- 1School of Basic Medical Sciences, Henan University, Kaifeng, China
- 2Henan International Joint Laboratory for Nuclear Protein Regulation, Henan University, Kaifeng, China
- 3Department of Biological Sciences, Faculty of Science, Dar es Salaam University College of Education, Dar es Salaam, Tanzania
- 4Kaifeng Key Laboratory of Infection and Biological Safety, School of Basic Medical Sciences, Henan University, Kaifeng, China
- 5School of Stomatology, Henan University, Kaifeng, China
Hydrogen sulfide (H2S), as one of the three known gaseous signal transduction molecules in organisms, has attracted a surging amount of attention. H2S is involved in a variety of physiological and pathological processes in the body, such as dilating blood vessels (regulating blood pressure), protecting tissue from ischemia-reperfusion injury, anti-inflammation, carcinogenesis, or inhibition of cancer, as well as acting on the hypothalamus and pancreas to regulate hormonal metabolism. The change of H2S concentration is related to a variety of endocrine disorders, and the change of hormone concentration also affects the synthesis of H2S. Understanding the effect of biosynthesis and the concentration of H2S on the endocrine system is useful to develop drugs for the treatment of hypertension, diabetes, and other diseases.
Introduction
It was not until the 1990s when endogenous and relatively high concentrations of hydrogen sulfide (H2S) were found in the brains of mice, humans, and cattle (1–3), that people’s inherent perception of H2S as a toxic gas changed. Exogenous H2S is a strong neurotoxin with a sturdy stimulating effect on mucosa. Inhalation of a small amount of high concentration of H2S can be fatal in a short time. A low concentration of H2S can affect the eyes, respiratory system, and central nervous system. With the progress of the research, more and more functions of endogenous H2S have been clarified, including vasodilation and angiogenesis (4, 5), cell death (6), intracellular signal transduction (7), inflammatory regulation (8), and mitochondrial energetics (9). Abnormal synthesis and decomposition of H2S can lead to a variety of diseases, including but not limited to hypertension, obesity, diabetes, arteriosclerosis, and cancer.
The endocrine syndrome occurs when the secretion and/or structure of the endocrine gland or endocrine tissue itself is abnormal. It also includes the syndromes of abnormal hormone sources, abnormal hormone receptors, and physiological disorders caused by the abnormal metabolism of hormones or substances. In the endocrine system, H2S can act on the thyroid (10), adrenal gland (11), and gonad (12) through the hypothalamus-pituitary axis, as well as on the pancreas (13), thereby participating in the regulation of many hormones in the body, and the hypothalamus-pituitary axis can, in turn, regulate the production of H2S (14).
The purpose of this paper is to discuss the effect of H2S on the endocrine system, hoping to have a better understanding of this new gas signal transduction molecule.
Synthesis and Metabolism of H2S in the Endocrine System
At present, three main known enzymes produce H2S in organisms: cystathionine β-synthase (CBS), cystathionine γ-lyase (CSE), and 3-mercaptopyruvate sulfurtransferase (3-MST) (15). The substrate of CBS and CSE is L-cysteine (16), while 3-MST can catalyze 3-mercaptopyruvate to produce H2S (17, 18). Recently, it has been discovered that a kind of human methanethiol oxidase-selenium binding protein 1 (SELENBP1) can convert methanethiol into H202, formaldehyde, and H2S. In adipocytes, H2S can be produced by SELENBP1 and is related to the expression of CBS, CSE, and 3-MST (19–21). But the amount of the enzyme that produces H2S varies in different tissues and organs. In endocrine glands and endocrine organs, the RNA and protein expression levels of CBS are the highest in the pancreas, especially in acinar cells (22). Moreover, the CBS is rarely distributed in other endocrine glands such as thyroid, parathyroid, adrenal gland, and pituitary gland (23); however, abnormally increased in thyroid carcinoma (10). The expression of CSE is the most abundant in the liver, but low in the thyroid, pancreas, testis, ovary, and other endocrine glands (24–26).3-MST is highly expressed in endocrine tissues (thyroid, parathyroid, adrenal), pancreas, gonad (testis, ovary) (27). H2S can affect the secretion of many hormones and participate in the occurrence and development of endocrine diseases (11), but the effect of H2S on the body may be biphasic (15), that is, the effect of too high or too low concentration is the opposite, so to ensure the normal physiological function of the body, it is necessary to maintain the concentration of H2S at an appropriate concentration. It is well known that H2S can be regulated in two ways: synthesis and consumption. In terms of synthesis, H2S is synthesized mainly through enzymatic and non-enzymatic pathways (such as reduction of sulfur-containing compounds) and, in a few cases, released by bound sulfur stored in cells (28). In the endocrine system, H2S is mainly produced by three enzymes that catalyze different substrates. In terms of consumption, H2S can remove protons from mitochondria and rapidly oxidize to thiosulfate, which is then converted to sulfite and eventually oxidized to sulfate (28). H2S can also accept the methyl of thiol-S-methyltransferase to constitute dimethylsulfide and methanethiol. Or metabolized by thiohemoglobin, a complex formed by H2S and methemoglobin (29). The half-life of H2S in vivo is very short (a few seconds to a few minutes) (30, 31). Nowadays, it mostly inhibits the activity of synthase or increases the donor in vitro, which brings great difficulties to the study of H2S.
Biological Effects of H2S in the Endocrine System
H2S can protect endocrine organs and regulate hormone secretion through anti-oxidative stress and ion channel regulation.
Antioxidant Stress
H2S protects cells from oxidative stress in two ways: (1) directly scavenging reactive oxygen species (ROS) and (2) up-regulating antioxidant defense. Excessive ROS can cause oxidative stress, resulting in DNA damage, protein misfolding, organelle lesion, and neuronal synaptic dysfunction (32). The main substances that produce ROS in cells are peroxide and nitric oxide (NO) (33). In the heart, H2S reduces lipid peroxidation by scavenging peroxides; directly scavenges nitrite peroxides in neuroblasts, and binds to NO-free radicals to reduce oxidative stress (34–36). H2S up-regulates antioxidant defense mainly by activating the nuclear-factor-E2-related factor-2 (Nrf2) pathway and increasing the amount of glutathione (GSH). Nrf2 is an antioxidant regulator which regulates the gene expression of a variety of enzymes used to reduce oxidative stress (37). GSH is the main substance of antioxidant stress in cells, and H2S can distribute GSH into mitochondria. The ROS produced in mitochondria can be scavenged by GSH (38).
Regulated Ion Channels
KATP channel is the first ion channel target of H2S (4), and many reported physiological effects of H2S are mediated by this channel: (1) vasodilation induced by H2S depends on its opening of KATP channel in vascular smooth muscle cells; (2) H2S opens KATP channel in rat atrial and ventricular myocytes; (3) electrophysiological studies have shown that NaHS increases KATP channel currents in rat aortic and smooth muscle cells. (4) the opening of the KATP channel also supports the relaxing effect of H2S on colonic and ocular smooth muscle (39–44). The Ca2+ channel is another ion channel regulated by H2S, which is elucidated in many types of cells, including nerve cells, cardiomyocytes, and endothelial cells. It is involved in cardiac contraction, angiogenesis, inflammation, and sensory transmission, and its effect may be related to the source of Ca2+ (extracellular or endoplasmic reticulum) (45–47). We will discuss the effects of the KATP channel and Ca2+ channel on insulin secretion below.
The Mechanism of H2S Participating in the Physiological Process of the Endocrine System
H2S plays a role in the endocrine system to (1) protect pancreatic cells, regulate insulin secretion, (2) maintain the function of the adrenal cortex, promote the release of catecholamine, (3) maintain reproduction, and (4) regulate the secretion of pituitary hormones and are regulated by hormones (Figure 1).
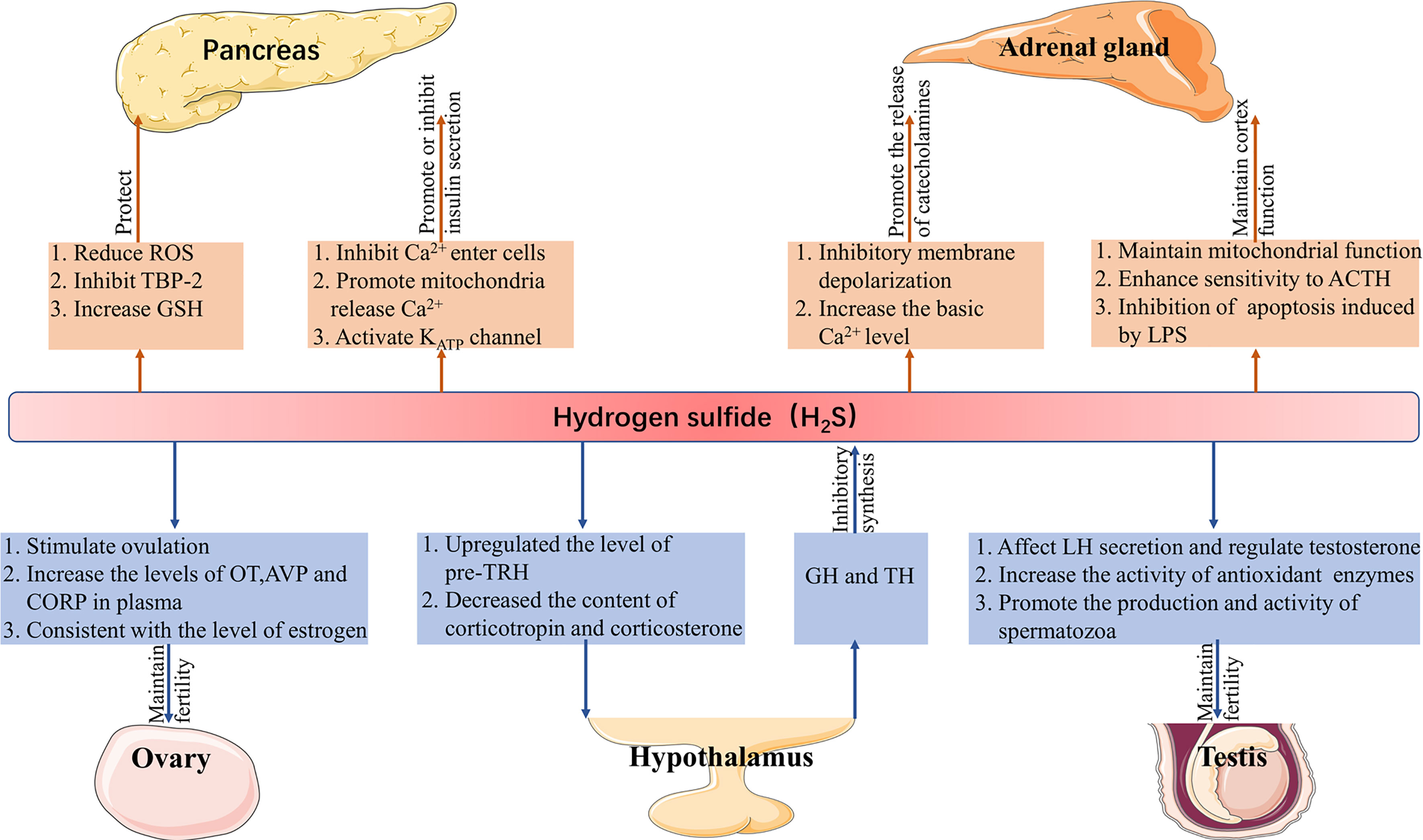
Figure 1 Function of H2S in the endocrine system. H2S, hydrogen sulfide; ROS, reactive oxygen species; TBP-2, thioredoxin binding protein-2; GSH, glutathione; ACTH, adrenocorticotropic hormone; LPS, lipopolysaccharide; LH, luteinizing hormone; pre-TRH, pre-thyrotropin-releasing hormone; GH, growth hormone; TH, thyroid hormone; OT, oxytocin; AVP, vasopressin; CORT, corticosterone.
H2S in the Pancreas
H2S in the pancreas was first found in mice, and CBS and CSE were subsequently detected in these tissues (48, 49). The main roles of H2S in the pancreas are protecting pancreatic β cells and regulating insulin secretion.H2S may protect pancreatic β cells in the following three ways: (1) reduce the production of ROS; (2) inhibit the expression of thioredoxin binding protein-2-a redox protein associated with diabetes that promotes apoptosis; and (3) increase the content of GSH, all of which reduce the damage of oxidative stress (50–53). On the contrary, a high concentration of H2S induces apoptosis of pancreatic β cells (54). Insulin secretion is affected by many factors. It is known that the concentration and oscillation of Ca2+, KATP channel is related to H2S. H2S can not only inhibit the entry of Ca2+ from the plasma membrane into cells, to reduce insulin secretion, but also promote the release of Ca2+ in mitochondria and increase insulin secretion (55). It is well known that a high concentration of glucose can promote insulin secretion. In rat insulinoma cell line INS-1E, a high concentration of glucose (16mM) not only inhibits the activity of the KATP channel but also reduces the concentration of H2S, meanwhile H2S supplementation can activate the KATP channel (56). Since the activity of the KATP channel is also negatively correlated with insulin secretion (57). Therefore, it is unequivocal H2S can induce its effects on insulin secretion by utilizing this channel.
The Role of H2S in the Adrenal Gland
In the adrenal gland, H2S can act on the adrenal cortex and chromaffin cells, respectively. For the adrenal cortex, inhibition of CBS/CSE can result in mitochondrial oxidative stress and dysfunction, while corticosterone response to the adrenocorticotropic hormone is weakened, which is reversed by morpholin-4-ium-methoxyphenyl-morpholino- phosphinodithioate (GYY4137, an H2S donor). Lipopolysaccharide (LPS) can lead to adrenal dysfunction induced by mitochondrial injury, and LPS inhibits the expression of CBS/CSE and the production of H2S in the adrenal gland, subsequent addition of GYY4137 inhibits apoptosis induced by LPS (58, 59). Calcium signaling plays a crucial role in the release of catecholamines (60). In mammals (mice and rats), the expression of CSE in chromaffin cells increased the oxygen sensitivity of the carotid body (61). Moreover, it has been proven that H2S can inhibit the depolarization of the membrane caused by IK+Ca and induce the release of catecholamines in rat chromaffin cells (62). In calve chromaffin cells, the basal Ca2+ level increased in the presence of NaHS (H2S donor), thus promoting exocytosis (63). In fish (rainbow trout), electrical stimulation and acute hypoxia-induced increased secretion of catecholamines and H2S, followed by the addition of amino-oxyacetic acid (AOAA, CBS inhibitor), which significantly inhibited the production of H2S, while the effect of DL-Propargylglycine (PAG, CSE inhibitor) was not obvious (64).
The Function of H2S in Gonads
The gonads mainly cover the testicles of men and the ovaries of women. Testes can secrete the male hormone testosterone, its main function is to promote the development of gonad and its accessory structure and the appearance of parasexual characteristics, as well as to promote protein synthesis (65). The expression of CBS and CSE are found in rat testes, but they are differentially expressed; CSE is mainly expressed in Sertoli cells and immature spermatogonia, while CBS is mainly distributed in Leydig cells, Sertoli cells, and germ cells (66). Sulfides in garlic regulate the secretion of testosterone by affecting the secretion of luteinizing hormone (LH), indicating that H2S may play an important role in the secretion of testosterone (67). Similarly, in rat testis, the provision of NaHS protected the testis from oxidative stress and inflammation induced by cisplatin and increased the activity of antioxidant enzymes (68). Subsequently, it has been found that the expression of CBS decreases in the spermatozoa of human asthenospermic patients, and AOAA inhibited the sperm motility, then the addition of GYY4137 weakens the effect of AOAA, suggesting that H2S could promote the production and activity of spermatozoa (69).
The decreased fertility of female offspring of CBS knockout mice suggests that H2S may have a greater effect on female fertility (70). In different mammals, H2S regulates female reproduction in many ways such as; the LH peak before ovulation in mice increases with the expression of H2S producing enzymes, the inhibition of CSE by hydroxylamine hydrochloride prevents ovulation, whereas, the subsequent addition of NaHS reverses the effect of the inhibitor, Na2S, an H2S donor, increases the plasma level of oxytocin, vasopressin, and corticosterone in rats; CBS increased the level of H2S in the uterine artery during sheep production, and the change of CBS protein expression was consistent with the estrous cycle and follicular estrogen level of sheep, so as the estrogen level of the human menstrual cycle (12, 71, 72).
Interaction Between H2S and Hypothalamus-Pituitary Axis
Abundant CSE and CBS were found in the hypothalamus (73). In the paraventricular nucleus (PVN), the high expression of CBS upregulates the level of pre-thyrotropin-releasing hormone (pre-TRH) and decreases the content of corticotropin and corticosterone in blood (74). Interestingly, in hypothalamic explants, NaHS did not affect the physiological secretion of corticotropin-releasing hormone (CRH) but could inhibit the release of CRH stimulated by KCl (75). The results show that the hormones produced by the hypothalamus-pituitary axis also affect the synthesis of H2S. Thyroid hormone (TH) and growth hormone (GH) regulates the production of H2S in the liver through TH receptor β1 and GH receptor, respectively. In mechanism, TH inhibits the expression of CSE, while GH inhibits the production of H2S through substrate availability control by autophagy (14). However, another study showed that in hyperthyroidism rats, the level of H2S in the liver increased (76), which may be due to the different effects of different concentrations of TH on the H2S producing enzyme.
Endocrine Diseases Involved in H2S
H2S can regulate glucose and fat metabolism through the pancreas, liver, adipose tissue, and skeletal muscle. In human thyroid and ovarian cancer, H2S appears to have a dual effect, which may be related to its concentration (or metabolic rate) in the cells. High concentrations of H2S can aggravate pancreatitis and induced lung injury, but protect osteoblasts, making it a potential drug for the prevention of osteoporosis.
H2S and Type 2 Diabetes
Pancreas, liver, adipose tissue, and skeletal muscle are involved in the regulation of plasma glucose, the enzyme-producing H2S is expressed in all the above tissues (48, 77–79). H2S can manage plasma glucose levels by protecting pancreatic β cells and regulating insulin secretion (see section 4.1). H2S also seems to have the opposite effect on liver glucose uptake, glycogen storage, and gluconeogenesis. One point of view is that H2S promotes liver glycogenesis: in hepatocellular carcinoma cell line HepG2, NaHS inhibits the activity of glucokinase, and CSE overexpression reduces glycogen content, while CSE knockout increases glycogen content (80, 81). Another point of view is that H2S inhibits gluconeogenesis in the liver: the lack of CSE promotes gluconeogenesis in the liver, knockout CSE of HepG2 increases glucose production, while the addition of NaHS weakens the knockout effect of CSE (82). The role of H2S in regulating plasma glucose through adipose tissue also seems to be twofold. On the one hand, H2S increases glucose uptake and fat production in adipocytes, for example: (1) in 3T3L1 adipocytes, H2S improves high glucose-induced insulin resistance by up-regulating phosphatidylinositol 3,4,5-trisphosphate level, (2) in diabetic insulin resistance rats, NaHS and H2S gas solution improve insulin resistance by activating insulin receptors (IR), (3) CSE/H2S converts glucose into triglycerides in adipocytes through peroxisome proliferator-activated receptor γ (PPARγ) and stores them in cells, (4) H2S promotes adipogenesis in 3T3L1 cells by increasing the expression of fatty acid protein 4 (83–86). On the other hand, H2S plays a vital role in adipocyte insulin resistance mediated by tumor necrosis factor-α (TNF-α) (87). H2S improved insulin resistance of skeletal muscle by increasing the sensitivity of the IR-PI3K-Akt signal pathway. In mouse myoblast C2C12, the addition of NaHS increased glucose uptake (84, 88). The above results show that H2S reduces the level of plasma glucose by regulating numerous cellular markers.
Effect of H2S on Thyroid Cancer
The effect of H2S on cancer is related to its concentration, that is, low concentration (or low production rate) and high concentration (or rapid production rate) can show opposite results (15).In thyroid cancer, two different H2S donors showed different results. Diallyl trisulfide (DATS), an H2S donor from garlic, has an inhibitory effect on both anaplastic and papillary thyroid cancer. In anaplastic cancer cell line 8505C, DATS induces the accumulation of ROS, which inhibits cell survival and increases apoptosis. In addition, it promotes cell DNA damage and arrests the cell cycle in the G2/M phase (89). In papillary carcinoma cell line KTC-1, treatment with diallyl trisulfide (DATS) activates NK-κB signaling pathway and increases the expression of NF-κB-dependent CSE, while H2S induced cell growth inhibition (90). While in another thyroid papillary carcinoma cell line BCPAP, DATS blocks the cell cycle in G0/G1 phase and promotes apoptosis of thyroid cancer cells by inducing mitochondrial apoptosis and activating the MAPK signal pathway (91). When NaHS is used as an H2S donor, a low concentration (≤50uM) of NaHS promotes the proliferation, migration, and invasion of thyroid cancer cells in vitro, while a high concentration (200uM) has the opposite result. In BALB/C nude mice with axillary tumorigenesis, the tumor mass increases, the doubling time shortens and the inhibition rate decreases in low concentration (≤2.8mg/kg/day) of NaHS, while in high concentration (≥5.6 mg/kg/day) the effect is reversed (92).
H2S Promotes Acute Pancreatitis and Its Induced Lung Injury
In rats and mice with acute pancreatitis (AP), it has been found that the concentration of serum H2S is increased and the expressions of CSE and TNF-α are also increased in the lungs. The pro-inflammatory effect of H2S may be related to the release of substance P (SP) and the activation of trypsinogen, while the release of SP is mediated by neurokinin-1 receptor (NK-1R) in pancreatic acini (48, 93, 94). In addition, the number of autophagosomes and autophagy vacuoles increases in AP, which is positively correlated with the level of H2S, which associates with the activation of the AMPK/mTOR signal pathway (95). The addition of PAG decreases the activity of peroxidase in the lung and pancreas, the expression of TNF-α, CSE, and NK-1R, and the concentration of H2S in serum (93, 94, 96). Diallyl disulfide in garlic can also trigger an anti-inflammatory effect in the pancreas and lung by reducing the expression of TNF-α, CSE, NK-1R, and the production of H2S (97). To sum up, H2S can aggravate AP and its induced lung injury in many ways, and inhibiting the production of H2S may be a potential method for the treatment of AP (Figure 2).
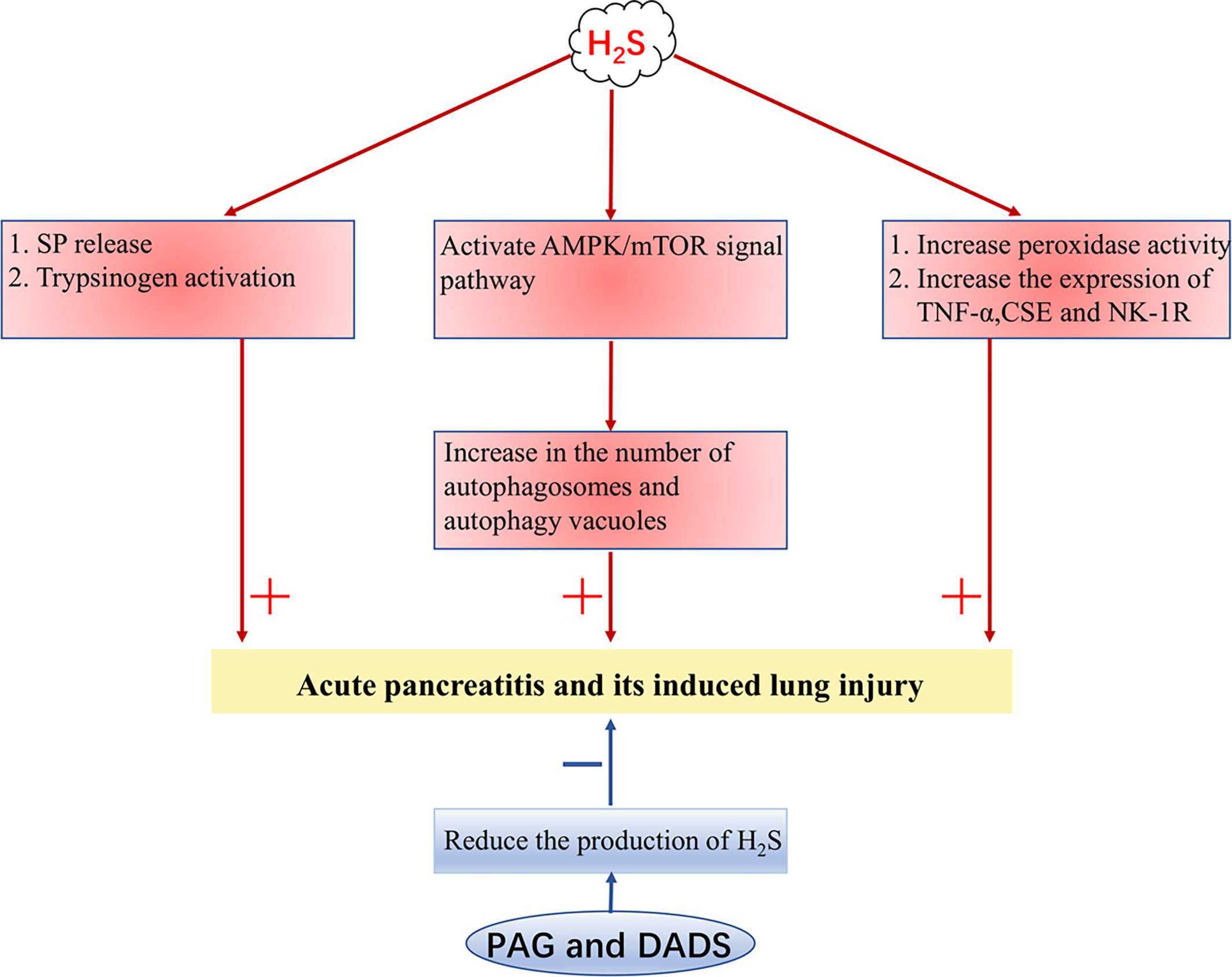
Figure 2 H2S aggravates acute pancreatitis. H2S, hydrogen sulfide; SP, substance P; TNF-α, tumor necrosis factor-α; NK-1R, neurokinin-1 receptor; CSE, cystathionine γ-lyase; PAG, DL-Propargylglycine; DADS, diallyl disulfide. + indicates aggravate, - indicates lighten.
H2S and Obesity
H2S can increase or decrease fat synthesis in many ways. It is described previously by regulating insulin sensitivity in adipocytes. The expression of H2S in different tissues can jointly regulate the formation of fat. Overexpression of CBS in PVN reduces obesity and insulin resistance induced by a high-fat diet (HFD). Mechanistically, leptin up-regulates CBS expression through FOXO3a, promotes pre-TRH production by inhibiting the mTOR pathway, and then increases TH production and decreases corticosterone production, thus reducing fat deposition and improving insulin resistance induced by FHD (74). However, in mouse adipocytes, H2S promotes adipogenesis by activating PPARγ, where when CSE is deficient, mice show resistance to FDH-induced obesity (98). Perivascular adipose tissue provides protection and support for blood vessels. In obese patients, the secretion of pro-inflammatory macrophages increases, resulting in increased consumption of H2S in vascular endothelium and smooth muscle, which directly damages endothelium-dependent vasodilation, and results in a loss of pulmonary vein function (99).
Uncertainty of the Effect of H2S on Ovarian Cancer
The expression of CBS is increased in ovarian cancer cell line A2780. After down-regulation of CBS in ovarian cancer cell line A2780, the content of GSH decreased, which aggravated the cascade of apoptosis triggered by oxidative stress and enhanced the sensitivity to cisplatin. In addition, mitochondrial function is inhibited and ATP synthesis decreases after down-regulation of CBS (100). Moreover, selenium-containing chrysin, as a compound with anticancer and antioxidant effects, shows an antitumor effect in many ovarian cancer cell lines, and its inhibitory effect is achieved by inhibiting the expression of CBS and promoting the transformation of GSH (101). However, another data shows that GYY4137 promotes the release of Ca2+ from the endoplasmic reticulum of A2780, induces endoplasmic reticulum stress, and drives apoptosis, while the content of GSH is decreased (102).
H2S Inhibits the Development of Osteoporosis
Osteoporosis occurs in postmenopausal women and can also occur in patients with specific hormone secretion disorders or patients treated with glucocorticoid drugs. Endogenous H2S protects osteoblasts MC3T3-E1 from cytotoxicity induced by ROS. NaHS safeguards osteoblasts from oxidative stress-induced cell injury and inhibition of proliferation and differentiation through the MAPK signal pathway (103). Therefore, H2S may be used as a drug to prevent osteoporosis.
Conclusions
The hypothalamic-pituitary-target organ axis is a complex biological structure, which plays an important role in endocrine regulation. H2S is distributed in multiple sites in the hypothalamus, pituitary-target organ, making it an important molecule in regulating hormone secretion, which is not only dual but also bi-directional, that is, H2S not only promotes the secretion of certain hormones but can also inhibit them. The complex effect of H2S on the endocrine system may be caused by its action on different organs. Current studies mainly focus on the effect of H2S on a single organ, the role of feedback regulation is unclear, which brings a difficult problem to explore the mechanism of action of H2S in the endocrine system.
There’s a growing body of evidence that H2S plays an important role in type 2 diabetes, but its effect on insulin secretion and insulin target organs has not been consensus, which may be related to its concentration and production rate in the target organs. Similarly, H2S is also involved in the occurrence and development of endocrine organ tumors, and its role in thyroid cancer is related to its concentration. Since the exact concentration of H2S in cells is difficult to detect, the effect of a specific concentration of H2S on endocrine organs remains to be further explained.
Although there are still many problems to be solved, existing studies have shown that H2S plays a key role in maintaining the function of endocrine organs and hormone secretion. Therefore, the regulation of H2S production may be a potential treatment for endocrine diseases.
Author Contributions
X-YJ and D-DW contributed to conception and design of the study. H-JC looked at the data and wrote the first draft. EN, LQ, TL, Y-ZQ, J-JZ, and KL wrote sections of the manuscript. D-DW and EN reviewed and revised the first draft. All authors contributed to the article and approved the submitted version.
Funding
This work was supported by grants from the National Natural Science Foundation of China (Nos. 81802718, 81670088, U1504817), the Foundation of Science & Technology Department of Henan Province, China (Nos.202102310480, 182102310335, 192102310151), the Training Program for Young Backbone Teachers of Institutions of Higher Learning in Henan Province, China (No. 2020GGJS038), and the Science Foundation for Young Talents of Henan University College of Medicine, China (No. 2019013).
Conflict of Interest
The authors declare that the research was conducted in the absence of any commercial or financial relationships that could be construed as a potential conflict of interest.
References
1. Warenycia MW, Goodwin LR, Benishin CG, Reiffenstein RJ, Francom DM, Taylor JD, et al. Acute Hydrogen Sulfide Poisoning. Demonstration of Selective Uptake of Sulfide by the Brainstem by Measurement of Brain Sulfide Levels. Biochem Pharmacol (1989) 38(6):973–81. doi: 10.1016/0006-2952(89)90288-8
2. Savage JC, Gould DH. Determination of Sulfide in Brain Tissue and Rumen Fluid by Ion-Interaction Reversed-Phase High-Performance Liquid Chromatography. J Chromatogr (1990) 526(2):540–5. doi: 10.1016/S0378-4347(00)82537-2
3. Goodwin LR, Francom D, Dieken FP, Taylor JD, Warenycia MW, Reiffenstein RJ, et al. Determination of Sulfide in Brain Tissue by Gas Dialysis/Ion Chromatography: Postmortem Studies and Two Case Reports. J Anal Toxicol (1989) 13(2):105–9. doi: 10.1093/jat/13.2.105
4. Zhao W, Zhang J, Lu Y, Wang R. The Vasorelaxant Effect of H(2)S as a Novel Endogenous Gaseous K(ATP) Channel Opener. EMBO J (2001) 20(21):6008–16. doi: 10.1093/emboj/20.21.6008
5. Cai WJ, Wang MJ, Moore PK, Jin HM, Yao T, Zhu YC. The Novel Proangiogenic Effect of Hydrogen Sulfide is Dependent on Akt Phosphorylation. Cardiovasc Res (2007) 76(1):29–40. doi: 10.1016/j.cardiores.2007.05.026
6. Kolluru GK, Shen X, Bir SC, Kevil CG. Hydrogen Sulfide Chemical Biology: Pathophysiological Roles and Detection. Nitric Oxide (2013) 35:5–20. doi: 10.1016/j.niox.2013.07.002
7. Wang R. Physiological Implications of Hydrogen Sulfide: A Whiff Exploration That Blossomed. Physiol Rev (2012) 92(2):791–896. doi: 10.1152/physrev.00017.2011
8. Zanardo RC, Brancaleone V, Distrutti E, Fiorucci S, Cirino G, Wallace JL. Hydrogen Sulfide is an Endogenous Modulator of Leukocyte-Mediated Inflammation. FASEB J (2006) 20(12):2118–20. doi: 10.1096/fj.06-6270fje
9. Szabo C, Ransy C, Módis K, Andriamihaja M, Murghes B, Coletta C, et al. Regulation of Mitochondrial Bioenergetic Function by Hydrogen Sulfide. Part I. Biochemical and Physiological Mechanisms. Br J Pharmacol (2014) 171(8):2099–122. doi: 10.1111/bph.12369
10. Turbat-Herrera EA, Kilpatrick MJ, Chen J, Meram AT, Cotelingam J, Ghali G, et al. Cystathione β-Synthase Is Increased in Thyroid Malignancies. Anticancer Res (2018) 38(11):6085–90. doi: 10.21873/anticanres.12958
11. Zhu X, Gu H, Ni X. Hydrogen Sulfide in the Endocrine and Reproductive Systems. Expert Rev Clin Pharmacol (2011) 4(1):75–82. doi: 10.1586/ecp.10.125
12. Estienne A, Portela VM, Choi Y, Zamberlam G, Boerboom D, Roussel V, et al. The Endogenous Hydrogen Sulfide Generating System Regulates Ovulation. Free Radic Biol Med (2019) 138:43–52. doi: 10.1016/j.freeradbiomed.2019.03.028
13. Kaneko Y, Kimura Y, Kimura H, Niki I. L-Cysteine Inhibits Insulin Release From the Pancreatic Beta-Cell: Possible Involvement of Metabolic Production of Hydrogen Sulfide, a Novel Gasotransmitter. Diabetes (2006) 55(5):1391–7. doi: 10.2337/db05-1082
14. Hine C, Kim HJ, Zhu Y, Harputlugil E, Longchamp A, Matos MS, et al. Hypothalamic-Pituitary Axis Regulates Hydrogen Sulfide Production. Cell Metab (2017) 25(6):1320–1333.e5. doi: 10.1016/j.cmet.2017.05.003
15. Hellmich MR, Szabo C. Hydrogen Sulfide and Cancer. Handb Exp Pharmacol (2015) 230:233–41. doi: 10.1007/978-3-319-18144-8_12
16. Stipanuk M, Beck P. Characterization of the Enzymic Capacity for Cysteine Desulphhydration in Liver and Kidney of the Rat. Biochem J (1982) 206(2):267–77. doi: 10.1042/bj2060267
17. Shibuya N, Koike S, Tanaka M, Ishigami-Yuasa M, Kimura Y, Ogasawara Y, et al. A Novel Pathway for the Production of Hydrogen Sulfide From D-Cysteine in Mammalian Cells. Nat Commun (2013) 4:1366. doi: 10.1038/ncomms2371
18. Shibuya N, Tanaka M, Yoshida M, Ogasawara Y, Togawa T, Ishii K, et al. 3-Mercaptopyruvate Sulfurtransferase Produces Hydrogen Sulfide and Bound Sulfane Sulfur in the Brain. Antioxidants Redox Signaling (2009) 11(4):703–14. doi: 10.1089/ars.2008.2253
19. Pol A, Renkema GH, Tangerman A, Winkel EG, Engelke UF, de Brouwer APM, et al. Mutations in SELENBP1, Encoding a Novel Human Methanethiol Oxidase, Cause Extraoral Halitosis. Nat Genet (2018) 50(1):120–9. doi: 10.1038/s41588-017-0006-7
20. Elhodaky M, Hong LK, Kadkol S, Diamond AM. Selenium-Binding Protein 1 Alters Energy Metabolism in Prostate Cancer Cells. Prostate (2020) 80(12):962–76. doi: 10.1002/pros.24028
21. Randi EB, Casili G, Jacquemai S, Szabo C. Selenium-Binding Protein 1 (SELENBP1) Supports Hydrogen Sulfide Biosynthesis and Adipogenesis. Antioxidants (Basel) (2021) 10(3):361. doi: 10.3390/antiox10030361
22. Bao L, Vlcek C, Paces V, Kraus JP. Identification and Tissue Distribution of Human Cystathionine Beta-Synthase mRNA Isoforms. Arch Biochem Biophys (1998) 350(1):95–103. doi: 10.1006/abbi.1997.0486
23. Uhlén M, Fagerberg L, Hallström BM, Lindskog C, Oksvold P, Mardinoglu A, et al. Proteomics. Tissue-Based Map of the Human Proteome. Science (2015) 347(6220):1260419. doi: 10.1126/science.1260419
24. Mustafa AK, Gadalla MM, Sen N, Kim S, Mu W, Gazi SK, et al. H2S Signals Through Protein S-Sulfhydration. Sci Signal (2009) 2(96):ra72. doi: 10.1126/scisignal.2000464
25. Yang G, Wu L, Jiang B, Yang W, Qi J, Cao K, et al. H2S as a Physiologic Vasorelaxant: Hypertension in Mice With Deletion of Cystathionine Gamma-Lyase. Science (2008) 322(5901):587–90. doi: 10.1126/science.1162667
26. Bos EM, Wang R, Snijder PM, Boersema M, Damman J, Fu M, et al. Cystathionine γ-Lyase Protects Against Renal Ischemia/Reperfusion by Modulating Oxidative Stress. J Am Soc Nephrol (2013) 24(5):759–70. doi: 10.1681/ASN.2012030268
27. Nagahara N. Multiple Role of 3-Mercaptopyruvate Sulfurtransferase: Antioxidative Function, H S and Polysulfide Production and Possible SO Production. Br J Pharmacol (2018) 175(4):577–89. doi: 10.1111/bph.14100
28. Li L, Rose P, Moore P. Hydrogen Sulfide and Cell Signaling. Annu Rev Pharmacol Toxicol (2011) 51:169–87. doi: 10.1146/annurev-pharmtox-010510-100505
29. Polhemus D, Lefer D. Emergence of Hydrogen Sulfide as an Endogenous Gaseous Signaling Molecule in Cardiovascular Disease. Circ Res (2014) 114(4):730–7. doi: 10.1161/CIRCRESAHA.114.300505
30. Wang R. Two’s Company, Three’s a Crowd: Can H2S be the Third Endogenous Gaseous Transmitter? FASEB Journal: Off Publ Fed Am Societies Exp Biol (2002) 16(13):1792–8. doi: 10.1096/fj.02-0211hyp
31. Insko M, Deckwerth TL, Hill P, Toombs CF, Szabo C. Detection of Exhaled Hydrogen Sulphide Gas in Rats Exposed to Intravenous Sodium Sulphide. Br J Pharmacol (2009) 157(6):944–51. doi: 10.1111/j.1476-5381.2009.00248.x
32. Maiese K. New Insights for Oxidative Stress and Diabetes Mellitus. Oxid Med Cell Longev (2015) 2015:875961. doi: 10.1155/2015/875961
33. Bodega G, Alique M, Puebla L, Carracedo J, Ramírez RM. Microvesicles: ROS Scavengers and ROS Producers. J Extracell Vesicles (2019) 8(1):1626654. doi: 10.1080/20013078.2019.1626654
34. Geng B, Chang L, Pan C, Qi Y, Zhao J, Pang Y, et al. Endogenous Hydrogen Sulfide Regulation of Myocardial Injury Induced by Isoproterenol. Biochem Biophys Res Commun (2004) 318(3):756–63. doi: 10.1016/j.bbrc.2004.04.094
35. Whiteman M, Armstrong JS, Chu SH, Jia-Ling S, Wong BS, Cheung NS, et al. The Novel Neuromodulator Hydrogen Sulfide: An Endogenous Peroxynitrite ‘Scavenger’? J Neurochem (2004) 90(3):765–8. doi: 10.1111/j.1471-4159.2004.02617.x
36. Kimura Y, Goto Y, Kimura H. Hydrogen Sulfide Increases Glutathione Production and Suppresses Oxidative Stress in Mitochondria. Antioxid Redox Signal (2010) 12(1):1–13. doi: 10.1089/ars.2008.2282
37. Ma Q. Role of Nrf2 in Oxidative Stress and Toxicity. Annu Rev Pharmacol Toxicol (2013) 53:401–26. doi: 10.1146/annurev-pharmtox-011112-140320
38. Kimura Y, Kimura H. Hydrogen Sulfide Protects Neurons From Oxidative Stress. FASEB J (2004) 18(10):1165–7. doi: 10.1096/fj.04-1815fje
39. Cheng Y, Ndisang JF, Tang G, Cao K, Wang R. Hydrogen Sulfide-Induced Relaxation of Resistance Mesenteric Artery Beds of Rats. Am J Physiol Heart Circ Physiol (2004) 287(5):H2316–23. doi: 10.1152/ajpheart.00331.2004
40. Fiorucci S, Antonelli E, Mencarelli A, Orlandi S, Renga B, Rizzo G, et al. The Third Gas: H2S Regulates Perfusion Pressure in Both the Isolated and Perfused Normal Rat Liver and in Cirrhosis. Hepatology (2005) 42(3):539–48. doi: 10.1002/hep.20817
41. Zhong GZ, Li YB, Liu XL, Guo LS, Chen ML, Yang XC. Hydrogen Sulfide Opens the KATP Channel on Rat Atrial and Ventricular Myocytes. Cardiology (2010) 115(2):120–6. doi: 10.1159/000260073
42. Distrutti E, Sediari L, Mencarelli A, Renga B, Orlandi S, Antonelli E, et al. Evidence That Hydrogen Sulfide Exerts Antinociceptive Effects in the Gastrointestinal Tract by Activating KATP Channels. J Pharmacol Exp Ther (2006) 316(1):325–35. doi: 10.1124/jpet.105.091595
43. Monjok EM, Kulkarni KH, Kouamou G, McKoy M, Opere CA, Bongmba ON, et al. Inhibitory Action of Hydrogen Sulfide on Muscarinic Receptor-Induced Contraction of Isolated Porcine Irides. Exp Eye Res (2008) 87(6):612–6. doi: 10.1016/j.exer.2008.09.011
44. Kubo S, Doe I, Kurokawa Y, Kawabata A. Hydrogen Sulfide Causes Relaxation in Mouse Bronchial Smooth Muscle. J Pharmacol Sci (2007) 104(4):392–6. doi: 10.1254/jphs.SC0070199
45. Yong QC, Cheong JL, Hua F, Deng LW, Khoo YM, Lee HS, et al. Regulation of Heart Function by Endogenous Gaseous Mediators-Crosstalk Between Nitric Oxide and Hydrogen Sulfide. Antioxid Redox Signal (2011) 14(11):2081–91. doi: 10.1089/ars.2010.3572
46. Lipscombe D, Helton TD, Xu W. L-Type Calcium Channels: The Low Down. J Neurophysiol (2004) 92(5):2633–41. doi: 10.1152/jn.00486.2004
47. Éva Sikura K, Combi Z, Potor L, Szerafin T, Hendrik Z, Méhes G, et al. Hydrogen Sulfide Inhibits Aortic Valve Calcification in Heart via Regulating RUNX2 by NF-κb, a Link Between Inflammation and Mineralization. J Adv Res (2021) 27:165–76. doi: 10.1016/j.jare.2020.07.005
48. Bhatia M, Wong FL, Fu D, Lau HY, Moochhala SM, Moore PK. Role of Hydrogen Sulfide in Acute Pancreatitis and Associated Lung Injury. FASEB J (2005) 19(6):623–5. doi: 10.1096/fj.04-3023fje
49. Yusuf M, Kwong Huat BT, Hsu A, Whiteman M, Bhatia M, Moore PK. Streptozotocin-Induced Diabetes in the Rat is Associated With Enhanced Tissue Hydrogen Sulfide Biosynthesis. Biochem Biophys Res Commun (2005) 333(4):1146–52. doi: 10.1016/j.bbrc.2005.06.021
50. Kaneko Y, Kimura T, Taniguchi S, Souma M, Kojima Y, Kimura Y, et al. Glucose-Induced Production of Hydrogen Sulfide may Protect the Pancreatic Beta-Cells From Apoptotic Cell Death by High Glucose. FEBS Lett (2009) 583(2):377–82. doi: 10.1016/j.febslet.2008.12.026
51. Taniguchi S, Kang L, Kimura T, Niki I. Hydrogen Sulphide Protects Mouse Pancreatic β-Cells From Cell Death Induced by Oxidative Stress, But Not by Endoplasmic Reticulum Stress. Br J Pharmacol (2011) 162(5):1171–8. doi: 10.1111/j.1476-5381.2010.01119.x
52. Yoshihara E, Fujimoto S, Inagaki N, Okawa K, Masaki S, Yodoi J, et al. Disruption of TBP-2 Ameliorates Insulin Sensitivity and Secretion Without Affecting Obesity. Nat Commun (2010) 1:127. doi: 10.1038/ncomms1127
53. Saxena G, Chen J, Shalev A. Intracellular Shuttling and Mitochondrial Function of Thioredoxin-Interacting Protein. J Biol Chem (2010) 285(6):3997–4005. doi: 10.1074/jbc.M109.034421
54. Yang G, Yang W, Wu L, Wang R. H2S, Endoplasmic Reticulum Stress, and Apoptosis of Insulin-Secreting Beta Cells. J Biol Chem (2007) 282(22):16567–76. doi: 10.1074/jbc.M700605200
55. Lu A, Chu C, Mulvihill E, Wang R, Liang W. ATP-Sensitive K(+) Channels and Mitochondrial Permeability Transition Pore Mediate Effects of Hydrogen Sulfide on Cytosolic Ca(2+) Homeostasis and Insulin Secretion in β-Cells. Pflugers Arch (2019) 471(11-12):1551–64. doi: 10.1007/s00424-019-02325-9
56. Yang W, Yang G, Jia X, Wu L, Wang R. Activation of KATP Channels by H2S in Rat Insulin-Secreting Cells and the Underlying Mechanisms. J Physiol (2005) 569(Pt 2):519–31. doi: 10.1113/jphysiol.2005.097642
57. Ritzel RA, Hansen JB, Veldhuis JD, Butler PC. Induction of Beta-Cell Rest by a Kir6.2/SUR1-Selective K(ATP)-Channel Opener Preserves Beta-Cell Insulin Stores and Insulin Secretion in Human Islets Cultured at High (11 Mm) Glucose. J Clin Endocrinol Metab (2004) 89(2):795–805. doi: 10.1210/jc.2003-031120
58. Wang CN, Liu YJ, Duan GL, Zhao W, Li XH, Zhu XY, et al. CBS and CSE are Critical for Maintenance of Mitochondrial Function and Glucocorticoid Production in Adrenal Cortex. Antioxid Redox Signal (2014) 21(16):2192–207. doi: 10.1089/ars.2013.5682
59. Wang C, Du J, Du S, Liu Y, Li D, Zhu X, et al. Endogenous H(2)S Resists Mitochondria-Mediated Apoptosis in the Adrenal Glands via ATP5A1 S-Sulfhydration in Male Mice. Mol Cell Endocrinol (2018) 474:65–73. doi: 10.1016/j.mce.2018.02.011
60. García AG, García-De-Diego AM, Gandía L, Borges R, García-Sancho J. Calcium Signaling and Exocytosis in Adrenal Chromaffin Cells. Physiol Rev (2006) 86(4):1093–131. doi: 10.1152/physrev.00039.2005
61. Peng YJ, Nanduri J, Raghuraman G, Souvannakitti D, Gadalla MM, Kumar GK, et al. H2S Mediates O2 Sensing in the Carotid Body. Proc Natl Acad Sci USA (2010) 107(23):10719–24. doi: 10.1073/pnas.1005866107
62. Zhu D, Yu X, Sun J, Li J, Ma X, Yao W, et al. H2S Induces Catecholamine Secretion in Rat Adrenal Chromaffin Cells. Toxicology (2012) 302(1):40–3. doi: 10.1016/j.tox.2012.07.008
63. de Pascual R, Baraibar AM, Méndez-López I, Pérez-Ciria M, Polo-Vaquero I, Gandía L, et al. Hydrogen Sulphide Facilitates Exocytosis by Regulating the Handling of Intracellular Calcium by Chromaffin Cells. Pflugers Arch (2018) 470(8):1255–70. doi: 10.1007/s00424-018-2147-7
64. Perry SF, McNeill B, Elia E, Nagpal A, Vulesevic B. Hydrogen Sulfide Stimulates Catecholamine Secretion in Rainbow Trout (Oncorhynchus Mykiss). Am J Physiol Regul Integr Comp Physiol (2009) 296(1):R133–40. doi: 10.1152/ajpregu.00185.2008
65. Mäkelä JA, Koskenniemi JJ, Virtanen HE, Toppari J. Testis Development. Endocr Rev (2019) 40(4):857–905. doi: 10.1210/er.2018-00140
66. Sugiura Y, Kashiba M, Maruyama K, Hoshikawa K, Sasaki R, Saito K, et al. Cadmium Exposure Alters Metabolomics of Sulfur-Containing Amino Acids in Rat Testes. Antioxid Redox Signal (2005) 7(5-6):781–7. doi: 10.1089/ars.2005.7.781
67. Oi Y, Imafuku M, Shishido K, Kominato K, Nishimura R, Iwai K. Garlic Supplementation Increases Testicular Testosterone and Decreases Plasma Corticosterone in Rats Fed a High Protein Diet. J Nutr (2001) 131(8):2150–6. doi: 10.1093/jn/131.8.2150
68. Azarbarz N, Shafiei Seifabadi Z, Moaiedi MZ, Mansouri E. Assessment of the Effect of Sodium Hydrogen Sulfide (Hydrogen Sulfide Donor) on Cisplatin-Induced Testicular Toxicity in Rats. Environ Sci Pollut Res Int (2020) 27(8):8119–28. doi: 10.1007/s11356-019-07266-5
69. Wang J, Wang W, Li S, Han Y, Zhang P, Meng G, et al. Hydrogen Sulfide As a Potential Target in Preventing Spermatogenic Failure and Testicular Dysfunction. Antioxid Redox Signal (2018) 28(16):1447–62. doi: 10.1089/ars.2016.6968
70. Watanabe M, Osada J, Aratani Y, Kluckman K, Reddick R, Malinow MR, et al. Mice Deficient in Cystathionine Beta-Synthase: Animal Models for Mild and Severe Homocyst(E)Inemia. Proc Natl Acad Sci USA (1995) 92p(5):1585–9. doi: 10.1073/pnas.92.5.1585
71. Coletti R, Almeida-Pereira G, Elias LL, Antunes-Rodrigues J. Effects of Hydrogen Sulfide (H2S) on Water Intake and Vasopressin and Oxytocin Secretion Induced by Fluid Deprivation. Horm Behav (2015) 67:12–20. doi: 10.1016/j.yhbeh.2014.11.008
72. Lechuga TJ, Qi QR, Magness RR, Chen DB. Ovine Uterine Artery Hydrogen Sulfide Biosynthesis In Vivo: Effects of Ovarian Cycle and Pregnancy†. Biol Reprod (2019) 100(6):1630–6. doi: 10.1093/biolre/ioz027
73. Heinonen K. Studies on Cystathionase Activity in Rat Liver and Brain During Development. Effects of Hormones and Amino Acids In Vivo. Biochem J (1973) 136(4):1011–5. doi: 10.1042/bj1361011
74. Zheng F, Han J, Lu H, Cui C, Yang J, Cui Q, et al. Cystathionine Beta Synthase-Hydrogen Sulfide System in Paraventricular Nucleus Reduced High Fatty Diet Induced Obesity and Insulin Resistance by Brain-Adipose Axis. Biochim Biophys Acta Mol Basis Dis (2018) 1864(10):3281–91. doi: 10.1016/j.bbadis.2018.07.014
75. Dello Russo C, Tringali G, Ragazzoni E, Maggiano N, Menini E, Vairano M, et al. Evidence That Hydrogen Sulphide can Modulate Hypothalamo-Pituitary-Adrenal Axis Function: In Vitro and In Vivo Studies in the Rat. J Neuroendocrinol (2000) 12(3):225–33. doi: 10.1046/j.1365-2826.2000.00441.x
76. Jeddi S, Gholami H, Gheibi S, Kashfi K, Ghasemi A. Altered Gene Expression of Hydrogen Sulfide-Producing Enzymes in the Liver and Muscles Tissues of Hyperthyroid Rats. J Cell Physiol (2019) 234(10):17937–45. doi: 10.1002/jcp.28426
77. Sun HJ, Wu ZY, Nie XW, Wang XY, Bian JS. Implications of Hydrogen Sulfide in Liver Pathophysiology: Mechanistic Insights and Therapeutic Potential. J Adv Res (2021) 27:127–35. doi: 10.1016/j.jare.2020.05.010
78. Feng X, Chen Y, Zhao J, Tang C, Jiang Z, Geng B. Hydrogen Sulfide From Adipose Tissue is a Novel Insulin Resistance Regulator. Biochem Biophys Res Commun (2009) 380(1):153–9. doi: 10.1016/j.bbrc.2009.01.059
79. Veeranki S, Tyagi SC. Role of Hydrogen Sulfide in Skeletal Muscle Biology and Metabolism. Nitric Oxide (2015) 46:66–71. doi: 10.1016/j.niox.2014.11.012
80. Zhang L, Yang G, Untereiner A, Ju Y, Wu L, Wang R. Hydrogen Sulfide Impairs Glucose Utilization and Increases Gluconeogenesis in Hepatocytes. Endocrinology (2013) 154(1):114–26. doi: 10.1210/en.2012-1658
81. Untereiner AA, Wang R, Ju Y, Wu L. Decreased Gluconeogenesis in the Absence of Cystathionine Gamma-Lyase and the Underlying Mechanisms. Antioxid Redox Signal (2016) 24(3):129–40. doi: 10.1089/ars.2015.6369
82. Guo W, Li D, You Y, Li W, Hu B, Zhang S, et al. Cystathionine γ-Lyase Deficiency Aggravates Obesity-Related Insulin Resistance via FoxO1-Dependent Hepatic Gluconeogenesis. FASEB J (2019) 33(3):4212–24. doi: 10.1096/fj.201801894R
83. Manna P, Jain SK. Hydrogen Sulfide and L-Cysteine Increase Phosphatidylinositol 3,4,5-Trisphosphate (PIP3) and Glucose Utilization by Inhibiting Phosphatase and Tensin Homolog (PTEN) Protein and Activating Phosphoinositide 3-Kinase (PI3K)/serine/threonine Protein Kinase (AKT)/protein Kinase Cζ/λ (Pkcζ/λ) in 3T3l1 Adipocytes. J Biol Chem (2011) 286(46):39848–59. doi: 10.1074/jbc.M111.270884
84. Xue R, Hao DD, Sun JP, Li WW, Zhao MM, Li XH, et al. Hydrogen Sulfide Treatment Promotes Glucose Uptake by Increasing Insulin Receptor Sensitivity and Ameliorates Kidney Lesions in Type 2 Diabetes. Antioxid Redox Signal (2013) 19(1):5–23. doi: 10.1089/ars.2012.5024
85. Cai J, Shi X, Wang H, Fan J, Feng Y, Lin X, et al. Cystathionine γ Lyase-Hydrogen Sulfide Increases Peroxisome Proliferator-Activated Receptor γ Activity by Sulfhydration at C139 Site Thereby Promoting Glucose Uptake and Lipid Storage in Adipocytes. Biochim Biophys Acta (2016) 1861(5):419–29. doi: 10.1016/j.bbalip.2016.03.001
86. Tsai CY, Peh MT, Feng W, Dymock BW, Moore PK. Hydrogen Sulfide Promotes Adipogenesis in 3T3L1 Cells. PloS One (2015) 10(3):e0119511. doi: 10.1371/journal.pone.0119511
87. Huang CY, Yao WF, Wu WG, Lu YL, Wan H, Wang W. Endogenous CSE/H2 S System Mediates TNF-α-Induced Insulin Resistance in 3T3-L1 Adipocytes. Cell Biochem Funct (2013) 31(6):468–75. doi: 10.1002/cbf.2920
88. Parsanathan R, Jain SK. Hydrogen Sulfide Increases Glutathione Biosynthesis, and Glucose Uptake and Utilisation in C(2)C(12) Mouse Myotubes. Free Radic Res (2018) 52(2):288–303. doi: 10.1080/10715762.2018.1431626
89. Zheng J, Cheng X, Xu S, Zhang L, Pan J, Yu H, et al. Diallyl Trisulfide Induces G2/M Cell-Cycle Arrest and Apoptosis in Anaplastic Thyroid Carcinoma 8505C Cells. Food Funct (2019) 10(11):7253–61. doi: 10.1039/C9FO00646J
90. Xu S, Pan J, Cheng X, Zheng J, Wang X, Guan H, et al. Diallyl Trisulfide, a H(2) S Donor, Inhibits Cell Growth of Human Papillary Thyroid Carcinoma KTC-1 Cells Through a Positive Feedback Loop Between H(2) S and Cystathionine-Gamma-Lyase. Phytother Res (2020) 34(5):1154–65. doi: 10.1002/ptr.6586
91. Pan J, Zhang L, Xu S, Cheng X, Yu H, Bao J, et al. Induction of Apoptosis in Human Papillary-Thyroid-Carcinoma BCPAP Cells by Diallyl Trisulfide Through Activation of the MAPK Signaling Pathway. J Agric Food Chem (2018) 66(23):5871–8. doi: 10.1021/acs.jafc.8b02243
92. Wu D, Li J, Zhang Q, Tian W, Zhong P, Liu Z, et al. Exogenous Hydrogen Sulfide Regulates the Growth of Human Thyroid Carcinoma Cells. Oxid Med Cell Longev (2019) 2019:6927298. doi: 10.1155/2019/6927298
93. Qu Z, Jiang Y, Wu BQ, Duan YF, Sun ZD, Luo GH. Cystathionine-Gamma-Lyase Inhibitor Attenuates Acute Lung Injury Induced by Acute Pancreatitis in Rats. Arch Med Sci (2014) 10(4):825–9. doi: 10.5114/aoms.2014.44873
94. Tamizhselvi R, Moore PK, Bhatia M. Hydrogen Sulfide Acts as a Mediator of Inflammation in Acute Pancreatitis: In Vitro Studies Using Isolated Mouse Pancreatic Acinar Cells. J Cell Mol Med (2007) 11(2):315–26. doi: 10.1111/j.1582-4934.2007.00024.x
95. Ji L, Li L, Qu F, Zhang G, Wang Y, Bai X, et al. Hydrogen Sulphide Exacerbates Acute Pancreatitis by Over-Activating Autophagy via AMPK/mTOR Pathway. J Cell Mol Med (2016) 20(12):2349–61. doi: 10.1111/jcmm.12928
96. Sundar V, Tamizhselvi R. Inhibition of Rb Phosphorylation Leads to H(2)S-Mediated Inhibition of NF-kB in Acute Pancreatitis and Associated Lung Injury in Mice. Pancreatology (2020) 20(4):647–58. doi: 10.1016/j.pan.2020.04.011
97. Mathan Kumar M, Tamizhselvi R. Protective Effect of Diallyl Disulfide Against Cerulein-Induced Acute Pancreatitis and Associated Lung Injury in Mice. Int Immunopharmacol (2020) 80:106136. doi: 10.1016/j.intimp.2019.106136
98. Yang G, Ju Y, Fu M, Zhang Y, Pei Y, Racine M, et al. Cystathionine Gamma-Lyase/Hydrogen Sulfide System is Essential for Adipogenesis and Fat Mass Accumulation in Mice. Biochim Biophys Acta Mol Cell Biol Lipids (2018) 1863(2):165–76. doi: 10.1016/j.bbalip.2017.11.008
99. Candela J, Wang R, White C. Microvascular Endothelial Dysfunction in Obesity Is Driven by Macrophage-Dependent Hydrogen Sulfide Depletion. Arterioscler Thromb Vasc Biol (2017) 37(5):889–99. doi: 10.1161/ATVBAHA.117.309138
100. Bhattacharyya S, Saha S, Giri K, Lanza IR, Nair KS, Jennings NB, et al. Cystathionine Beta-Synthase (CBS) Contributes to Advanced Ovarian Cancer Progression and Drug Resistance. PloS One (2013) 8(11):e79167. doi: 10.1371/journal.pone.0079167
101. Santos I, Ramos C, Mendes C, Sequeira CO, Tomé CS, Fernandes DGH, et al. Targeting Glutathione and Cystathionine β-Synthase in Ovarian Cancer Treatment by Selenium-Chrysin Polyurea Dendrimer Nanoformulation. Nutrients (2019) 11(10):2523. doi: 10.3390/nu11102523
102. Lencesova L, Vlcek M, Krizanova O, Hudecova S, et al. Hypoxic Conditions Increases H₂S-Induced ER Stress in A2870 Cells. Mol Cell Biochem (2016) 414(1-2):67–76. doi: 10.1007/s11010-016-2659-4
Keywords: hydrogen sulfide, hormone, endocrine system, hypothalamus, pancreas
Citation: Chen H-J, Ngowi EE, Qian L, Li T, Qin Y-Z, Zhou J-J, Li K, Ji X-Y and Wu D-D (2021) Role of Hydrogen Sulfide in the Endocrine System. Front. Endocrinol. 12:704620. doi: 10.3389/fendo.2021.704620
Received: 03 May 2021; Accepted: 25 June 2021;
Published: 16 July 2021.
Edited by:
Brian J Arey, Bristol Myers Squibb, United StatesReviewed by:
Andrei Tica, University of Craiova, RomaniaWendong Huang, Beckman Research Institute, City of Hope, United States
Copyright © 2021 Chen, Ngowi, Qian, Li, Qin, Zhou, Li, Ji and Wu. This is an open-access article distributed under the terms of the Creative Commons Attribution License (CC BY). The use, distribution or reproduction in other forums is permitted, provided the original author(s) and the copyright owner(s) are credited and that the original publication in this journal is cited, in accordance with accepted academic practice. No use, distribution or reproduction is permitted which does not comply with these terms.
*Correspondence: Dong-Dong Wu, ZGR3dWJpb21lZDIwMTBAMTYzLmNvbQ==; Xin-Ying Ji, MTAxOTAwOTZAdmlwLmhlbnUuZWR1LmNu