- Division of Cancer Epidemiology and Genetics, National Cancer Institute, NIH, DHHS, Bethesda, MD, United States
Introduction: The Chernobyl accident resulted in a considerable release of radioactivity to the atmosphere, particularly of Iodine-131 (131I), with the greatest contamination occurring in Belarus, Ukraine, and western part of Russia.
Material and Methods: Increase in thyroid cancer and other thyroid diseases incidence in population exposed to Chernobyl fallout in these counties was the major health effect of the accident. Therefore, a lot of attention was paid to the thyroid doses, mainly, the 131I intake during two months after the accident. This paper reviews thyroid doses, both the individual for the subjects of radiation epidemiological studies and population-average doses. Exposure to 131I intake and other exposure pathways to population of affected regions and the Chernobyl cleanup workers (liquidators) are considered.
Results: Individual thyroid doses due to 131I intake varied up to 42 Gy and depended on the age of the person, the region where a person was exposed, and their cow’s milk consumption habits. Population-average thyroid doses among children of youngest age reached up to 0.75 Gy in the most contaminated area, the Gomel Oblast, in Belarus. Intake of 131I was the main pathway of exposure to the thyroid gland; its mean contribution to the thyroid dose in affected regions was more than 90%. The mean thyroid dose from inhalation of 131I for early Chernobyl cleanup workers was estimated to be 0.18 Gy. Individual thyroid doses due to different exposure pathways varied among 1,137 cleanup workers included in the epidemiological studies up to 9 Gy. Uncertainties associated with dose estimates, in terms of mean geometric standard deviation of individual stochastic doses, varied in range from 1.6 for doses based on individual-radiation measurements to 2.6 for “modelled” doses.
Conclusion: The 131I was the most radiologically important radionuclide that resulted in radiation exposure to the thyroid gland and cause an increase in the of rate of thyroid cancer and other thyroid diseases in population exposed after the Chernobyl accident.
Introduction
The Chernobyl accident that occurred on 26 April 1986 led to widescale radioactive contamination of territories in Belarus, Ukraine and the western part of Russia. The accident resulted in the release from the damaged reactor of a large amount of radionuclides into the atmosphere, including the radiologically significant short-lived Iodine-131 (131I), Tellurium-132 (132Te), Iodine-133 (133I), and long-lived Caesium-134 and Caesium-137 (134Cs and 137Cs) (1). Two main groups of people were exposed to radioactive fallout: (1) representatives of the population in the contaminated territories in Belarus, Ukraine, and Russia; and (2) cleanup workers (emergency and recovery workers or liquidators) who were the first responders or participated in cleanup activities at the site of the Chernobyl nuclear power plant (NPP) and in the 30-km zone around the NPP. Young children were among the population groups most affected by accident as they consumed cow’s milk contaminated with 131I.
The increase of thyroid cancer among persons who were exposed to Chernobyl fallout during childhood and adolescence were reported a few years after the accident, first in Belarus (2) and in Ukraine (3), and later in Russia (4). A number of radiation epidemiology studies have demonstrated an increased risk of thyroid cancer and other thyroid diseases associated with exposure of the thyroid gland to 131I (5–12). Results from these and other studies conducted in the affected population (13–15) suggested that an increase in the incidence of thyroid cancer in individuals exposed in childhood and adolescence was the main health effect of the Chernobyl accident. The excess odds ratios (EOR) of radiation-related thyroid cancer derived in these cohort and case-control studies were similar within the range of uncertainties and varied from 1.36 Gy-1 (95% confidence interval (CI): 0.39–4.15) (10) to 8.4 Gy-1 (95% CI: 4.1–17.3) (14). An increased risk of thyroid cancer, not statistically significant, (EOR/Gy = 3.91, 95% CI: –1.49, 65.7) has been reported in individuals exposed in utero (16, 17). Studies among Chernobyl cleanup workers, who were exposed as adults, also reported an increased risk of thyroid cancer after exposure to external irradiation and internal irradiation from 131I intake (18–20).
Since the main health effect of the Chernobyl accident is an increase in the incidence of thyroid cancer and other thyroid diseases, a lot of efforts have been devoted to the assessment of radiation thyroid doses. The main purpose of this paper is to summarize the methods and results of reconstruction of radiation doses to the thyroid of the population exposed to the Chernobyl accident.
Thyroid Doses to The Members of The General Public
In the aftermath of the Chernobyl accident, the radiation absorbed dose to the thyroid gland for the members of the general public resulted mainly from intake of 131I. In brief, the radionuclides, which were released into atmosphere during the accident, deposited on the ground surface, and contaminated the pasture grass and leafy vegetables covering the ground. The grazing cows ate contaminated grass and some fraction of the radioactivity was transferred to their milk. The consumption of fresh cow’s milk contaminated with 131I was the main pathway of thyroid exposure while the 131I intake with leafy vegetables and inhaled contaminated air playing a minor role. The radiation dose due to 131I intake is the highest for the thyroid gland as iodine accumulates in this organ. Thyroid doses in children are higher than that of adults is because of the smaller size of the thyroid gland in children. Since the half-life of 131I is 8.02 days, radiation exposure to the thyroid gland occurred during the first two months after the accident when the activity of 131I in the environment became negligible.
In addition, there were other contributors to the thyroid exposure, which were typically rather small for most individuals, but relatively important for those with no or little milk consumption: (1) internal irradiation due to intake of short-lived radioiodine and radiotellurium isotopes (132I, 133I, 135I, 131mTe, and 132Te); (2) external irradiation from gamma-emitted radionuclides deposited on the ground; and (3) internal irradiation resulting from intake of long-lived 134Cs and 137Cs.
There are two types of doses to the members of general public: (1) an individual dose for a specified person that takes into account (i) information on individual whereabouts and consumption history collected, typically, by means of personal interview and (ii) individual-based radiation measurements, if available; and (2) a population-average dose for an unspecified individual that is estimated using generic values of dosimetry models. Estimates of individual dose are required for radiation epidemiological studies while the population-average doses are used for the radiation protection of population by comparing of exposure levels in population groups of different ages living in different territories.
Radiation thyroid doses are also classified as “instrumental” doses, which are estimated using individual-based radiation measurements, and ‘modelled’ doses, which are estimated using dosimetry models.
Radiation and Thyroid Volume Measurements Available for Exposure Assessment
The following information was available to reconstruct radiation doses to the population in Belarus, Ukraine and Russia:
– About 400,000 measurements of 131I thyroidal activity that were derived from gamma-spectrometric and radiometric measurements (called “direct thyroid measurements”) made between 26 April and 30 June 1986 among the population resided in contaminated areas (21–23). These measurements were used to estimate the thyroid doses due to 131I intake for measured individuals.
– 137Cs ground deposition density measured in almost every contaminated settlement in Belarus, Ukraine and Russia (24).
– Ground deposition densities of 131I and other gamma-emitting radionuclides (95Zr, 95Nb, 103Ru, 106Ru, 134Cs, 141Ce, 144Ce), measured in some locations (25–28).
– Activity concentration of 131I and total beta-activity in cow’s milk (29–31).
– More than 600,000 measurements of radiocesium activity in individuals living in contaminated territories made using whole-body counter (32–35);
– Thyroid volume-values measured by the Sasakawa Memorial Health Foundation in Belarus, Ukraine, and Russia in the 1990s (36–38).
Thyroid Doses from Intake of 131I
Individual Thyroid Doses for the Subjects of Epidemiological Studies
The most reliable individual thyroid doses were estimated based on the results of measurements of 131I thyroidal activity carried out in the most contaminated oblasts (regions) in Belarus, Ukraine, and Russia (Figure 1). There are two cohort studies that used estimates of individual 131I thyroidal activity available for each cohort member and provided the uncertainties associated with estimates of thyroid doses due to 131I intake. These two thyroid screening cohorts consist of people who were 0–18 years old at the time of the accident (ATA), 11,732 individuals in the Belarusian-American (BelAm) cohort and 13,204 individuals in the Ukrainian-American (UkrAm) cohort (39). The BelAm cohort includes persons who resided ATA in Gomel and Mogilev Oblasts as well as in the city of Minsk; UkrAm cohort consists of residents of Chernihiv, Kyiv, and Zhytomyr Oblasts (see Figure 1).
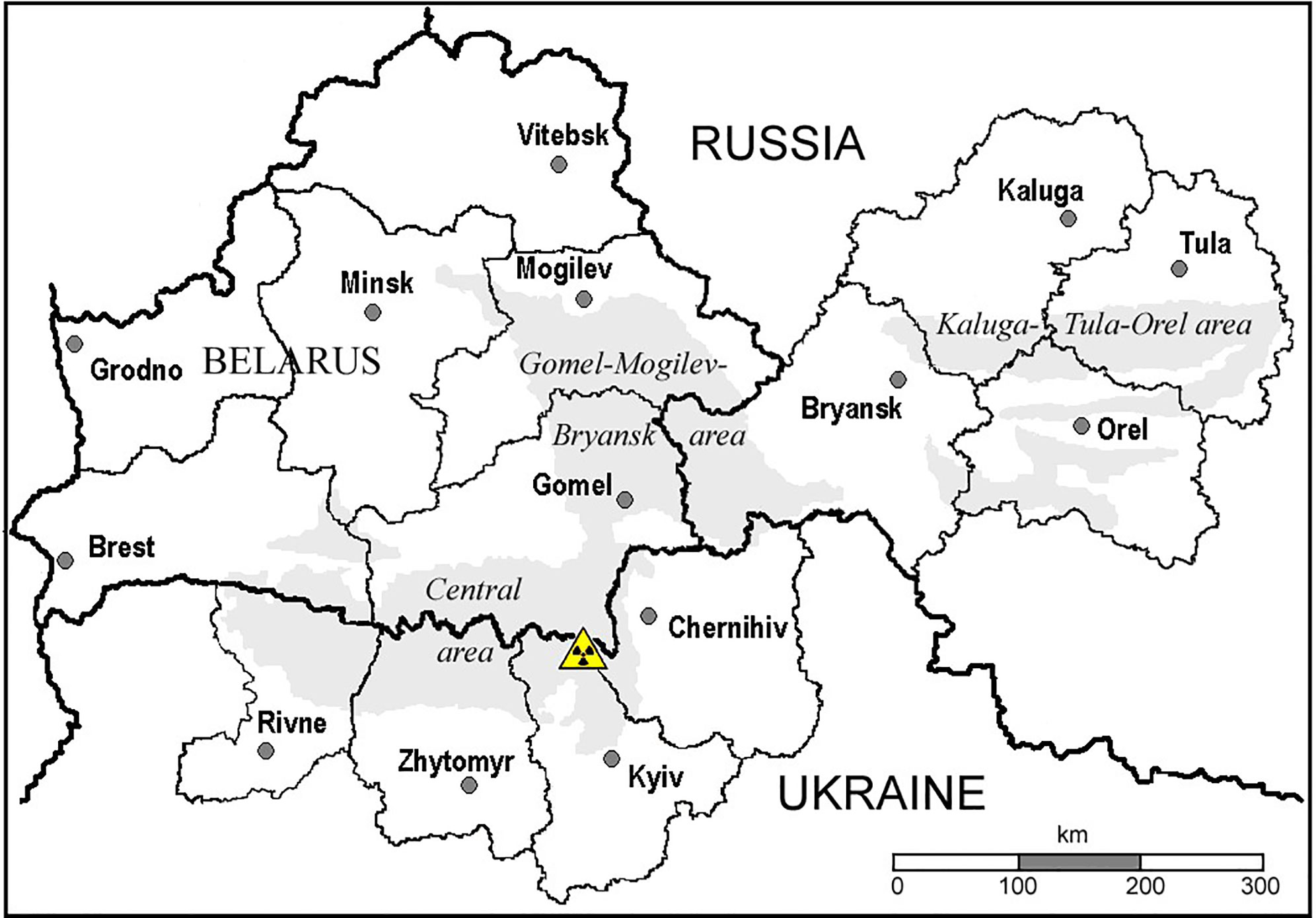
Figure 1 Areas in Belarus, Ukraine, and Russia. Territories with 137Cs ground deposition density greater than 37 kBq m-2 are shown in gray.
Information on residential history, consumption of cows’ milk, dairy products, and leafy vegetables as well as the administration of stable iodine necessary for the assessment of the individual thyroid doses was collected for each cohort member by means of personal interviews (40, 41). Another two screening thyroid cohorts consist of persons exposed in utero, 2,965 and 2,582 individuals in Belarus and Ukraine, respectively (16, 42). A fraction of the cohorts’ members, around 10% in Belarus and 28% in Ukraine, was subject to direct thyroid measurements (43, 44). Practically the same methodology was used to assess the individual thyroid doses in the BelAm and UkrAm cohorts as well as in the Belarusian and Ukrainian in utero cohorts.
In the BelAm and UkrAm studies, thyroid doses due to 131I intake were calculated in a stochastic mode using a Monte-Carlo simulation procedure that provides an estimate of the uncertainties (41, 45). In accordance with this procedure, 1,000 individual stochastic thyroid doses were calculated, considering the classification of errors as shared or unshared. The distribution of individual stochastic thyroid doses for cohort members was approximately lognormal; the geometric standard deviation (GSD) of this distribution characterized the uncertainty of dose estimates.
Table 1 shows the distribution of individual thyroid doses in these four cohorts. More than 2/3 of the subjects of the BelAm and UkrAm cohorts received thyroid doses less than 0.5 Gray (Gy) and of the Belarusian and Ukrainian in utero cohorts received thyroid doses less than 0.05 Gy. Individual thyroid doses varied widely from 0 to 39, 42, 15, and 3.2 Gy in the BelAm, UkrAm, Belarusian and Ukrainian in utero cohorts, respectively.
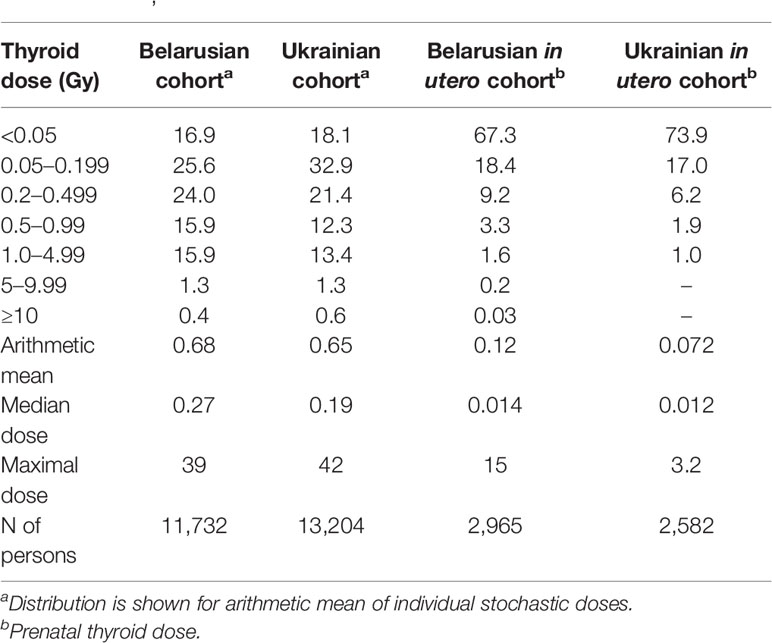
Table 1 Distribution (%) of thyroid doses from intake of 131I and mean, median, and maximal dose among subjects of the screening cohorts in Belarus and Ukraine (41, 43–45).
The individual stochastic doses were characterized by the GSDs from 1.3 to 5.1 with (an arithmetic mean (AM) of 1.8, a geometric mean (GM) of 1.7) for the BelAm cohort, and from 1.3 to 10.6 (AM of 1.6, GM of 1.5) for the UkrAm cohort. The uncertainties in thyroid doses were mainly defined by sources of unshared (classical) errors: the derivation of 131I activity in the cohort member’s thyroid from direct thyroid measurements and the values of thyroid mass (41, 45, 46).
If the result of measurement of 131I thyroidal activity was not available for the individual, thyroid doses were estimated using two types of models:
– Purely empirical models that were based on the correlation between environmental contamination (deposition density of 131I or 137Cs, 131I activity concentration in cow’s milk) and thyroid doses derived from direct thyroid measurements done among individuals of different ages (21, 22, 29, 47–49); and
– An environmental transfer model that considers a process of 131I activity transfer to the human thyroid with contaminated milk and/or leafy vegetables for ingestion or with contaminated ground-level air for inhalation (50, 51).
To estimate the individual modelled thyroid doses, a personal interview was conducted to collect information on the whereabouts and consumption history for a given person. Individual thyroid doses estimated using the models, called “modelled” doses, were associated with uncertainties arose mainly from the estimates of 131I ground deposition density in the place of residence, the transfer of 131I to cow’s milk, and estimates of the thyroid mass (46, 47, 52).
Table 2 presents the thyroid doses due to 131I by age and by country of residence among the subjects of the case-control study of thyroid cancer in Belarus and Russia (Figure 1), which was coordinated by the International Agency for Research on Cancer (IARC). Data from the table show that the thyroid dose decreased with increasing age. The thyroid dose in Russia was estimated to be more than five times lower than that in Belarus, 0.10 vs. 0.54 Gy for mean. The individual stochastic doses were characterized by the GSDs from 1.59 to 3.61 (AM of 1.94, GM of 1.89). The uncertainties in thyroid doses were defined by the shared (Berkson) errors in parameters of the model and by unshared (classical) errors related to the estimates of thyroid-mass values (52, 53).
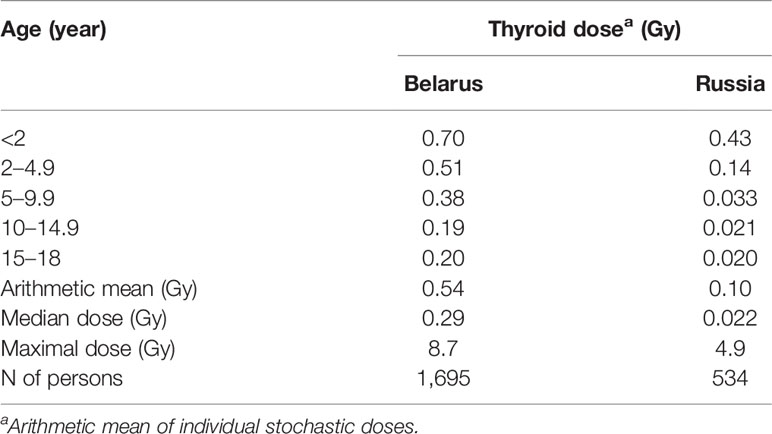
Table 2 Thyroid doses due to 131I intake at different ages and by country of residence among subjects of the case-control study of thyroid cancer in Belarus and Russia (53).
Population Average Dose Estimates
Thyroid doses from intake of 131I for population groups were estimated using a combination of the methods indicated above. The following groups of population were considered (54, 55): evacuees from the 30-km zone around Chernobyl NPP and residents of the contaminated areas in the most affected countries, Belarus, Ukraine and Russia.
Evacuees. More than 100,000 persons were evacuated in the weeks after the accident from the most contaminated 30-km zone around the Chernobyl NPP in Ukraine and Belarus. The thyroid doses varied with place of residence, date of evacuation, and the age of the evacuees. Evacuees from Belarusian villages received the highest doses, the average thyroid dose was estimated to be 0.68 Gy for adults and 3.1 Gy for young children (0–7 years old) vs. 0.28 Gy and 1.2, respectively, for evacuees from Ukrainian villages (54). The thyroid doses for the residents evacuated from the town of Pripyat were 0.28 Gy for adults and 0.99 Gy for young children received mainly due to the 131I intake with cow’s milk during their stay in the villages where they were evacuated (56). The population-weighted average thyroid dose for the entire evacuated population was 0.47 Gy. It should be noted that dose estimates were based on direct thyroid measurements carried out among evacuated persons.
Residents of the Contaminated Areas. Table 3 shows estimates of thyroid doses due to 131I for the populations of Belarus, Ukraine, and Russia (1, 57). Population-average thyroid doses depended on the varied from region to region dates of fallout and 131I ground deposition densities, and on the dates when pasture grazing season started. The latter is vital, since cow’s milk was the main source of 131I intake to the exposed population. The highest Oblast-average thyroid dose due to 131I intake among the three countries was realized in the most contaminated Gomel Oblast in Belarus, it varied from 0.15 Gy for adults to 0.75 Gy for young children. The highest dose in Ukraine was found in Zhytomyr Oblast, 0.06 Gy for adults and 0.23 Gy for children 0–7 years old; and in Russia in Bryansk Oblast, 0.026 Gy for adults and 0.16 Gy for children 0–7 years old.
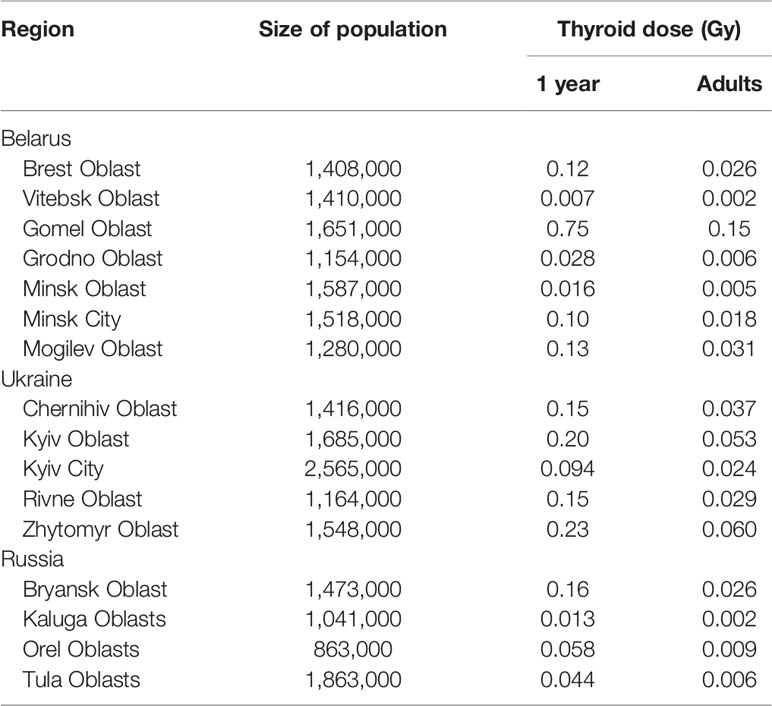
Table 3 Estimates of thyroid doses due to 131I intake for the populations of Belarus, Ukraine, and Russia (1, 57).
Thyroid Doses from Pathways Other than Intake of 131I
Thyroid doses for most individuals were mainly due to 131I intake. However, there were other exposure pathways with typically small contribution to the thyroid dose: (1) intake of short-lived radioiodine and radiotellurium isotopes (132I, 133I, 135I, 131mTe, and 132Te); (2) external irradiation from gamma-emitting radionuclides deposited on the ground (mainly 140Ba+140La, 95Zr+90Nb, and 132Te+132I shortly after the accident and 134Cs and 137Cs in the long term); and (3) intake of long-lived 134Cs and 137Cs. The methods used to estimate thyroid doses from these pathways are described elsewhere (28, 33, 34, 47, 58, 59).
Table 4 shows the contribution of minor exposure pathways to the individual thyroid doses reconstructed for the subjects of epidemiological studies. The contribution varied from 5 to 8% for subjects of the studies conducted in Belarus and was about 10% for subjects in Russia.
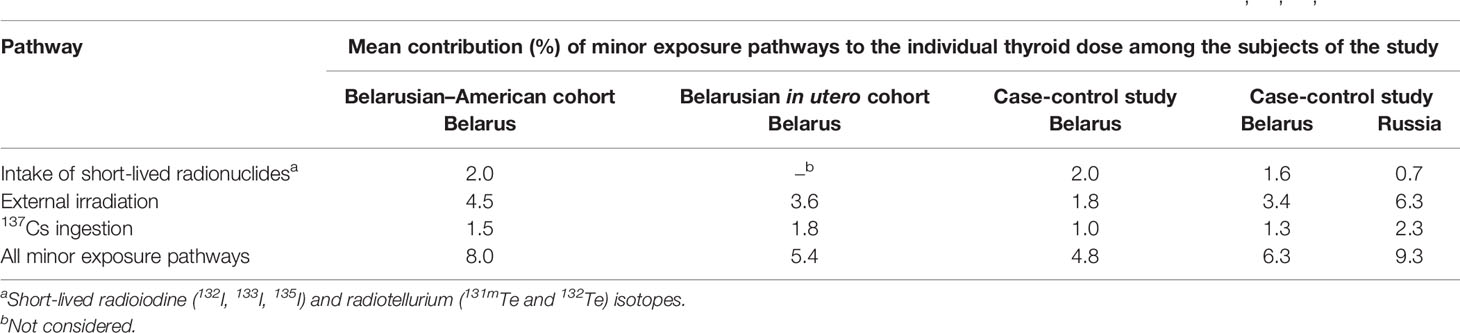
Table 4 Contribution of minor exposure pathways to the individual thyroid dose reconstructed for the subjects of epidemiological studies (34, 40, 43, 60).
However, the contribution of minor pathways may be substantial for some individuals. For evacuees from Pripyat-town, which is located near the Chernobyl NPP, the inhalation intake of short-lived radioiodine and radiotellurium isotopes (132I, 133I, and 132Te) contributed about 30% to the total thyroid dose due to inhalation (61). For the IARC coordinated case-control study of thyroid cancer in Belarus and Russia, it was estimated that for 19 out of 1,615 (1.3% of the total) study subjects, the contribution of external irradiation and ingestion of radiocesium isotopes to the thyroid dose was higher than 50% (60). These individuals were relocated from contaminated residents shortly after the accident or did not consume locally produced foodstuffs and, therefore, were exposed to relatively small doses from 131I intake in April–June 1986 but received high doses from long-lived sources of exposure in subsequent years.
Thyroid Doses to The Chernobyl Cleanup Workers
Following the Chernobyl accident, more than 500,000 cleanup workers participated from 26 April 1986 to 31 December 1990 in cleanup activities on the reactor site and in the restricted 30-km zone around the Chernobyl NPP (1). Cleanup workers consisted of different occupational groups, including Chernobyl NPP personnel, nuclear workers, military, construction workers, and support staff, who performed work on decontamination and maintenance at the Chernobyl site, construction and safeguard at various locations (62, 63).
Basically, the cleanup workers received doses due to external irradiation (64–66). However, during the 10-day period of atmospheric releases of radioactivity from destroyed Unit 4, the cleanup workers also were exposed to internal irradiation due to inhalation of 131I and short-lived 132I, 133I, 135I, 131mTe, and 132Te. In addition, cleanup workers, who resided in contaminated areas in Belarus, Ukraine and Russia received thyroid doses due to 131I intake with cow’s milk, dairy products and leafy vegetables produced at their places of residence.
Cleanup Workers With Direct Thyroid Measurements
A group of 594 Chernobyl cleanup workers was measured for 131I thyroidal activity between 30 April and 5 May 1986 (67). At the time of the direct thyroid measurement, each person provided information on his or her cleanup activities since 26 April 1986 as well as on stable iodine administration. As measured individuals were the operation personnel of the Chernobyl NPP, only inhalation intake of 131I with contaminated air was considered in calculation of the thyroid doses.
Table 5 shows the distribution of thyroid doses for cleanup workers with direct thyroid measurements. The average thyroid dose among the cleanup workers was 0.18 Gy and the median was 0.11 Gy. About 73% of the cleanup workers received thyroid doses between 0.05 and 0.50 Gy. The highest individual thyroid dose due to 131I intake estimated among Chernobyl cleanup workers based on direct thyroid measurement was 4.5 Gy.
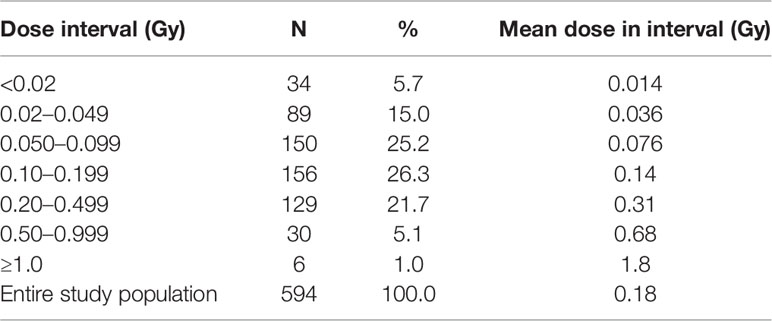
Table 5 Distribution of the thyroid doses from inhalation of 131I for the 594 Chernobyl cleanup workers with direct thyroid measurements (67).
Thyroid Doses for the Subjects of Epidemiological Studies
Two case-control thyroid-cancer studies were conducted among Chernobyl cleanup workers: (1) a study nested within cohorts of Belarusian, Russian, and Baltic (Latvia, Lithuania and Estonia) cleanup workers that was coordinated by the IARC and included 530 subjects (19); and (2) a collaborative study of the National Research Center for Radiation Medicine (Kyiv, Ukraine) and the U.S. National Cancer Institute that was nested in a cohort of Ukrainian cleanup workers and included 607 subjects (68). Individual thyroid doses were estimated for the study subjects due to (a) external irradiation during a cleanup mission using the RADRUE method (65), (b) internal irradiation during a cleanup mission due to inhalation of (b) 131I and (c) short-lived radioiodine and radiotellurium isotopes as well as from (d) intake of 131I during residence (69). A dosimetry questionnaire was administrated to each cleanup worker or his proxy to collect detailed information about (a) the cleanup worker’s routes to and from his or her work place(s) at the Chernobyl site and in the 30-km zone, (b) the details about the cleanup activities, (c) residential history between 26 April and 30 June 1986, and (d) consumption of locally produced foodstuffs (only for Ukrainian cleanup workers). To estimate the uncertainties in doses, individual stochastic doses for each considered exposure pathway were calculated using Monte Carlo simulations.
Table 6 provides the thyroid doses from different exposure pathways reconstructed for the subjects of epidemiological studies among the Chernobyl cleanup workers. The mean thyroid dose from all exposure pathways was 0.19, 0.10, 0.058, and 0.20 Gy for cleanup workers from Belarus, Russia, Baltic States and Ukraine, respectively. The thyroid dose was formed mainly due to external irradiation, except for Belarusian cleanup workers who were, mainly, residents of the 30-km zone and their dose was defined by 131I intake during cleanup mission and residence. The thyroid doses during residence were not calculated for Russian and Baltic States cleanup workers as 131I deposition densities in their locations of residence were negligible compared to those of Belarusian and Ukrainian cleanup workers.
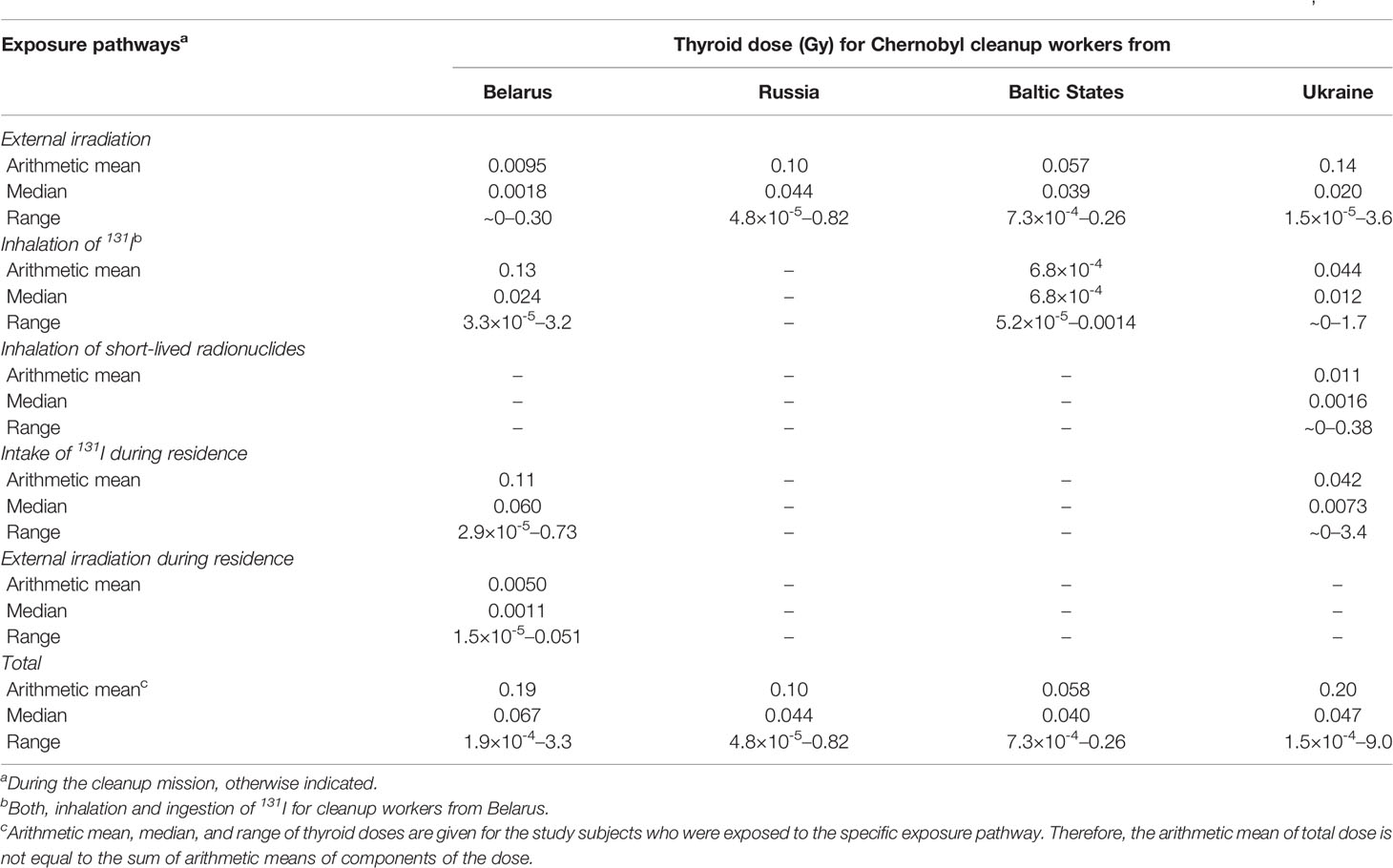
Table 6 Thyroid doses from different exposure pathways reconstructed for subjects of case-control studies of thyroid cancer among Chernobyl cleanup workers (19, 69).
For the IARC-coordinated study, the GSDs of the individual stochastic doses due to external irradiation varied from 1.1 to 5.8 with a mean of 1.9, while for the thyroid doses due to 131I intake during residence, the GSDs varied from 1.9 to 2.5 with a mean of 2.2 (70). The uncertainties of the individual stochastic doses in the Ukrainian-American study were characterized by a mean GSD of 2.0, 1.8, 2.0 and 2.6 for external irradiation, inhalation of 131I, inhalation of short-lived radionuclides, and exposure to 131I intake during residence, respectively (69).
Conclusions
This paper considers the radiation exposure to the thyroid after the Chernobyl accident. The most important radiological consequence of the accident was exposure to 131I, which led to an increase in the rate of thyroid cancer and other thyroid diseases in the exposed population. The thyroid doses were mainly defined by the consumption of cow’s milk contaminated with 131I. Individual thyroid doses due to 131I intake varied up to 42 Gy and depended on the age of person, the region where people were exposed, and their cow’s milk consumption habits. In addition to exposure due to 131I, the intake of short-lived radioiodine and radiotellurium isotopes, external irradiation, and the intake of long-lived 134Cs and 137Cs contributed to the thyroid dose of the members of public, typically, not more than 10%.
The mean thyroid dose due to 131I inhalation in a group of 594 early Chernobyl cleanup workers was 0.18 Gy. Individual thyroid doses were also reconstructed for 1,137 cleanup workers included in two case-control studies of thyroid cancer. Their thyroid dose from different exposure pathways, i.e., external irradiation, inhalation of 131I and short-lived radionuclides during the cleanup mission, and 131I intake during residence, varied within a wide range from essential zero to 9 Gy.
Author Contributions
The author confirms being the sole contributor of this work and has approved it for publication.
Funding
This work was funded by the Intramural Research Program of the National Cancer Institute (USA), Division of Cancer Epidemiology and Genetics.
Conflict of Interest
The author declares that the research was conducted in the absence of any commercial or financial relationships that could be construed as a potential conflict of interest.
Acknowledgments
The author gratefully acknowledges the contribution of the late Drs. Yuri Gavrilin, Valeri Khrouch, Ilya Likhtarov, and Nickolas Luckyanov to the design, development, and implementation of the dosimetry for the studies considered in the paper. The author also gratefully acknowledges the contribution of Lynn Anspaugh, Elena Bakhanova, André Bouville, Mikhail Balonov, Vadim Chumak, Mykola Chepurny, Ivan Golovanov, Victor Kryuchkov, Lionella Kovgan, Semion Kutsen, Sergii Masiuk, Victor Minenko, Sergey Shinkarev, Valeri Stepanenko, Paul Voillequé, Irina Zvonova, and many others to the dosimetry methods applied in the reviewed studies.
References
1. United Nations Scientific Committee on the Effects of Atomic Radiation (UNSCEAR). Sources and Effects of Ionizing Radiation, UNSCEAR 2008 Report. Annex D: Health effects due to radiation from the Chernobyl accident. Sales No. E.11.IX.3. New York: United Nations (2011).
2. Kazakov VS, Demidchik EP, Astakhova LN. Thyroid cancer after Chernobyl. Nature (1992) 359:21. doi: 10.1038/359021a0
3. Prisyazhiuk A, Pjatak OA, Buzanov VA, Reeves GK, Beral V. Cancer in the Ukraine, post Chernobyl. Lancet (1991) 338:1334–5. doi: 10.1016/0140-6736(91)92632-c
4. Ivanov VK, Tsyb AF, Matveenko YG, Parshkov YM, Maksyutov MA, Gorskiy AI, et al. Radiation epidemiology of cancer- and non-cancer thyroid diseases in Russia after the ChNPP accident: Prognostication and risk estimation. Radiat Risk (1995) 1:3–29.
5. Brenner AV, Tronko MD, Hatch M, Bogdanova TI, Oliynik VA, Lubin JH, et al. I-131 dose response for incident thyroid cancers in Ukraine related to the Chornobyl accident. Environ Health Perspect (2011) 119:933–9. doi: 10.1289/ehp.1002674
6. Cahoon EK, Nadirov EA, Polanskaya ON, Yauseyenka VV, Velalkin IV, Minenko VF, et al. Risk of prevalent thyroid nodules in residents of Belarus exposed to Chernobyl fallout as children and adolescents. J Clin Endocrinol Metab (2017) 102:2207–21. doi: 10.1210/jc.2016-3842
7. Ostroumova E, Brenner A, Oliynyk V, McConnell R, Robbins J, Terekhova G, et al. Subclinical hypothyroidism after radioiodine exposure: Ukrainian-American cohort study of thyroid cancer and other thyroid diseases after the Chornobyl accident (1998-2000). Environ Health Perspect (2009) 117:745–50. doi: 10.1289/ehp.0800184
8. Ostroumova E, Rozhko A, Hatch M, Furukawa K, Polyanskaya O, McConnell RJ, et al. Measures of thyroid function among Belarusian children and adolescents exposed to Iodine-131 from the accident at the Chernobyl nuclear plant. Environ Health Perspect (2013) 121:865–71. doi: 10.1289/ehp.1205783
9. Tronko MD, Howe GR, Bogdanova TI, Bouville AC, Epstein OV, Brill AB, et al. A cohort study of thyroid cancer and other thyroid diseases after the Chornobyl accident: thyroid cancer in Ukraine detected during first screening. J Natl Cancer Inst (2006) 98:896–903. doi: 10.1093/jnci/djj244
10. Tronko M, Brenner A, Bogdanova T, Shpak V, Hatch M, Oliynyk V, et al. Thyroid neoplasia risk is increased nearly 30 years after the Chernobyl accident. Int J Cancer (2017) 141:1585–8. doi: 10.1002/ijc.30857
11. Zablotska LB, Ron E, Rozhko AV, Hatch M, Polyanskaya ON, Brenner AV, et al. Thyroid cancer risk in Belarus among children and adolescents exposed to radioiodine after the Chornobyl Accident. Br J Cancer (2011) 104:181–7. doi: 10.1038/sj.bjc.6605967
12. Zablotska LB, Nadyrov EA, Polyanskaya ON, McConnell RJ, O’Kane P, Lubin J, et al. Risk of thyroid follicular adenoma among children and adolescents in Belarus exposed to Iodine-131 after the Chornobyl accident. Am J Epidemiol (2015) 182:781–90. doi: 10.1093/aje/kwv127
13. Astakhova LN, Anspaugh LR, Beebe GW, Bouville A, Drozdovitch VV, Garber V, et al. Chernobyl-related thyroid cancer in children of Belarus: a case-control study. Radiat Res (1998) 150:349–56. doi: 10.2307/3579983
14. Cardis E, Kesminiene A, Ivanov V, Malakhova I, Shibata Y, Khrouch V, et al. Risk of thyroid cancer after exposure to 131I in childhood. J Natl Cancer Inst (2005) 97:724–32. doi: 10.1093/jnci/dji129
15. Davis S, Stepanenko V, Rivkind N, Kopecky KJ, Voillequé P, Shakhtarin V, et al. Risk of thyroid cancer in the Bryansk Oblast of the Russian Federation after the Chernobyl power station accident. Radiat Res (2004) 162:241–8. doi: 10.1667/rr3233
16. Hatch M, Brenner A, Bogdanova T, Derevyanko A, Kuptsova N, Likhtarev I, et al. A screening study of thyroid cancer and other thyroid diseases among individuals exposed in utero to Iodine-131 from Chornobyl fallout. J Clin Endocrinol Metab (2009) 94:899–906. doi: 10.1210/jc.2008-2049
17. Hatch M, Brenner AV, Cahoon EK, Drozdovitch V, Little MP, Bogdanova T, et al. Thyroid cancer and benign nodules after exposure in utero to fallout from Chernobyl benign thyroid nodules. J Clin Endocrinol Metab (2019) 104:41–8. doi: 10.1210/jc.2018-00847
18. Ivanov VK, Tsyb AF, Gorsky AI, Maksyutov MA, Rastopchin EM, Konogorov AP, et al. Thyroid cancer among “liquidators” of the Chernobyl accident. Br J Radiol (1997) 70:937–41. doi: 10.1259/bjr.70.837.9486071
19. Kesminiene A, Evrard AS, Ivanov VK, Malakhova IV, Kurtinaitis J, Stengrevics A, et al. Risk of thyroid cancer among Chernobyl liquidators. Radiat Res (2012) 178:425–36. doi: 10.1667/RR2975.1
20. Ostroumova E, Gudzenko N, Brenner A, Gorokh Y, Hatch M, Prysyazhnyuk A, et al. Thyroid cancer incidence in Chornobyl liquidators in Ukraine: SIR analysis, 1986-2010. Eur J Epidemiol (2014) 29:337–42. doi: 10.1007/s10654-014-9896-1
21. Gavrilin YI, Khrouch VT, Shinkarev SM, Krysenko NA, Skryabin AM, Bouville A, et al. Chernobyl accident: Reconstruction of thyroid dose for inhabitants of the Republic of Belarus. Health Phys (1999) 76:105–19. doi: 10.1097/00004032-199902000-00002
22. Likhtarov I, Kovgan L, Vavilov S, Chepurny M, Bouville A, Luckyanov N, et al. Post-Chornobyl thyroid cancers in Ukraine. Report 1. Estimation of thyroid doses. Radiat Res (2005) 163:125–36. doi: 10.1667/rr3291
23. Bratilova AA, Zvonova IA, Balonov MI, Shishkanov NG, Trushin VI, Hoshi M. 131I content in the human thyroid estimated from direct measurements of the inhabitants of Russian areas contaminated due to the Chernobyl accident. Radiat Prot Dosimetry (2003) 105:623–6. doi: 10.1093/oxfordjournals.rpd.a006315
24. European Commission. Atlas on 137Cs deposition on Europe after the Chernobyl accident. Brussels, Luxembourg: European Commission (1998).
25. Drozdovitch V, Zhukova O, Germenchuk M, Khrutchinsky A, Kukhta T, Luckyanov N, et al. Database of meteorological and radiation measurements made in Belarus during the first three months following the Chernobyl accident. J Environ Radioact (2013) 116:84–92. doi: 10.1016/j.jenvrad.2012.09.010
26. Khrushchinskii AA, Kuten SA, Minenko VF, Zhukova OM, Podgaiskaya AA, Germenchuk MG, et al. Radionuclide ratios in precipitation on the territory of Belarus after the Chernobyl accident: Calculation from gamma-spectrometric measurements on soil in May–July 1986. At Energy (2014) 117:143–8. doi: 10.1007/s10512-014-9902-4
27. Mück K, Pröhl G, Likhtarev I, Kovgan L, Meckbach R, Golikov V. A consistent radionuclide vector after the Chernobyl accident. Health Phys (2002) 82:141–56. doi: 10.1097/00004032-200202000-00002
28. Pitkevich VA, Guba VV, Ivanov VK, Chekin SY, Tsyb AF, Vakulovsky SM, et al. Reconstruction of the composition of the Chernobyl radionuclide fallout and external radiation absorbed doses to the population in areas of Russia. Radiat Prot Dosimetry (1996) 64:69–92. doi: 10.1093/oxfordjournals.rpd.a031568
29. Drozdovitch V, Germenchuk M, Bouville A. Using total beta-activity measurements in milk to derive thyroid doses from Chernobyl fallout. Radiat Prot Dosimetry (2006) 118:402–11. doi: 10.1093/rpd/nci360
30. Minenko V, Viarenich K, Zhukova O, Kukhta T, Podgaiskaya M, Khrutchinsky A, et al. Activity concentrations of 131I and other radionuclides in cow’s milk in Belarus during the first month following the Chernobyl accident. J Environ Radioact (2020) 220-221:106264. doi: 10.1016/j.jenvrad.2020.106264
31. Zvonova IA, Bratilova AA, Balonov MI, Shutov VN, Kotik DS, Shaposhnikova EN, et al. 131I concentration in milk in Russian areas after the Chernobyl accident. In: Full papers of IRPA-11 International Congress. Madrid, Spain, 23-28 May 2004, Madrid: IRPA (2004) ISBN: 84-87078-05-2.
32. Likhtarev IA, Kovgan LN, Vavilov ES, Gluvchinsky RR, Perevoznikov ON, Litvinets LN, et al. Internal exposure from ingestion of foods contaminated by 137Cs after the Chernobyl accident. Report I. General model: Ingestion doses and countermeasure effectiveness for the adults of Rovno oblast of Ukraine. Health Phys (1996) 70:297–317. doi: 10.1097/00004032-199603000-00001
33. Likhtarev IA, Kovgan LN, Vavilov ES, Perevoznikov ON, Litvinets LN, Anspaugh LR, et al. Internal exposure from ingestion of foods contaminated by 137Cs after the Chernobyl accident. Report II. Ingestion doses of the rural population of Ukraine up to 12 y after the accident (1986-1997). Health Phys (2000) 79:341–57. doi: 10.1097/00004032-200010000-00002
34. Minenko VF, Ulanovsky A, Drozdovitch V, Shemiakina E, Gavrilin Y, Khrouch V, et al. Individual thyroid dose estimates for a case-control study of Chernobyl-related thyroid cancer among children of Belarus. Part II. Contributions from long-lived radionuclides and external radiation. Health Phys (2006) 90:312–27. doi: 10.1097/01.HP.0000183761.30158.c1
35. Sekitani Y, Hayashida N, Karevskaya IV, Vasilitsova OA, Kozlovsky A, Omiya M, et al. Evaluation of 137Cs body burden in inhabitants of Bryansk Oblast, Russian Federation, where a high incidence of thyroid cancer was observed after the accident at the Chernobyl nuclear power plant. Radiat Prot Dosimetry (2010) 141:36–42. doi: 10.1093/rpd/ncq137
36. Ashizawa K, Shibata Y, Yamashita S, Namba H, Hoshi M, Yokoyama N, et al. Prevalence of goiter and urinary iodine excretion levels in children around Chernobyl. J Clin Endocrinol Metab (1997) 82:3430–3. doi: 10.1210/jcem.82.10.4285
37. Likhtarov I, Kovgan L, Masiuk S, Chepurny M, Ivanova O, Gerasymenko V, et al. Estimating thyroid masses for children, infants, and fetuses in Ukraine exposed to (131)I from the Chernobyl accident. Health Phys (2013) 104:78–86. doi: 10.1097/HP.0b013e31826e188e
38. Skryabin AM, Drozdovitch V, Belsky Y, Leshcheva SV, Mirkhaidarov AK, Voillequé P, et al. Thyroid mass in children and adolescents living in the most exposed areas to Chernobyl fallout in Belarus. Radiat Prot Dosimetry (2010) 142:292–9. doi: 10.1093/rpd/ncq209
39. Stezhko VA, Buglova EE, Danilova LI, Drozd VM, Krysenko NA, Lesnikova NR, et al. A cohort study of thyroid cancer and other thyroid diseases following the Chornobyl accident: objectives, design, and methods. Radiat Res (2004) 161:481–92. doi: 10.1667/3148
40. Drozdovitch V, Minenko V, Khrouch V, Leshcheva S, Gavrilin Y, Khrutchinsky A, et al. Thyroid dose estimates for a cohort of Belarusian children exposed to radiation from the Chernobyl accident. Radiat Res (2013) 179:597–609. doi: 10.1667/RR3153.1
41. Likhtarov I, Kovgan L, Masiuk S, Talerko M, Chepurny M, Ivanova O, et al. Thyroid cancer study among Ukrainian children exposed to radiation after the Chornobyl accident: improved estimates of the thyroid doses to the cohort members. Health Phys (2014) 106:370–96. doi: 10.1097/HP.0b013e31829f3096
42. Yauseyenka V, Drozdovitch V, Ostroumova E, Polyanskaya O, Minenko V, Brenner A, et al. Belarusian in utero cohort: new opportunity to evaluate health effects of prenatal and early-life exposure to ionizing radiation. J Radiol Prot (2020) 40:280–95. doi: 10.1088/1361-6498/ab5c08
43. Drozdovitch V, Minenko V, Kukhta T, Trofimik S, Grakovitch R, Hatch M, et al. Thyroid dose estimates for a cohort of Belarusian persons exposed in utero and during early life to Chernobyl fallout. Health Phys (2020) 118:170–84. doi: 10.1097/HP.0000000000001135
44. Likhtarov I, Kovgan L, Chepurny M, Ivanova O, Boyko Z, Ratia G, et al. Estimation of the thyroid doses for Ukrainian children exposed in utero after the Chornobyl accident. Health Phys (2011) 100:583–93. doi: 10.1097/HP.0b013e3181ff391a
45. Drozdovitch V, Minenko V, Golovanov I, Khrutchinsky A, Kukhta T, Kutsen S, et al. Thyroid dose estimates for a cohort of Belarusian children exposed to 131I from the Chernobyl accident: Assessment of uncertainties. Radiat Res (2015) 184:203–18. doi: 10.1667/rr13791.1
46. Likhtarev I, Minenko V, Khrouch V, Bouville A. Uncertainties in thyroid dose reconstruction after Chernobyl. Radiat Prot Dosimetry (2003) 105:601–8. doi: 10.1093/oxfordjournals.rpd.a006310
47. Gavrilin Y, Khrouch V, Shinkarev S, Drozdovitch V, Minenko V, Shemiakina E, et al. Individual thyroid dose estimation for a case-control study of Chernobyl-related thyroid cancer among children of Belarus. Part 1: 131I, short-lived radioiodines (132I, 133I, 135I), and short-lived radiotelluriums (131mTe and 132Te). Health Phys (2004) 86:565–85. doi: 10.1097/00004032-200406000-00002
48. Stepanenko VF, Voilleque PG, Gavrilin Y, Khrouch VT, Shinkarev SM, Orlov MY, et al. Estimating individual thyroid doses for a case-control study of childhood thyroid cancer in Bryansk Oblast, Russia. Radiat Prot Dosimetry (2004) 108:143–60. doi: 10.1093/rpd/nch017
49. Zvonova IA, Balonov MI, Bratilova AA. Thyroid dose reconstruction for the population of Russia after the Chernobyl accident. Radiat Prot Dosimetry (1998) 79:175–8. doi: 10.1093/oxfordjournals.rpd.a032386
50. Drozdovitch VV, Goulko GM, Minenko VF, Paretzke HG, Voigt G, Kenigsberg Y. Thyroid dose reconstruction for the population of Belarus after the Chernobyl accident. Radiat Environ Biophys (1997) 36:17–23. doi: 10.1007/s004110050050
51. Vlasov OK, Pitkevich VA. Agro-climate model for estimation of radionuclides transport on food chain and for formation of internal exposure to population. Radiat Risk (1999) 11:65–85.
52. Drozdovitch V, Maceika E, Khrouch V, Zvonova I, Vlasov O, Bouville A, et al. Uncertainties in individual doses in a case-control study of thyroid cancer after the Chernobyl accident. Radiat Prot Dosimetry (2007) 127:540–3. doi: 10.1093/rpd/ncm360
53. Drozdovitch V, Kesminiene A, Moissonnier M, Veyalkin I, Ostroumova E. Uncertainties in radiation doses for a case-control study of thyroid cancer among persons exposed in childhood to Iodine-131 from Chernobyl fallout. Health Phys (2020) 119:222–35. doi: 10.1097/HP.0000000000001206
54. Bouville A, Likhtarev I, Kovgan L, Minenko V, Shinkarev S, Drozdovitch V. Radiation dosimetry for highly contaminated Ukrainian, Belarusian and Russian populations, and for less contaminated populations in Europe. Health Phys (2007) 93:487–501. doi: 10.1097/01.HP.0000279019.23900.62
55. Cardis E, Howe G, Ron E, Bebeshko V, Bogdanova T, Bouville A, et al. Cancer consequences of the Chernobyl accident: 20 years after. J Radiol Prot (2006) 26:127–40. doi: 10.1088/0952-4746/26/2/001
56. Goulko GM, Chumak VV, Chepurny NI, Henrichs K, Jacob P, Kairo IA, et al. Estimation of 131I thyroid doses for the evacuees from Pripjat. Radiat Environ Biophys (1996) 35:81–7. doi: 10.1007/BF02434029
57. Drozdovitch V, Bouville A, Chobanova N, Filistovic V, Ilus T, Kovačić M, et al. Radiation exposure to the population of Europe following the Chernobyl accident. Radiat Prot Dosimetry (2007) 123:515–28. doi: 10.1093/rpd/ncl528
58. Golikov VY, Balonov MI, Jacob P. External exposure of the population living in areas of Russia contaminated due to the Chernobyl accident. Radiat Environ Biophys (2002) 41:185–93. doi: 10.1007/s00411-002-0167-2
59. Likhtarev IA, Kovgan LN, Jacob P, Anspaugh LR. Chornobyl accident: retrospective and prospective estimates of external dose of the population of Ukraine. Health Phys (2002) 82:290–303. doi: 10.1097/00004032-200203000-00002
60. Drozdovitch V, Khrouch V, Maceika E, Zvonova I, Vlasov O, Bratilova A, et al. Reconstruction of radiation doses in a case-control study of thyroid cancer following the Chernobyl accident. Health Phys (2010) 99:1–16. doi: 10.1097/HP.0b013e3181c910dd
61. Balonov M, Kaidanovsky G, Zvonova I, Kovtun A, Bouville A, Luckyanov N, et al. Contributions of short-lived radioiodines to thyroid doses received by evacuees from the Chernobyl area estimated using early in-vivo measurements. Radiat Prot Dosimetry (2003) 105:593–9. doi: 10.1093/oxfordjournals.rpd.a006309
62. Bouville A, Kryuchkov V. Increased occupational radiation doses: nuclear fuel cycle. Health Phys (2014) 106:259–71. doi: 10.1097/HP.0000000000000066
63. Chumak VV. Physical dosimetry of Chernobyl cleanup workers. Health Phys (2007) 93:452–61. doi: 10.1097/01.HP.0000278842.39156.93
64. Chumak VV, Sholom SV, Bakhanova EV, Pasalskaya LF, Musijachenko AV. High precision EPR dosimetry as a reference tool for validation of other techniques. Appl Radiat Isot (2005) 62:141–6. doi: 10.1016/j.apradiso.2004.08.029
65. Kryuchkov V, Chumak V, Maceika E, Anspaugh LR, Cardis E, Bakhanova E, et al. RADRUE method for reconstruction of gamma external doses to Chernobyl liquidators in epidemiological studies. Health Phys (2009) 97:275–98. doi: 10.1097/HP.0b013e3181ac9306
66. Kryuchkov VP, Kochetkov OA, Tovijanov AG. Mitigation of accident consequences at Chernobyl NPP: radiation and dosimetry issues. Moscow: Izdat (2012).
67. Drozdovitch V, Kryuchkov V, Chumak V, Kutsen S, Golovanov I, Bouville A. Thyroid doses due to Iodine-131 inhalation among Chernobyl cleanup workers. Radiat Environ Biophys (2019) 58:183–94. doi: 10.1007/s00411-019-00781-6
68. Cahoon E, Mabuchi K, Drozdovitch V, Little M, Hatch M, Gudzenko N, et al. Risk of thyroid cancer among Chernobyl clean-up workers in Ukraine. Environ Epidemiol (2019) 3:49. doi: 10.1097/01.EE9.0000606172.11860.ac
69. Drozdovitch V, Kryuchkov V, Bakhanova E, Golovanov I, Bazyka D, Gudzenko N, et al. Reconstruction of individual thyroid doses for case-control study of thyroid cancer among Ukrainian Chernobyl cleanup workers. Health Phys (2020) 118:18–35. doi: 10.1097/HP.0000000000001120
Keywords: Chernobyl, thyroid, radiation, exposure, Iodine-131
Citation: Drozdovitch V (2021) Radiation Exposure to the Thyroid After the Chernobyl Accident. Front. Endocrinol. 11:569041. doi: 10.3389/fendo.2020.569041
Received: 02 June 2020; Accepted: 17 November 2020;
Published: 05 January 2021.
Edited by:
Christoph Reiners, University Hospital Würzburg, GermanyReviewed by:
Michele Minuto, University of Genoa, ItalyFrancesco Frasca, University of Catania, Italy
Copyright © 2021 Drozdovitch. This is an open-access article distributed under the terms of the Creative Commons Attribution License (CC BY). The use, distribution or reproduction in other forums is permitted, provided the original author(s) and the copyright owner(s) are credited and that the original publication in this journal is cited, in accordance with accepted academic practice. No use, distribution or reproduction is permitted which does not comply with these terms.
*Correspondence: Vladimir Drozdovitch, ZHJvemRvdnZAbWFpbC5uaWguZ292