- The First Affiliated Hospital of USTC, Division of Life Sciences and Medicine, University of Science and Technology of China, Hefei, China
Sustaining and maintaining the intricate process of spermatogenesis is liable upon hormones and growth factors acting through endocrine and paracrine pathways. The Sertoli cells (SCs) are the major somatic cells present in the seminiferous tubules and are considered to be the main regulators of spermatogenesis. As each Sertoli cell supports a specific number of germ cells, thus, the final number of Sertoli cells determines the sperm production capacity. Similarly, sex hormones are also major regulators of spermatogenesis and they can determine the proliferation of Sertoli cells. In the present review, we have critically and comprehensively discussed the role of sex hormones and some other factors that are involved in Sertoli cell proliferation, differentiation and maturation. Furthermore, we have also presented a model of Sertoli cell development based upon the recent advancement in the field of reproduction. Hence, our review article provides a general overview regarding the sex hormonal pathways governing Sertoli cell proliferation and development.
Background
Testes are destined to perform two important roles: to produce testosterone (steroidogenesis) and to maintain germ cell development (1). These functions are supported by the testicular somatic cells, Sertoli cells (SCs), which are located within the seminiferous tubules of testes (2, 3). Sertoli cells are considered as the most complex type of cells in an organism on the bases of their three-dimensional structure and their production of a microenvironment for germ cell development (3–5). Dependence of germ cells to obtain nutritional contents from Sertoli cells is owing to the presence of blood testes barrier (BTB) which physically portioned the seminiferous tubules into basal and adluminal compartments (6). The BTB is constituted by tight junction, ectoplasmic specialization (N-cadherin), desmosomes and gap junctions that are present in Sertoli cells (7–10). The SC–SC junctional complex has been studied and is known to undertake an indispensable job in testis directional morphogenesis (11, 12). Thus, Sertoli cells encompass all sorts of germ cells and have a chief assistive role in spermatogenesis.
Furthermore, developing germ cells cannot metabolize macromolecules such as lipids, carbohydrates and proteins, and most preferable energy source for germ cells is lactate molecule which is produced by Sertoli cells (13, 14). On the other hand, Sertoli cells not only provide lactate to the developing germ cells for energy production but they also supply other nutrients including amino acids, vitamins and metal ions (14–16). Another important task of Sertoli cells is to generate and produce signaling molecules including growth factors and inflammatory cytokines which are involved in a cascade of events that are necessary for the spermatogenic process (17–19). Thus, accurate establishment and proper functioning of Sertoli cells is crucial for the developing germ cells to sustain the process of spermatogenesis.
Proliferation and Maturation of Sertoli Cells
The proper proliferation of Sertoli cells takes place during their immature period and can be mediated by specific factors (20, 21). The proliferative phase of Sertoli cells varies between species and two periods of Sertoli cells proliferation (one during fetal or neonatal period and other before pubertal period) are generally observed in various species (4, 6). Furthermore, marked variations exist between mature and immature Sertoli cells especially in terms of morphological and biochemical aspects. Generally, immature Sertoli cells reside on the basement and possess cytoplasmic projections which fill up the space of seminiferous cords (20, 22). In addition to immature Sertoli cells, seminiferous tubules also contain peritubular and germ stem cells which give solid appearance with the absence of lumen (23). After puberty, the Sertoli cells start to elongate and BTB begins to establish (8). Finally, Sertoli cells switch from their immature stage to mature phase and their proliferative state is stopped (6). At this stage, mature Sertoli cells represent radical changes within their morphology and functions. Further changes occurred in the nucleus and nucleoli become large in size along with the completion of tight junction which makes the fluid filled lumen space. The whole process of Sertoli cell proliferation and maturation is regulated under strict control and any impairment in the process of Sertoli cell development or proliferation can causes pathological events which may lead to the reduction of sperm count and semen quality (6, 20, 24–26).
Sertoli cells can serve as the organizing center for testis differentiation and signalings from Sertoli cells also regulate the differentiation events of testicular cord formation and testis organogenesis (5, 27). The Sertoli cells also provide a means of canalizing gonadal fate to coordinate testis development (5). Interestingly, Sertoli cell fate, once specified, is not permanent but instead needs to be constantly reinforced (3, 5).
Testicular development and spermatogenesis are influenced by various hormones which are generally mediated by the hypothalamus–pituitary–gonad (HPG) axis (14, 27) (Figure 1). HPG axis establishes a connection between brain and testes (28, 29). The gonadotropin leuitinizing hormone (LH) and follicle stimulating hormone (FSH) are secreted by adenohypophysis which are considered to be the important regulators of testicular function (30). It has been demonstrated that FSH mainly controls the proliferation of Sertoli cells while LH regulates testosterone production (14, 27, 31). Thus, the pre-pubertal decrease of LH and subsequent FSH secretion tends to cause a disturbance in the pulsatile release of gonadotropin-releasing hormone (GnRH). This hypothalamic-releasing agent provides the main push to the gonadotropin-secreting cells of the anterior pituitary gland (32). What is more, the HPG axis also works in association with local endocrine system to mechanistically regulate the complete process of Sertoli cell maturation and testis development (30). Paracrine system intercedes with various types of cells including germ cells, peritubular myoid cells and Leydig cells. Thus, proper hormone levels and their regulation are necessary for these complex processes which further ensure the accurate and smooth development of Sertoli cells to support spermatogenic process.
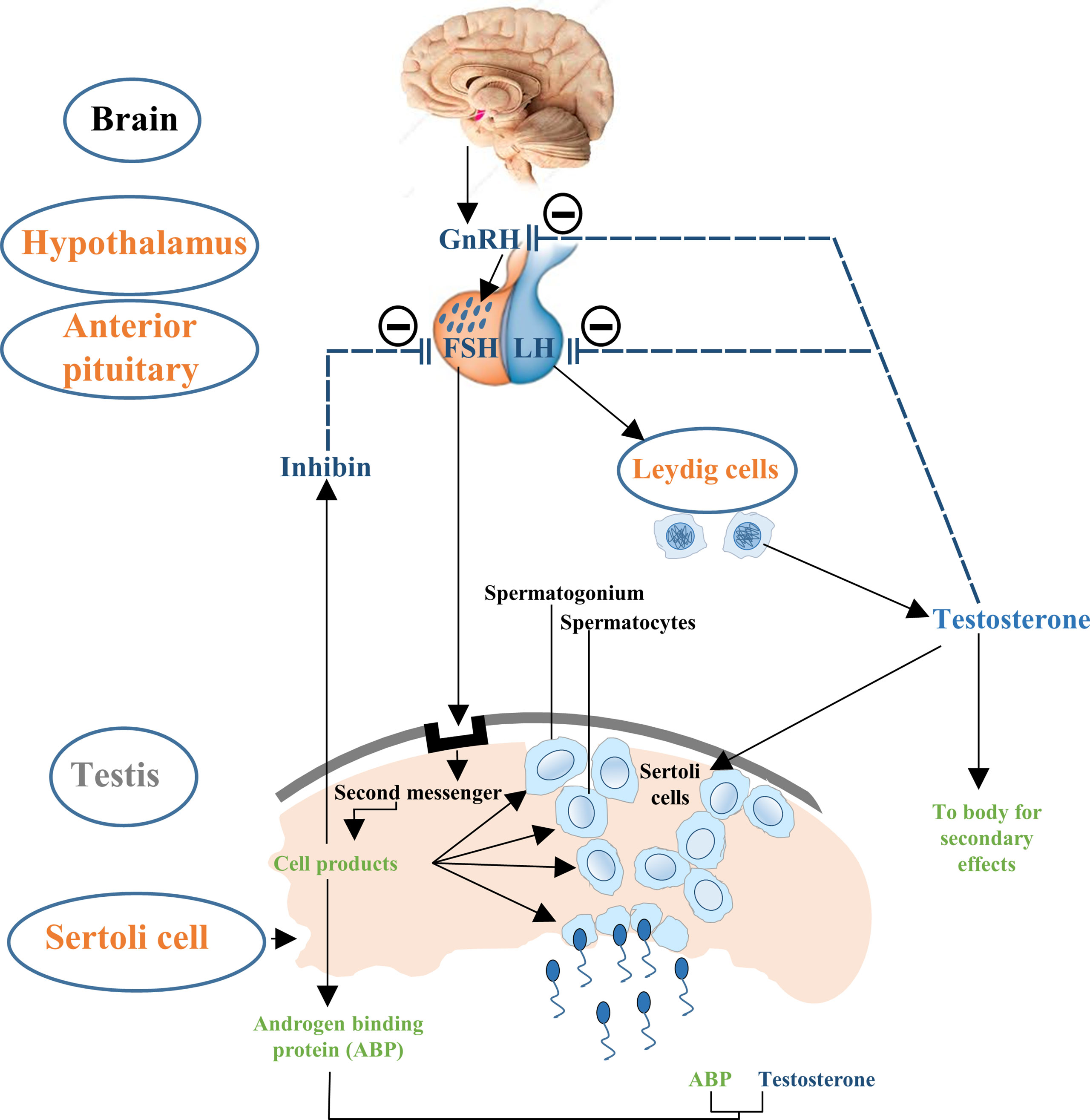
Figure 1 Flow chart description the control of hypothalamus–pituitary–testis axis on Sertoli cell proliferation. The hypothalamic GnRH modulates the biosynthesis and secretion of pituitary hormones i.e., LH and FSH. LH induces secretion of testosterone in Leydig cells and is involved in the late Sertoli cell proliferation period, followed by negative feedback reducing GnRH and LH production. FSH primarily stimulates the seminiferous tubules to form steroid hormones such as inhibin and further sustain the process of spermatogenesis. Steroid hormones i.e., testosterone and inhibin exert negative feedback effects on GnRH.
Sex Hormones in Sertoli Cell Development and Proliferation
The complex process of reproduction is generally regulated by various factors including autocrine, paracrine, juxtracrine and endocrine environment within the gonads (33). Though these processes are well inter-connected, the major function is performed by sex hormones such as leuitinizing hormone, follicle stimulating hormone and prolactin that orchestrate and coordinate sexual development, sexuality and reproduction (34–36). Sex hormones are also playing key roles in development and maturation of Sertoli cells by modulating either Sertoli cell metabolism or influencing growth signaling pathways (14, 27, 31, 35–38). These hormones also create adequate ionic environment in Sertoli cells which is required for germ cell development. In this review, we have discussed the role of reproductive hormones in association with Sertoli cell development, proliferation and maturation (Figure 2).
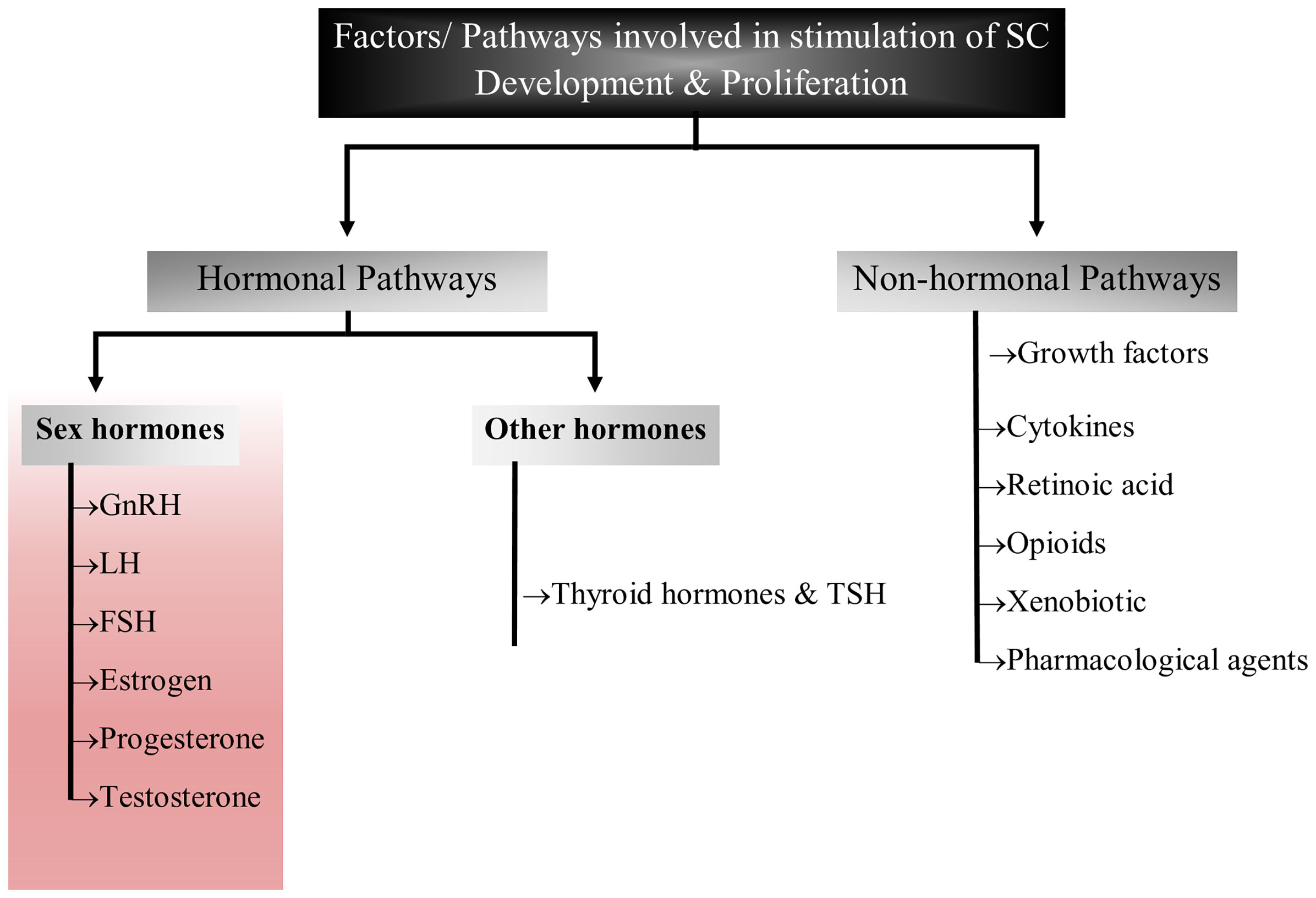
Figure 2 Flow chart diagram representing the factors/pathways involved in Sertoli cell development and proliferation. This figure summarized the role of sex hormones, hormones other than sex hormones and non-hormonal pathways that have been implicated in Sertoli cell development.
Follicle Stimulating Hormone (FSH)
FSH plays a crucial role in fertility as it influences the proliferation of Sertoli cells during perinatal life and also stimulates the production of Sertoli cell derived factors that are required for the development of germ cells and testes (39). FSH, LH, thyroid-stimulating hormone (TSH) and chorionic gonadotropin (hCG), belong to pituitary glycoprotein hormone family and these hormones are known to perform important function during Sertoli cell development, thus, directly or indirectly influencing male reproductive health. These hormones are usually existed in the form of a heterodimer which consists of a α-subunit that has the ability to associate with β-subunit (40).
The mechanism of action in which FSH binds and stimulates membrane receptor belonging to the G protein-coupled receptor (GPCR) superfamily (41). It was noted that FSH receptor (FSHR) presents tissue specificity as it is majorly expressed in granulosa cells (female) and Sertoli cells (male) (42). Furthermore, FSHR has the capability to subordinate with other type of G proteins including Gαi to initiate signaling cascade events that modulate Sertoli cell function. Impaired secretion of FSH due to homozygous mutation in the gene encoding β-subunit leads to bilateral small and soft testicles, androgen deficiency, elevated level of LH in serum, low level of testosterone, as well as azoospermia in human (43, 44). Furthermore, homozygous FSHR mutations lead to male infertility in few cases, while the A189V FSHR mutation in males is linked with subfertility but not azoospermia (45). Interestingly, Fshr knockout mice still had sperm production albeit sperm reduction was observed (46–48).
It is a well-known fact that FSH is the factor necessary for Sertoli cell mitogen which stimulates the expression of various Sertoli cell markers such as c-Myc, Cyclin A2, Cyclin D1, and proliferating cell nuclear antigen (PCNA) (39, 49). Moreover, it has been described that FSH level and FSHR expression become stable after puberty, however, a change has been observed in signaling pathways triggered by FSH during transition of Sertoli cells from proliferation to differentiation stage (50). Consistently, some pathways such as FSH-mediated ERK activation and calcium uptake are exclusively activated in immature Sertoli cells during proliferative phase. The opposite action of FSH in immature and mature Sertoli cells is related to the cAMP kinetics (51). It was found that cAMP level was low in immature rat Sertoli cells. On the other hand, higher basal concentration of cAMP was observed in 20 days old Sertoli cells along with almost 4-fold increased activity of phosphodiesterase and completely abolished in older rat Sertoli cells (52–55). Hence, it is assumed that diverse function of Sertoli cells in response to FSH might be responsible for robust onset of germ cell differentiation during prepubertal testicular maturation in rats. What is more, Gαs and Ric8b, which activate adenylate cyclase for supplementing cAMP production and gene transcription, can also cause constrained FSH action during infancy in primates (56). Thus, the FSH action on Sertoli cell development and maturation is complicated and it is still difficult to investigate the complete array of signaling events in vivo.
In fact, it is hard to differentiate the overlap signaling pathways in vivo that are triggered during Sertoli cell proliferation and maturation. Most of the studies are conducted in vitro and these studies have demonstrated some of the major signaling pathways that are stimulated by FSH. In this regard, a study described that FSH binds with its receptor (FSHR) to form Gα protein, which is further dissociated into two heteromeric molecules, Gα-subunit and Gβ/γ unit. This dissociation further stimulates a cascade signaling mechanism by activating mitogen-activated protein kinase (MAPK), or phosphoinositide 3-kinase (PI3K)/protein kinase B (PKB) and adenylate cyclase/cyclic adenosine monophosphate (cAMP)/protein kinase A (PKA) which cause a change of Sertoli cell membrane potential and calcium influx. During this process, each subunit of FSH heterodimer protein is destined to perform specific function such as Gα subunit is responsible for the activation of adenylate cyclase which further initiates the formation of cAMP and phosphorylation of PKA (57, 58). Furthermore, PKA activates structural proteins, transcription factors and enzymes which trigger diverse biological processes with varying effects on Sertoli cells (37). More specifically, FSH has biphasic effects on membrane potential of immature rat Sertoli cells, which are manifested by membrane hyperpolarization (59).
FSH was also found to stimulate cAMP/PKA which intercedes various protein phosphorylation to trigger calcium channels and their regulators. But the complete scenario of FSH stimulation of cAMP/PKA and subsequent voltage gated calcium channels (VDCC) modulation is still not clear. Previous reports described that PKA system phosphorylates α1-subunit of the VDCC resulting in calcium potentiation (60, 61). However, up till now, no research has been conducted to investigate this mechanism in Sertoli cells. The addition of PKA and adenylate cyclase inhibitors [MDL, (Bu)2cAMP and staurosporine] in cultured Sertoli cells can partially impede FSH mediated calcium uptake, indicating involvement of other mechanisms in calcium influx during Sertoli cell proliferation (62). Further evidence showed that Sertoli cell proliferation is not only depend upon AC/cAMP/PKA pathway, some alternative mechanisms also exist, such as FSH-mediated dissociation of the Gαi-GGβ/γ heterodimer which causes calcium influx through L-type VDCC and [14C]-MeAIB transport system (63, 64). Moreover, FSH has the ability to transport small amino acids through activation of system A (which is basically designed for the transport of neutral amino acids with small side chains such as alanine, serine and glutamine). System A activation by FSH can provide nitrogen from alanine and other amino acids for biosynthesis of proteins and nucleotides which are essential for cellular growth (65, 66). Similarly, alanine is converted into pyruvate and is used as energy substrate by Sertoli cells. The presence of this alternative mechanism of Sertoli cell proliferation has been validated by inhibition of [14C]-MeAIB transport system (67). FSH activates PI3K downstream target, PKB, which further stimulates enhanced uptake of glucose, calcium and small amino acids in cultured Sertoli cells (68). The active PI3K/AKT signaling pathway is required to stimulate the actions of FSH, whereas an active ERK/MAPK pathway can inhibit the expression of aromatase (such as Cyp19a1) (69). Altogether, these pathways are essential for proliferation and differentiation of immature Sertoli cells that pave the way for successful spermatogenesis (70). Taking consideration of all these studies, a comprehensive diagram explaining the role of FSH and other factors in Sertoli cell proliferation can be proposed (27) (Figure 3).
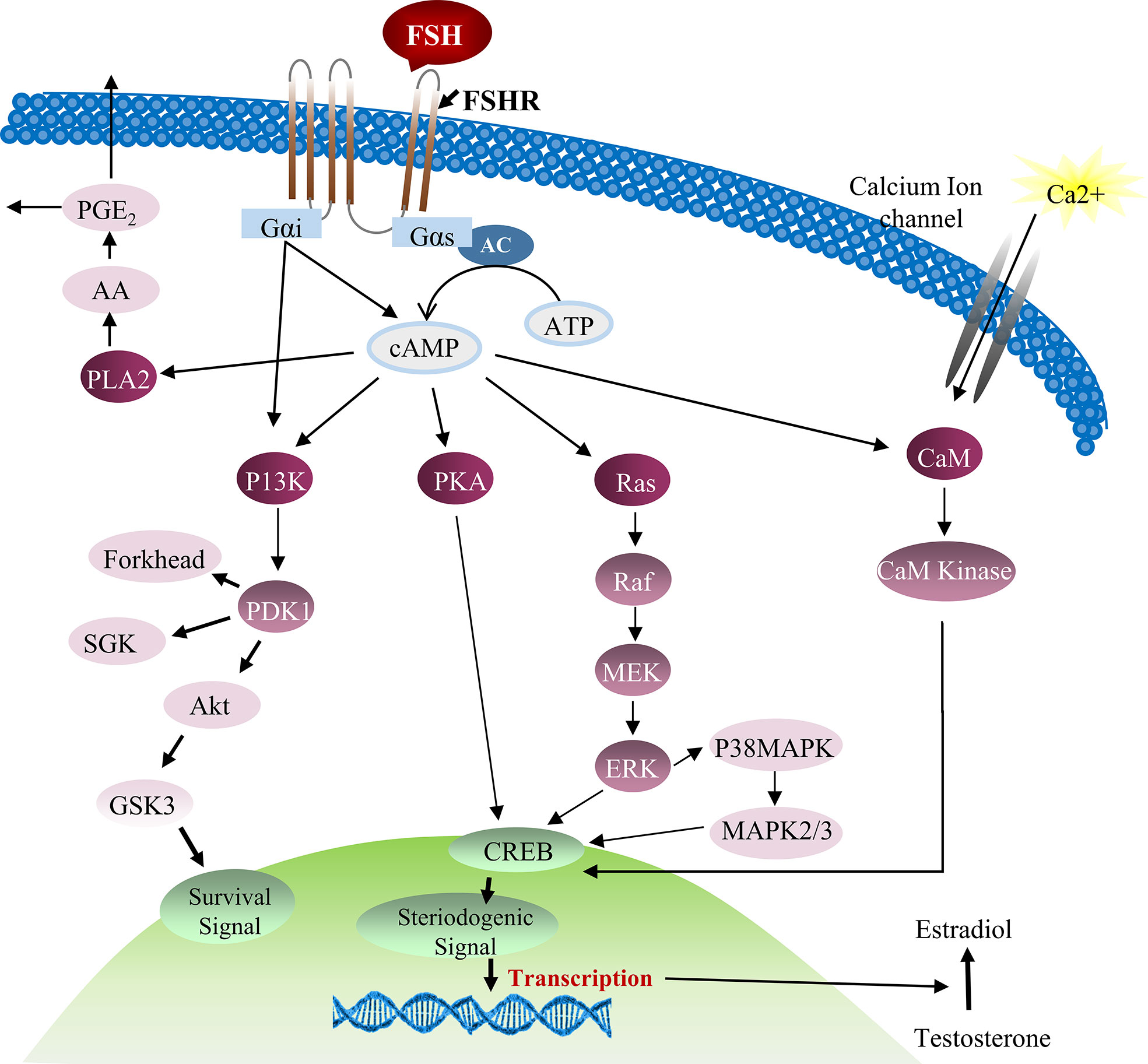
Figure 3 FSH and testosterone signaling pathways in Sertoli cell proliferation. Initially FSH binding to the FSH receptor causes receptor coupled G proteins to activate adenylate cyclase (AC) and increase intracellular cAMP levels. Multiple factors can be activated by cAMP in Sertoli cells including PKA that can phosphorylate a number of proteins and also regulate the expression and activity of numerous transcription factors including CREB. FSH also causes Ca2+ influx into Sertoli cells that is mediated by cAMP and perhaps PKA modification of surface Ca2+ channels. Depolarization of the cell is also involved in Ca2+ influx. Elevated Ca2+ levels can activate calmodulin and CaM kinases that have multiple potential downstream effects including the phosphorylation of CREB. During puberty, FSH activates the MAP kinase cascade and ERK kinase in Sertoli cells most likely via cAMP interactions with guanine nucleotide exchange factors (GEFs) and activation of Ras-like G proteins. ERK is capable of activating transcription factors including SRF, c-jun and CREB. FSH and cAMP likely act through GEFs to activate PI3-K and then phosphoinositide dependent protein kinase (PDK1) and PKB in Sertoli cells. FSH also mediates the induction of PLA2 and the subsequent release of arachadonic acid (71, 72).
Androgens
Cessation of proliferative phase of Sertoli cells is mediated by changes in gene expression and establishment of BTB and finally Sertoli cells become able to sustain developing germ cells. Thus, it is imperative to investigate the factors that are involved in transition of Sertoli cells from proliferation to maturation phase. In this regard, some studies have demonstrated that androgens and their derivative products are key mediators for Sertoli cell proliferative phase cessation in diverse species (73, 74). In fact, androgens play important functions that reach far beyond the reproductive process, for example 5α-dihydrotestosterone (DHT) regulates glucose consumption and lactate production in cultured rat Sertoli cells (35, 75). Similarly, it is also reported that long time treatment of DHT in cultured human Sertoli cells can cause decrease expression of lactate dehydrogenase A and monocarboxylate transporter 4 (MCT4) levels (76).
The function of androgens is intensively investigated in terms of fertility and spermatogenesis while its role in Sertoli cell maturation and development generally remains elusive, instead of knowing that high amounts of androgens is produced by Leydig cells in the form of testosterone. The dynamic level of testosterone is observed during different developmental stages of organism such as its concentration increases at the end of fetal life, and starts to decrease from birth until puberty, and then increases again (77, 78). Testosterone performs its function through classical and non-classical mechanism. Non-genomic signaling of testosterone can activate gene transcription through CREB mediated pathway (79, 80). Furthermore, zinc transporter ZIP9 subfamily protein that is localized on the plasma membrane also has ability to mediate testosterone level (81).
Testosterone can also function in a non-classical pathway through the androgen receptor to rapidly activate kinases. For example, by increasing testosterone levels, the MAP kinase cascade is rapidly activated in Sertoli cells. An inhibitor of non-classical testosterone signaling blocked meiosis in pubertal mice and caused germ cell loss in adult mouse testes, while a classical pathway inhibitor caused the premature release of immature germ cells. Thus, classical and nonclassical testosterone signaling have overlapping and distinct functions that are required for the spermatogenesis and male fertility. Furthermore, some findings suggested that the non-classical testosterone signaling can act via Src and ERK kinases to facilitate the adhesion of germ cells to Sertoli cells (82, 83). On the other hand, the non-classical signaling of androgens alter the cellular process within seconds to minutes (84). This system can increase calcium influx by activation of phospholipase C which causes hydrolysis of phosphatidylinositol 4,5-biphosphate (PIP2) (85). The absence of PIP2 decreases negative charges on membranes and causes closing of K+ ATP channels and opening of the VDCC, which results in calcium influx (86). Similarly, the calcium influx stirred by testosterone may be involved in several other biological processes such as cytoskeleton rearrangement, gene transcription and cell proliferation (27, 42). Thus, it is believed that non-classical action of androgens is more closely related to Sertoli cell maturation and spermatogenesis.
Studies that in vivo treatment of testosterone caused reduced incorporation of [H3] thymidine by Sertoli cells in some species suggesting that testosterone can inhibit the proliferation of Sertoli cells (35, 87). Further work by Buzzard and colleagues displayed that the addition of testosterone in cultured Sertoli cells leads to strong inhibition of proliferation as well as increased expression of cell cycle inhibitor markers such as p27Kip1 and p21Cip1, while it also induces the enhanced expression of GATA-1 which is a marker for Sertoli cell differentiation (88). By crossing hypogonadal (hpg) mice that lack gonadotrophins and intratesticular androgen with mice lacking ubiquitous AR (ARKO) or specifically in Sertoli cells (SCARKO), O’Shaughnessy et al. found that dihydrotestosterone has no effect on germ cell numbers in hpgSCARKO and hpgARKO mice, while testosterone increased germ cell numbers in hpgSCARKO and hpgARKO mice, and this was associated with stimulation of FSH release (89). Thus, androgen stimulation of spermatogenesis requires direct androgen action on the Sertoli cells. However, some studies on mouse model demonstrated controversial results related to androgens involvement in Sertoli cell proliferation. For example, Tfm mutant mice lacking functional androgen receptor and AR knockout mice displayed reduced Sertoli cell number (90–92). But the observed phenotypes of Tfm and AR knockout mice could not be attributed entirely to the androgen effect on Sertoli cells since androgens are also known to be produced by peritubular cells. Nevertheless, specific deletion of AR in mouse did not show any aberration in Sertoli cell number as well as the expression of Sertoli cell maturation markers (90). These results demonstrated that androgens may affect Sertoli cell proliferation through an indirect way because peritubular cells secrete Activin A which also influences Sertoli cell physiology (93–95). Furthermore, SCAR KO mice showed minor changes which further suggests that the effect of androgen on number of Sertoli cells is not regulated by the direct action. Subsequently, TgSCAR (transgenic mouse with gain of function) mice showed reduced Sertoli cell proliferation which further lead to decreased testis size (96). Altogether, it can be deduced that AR expression in Sertoli cells is wisely orchestrated to avoid early maturation of Sertoli cells.
The synergistic actions of testosterone and FSH via testicular Sertoli cells regulate male fertility (53). FSH acts through receptors (FSHR) on the Sertoli cell to stimulate spermatogenesis while androgens promote testis growth through receptors (AR) on the Sertoli cells, Leydig cells and peritubular myoid cells. By examining the effects on testis development of ablating FSHRs (FSHRKO mice) and/or ARs ubiquitously (ARKO mice) or specifically on the Sertoli cells (SCARKO mice), results showed that development of most testicular parameters is more dependent on FSH action than androgen action mediated through the Sertoli cells prior to puberty. Post-pubertally, germ cell numbers and spermatogenesis are dependent on FSH and androgen action through the Sertoli cells (91). Furthermore, through the analysis of mice lacking both FSH receptors and androgen receptors in Sertoli cells (FSHRKO-SCARKO), Abel et al. found that FSH and androgen act through redundant, additive, and synergistic regulation in spermatogenesis and Sertoli cell activity (97). Additionally, in pubertal primate (Macaca mulatta) Sertoli cells, prolonged stimulation of testosterone significantly elevated the expression of genes involved in FSH signaling pathway such as FSHR, GNAS and RIC8B, and this was associated with a rise in cAMP production. Testosterone also augmented FSH induced expression of genes like SCF, GDNF, ABP and Transferrin. Such a coordinated network of hormonal signaling in Sertoli cells may facilitate the timely onset of the first spermatogenic wave in pubertal primates and is responsible for normal spermatogenesis (53). On the other hand, it has been reported that insufficient FSH and androgen are associated with azoospermia in infantile primate testes (98). Thus, it is assumed that infant primate Sertoli cells may have insufficient number of AR and the binding ability of testosterone to AR might be compromised during primate infancy.
Luteinizing Hormone
Luteinizing hormone (LH) belongs to the family of glycoproteins, with α subunit and hormone-specific β subunit. LH and FSH both were isolated as molecules in 1942 and these two gonadotrophins are involved in synthesis of estradiol and ultimately form the androgens. LH accelerates testosterone production in Leydig cells, thus, helping in spermatogenesis by directly impacting on Sertoli cells. Knockout mice for LH receptor (Lhr) have no testosterone production with disrupted spermatogenesis. This LH-dependent testosterone absence leads to azoospermia, however, in some cases absence of LH signaling does not disrupt the pathway fully and results in oligozoospermia with low testosterone production (99). But knockout mice for luteinizing hormone/choriogonadotropin receptor (Lhcgr) had elevated levels of Wnt5a (wingless-type MMTV integration site family member 5A) in Sertoli cells that favors cell proliferation. It was also noted that absence of LH caused alternations in genes associated with Sertoli cell development and proliferation (100, 101).
Three genetically modified mouse model were generated to study the effect of LH on Sertoli cell development by completely or partially reducing its activity. Two distinct strategies were used to generate these mutant models; one with LH-deficient hpg-(hypogonadal) mice to selectively study either pituitary-independent transgenic-(tg) FSH or ligand-independent activated tg FSH receptor (FSHR) expression, and second model used LH receptor (Lhr)-deficient mice in which their gonads were isolated to examine endogenous mouse FSH effects on gonad development. Analysis of these models showed subtle differences in gem cell maturation between tg-hpg and Lhr-null mouse models, indicating that the FSH cannot fully restore Sertoli cell number in absence of LH activity (102). Thus, the synergistic effect of both LH and FSH is important for proper proliferation and development of Sertoli cells.
Estrogens
Estrogens are steroid pleiotropic hormones, present in ovary and testis. These hormones act by cytosolic estrogen receptors (ERs). Alpha (α) and beta (β) receptors are found in animals while in fishes, ERγ has been discovered as the third type receptor. ERα and ERβ are located in the cell membrane; either as homodimers (ERα-ERα or ERβ-ERβ) or as heterodimers (ERα-ERβ). These hormones play their roles in production, regulation as well as maintenance of concentration of testicular liquid (36).
A study conducted by Royer et al. indicated that estradiol initiates the proliferation of Sertoli cells by activating classical estrogen receptors and G protein-coupled estrogen receptor which further induce a cascade of signaling events through CREB activation (103). It is important to mention that estrogen expression in testis is dynamic and varies from postnatal to adult life. Its concentration increases from 20 days of post-partum and continues to increase till 30 days old while aromatase transcripts has not been detected in adult rat Sertoli cells. However, in adult rats, aromatase expression is noted in Leydig, pachytene spermatocytes and round spermatids (104). It is suggested that Sertoli cells may produce estrogen in immature animals while the source of estrogens in adult animals comes from Leydig or germ cells (105). Hence, complete understanding of physiological effects of estrogens is necessary to investigate its actual function in postnatal testis development.
Studies found that ERα knockout mice or rats are infertile while ERβ knockout mice or rats have no such abnormalities, which indicates that ERα subunit is essential for fertility and reproduction. This function is evident in 15 days old rat Sertoli cells in which ERα promotes cell proliferation by acting on NF-kB (nuclear factor-kB) in P13K and ERK1/2 (extracellular signal-regulated kinase 1/2) manner and ultimately increasing the levels of Cyclin D1. On the other hand, ERβ promotes cell cycle arrest by interacting with 17β-estradiol (E2) (106).
Progesterone
Progesterone is a major cholesterol-derivative steroid and is specifically involved in reproduction. The receptors of this hormone are localized in the nucleus and cytoplasm of spermatogenic cells, Sertoli cells and occasionally in the Leydig cells. Structurally, two isoform receptors of this hormone exist; namely PR-A and PR-B and these intracellular proteins belongs to nuclear receptor superfamily of transcription factor (107). High level of progesterone has inhibitory role in spermatogenesis by limiting the production of Leydig cells and Sertoli cells at developmental stage (108). The effect of progesterone was examined by generating progesterone receptor (PR) knockout mice. PR knockout mice displayed large testis size, increased total sperm contents and increased number of Sertoli cells. On the other hand, synthetic progestins such as levonorgestrel (LNG) in combination with testosterone caused suppression of spermatogenesis and increased germ cell apoptosis (108).
Prolactin
Prolactin is a type of polypeptide hormone that is involved in wide range of biological functions including lactation, osmoregulation, immune articulation and reproduction (109). Prolactin receptors (PLR) are present on Sertoli cells and prolactin through its receptors mediates proliferation of Sertoli cells (110). Various reports reveled its biological function in reproduction and elevated level of prolactin leads to hypogonadism and male infertility (111). It is highly recognized that prolactin regulates testicular function by two ways either altering pituitary function by inducing LH and FSH production or Leydig cells through modulation of testosterone hormone (1). Furthermore, targetted mutation of prolactin receptor in model organism displayed mild phenotype indicating that prolactin has partial effects on male reproductive health (112).
Other Regulatory Factors Involved in Sertoli Cell Development and Proliferation
Besides the mentioned hormones, many other factors such as growth factors, cytokines, xenobiotic and pharmacological agents, have been identified and are involved in Sertoli cell development process. Opioids, such as α-melanocyte-stimulating hormone (αMSH), β-endorphin and proopiomelanocortin (POMC), mainly produced in Leydig cells, exert direct paracrine actions on Sertoli cell proliferation (113–115). The in vitro exposure of fetal human testis to ibuprofen does not modify the number of Sertoli cells but decreases AMH and SOX9 expression, suggesting a role in Sertoli cell maturation (116).
Insulin Receptor Signaling Family
The insulin receptor tyrosine kinase family consists of insulin receptor (IR), IGF-1R and insulin related receptor (Irr). These receptors are present in all types of cells in eutherian mammals (117). Mice lacking IR and IGF-1R die within four days after birth due to ketoacidosis and respiratory failure, respectively (118). A lot of studies had investigated the function of these hormones and new insights regarding their involvement in reproductive system (29, 50, 117, 119–121). Recently, a study investigated the in vivo function of IR and IGF-1R in which both factors work in a synergistic way to regulate the Sertoli cell number and testis size. Furthermore, the study also described that both receptors and their downstream molecules are critical for the development of male gonads and sexual differentiation (122). Similarly, the insulin-related peptide hormone relaxin (Rlx) has also been recognized to perform an essential role in reproduction and it precipitates in the regulation of the cyclic adenosine monophosphate and nitric oxide pathways that are implicated in Sertoli cell proliferation (123).
It has been recognized that insulin is involved in energy metabolism and also regulates cell proliferation and differentiation. Generally, the insulin function is interceded by IR through phosphorylation that further activates classical signaling mechanism involving adaptor protein such as insulin receptor substrate-1 (IRS-1) (28). Different studies have reported the function of insulin in testicular development, in modulating testicular cell function (38, 124, 125), or even influencing HPG axis function (28, 29). Furthermore, the compromised function of insulin is the leading cause of Diabetes Mellitus (DM) which is usually accompanied by aberrant testosterone levels (126). Thus, it can be deduced that insulin could regulate testosterone secretion in human and animal models. A study demonstrated that insulin directly influences Sertoli cell metabolism by affecting amino acid accumulation, glucose transport and lactate production either through the modification of glucose transporter expression or altering important glycolytic enzyme activity (121). Further studies indicated that cultured Sertoli cells can cause reduced lactate production and altered caspase-dependent apoptotic signaling (75, 127). Similarly, it has also been reported that insulin activate calcium-dependent membrane depolarization in immature Sertoli cells, which is mostly induced through IGF-1R activation (120). Altogether, these findings clearly indicate the importance of insulin function in regulating Sertoli cell metabolism which is further manifested by Sertoli cell proliferation.
Cytokines
Various studies reported that inflammatory cytokines are not only produced by macrophages in response to inflammatory signals but these cytokines are also secreted from Sertoli cells and appear to take part in the regulation of Sertoli cell proliferation (1, 19). For example, interleukin-1, 6 (IL-1 and IL-6) and tumor necrosis factor α (TNF-α) are produced by Sertoli cells and in vitro studies demonstrated that all of these cytokines are involved in Sertoli cell metabolism by activating the production of transferrin. Furthermore, it was also noted that only IL-1, neither IL-6 nor TNF-α, enhanced lactate production and secretion during Sertoli cell proliferation (1). Notably, IL-1 activity in Sertoli cells can be specifically neutralized by IL-1α antiserum, implying that IL-1α is the major isoform of IL-1 in Sertoli cells (17). But the underlying pathophysiological mechanism is still not completely understood due to the lack of in vivo studies. Interestingly, animal model studies with disrupted interleukin or tumor necrosis factors displayed no obvious alterations in testicular development. Thus, the actual function of these cytokines in relevance to Sertoli cell development is still obscure and needs more investigations.
Thyroid Hormones
It has been described that thyroid hormones regulate lactate production, glucose transporter type 1 mRNA levels, aromatase activity, Sertoli cell proliferation and other processes of Sertoli cells in various mammalian species (128–130). The involvement of thyroid hormones in establishing the Sertoli cell population have been extensively investigated and results indicated that thyroid hormones can affect Sertoli cell proliferation through direct or indirect ways. Generally, indirect way of thyroid hormone on Sertoli cells is mediated by triiodothyronine (T3) that inhibits FSH production and leads to reduced Sertoli cell proliferation (128). Some studies demonstrated that T3 treatment can reduce Sertoli cell proliferation activity, as well as Sertoli cell proliferation period and Sertoli cell number (88, 128, 131). Similarly, it has been described that T3 also stimulates the maturation of Sertoli cells in vitro implying that T3 can terminates Sertoli cell proliferation and favors the terminal maturation of Sertoli cells (132). Thyroid hormones can halt Sertoli cell proliferation by accelerating the accumulation of cell cycle inhibitors p27Kip1 and p21Cip1 (88, 133, 134). To be noted, thyroid hormone, retinoic acid, and testosterone share similar suppressive effects on the rate of Sertoli cell division without any apparent additive effects (88). Another study displayed that Connexins 43 (Cx43) could be an intermediate target of T3 in the inhibition of Sertoli cell proliferation (135). Thus, a balance level of thyroid hormones during early life of development is essential for the terminal differentiation of Sertoli cells.
WNT and BMP Signaling Pathways
The vertebrate WNT (Wingless-related integration site) family consists of 19 secreted cysteine-rich glycoproteins (136). Though WNT signaling exerts an antagonistic effect on testis-determining pathways in sex determination during the embryonic stage, it promotes sperm maturation in adult epididymis (137). During the development of seminiferous tubules, Wnt/β-catenin can play an important role in the differentiation of Sertoli cells. However, these findings appear to be inconsistent about the influence of Wnt/β-catenin signaling. For example, several studies have shown that β-catenin deletion does not induce aberration in Sertoli cells, but β-catenin stabilization results in immaturity, inadequate differentiation and irregular cellular interaction in Sertoli cells, as well as reduced proliferation and increased apoptosis of germ cells (138–141). Similar findings have also been observed when the Wnt/β-catenin pathway is activated in APC (adenomatous polyposis coli-conditional) knockout mice (142). Therefore, the suppression Wnt/β-catenin pathway is required to sustain normal maturation and proliferation of Sertoli cells (143).
Bone morphogenetic proteins (BMPs) and transforming growth factor-beta superfamily (TGF-β) also have pivotal roles in reproductive biology. Their roles have been established by various in vivo and in vitro studies. BMP2, BMP4, BMP8a and BMP8b are involved in specification of primordial germ cell (PGC), acceleration of spermatogonial proliferation as well as are responsible for adult spermatogenesis in vivo (144).
A study found that Bmp4 was expressed in postnatal days 4 and 7 isolated Sertoli cells implying that Bmp4 perform important role in early postnatal testis development. In testes, multiple BMP genes are expressed and BMP7 and BMP8 a/b transcripts were specifically found in germ cells at various stages of differentiation (145, 146), thus indicating that these molecules may mediate paracrine interactions which are secreted by Sertoli cells. Furthermore, mice lacking BMP8b had smaller testes, similar type of phenotype was also observed in BMP8a null mice (147, 148). A recent study explored the role of Sclerostin domain containing 1 protein (Sostdc1) in modulating the Sertoli cell gene expression and its possible outcomes on mouse spermatogenesis. Interestingly, Pradhan et al. found that Sostdc1 is a negative regulator of spermatogenesis, and found that down regulation of Sostdc1 during puberty is necessary for quantitatively and qualitatively normal spermatogenesis (149). Thus, it is argued that Sostdc1 is a dual BMP/Wnt regulator and plays indirect role in mouse spermatogenesis by influencing Sertoli cells.
Activin and Inhibin
Activins are dimeric glycoproteins, consisting of β subunits and members of TGF-β superfamily. Activins mediate FSH production by a cascade of interacting proteins event (150). Their ability to bind with type II receptor causes phosphorylation of type I receptor, starting a series of phosphorylation of SMAD proteins (SMAD2, SMAD3, SMAD4) which ultimately triggers the transcription of FSHβ encoding gene (150, 151). On the other hand, inhibins and follistatin are considered as antagonists of activins (152). They are also glycoproteins but structurally different from activins. Inhibins compete at binding sites for activins which ultimately affects its activation. Thus, activins, inhibins and follistatin collectively form a complex autocrine network that plays a vital role in fertility. The interruptions of these can cause lower testis size, progressive sterility, delayed fertility as well as other fertility-related issues due to defective Sertoli cell development and proliferation (153, 154).
Retinoic Acid
Retinoic acid (biologically active component of vitamin A) is a major factor that control the complex process of spermatogenesis and is also important driven force of Sertoli cell development (155). RA induce the initiation of spermatogonia differentiation in the mammals and its activity is generally governed by FSH (156). The functional role of RA was verified by generating vitamin A-deficient (VAD) mice that were infertile due to spermatogonia differentiation arrest at the Aaligned stage and treating them with RA results in the complete recovery of spermatogenesis (157).
Summary
Sex hormonal regulation of Sertoli cell proliferation, differentiation and maturation is an intricate process which requires synergistic effects of these hormones along with the regulatory factors including IGF-1R, insulin, thyroid hormones and cytokines. All these hormones and factors have been implicated in various stages of Sertoli cell development and their balanced action of mechanism is mandatory for ensuring accurate Sertoli cell number, establishment of BTB and maintaining spermatogenesis. Although, recent in vivo studies explained the involvement of FSH, androgen, estrogen and IGF-1R to be essential for Sertoli cell development, still the complete scenario of this complex process is unresolved. Thus, it is suggested that there are some additional factors needs to be elucidated in future. Similarly, in vivo description of some factors such as TGF-α family members, TGF-β, TNF-α, and IL-1 may shed light on complex process of Sertoli cell proliferation and testis development. Subsequently, the detailed mechanism of action of these hormones might give us insights into a better comprehension of hormonal regulation in Sertoli cell proliferation, as well as provide possible therapeutic molecules for human infertility.
Author Contributions
RK and XJ conceived the review. WS, BS, AK, and SD collected the information. WS, RK, and XJ wrote the paper. JW, XJ, WL, and RK modified the manuscript. All authors contributed to the article and approved the submitted version.
Funding
This work was supported by the National Key Research and Developmental Program of China (2018YFC1004700), National Natural Science Foundation of China (31890780 and 82071709), the Open Project Fund from Key Laboratory of Reproduction Regulation of NHC (KF2020-07) and Key Laboratory of Male Reproduction and Genetics of NHC (KF202003), and Natural Science Foundation of Qinghai (2019-HZ-823).
Conflict of Interest
The authors declare that the research was conducted in the absence of any commercial or financial relationships that could be construed as a potential conflict of interest.
References
1. Petersen C, Soder O. The Sertoli Cell–a Hormonal Target and ‘Super’ Nurse for Germ Cells That Determines Testicular Size. Horm Res (2006) 66:153–61. doi: 10.1159/000094142
2. Vogl AW, Vaid KS, Guttman JA. The Sertoli Cell Cytoskeleton. Adv Exp Med Biol (2008) 636:186–211. doi: 10.1007/978-0-387-09597-4_11
3. Makela JA, Koskenniemi JJ, Virtanen HE, Toppari J. Testis Development. Endocr Rev (2019) 40:857–905. doi: 10.1210/er.2018-00140
4. Koskenniemi JJ, Virtanen HE, Toppari J. Testicular Growth and Development in Puberty. Curr Opin Endocrinol Diabetes Obes (2017) 24:215–24. doi: 10.1097/MED.0000000000000339
5. Svingen T, Koopman P. Building the Mammalian Testis: Origins, Differentiation, and Assembly of the Component Cell Populations. Genes Dev (2013) 27:2409–26. doi: 10.1101/gad.228080.113
6. Sharpe RM, McKinnell C, Kivlin C, Fisher JS. Proliferation and Functional Maturation of Sertoli Cells, and Their Relevance to Disorders of Testis Function in Adulthood. Reproduction (2003) 125:769–84. doi: 10.1530/rep.0.1250769
7. Mruk DD, Cheng CY. Sertoli-Sertoli and Sertoli-Germ Cell Interactions and Their Significance in Germ Cell Movement in the Seminiferous Epithelium During Spermatogenesis. Endocr Rev (2004) 25:747–806. doi: 10.1210/er.2003-0022
8. Parte P, Balasinor N, Gill-Sharma MK, Maitra A, Juneja HS. Temporal Effect of Tamoxifen on Cytochrome P450 Side Chain Cleavage Gene Expression and Steroid Concentration in Adult Male Rats. J Steroid Biochem Mol Biol (2002) 82:349–58. doi: 10.1016/S0960-0760(02)00193-0
9. Jiang XH, Bukhari I, Zheng W, Yin S, Wang Z, Cooke HJ, et al. Blood-Testis Barrier and Spermatogenesis: Lessons From Genetically-Modified Mice. Asian J Androl (2014) 16:572–80. doi: 10.4103/1008-682X.125401
10. Jiang X, Ma T, Zhang Y, Zhang H, Yin S, Zheng W, et al. Specific Deletion of Cdh2 in Sertoli Cells Leads to Altered Meiotic Progression and Subfertility of Mice. Biol Reprod (2015) 92:79. doi: 10.1095/biolreprod.114.126334
11. Gerber J, Weider K, Hambruch N, Brehm R. Loss of Connexin43 (Cx43) in Sertoli Cells Leads to Spatio-Temporal Alterations in Occludin Expression. Histol Histopathol (2014) 29:935–48. doi: 10.14670/HH-29.935
12. Rode K, Sieme H, Richterich P, Brehm R. Characterization of the Equine Blood-Testis Barrier During Tubular Development in Normal and Cryptorchid Stallions. Theriogenology (2015) 84:763–72. doi: 10.1016/j.theriogenology.2015.05.009
13. Cheng CY, Mruk DD. The Blood-Testis Barrier and its Implications for Male Contraception. Pharmacol Rev (2012) 64:16–64. doi: 10.1124/pr.110.002790
14. Alves MG, Rato L, Carvalho RA, Moreira PI, Socorro S, Oliveira PF. Hormonal Control of Sertoli Cell Metabolism Regulates Spermatogenesis. Cell Mol Life Sci (2013) 70:777–93. doi: 10.1007/s00018-012-1079-1
15. Rato L, Alves MG, Socorro S, Duarte AI, Cavaco JE, Oliveira PF. Metabolic Regulation is Important for Spermatogenesis. Nat Rev Urol (2012) 9:330–8. doi: 10.1038/nrurol.2012.77
16. Sylvester SR, Griswold MD. The Testicular Iron Shuttle: A “Nurse” Function of the Sertoli Cells. J Androl (1994) 15:381–5.
17. Gerard N, Syed V, Bardin W, Genetet N, Jegou B. Sertoli Cells are the Site of Interleukin-1 Alpha Synthesis in Rat Testis. Mol Cell Endocrinol (1991) 82:R13–6. doi: 10.1016/0303-7207(91)90019-O
18. Galdieri M, Monaco L, Stefanini M. Secretion of Androgen Binding Protein by Sertoli Cells is Influenced by Contact With Germ Cells. J Androl (1984) 5:409–15. doi: 10.1002/j.1939-4640.1984.tb00806.x
19. Wu H, Wang D, Shu Z, Zhou H, Zuo H, Wang S, et al. Cytokines Produced by Microwave-Radiated Sertoli Cells Interfere With Spermatogenesis in Rat Testis. Andrologia (2012) 44 Suppl 1:590–9. doi: 10.1111/j.1439-0272.2011.01232.x
20. Fortunati N. Sex Hormone-Binding Globulin: Not Only a Transport Protein. What News is Around the Corner? J Endocrinol Invest (1999) 22:223–34. doi: 10.1007/BF03343547
21. Sun H, Zhang G, Dong F, Wang F, Cao W. Reprogramming Sertoli Cells Into Pluripotent Stem Cells. Cell Reprogram (2014) 16:196–205. doi: 10.1089/cell.2013.0083
22. Bouraima-Lelong H, Vanneste M, Delalande C, Zanatta L, Wolczynski S, Carreau S. Aromatase Gene Expression in Immature Rat Sertoli Cells: Age-Related Changes in the FSH Signalling Pathway. Reprod Fertil Dev (2010) 22:508–15. doi: 10.1071/RD09168
23. Bhushan S, Aslani F, Zhang Z, Sebastian T, Elsasser HP, Klug J. Isolation of Sertoli Cells and Peritubular Cells From Rat Testes. J Vis Exp (2016) 8:e53389. doi: 10.3791/53389
24. Aleem M, Padwal V, Choudhari J, Balasinor N, Parte P, Gill-Sharma M. Cyproterone Acetate Affects Protamine Gene Expression in the Testis of Adult Male Rat. Contraception (2005) 71:379–91. doi: 10.1016/j.contraception.2004.11.003
25. Jiang X, Yin S, Fan S, Bao J, Jiao Y, Ali A, et al. Npat-Dependent Programmed Sertoli Cell Proliferation is Indispensable for Testis Cord Development and Germ Cell Mitotic Arrest. FASEB J (2019) 33:9075–86. doi: 10.1096/fj.201802289RR
26. Zheng W, Nazish J, Wahab F, Khan R, Jiang X, Shi Q. DDB1 Regulates Sertoli Cell Proliferation and Testis Cord Remodeling by Tgfbeta Pathway. Genes (Basel) (2019) 10:974. doi: 10.3390/genes10120974
27. Escott GM, da Rosa LA, Loss Eda S. Mechanisms of Hormonal Regulation of Sertoli Cell Development and Proliferation: A Key Process for Spermatogenesis. Curr Mol Pharmacol (2014) 7:96–108. doi: 10.2174/1874467208666150126155032
28. Ballester J, Munoz MC, Dominguez J, Rigau T, Guinovart JJ, Rodriguez-Gil JE. Insulin-Dependent Diabetes Affects Testicular Function by FSH- and LH-Linked Mechanisms. J Androl (2004) 25:706–19. doi: 10.1002/j.1939-4640.2004.tb02845.x
29. Schoeller EL, Albanna G, Frolova AI, Moley KH. Insulin Rescues Impaired Spermatogenesis Via the Hypothalamic-Pituitary-Gonadal Axis in Akita Diabetic Mice and Restores Male Fertility. Diabetes (2012) 61:1869–78. doi: 10.2337/db11-1527
30. Pivonello R, Menafra D, Riccio E, Garifalos F, Mazzella M, de Angelis C, et al. Metabolic Disorders and Male Hypogonadotropic Hypogonadism. Front Endocrinol (Lausanne) (2019) 10:345. doi: 10.3389/fendo.2019.00345
31. Meroni SB, Galardo MN, Rindone G, Gorga A, Riera MF, Cigorraga SB. Molecular Mechanisms and Signaling Pathways Involved in Sertoli Cell Proliferation. Front Endocrinol (Lausanne) (2019) 10:224. doi: 10.3389/fendo.2019.00224
32. Plant TM. Hypothalamic Control of the Pituitary-Gonadal Axis in Higher Primates: Key Advances Over the Last Two Decades. J Neuroendocrinol (2008) 20:719–26. doi: 10.1111/j.1365-2826.2008.01708.x
33. Eertmans F, Dhooge W, Stuyvaert S, Comhaire F. Endocrine Disruptors: Effects on Male Fertility and Screening Tools for Their Assessment. Toxicol Vitro (2003) 17:515–24. doi: 10.1016/S0887-2333(03)00121-8
35. O’Shaughnessy PJ. Hormonal Control of Germ Cell Development and Spermatogenesis. Semin Cell Dev Biol (2014) 29:55–65. doi: 10.1016/j.semcdb.2014.02.010
36. Amenyogbe E, Chen G, Wang Z, Lu X, Lin M, Lin AY. A Review on Sex Steroid Hormone Estrogen Receptors in Mammals and Fish. Int J Endocrinol (2020) 2020:5386193. doi: 10.1155/2020/5386193
37. Ulloa-Aguirre A, Reiter E, Crepieux P. FSH Receptor Signaling: Complexity of Interactions and Signal Diversity. Endocrinology (2018) 159:3020–35. doi: 10.1210/en.2018-00452
38. Ipsa E, Cruzat VF, Kagize JN, Yovich JL, Keane KN. Growth Hormone and Insulin-Like Growth Factor Action in Reproductive Tissues. Front Endocrinol (Lausanne) (2019) 10:777. doi: 10.3389/fendo.2019.00777
39. Meachem SJ, Ruwanpura SM, Ziolkowski J, Ague JM, Skinner MK, Loveland KL. Developmentally Distinct In Vivo Effects of FSH on Proliferation and Apoptosis During Testis Maturation. J Endocrinol (2005) 186:429–46. doi: 10.1677/joe.1.06121
40. Alevizaki M, Huhtaniemi I. Structure-Function Relationships of Glycoprotein Hormones; Lessons From Mutations and Polymorphisms of the Thyrotrophin and Gonadotrophin Subunit Genes. Hormones (Athens) (2002) 1:224–32. doi: 10.14310/horm.2002.1171
41. Ulloa-Aguirre A, Dias JA, Bousfield G, Huhtaniemi I, Reiter E. Trafficking of the Follitropin Receptor. Methods Enzymol (2013) 521:17–45. doi: 10.1016/B978-0-12-391862-8.00002-8
42. Simoni M, Gromoll J, Nieschlag E. The Follicle-Stimulating Hormone Receptor: Biochemistry, Molecular Biology, Physiology, and Pathophysiology. Endocr Rev (1997) 18:739–73. doi: 10.1210/edrv.18.6.0320
43. Phillip M, Arbelle JE, Segev Y, Parvari R. Male Hypogonadism Due to a Mutation in the Gene for the Beta-Subunit of Follicle-Stimulating Hormone. N Engl J Med (1998) 338:1729–32. doi: 10.1056/NEJM199806113382404
44. Zheng J, Mao J, Cui M, Liu Z, Wang X, Xiong S, et al. Novel Fshbeta Mutation in a Male Patient With Isolated FSH Deficiency and Infertility. Eur J Med Genet (2017) 60:335–9. doi: 10.1016/j.ejmg.2017.04.004
45. Tapanainen JS, Aittomaki K, Min J, Vaskivuo T, Huhtaniemi IT. Men Homozygous for an Inactivating Mutation of the Follicle-Stimulating Hormone (FSH) Receptor Gene Present Variable Suppression of Spermatogenesis and Fertility. Nat Genet (1997) 15:205–6. doi: 10.1038/ng0297-205
46. Kumar TR, Wang Y, Lu N, Matzuk MMJNG. Follicle Stimulating Hormone is Required for Ovarian Follicle Maturation But Not Male Fertility. Nat Genet (1997) 15:201–4. doi: 10.1038/ng0297-201
47. Dierich A, Sairam MR, Monaco L, Fimia GM, Gansmuller A, LeMeur M, et al. Impairing Follicle-Stimulating Hormone (FSH) Signaling In Vivo: Targeted Disruption of the FSH Receptor Leads to Aberrant Gametogenesis and Hormonal Imbalance. Proc Natl Acad Sci USA (1998) 95:13612–7. doi: 10.1073/pnas.95.23.13612
48. Abel MH, Wootton AN, Wilkins V, Huhtaniemi I, Knight PG, Charlton HMJE. The Effect of a Null Mutation in the Follicle-Stimulating Hormone Receptor Gene on Mouse Reproduction. Endocrinology (2000) 141:1795–803. doi: 10.1210/endo.141.5.7456
49. Dahia CL, Rao AJ. Regulation of FSH Receptor, Pkibeta, IL-6 and Calcium Mobilization: Possible Mediators of Differential Action of FSH. Mol Cell Endocrinol (2006) 247:73–81. doi: 10.1016/j.mce.2005.10.029
50. Riera MF, Regueira M, Galardo MN, Pellizzari EH, Meroni SB, Cigorraga SB. Signal Transduction Pathways in FSH Regulation of Rat Sertoli Cell Proliferation. Am J Physiol Endocrinol Metab (2012) 302:E914–23. doi: 10.1152/ajpendo.00477.2011
51. Volpato KC, Menegaz D, Leite LD, Barreto KP, de Vilhena Garcia E, Silva FR. Involvement of K+ Channels and Calcium-Dependent Pathways in the Action of T3 on Amino Acid Accumulation and Membrane Potential in Sertoli Cells of Immature Rat Testis. Life Sci (2004) 74:1277–88. doi: 10.1016/j.lfs.2003.08.005
52. Levallet G, Levallet J, Bouraima-Lelong H, Bonnamy PJ. Expression of the Camp-Phosphodiesterase PDE4D Isoforms and Age-Related Changes in Follicle-Stimulating Hormone-Stimulated PDE4 Activities in Immature Rat Sertoli Cells. Biol Reprod (2007) 76:794–803. doi: 10.1095/biolreprod.106.055343
53. Bhattacharya I, Basu S, Pradhan BS, Sarkar H, Nagarajan P, Majumdar SS. Testosterone Augments FSH Signaling by Upregulating the Expression and Activity of FSH-Receptor in Pubertal Primate Sertoli Cells. Mol Cell Endocrinol (2019) 482:70–80. doi: 10.1016/j.mce.2018.12.012
54. Levallet G, Bonnamy PJ, Levallet J. Alteration of Cell Membrane Proteoglycans Impairs FSH Receptor/Gs Coupling and ERK Activation Through PP2A-Dependent Mechanisms in Immature Rat Sertoli Cells. Biochim Biophys Acta (2013) 1830:3466–75. doi: 10.1016/j.bbagen.2013.02.027
55. Bhattacharya I, Pradhan BS, Sarda K, Gautam M, Basu S, SSJAJoP-E M, et al. A Switch in Sertoli Cell Responsiveness to FSH may be Responsible for Robust Onset of Germ Cell Differentiation During Prepubartal Testicular Maturation in Rats. Am J Physiol Endocrinol Metab (2012) 303:E886–E98. doi: 10.1152/ajpendo.00293.2012
56. Bhattacharya I, Basu S, Sarda K, Gautam M, Nagarajan P, Pradhan BS, et al. Low Levels of Galphas and Ric8b in Testicular Sertoli Cells may Underlie Restricted FSH Action During Infancy in Primates. Endocrinology (2015) 156:1143–55. doi: 10.1210/en.2014-1746
57. Ulloa-Aguirre A, Zarinan T, Pasapera AM, Casas-Gonzalez P, Dias JA. Multiple Facets of Follicle-Stimulating Hormone Receptor Function. Endocrine (2007) 32:251–63. doi: 10.1007/s12020-008-9041-6
58. Ma Y, Wang H. PI3K/Akt/Foxo: A Novel Participant in Signal Transduction in Bone Cells Under Mechanical Stimulation. Cell Biol Int (2012) 36:923–6. doi: 10.1042/CBI20120078
59. Nichols CG. KATP Channels as Molecular Sensors of Cellular Metabolism. Nature (2006) 440:470–6. doi: 10.1038/nature04711
60. Sculptoreanu A, Rotman E, Takahashi M, Scheuer T, Catterall WA. Voltage-Dependent Potentiation of the Activity of Cardiac L-Type Calcium Channel Alpha 1 Subunits Due to Phosphorylation by Camp-Dependent Protein Kinase. Proc Natl Acad Sci USA (1993) 90:10135–9. doi: 10.1073/pnas.90.21.10135
61. Yang L, Liu G, Zakharov SI, Bellinger AM, Mongillo M, Marx SO. Protein Kinase G Phosphorylates Cav1.2 Alpha1c and Beta2 Subunits. Circ Res (2007) 101:465–74. doi: 10.1161/CIRCRESAHA.107.156976
62. Lai TH, Lin YF, Wu FC, Tsai YH. Follicle-Stimulating Hormone-Induced Galphah/Phospholipase C-Delta1 Signaling Mediating a Noncapacitative Ca2+ Influx Through T-Type Ca2+ Channels in Rat Sertoli Cells. Endocrinology (2008) 149:1031–7. doi: 10.1210/en.2007-1244
63. Sharma OP, Flores JA, Leong DA, Veldhuis JD. Cellular Basis for Follicle-Stimulating Hormone-Stimulated Calcium Signaling in Single Rat Sertoli Cells: Possible Dissociation From Effects of Adenosine 3’,5’-Monophosphate. Endocrinology (1994) 134:1915–23. doi: 10.1210/endo.134.4.8137759
64. Touyz RM, Jiang L, Sairam MR. Follicle-Stimulating Hormone Mediated Calcium Signaling by the Alternatively Spliced Growth Factor Type I Receptor. Biol Reprod (2000) 62:1067–74. doi: 10.1095/biolreprod62.4.1067
65. Yahyaoui R, Perez-Frias J. Amino Acid Transport Defects in Human Inherited Metabolic Disorders. Int J Mol Sci (2019) 21:119. doi: 10.3390/ijms21010119
66. Kilberg MS, Stevens BR, Novak DA. Recent Advances in Mammalian Amino Acid Transport. Annu Rev Nutr (1993) 13:137–65. doi: 10.1146/annurev.nu.13.070193.001033
67. Jacobus AP, Loss ES, Wassermann GF. Pertussis Toxin Nullifies the Depolarization of the Membrane Potential and the Stimulation of the Rapid Phase of Ca Entry Through L-Type Calcium Channels That are Produced by Follicle Stimulating Hormone in 10- to 12-Day-Old Rat Sertoli Cells. Front Physiol (2010) 1:138. doi: 10.3389/fphys.2010.00138
68. Meroni SB, Riera MF, Pellizzari EH, Cigorraga SB. Regulation of Rat Sertoli Cell Function by FSH: Possible Role of Phosphatidylinositol 3-Kinase/Protein Kinase B Pathway. J Endocrinol (2002) 174:195–204. doi: 10.1677/joe.0.1740195
69. McDonald CA, Millena AC, Reddy S, Finlay S, Vizcarra J, Khan SA, et al. Follicle-Stimulating Hormone-Induced Aromatase in Immature Rat Sertoli Cells Requires an Active Phosphatidylinositol 3-Kinase Pathway and is Inhibited Via the Mitogen-Activated Protein Kinase Signaling Pathway. Mol Endocrinol (2006) 20:608–18. doi: 10.1210/me.2005-0245
70. Hirsch E, Costa C, Ciraolo E. Phosphoinositide 3-Kinases as a Common Platform for Multi-Hormone Signaling. J Endocrinol (2007) 194:243–56. doi: 10.1677/JOE-07-0097
71. Smith LB, Walker WH. The Regulation of Spermatogenesis by Androgens. Semin Cell Dev Biol (2014) 30:2–13. doi: 10.1016/j.semcdb.2014.02.012
72. De Gendt K, Verhoeven GJM. Tissue-and Cell-Specific Functions of the Androgen Receptor Revealed Through Conditional Knockout Models in Mice. Mol Cell Endocrinol (2012) 352:13–25. doi: 10.1016/j.mce.2011.08.008
73. Martins AD, Alves MG, Simoes VL, Dias TR, Rato L, Moreira PI, et al. Control of Sertoli Cell Metabolism by Sex Steroid Hormones is Mediated Through Modulation in Glycolysis-Related Transporters and Enzymes. Cell Tissue Res (2013) 354:861–8. doi: 10.1007/s00441-013-1722-7
74. Berger T. Testicular Estradiol and the Pattern of Sertoli Cell Proliferation in Prepuberal Bulls. Theriogenology (2019) 136:60–5. doi: 10.1016/j.theriogenology.2019.06.031
75. Alves MG, Socorro S, Silva J, Barros A, Sousa M, Cavaco JE, et al. In Vitro Cultured Human Sertoli Cells Secrete High Amounts of Acetate That is Stimulated by 17beta-Estradiol and Suppressed by Insulin Deprivation. Biochim Biophys Acta (2012) 1823:1389–94. doi: 10.1016/j.bbamcr.2012.06.002
76. Rato L, Alves MG, Socorro S, Carvalho RA, Cavaco JE, Oliveira PF. Metabolic Modulation Induced by Oestradiol and DHT in Immature Rat Sertoli Cells Cultured In Vitro. Biosci Rep (2012) 32:61–9. doi: 10.1042/BSR20110030
77. Tapanainen J, Kuopio T, Pelliniemi LJ, Huhtaniemi I. Rat Testicular Endogenous Steroids and Number of Leydig Cells Between the Fetal Period and Sexual Maturity. Biol Reprod (1984) 31:1027–35. doi: 10.1095/biolreprod31.5.1027
78. Lee VW, de Kretser DM, Hudson B, Wang C. Variations in Serum FSH, LH and Testosterone Levels in Male Rats From Birth to Sexual Maturity. J Reprod Fertil (1975) 42:121–6. doi: 10.1530/jrf.0.0420121
79. Walker WH. Non-Classical Actions of Testosterone and Spermatogenesis. Philos Trans R Soc Lond B Biol Sci (2010) 365:1557–69. doi: 10.1098/rstb.2009.0258
80. Fix C, Jordan C, Cano P, Walker W. Testosterone Activates Mitogen-Activated Protein Kinase and the Camp Response Element Binding Protein Transcription Factor in Sertoli Cells. Proc Natl Acad Sci USA (2004) 101:10919–24. doi: 10.1073/pnas.0404278101
81. Berg AH, Rice CD, Rahman MS, Dong J, Thomas P. Identification and Characterization of Membrane Androgen Receptors in the ZIP9 Zinc Transporter Subfamily: I. Discovery in Female Atlantic Croaker and Evidence ZIP9 Mediates Testosterone-Induced Apoptosis of Ovarian Follicle Cells. Endocrinology (2014) 155:4237–49. doi: 10.1210/en.2014-1198
82. Shupe J, Cheng J, Puri P, Kostereva N, Walker WH. Regulation of Sertoli-Germ Cell Adhesion and Sperm Release by FSH and Nonclassical Testosterone Signaling. Mol Endocrinol (2011) 25:238–52. doi: 10.1210/me.2010-0030
83. Toocheck C, Clister T, Shupe J, Crum C, Ravindranathan P, Lee TK, et al. Mouse Spermatogenesis Requires Classical and Nonclassical Testosterone Signaling. Biol Reprod (2016) 94:11. doi: 10.1095/biolreprod.115.132068
84. Lucas-Herald AK, Alves-Lopes R, Montezano AC, Ahmed SF, Touyz RM. Genomic and non-Genomic Effects of Androgens in the Cardiovascular System: Clinical Implications. Clin Sci (Lond) (2017) 131:1405–18. doi: 10.1042/CS20170090
85. Loss ES, Jacobsen M, Costa ZS, Jacobus AP, Borelli F, Wassermann GF. Testosterone Modulates K(+)ATP Channels in Sertoli Cell Membrane Via the PLC-PIP2 Pathway. Horm Metab Res (2004) 36:519–25. doi: 10.1055/s-2004-825753
86. Loss ES, Jacobus AP, Wassermann GF. Rapid Signaling Responses in Sertoli Cell Membranes Induced by Follicle Stimulating Hormone and Testosterone: Calcium Inflow and Electrophysiological Changes. Life Sci (2011) 89:577–83. doi: 10.1016/j.lfs.2011.05.017
87. Orth JM, Higginbotham CA, Salisbury RL. Hemicastration Causes and Testosterone Prevents Enhanced Uptake of [3H] Thymidine by Sertoli Cells in Testes of Immature Rats. Biol Reprod (1984) 30:263–70. doi: 10.1095/biolreprod30.1.263
88. Buzzard JJ, Wreford NG, Morrison JR. Thyroid Hormone, Retinoic Acid, and Testosterone Suppress Proliferation and Induce Markers of Differentiation in Cultured Rat Sertoli Cells. Endocrinology (2003) 144:3722–31. doi: 10.1210/en.2003-0379
89. O’Shaughnessy PJ, Verhoeven G, De Gendt K, Monteiro A, Abel MH. Direct Action Through the Sertoli Cells is Essential for Androgen Stimulation of Spermatogenesis. Endocrinology (2010) 151:2343–8. doi: 10.1210/en.2009-1333
90. Tan KA, De Gendt K, Atanassova N, Walker M, Sharpe RM, Saunders PT, et al. The Role of Androgens in Sertoli Cell Proliferation and Functional Maturation: Studies in Mice With Total or Sertoli Cell-Selective Ablation of the Androgen Receptor. Endocrinology (2005) 146:2674–83. doi: 10.1210/en.2004-1630
91. O’Shaughnessy PJ, Monteiro A, Abel M. Testicular Development in Mice Lacking Receptors for Follicle Stimulating Hormone and Androgen. PloS One (2012) 7:e35136. doi: 10.1371/journal.pone.0035136
92. Johnston H, Baker PJ, Abel M, Charlton HM, Jackson G, Fleming L, et al. Regulation of Sertoli Cell Number and Activity by Follicle-Stimulating Hormone and Androgen During Postnatal Development in the Mouse. Endocrinology (2004) 145:318–29. doi: 10.1210/en.2003-1055
93. Buzzard JJ, Farnworth PG, De Kretser DM, O’Connor AE, Wreford NG, Morrison JR. Proliferative Phase Sertoli Cells Display a Developmentally Regulated Response to Activin In Vitro. Endocrinology (2003) 144:474–83. doi: 10.1210/en.2002-220595
94. de Winter JP, Vanderstichele HM, Verhoeven G, Timmerman MA, Wesseling JG, de Jong FH. Peritubular Myoid Cells From Immature Rat Testes Secrete Activin-a and Express Activin Receptor Type II In Vitro. Endocrinology (1994) 135:759–67. doi: 10.1210/endo.135.2.8033824
95. Nicholls PK, Stanton PG, Chen JL, Olcorn JS, Haverfield JT, Qian H, et al. Activin Signaling Regulates Sertoli Cell Differentiation and Function. Endocrinology (2012) 153:6065–77. doi: 10.1210/en.2012-1821
96. Hazra R, Corcoran L, Robson M, McTavish KJ, Upton D, Handelsman DJ, et al. Temporal Role of Sertoli Cell Androgen Receptor Expression in Spermatogenic Development. Mol Endocrinol (2013) 27:12–24. doi: 10.1210/me.2012-1219
97. Abel MH, Baker PJ, Charlton HM, Monteiro A, Verhoeven G, De Gendt K, et al. Spermatogenesis and Sertoli Cell Activity in Mice Lacking Sertoli Cell Receptors for Follicle-Stimulating Hormone and Androgen. Endocrinology (2008) 149:3279–85. doi: 10.1210/en.2008-0086
98. Majumdar SS, Sarda K, Bhattacharya I, Plant T. Insufficient Androgen and FSH Signaling may be Responsible for the Azoospermia of the Infantile Primate Testes Despite Exposure to an Adult-Like Hormonal Milieu. Hum Reprod (2012) 27:2515–25. doi: 10.1093/humrep/des184
99. Syed V, Khan SA, Lindh M, Ritzen EM. Ontogeny and Cellular Origin of a Rat Seminiferous Tubule Factor(s) That Inhibits LH-Dependent Testosterone Production by Interstitial Cells In Vitro. Int J Androl (1987) 10:711–20. doi: 10.1111/j.1365-2605.1987.tb00373.x
100. Tanaka T, Kanatsu-Shinohara M, Lei Z, Rao CV, Shinohara T. The Luteinizing Hormone-Testosterone Pathway Regulates Mouse Spermatogonial Stem Cell Self-Renewal by Suppressing WNT5A Expression in Sertoli Cells. Stem Cell Rep (2016) 7:279–91. doi: 10.1016/j.stemcr.2016.07.005
101. Ma X, Dong Y, Matzuk MM, Kumar TR. Targeted Disruption of Luteinizing Hormone Beta-Subunit Leads to Hypogonadism, Defects in Gonadal Steroidogenesis, and Infertility. Proc Natl Acad Sci USA (2004) 101:17294–9. doi: 10.1073/pnas.0404743101
102. Allan CM, Garcia A, Spaliviero J, Zhang FP, Jimenez M, Huhtaniemi I, et al. Complete Sertoli Cell Proliferation Induced by Follicle-Stimulating Hormone (FSH) Independently of Luteinizing Hormone Activity: Evidence From Genetic Models of Isolated FSH Action. Endocrinology (2004) 145:1587–93. doi: 10.1210/en.2003-1164
103. Royer C, Lucas TF, Lazari MF, Porto CS. 17Beta-Estradiol Signaling and Regulation of Proliferation and Apoptosis of Rat Sertoli Cells. Biol Reprod (2012) 86:108. doi: 10.1095/biolreprod.111.096891
104. Bois C, Delalande C, Nurmio M, Parvinen M, Zanatta L, Toppari J, et al. Age- and Cell-Related Gene Expression of Aromatase and Estrogen Receptors in the Rat Testis. J Mol Endocrinol (2010) 45:147–59. doi: 10.1677/JME-10-0041
105. Carreau S, Delalande C, Silandre D, Bourguiba S, Lambard S. Aromatase and Estrogen Receptors in Male Reproduction. Mol Cell Endocrinol (2006) 246:65–8. doi: 10.1016/j.mce.2005.11.021
106. Macheroni C, Lucas TFG, Porto CS. The Role of Estrogen Receptors in Rat Sertoli Cells at Different Stages of Development. Heliyon (2020) 6:e05363. doi: 10.1016/j.heliyon.2020.e05363
107. Liu T, Shi F, Ying Y, Chen Q, Tang Z, Lin H. Mouse Model of Menstruation: An Indispensable Tool to Investigate the Mechanisms of Menstruation and Gynaecological Diseases (Review). Mol Med Rep (2020) 22:4463–74. doi: 10.3892/mmr.2020.11567
108. Lue Y, Wang C, Lydon JP, Leung A, Li J, Swerdloff RS. Functional Role of Progestin and the Progesterone Receptor in the Suppression of Spermatogenesis in Rodents. Andrology (2013) 1:308–17. doi: 10.1111/j.2047-2927.2012.00047.x
109. Guillaumot P, Benahmed M. Prolactin Receptors are Expressed and Hormonally Regulated in Rat Sertoli Cells. Mol Cell Endocrinol (1999) 149:163–8. doi: 10.1016/S0303-7207(98)00246-9
110. Guillaumot P, Tabone E, Benahmed M. Sertoli Cells as Potential Targets of Prolactin Action in the Testis. Mol Cell Endocrinol (1996) 122:199–206. doi: 10.1016/0303-7207(96)03891-9
111. Scarabelli L, Caviglia D, Bottazzi C, Palmero S. Prolactin Effect on Pre-Pubertal Sertoli Cell Proliferation and Metabolism. J Endocrinol Invest (2003) 26:718–22. doi: 10.1007/BF03347352
112. Huhtaniemi I, Bartke A. Perspective: Male Reproduction. Endocrinology (2001) 142:2178–83. doi: 10.1210/endo.142.6.8228
113. Tsong SD, Phillips D, Halmi N, Liotta AS, Margioris A, Bardin CW, et al. ACTH and Beta-Endorphin-Related Peptides are Present in Multiple Sites in the Reproductive Tract of the Male Rat. Endocrinology (1982) 110:2204–6. doi: 10.1210/endo-110-6-2204
114. Bardin CW, Shaha C, Mather J, Salomon Y, Margioris AN, Liotta AS, et al. Identification and Possible Function of Pro-Opiomelanocortin-Derived Peptides in the Testis. Ann N Y Acad Sci (1984) 438:346–64. doi: 10.1111/j.1749-6632.1984.tb38296.x
115. Fabbri A, Knox G, Buczko E, Dufau ML. Beta-Endorphin Production by the Fetal Leydig Cell: Regulation and Implications for Paracrine Control of Sertoli Cell Function. Endocrinology (1988) 122:749–55. doi: 10.1210/endo-122-2-749
116. Ben Maamar M, Lesne L, Hennig K, Desdoits-Lethimonier C, Kilcoyne KR, Coiffec I, et al. Ibuprofen Results in Alterations of Human Fetal Testis Development. Sci Rep (2017) 7:44184. doi: 10.1038/srep44184
117. Nef S, Verma-Kurvari S, Merenmies J, Vassalli JD, Efstratiadis A, Accili D, et al. Testis Determination Requires Insulin Receptor Family Function in Mice. Nature (2003) 426:291–5. doi: 10.1038/nature02059
118. Rother KI, Accili D. Role of Insulin Receptors and IGF Receptors in Growth and Development. Pediatr Nephrol (2000) 14:558–61. doi: 10.1007/s004670000351
119. Pitetti JL, Calvel P, Zimmermann C, Conne B, Papaioannou MD, Aubry F, et al. An Essential Role for Insulin and IGF1 Receptors in Regulating Sertoli Cell Proliferation, Testis Size, and FSH Action in Mice. Mol Endocrinol (2013) 27:814–27. doi: 10.1210/me.2012-1258
120. Escott GM, de Castro AL, Jacobus AP, Loss ES. Insulin and IGF-I Actions on IGF-I Receptor in Seminiferous Tubules From Immature Rats. Biochim Biophys Acta (2014) 1838:1332–7. doi: 10.1016/j.bbamem.2014.02.002
121. Oliveira PF, Alves MG, Rato L, Laurentino S, Silva J, Sa R, et al. Effect of Insulin Deprivation on Metabolism and Metabolism-Associated Gene Transcript Levels of In Vitro Cultured Human Sertoli Cells. Biochim Biophys Acta (2012) 1820:84–9. doi: 10.1016/j.bbagen.2011.11.006
122. Griffeth RJ, Carretero J, Burks DJ. Insulin Receptor Substrate 2 is Required for Testicular Development. PloS One (2013) 8:e62103. doi: 10.1371/journal.pone.0062103
123. Dschietzig T, Bartsch C, Baumann G, Stangl K. Relaxin-a Pleiotropic Hormone and its Emerging Role for Experimental and Clinical Therapeutics. Pharmacol Ther (2006) 112:38–56. doi: 10.1016/j.pharmthera.2006.03.004
124. Meneses MJ, Borges DO, Dias TR, Martins FO, Oliveira PF, Macedo MP, et al. Knockout of Insulin-Degrading Enzyme Leads to Mice Testicular Morphological Changes and Impaired Sperm Quality. Mol Cell Endocrinol (2019) 486:11–7. doi: 10.1016/j.mce.2019.02.011
125. Cannarella R, Condorelli RA, La Vignera S, Calogero AE. Effects of the Insulin-Like Growth Factor System on Testicular Differentiation and Function: A Review of the Literature. Andrology (2018) 6:3–9. doi: 10.1111/andr.12444
126. Lopez-Alvarenga JC, Zarinan T, Olivares A, Gonzalez-Barranco J, Veldhuis JD, Ulloa-Aguirre A. Poorly Controlled Type I Diabetes Mellitus in Young Men Selectively Suppresses Luteinizing Hormone Secretory Burst Mass. J Clin Endocrinol Metab (2002) 87:5507–15. doi: 10.1210/jc.2002-020803
127. Dias TR, Rato L, Martins AD, Simoes VL, Jesus TT, Alves MG, et al. Insulin Deprivation Decreases Caspase-Dependent Apoptotic Signaling in Cultured Rat Sertoli Cells. ISRN Urol (2013) 2013:970370. doi: 10.1155/2013/970370
128. van Haaster LH, de Jong FH, Docter R, de Rooij DG. High Neonatal Triiodothyronine Levels Reduce the Period of Sertoli Cell Proliferation and Accelerate Tubular Lumen Formation in the Rat Testis, and Increase Serum Inhibin Levels. Endocrinology (1993) 133:755–60. doi: 10.1210/endo.133.2.8344214
129. Van Haaster LH, De Jong FH, Docter R, De Rooij DG. The Effect of Hypothyroidism on Sertoli Cell Proliferation and Differentiation and Hormone Levels During Testicular Development in the Rat. Endocrinology (1992) 131:1574–6. doi: 10.1210/endo.131.3.1505485
130. Ando S, Sirianni R, Forastieri P, Casaburi I, Lanzino M, Rago V, et al. Aromatase Expression in Prepuberal Sertoli Cells: Effect of Thyroid Hormone. Mol Cell Endocrinol (2001) 178:11–21. doi: 10.1016/S0303-7207(01)00443-9
131. Palmero S, Prati M, Bolla F, Fugassa E. Tri-Iodothyronine Directly Affects Rat Sertoli Cell Proliferation and Differentiation. J Endocrinol (1995) 145:355–62. doi: 10.1677/joe.0.1450355
132. Holsberger DR, Kiesewetter SE, Cooke PS. Regulation of Neonatal Sertoli Cell Development by Thyroid Hormone Receptor Alpha1. Biol Reprod (2005) 73:396–403. doi: 10.1095/biolreprod.105.041426
133. Holsberger DR, Cooke PS. Understanding the Role of Thyroid Hormone in Sertoli Cell Development: A Mechanistic Hypothesis. Cell Tissue Res (2005) 322:133–40. doi: 10.1007/s00441-005-1082-z
134. Sun Y, Yang W, Luo H, Wang X, Chen Z, Zhang J, et al. Thyroid Hormone Inhibits the Proliferation of Piglet Sertoli Cell Via PI3K Signaling Pathway. Theriogenology (2015) 83:86–94. doi: 10.1016/j.theriogenology.2014.08.003
135. Gilleron J, Nebout M, Scarabelli L, Senegas-Balas F, Palmero S, Segretain D, et al. A Potential Novel Mechanism Involving Connexin 43 Gap Junction for Control of Sertoli Cell Proliferation by Thyroid Hormones. J Cell Physiol (2006) 209:153–61. doi: 10.1002/jcp.20716
136. Lombardi AP, Royer C, Pisolato R, Cavalcanti FN, Lucas TF, Lazari MF, et al. Physiopathological Aspects of the Wnt/Beta-Catenin Signaling Pathway in the Male Reproductive System. Spermatogenesis (2013) 3:e23181. doi: 10.4161/spmg.23181
137. Kerr GE, Young JC, Horvay K, Abud HE, Loveland KL. Regulated Wnt/Beta-Catenin Signaling Sustains Adult Spermatogenesis in Mice. Biol Reprod (2014) 90:3. doi: 10.1095/biolreprod.112.105809
138. Chang H, Gao F, Guillou F, Taketo MM, Huff V, Behringer RR. Wt1 Negatively Regulates Beta-Catenin Signaling During Testis Development. Development (2008) 135:1875–85. doi: 10.1242/dev.018572
139. Tanwar PS, Kaneko-Tarui T, Zhang L, Rani P, Taketo MM, Teixeira J. Constitutive WNT/Beta-Catenin Signaling in Murine Sertoli Cells Disrupts Their Differentiation and Ability to Support Spermatogenesis. Biol Reprod (2010) 82:422–32. doi: 10.1095/biolreprod.109.079335
140. Boyer A, Yeh JR, Zhang X, Paquet M, Gaudin A, Nagano MC, et al. CTNNB1 Signaling in Sertoli Cells Downregulates Spermatogonial Stem Cell Activity Via WNT4. PloS One (2012) 7:e29764. doi: 10.1371/journal.pone.0029764
141. Das DS, Wadhwa N, Kunj N, Sarda K, Pradhan BS, Majumdar SS. Dickkopf Homolog 3 (DKK3) Plays a Crucial Role Upstream of WNT/Beta-CATENIN Signaling for Sertoli Cell Mediated Regulation of Spermatogenesis. PloS One (2013) 8:e63603. doi: 10.1371/journal.pone.0063603
142. Tanwar PS, Zhang L, Teixeira JM. Adenomatous Polyposis Coli (APC) is Essential for Maintaining the Integrity of the Seminiferous Epithelium. Mol Endocrinol (2011) 25:1725–39. doi: 10.1210/me.2011-0057
143. Dong WL, Tan FQ, Yang WX. Wnt Signaling in Testis Development: Unnecessary or Essential? Gene (2015) 565:155–65. doi: 10.1016/j.gene.2015.04.066
144. Ducy P, Karsenty G. The Family of Bone Morphogenetic Proteins. Kidney Int (2000) 57:2207–14. doi: 10.1046/j.1523-1755.2000.00081.x
145. Zhao GQ, Hogan BL. Evidence That Mouse Bmp8a (Op2) and Bmp8b are Duplicated Genes That Play a Role in Spermatogenesis and Placental Development. Mech Dev (1996) 57:159–68. doi: 10.1016/0925-4773(96)00543-6
146. Zhao GQ, Chen YX, Liu XM, Xu Z, Qi X. Mutation in Bmp7 Exacerbates the Phenotype of Bmp8a Mutants in Spermatogenesis and Epididymis. Dev Biol (2001) 240:212–22. doi: 10.1006/dbio.2001.0448
147. Zhao GQ, Deng K, Labosky PA, Liaw L, Hogan BL. The Gene Encoding Bone Morphogenetic Protein 8B is Required for the Initiation and Maintenance of Spermatogenesis in the Mouse. Genes Dev (1996) 10:1657–69. doi: 10.1101/gad.10.13.1657
148. Zhao GQ, Liaw L, Hogan BL. Bone Morphogenetic Protein 8A Plays a Role in the Maintenance of Spermatogenesis and the Integrity of the Epididymis. Development (1998) 125:1103–12. doi: 10.1242/dev.125.6.1103
149. Pradhan BS, Bhattacharya I, Sarkar R, Majumdar S. Downregulation of Sostdc1 in Testicular Sertoli Cells is Prerequisite for Onset of Robust Spermatogenesis at Puberty. Sci Rep (2019) 9:1–11. doi: 10.1038/s41598-019-47930-x
150. Marino G, Zanghi A. Activins and Inhibins: Expression and Role in Normal and Pathological Canine Reproductive Organs: A Review. Anat Histol Embryol (2013) 42:1–8. doi: 10.1111/j.1439-0264.2012.01161.x
151. Namwanje M, Brown CW. Activins and Inhibins: Roles in Development, Physiology, and Disease. Cold Spring Harb Perspect Biol (2016) 8:a021881. doi: 10.1101/cshperspect.a021881
152. Ying SY. Inhibins, Activins and Follistatins. J Steroid Biochem (1989) 33:705–13. doi: 10.1016/0022-4731(89)90481-0
153. de Kretser DM, Loveland KL, Meehan T, O’Bryan MK, Phillips DJ, Wreford NG. Inhibins, Activins and Follistatin: Actions on the Testis. Mol Cell Endocrinol (2001) 180:87–92. doi: 10.1016/S0303-7207(01)00502-0
154. Vale W, Wiater E, Gray P, Harrison C, Bilezikjian L, Choe SY. Understanding and Optimizing Human Development: From Cells to Patients to Populations. Proceedings of the National Institute of Child Health and Human Development 40th Anniversary Scientific Symposium, September 8, 2003, Bethesda, Maryland, USA. Ann N Y Acad Sci (2004) 1038:1–234. doi: 10.1196/annals.1315.023
155. Raverdeau M, Gely-Pernot A, Feret B, Dennefeld C, Benoit G, Davidson I, et al. Retinoic Acid Induces Sertoli Cell Paracrine Signals for Spermatogonia Differentiation But Cell Autonomously Drives Spermatocyte Meiosis. Proc Natl Acad Sci USA (2012) 109:16582–7. doi: 10.1073/pnas.1214936109
156. Khanehzad M, Abbaszadeh R, Holakuyee M, Modarressi MH, Nourashrafeddin SM. FSH Regulates RA Signaling to Commit Spermatogonia Into Differentiation Pathway and Meiosis. Reprod Biol Endocrinol (2021) 19:4. doi: 10.1186/s12958-020-00686-w
Keywords: Sertoli cells, fertility, sex hormone, spermatogenesis, testis
Citation: Shah W, Khan R, Shah B, Khan A, Dil S, Liu W, Wen J and Jiang X (2021) The Molecular Mechanism of Sex Hormones on Sertoli Cell Development and Proliferation. Front. Endocrinol. 12:648141. doi: 10.3389/fendo.2021.648141
Received: 31 December 2020; Accepted: 17 May 2021;
Published: 23 July 2021.
Edited by:
Tatjana S. Kostic, University of Novi Sad, SerbiaReviewed by:
Bhola Shankar Pradhan, Łukasiewicz Research Network - PORT, The Polish Center for Technology Development, PolandIndrashis Bhattacharya, Hemwati Nandan Bahuguna Garhwal University, India
Copyright © 2021 Shah, Khan, Shah, Khan, Dil, Liu, Wen and Jiang. This is an open-access article distributed under the terms of the Creative Commons Attribution License (CC BY). The use, distribution or reproduction in other forums is permitted, provided the original author(s) and the copyright owner(s) are credited and that the original publication in this journal is cited, in accordance with accepted academic practice. No use, distribution or reproduction is permitted which does not comply with these terms.
*Correspondence: Xiaohua Jiang, YmlvanhoQHVzdGMuZWR1LmNu; Ranjha Khan, cmFuamhhQHVzdGMuZWR1LmNu; Jie Wen, amlld2VuQHVzdGMuZWR1LmNu
†These authors have contributed equally to this work