- 1IVI Foundation, Insituto de Investigación Sanitaria La Fe, Valencia, Spain
- 2IVI RMA, Madrid, Spain
- 3Department of Obstetrics and Gynecology, Rey Juan Carlos University, Madrid, Spain
Premature ovarian insufficiency is a common disorder affecting young women and represents the worst-case ovarian scenario due to the substantial impact on the reproductive lifespan of these patients. Due to the complexity of this condition, which is not fully understood, non-effective treatments have yet been established for these patients. Different experimental approaches are being explored and strategies based on stem cells deserve special attention. The regenerative and immunomodulatory properties of stem cells have been successfully tested in different tissues, including ovary. Numerous works point out to the efficacy of stem cells in POI treatment, and a wide range of clinical trials have been developed in order to prove safety and effectiveness of stem cells therapy—in diminished ovarian reserve and POI women. The main purpose of this review is to describe the state of the art of the treatment of POI involving stem cells, especially those that use mobilization of stem cells or paracrine signaling.
Introduction
In humans, oocyte development begins during fetal life and follicle pool reaches its maximum at 16/20 weeks of fetal development (1). Follicular decline is initiated before birth, so that, at the time of delivery, only about 1 million of follicles remain in the ovary of the baby. By the time of menarche, each ovary contains about 400,000 follicles and ovarian reserve continues decreasing as women age (2). Thereby, the decline in oocyte quantity and quality during women reproductive life is a physiological process; however, in some women, ovary deterioration occurs in an abrupt way and they become prematurely infertile.
Primary Ovarian Insufficiency (POI), also known as Premature Ovarian Failure (POF) or premature menopause, is a reproductive disorder, characterized by oligo-amenorrhea and high levels of serum FSH, leading to a cessation of ovarian function before the age of 40 (3). This condition affects 1% of women under the age of 40 years, and 1 out of 250 women under the age of 35 years (4).
POI is characterized by a hypergonadotropic hypogonadism state, which can be diagnosed by a triad of features in a woman under the age of 40: (a) postmenopausal levels of follicle-stimulating hormone (FSH) (>40 UI/L in two different samples taken separately in the time), (b) 4 or more months of amenorrhea, and (c) decreased estradiol serum concentrations (3). These patients present low AMH serum levels and a low antral follicle count (AFC).
This condition represents a dramatic scenario, as ovarian dysfunction leads to female infertility in POI patients. Laparoscopy shows a lack of follicle development in POI patients and dysfunctional ovaries lead to estrogen deficiency. The uterus and vaginal mucosa undergo atrophy, which is very often associated with dyspareunia (5, 6). In addition, POI involves menopausal syndrome, which may include hot flushes, night sweats, heart palpitations, insomnia, or headaches. Moreover, POI is associated with long-term negative consequences in female health, such as an increased risk of immunological disorders, cardiovascular diseases, and osteoporosis (7).
It should be noted that 5–10% of women with POI might have spontaneous follicular development, menses resumption, or spontaneous pregnancies, especially during the first year after diagnosis (8). This could be due to the fact that ovarian biopsies from POI patients revealed that up to 9% of women have plenty primordial follicles, and 30% have some primordial follicles (5, 9). However, ovulation is unpredictable and most women with POI have a low chance of pregnancy (5, 6, 10).
There are limited options for POI patients, whose treatment may be oriented to reduce the impact of endocrine dysfunction—by means of therapy hormone replacement—and/or to overcome infertility. None of these strategies are absent from limitations. On the one hand, hormone replacement therapy has been associated with an increased risk of reproductive cancer (11–15). On the other hand, the treatment of POI-associated infertility by reproductive techniques with autologous gametes represents a major challenge in reproductive medicine, and usually involves prolonged protocols and inconsistent clinical outcomes. Despite the intensive effort to develop variants of stimulation protocols to improve reproductive outcomes in poor responder patients, intrinsic characteristics of POI patients make its management even more difficult; probably, because of the absence of antral follicles responding to stimulation. To date, there is no enough evidence to recommend most of these strategies in order to improve pregnancy rates in POF patients (16, 17). In most cases, the only options for these patients to achieve desired motherhood is oocyte donation or adoption, which are not always accepted due to ethical, cultural, or religious issues. Thus, because of the complexity of this disorder, a standard and effective treatment has not yet been established; but an active management of these patients and an intensive search for new strategies may open new doors for them.
Etiology
The human ovary contains a limited and non-renewable pool of quiescent follicles determined at birth. Folliculogenesis is a complex process that should be extremely regulated. During this process, granulose and theca cells assist the oocyte, in order to promote primordial follicle development towards antral stage and ovulation (10) (Figure 1). Intraovarian mechanisms activate a small number of primordial follicles (≈1,000/month) and although most underwent atresia, a few of them achieved the advanced maturation stage before ovulation (18). Follicle depletion occurs at menopause when less than 1,000 quiescent follicles remain (19). In POI, this process is altered. It is suggested that follicular dysfunction and altered follicle depletion may underlie POI (20). Although scientific knowledge is limited about factors controlling oocyte pool and the cause of POI is not yet completely understood, different factors could alter follicle maintenance and development. In fact, POI can appear spontaneously or induced by different factors (21).
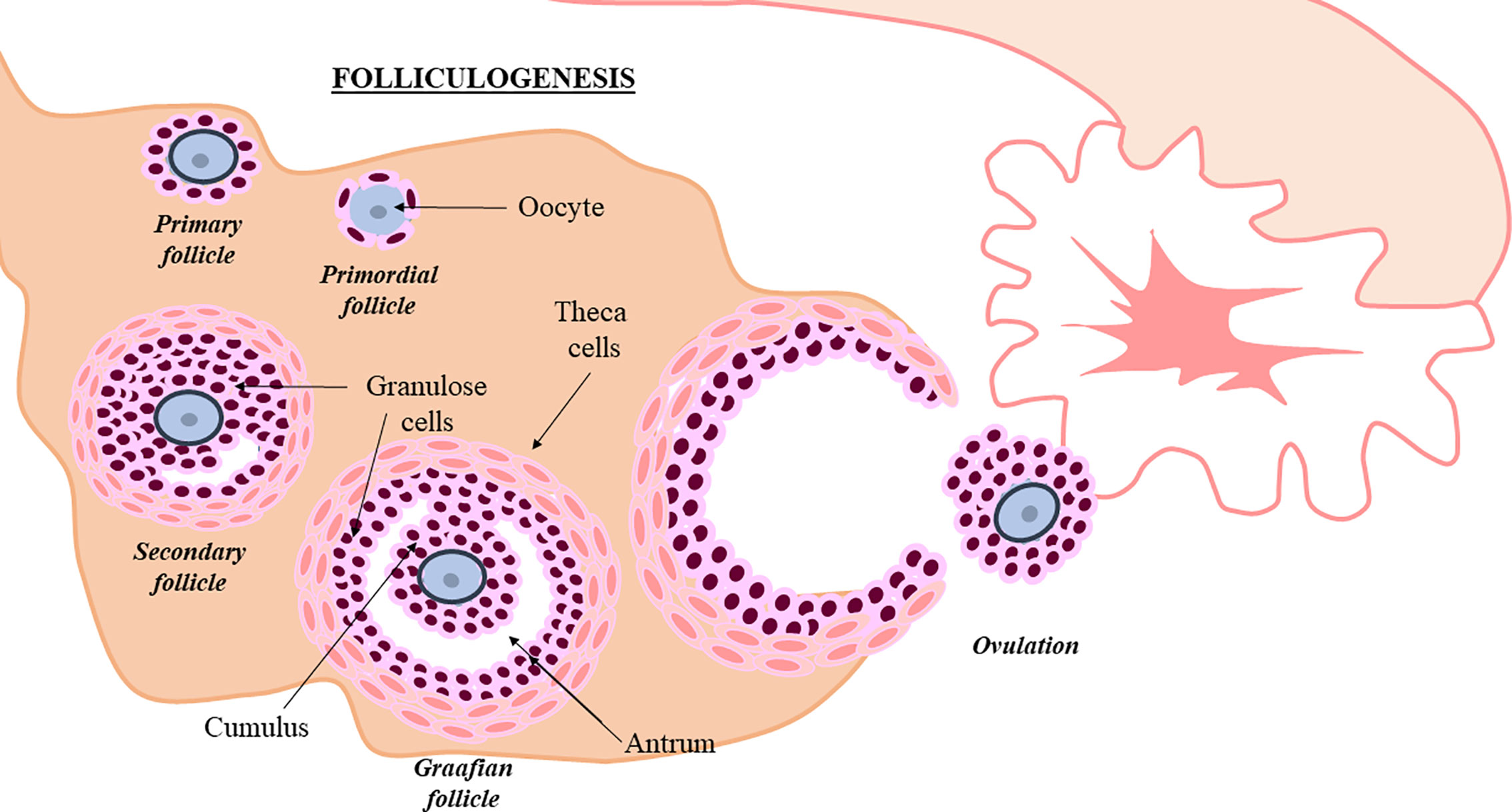
Figure 1 Follicullogenis. Granulosa and theca cells assist oocyte progression towards ovulation (10). Follicle development is shown in the picture.
The most common cause of POI are oncologic treatments with high doses of chemo- and radiotherapy (22). The increased survival rates (>80%) of oncologic patients associate a growing percentage of young women facing gonadotoxic side effects of cancer therapies without having accomplished their reproductive project. Deleterious effects on the ovary depend on the age of the patients—the risk to develop POI after cancer therapy increases with the age—the dosage and type of toxic agent (having the alkylating agents the highest risk for developing POI). A main mechanism of chemotherapy-induced ovarian failure is based on the damage induced to DNA of primordial follicles, leading to apoptosis, and promoting a massive activation of follicles followed by atresia and elimination. This follicular depletion also associates an impairment of ovarian vascularization, fibrosis, or interrupting cross talk communication between follicular cells (23, 24), leading to a cessation of ovarian function
Iatrogenic factors, such as laparoscopy, ovarian drilling, or surgery for ovarian endometriosis or cysts, may also lead to POI (25). Others environmental factors such as viral infections or pollutants can result in POI, although the real incidence of these cases is not clear (26, 27).
Genetic defects, including X chromosome aneuploidies (Turner syndrome, trisomy X) (28–30), structural X chromosome anomalies (isochromosome, deletions, inversions, duplications) (31), mutations or premutations of X linked genes (Fragile X syndrome) (31), and single mutations in genes related to reproductive function (FSH receptor, LH receptor, inhibin, galactosaemia) constitute another cause of POI (32). Enzymatic deficiencies in the steroidogenesis pathway could also lead to POI (22).
Finally, autoimmune mechanisms are involved in pathogenesis of more than a 4% of POI cases (33) and autoimmune disorders such as myasthenia gravis, celiac disease, vitiligo, lupus, Addison’s disease, or autoimmune polyglandular syndrome, have been seen in a percentage of women diagnosed with POI (22, 34). In these patients, immune alterations including an increase in CD4+ T cells and B cells, macrophage and dendrite cells disorders, lymphocytes oophoritis, and inappropriate expression of class II MHC antigens by granulose cells have been found (35–37). In fact, anti-ovary antibodies—with several targets—have been detected in 50% of unexplained infertile patients and several studies report that the presence of autoantibodies increases the risk to develop POI in patients with autoimmune disease (22, 34).
However, most cases of POI are idiopathic (22), which promote further investigations to utterly understand this entity, in order to explore new strategies to solve it. Even in cases with a diagnosed cause, the diversity of disorders associated with POF indicates the heterogeneity of this entity. This fact underlines the need not only to develop different strategies to improve clinical management of these patients, but also the importance of the selection of the right population of POI patients, who can benefit from each approach.
Novel Strategies for POI Management
Recent research has focused the attention on the residual quiescent pool of follicles that remain even when the ovary loses their ability to ovulate and function. Based on the successful protocol of in vitro activation (IVA) of primordial dormant follicles, Kamawura et al. was the first group exploring the combination of IVA with mechanical ovarian fragmentation, to inhibit the Hippo pathway, in menopausal women (38). Additional research has been developed to improve the success of the technique and to design a less invasive procedure, named as one step IVA or ovarian fragmentation for follicular activation (OFFA). The technique consists in a unique surgery for ovarian cortex retrieval, followed by mechanical fragmentation into small pieces and transplant into an ovarian grafting site. By means of this strategy, 10 premenopausal women have achieved a pregnancy (39–43) as well as several poor ovarian responders (POR) (44). OFFA pursues not only fertility recovery but also endocrine function and as avoids ovarian cryopreservation, the main concern of ischemia-associated follicle death is also overcome.
Artificial ovary is also a promising alternative that will be used to in vitro growth and maturation approaches or to improve ovarian transplant in the future. Although it has been applied successfully in animal models, its efficacy and safety have to be proved before it becomes a reality for patients (45).
Another strategy, closely related to the previous one, is the generation of artificial gametes in patients who are not able to produce functional gametes. Artificial gametes could be generated from induced pluripotent stem cells (iPSCs), embryonic stem cells (ESCs) derived from blastocysts inner cell mass, or the putative germline stem cells (GSCs) (45). In animals, artificial gametes have been generated from GSCs (46), ESCs (47), and iPSCs (48, 49). The implementation of this technique in animal preclinical models has even achieved the birth of viable offspring (48, 49). The main limitation of the technique—apart from multiple ethical concerns—is the low efficacy of differentiation. In humans, artificial oocyte-like cells have been developed from ESCs (50) and GSCs (46) and the successful fertilization of artificial oocyte-like cells has been reported (51). However, development potential and human offspring from artificial gametes is to date far from reality (52). In relation to this strategy, transplantation of ovarian granulosa-like cells derived from human IPSCs has been reported to repair ovarian niche and to promote follicular development in POF mice (53).
Different studies have described a lower telomere length and telomerase activity in POF patients (54–56). Concerning this pathway, the reactivation of telomerase—which maintains telomere length—has been described to resume fertility in telomerase-deficient mice, which present impaired fertility (57, 58), opening a new future possibility to ovarian rejuvenation by means of telomerase reactivation (59–61).
Based on the autoimmune dysregulation associated to a wide percentage of POI cases, together with the close association of fibrosis with ovarian failure, some authors suggested the possible effect of immunomodulating therapy for ovarian function recovery in POF patients, especially in those with autoimmune-related POF (34, 62, 63). In fact, use of the anti-inflammatory and antioxidant properties of several agents has been proved to improve ovarian function in a POI mice model (62, 64).
Stem Cell–Based Approaches
Preclinical Studies
Most of these strategies highlighted the relevance of the ovarian niche as a key parameter to promote an adequate follicle development in order to restore ovarian function. Following this idea, one of the most promising strategies pursues the regeneration of ovarian niche using Stem Cells (SCs) in order to promote development of remaining follicles within the ovary.
With the rise of regenerative medicine, different types of SCs have been tested for follicular rescue and regeneration of the ovarian niche. Among them, mesenchymal stem cells (MSCs) have been the most widely used for these strategies. MSCs are a population of SCs that can be derived from different adult tissues, and that have proliferative, self-renewal and differentiation to different lineages properties (65). Different studies in animal models with different degrees of ovarian damage describe the ability of MSCs to restore ovarian function in these animals.
Mesenchymal stem cells from human and murine amniotic fluid have shown the ability to survive and proliferate in the ovary and to rescue short-term fertility of mice with chemotherapy (QT)-induced POF after injection into the ovarian artery (66, 67). Wang et al. report the ability of amniotic epithelial stem cells (AESCs) to infiltrate the damaged ovary after injection into the tail of mice with POF (68), leading to the recovery of folliculogenesis and differentiation towards granulosa cells (68, 69). Ding et al. show the recovery of the follicle pool in all its developmental stages and hormonal restoration after the tail injection of both AESCs and amniotic mesenchymal stem cells (AMSCs) in mice with different degrees of ovarian failure induced by chemotherapy (70). Although AESCs seem to show less immunological rejection, AMSCs show a higher efficacy in the recovery of ovarian function, especially in the most drastic cases of POF (70). Ling et al. evaluate the improvement of the treatment with human AMSCs by pretreating them with low intensity pulsed ultrasound (LIPUS). Both LIPUS-pretreated AMSCs and non-pretreated AMSCs have been reported to increase reproductive organ weights, reduce granulosa cells (GCs) apoptosis and ovarian inflammation, and improve ovarian function in POI rats (71).
MSCs can also be obtained from the umbilical cord. The injection of umbilical cord mesenchymal stem cells (UCMSCs) into the tail vein allows improvement of the ovarian structure and ovarian function—at the hormonal and follicular level—in mice with POF induced by QT and in rats with natural ovarian aging. GCs apoptosis reduction and cytokines secretion leading by UCMSCs are proposed as possible mechanisms of action (72–75). Zhu et al. report that the recovery of ovarian function and fertility after UCMSCs transplantation occurs sooner when UCMSCs are injected directly into the ovarian artery (76). It has also been reported a long-term survival of UCMSCs in the rat ovary after the transplant (73) and stabilization of the ovarian epithelium by these SCs (74). Both human UCMSCs and AMSCs interventions restore ovarian morphology elasticity and toughness, and involve a slight recovery of ovarian function in QT damaged ovaries (77).
Menstrual blood is another possible source of MSCs. Human menstrual blood-derived stem cells (MenSCs) are endometrial MSCs, which have also been used for the treatment of POI. Thus, the ability of these cells to migrate to the ovary has been reported, and ovary infiltration by MenSCs is followed by hormone levels restoration and follicular count increase in mice with QT-induced POF (78–80), as well as the restoration of fertility in these mice (78). The reduction of both fibrosis and apoptosis through cytokine secretion has been suggested as possible mechanisms to restore ovarian function by MenSCs (78, 79). Feng et al. also described a possible role of these cells in the regulation of folliculogenesis (80). Recovery of ovarian function has been also achieved by injecting only the culture medium of MenSCs, which reinforces the idea of paracrine action of these cells (79). Thus, MesSCs would represent an interesting alternative due to the possibility of non-invasive collection. Nevertheless, most of POI patients present amenorrhea or oligomenorrhea, which reduces the application of this strategy.
Adipose tissue-derived mesenchymal stem cells (ADMSCs) have also shown the ability to restore ovarian function, increasing the number of follicles after injection into the ovary in QT induced POF mice and rats (81–83). An improvement in estradiol serum levels and an increase in the gestation rate have been reported after ADMSCs transplant (82, 83). Fouad et al. report a therapeutic efficacy of both human AMSCs and ADSCs, but with a greater efficacy of the former, which achieve not only an increase in estrogen levels, but also a decrease in FSH levels in mice with POF (83). ADMSCs have been suggested to produce cytokines and reduce apoptosis in GCs (81). However, a low long-term permanence of these cells in the ovary has been reported (81). The transplantation of soluble collagen with ADSCs improves the short-term permanence of ADSCs in the ovaries and contributes to the restoration of ovarian function (82). Takehara et al. also describe a restoration of ovarian function after local injection of male ADMSCs into female mice with QT induced POF, and note that the Y chromosome only appears in theca cells and not inside the follicle. They report an increase in secreted levels of vascular endothelial growth factor (VEGF), insulin-like growth factor (IGF), and hepatocyte growth factor (HGF) (84).
Although promising results obtained for ovarian rescue with different types of MSC, their clinical application requires cell culture procedures to reach clinically relevant cell numbers for transplant. This represents a main limitation for use, as the accumulation of genomic and epigenomic alterations and degeneration in progenitor potency in human SCs have been associated to cell expansion procedures (85).
Bone marrow derived stem cells (BMDSCs) present an interesting alternative for transplantation in women with POI (Table 1). The possibility of obtaining a large number of BMDSCs, from an autologous source, by means of well-established clinical protocols—used for BM transplant after QT—makes them a valuable candidate (96). This possibility lets us avoid cell expansion steps, which is associated with genetic instability (97). In 2007, Lee et al. described fertility rescue in a QT-induced POF rat model after injection into the tail of BMDSCs derived from another rat. All the offspring belonged genetically to the recipient rat, although donor immature oocytes were described (86). Fu el al., in 2008 describe the improvement of ovarian function after the local injection in the ovary of BMDSCs derived from human in mouse with POF, possibly mediated by the reduction of apoptosis in GCs (87). The ovarian function improvement with BMDSCs has also been proved by prolongation of reproductive potential in mice beyond the common age of reproductive senescence with monthly infusions of BMDSCs from young mice (88). The increase in the number of follicles and the size of the reproductive organs, as well as the restoration of hormonal levels have been described in genetically generated POF mice, and QT induced POF mice, rats, and rabbits after heterologous distal BMDSCs transplantation (90, 91, 98). In 2014, Liu et al. describe for the first time the ability of BMDSCs to migrate into POF-damaged rat’s ovary, where they were not distributed in a uniform manner. Their presence was described to be higher in the medulla than in the cortex. Following BMDSCs transplantation, increased estradiol levels and antral follicle count were reported (89). Different studies describe not only the regenerative potential of BMDSCs, but also a protective property, with a reduction of apoptosis in ovarian cells and lower germ cell DNA damage when combining chemotherapy with injection of BMDSCs (99, 100). Heterologous transplant of BMDSCs also shows the potential to reverse the hormonal dysfunction caused by QT in mice and an increase in the number of healthy follicles has been showed after BMDSCs tail vein injection (92). The ability of autologous BMDSCs transplant to restore fertility and shorten estrous cycles has also been reported after SCs injection in the ovarian artery in a QT-induced POF mouse model (93). After that, the ability of human BMDSCs transplant to increase ovarian weight and follicular count, and to improve pregnancy rate after injection into the ovarian artery has been shown in a mouse model with QT-induced POF (94). Herraiz et al. described the restoration of fertility in a mouse model with ovarian damage after transplantation of human BMDSCs, with an increase in the number of preovulatory follicles, MII oocytes, spontaneous pregnancy rate, and number of healthy offspring (95). Furthermore, this study shows for the first time, the ability of BMDSCs to migrate towards the follicles and vessels in human tissue POR women xenografted into immunodeficient mice, promoting follicular development, ovarian local vascularization, estradiol secretion, and reducing apoptosis (95).
In spite of the greater potential of MSCs, others SCs sources have been explored. Liu et al., described the potential of human embryonic stem cell (ESCs) to restore hormone levels and increase follicular count in mice, after the vein injection in vesicles, possibly by means of the apoptosis reduction (101). However, the use of ESCs, which may be obtained from the blastocysts inner cell mass is not exempt from ethical concerns.
In light of reported studies and advances in this field by using preclinical animal models, there are higher expectations regarding the use of MSCs, and especially BMDSCs to restore ovarian function in humans.
Pilot Studies and Clinical Trials in POI Patients
The firsts clinical trials developed in humans using MSCs from bone marrow (BM) required iliac crest aspiration for cell collection followed by SCs isolation and in vitro culture procedures to reach clinically relevant cell numbers (Tables 2.1 and 2.2).
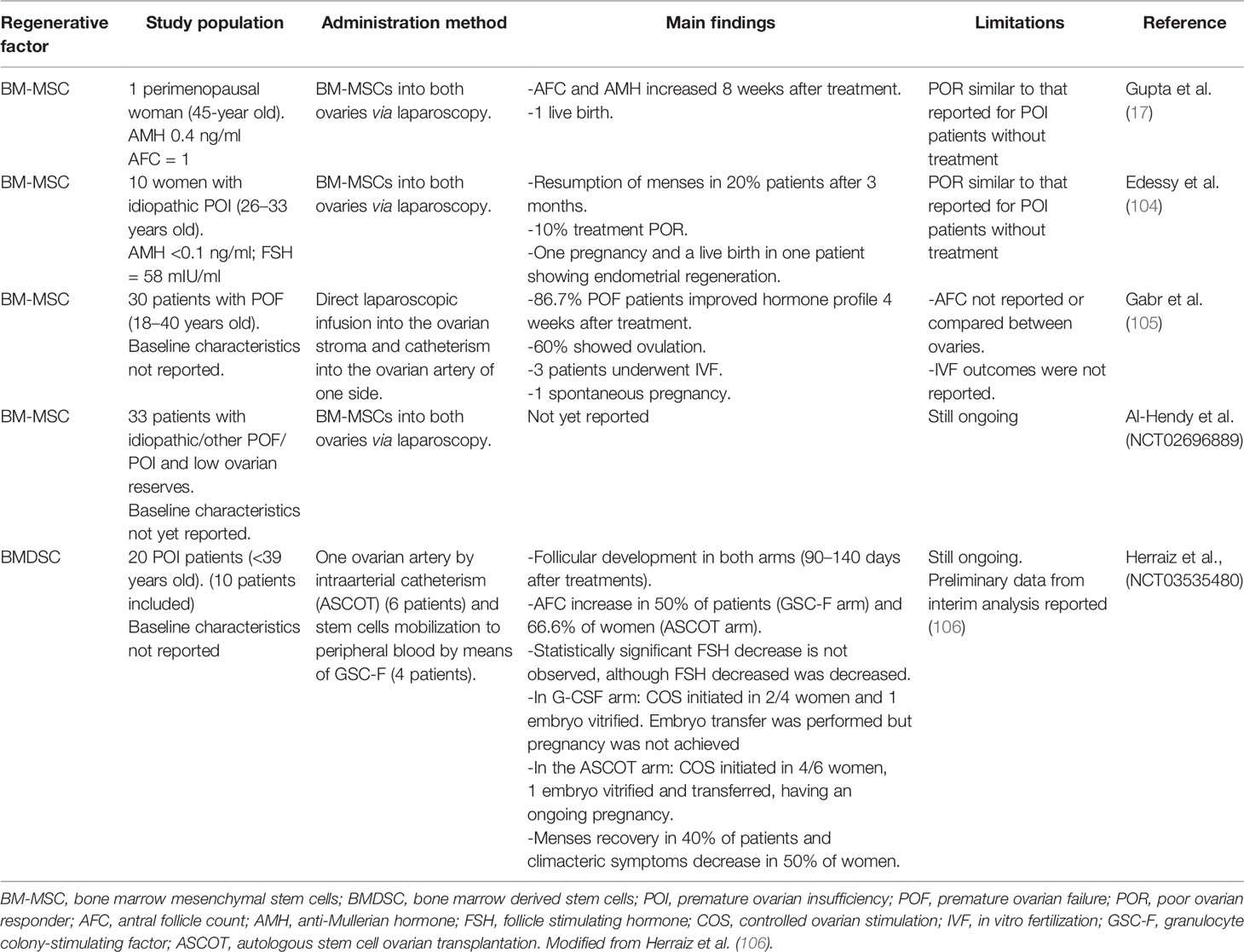
Table 2.2 Human studies involving bone marrow stem cell treatment for POI and perimenopausal patients.
Gupta et al. (17) published a live birth in a postmenopausal woman (45 years old) underwent this technique and IVF treatment. They injected cells in both ovaries by laparoscopy. This means to expose the patient to two different invasive procedures: first the iliac crest aspiration, and second the laparoscopy. Edessy’s group followed the same technique, in 10 POI younger women (26–33 years old) with positive results showing a return of menses in two patients and one ongoing pregnancy, with one live birth (104). Gabr et al. (105) later applied this same method in 30 POI women (18–40 years old). This study, instead, had two branches: one arm received these cells by direct ovarian injection through laparoscopy, while the second arm had cells injected through de ovarian artery. One spontaneous pregnancy was obtained. Al Hendy and colleagues are carrying out similar studies as the above described in POR and POI patients, after their promising results in animals, but their investigations are still ongoing.
We recently described that infusion of BMDSC promotes human and mouse follicular growth by increasing ovarian vascularization, stromal cell proliferation, and reducing cell death (95). Based on this information, a prospective pilot study in 17 POR women was developed by our group to evaluate the effects of autologous stem cell ovarian transplant (ASCOT) on ovarian reserve (102). ASCOT improved ovarian function biomarkers (AMH and AFC) in 81.3% of women and a total of six pregnancies and three healthy babies were achieved. ASCOT improved follicle and oocyte quantity enabling pregnancy in POR women previously limited to oocyte donation. In the context of ovarian tissue, stem cell paracrine actions should be evaluated for their capacity to activate the pre-existing quiescent follicles based on the ability of BMDSCs to produce and secrete a broad variety of growth factors involved in follicular growth, angiogenesis, viability, and ovarian response to Controlled Ovarian Stimulation (COS) (107). In fact, our results suggest that ASCOT optimized the growth of existing follicles, mediated the presence of specific stem cell secreted factors such as FGF-2 and THSP-1 within aphaeresis. Based on that, a randomized pilot study (NCT03535480) has been designed with 20 POI women younger than 39 (106). Patients will be randomized to the ASCOT or only stem cell mobilization based on the ability of ovarian niche to attract undifferentiated cells from BM in a process known as “homing’’ (89).
It is important to highlight that many other stem cell origins are also being tested worldwide in several RCT involving POI women. Nevertheless, most of them are still ongoing and therefore results have not been yet reported (Table 3).
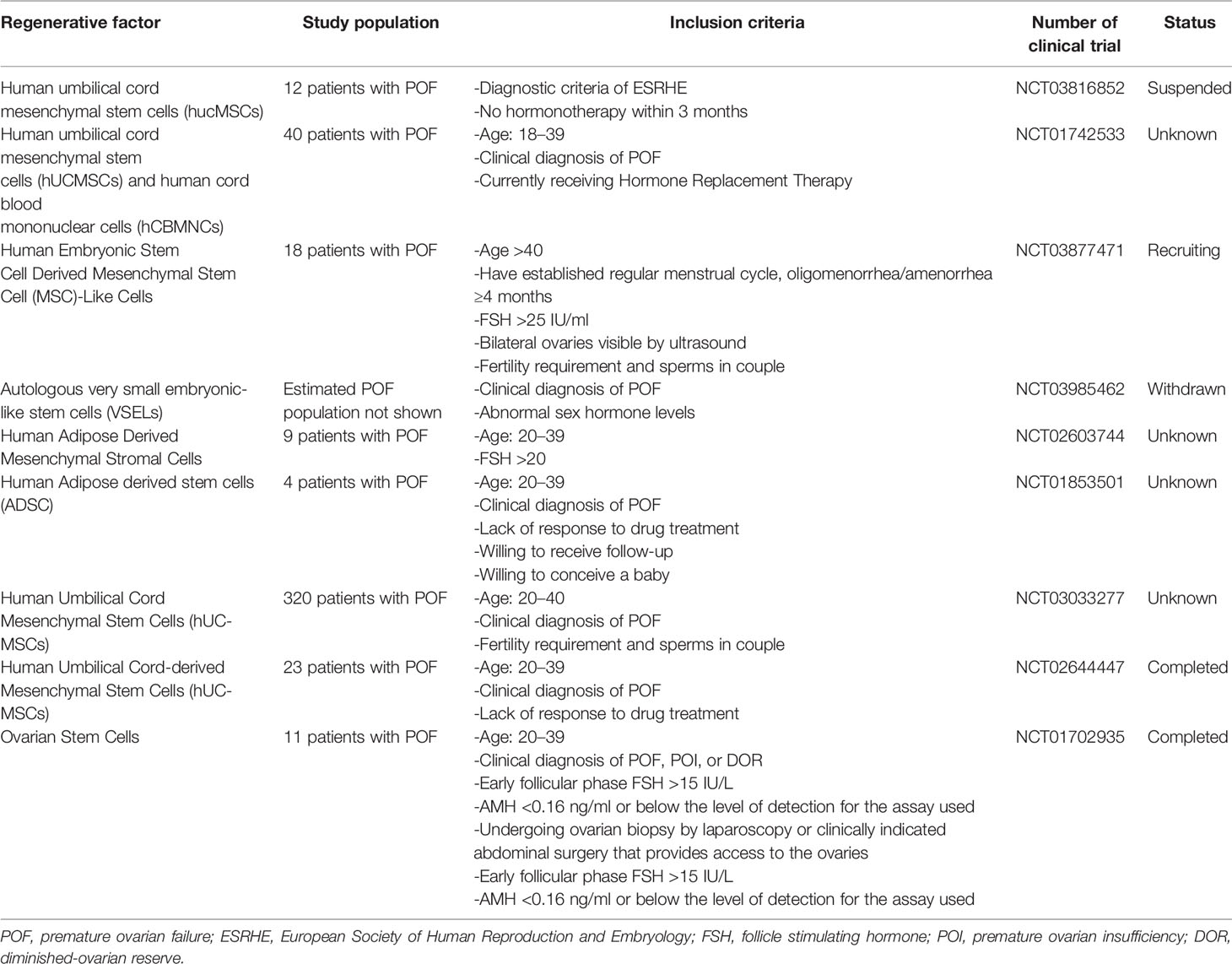
Table 3 Registered Randomized Clinical Trials involving different sources of SCs—apart from Bone Marrow Derived Stem Cells—for POF/POI patients.
Proposed Mechanisms for Stem Cell Therapy
As it has been described, different studies showed that BMDSCs are effective for POI treatment in animals (and present promising results in humans) (103). To understand the underlying mechanisms would allow us to optimize these strategies and to find the optimal cohort of patients who will be benefited.
Overall, SCs show the ability to act in a paracrine manner thanks to the secretion of soluble factors and chemokines (75, 79, 81, 91). Paracrine action could help to restore damaged tissue, in this case the ovarian niche, by regulating different vital processes in this microenvironment. In this context, different studies show the involvement of BMDSCs in the regulation of angiogenesis, apoptosis, the regulation of the immune system, and fibrosis in the ovary (108) (Figure 2).
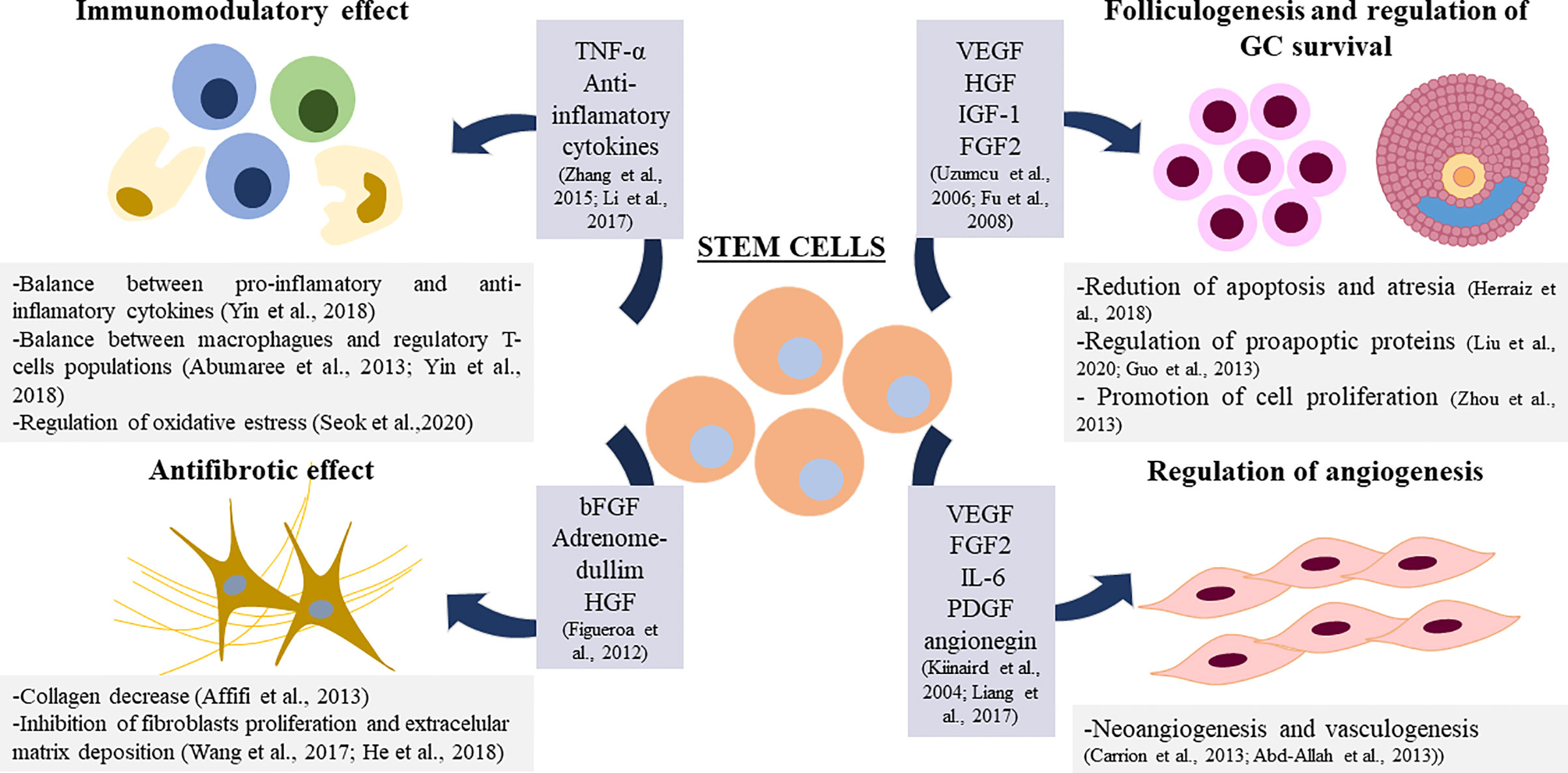
Figure 2 Proposed mechanisms for Stem Cell Therapy in ovarian damage. TNF-α: Tumor Necrosis Factor α, VEGF: Vascular Endothelial Growth Factor, HGF: Hepatocyte Growth Factor, IGF-1: Insuline Like Growth Factor 1, bFGF: Basic Fibrolast Growth factor, FGF2: Fibrolast Growth Factor 2, IL-6: Interkuline 6, PDGF: Platelet Derived Growth Factor.
In the context of angiogenesis regulation in the ovary mediated by BMDSCs, the increase of vascularization in the ovarian niche, improve the healing process that occurs in a cyclic manner, and it may be beneficial for ovarian recovery (91). It has been reported that factors produced by these cells such as Vascular Endothelial Growth Factor (VEGF), Fibroblast Growth Factor-2 (FGF2) and Interleukine-6 (IL-6) promote arteriogenesis in vitro and in vivo (109). BMDSCs have been shown to promote angiogenesis in vitro through the α5 β1 receptor (110) and through Platelet Derived Growth Factor (PDGF) (111). In ovarian tissue, angiogenin produced by BMDSCs has been reported to play a positive role in angiogenesis after transplantation (112).
Regarding the antiapoptotic-promotion property of BMDSCs in GCs in the ovary (72, 81, 87, 95, 101), it has been reported that the coculture with BMDSCs decreases the levels of the proapoptotic proteins P21 and BAX and increase the levels of the proto-oncogene c-myc in GCs (100, 101). It has been observed that different cytokines present in the BMDSCs culture medium—VEGF, HGF, IGF-1—are able to decrease apoptosis of granulosa cells in vitro (113) and in vivo (87) and promote their proliferation (114); which suggests that the secretion of these growth factors may be underlying the apoptosis decrease found after BMDSCs in the ovary.
The immunomodulatory effects of BMDSCs have been tested in vitro and in vivo in different diseases (115, 116). The regulation of the balance between different populations of immune cells or between pro-inflammatory and anti-inflammatory cytokines mediated by BMDSCs could underlie this immunomodulatory effect (108). MSCs have been reported to have immunoregulatory properties in the ovarian niche by regulating populations of macrophages, regulatory T lymphocytes, and associated cytokines (117, 118). TNF-alpha has also been associated with the immunoregulatory function of human MSCs in the ovary (119). MSCs have been reported to reduce SOD dismutase in the ovary after transplantation, suggesting that the recovery of the ovarian niche could also be due to a regulation of oxidative stress in this microenvironment (108, 120).
In relation to fibrosis decrease, after BMDSCs transplantation, a decrease in collagen levels has been observed (121), suggesting that the mechanism of action of these cells in the recovery of ovarian damage could also involve an antifibrotic effect (79). In fact, BMSCs may inhibit fibroblasts proliferation and decrease the level of extracellular matrix deposition (108).This antifibrotic effect has been associated with certain soluble factors such as HGF, adrenomedullim and Basic Fibroblast Growth Factor (bFGF) (122).
As it is said, the paracrine action of BMDSCs in the ovarian niche has been demonstrated by injecting only soluble factors from the culture medium of the SCs, obtaining similar results as with SCs transplant (79). In fact, the ASCOT clinical trial in POR women highlighted the fact that aphaeresis provided relevant components for success, including specific BMDSC-secreted soluble factors, which acting in a paracrine manner promote growth of the already existing residual follicles in impaired ovaries. This study found that positive response was not limited to the injected ovary, as circulating SCs during the mobilization phase also reached the non-injected ovary producing an increase in the AFC in both sides (102). Furthermore, there are recent reports of nervous tissue rejuvenation and repair by injection of young growth factor enriched plasma, umbilical cord blood plasma, and plasma specific proteins into damaged and aged organisms (123–125).
This fact opens up new possibilities to combat damage in ovarian tissue such as the injection of soluble factors or platelet-rich plasma (PRP).
The PRP Approach
PRP injection has been used for years in several fields of Medicine (orthopedics, sports Medicine, aesthetics, etc.) but in the context of assisted reproductive techniques (ART), intraovarian injection of autologous PRP has been recently proposed as an alternative to restore ovarian function in POI women (Tables 4.1 and 4.2). This approach is also based on the paracrine signaling, as PRP is a concentrate composed by platelet-enclosed growth factors, which could promote tissue healing, angiogenesis and cell growth (132, 133). Pantos et al. (129) introduced by first time this new approach without the direct use of SCs to reactivate folliculogenesis in perimenopausal women. In this study, the ovaries of eight perimenopausal women of advanced maternal age (41–49 years old) were infused with platelet-rich plasma by transvaginal ultrasound-guided injection. Treatment resulted in restoration of menses, with presence of ovarian follicles that allowed oocyte retrieval after IVF treatment in all patients and cryopreservation of 1.50 ± 0.71 embryos. However, a limitation of the study is that it only included eight women and did not document their previous ovarian reserves. These effects might be due to an increased in ovarian vascularization, with a key role in ovarian function as well as in promoting follicle development increasing follicular cell proliferation and survival (95). After this first report, ovarian and endocrine positive effects and live births have been also reported in several series of patients with impaired ovarian function such as POR (127) and POI women (130, 134).
Sills et al. (126) showed, in a study population of aged women (42 ± 4 years; infertility duration 60 ± 25) with diminished ovarian reserves, that intraovarian administration of PRP was able to induce an increase in serum AMH and a decrease in serum FSH, sufficient to permit oocyte retrieval (5.3 ± 1.3 MII) and blastocyst cryopreservation in all recruited patients 2 months after treatment.
The firsts controlled clinical trials involving a relevant number of patients with different and properly characterized ovarian phenotypes have been published in the last year. Sfakianoudis et al. (128) reported four pilot studies on POR, POI, perimenopausal, and menopausal women with a total of 120 participants recruited (n = 30 each). In the case of POR women (38.4 ± 2.0 y.o.), they found that PRP injection was able to improve ovarian reserve biomarkers, as AMH levels as well as AFC increased in the first and second menstrual and remained stable in the third while FSH and LH levels were reduced in the first menstrual cycle and remained stable. The main ICSI cycle outcomes were increased, especially the number of oocytes retrieved and the number of MII and embryos, all together with a reduction of the cancelation rate, a main concern in POR women. Overall, the reported clinical pregnancy rate for POR was 46.6% (14 out of 30 women) with 12 participants having a live birth.
For the other three pilot studies included, the reported primary outcomes were different according to the diagnosis of the recruited patients, as for menopause and POI women menses recovery and FSH levels became a principal result. In the POI population (35.9 ± 1.9 y.o.), they observed that 18 women (60%) positively responded to PRP treatment when considered as menstrual cycle restoration and reduced FSH levels, with a total of three pregnancies and three live births (PR:10%). These results slightly improved in the perimenopausal women (43.4 ± 1.4 y.o.), where 24 women (80%) positively responded to PRP treatment. For these women menstrual cycle regulation as well as FSH level reduction was observed having four natural conceptions and three live births (PR:13.3%). Finally, in the menopausal group (48.8 ± 1.6 y.o.), 13 women (43.3%) positively responded to PRP treatment with one pregnancy and one live birth (1%).
To date, the largest study has been developed by Cakiroglu et al. (131), in a population of 311 women (34.8 ± 4.3 y.o.) with POI diagnosis based on the ESHRE criteria. After intraovarian injection of autologous PRP, the 7.4% of POI women (23/311) achieved a spontaneous pregnancy one or two menstrual cycles after treatment with 7 miscarriages and 16 live births reported. From the remaining patients, development of at least one antral follicle was noticed in 201 allowing the initiation of controlled ovarian stimulation from the second to the sixth menstrual cycles after intervention, although oocyte retrieval was only achieved in 130 and MII-oocytes obtained in 93 women. The 40.8% of stimulated women obtained at least one cleavage stage embryo scored as A/B according to morphological criteria. To date, only 57 of these patients underwent embryo transfer (both fresh or frozen) as the remaining ones having embryos decided to cryopreserve them for a later transfer. A total of 13 achieved a pregnancy after ET (22.8%) although 4 experienced a miscarriage. Nevertheless, FSH levels did not improve after treatment when compared to previous values, although AMH and AFC increased. Overall, this study reported a total of 36 pregnancies in 311 women (11.5%PR) and 8% of live birth rate or sustained implantation, which although opening a new path for the management of POI women. It is relevant for the overall evaluation of these rates to highlight that at the moment of publication several patients still had their embryos cryopreserved for future transfer.
All together, the studies evaluating PRP ovarian injection are encouraging as they open a new path to a clinical alternative more easily applied than the stem cell based therapies as ovarian injection is performed in a similar intervention to oocytes collection. Nevertheless, their results should be evaluated with caution as for now there are no experimental studies evaluating the wide spectrum of PRP effects, duration, and mechanism in the ovarian tissue, and the reported human studies lack from an adequate control group to properly establish the efficiency of the technique. Thus, results of placebo in double blinded randomized clinical trials should be obtained and carefully evaluated before proposing PRP as a routine treatment for POI and DOR patients in ART clinics. Furthermore, it is important to bear in mind that POI pregnancy rates across studies ranged from 2.2 to 14.2% and spontaneous resumption of ovarian function occurs in 25% of patients, and primordial and pre-antral follicles are frequently found in ovarian biopsies from women diagnosed as having POI (8).
Conclusions
This field of investigation opens new opportunities for ovarian rescue in women with impaired ovarian reserve, such as POR and POI patients, by different strategies focused on the rescue of already existing follicles. These approaches include the inhibition of molecular pathways by IVA and tissue mechanical fragmentation, stem cell administration, and PRP ovarian injection. Although heterogeneous, all the techniques have a common characteristic, to promote growth of follicular cells by activating different paracrine signaling mechanisms. This finding is of paramount relevance for the future design of feasible and less invasive clinical options. Nevertheless, these proposals should be previously supported by comprehensive experimental and mechanistic studies. The inclusion of a proper control group should be mandatory in future randomized clinical trials for a realistic evaluation of the technique’s efficacy in selected group of patients.
Author Contributions
AP has performed a bibliography search and analysis and she has written the manuscript. JG-V has critically revised the manuscript, and he has coordinated the study. SH has performed a literature search and analysis, she has written and critically revised the manuscript, and she has coordinated the study. All authors contributed to the article and approved the submitted version.
Funding
This work was partially supported by grants FPU18/02904 and CP19/00141 from the Spanish Ministry of Science, Innovation and Universities for the participation of AP and SH, respectively.
Conflict of Interest
The authors declare that the research was conducted in the absence of any commercial or financial relationships that could be construed as a potential conflict of interest.
References
1. Broekmans FJ, Knauff EA, te Velde ER, Macklon NS, Fauser BC. Female reproductive ageing: current knowledge and future trends. Trends Endocrinol Metab (2007) 18(2):58–65. doi: 10.1016/j.tem.2007.01.004
2. Hansen KR, Knowlton NS, Thyer AC, Charleston JS, Soules MR, Klein NA. A new model of reproductive aging: the decline in ovarian non-growing follicle number from birth to menopause. Hum Reprod (2008) 23(3):699–708. doi: 10.1093/humrep/dem408
3. Cohen J, Chabbert-Buffet N, Darai E. Diminished ovarian reserve, premature ovarian failure, poor ovarian responder–a plea for universal definitions. J Assist Reprod Genet (2015) 32(12):1709–12. doi: 10.1007/s10815-015-0595-y
4. Coulam CB, Adamson SC, Annegers JF. Incidence of premature ovarian failure. Obstet Gynecol (1986) 67(4):604–6.
5. Rebar RW, Erickson GF, Yen SS. Idiopathic premature ovarian failure: clinical and endocrine characteristics. Fertil Steril (1982) 37(1):35–41. doi: 10.1016/S0015-0282(16)45973-X
6. Rebar RW, Connolly HV. Clinical features of young women with hypergonadotropic amenorrhea. Fertil Steril (1990) 53(5):804–10. doi: 10.1016/S0015-0282(16)53513-4
7. Podfigurna-Stopa A, Czyzyk A, Grymowicz M, Smolarczyk R, Katulski K, Czajkowski K, et al. Premature ovarian insufficiency: the context of long-term effects. J Endocrinol Invest (2016) 39(9):983–90. doi: 10.1007/s40618-016-0467-z
8. Bachelot A, Nicolas C, Bidet M, Dulon J, Leban M, Golmard JL, et al. Long-term outcome of ovarian function in women with intermittent premature ovarian insufficiency. Clin Endocrinol (Oxf) (2017) 86(2):223–8. doi: 10.1111/cen.13105
9. van Kasteren YM, Schoemaker J. Premature ovarian failure: a systematic review on therapeutic interventions to restore ovarian function and achieve pregnancy. Hum Reprod Update (1999) 5(5):483–92. doi: 10.1093/humupd/5.5.483
10. Baerwald AR, Adams GP, Pierson RA. Ovarian antral folliculogenesis during the human menstrual cycle: a review. Hum Reprod Update (2012) 18(1):73–91. doi: 10.1093/humupd/dmr039
11. Krieger N, Lowy I, Aronowitz R, Bigby J, Dickersin K, Garner E, et al. Hormone replacement therapy, cancer, controversies, and women’s health: historical, epidemiological, biological, clinical, and advocacy perspectives. J Epidemiol Community Health (2005) 59(9):740–8. doi: 10.1136/jech.2005.033316
12. Collaborative Group on Hormonal Factors in Breast Cancer. Breast cancer and hormone replacement therapy: collaborative reanalysis of data from 51 epidemiological studies of 52 705 women with breast cancer and 108 411 women without breast cancer. Lancet (1997) 350(9084):1047–59. doi: 10.1016/S0140-6736(97)08233-0
13. Ewertz M, Mellemkjaer L, Poulsen AH, Friis S, Sorensen HT, Pedersen L, et al. Hormone use for menopausal symptoms and risk of breast cancer. A Danish cohort Study Br J Cancer (2005) 92(7):1293–7. doi: 10.1038/sj.bjc.6602472
14. Rossouw JE, Anderson GL, Prentice RL, LaCroix AZ, Kooperberg C, Stefanick ML, et al. Risks and benefits of estrogen plus progestin in healthy postmenopausal women: principal results From the Women’s Health Initiative randomized controlled trial. JAMA (2002) 288(3):321–33. doi: 10.1001/jama.288.3.321
15. Beral V. Million Women Study Collaborators. Breast cancer and hormone-replacement therapy in the Million Women Study. Lancet (2003) 362(9382):419–27. doi: 10.1016/S0140-6736(03)14065-2
16. Kyrou D, Kolibianakis EM, Venetis CA, Papanikolaou EG, Bontis J, Tarlatzis BC. How to improve the probability of pregnancy in poor responders undergoing in vitro fertilization: a systematic review and meta-analysis. Fertil Steril (2009) 91(3):749–66. doi: 10.1016/j.fertnstert.2007.12.077
17. Gupta S, Lodha P, Karthick MS, Tandulwadkar SR. Role of Autologous Bone Marrow-Derived Stem Cell Therapy for Follicular Recruitment in Premature Ovarian Insufficiency: Review of Literature and a Case Report of World’s First Baby with Ovarian Autologous Stem Cell Therapy in a Perimenopausal Woman of Age 45 Year. J Hum Reprod Sci (2018) 11(2):125–30. doi: 10.4103/jhrs.JHRS_57_18
18. Zhang H, Risal S, Gorre N, Busayavalasa K, Li X, Shen Y, et al. Somatic cells initiate primordial follicle activation and govern the development of dormant oocytes in mice. Curr Biol (2014) 24(21):2501–8. doi: 10.1016/j.cub.2014.09.023
19. Macklon NS, Fauser BC. Aspects of ovarian follicle development throughout life. Horm Res (1999) 52(4):161–70. doi: 10.1159/000023456
20. Nelson LM. Clinical practice. Primary ovarian insufficiency. N Engl J Med (2009) 360(6):606–14. doi: 10.1056/NEJMcp0808697
21. De Vos M, Devroey P, Fauser BC. Primary ovarian insufficiency. Lancet (2010) 376(9744):911–21. doi: 10.1016/S0140-6736(10)60355-8
22. Sheikhansari G, Aghebati-Maleki L, Nouri M, Jadidi-Niaragh F, Yousefi M. Current approaches for the treatment of premature ovarian failure with stem cell therapy. BioMed Pharmacother (2018) 102:254–62. doi: 10.1016/j.biopha.2018.03.056
23. Sonigo C, Beau I, Binart N, Grynberg M. The Impact of Chemotherapy on the Ovaries: Molecular Aspects and the Prevention of Ovarian Damage. Int J Mol Sci (2019) 20(21):10. doi: 10.3390/ijms20215342
24. Blumenfeld Z. Chemotherapy and fertility. Best Pract Res Clin Obstet Gynaecol (2012) 26(3):379–90. doi: 10.1016/j.bpobgyn.2011.11.008
25. Ebrahimi M, Akbari Asbagh F. Pathogenesis and causes of premature ovarian failure: an update. Int J Fertil Steril (2011) 5(2):54–65.
26. Gruber N, Shoenfeld Y. A link between human papilloma virus vaccination and primary ovarian insufficiency: current analysis. Curr Opin Obstet Gynecol (2015) 27(4):265–70. doi: 10.1097/GCO.0000000000000183
27. Kaufman DW, Slone D, Rosenberg L, Miettinen OS, Shapiro S. Cigarette smoking and age at natural menopause. Am J Public Health (1980) 70(4):420–2. doi: 10.2105/AJPH.70.4.420
28. Cunniff C, Jones KL, Benirschke K. Ovarian dysgenesis in individuals with chromosomal abnormalities. Hum Genet (1991) 86(6):552–6. doi: 10.1007/BF00201540
29. Holland CM. 47,XXX in an adolescent with premature ovarian failure and autoimmune disease. J Pediatr Adolesc Gynecol (2001) 14(2):77–80. doi: 10.1016/S1083-3188(01)00075-4
30. Modi DN, Sane S, Bhartiya D. Accelerated germ cell apoptosis in sex chromosome aneuploid fetal human gonads. Mol Hum Reprod (2003) 9(4):219–25. doi: 10.1093/molehr/gag031
31. Zinn AR. The X chromosome and the ovary. J Soc Gynecol Investig (2001) 8(1 Suppl Proceedings):S34–6. doi: 10.1177/1071557601008001S11
32. Huhtaniemi IT, Aittomaki K. Mutations of follicle-stimulating hormone and its receptor: effects on gonadal function. Eur J Endocrinol (1998) 138(5):473–81. doi: 10.1530/eje.0.1380473
33. Kirshenbaum M, Orvieto R. Premature ovarian insufficiency (POI) and autoimmunity-an update appraisal. J Assist Reprod Genet (2019) 36(11):2207–15. doi: 10.1007/s10815-019-01572-0
34. Ebrahimi M, Akbari Asbagh F. The role of autoimmunity in premature ovarian failure. Iran J Reprod Med (2015) 13(8):461–72.
35. Chernyshov VP, Radysh TV, Gura IV, Tatarchuk TP, Khominskaya ZB. Immune disorders in women with premature ovarian failure in initial period. Am J Reprod Immunol (2001) 46(3):220–5. doi: 10.1034/j.1600-0897.2001.d01-5.x
36. Wu R, Van der Hoek KH, Ryan NK, Norman RJ, Robker RL. Macrophage contributions to ovarian function. Hum Reprod Update (2004) 10(2):119–33. doi: 10.1093/humupd/dmh011
37. Yan G, Schoenfeld D, Penney C, Hurxthal K, Taylor AE, Faustman D. Identification of premature ovarian failure patients with underlying autoimmunity. J Womens Health Gend Based Med (2000) 9(3):275–87. doi: 10.1089/152460900318461
38. Kawashima I, Kawamura K. Regulation of follicle growth through hormonal factors and mechanical cues mediated by Hippo signaling pathway. Syst Biol Reprod Med (2018) 64(1):3–11. doi: 10.1080/19396368.2017.1411990
39. Fabregues F, Ferreri J, Calafell JM, Moreno V, Borras A, Manau D, et al. Pregnancy after drug-free in vitro activation of follicles and fresh tissue autotransplantation in primary ovarian insufficiency patient: a case report and literature review. J Ovarian Res (2018) 11(1):76–018-0447-3. doi: 10.1186/s13048-018-0447-3
40. Li J, Kawamura K, Cheng Y, Liu S, Klein C, Liu S, et al. Activation of dormant ovarian follicles to generate mature eggs. Proc Natl Acad Sci USA (2010) 107(22):10280–4. doi: 10.1073/pnas.1001198107
41. Suzuki N, Yoshioka N, Takae S, Sugishita Y, Tamura M, Hashimoto S, et al. Successful fertility preservation following ovarian tissue vitrification in patients with primary ovarian insufficiency. Hum Reprod (2015) 30(3):608–15. doi: 10.1093/humrep/deu353
42. Zhai J, Yao G, Dong F, Bu Z, Cheng Y, Sato Y, et al. In Vitro Activation of Follicles and Fresh Tissue Auto-transplantation in Primary Ovarian Insufficiency Patients. J Clin Endocrinol Metab (2016) 101(11):4405–12. doi: 10.1210/jc.2016-1589
43. Ferreri J, Fabregues F, Calafell JM, Solernou R, Borras A, Saco A, et al. Drug-free in-vitro activation of follicles and fresh tissue autotransplantation as a therapeutic option in patients with primary ovarian insufficiency. Reprod BioMed Online (2020) 40(2):254–60. doi: 10.1016/j.rbmo.2019.11.009
44. Lunding SA, Pors SE, Kristensen SG, Landersoe SK, Jeppesen JV, Flachs EM, et al. Biopsying, fragmentation and autotransplantation of fresh ovarian cortical tissue in infertile women with diminished ovarian reserve. Hum Reprod (2019) 34(10):1924–36. doi: 10.1093/humrep/dez152
45. Sfakianoudis K, Rapani A, Grigoriadis S, Retsina D, Maziotis E, Tsioulou P, et al. Novel Approaches in Addressing Ovarian Insufficiency in 2019: Are We There Yet? Cell Transplant (2020) 29:963689720926154. doi: 10.1177/0963689720926154
46. White YA, Woods DC, Takai Y, Ishihara O, Seki H, Tilly JL. Oocyte formation by mitotically active germ cells purified from ovaries of reproductive-age women. Nat Med (2012) 18(3):413–21. doi: 10.1038/nm.2669
47. Hubner K, Fuhrmann G, Christenson LK, Kehler J, Reinbold R, De La Fuente R, et al. Derivation of oocytes from mouse embryonic stem cells. Science (2003) 300(5623):1251–6. doi: 10.1126/science.1083452
48. Hayashi K, Ogushi S, Kurimoto K, Shimamoto S, Ohta H, Saitou M. Offspring from oocytes derived from in vitro primordial germ cell-like cells in mice. Science (2012) 338(6109):971–5. doi: 10.1126/science.1226889
49. Hayashi K, Saitou M. Generation of eggs from mouse embryonic stem cells and induced pluripotent stem cells. Nat Protoc (2013) 8(8):1513–24. doi: 10.1038/nprot.2013.090
50. Aflatoonian B, Ruban L, Jones M, Aflatoonian R, Fazeli A, Moore HD. In vitro post-meiotic germ cell development from human embryonic stem cells. Hum Reprod (2009) 24(12):3150–9. doi: 10.1093/humrep/dep334
51. Tesarik J, Nagy ZP, Sousa M, Mendoza C, Abdelmassih R. Fertilizable oocytes reconstructed from patient’s somatic cell nuclei and donor ooplasts. Reprod BioMed Online (2001) 2(3):160–4. doi: 10.1016/S1472-6483(10)61950-5
52. Hendriks S, Dancet EA, van Pelt AM, Hamer G, Repping S. Artificial gametes: a systematic review of biological progress towards clinical application. Hum Reprod Update (2015) 21(3):285–96. doi: 10.1093/humupd/dmv001
53. Liu T, Li Q, Wang S, Chen C, Zheng J. Transplantation of ovarian granulosalike cells derived from human induced pluripotent stem cells for the treatment of murine premature ovarian failure. Mol Med Rep (2016) 13(6):5053–8. doi: 10.3892/mmr.2016.5191
54. Butts S, Riethman H, Ratcliffe S, Shaunik A, Coutifaris C, Barnhart K. Correlation of telomere length and telomerase activity with occult ovarian insufficiency. J Clin Endocrinol Metab (2009) 94(12):4835–43. doi: 10.1210/jc.2008-2269
55. Hanna CW, Bretherick KL, Gair JL, Fluker MR, Stephenson MD, Robinson WP. Telomere length and reproductive aging. Hum Reprod (2009) 24(5):1206–11. doi: 10.1093/humrep/dep007
56. Xu X, Chen X, Zhang X, Liu Y, Wang Z, Wang P, et al. Impaired telomere length and telomerase activity in peripheral blood leukocytes and granulosa cells in patients with biochemical primary ovarian insufficiency. Hum Reprod (2017) 32(1):201–7. doi: 10.1093/humrep/dew283
57. Bernardes de Jesus B, Vera E, Schneeberger K, Tejera AM, Ayuso E, Bosch F, et al. Telomerase gene therapy in adult and old mice delays aging and increases longevity without increasing cancer. EMBO Mol Med (2012) 4(8):691–704. doi: 10.1002/emmm.201200245
58. Jaskelioff M, Muller FL, Paik JH, Thomas E, Jiang S, Adams AC, et al. Telomerase reactivation reverses tissue degeneration in aged telomerase-deficient mice. Nature (2011) 469(7328):102–6. doi: 10.1038/nature09603
59. Calado RT, Yewdell WT, Wilkerson KL, Regal JA, Kajigaya S, Stratakis CA, et al. Sex hormones, acting on the TERT gene, increase telomerase activity in human primary hematopoietic cells. Blood (2009) 114(11):2236–43. doi: 10.1182/blood-2008-09-178871
60. Townsley DM, Dumitriu B, Young NS. Danazol Treatment for Telomere Diseases. N Engl J Med (2016) 375(11):1095–6. doi: 10.1056/NEJMc1607752
61. Polonio AM, Chico-Sordo L, Cordova-Oriz I, Medrano M, Garcia-Velasco JA, Varela E. Impact of Ovarian Aging in Reproduction: From Telomeres and Mice Models to Ovarian Rejuvenation. Yale J Biol Med (2020) 93(4):561–9.
62. Briley SM, Jasti S, McCracken JM, Hornick JE, Fegley B, Pritchard MT, et al. Reproductive age-associated fibrosis in the stroma of the mammalian ovary. Reproduction (2016) 152(3):245–60. doi: 10.1530/REP-16-0129
63. Zhang Z, Schlamp F, Huang L, Clark H, Brayboy L. Inflammaging is associated with shifted macrophage ontogeny and polarization in the aging mouse ovary. Reproduction (2020) 159(3):325–37. doi: 10.1530/REP-19-0330
64. He L, Ling L, Wei T, Wang Y, Xiong Z. Ginsenoside Rg1 improves fertility and reduces ovarian pathological damages in premature ovarian failure model of mice. Exp Biol Med (Maywood) (2017) 242(7):683–91. doi: 10.1177/1535370217693323
65. Augello A, Kurth TB, De Bari C. Mesenchymal stem cells: a perspective from in vitro cultures to in vivo migration and niches. Eur Cell Mater (2010) 20:121–33. doi: 10.22203/eCM.v020a11
66. Liu T, Huang Y, Guo L, Cheng W, Zou G. CD44+/CD105+ human amniotic fluid mesenchymal stem cells survive and proliferate in the ovary long-term in a mouse model of chemotherapy-induced premature ovarian failure. Int J Med Sci (2012) 9(7):592–602. doi: 10.7150/ijms.4841
67. Xiao GY, Liu IH, Cheng CC, Chang CC, Lee YH, Cheng WT, et al. Amniotic fluid stem cells prevent follicle atresia and rescue fertility of mice with premature ovarian failure induced by chemotherapy. PloS One (2014) 9(9):e106538. doi: 10.1371/journal.pone.0106538
68. Wang F, Wang L, Yao X, Lai D, Guo L. Human amniotic epithelial cells can differentiate into granulosa cells and restore folliculogenesis in a mouse model of chemotherapy-induced premature ovarian failure. Stem Cell Res Ther (2013) 4(5):124. doi: 10.1186/scrt335
69. Lai D, Wang F, Chen Y, Wang L, Wang Y, Cheng W. Human amniotic fluid stem cells have a potential to recover ovarian function in mice with chemotherapy-induced sterility. BMC Dev Biol (2013) 13:34–213X-13-34. doi: 10.1186/1471-213X-13-34
70. Ding C, Li H, Wang Y, Wang F, Wu H, Chen R, et al. Different therapeutic effects of cells derived from human amniotic membrane on premature ovarian aging depend on distinct cellular biological characteristics. Stem Cell Res Ther (2017) 8(1):173–017-0613-3. doi: 10.1186/s13287-017-0613-3
71. Ling L, Feng X, Wei T, Wang Y, Wang Y, Zhang W, et al. Effects of low-intensity pulsed ultrasound (LIPUS)-pretreated human amnion-derived mesenchymal stem cell (hAD-MSC) transplantation on primary ovarian insufficiency in rats. Stem Cell Res Ther (2017) 8(1):283–017-0739-3. doi: 10.1186/s13287-017-0739-3
72. Wang S, Yu L, Sun M, Mu S, Wang C, Wang D, et al. The therapeutic potential of umbilical cord mesenchymal stem cells in mice premature ovarian failure. BioMed Res Int (2013) 2013:690491. doi: 10.1155/2013/690491
73. Song D, Zhong Y, Qian C, Zou Q, Ou J, Shi Y, et al. Human Umbilical Cord Mesenchymal Stem Cells Therapy in Cyclophosphamide-Induced Premature Ovarian Failure Rat Model. BioMed Res Int (2016) 2016:2517514. doi: 10.1155/2016/2517514
74. Elfayomy AK, Almasry SM, El-Tarhouny SA, Eldomiaty MA. Human umbilical cord blood-mesenchymal stem cells transplantation renovates the ovarian surface epithelium in a rat model of premature ovarian failure: Possible direct and indirect effects. Tissue Cell (2016) 48(4):370–82. doi: 10.1016/j.tice.2016.05.001
75. Li J, Mao Q, He J, She H, Zhang Z, Yin C. Human umbilical cord mesenchymal stem cells improve the reserve function of perimenopausal ovary via a paracrine mechanism. Stem Cell Res Ther (2017) 8(1):55–017-0514-5. doi: 10.1186/s13287-017-0514-5
76. Zhu SF, Hu HB, Xu HY, Fu XF, Peng DX, Su WY, et al. Human umbilical cord mesenchymal stem cell transplantation restores damaged ovaries. J Cell Mol Med (2015) 19(9):2108–17. doi: 10.1111/jcmm.12571
77. Pan Y, Zhang L, Zhang X, Hu C, Liu R. Biological and biomechanical analysis of two types of mesenchymal stem cells for intervention in chemotherapy-induced ovarian dysfunction. Arch Gynecol Obstet (2017) 295(1):247–52. doi: 10.1007/s00404-016-4224-5
78. Lai D, Wang F, Yao X, Zhang Q, Wu X, Xiang C. Human endometrial mesenchymal stem cells restore ovarian function through improving the renewal of germline stem cells in a mouse model of premature ovarian failure. J Transl Med (2015) 13:155–015-0516-y. doi: 10.1186/s12967-015-0516-y
79. Wang Z, Wang Y, Yang T, Li J, Yang X. Study of the reparative effects of menstrual-derived stem cells on premature ovarian failure in mice. Stem Cell Res Ther (2017) 8(1):11–016-0458-1. doi: 10.1186/s13287-016-0458-1
80. Feng P, Li P, Tan J. Human Menstrual Blood-Derived Stromal Cells Promote Recovery of Premature Ovarian Insufficiency Via Regulating the ECM-Dependent FAK/AKT Signaling. Stem Cell Rev Rep (2019) 15(2):241–55. doi: 10.1007/s12015-018-9867-0
81. Sun M, Wang S, Li Y, Yu L, Gu F, Wang C, et al. Adipose-derived stem cells improved mouse ovary function after chemotherapy-induced ovary failure. Stem Cell Res Ther (2013) 4(4):80. doi: 10.1186/scrt231
82. Su J, Ding L, Cheng J, Yang J, Li X, Yan G, et al. Transplantation of adipose-derived stem cells combined with collagen scaffolds restores ovarian function in a rat model of premature ovarian insufficiency. Hum Reprod (2016) 31(5):1075–86. doi: 10.1093/humrep/dew041
83. Fouad H, Sabry D, Elsetohy K, Fathy N. Therapeutic efficacy of amniotic membrane stem cells and adipose tissue stem cells in rats with chemically induced ovarian failure. J Adv Res (2016) 7(2):233–41. doi: 10.1016/j.jare.2015.05.002
84. Takehara Y, Yabuuchi A, Ezoe K, Kuroda T, Yamadera R, Sano C, et al. The restorative effects of adipose-derived mesenchymal stem cells on damaged ovarian function. Lab Invest (2013) 93(2):181–93. doi: 10.1038/labinvest.2012.167
85. Lund RJ, Narva E, Lahesmaa R. Genetic and epigenetic stability of human pluripotent stem cells. Nat Rev Genet (2012) 13(10):732–44. doi: 10.1038/nrg3271
86. Lee HJ, Selesniemi K, Niikura Y, Niikura T, Klein R, Dombkowski DM, et al. Bone marrow transplantation generates immature oocytes and rescues long-term fertility in a preclinical mouse model of chemotherapy-induced premature ovarian failure. J Clin Oncol (2007) 25(22):3198–204. doi: 10.1200/JCO.2006.10.3028
87. Fu X, He Y, Xie C, Liu W. Bone marrow mesenchymal stem cell transplantation improves ovarian function and structure in rats with chemotherapy-induced ovarian damage. Cytotherapy (2008) 10(4):353–63. doi: 10.1080/14653240802035926
88. Selesniemi K, Lee HJ, Niikura T, Tilly JL. Young adult donor bone marrow infusions into female mice postpone age-related reproductive failure and improve offspring survival. Aging (Albany NY) (2008) 1(1):49–57. doi: 10.18632/aging.100002
89. Liu J, Zhang H, Zhang Y, Li N, Wen Y, Cao F, et al. Homing and restorative effects of bone marrow-derived mesenchymal stem cells on cisplatin injured ovaries in rats. Mol Cells (2014) 37(12):865–72. doi: 10.14348/molcells.2014.0145
90. Ghadami M, El-Demerdash E, Zhang D, Salama SA, Binhazim AA, Archibong AE, et al. Bone marrow transplantation restores follicular maturation and steroid hormones production in a mouse model for primary ovarian failure. PloS One (2012) 7(3):e32462. doi: 10.1371/journal.pone.0032462
91. Abd-Allah SH, Shalaby SM, Pasha HF, El-Shal AS, Raafat N, Shabrawy SM, et al. Mechanistic action of mesenchymal stem cell injection in the treatment of chemically induced ovarian failure in rabbits. Cytotherapy (2013) 15(1):64–75. doi: 10.1016/j.jcyt.2012.08.001
92. Bao R, Xu P, Wang Y, Wang J, Xiao L, Li G, et al. Bone marrow derived mesenchymal stem cells transplantation rescues premature ovarian insufficiency induced by chemotherapy. Gynecol Endocrinol (2018) 34(4):320–6. doi: 10.1080/09513590.2017.1393661
93. El Andaloussi A, Igboeli P, Amer A, Al-Hendy A. Intravenous Infusion of Nucleated Peripheral Blood Cells Restores Fertility in Mice with Chemotherapy-Induced Premature Ovarian Failure. Biomedicines (2018) 6(3):10.3390/biomedicines6030093. doi: 10.3390/biomedicines6030093
94. Mohamed SA, Shalaby SM, Abdelaziz M, Brakta S, Hill WD, Ismail N, et al. Human mesenchymal stem cells partially reverse infertility in chemotherapy-induced ovarian failure. Reprod Sci (2018) 25(1):51–63. doi: 10.1177/1933719117699705
95. Herraiz S, Buigues A, Diaz-Garcia C, Romeu M, Martinez S, Gomez-Segui I, et al. Fertility rescue and ovarian follicle growth promotion by bone marrow stem cell infusion. Fertil Steril (2018) 109(5):908–918.e2. doi: 10.1016/j.fertnstert.2018.01.004
96. Cashen AF, Lazarus HM, Devine SM. Mobilizing stem cells from normal donors: is it possible to improve upon G-CSF? Bone Marrow Transplant (2007) 39(10):577–88. doi: 10.1038/sj.bmt.1705616
97. Hladik D, Hofig I, Oestreicher U, Beckers J, Matjanovski M, Bao X, et al. Long-term culture of mesenchymal stem cells impairs ATM-dependent recognition of DNA breaks and increases genetic instability. Stem Cell Res Ther (2019) 10(1):218–019-1334-6. doi: 10.1186/s13287-019-1334-6
98. Gabr H, Rateb MA, El Sissy MH, Ahmed Seddiek H, Ali Abdelhameed Gouda S. The effect of bone marrow-derived mesenchymal stem cells on chemotherapy induced ovarian failure in albino rats. Microsc Res Tech (2016) 79(10):938–47. doi: 10.1002/jemt.22725
99. Kilic S, Pinarli F, Ozogul C, Tasdemir N, Naz Sarac G, Delibasi T. Protection from cyclophosphamide-induced ovarian damage with bone marrow-derived mesenchymal stem cells during puberty. Gynecol Endocrinol (2014) 30(2):135–40. doi: 10.3109/09513590.2013.860127
100. Guo JQ, Gao X, Lin ZJ, Wu WZ, Huang LH, Dong HY, et al. BMSCs reduce rat granulosa cell apoptosis induced by cisplatin and perimenopause. BMC Cell Biol (2013) 14:18–2121-14-18. doi: 10.1186/1471-2121-14-18
101. Liu M, Qiu Y, Xue Z, Wu R, Li J, Niu X, et al. Small extracellular vesicles derived from embryonic stem cells restore ovarian function of premature ovarian failure through PI3K/AKT signaling pathway. Stem Cell Res Ther (2020) 11(1):3–019-1508-2. doi: 10.1186/s13287-019-1508-2
102. Herraiz S, Romeu M, Buigues A, Martinez S, Diaz-Garcia C, Gomez-Segui I, et al. Autologous stem cell ovarian transplantation to increase reproductive potential in patients who are poor responders. Fertil Steril (2018) 110(3):496–505.e1. doi: 10.1016/j.fertnstert.2018.04.025
103. Herraiz S, Pellicer N, Romeu M, Pellicer A. Treatment potential of bone marrow-derived stem cells in women with diminished ovarian reserves and premature ovarian failure. Curr Opin Obstet Gynecol (2019) 31(3):156–62. doi: 10.1097/GCO.0000000000000531
104. Edessy M, Hosni H, Shady Y, Waf Y, Bakr S, Samel M. Autologous stem cells therapy, The first baby of idiopathic premature ovarian failure. Acta Med Int (2016) 3(3):19–23. doi: 10.5530/ami.2016.1.7
105. Gabr H, Elkheir W, El-Gazzar A. Autologous stem cell transplantation in patients with idiopathic premature ovarian failure. J Tissue Sci Eng (2016) 7(3 Suppl):27.
106. Pellicer De Castellvi N, Herraiz S, Romeu M, Martinez S, Buigues S, Gomez-Seguí I. Bone marrow derived stem cells restore ovarian function and fertility in premature ovarian insufficiency women. Interim report of a randomized trial: mobilization versus ovarian injection. In: Human Reproduction. Oxford OX2 6DP, England: Oxford Univ Press Great Clarendon St.
107. Gnecchi M, Zhang Z, Ni A, Dzau VJ. Paracrine mechanisms in adult stem cell signaling and therapy. Circ Res (2008) 103(11):1204–19. doi: 10.1161/CIRCRESAHA.108.176826
108. He Y, Chen D, Yang L, Hou Q, Ma H, Xu X. The therapeutic potential of bone marrow mesenchymal stem cells in premature ovarian failure. Stem Cell Res Ther (2018) 9(1):263–018-1008-9. doi: 10.1186/s13287-018-1008-9
109. Kinnaird T, Stabile E, Burnett MS, Lee CW, Barr S, Fuchs S, et al. Marrow-derived stromal cells express genes encoding a broad spectrum of arteriogenic cytokines and promote in vitro and in vivo arteriogenesis through paracrine mechanisms. Circ Res (2004) 94(5):678–85. doi: 10.1161/01.RES.0000118601.37875.AC
110. Carrion B, Kong YP, Kaigler D, Putnam AJ. Bone marrow-derived mesenchymal stem cells enhance angiogenesis via their alpha6beta1 integrin receptor. Exp Cell Res (2013) 319(19):2964–76. doi: 10.1016/j.yexcr.2013.09.007
111. Liang T, Zhu L, Gao W, Gong M, Ren J, Yao H, et al. Coculture of endothelial progenitor cells and mesenchymal stem cells enhanced their proliferation and angiogenesis through PDGF and Notch signaling. FEBS Open Bio (2017) 7(11):1722–36. doi: 10.1002/2211-5463.12317
112. Zhang Y, Xia X, Yan J, Yan L, Lu C, Zhu X, et al. Mesenchymal stem cell-derived angiogenin promotes primodial follicle survival and angiogenesis in transplanted human ovarian tissue. Reprod Biol Endocrinol (2017) 15(1):1–12. doi: 10.1186/s12958-017-0235-8
113. Uzumcu M, Pan Z, Chu Y, Kuhn PE, Zachow R. Immunolocalization of the hepatocyte growth factor (HGF. system in the rat ovary and the anti-apoptotic effect of HGF in rat ovarian granulosa cells in vitro. Reproduction (2006) 132(2):291–9. doi: 10.1530/rep.1.00989
114. Zhou P, Baumgarten SC, Wu Y, Bennett J, Winston N, Hirshfeld-Cytron J, et al. IGF-I signaling is essential for FSH stimulation of AKT and steroidogenic genes in granulosa cells. Mol Endocrinol (2013) 27(3):511–23. doi: 10.1210/me.2012-1307
115. Zhao N, Liu Y, Liang H, Jiang X. Bone marrow-derived mesenchymal stem cells reduce immune reaction in a mouse model of allergic rhinitis. Am J Transl Res (2016) 8(12):5628–36.
116. Nemeth K, Leelahavanichkul A, Yuen PS, Mayer B, Parmelee A, Doi K, et al. Bone marrow stromal cells attenuate sepsis via prostaglandin E(2)-dependent reprogramming of host macrophages to increase their interleukin-10 production. Nat Med (2009) 15(1):42–9. doi: 10.1038/nm.1905
117. Yin N, Zhao W, Luo Q, Yuan W, Luan X, Zhang H. Restoring Ovarian Function With Human Placenta-Derived Mesenchymal Stem Cells in Autoimmune-Induced Premature Ovarian Failure Mice Mediated by Treg Cells and Associated Cytokines. Reprod Sci (2018) 25(7):1073–82. doi: 10.1177/1933719117732156
118. Abumaree MH, Al Jumah MA, Kalionis B, Jawdat D, Al Khaldi A, Abomaray FM, et al. Human placental mesenchymal stem cells (pMSCs) play a role as immune suppressive cells by shifting macrophage differentiation from inflammatory M1 to anti-inflammatory M2 macrophages. Stem Cell Rev Rep (2013) 9(5):620–41. doi: 10.1007/s12015-013-9455-2
119. Zhang Q, Xu M, Yao X, Li T, Wang Q, Lai D. Human amniotic epithelial cells inhibit granulosa cell apoptosis induced by chemotherapy and restore the fertility. Stem Cell Res Ther (2015) 6:152–015-0148-4. doi: 10.1186/s13287-015-0148-4
120. Seok J, Park H, Choi JH, Lim JY, Kim KG, Kim GJ. Placenta-Derived Mesenchymal Stem Cells Restore the Ovary Function in an Ovariectomized Rat Model via an Antioxidant Effect. Antioxid (Basel) (2020) 9(7). doi: 10.3390/antiox9070591
121. Afifi N, Reyad O. Role of mesenchymal stem cell therapy in restoring ovarian function in a rat model of chemotherapy-induced ovarian failure:a histological and immunohistochemical study. Egyptian J Histol (2013) 36:114–26. doi: 10.1097/01.EHX.0000423979.18253.10
122. Figueroa FE, Carrion F, Villanueva S, Khoury M. Mesenchymal stem cell treatment for autoimmune diseases: a critical review. Biol Res (2012) 45(3):269–77. doi: 10.4067/S0716-97602012000300008
123. Castellano JM, Kirby ED, Wyss-Coray T. Blood-Borne Revitalization of the Aged Brain. JAMA Neurol (2015) 72(10):1191–4. doi: 10.1001/jamaneurol.2015.1616
124. Villeda SA, Plambeck KE, Middeldorp J, Castellano JM, Mosher KI, Luo J, et al. Young blood reverses age-related impairments in cognitive function and synaptic plasticity in mice. Nat Med (2014) 20(6):659–63. doi: 10.1038/nm.3569
125. Castellano JM, Mosher KI, Abbey RJ, McBride AA, James ML, Berdnik D, et al. Human umbilical cord plasma proteins revitalize hippocampal function in aged mice. Nature (2017) 544(7651):488–92. doi: 10.1038/nature22067
126. Sills ES, Rickers NS, Li X, Palermo GD. First data on in vitro fertilization and blastocyst formation after intraovarian injection of calcium gluconate-activated autologous platelet rich plasma. Gynecol Endocrinol (2018) 34(9):756–60. doi: 10.1080/09513590.2018.1445219
127. Farimani M, Heshmati S, Poorolajal J, Bahmanzadeh M. A report on three live births in women with poor ovarian response following intra-ovarian injection of platelet-rich plasma (PRP). Mol Biol Rep (2019) 46(2):1611–6. doi: 10.1007/s11033-019-04609-w
128. Sfakianoudis K, Simopoulou M, Grigoriadis S, Pantou A, Tsioulou P, Maziotis E, et al. Reactivating Ovarian Function through Autologous Platelet-Rich Plasma Intraovarian Infusion: Pilot Data on Premature Ovarian Insufficiency, Perimenopausal, Menopausal, and Poor Responder Women. J Clin Med (2020) 9(6). doi: 10.3390/jcm9061809
129. Pantos K, Nitsos N, Kokkali G, Vaxevanoglou T, Markomichali C, Pantou A. Ovarian rejuvenation and folliculogenesis reactivation in peri-menopausal women after autologous platelet-rich plasma treatment. In: Abstracts, ESHRE 32nd Annual Meeting.
130. Pantos K, Simopoulou M, Pantou A, Rapani A, Tsioulou P, Nitsos N, et al. A case series on natural conceptions resulting in ongoing pregnancies in menopausal and prematurely menopausal women following platelet-rich plasma treatment. Cell Transplant (2019) 28(9-10):1333–40. doi: 10.1177/0963689719859539
131. Cakiroglu Y, Saltik A, Yuceturk A, Karaosmanoglu O, Kopuk SY, Scott RT, et al. Effects of intraovarian injection of autologous platelet rich plasma on ovarian reserve and IVF outcome parameters in women with primary ovarian insufficiency. Aging (Albany NY) (2020) 12(11):10211–22. doi: 10.18632/aging.103403
132. Boswell SG, Cole BJ, Sundman EA, Karas V, Fortier LA. Platelet-rich plasma: a milieu of bioactive factors. Arthroscopy (2012) 28(3):429–39. doi: 10.1016/j.arthro.2011.10.018
133. Sundman EA, Cole BJ, Karas V, Della Valle C, Tetreault MW, Mohammed HO, et al. The anti-inflammatory and matrix restorative mechanisms of platelet-rich plasma in osteoarthritis. Am J Sports Med (2014) 42(1):35–41. doi: 10.1177/0363546513507766
Keywords: follicular rescue, ovarian rejuvenation, premature ovarian insufficiency, stem cells, autologous stem cell ovarian transplant, mobilization
Citation: Polonio AM, García-Velasco JA and Herraiz S (2021) Stem Cell Paracrine Signaling for Treatment of Premature Ovarian Insufficiency. Front. Endocrinol. 11:626322. doi: 10.3389/fendo.2020.626322
Received: 05 November 2020; Accepted: 31 December 2020;
Published: 24 February 2021.
Edited by:
Annalisa Racca, University Hospital Brussels, BelgiumReviewed by:
Sezcan Mumusoglu, Hacettepe University, TurkeyNecati Findikli, Bahçeci Fulya IVF Center, Turkey
Copyright © 2021 Polonio, García-Velasco and Herraiz. This is an open-access article distributed under the terms of the Creative Commons Attribution License (CC BY). The use, distribution or reproduction in other forums is permitted, provided the original author(s) and the copyright owner(s) are credited and that the original publication in this journal is cited, in accordance with accepted academic practice. No use, distribution or reproduction is permitted which does not comply with these terms.
*Correspondence: Alba M. Polonio, YWxiYW1fcG9sb25pb0BpaXNsYWZlLmVz