- 1Section on Medical Neuroendocrinology, Eunice Kennedy Shriver National Institute of Child Health and Human Development, National Institutes of Health, Bethesda, MD, United States
- 2Scientific Department, Medica Scientia Innovation Research (MedSIR), Barcelona, Spain
- 3Section of Medical Oncology, Consorcio Hospitalario Provincial of Castellon, Castellon, Spain
- 4Department of Nuclear Medicine, La Timone University Hospital, CERIMED, Aix-Marseille University, Marseille, France
Neuroendocrine tumors overexpress somatostatin receptors, which serve as important and unique therapeutic targets for well-differentiated advanced disease. This overexpression is a well-established finding in gastroenteropancreatic neuroendocrine tumors which has guided new medical therapies in the administration of somatostatin analogs, both “cold”, particularly octreotide and lanreotide, and “hot” analogs, chelated to radiolabeled isotopes. The binding of these analogs to somatostatin receptors effectively suppresses excess hormone secretion and tumor cell proliferation, leading to stabilization, and in some cases, tumor shrinkage. Radioisotope-labeled somatostatin analogs are utilized for both tumor localization and peptide radionuclide therapy, with 68Ga-DOTATATE and 177Lu-DOTATATE respectively. Benign and malignant pheochromocytomas and paragangliomas also overexpress somatostatin receptors, irrespective of embryological origin. The pattern of somatostatin receptor overexpression is more prominent in succinate dehydrogenase subunit B gene mutation, which is more aggressive than other subgroups of this disease. While the Food and Drug Administration has approved the use of 68Ga-DOTATATE as a radiopharmaceutical for somatostatin receptor imaging, the use of its radiotherapeutic counterpart still needs approval beyond gastroenteropancreatic neuroendocrine tumors. Thus, patients with pheochromocytoma and paraganglioma, especially those with inoperable or metastatic diseases, depend on the clinical trials of somatostatin analogs. The review summarizes the advances in the utilization of somatostatin receptor for diagnostic and therapeutic approaches in the neuroendocrine tumor subset of pheochromocytoma and paraganglioma; we hope to provide a positive perspective in using these receptors as targets for treatment in this rare condition.
Introduction
The theranostic revolution began over three decades ago, following the medical conception of somatostatin receptors (SSTRs) and their analogs (SSA). The identification of specific tumor targets for diagnosis and therapy of advanced diseases has been a continuing trend in oncology since its innovation. Neuroendocrine tumors (NETs) with the overexpression of SSTRs are ideal cancer models for discovering the dual ability of diagnosis and treatment using SSAs.
Two decades after the identification of somatostatin (SST) as the central regulator of neuroendocrine cell physiology in the early seventies, five SSTR subtypes were discovered (1–4). The discovery of SSTRs led to the successful introduction of somatostatin analogs (SSAs), initially as antisecretory agents, and recently as antiproliferative agents based on the results of two large phase III trials (5–7).
Through recognition of the SST molecular pathway, we can extrapolate how SSAs exert these physiologic functions. SST inhibits the secretion of neuroendocrine hormones by activating seven-transmembrane somatostatin receptors, a type of G-protein coupled receptor (GPCR). Activation of GPCR initiates a cascade of inhibiting adenyl cyclase, lowering intracellular cAMP, decreasing protein kinase A (PKA) activity, and inhibiting/activating Ca2 and K+ channels, respectively. This sequence leads to a decrease in exocytosis of peptides, effectors, or ligands resulting in a reduction of hormone secretion (8–17). SST antiproliferative effect has been much more difficult to elucidate, involving various pathways that result in a global imbalance toward increased apoptosis, cell growth modulation, and decreased angiogenesis. Besides the reduction in growth factors (GF) release, SST exerts the effect through SSTR2 triggering and subsequent activation of phosphotyrosine phosphatases (PTPs). This causes a downregulation of the mitogen-activated protein kinase (MAPK) pathway and of tyrosine kinase receptor (TKR) phosphorylation, inducing cell cycle arrest and decreased cell proliferation (18–27).
Moreover, clinical imaging using radiolabeled SSAs to target SSTRs, known as somatostatin receptor imaging (SRI), became a prominent method in the diagnosis and management of NETs. The earliest success of SRI was pivotal in gastroenteropancreatic (GEP)-NETs and glomus paraganglioma (PGL) localization using 111In-pentetreotide (Octreoscan®) (28, 29). The progression of SRI in NETs increased with the introduction of radiolabeled isotope 68Ga-SSAs for positron emission tomography (PET) imaging. Then, Lutetium-177 (177Lu)-SSA was developed for peptide receptor radionuclide therapy (PRRT). A particular SST-based PRRT, 177Lu-DOTA0-Tyr3-Octreotate (177Lu-DOTATATE), was shown to be superior to other modalities in terms of progression-free survival (PFS) in a subset of GEPNETs (30). In 2018, based on the results of the NETTER-1 trial, 177Lu-DOTATATE (Lutathera®) was approved by the FDA for foregut, midgut, and hindgut GEPNET treatment. Current management algorithms for GEPNET patients use radiolabeled, and “cold” or unlabeled SSAs for their antiproliferative and cytotoxic abilities.
The discovery of SSTR overexpression in pheochromocytomas and paragangliomas (PPGLs) occurred in the 1990s (31), predicting a limitless therapeutic potential of SSA; however, its role in PPGL management was not developed in parallel with GEPNETs. Initial efficacy testing of SSAs, both cold and radiolabeled, was futile, mostly due to small clinical trials without any clear accrual of therapeutic benefits (32–34) in PPGLs. Despite the therapeutic responses of SSAs in GEPNETs showing significant success (35–39), the application of cold and radiolabeled SSA in PPGL was prematurely abandoned. In the last decade, there was a rise in the use of octreotide and radiolabeled SSA for recommended therapies approved by the FDA for both functioning and nonfunctioning GEPNETs, without enough studies confirming the clinical benefits of these compounds in PPGLs for federal approval. Figure 1 is a timeline comparing important findings and trials in SSTRs and 121 SSAs between NETs and PPGLs.
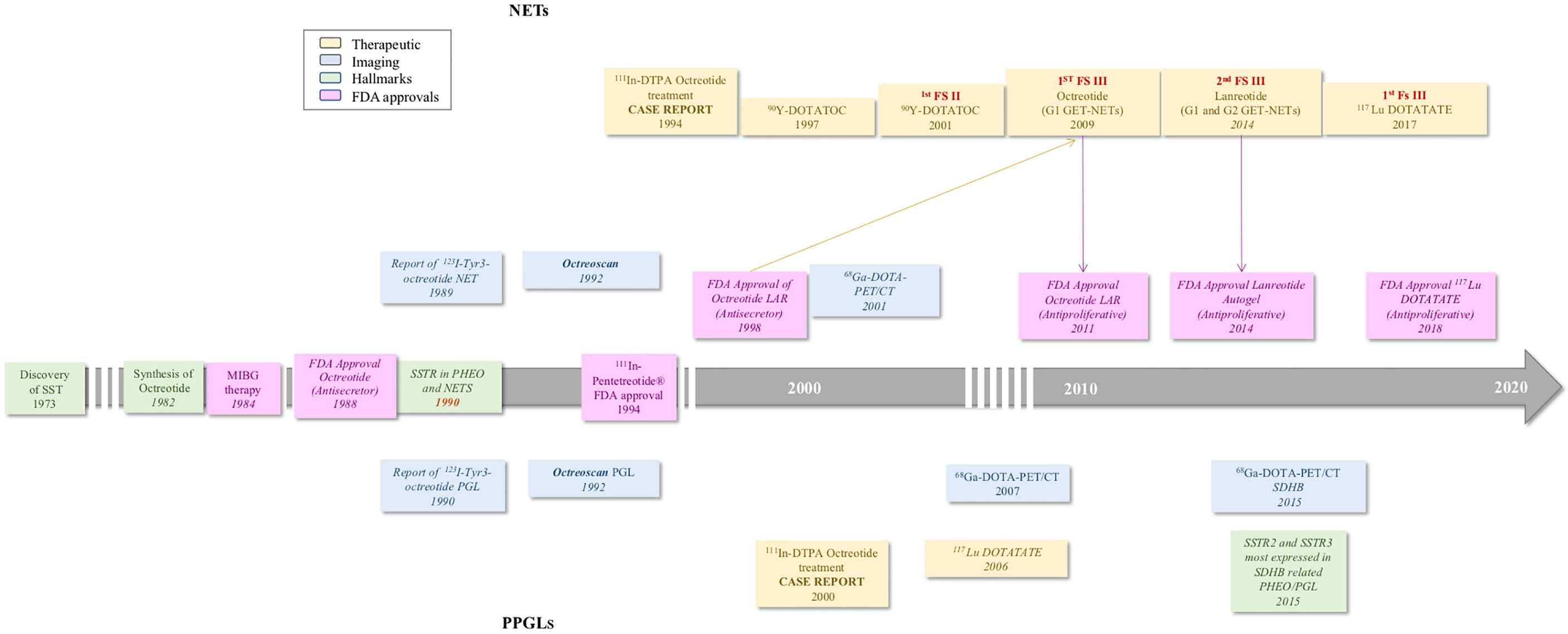
Figure 1 Timeline of important events in the development of somatostatin receptors and targeting analogs in neuroendocrine tumors and Pheochromocytoma and Paragangliomas (1–7, 30, 40–48).
The primary therapy of choice for PPGL is surgical resection, but not in the case of unresectable advanced and metastatic tumors. A significant proportion of patients with PPGL is due to an inheritable genetic component, where the incidence of metastatic PPGL (mPPGL) occurs due to succinate dehydrogenase subunit B (SDHB) germline mutation patterns (49). Interestingly, SDHB-related PPGLs overexpress SSTRs, mainly SSTR2 (48). To advance and expand the clinical utilization of SSAs in this PPGL, it is imperative to view the SDHB subgroup as a prime example of clinical benefits that these analogs could provide.
This review summarizes the studies on the role of SSTRs focusing on PPGLs. We detail the discovery of PPGL receptors and the creation of diagnostic and therapeutic radionuclide-bound moieties to target these receptors. We also explore future perspectives for SSTRs and SSAs in driving precision-based care of PPGL patients.
Pheochromocytoma and Paraganglioma
PPGLs are rare NETs arising from neural crest cells, specifically chromaffin cells. Differentiated based on anatomic locations, tumors from the adrenal medulla are defined as pheochromocytoma (PCC), whereas tumors from the sympathetic and parasympathetic ganglia are known as paraganglioma (PGL). While both these tumors present with similar molecular findings on pathology, they vary in manifested symptoms based on their biochemical profile (50).
More than 20 susceptibility genes (SDHA, SDHB, SDHC, SDHD, SDHAF2, FH, VHL, EPAS1, CSDE1, MAML3, RET, NF1, MAX, HRAS, TMEM127, HIF2A, PHD1/2) indicate predisposition to PPGLs (50). SDHB-related PPGLs are considered aggressive, causing more than 40% of all the metastatic cases (47). The risk of metastatic progression necessitates early diagnosis and intervention for obtaining good outcome in patients. It is important to identify symptoms and perform laboratory tests using plasma or urine metanephrines to confirm the diagnosis, followed by tumor localization through imaging.
Imaging allows personalized therapy by assisting clinicians in deciding whether surgical interventions can render the patient disease-free. PPGLs occur in a wide range of anatomical locations, from the base of the skull to the bladder, making computed tomography (CT) with intravenous contrast the initial choice of imaging modality. However, magnetic resonance imaging (MRI) with or without gadolinium is recommended if there are contraindications to CT imaging, for example contrast allergy, pregnancy, young age, and surgical clip artifacts (51).
Several predictors increase the risk of metastases: PPGL tumor > 5 cm, noradrenergic phenotype, dopaminergic phenotype, familial PPGLs (especially SDHB and SDHA), young age at initial diagnosis, multiple tumors, and recurrent disease (52, 53). PPGLs are more likely to metastasize to the lungs, liver, bones, and lymph nodes (54). While MRI has high sensitivity and specificity for PPGLs, functional imaging has shown to surpass it (55, 56). The advent of functional imaging utilizing SSTRs dramatically improved PPGL localization and identification, enabling clinicians to guide precision medicine.
Advent of SSTR-Based Imaging in Pheochromocytoma and Paraganglioma
Success in nuclear imaging of PPGLs was achieved in 1990, when Lamberts et al. conducted a study on three NETs, including one PGL, by labeling Tyr3-octreotide with radioisotope 123Iodine (123I-Tyr3-octreotide) to target SSTRs and capturing them using gamma cameras to produce single photon emission computed tomography (SPECT) and planar images. Results showed that 29 of the 31 possible PGLs were identified, and the two missed lesions were less than 5 mm in size (40). Although it was a relatively small study in terms of patient number, these findings on SRI-related PGLs could not be ignored. Subsequent studies improved the radiolabeled nucleotide by substituting 123Iodide with 111Indium in octreotide (111In-pentetreotide), chelated by a diethylene triamine penta-acetic acid (DTPA) group, thus solving the problems of short half-life half-life: 13 hours for 123Iodide versus 24-48 hours for 111Indium and obscured pathology identification due to biliary excretion with subsequent accumulation in the intestines (57). A study detected 94% of PGLs in 25 patients, and an additional 36% of tumors that were not recognized with conventional imaging [CT, ultrasound, 123I-metaiodobenzylguanidine (123I-MIBG), MRI, and bone scanning] were detected using 111In-pentetreotide. The study showed that using 111In-pentetreotide could identify the PGLs identified by conventional imaging and others that were not initially visualized (58). In PCCs, 123I-MIBG significantly outperformed 111In-pentetreotide in detection (57). 111In-pentetreotide had higher sensitivity than 123I-MIBG in detecting head and neck PGLs (HNPGLs) (59–61) and mPPGL (62, 63). The ability of 111In-pentetreotide to bind with SSTRs, especially SSTR2, provided an additional diagnostic tool for clinicians to identify PPGL; however, their sole gamma-emitting capability allows the application of only SPECT to visualize them. SPECT images do not provide spatial resolution to pinpoint the precise anatomical location of PPGL.
68Ga-Based-SSA: A Preferred Imaging Radioisotope in PPGL
PET, which captures emitted positrons from radiotracers and combines them with low dose CT (PET-CT) for targeted receptor localization, was developed in the late nineties (64). Not only does PET have better spatial resolution than SPECT, it can also quantify radiotracer uptake in the form of a standardized uptake value (SUV) (65). To utilize PET-CT hybridized imaging, radiotracers -emitting positrons and targeting SSTRs were created. The first discovered radiotracer was a somatostatin analog 1-Nal3-octreotide (NOC) combined with 68Gallium (68Ga)-labeled 1,4,7,10-tetraazacyclododecane-1,4,7,10-tetraacetic acid (DOTA), better known as 68Ga-DOTANOC. 68Ga-DOTANOC targets SSTR2,3, and 5 (66, 67) subtypes, while another moiety, 68Ga-labeled DOTA-Tyr3-octreotide (68Ga-DOTATOC), showed affinity for SSTR5, which is not specific to PPGLs. The last moiety of the three 68Ga-labeled DOTA peptides is 68Ga-DOTA-Tyr3-octreotate (68Ga-DOTATATE), which showed a strong tendency to bind with SSTR2 and is ideally suited for PPGLs because of the preferential expression of these SSTR subtypes (68). These three radiolabeled somatostatin analogs were compared with previous somatostatin-targeting 111In-pentetreotide. The overall sensitivities for NET detection, including metastatic lesions, were much higher with 68Ga-labeled DOTA-peptides by PET imaging than 111In-pentetreotide by SPECT imaging (69–75). While these studies were not specific to PPGL tumors, one study found 16 and 12 additional PGLs on 68Ga-DOTATATE compared to only two on 111In-pentetreotide (71). The other study included two patients with PGLs, comparing 68Ga-DOTATOC to 99mTechnetium-labeled hydrazinonicotinyl-Tyr3-octreotide (99mTc-HYNIC-TOC). While the study proved that 68Ga-labeled DOTA-peptide was superior, individual details of these PGL patients cannot be inferred from the analysis because it was performed on a regional basis, and on other NETs (75). There were no studies comparing the efficiency of 68Ga-DOTATATE to that of its predecessor, 111In-pentetreotide, but it was widely shown to be effective in tumors that express SSTRs. In an individual case of metastatic PGL with SDHD germline mutation, 68Ga-DOTATATE PET/CT produced higher resolution of tumors than Octreoscan®, as seen in Figure 2.
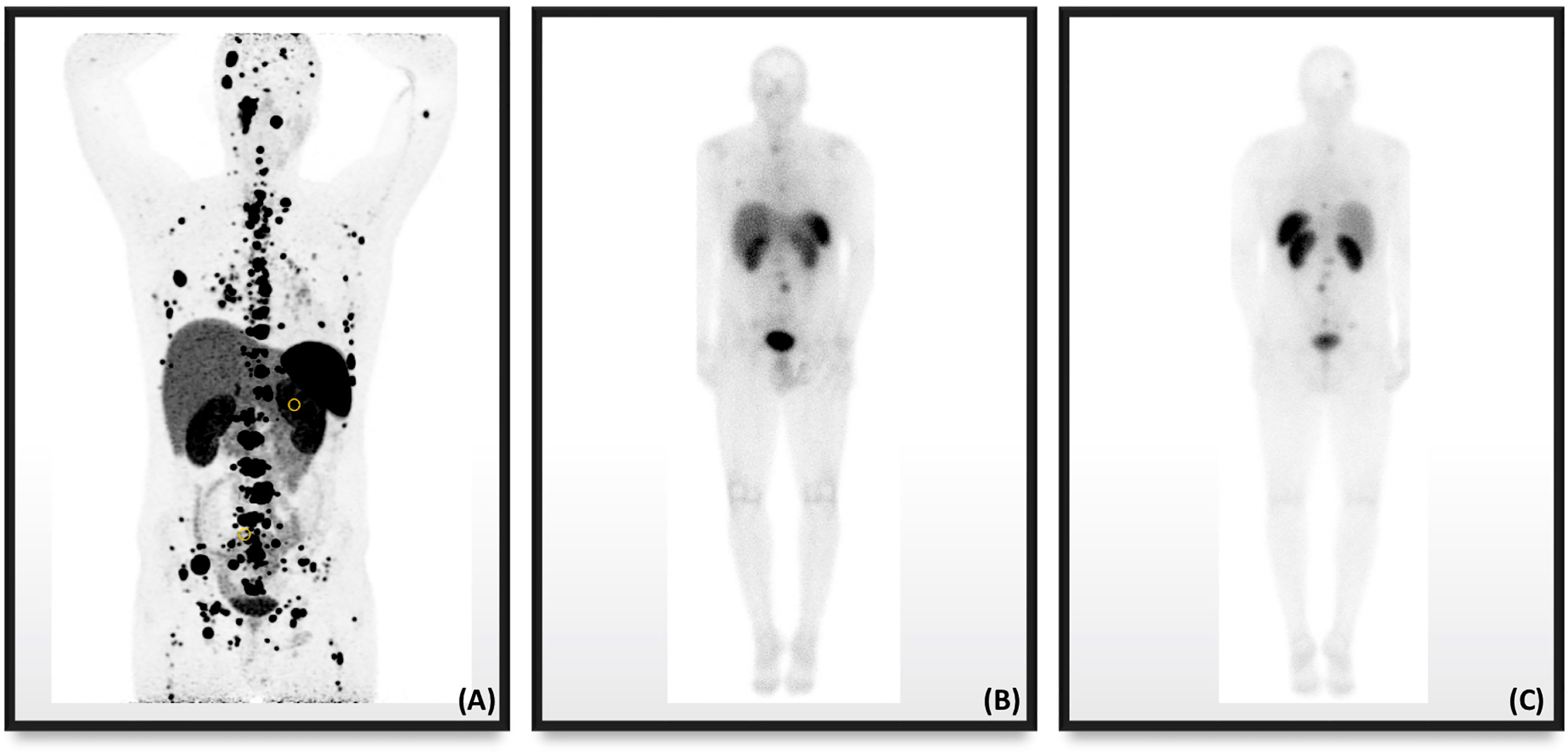
Figure 2 Nuclear imaging in a male patient with metastatic PPGL in the setting of SDHD showing the superiority in resolution of (A) 68Ga-DOTATATE PET/CT compared to Octreoscan® in the (B) anterior anatomical plane and (C) posterior anatomical plane.
Among the three radiolabeled somatostatin analogs, 68Ga-DOTATATE provided a brighter outlook for PPGL evaluation. SSTR expression by PPGLs, mainly extra-adrenal PGLs and mPPGLs, was found to be the subtype 2 variety (76). This subtype was the preferred target of 68Ga-DOTATATE (68). 68Ga-DOTATATE was shown to be superior to alternative PET radiotracers in imaging for genotypes, phenotypes, metastases, and PGL-predominant diseases. The two alternative PET radiotracers used to diagnose PPGLs, 18Flourine-fluorodeoxyglucose (18F-FDG) and 18F-fluorodihydroxyphenylalanine (18F-FDOPA), were inferior to 68Ga-DOTATATE in the following cohorts of patients with:
i. sporadic metastatic PPGL (77)
ii. PGLs (78)
iv. metastatic SDHB PPGL (47)
v. SDHA PPGL (80)
vi. SDHD PPGL (81)
vii. pediatric SDHx PPGL (82).
At a molecular level, the utility of 68Ga-based SRI in these patient cohorts can be explained by the current knowledge that SDHx-based lesions and extra-adrenal PGLs have higher proportions of SSTR2 than other PPGL types. Even though 68Ga-DOTATATE has lower sensitivity in other types of PPGLs than 18F-FDOPA, it remains the secondary radiopharmaceutical of choice in the evaluation of PPGL genotypic and phenotypic subtypes that do not fit in the cohorts mentioned above.
In two recent meta-analyses, 68Ga-DOTA-SSA had outperformed several radiotracers, including 18F-FDOPA and 18F-FDG. The pooled detection rate of unknown genetic mutational status in 68Ga-DOTA-SSA was 93% ([95% CI, 91%-95%], P < 0.005), higher than 80% in 18F-FDOPA ([95% CI, 69%–88%], P < 0.005) or 74% in 18F-FDG PET ([95% CI, 46%–91%], P < 0.005). The analyses showed that while genetic mutations can help select the type of radiotracers to be used in staging and diagnosing PPGL, it was not always required prior to the selection of 68Ga-DOTATATE, 68Ga-DOTATOC, and 68Ga-DOTANOC PET exams (83). A second meta-analysis pooled results of mPPGLs with germline mutational status, and the outcomes showed that 68Ga-DOTA-SSA PET/CT (0.97 [95% CI: 0.94-0.98]) detected more lesions than 18F-FDG PET/CT (0.79 [95% CI: 0.69–0.87]) (84).
68Ga-DOTATATE PET/CT proved to be more than a complementary imaging modality to traditional CT and MRI imaging modalities. 68Ga-DOTATATE PET/CT has taken the place of 111In-pentetreotide (Octreoscan®) in becoming the SRI modality of choice in PPGLs, subject to the availability of a PET/CT scanner and radiotracer. It also outperformed 18F-FDOPA and 18F-FDG for detection of PGLs, mPGLs, HNPGLs, and SDHx PPGLs in adults and children. Figure 3 illustrates the superiority of 68Ga-DOTATATE PET/CT compared to 18F-FDOPA and 18F-FDG of metastatic lesions in a PPGL patient with a SDHB mutation. While 68Ga-DOTATATE PET/CT effectively localizes PPGL tumors, the benefit was ultimately attributed in conversion of the 68Ga radiometal to a stronger beta-emitting one for therapeutic purposes.
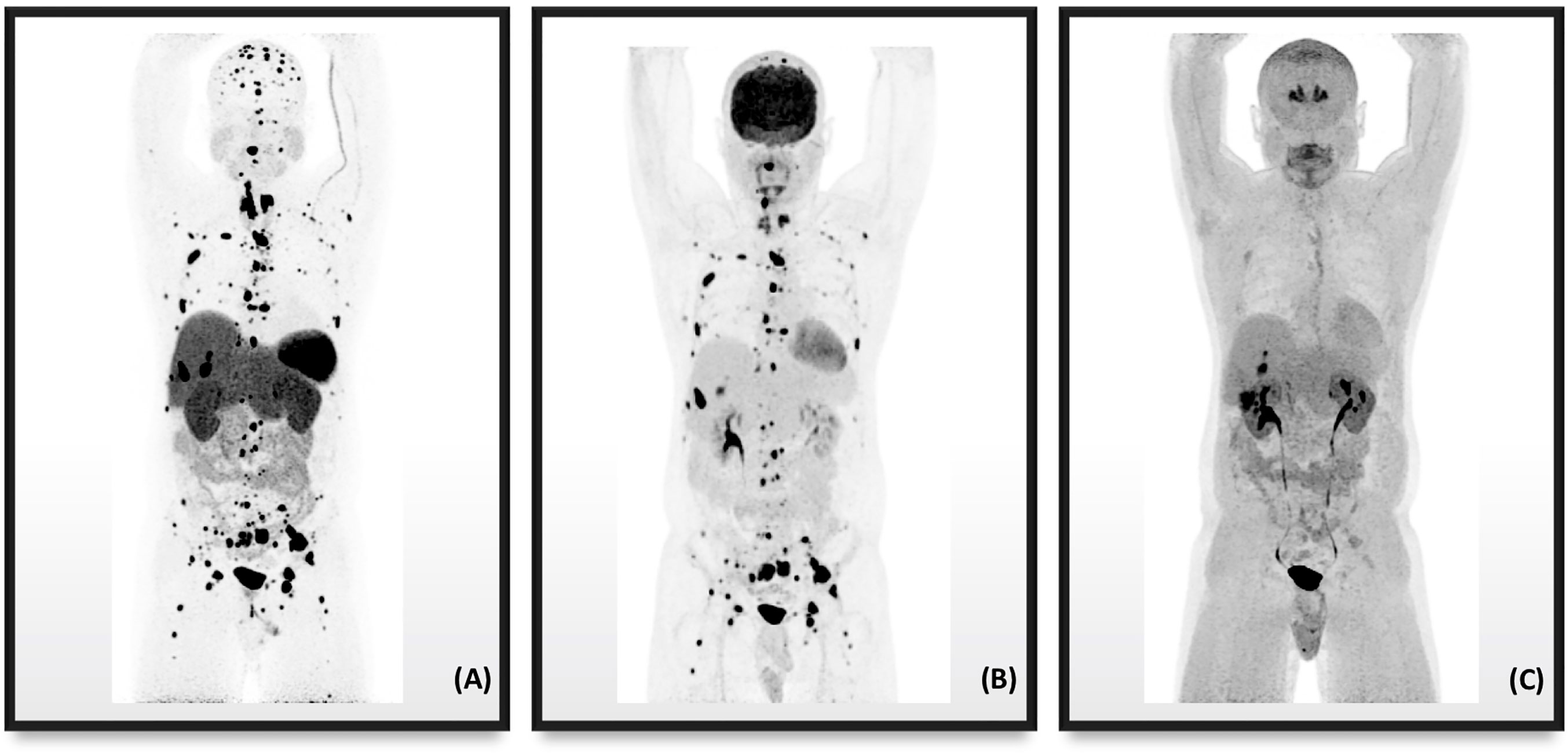
Figure 3 PET/CT radiotracer imaging of a 41-year-old male with metastatic PPGL in the setting of SDHB mutation. (A) 68Ga-DOTATATE displaying more metastatic disease than radiotracers (B) 18F-FDG and (C) 18F-FDOPA.
Experiences With Peptide Receptor Radionuclide Therapy Using Somatostatin Analogs in PPGLs
An interchange of radiolabeling on a chelated SSA (e.g., DOTA-SSA) caused a functional switch of the molecular compound from diagnostic to therapeutic capabilities. 68Ga-DOTA-SSA precisely located SSTRs on the surface of PPGL lesions through the capture of 68Ga-beta emissions by PET/CT scanners. A change in radiometal to 177Lutetium (177Lu) or 90Yttrium (90Y) gave radiolabeled DOTA-SSA the ability to emit not only imageable radiations but also deliver beta radiations to the target lesions. Lutathera® was approved by the FDA for GEPNET treatment; hopefully, it is only a matter of larger-model experiences and extensive reporting until its approval in surgically unamenable or metastatic PPGLs. More trials and research are needed to determine its actual applicability in PPGLs and to support the studies mentioned in this section.
177Lu-Based-SSA PRRT
A recent 2020 report by Basu et al. reviewed 1000 patients with NETs treated with 177Lu-DOTATATE; 15 were diagnosed with PPGL. A particular case of metastatic HNPGL was detailed in the review, displaying stabilization of the disease after two cycles of 177Lu-DOTATATE on 68Ga-DOTATATE PET/CT (85) and should be considered in metastatic HNPGL with associated SDHB mutations (86). The same team recently published a retrospective study highlighting disease control of progressive mPGL in 6 out of 9 patients treated with 177Lu-DOTATATE with negative 131I-MIBG scans. These patients tolerated treatment without any significant adverse events (87).
A retrospective study in 2019 by Vyakaranam et al. involved 22 PPGL patients (nine with progressive disease and 13 with stable disease at the start of PRRT) and their responses to 177Lu-DOTATATE. The response rates of the therapy, such as biochemical response, scintigraphy, response evaluation criteria in solid tumors (RECIST), overall survival (OS), and progression-free survival (PFS) showed favorable outcomes. 177Lu-DOTATATE showed that only one of the 19 patients reviewed with SPECT/CT had progressive disease, while with CT, according to RECIST 1.1, all patients either had stable disease (n=20) or partial response (n=2). The median OS calculated was 49.6 months and median PFS was 21.6 (88); these were not established in other recent studies (87, 89, 90).
Another retrospective study focused on 30 patients with either parasympathetic PGL, sympathetic PGL, or PCC; after four cycles of 177Lu-DOTATATE, results showed either stable disease or partial response in 90% of these patients. Among these patients, 20 had progressive disease prior to the start of 177Lu-DOTATATE, of which 85% showed the disease controlled post-treatment (91).
90Y-Based-SSA PRRT
The alternative beta-emitting radiometal, 90Y, was utilized and studied in SSA-based PRRT. 90Y had shorter half-life, longer path length, and greater emitted energy compared to 177Lu (92, 93). 90Y also cannot be imaged using gamma cameras post-therapy because of its inherent property of being a sole beta emitter (93). With longer half-life, shorter path length, lower beta emission, and partial gamma emission, 177Lu had a significant advantage over 90Y; however, studies showed the therapeutic benefit of 90Y-labeled SSA as PRRT.
In a prospective study from 2019 by Kolasinska-Cwikla et al., 13 patients with metastatic SDHB and SDHD (n=5 and 8, respectively) were treated with 90Y-DOTATATE, with an 82% response of stable disease after 1 year. The median OS and PFS were 68 months and 35 months, respectively, with no difference in the endpoints in patients who were either secretory or non-secretory (94). A retrospective study assessing 90Y-DOTATATE and 131I-MIBG concluded that mPGLs were best suited for treatment by SSA-based PRRT. The study reviewed the treatment responses of 22 patients with mPCC or mPGL after three different targeted radionuclide therapies. While only two patients received 177Lu-DOTATATE, 90Y-DOTATATE performed better in terms of median PFS and RECIST 1.1 base response to treatment compared to 131I-MIBG (these were the two statistically significant findings) in mPGL with no significant difference observed when considering all the mPPGL patients (95).
These studies showed some positive responses to either 177Lu- or 90Y-based SSA therapy (Table 1, summarizing experiences using SSA-based PRRT therapies in PPGL). There are still insufficient data for FDA approval of these therapies for PPGLs.
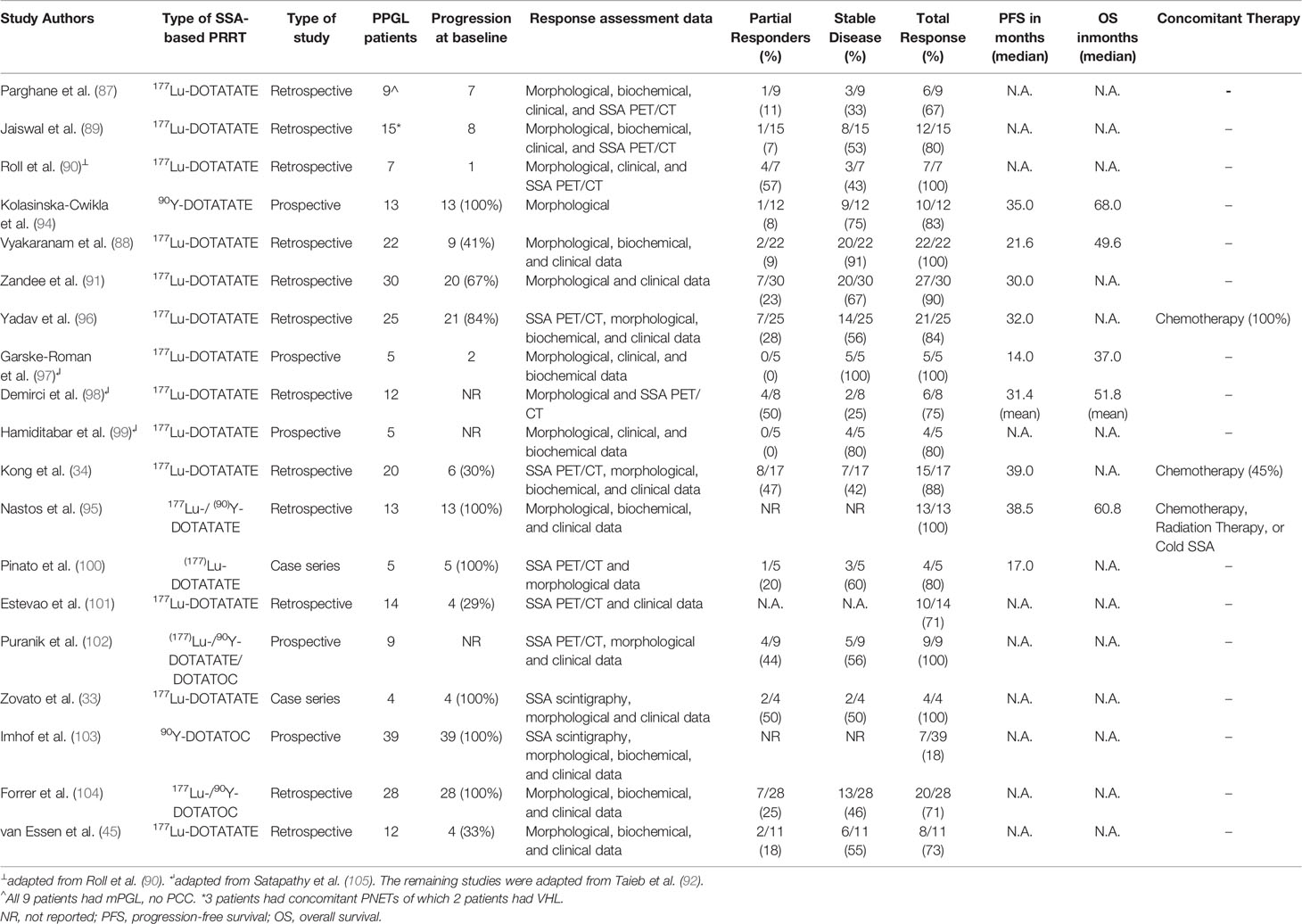
Table 1 Somatostatin-based PRRT experiences with pheochromocytoma and paraganglioma in the order of year of publication (2020 to 2006).
Clinical Side Effects of Somatostatin Analog Based Peptide Receptor Nucleotide Therapy
The clinical side effects of SSA-based PRRT include nausea, vomiting, fatigue, and abdominal pain (106). Nausea and vomiting have been attributed to commercial amino acid infusion for renal protection prior to infusion of the selected PRRT. The occurrence of nausea and vomiting can be reduced by substituting the commercial amino acid infusion with an alternative containing L-lysine and L-arginine. More serious side effects include neutropenia, lymphopenia, thrombocytopenia, and nephrotoxicity. In a review of 45 PPGL patients treated with PRRT, 3% had grade 3/4 neutropenia, 9% had thrombocytopenia, 11% had lymphopenia, and 4% had nephrotoxicity. A long-term complication of myelodysplastic syndrome was also observed in an unreported number of PPGL patients receiving the therapy (105). In a case report by Wolf et al., a dangerous side effect of Lutathera® in two mPGL patients was hyperprogression of mPGL disease after three cycles of 90Y/177Lu-DOTATOC (cycle one was 90Y, and cycle two and three were 177Lu) in patient A and two cycles of 177Lu-DOTATATE in patient B (107). Future reporting of adverse effects of SSA-based PRRT is important in assessing the safety of this therapy in PPGL patients to determine whether the therapy can be effectuated in patients, without life-threatening side effects.
Future Avenues of Somatostatin-Based Therapy in PPGLs
The following section will focus on ongoing studies that focus on the targeting of SSTRs by SSA based therapeutic compounds.
Ongoing PRRT Clinical Trials
An ongoing phase II study at the National Institutes of Health (NIH), NCT03206060, could make a strong case for federal approval. The study is using Lutathera® for treating progressive and inoperable PPGL patients with either germline SDHx mutation or sporadic disease. This prospective clinical trial will identify important clinical benefits of this treatment, focusing on the primary endpoint of PFS and several secondary endpoints, such as safety profile, OS, and quality of life. There are two other trials on Lutathera® currently recruiting children (NCT03923257 in Iowa, USA) and adults (NCT04029428 in Warsaw, Poland) with nonresectable or treatment-refractory SSTR-positive PPGLs. Similar prospective clinical studies should be conducted to uncover the therapeutic potential of SSTR-targeting radiotherapy.
Ongoing Lanreotide Clinical Trial
The long history of adoption and trial of SSA with good outcomes perpetuated an environment of ongoing clinical research and investigation. This culminated in large studies, such as the PROMID and CLARINET trials, which showed the clinical benefit of SSAs in GEPNETs (6, 7). However, the subset of NETs focused on in this review did not have extensive trials for testing the efficacy of cold SSA. There are reports of clinical stabilization of surgically unamenable PPGLs, two of which were patient experiences observed by our clinical team (80, 108), but there were no prospective or retrospective studies to either strengthen or refute these claims (80, 108–111). A prospective clinical trial (NCT03946527 in New York, USA) will evaluate the effectiveness of lanreotide in mPPGLs (LAMPARA) by observing tumor growth rate, overall survival, overall response rate, progression-free survival, and biochemical response.
Next Generation Cold SSAs
Overexpression of SSTRs on the cell surfaces of PPGLs has led to ongoing investigations that target and manipulate these receptors. The antiproliferative and apoptotic effects of somatostatin and its analogs upon binding with SSTRs were identified through extensive and detailed studies (112, 113). Targeting SSTR2 due to their preferential expression is the current and future direction of therapeutic management in these tumors (93, 114, 115). Cold SSAs, such as octreotide and lanreotide, have a proclivity to target SSTR2, which have been studied and utilized in various endocrine-related diseases, including GEPNETs and acromegaly (116). Pasireotide, a second-generation SSA, targets five SSTR subtypes, unlike octreotide and lanreotide. Although it was not superior to octreotide in terms of therapy or safety profile, it could be beneficial in tumors with broader expression of SSTR subtypes, including SSTR1, SSTR2, SSTR3, and SSTR5 (117, 118). Somatoprim, another second-generation SSA, is a multi-receptor targeting analog with a preference for SSTR2, SSTR4, and SSTR5, which was trialed in vitro on growth hormone (GH)-secreting pituitary adenomas. The results showed that it had anti-secretory effects on GH adenomas that were not controlled by octreotide (119). It would be worthwhile to investigate whether somatoprim has the same antisecretory effect in PPGLs. Dopastatin, a novel chimeric analog with dual binding ability to SSTR2 and dopamine receptors (D2), also exhibited an antisecretory effect on GH in acromegaly patients (120), and antitumor effects in midgut carcinoid cells in vitro (121). D2 receptors were expressed in larger amounts in 52 PPGL patients than 35 GEPNET patients (122), providing another targetable receptor for dopastatin analogs through radiopeptide imaging and therapy.
SSTR Antagonists
Development and research on SSA, which were recognized to antagonistically bind to SSTRs, are ongoing. According to an in vitro study by Ginj et al. (123), SSTR antagonists (SSTR-ANs) bound to NET SSTRs (especially SSTR2 and SSTR3) better than agonists but did not undergo subsequent internalization. These antagonists, sst3-ODN-8 and sst2-ANT were chelated to In by DOTA to create a receptor-targeting radioligand. These findings captured by gamma cameras were impressive in displaying antagonist-based radioligands, which bound more receptors for longer durations than their counterparts (123). The study caused a shift from the traditional theory that better binding and more benefits are derived from agonist-based analogs, mainly due to their ability to internalize the compound. A subsequent clinical comparison showed an antagonist-based SST ligand, 111In-DOTA-BASS, which allowed better visualization and had higher uptake in NETs than 111In-pentreotide (124). Based on the impressive results from first-generation SSTR-ANs, second-generation ones, such as LM3, JR10, and JR11, were developed. These second-generation SSTR-ANs were further improved in their SSTR binding capacity by using the chelator NODAGA (125). A comparative study showed that 68Ga-NODAGA-JR11 had higher tumoral uptake despite its lower affinity to SSTR2 than 68Ga-DOTATATE (126). The benefits were just as clear when 177Lu-DOTA-JR11 was used for treating four patients with 18 advanced NETs, with a ten-fold higher dose than 177Lu-DOTATATE and with reversible adverse events (127). A phase I/II study (NCT 02592707) focusing on the endpoints of safety, tolerability, efficacy, biodistribution, and dosimetry of 177Lu-OPS201 (also known as 177Lu-DOTA-JR11) in unresectable GEPNETs, lung carcinoids, and PPGLs is currently underway. This study could provide an additional research perspective to identify therapeutic options for PPGLs.
Alpha Emitting 255Ac-DOTATATE PRRT
Alpha-emitting radiometals are also being explored in the treatment of GEPNETs and PPGLs. A study explored the utility of 225Actinium (225Ac)-DOTATATE, a targeted alpha therapy (TAT), in 32 patients with metastatic GEPNETs refractory or stable after 177Lu-DOTATATE therapy. Four patients with paraganglioma received TAT but were excluded from the analysis. Of the 32 GEPNET patients, 24 were assessed by RECIST 1.1 and found to have either stable or partial response. A positive biochemical response in chromogranin A (CgA) was observed as well, showing stable or decreased levels in 32 patients. There were also minimal grade III/IV toxicities reported in patients, which included gastritis in 7, weight loss in 5, flushing in 3, and headaches in 2 (128). Another study used 225Ac-DOTATATE as compassionate care in two patients with progressive PCC after 3 cycles of 177Lu-DOTATATE; however, results on the effectiveness and toxicity were not published (89).
Cytotoxic Compounds Conjugated to SSA
Another frontier of therapeutic innovation in SSTR targeting was that of compounds linking SSA and cytotoxic agents. The SSA, Tyr3-octreotate, was conjugated with a microtubule-targeting agent, DM1, creating PEN-221. SSTR2 targeting of this agent was accomplished by the Tyr3-octreotate analog of the compound; after endocytosis, the DM1 portion induced a toxic payload within the targeted cells (129). A current phase I/II study (NCT 02936323) is ongoing for investigating the utility of PEN-221 in advanced NETs, including PPGLs.
Conclusion
The “Old Players” in the title of this review shows that SSAs have a historic role in treating and managing NETs. The review hopes to restore clinical awareness of these analogs through successes achieved in PPGLs. The theranostic utility of SSAs in PPGLs can be realized once federal approval is achieved. However, research and innovation should not be halted once an approval of Lutathera® for unresectable PPGLs is garnered. Research should be continued for targeting SSTRs with second-generation SSAs, SSTR-ANs, chimeric dual receptor-targeting peptides, chemotactic delivery through SSTRs, and other novel methods.
Author Contributions
MP and IT share first co-authorship; they contributed to the conception of the idea, creation of the outline, writing, reviewing, and editing. AJ contributed to creating an outline, conceptualization, reviewing, and editing. DT contributed to reviewing. KP contributed to creating an outline, conceptualization, review, and edit of the manuscript. All authors contributed to the article and approved the submitted version.
Funding
This research was funded by the Eunice Kennedy Shriver National Institute of Child Health and Human Development at the National Institutes of Health.
Conflict of Interest
The authors declare that the research was conducted in the absence of any commercial or financial relationships that could be construed as a potential conflict of interest.
The handling editor declared a past co-authorship with one of the authors KP.
References
1. Patel YC. Somatostatin and its receptor family. Front Neuroendocrinol (1999) 20(3):157–98. doi: 10.1006/frne.1999.0183
2. Krenning EP, Valkema R, Kooij PP, Breeman WA, Bakker WH, deHerder WW, et al. Scintigraphy and radionuclide therapy with [indium-111-labelled-diethyl triamine penta-acetic acid-D-Phe1]-octreotide. Ital J Gastroenterol Hepatol (1999) 31(Suppl 2):S219–223.
3. Vanetti M, Kouba M, Wang X, Vogt G, Hollt V. Cloning and expression of a novel mouse somatostatin receptor (SSTR2B). FEBS Lett (1992) 311(3):290–4. doi: 10.1016/0014-5793(92)81122-3
4. Krenning EP, Bakker WH, Breeman WA, Koper JW, Kooij PP, Ausema L, et al. Localisation of endocrine-related tumours with radioiodinated analogue of somatostatin. Lancet (1989) 1(8632):242–4. doi: 10.1016/S0140-6736(89)91258-0
5. Rinke A, Muller HH, Schade-Brittinger C, Klose KJ, Barth P, Wied M, et al. Placebo-controlled, double-blind, prospective, randomized study on the effect of octreotide LAR in the control of tumor growth in patients with metastatic neuroendocrine midgut tumors: a report from the PROMID Study Group. J Clin Oncol (2009) 27(28):4656–63. doi: 10.1200/JCO.2009.22.8510
6. Caplin ME, Pavel M, Cwikla JB, Phan AT, Raderer M, Sedlackova E, et al. Lanreotide in metastatic enteropancreatic neuroendocrine tumors. N Engl J Med (2014) 371(3):224–33. doi: 10.1056/NEJMoa1316158
7. Rinke A, Wittenberg M, Schade-Brittinger C, Aminossadati B, Ronicke E, Gress TM, et al. Placebo-Controlled, Double-Blind, Prospective, Randomized Study on the Effect of Octreotide LAR in the Control of Tumor Growth in Patients with Metastatic Neuroendocrine Midgut Tumors (PROMID): Results of Long-Term Survival. Neuroendocrinology (2017) 104(1):26–32. doi: 10.1159/000443612
8. Kagimoto S, Yamada Y, Kubota A, Someya Y, Ihara Y, Yasuda K, et al. Human somatostatin receptor, SSTR2, is coupled to adenylyl cyclase in the presence of Gi alpha 1 protein. Biochem Biophys Res Commun (1994) 202(2):1188–95. doi: 10.1006/bbrc.1994.2054
9. Kubota A, Yamada Y, Kagimoto S, Seino S, Seino Y. Effector coupling of somatostatin receptor subtypes on human endocrine tumors. Metabolism (1996) 45(8 Suppl 1):42–5. doi: 10.1016/S0026-0495(96)90078-5
10. Law SF, Yasuda K, Bell GI, Reisine T. Gi alpha 3 and G(o) alpha selectively associate with the cloned somatostatin receptor subtype SSTR2. J Biol Chem (1993) 268(15):10721–7. doi: 10.1016/S0021-9258(18)82045-2
11. Tentler JJ, Hadcock JR, Gutierrez-Hartmann A. Somatostatin acts by inhibiting the cyclic 3’,5’-adenosine monophosphate (cAMP)/protein kinase A pathway, cAMP response element-binding protein (CREB) phosphorylation, and CREB transcription potency. Mol Endocrinol (1997) 11(7):859–66. doi: 10.1210/mend.11.7.9943
12. Tomura H, Okajima F, Akbar M, Majid MA, Sho KM, Kondo Y. Transfected Human Somatostatin Receptor Type 2, SSTR2, Not Only Inhibits Adenylate Cyclase but Also Stimulates Phospholipase C and Ca2+ Mobilization. Biochem Biophys Res Commun (1994) 200(2):986–92. doi: 10.1006/bbrc.1994.1547
13. Yang SK, Parkington HC, Blake AD, Keating DJ, Chen C. Somatostatin increases voltage-gated K+ currents in GH3 cells through activation of multiple somatostatin receptors. Endocrinology (2005) 146(11):4975–84. doi: 10.1210/en.2005-0696
14. Van Op den Bosch J, Adriaensen D, Van Nassauw L, Timmermans JP. The role(s) of somatostatin, structurally related peptides and somatostatin receptors in the gastrointestinal tract: a review. Regul Pept (2009) 156(1-3):1–8. doi: 10.1016/j.regpep.2009.04.003
15. Koch BD, Schonbrunn A. Characterization of the cyclic AMP-independent actions of somatostatin in GH cells. II. An increase in potassium conductance initiates somatostatin-induced inhibition of prolactin secretion. J Biol Chem (1988) 263(1):226–34. doi: 10.1016/S0021-9258(19)57382-3
16. Pace CS, Tarvin JT. Somatostatin: mechanism of action in pancreatic islet beta-cells. Diabetes (1981) 30(10):836–42. doi: 10.2337/diabetes.30.10.836
17. Yamashita N, Shibuya N, Ogata E. Hyperpolarization of the membrane potential caused by somatostatin in dissociated human pituitary adenoma cells that secrete growth hormone. Proc Natl Acad Sci U S A (1986) 83(16):6198–202. doi: 10.1073/pnas.83.16.6198
18. Sellers LA, Alderton F, Carruthers AM, Schindler M, Humphrey PP. Receptor isoforms mediate opposing proliferative effects through gbetagamma-activated p38 or Akt pathways. Mol Cell Biol (2000) 20(16):5974–85. doi: 10.1128/MCB.20.16.5974-5985.2000
19. Reubi JC, Laissue JA. Multiple actions of somatostatin in neoplastic disease. Trends Pharmacol Sci (1995) 16(3):110–5. doi: 10.1016/S0165-6147(00)88992-0
20. Buscail L, Esteve JP, Saint-Laurent N, Bertrand V, Reisine T, O’Carroll AM, et al. Inhibition of cell proliferation by the somatostatin analogue RC-160 is mediated by somatostatin receptor subtypes SSTR2 and SSTR5 through different mechanisms. Proc Natl Acad Sci U S A (1995) 92(5):1580–4. doi: 10.1073/pnas.92.5.1580
21. Cattaneo MG, Amoroso D, Gussoni G, Sanguini AM, Vicentini LM. A somatostatin analogue inhibits MAP kinase activation and cell proliferation in human neuroblastoma and in human small cell lung carcinoma cell lines. FEBS Lett (1996) 397(2-3):164–8. doi: 10.1016/S0014-5793(96)01159-3
22. Pola S, Cattaneo MG, Vicentini LM. Anti-migratory and anti-invasive effect of somatostatin in human neuroblastoma cells: involvement of Rac and MAP kinase activity. J Biol Chem (2003) 278(42):40601–6. doi: 10.1074/jbc.M306510200
23. Douziech N, Calvo E, Coulombe Z, Muradia G, Bastien J, Aubin RA, et al. Inhibitory and stimulatory effects of somatostatin on two human pancreatic cancer cell lines: a primary role for tyrosine phosphatase SHP-1. Endocrinology (1999) 140(2):765–77. doi: 10.1210/endo.140.2.6492
24. Barbieri F, Pattarozzi A, Gatti M, Porcile C, Bajetto A, Ferrari A, et al. Somatostatin receptors 1, 2, and 5 cooperate in the somatostatin inhibition of C6 glioma cell proliferation in vitro via a phosphotyrosine phosphatase-eta-dependent inhibition of extracellularly regulated kinase-1/2. Endocrinology (2008) 149(9):4736–46. doi: 10.1210/en.2007-1762
25. Bousquet C, Delesque N, Lopez F, Saint-Laurent N, Esteve JP, Bedecs K, et al. sst2 somatostatin receptor mediates negative regulation of insulin receptor signaling through the tyrosine phosphatase SHP-1. J Biol Chem (1998) 273(12):7099–106. doi: 10.1074/jbc.273.12.7099
26. Shimon I, Taylor JE, Dong JZ, Bitonte RA, Kim S, Morgan B, et al. Somatostatin receptor subtype specificity in human fetal pituitary cultures. Differential role of SSTR2 and SSTR5 for growth hormone, thyroid-stimulating hormone, and prolactin regulation. J Clin Invest (1997) 99(4):789–98. doi: 10.1172/JCI119225
27. Murray RD, Kim K, Ren SG, Chelly M, Umehara Y, Melmed S. Central and peripheral actions of somatostatin on the growth hormone-IGF-I axis. J Clin Invest (2004) 114(3):349–56. doi: 10.1172/JCI19933
28. Krenning EP, Kwekkeboom DJ, Reubi JC, Van Hagen PM, van Eijck CH, Oei HY, et al. 111In-octreotide scintigraphy in oncology. Metabolism. (1992) 41(9 Suppl 2):83–6. doi: 10.1016/0026-0495(92)90038-C
29. Westlin JE, Janson ET, Arnberg H, Ahlstrom H, Oberg K, Nilsson S. Somatostatin receptor scintigraphy of carcinoid tumours using the [111In-DTPA-D-Phe1]-octreotide. Acta Oncol (Stockholm Sweden) (1993) 32(7-8):783–6. doi: 10.3109/02841869309096136
30. Strosberg J, El-Haddad G, Wolin E, Hendifar A, Yao J, Chasen B, et al. Phase 3 Trial of 177Lu-Dotatate for Midgut Neuroendocrine Tumors. N Engl J Med (2017) 376(2):125–35. doi: 10.1056/NEJMoa1607427
31. Reubi JC, Waser B, Khosla S, Kvols L, Goellner JR, Krenning E, et al. In vitro and in vivo detection of somatostatin receptors in pheochromocytomas and paragangliomas. J Clin Endocrinol Metab (1992) 74(5):1082–9. doi: 10.1210/jc.74.5.1082
32. Baulieu J, Resche I, Bardies M, Chauvet AF, Lecloirec J, Malhaire J, et al. [131I]-TYR3-octreotide: clinical dosimetry and use for internal radiotherapy of metastatic paraganglioma and carcinoid tumors. Nucl Med Biol (2000) 27(8):809–13. doi: 10.1016/S0969-8051(00)00150-5
33. Zovato S, Kumanova A, Dematte S, Sansovini M, Bodei L, Di Sarra D, et al. Peptide receptor radionuclide therapy (PRRT) with 177Lu-DOTATATE in individuals with neck or mediastinal paraganglioma (PGL). Horm Metab Res (2012) 44(5):411–4. doi: 10.1055/s-0032-1311637
34. Kong G, Grozinsky-Glasberg S, Hofman MS, Callahan J, Meirovitz A, Maimon O, et al. Efficacy of Peptide Receptor Radionuclide Therapy (PRRT) for Functional Metastatic Paraganglioma and Phaeochromocytoma. J Clin Endocrinol Metab (2017) 102(9):3278–3287. doi: 10.1210/jc.2017-00816
35. Faiss S, Rath U, Mansmann U, Caird D, Clemens N, Riecken EO, et al. Ultra-high-dose lanreotide treatment in patients with metastatic neuroendocrine gastroenteropancreatic tumors. Digestion (1999) 60(5):469–76. doi: 10.1159/000007693
36. Khan S, Krenning EP, van Essen M, Kam BL, Teunissen JJ, Kwekkeboom DJ. Quality of life in 265 patients with gastroenteropancreatic or bronchial neuroendocrine tumors treated with [177Lu-DOTA0,Tyr3]octreotate. J Nucl Med (2011) 52(9):1361–8. doi: 10.2967/jnumed.111.087932
37. Kvols LK, Moertel CG, O’Connell MJ, Schutt AJ, Rubin J, Hahn RG. Treatment of the malignant carcinoid syndrome. Evaluation of a long-acting somatostatin analogue. N Engl J Med (1986) 315(11):663–6. doi: 10.1056/NEJM198609113151102
38. Ruszniewski P, Ducreux M, Chayvialle JA, Blumberg J, Cloarec D, Michel H, et al. Treatment of the carcinoid syndrome with the longacting somatostatin analogue lanreotide: a prospective study in 39 patients. Gut (1996) 39(2):279–83. doi: 10.1136/gut.39.2.279
39. Wymenga AN, Eriksson B, Salmela PI, Jacobsen MB, Van Cutsem EJ, Fiasse RH, et al. Efficacy and safety of prolonged-release lanreotide in patients with gastrointestinal neuroendocrine tumors and hormone-related symptoms. J Clin Oncol (1999) 17(4):1111. doi: 10.1200/JCO.1999.17.4.1111
40. Lamberts SW, Bakker WH, Reubi JC, Krenning EP. Somatostatin-receptor imaging in the localization of endocrine tumors. New Engl J Med (1990) 323(18):1246–9. doi: 10.1056/NEJM199011013231805
41. Lamberts SW, Reubi JC, Krenning EP. Somatostatin receptor imaging in the diagnosis and treatment of neuroendocrine tumors. J Steroid Biochem Mol Biol (1992) 43(1–3):185–8.
42. Krenning EP, Kooij PP, Bakker WH, Breeman WA, Postema PT, Kwekkeboom DJ, et al. Radiotherapy with a radiolabeled somatostatin analogue, [111In-DTPA-D-Phe1]-octreotide. A case history. Ann N Y Acad Sci (1994) 733:496–506.
43. Otte A, Mueller-Brand J, Dellas S, Nitzsche EU, Herrmann R, Maecke HR. Yttrium-90-abelled somatostatin-analogue for cancer treatment. Lancet (1998) 351(9100):417–8.
44. Waldherr C, Pless M, Maecke HR, Haldemann A, Mueller-Brand J. The clinical value of [90Y-DOTA]-D-Phe1-Tyr3-octreotide (90Y-DOTATOC) in the treatment of neuroendocrine tumours: a clinical phase II study. Ann Oncol (2001) 12(7):941–5.
45. van Essen M, Krenning EP, Kooij PP, Bakker WH, Feelders RA, de Herder WW, et al. Effects of therapy with [177Lu-DOTA0, Tyr3]octreotate in patients with paraganglioma, meningioma, small cell lung carcinoma, and melanoma. J Nucl Med (2006) 47(10):1599–606.
46. Win Z, Al-Nahhas A, Towey D, Todd JF, Rubello D, Lewington V, et al. 68Ga-DOTATATE PET in neuroectodermal tumours: first experience. Nucl Med Commun (2007) 28(5):359–63.
47. Janssen I, Blanchet EM, Adams K, Chen CC, Millo CM, Herscovitch P, et al. Superiority of [68Ga]-DOTATATE PET/CT to Other Functional Imaging Modalities in the Localization of SDHB-Associated Metastatic Pheochromocytoma and Paraganglioma. Clin Cancer Res (2015) 21(17):3888–95. doi: 10.1158/1078-0432.CCR-14-2751
48. Elston MS, Meyer-Rochow GY, Conaglen HM, Clarkson A, Clifton-Bligh RJ, Conaglen JV, et al. Increased SSTR2A and SSTR3 expression in succinate dehydrogenase-deficient pheochromocytomas and paragangliomas. Hum Pathol (2015) 46(3):390–6. doi: 10.1016/j.humpath.2014.11.012
49. Turkova H, Prodanov T, Maly M, Martucci V, Adams K, Widimsky J Jr, et al. Characteristics and Outcomes of Metastatic Sdhb and Sporadic Pheochromocytoma/Paraganglioma: An National Institutes of Health Study. Endocrine Pract Off J Am Coll Endocrinol Am Assoc Clin Endocrinologists (2016) 22(3):302–14. doi: 10.4158/EP15725.OR
50. Crona J, Taieb D, Pacak K. New Perspectives on Pheochromocytoma and Paraganglioma: Toward a Molecular Classification. Endocr Rev (2017) 38(6):489–515. doi: 10.1210/er.2017-00062
51. Lenders JW, Duh QY, Eisenhofer G, Gimenez-Roqueplo AP, Grebe SK, Murad MH, et al. Pheochromocytoma and paraganglioma: an endocrine society clinical practice guideline. J Clin Endocrinol Metab (2014) 99(6):1915–42. doi: 10.1210/jc.2014-1498
52. Havekes B, King K, Lai EW, Romijn JA, Corssmit EP, Pacak K. New imaging approaches to phaeochromocytomas and paragangliomas. Clin Endocrinol (Oxf) (2010) 72(2):137–45. doi: 10.1111/j.1365-2265.2009.03648.x
53. Nolting S, Ullrich M, Pietzsch J, Ziegler CG, Eisenhofer G, Grossman A, et al. Current Management of Pheochromocytoma/Paraganglioma: A Guide for the Practicing Clinician in the Era of Precision Medicine. Cancers (Basel) (2019) 11(10). doi: 10.3390/cancers11101505
55. Lumachi F, Tregnaghi A, Zucchetta P, Cristina Marzola M, Cecchin D, Grassetto G, et al. Sensitivity and positive predictive value of CT, MRI and 123I-MIBG scintigraphy in localizing pheochromocytomas: a prospective study. Nucl Med Commun (2006) 27(7):583–7. doi: 10.1097/00006231-200607000-00006
56. Timmers HJ, Chen CC, Carrasquillo JA, Whatley M, Ling A, Eisenhofer G, et al. Staging and functional characterization of pheochromocytoma and paraganglioma by 18F-fluorodeoxyglucose (18F-FDG) positron emission tomography. J Natl Cancer Inst (2012) 104(9):700–8. doi: 10.1093/jnci/djs188
57. Krenning EP, Kwekkeboom DJ, Bakker WH, Breeman WA, Kooij PP, Oei HY, et al. Somatostatin receptor scintigraphy with [111In-DTPA-D-Phe1]- and [123I-Tyr3]-octreotide: the Rotterdam experience with more than 1000 patients. Eur J Nucl Med (1993) 20(8):716–31. doi: 10.1007/BF00181765
58. Kwekkeboom DJ, van Urk H, Pauw BK, Lamberts SW, Kooij PP, Hoogma RP, et al. Octreotide scintigraphy for the detection of paragangliomas. J Nucl Med (1993) 34(6):873–8.
59. Muros MA, Llamas-Elvira JM, Rodriguez A, Ramirez A, Gomez M, Arraez MA, et al. 111In-pentetreotide scintigraphy is superior to 123I-MIBG scintigraphy in the diagnosis and location of chemodectoma. Nucl Med Commun (1998) 19(8):735–42. doi: 10.1097/00006231-199808000-00003
60. Telischi FF, Bustillo A, Whiteman ML, Serafini AN, Reisberg MJ, Gomez-Marin O, et al. Octreotide scintigraphy for the detection of paragangliomas. Otolaryngol Head Neck Surg (2000) 122(3):358–62. doi: 10.1067/mhn.2000.102575
61. Schmidt M, Fischer E, Dietlein M, Michel O, Weber K, Moka D, et al. Clinical value of somatostatin receptor imaging in patients with suspected head and neck paragangliomas. Eur J Nucl Med Mol Imaging (2002) 29(12):1571–80. doi: 10.1007/s00259-002-0939-6
62. van der Harst E, de Herder WW, Bruining HA, Bonjer HJ, de Krijger RR, Lamberts SW, et al. [(123)I]metaiodobenzylguanidine and [(111)In]octreotide uptake in begnign and malignant pheochromocytomas. J Clin Endocrinol Metab (2001) 86(2):685–93. doi: 10.1210/jc.86.2.685
63. Ilias I, Chen CC, Carrasquillo JA, Whatley M, Ling A, Lazurova I, et al. Comparison of 6-18F-fluorodopamine PET with 123I-metaiodobenzylguanidine and 111in-pentetreotide scintigraphy in localization of nonmetastatic and metastatic pheochromocytoma. J Nucl Med (2008) 49(10):1613–9. doi: 10.2967/jnumed.108.052373
64. Townsend DW. Combined positron emission tomography-computed tomography: the historical perspective. Semin Ultrasound CT MR (2008) 29(4):232–5. doi: 10.1053/j.sult.2008.05.006
65. Johnbeck CB, Knigge U, Kjaer A. PET tracers for somatostatin receptor imaging of neuroendocrine tumors: current status and review of the literature. Future Oncol (2014) 10(14):2259–77. doi: 10.2217/fon.14.139
66. Wild D, Schmitt JS, Ginj M, Macke HR, Bernard BF, Krenning E, et al. DOTA-NOC, a high-affinity ligand of somatostatin receptor subtypes 2, 3 and 5 for labelling with various radiometals. Eur J Nucl Med Mol Imaging (2003) 30(10):1338–47. doi: 10.1007/s00259-003-1255-5
67. Wild D, Macke HR, Waser B, Reubi JC, Ginj M, Rasch H, et al. 68Ga-DOTANOC: a first compound for PET imaging with high affinity for somatostatin receptor subtypes 2 and 5. Eur J Nucl Med Mol Imaging (2005) 32(6):724. doi: 10.1007/s00259-004-1697-4
68. Reubi JC, Schar JC, Waser B, Wenger S, Heppeler A, Schmitt JS, et al. Affinity profiles for human somatostatin receptor subtypes SST1-SST5 of somatostatin radiotracers selected for scintigraphic and radiotherapeutic use. Eur J Nucl Med (2000) 27(3):273–82. doi: 10.1007/s002590050034
69. Sadowski SM, Neychev V, Millo C, Shih J, Nilubol N, Herscovitch P, et al. Prospective Study of 68Ga-DOTATATE Positron Emission Tomography/Computed Tomography for Detecting Gastro-Entero-Pancreatic Neuroendocrine Tumors and Unknown Primary Sites. J Clin Oncol (2016) 34(6):588–96. doi: 10.1200/JCO.2015.64.0987
70. Deppen SA, Liu E, Blume JD, Clanton J, Shi C, Jones-Jackson LB, et al. Safety and Efficacy of 68Ga-DOTATATE PET/CT for Diagnosis, Staging, and Treatment Management of Neuroendocrine Tumors. J Nucl Med (2016) 57(5):708–14. doi: 10.2967/jnumed.115.163865
71. Srirajaskanthan R, Kayani I, Quigley AM, Soh J, Caplin ME, Bomanji J. The role of 68Ga-DOTATATE PET in patients with neuroendocrine tumors and negative or equivocal findings on 111In-DTPA-octreotide scintigraphy. J Nucl Med (2010) 51(6):875–82. doi: 10.2967/jnumed.109.066134
72. Morgat C, Velayoudom-Cephise FL, Schwartz P, Guyot M, Gaye D, Vimont D, et al. Evaluation of (68)Ga-DOTA-TOC PET/CT for the detection of duodenopancreatic neuroendocrine tumors in patients with MEN1. Eur J Nucl Med Mol Imaging (2016) 43(7):1258–66. doi: 10.1007/s00259-016-3319-3
73. Van Binnebeek S, Vanbilloen B, Baete K, Terwinghe C, Koole M, Mottaghy FM, et al. Comparison of diagnostic accuracy of (111)In-pentetreotide SPECT and (68)Ga-DOTATOC PET/CT: A lesion-by-lesion analysis in patients with metastatic neuroendocrine tumours. Eur Radiol (2016) 26(3):900–9. doi: 10.1007/s00330-015-3882-1
74. Buchmann I, Henze M, Engelbrecht S, Eisenhut M, Runz A, Schafer M, et al. Comparison of 68Ga-DOTATOC PET and 111In-DTPAOC (Octreoscan) SPECT in patients with neuroendocrine tumours. Eur J Nucl Med Mol Imaging (2007) 34(10):1617–26. doi: 10.1007/s00259-007-0450-1
75. Gabriel M, Decristoforo C, Kendler D, Dobrozemsky G, Heute D, Uprimny C, et al. 68Ga-DOTA-Tyr3-octreotide PET in neuroendocrine tumors: comparison with somatostatin receptor scintigraphy and CT. J Nucl Med (2007) 48(4):508–18. doi: 10.2967/jnumed.106.035667
76. Leijon H, Remes S, Hagstrom J, Louhimo J, Maenpaa H, Schalin-Jantti C, et al. Variable somatostatin receptor subtype expression in 151 primary pheochromocytomas and paragangliomas. Hum Pathol (2019) 86:66–75. doi: 10.1016/j.humpath.2018.11.020
77. Janssen I, Chen CC, Millo CM, Ling A, Taieb D, Lin FI, et al. PET/CT comparing (68)Ga-DOTATATE and other radiopharmaceuticals and in comparison with CT/MRI for the localization of sporadic metastatic pheochromocytoma and paraganglioma. Eur J Nucl Med Mol Imaging (2016) 43(10):1784–91. doi: 10.1007/s00259-016-3357-x
78. Archier A, Varoquaux A, Garrigue P, Montava M, Guerin C, Gabriel S, et al. Prospective comparison of (68)Ga-DOTATATE and (18)F-FDOPA PET/CT in patients with various pheochromocytomas and paragangliomas with emphasis on sporadic cases. Eur J Nucl Med Mol Imaging (2016) 43(7):1248–57. doi: 10.1007/s00259-015-3268-2
79. Janssen I, Chen CC, Taieb D, Patronas NJ, Millo CM, Adams KT, et al. 68Ga-DOTATATE PET/CT in the Localization of Head and Neck Paragangliomas Compared with Other Functional Imaging Modalities and CT/MRI. J Nucl Med (2016) 57(2):186–91. doi: 10.2967/jnumed.115.161018
80. Jha A, de Luna K, Balili CA, Millo C, Paraiso CA, Ling A, et al. Clinical, Diagnostic, and Treatment Characteristics of SDHA-Related Metastatic Pheochromocytoma and Paraganglioma. Front Oncol (2019) 9:53. doi: 10.3389/fonc.2019.00053
81. Jha A, Ling A, Millo C, Chen C, Gupta G, Viana B, et al. Superiority of 68Ga-DOTATATE PET/CT to other functional and anatomic imaging modalities in the detection ofSDHD-related pheochromocytoma and paraganglioma-A comparative prospective study. J Nucl Med (2018) 59(supplement 1):46–6.
82. Jha A, Ling A, Millo C, Gupta G, Viana B, Lin FI, et al. Superiority of (68)Ga-DOTATATE over (18)F-FDG and anatomic imaging in the detection of succinate dehydrogenase mutation (SDHx )-related pheochromocytoma and paraganglioma in the pediatric population. Eur J Nucl Med Mol Imaging (2018) 45(5):787–97. doi: 10.1007/s00259-017-3896-9
83. Han S, Suh CH, Woo S, Kim YJ, Lee JJ. Performance of (68)Ga-DOTA-Conjugated Somatostatin Receptor-Targeting Peptide PET in Detection of Pheochromocytoma and Paraganglioma: A Systematic Review and Metaanalysis. J Nucl Med (2019) 60(3):369–76. doi: 10.2967/jnumed.118.211706
84. Kan Y, Zhang S, Wang W, Liu J, Yang J, Wang Z. (68)Ga-somatostatin receptor analogs and (18)F-FDG PET/CT in the localization of metastatic pheochromocytomas and paragangliomas with germline mutations: a meta-analysis. Acta Radiol (2018) 59(12):1466–74. doi: 10.1177/0284185118764206
85. Basu S, Chakraborty S, Parghane RV, Kamaldeep, Ranade R, Thapa P, et al. One decade of ‘Bench-to-Bedside’ peptide receptor radionuclide therapy with indigenous [(177)Lu]Lu-DOTATATE obtained through ‘Direct’ neutron activation route: lessons learnt including practice evolution in an Indian setting. Am J Nucl Med Mol Imaging (2020) 10(4):178–211.
86. Jha A, Nilubol N, Pacak K. Some Considerations in Treating Malignant Head and Neck Paragangliomas. JAMA Otolaryngol Head Neck Surg (2019) 146(2):209–210. doi: 10.1001/jamaoto.2019.3444
87. Parghane RV, Talole S, Basu S. (131)I-MIBG negative progressive symptomatic metastatic paraganglioma: response and outcome with (177)Lu-DOTATATE peptide receptor radionuclide therapy. Ann Nucl Med (2020) 35(1):92–101. doi: 10.1007/s12149-020-01541-z
88. Vyakaranam AR, Crona J, Norlen O, Granberg D, Garske-Roman U, Sandstrom M, et al. Favorable Outcome in Patients with Pheochromocytoma and Paraganglioma Treated with (177)Lu-DOTATATE. Cancers (Basel) (2019) 11(7). doi: 10.3390/cancers11070909
89. Jaiswal SK, Sarathi V, Memon SS, Garg R, Malhotra G, Verma P, et al. 177Lu-DOTATATE therapy in metastatic/inoperable pheochromocytoma-paraganglioma. Endocr Connect (2020) 9(9):864–73. doi: 10.1530/EC-20-0292
90. Roll W, Muther M, Sporns PB, Zinnhardt B, Suero Molina E, Seifert R, et al. Somatostatin Receptor-Targeted Radioligand Therapy in Head and Neck Paraganglioma. World Neurosurg (2020) 143:e391–9. doi: 10.1016/j.wneu.2020.07.165
91. Zandee WT, Feelders RA, Smit Duijzentkunst DA, Hofland J, Metselaar RM, Oldenburg RA, et al. Treatment of inoperable or metastatic paragangliomas and pheochromocytomas with peptide receptor radionuclide therapy using 177Lu-DOTATATE. Eur J Endocrinol (2019) 181(1):45–53. doi: 10.1530/EJE-18-0901
92. Taieb D, Jha A, Treglia G, Pacak K. Molecular imaging and radionuclide therapy of pheochromocytoma and paraganglioma in the era of genomic characterization of disease subgroups. Endocrine-related cancer (2019) 26(11):R627–52. doi: 10.1530/ERC-19-0165
93. Van Essen M, Krenning EP, De Jong M, Valkema R, Kwekkeboom DJ. Peptide Receptor Radionuclide Therapy with radiolabelled somatostatin analogues in patients with somatostatin receptor positive tumours. Acta Oncol (Stockholm Sweden) (2007) 46(6):723–34. doi: 10.1080/02841860701441848
94. Kolasinska-Cwikla A, Peczkowska M, Cwikla JB, Michalowska I, Palucki JM, Bodei L, et al. A Clinical Efficacy of PRRT in Patients with Advanced, Nonresectable, Paraganglioma-Pheochromocytoma, Related to SDHx Gene Mutation. J Clin Med (2019) 8(7). doi: 10.3390/jcm8070952
95. Nastos K, Cheung VTF, Toumpanakis C, Navalkissoor S, Quigley AM, Caplin M, et al. Peptide Receptor Radionuclide Treatment and (131)I-MIBG in the management of patients with metastatic/progressive phaeochromocytomas and paragangliomas. J Surg Oncol (2017) 115(4):425–34. doi: 10.1002/jso.24553
96. Yadav MP, Ballal S, Bal C. Concomitant (177)Lu-DOTATATE and capecitabine therapy in malignant paragangliomas. EJNMMI Res (2019) 9(1):13. doi: 10.1186/s13550-019-0484-y
97. Garske-Roman U, Sandstrom M, Fross Baron K, Lundin L, Hellman P, Welin S, et al. Prospective observational study of (177)Lu-DOTA-octreotate therapy in 200 patients with advanced metastasized neuroendocrine tumours (NETs): feasibility and impact of a dosimetry-guided study protocol on outcome and toxicity. Eur J Nucl Med Mol Imaging (2018) 45(6):970–88. doi: 10.1007/s00259-018-3945-z
98. Demirci E, Kabasakal L, Toklu T, Ocak M, Sahin OE, Alan-Selcuk N, et al. 177Lu-DOTATATE therapy in patients with neuroendocrine tumours including high-grade (WHO G3) neuroendocrine tumours: response to treatment and long-term survival update. Nucl Med Commun (2018) 39(8):789–96. doi: 10.1097/MNM.0000000000000874
99. Hamiditabar M, Ali M, Roys J, Wolin EM, O’Dorisio TM, Ranganathan D, et al. Peptide Receptor Radionuclide Therapy With 177Lu-Octreotate in Patients With Somatostatin Receptor Expressing Neuroendocrine Tumors: Six Years’ Assessment. Clin Nucl Med (2017) 42(6):436–43. doi: 10.1097/RLU.0000000000001629
100. Pinato DJ, Black JR, Ramaswami R, Tan TM, Adjogatse D, Sharma R. Peptide receptor radionuclide therapy for metastatic paragangliomas. Med Oncol (2016) 33(5):47. doi: 10.1007/s12032-016-0737-9
101. Estevao R, Duarte H, Lopes F, Fernandes J, Monteiro E. Peptide receptor radionuclide therapy in head and neck paragangliomas - Report of 14 cases. Rev Laryngol Otol Rhinol (Bord) (2015) 136(4):155–8.
102. Puranik AD, Kulkarni HR, Singh A, Baum RP. Peptide receptor radionuclide therapy with (90)Y/ (177)Lu-labelled peptides for inoperable head and neck paragangliomas (glomus tumours). Eur J Nucl Med Mol Imaging (2015) 42(8):1223–30. doi: 10.1007/s00259-015-3029-2
103. Imhof A, Brunner P, Marincek N, Briel M, Schindler C, Rasch H, et al. Response, survival, and long-term toxicity after therapy with the radiolabeled somatostatin analogue [90Y-DOTA]-TOC in metastasized neuroendocrine cancers. J Clin Oncol (2011) 29(17):2416–23. doi: 10.1200/JCO.2010.33.7873
104. Forrer F, Riedweg I, Maecke HR, Mueller-Brand J. Radiolabeled DOTATOC in patients with advanced paraganglioma and pheochromocytoma. Q J Nucl Med Mol Imaging (2008) 52(4):334–40.
105. Satapathy S, Mittal BR, Bhansali A. ‘Peptide receptor radionuclide therapy in the management of advanced pheochromocytoma and paraganglioma: A systematic review and meta-analysis’. Clin Endocrinol (Oxf) (2019) 91(6):718–27. doi: 10.1111/cen.14106
106. Taieb D, Pacak K. Molecular imaging and theranostic approaches in pheochromocytoma and paraganglioma. Cell Tissue Res (2018) 372(2):393–401. doi: 10.1007/s00441-018-2791-4
107. Wolf KI, Jha A, van Berkel A, Wild D, Janssen I, Millo CM, et al. Eruption of Metastatic Paraganglioma After Successful Therapy with (177)Lu/(90)Y-DOTATOC and (177)Lu-DOTATATE. Nucl Med Mol Imaging (2019) 53(3):223–30. doi: 10.1007/s13139-019-00579-w
108. Jha A, Patel M, Baker E, Gonzales MK, Ling A, Millo C, et al. Role of (68)Ga-DOTATATE PET/CT in a Case of SDHB-Related Pterygopalatine Fossa Paraganglioma Successfully Controlled with Octreotide. Nucl Med Mol Imaging (2020) 54(1):48–52. doi: 10.1007/s13139-019-00629-3
109. Tonyukuk V, Emral R, Temizkan S, Sertcelik A, Erden I, Corapcioglu D. Case report: patient with multiple paragangliomas treated with long acting somatostatin analogue. Endocr J (2003) 50(5):507–13. doi: 10.1507/endocrj.50.507
110. van Hulsteijn LT, van Duinen N, Verbist BM, Jansen JC, van der Klaauw AA, Smit JW, et al. Effects of octreotide therapy in progressive head and neck paragangliomas: case series. Head Neck (2013) 35(12):E391–6. doi: 10.1002/hed.23348
111. Narayan V, Fishbein L, Nathanson K, Bennett B, Pryma D, Cohen D. Use of Somatostatin Analog Therapy in Patients With Advanced Pheochromocytoma/Paraganglioma and Somatostatin-Receptor Avid Disease. Paper presented at: PANCREAS (2018).
112. Moller LN, Stidsen CE, Hartmann B, Holst JJ. Somatostatin receptors. Biochim Biophys Acta (2003) 1616(1):1–84. doi: 10.1016/S0005-2736(03)00235-9
113. Florio T. Molecular mechanisms of the antiproliferative activity of somatostatin receptors (SSTRs) in neuroendocrine tumors. Front Biosci (2008) 13:822–40. doi: 10.2741/2722
114. Unger N, Serdiuk I, Sheu SY, Walz MK, Schulz S, Saeger W, et al. Immunohistochemical localization of somatostatin receptor subtypes in benign and malignant adrenal tumours. Clin Endocrinol (Oxf) (2008) 68(6):850–7. doi: 10.1111/j.1365-2265.2007.03124.x
115. Ziegler CG, Brown JW, Schally AV, Erler A, Gebauer L, Treszl A, et al. Expression of neuropeptide hormone receptors in human adrenal tumors and cell lines: antiproliferative effects of peptide analogues. Proc Natl Acad Sci U S A (2009) 106(37):15879–84. doi: 10.1073/pnas.0907843106
116. Hofland LJ, Lamberts SW. The pathophysiological consequences of somatostatin receptor internalization and resistance. Endocr Rev (2003) 24(1):28–47. doi: 10.1210/er.2000-0001
117. Stueven AK, Kayser A, Wetz C, Amthauer H, Wree A, Tacke F, et al. Somatostatin Analogues in the Treatment of Neuroendocrine Tumors: Past, Present and Future. Int J Mol Sci (2019) 20(12):1–13. doi: 10.3390/ijms20123049
118. Vitale G, Dicitore A, Sciammarella C, Di Molfetta S, Rubino M, Faggiano A, et al. Pasireotide in the treatment of neuroendocrine tumors: a review of the literature. Endocrine-related cancer (2018) 25(6):R351–64. doi: 10.1530/ERC-18-0010
119. Plockinger U, Hoffmann U, Geese M, Lupp A, Buchfelder M, Flitsch J, et al. DG3173 (somatoprim), a unique somatostatin receptor subtypes 2-, 4- and 5-selective analogue, effectively reduces GH secretion in human GH-secreting pituitary adenomas even in Octreotide non-responsive tumours. Eur J Endocrinol (2012) 166(2):223–34. doi: 10.1530/EJE-11-0737
120. Toumpanakis C, Caplin ME. Update on the role of somatostatin analogs for the treatment of patients with gastroenteropancreatic neuroendocrine tumors. Semin Oncol (2013) 40(1):56–68. doi: 10.1053/j.seminoncol.2012.11.006
121. Zitzmann K, Andersen S, Vlotides G, Spottl G, Zhang S, Datta R, et al. The novel somatostatin receptor 2/dopamine type 2 receptor chimeric compound BIM-23A758 decreases the viability of human GOT1 midgut carcinoid cells. Neuroendocrinology (2013) 98(2):128–36. doi: 10.1159/000353784
122. Saveanu A, Muresan M, De Micco C, Taieb D, Germanetti AL, Sebag F, et al. Expression of somatostatin receptors, dopamine D(2) receptors, noradrenaline transporters, and vesicular monoamine transporters in 52 pheochromocytomas and paragangliomas. Endocrine-related cancer (2011) 18(2):287–300. doi: 10.1530/ERC-10-0175
123. Ginj M, Zhang H, Waser B, Cescato R, Wild D, Wang X, et al. Radiolabeled somatostatin receptor antagonists are preferable to agonists for in vivo peptide receptor targeting of tumors. Proc Natl Acad Sci U S A (2006) 103(44):16436–41. doi: 10.1073/pnas.0607761103
124. Wild D, Fani M, Behe M, Brink I, Rivier JE, Reubi JC, et al. First clinical evidence that imaging with somatostatin receptor antagonists is feasible. J Nucl Med (2011) 52(9):1412–7. doi: 10.2967/jnumed.111.088922
125. Fani M, Del Pozzo L, Abiraj K, Mansi R, Tamma ML, Cescato R, et al. PET of somatostatin receptor-positive tumors using 64Cu- and 68Ga-somatostatin antagonists: the chelate makes the difference. J Nucl Med (2011) 52(7):1110–8. doi: 10.2967/jnumed.111.087999
126. Fani M, Braun F, Waser B, Beetschen K, Cescato R, Erchegyi J, et al. Unexpected sensitivity of sst2 antagonists to N-terminal radiometal modifications. J Nucl Med (2012) 53(9):1481–9. doi: 10.2967/jnumed.112.102764
127. Wild D, Fani M, Fischer R, Del Pozzo L, Kaul F, Krebs S, et al. Comparison of somatostatin receptor agonist and antagonist for peptide receptor radionuclide therapy: a pilot study. J Nucl Med (2014) 55(8):1248–52. doi: 10.2967/jnumed.114.138834
128. Ballal S, Yadav MP, Bal C, Sahoo RK, Tripathi M. Broadening horizons with (225)Ac-DOTATATE targeted alpha therapy for gastroenteropancreatic neuroendocrine tumour patients stable or refractory to (177)Lu-DOTATATE PRRT: first clinical experience on the efficacy and safety. Eur J Nucl Med Mol Imaging (2020) 47(4):934–46.
Keywords: pheochromocytoma, paraganglioma, somatostatin receptors, somatostatin analog, peptide receptor radionuclide therapy, 68Ga-DOTATATE, theranostic, PET/CT
Citation: Patel M, Tena I, Jha A, Taieb D and Pacak K (2021) Somatostatin Receptors and Analogs in Pheochromocytoma and Paraganglioma: Old Players in a New Precision Medicine World. Front. Endocrinol. 12:625312. doi: 10.3389/fendo.2021.625312
Received: 02 November 2020; Accepted: 04 March 2021;
Published: 29 March 2021.
Edited by:
Naris Nilubol, National Cancer Institute, National Institutes of Health (NIH), United StatesReviewed by:
Amit Tirosh, Sheba Medical Center, IsraelCorinne Bousquet, Institut National de la Santé et de la Recherche Médicale (INSERM), France
Copyright © 2021 Patel, Tena, Jha, Taieb and Pacak. This is an open-access article distributed under the terms of the Creative Commons Attribution License (CC BY). The use, distribution or reproduction in other forums is permitted, provided the original author(s) and the copyright owner(s) are credited and that the original publication in this journal is cited, in accordance with accepted academic practice. No use, distribution or reproduction is permitted which does not comply with these terms.
*Correspondence: Karel Pacak, a2FyZWxAbWFpbC5uaWguZ292
†These authors have contributed equally to this work and share first authorship