- 1Institute of Orthopaedics and Traumatology, The First Affiliated Hospital of Zhejiang Chinese Medical University, Hangzhou, China
- 2Department of Pharmacy, The Second Affiliated Hospital, School of Medicine, Zhejiang University, Hangzhou, China
- 3The Fourth Clinical Medical College, Zhejiang Chinese Medical University, Hangzhou, China
- 4The Second Clinical Medical College, Zhejiang Chinese Medical University, Hangzhou, China
- 5Department of Physiology, College of Basic Medical Sciences, Zhejiang Chinese Medical University, Hangzhou, China
- 6Research Institute of Orthopedics, The Affiliated Jiang Nan Hospital of Zhejiang Chinese Medical University, Hangzhou, China
- 7Department of Orthopedics, The First Affiliated Hospital of Zhejiang Chinese Medical University, Hangzhou, China
- 8Department of Orthopedics and Traumatology, Shanghai Municipal Hospital of Traditional Chinese Medicine, Shanghai University of Traditional Chinese Medicine, Shanghai, China
Osteoporosis (OP) is a common skeletal disease, characterized by decreased bone formation and increased bone resorption. As a novel Chinese medicine formula, Zhuanggu Busui formula (ZGBSF) has been proved to be an effective prescription for treating OP in clinic, however, the pharmacological mechanisms underlying the beneficial effects remain obscure. In this study, we explored the pharmacological mechanisms of ZGBSF against OP via network pharmacology analysis coupled with in vivo experimental validation. The results of the network pharmacology analysis showed that a total of 86 active ingredients and 164 targets of ZGBSF associated with OP were retrieved from the corresponding databases, forming an ingredient-target-disease network. The protein-protein interaction (PPI) network manifested that 22 core targets, including Caspase-3, BCL2L1, TP53, Akt1, etc, were hub targets. Moreover, functional enrichment analyses revealed that PI3K-Akt and apoptosis signalings were significantly enriched by multiple targets and served as the targets for in vivo experimental study validation. The results of animal experiments revealed that ZGBSF not only reversed the high expression of Caspase-3, Bax, Prap, and low expression of Bcl-2 in osteoblasts of the OP mouse model but also contributed to the phosphorylation of Akt1 and expression of PI3K, thereby promoting osteogenesis and ameliorating the progression of OP. In conclusion, this study systematically and intuitively illustrated that the possible pharmacological mechanisms of ZGBSF against OP through multiple ingredients, targets, and signalings, and especially the inhibition of the apoptosis and the activation of PI3K-Akt signaling.
Introduction
Osteoporosis (OP), a common metabolic bone disease, is featured with decreased bone density and increased brittleness of bone, resulting in an increased risk of fracture (1, 2). It’s estimated that approximately 200 million people worldwide are suffering from OP (10.4% for males, 31.2% for females) and the number is climbing, representing a major worldwide public health problem (3, 4). The homeostasis of bone mass during bone metabolism is dynamically regulated by the osteoblast-mediated bone formation and osteoclast-mediated bone resorption (5, 6), and OP is a pathological state in which bone resorption is greater than bone formation (7, 8). Current strategies for OP fall into two categories, including anti-resorptive drugs and osteogenic drugs (9). Although these drugs are available, some are limited by side effects, such as dizziness, heart palpitations, and nausea (10). Thus, it is of importance to find new alternative therapies for OP (11).
Traditional Chinese medicine (TCM) herb formulae have their advantages and characteristics in fewer side-effects and early intervention (12, 13). “Kidney dominating bone, generating marrow” is the theoretical basis of TCM in the treatment of OP, and the deficiency of kidney function leads to OP (14). Zhuanggu Busui formula (ZGBSF) is a novel TCM formula composed of Epimedium (Yin Yang Huo), Dipsaci Radix (Xu Duan), Paeoniae Radix Alba (Bai Shao), Chuanxiong Rhizoma (Chuan Xiong), Hedysarum Multijugum Maxim. (Huang Qi), Rehmanniae Radix Praeparata (Shu Di Huang), Eucommiae Cortex (Du Zhong), Achyranthis Bidentatae Radix (Niu Xi), Carthami Flos (Hong Hua), Angelicae Sinensis Radix (Dang Gui), which is used for tonifying kidney and strengthening bone. Clinical practice has confirmed that ZGBSF is an effective prescription for treating OP. Recent system pharmacology works have shown that Eucommiae Cortex, Dipsaci Radix and Epimedium, several main components in ZGBSF, could promote osteogenic differentiation and ameliorate bone loss due to their anti-inflammatory and antioxidant properties (15–17). However, the underlying integrated pharmacological mechanisms of ZGBSF on OP remain largely elusive.
Because of multiple components and targets of TCM formulae, it is a challenge to explore their pharmacological mechanisms using conventional pharmacological methods (18, 19). With the rapid development of computer technology and system biology theory, network pharmacology, an emerging interdisciplinary subject, has great advantages to decipher the pharmacologic mechanisms of TCM with multi-components, multi-targets, and multi-pathways. A study reported that 16 compounds and 28 targets in Luohua Zizhu granule associated with colorectal adenoma were screened by network pharmacology, which revealed the mechanism of Luohua Zizhu granule against colorectal adenoma (20). Our previous study in the mouse model showed that apoptosis screened by network pharmacology had been validated to be the key signaling pathway of Liuwei Dihuang Decoction against intervertebral disc degeneration (21). Therefore, all evidence illustrated that network pharmacology analysis may be a good way to explore pharmacological mechanisms of ZGBSF in the treatment of OP.
In this study, the active compounds of ZGBSF were firstly obtained according to the screening criteria (oral bioavailability (OB) ≥ 30% and drug-likeness (DL) ≥ 0.18) (22), and the core targets of ZGBSF associated with OP were screened by the PPI network. Next, the GO and KEGG functional enrichment analyses were utilized to screen the core signaling pathways of ZGBSF against OP. Finally, the core target proteins related to the predicted pathway were further verified in OVX mice treated with ZGBSF. A flowchart of this research was shown in Figure 1.
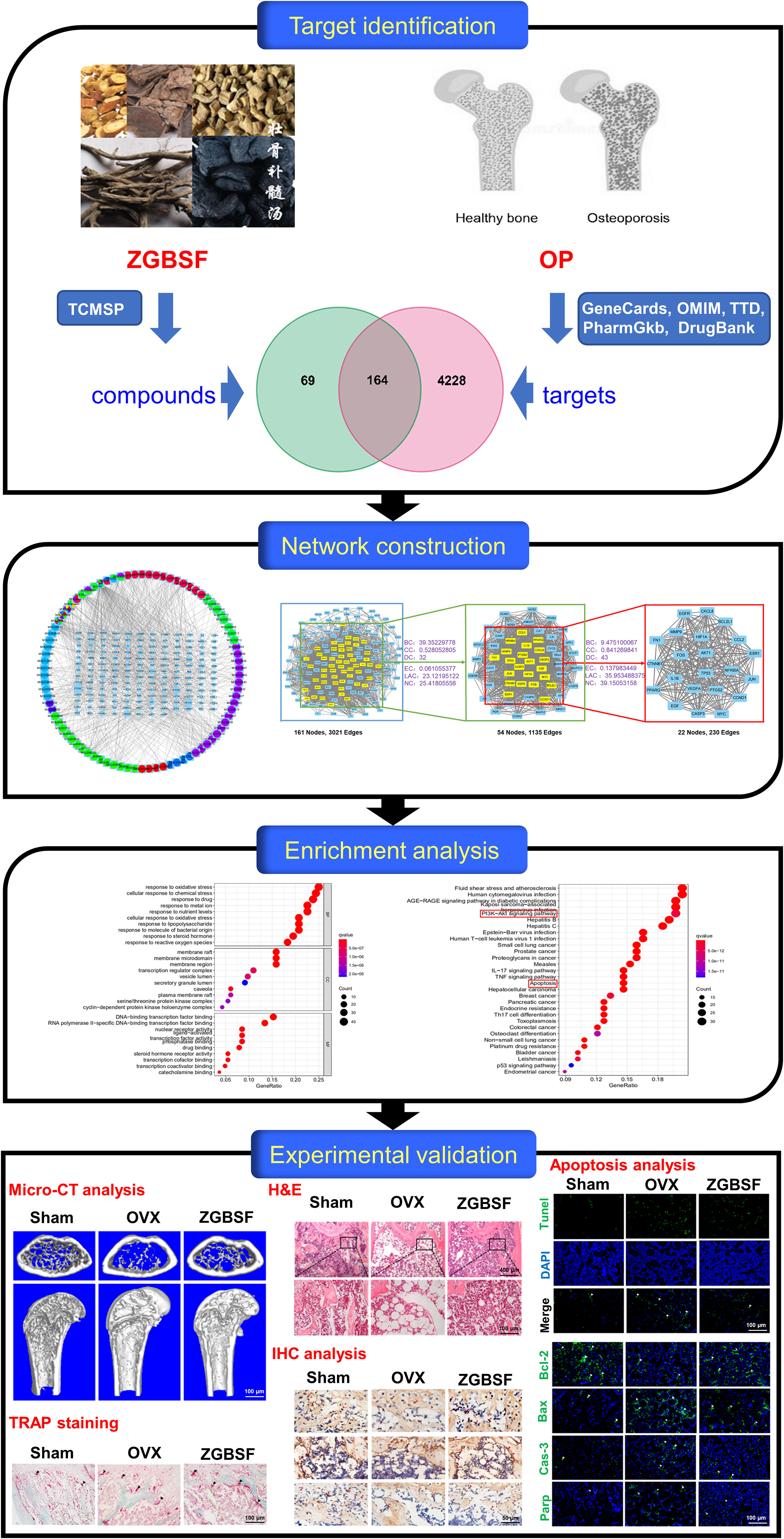
Figure 1 A flowchart for explaining the mechanism of ZGBSF against OP through targets identification, network construction, enrichment analysis, and experimental validation.
Materials and Methods
Screening for Active Compounds of ZGBSF and Target Prediction
The active compounds of ZGBSF were retrieved from the Traditional Chinese Medicine Systems Pharmacology (TCMSP) Database and Analysis Platform (http://tcmspw.com/tcmsp.php) (23) by using ‘Yin Yang Huo’, ‘Xu Duan’, ‘Bai Shao’, ‘Chuan Xiong’, ‘Huang Qi’, ‘Shu Di Huang’, ‘Du Zhong’, ‘Niu Xi’, ‘Hong Hua’ and ‘Dang Gui’ as keywords to screen targets correlated with ZGBSF. All target names were standardized through the UniProt database (https://sparql.uniprot.org/) (24).
Screening of Potential Targets for OP
The potential targets related to OP were screened from DrugBank (https://go.drugbank.com/) (25), Genecards (https://www.genecards.org/) (26), Therapeutic Target Database (TTD, http://bidd.nus.edu.sg/group/ttd/ttd.asp) (27), PharmGkb (https://www.pharmgkb.org/) (28) and Online Mendelian Inheritance in Man (OMIM, https://www.genecards.org/) (29). After elimination of repetitive targets, the potential targets correlated with OP were obtained.
Drug-Target-Disease Network Construction
A drug-target-disease interaction network was constructed by inputting active compounds in ZGBSF and common targets of these active compounds and OP into Cytoscape 3.8 software. The degree was used to evaluate the importance of compounds and targets, which represented the total number of routes associated with the node and other nodes. And the higher the degree, the more important it was (30).
Protein-Protein Interaction Network
To clarify the functional interactions among the screened potential proteins, they were all imported to the String database (https://string-db.org/) to construct Protein-Protein Interaction (PPI) network (31). Then the PPI network was inputted into Cytoscape 3.8 software, and key proteins were identified by CytoNCA software according to betweenness centrality (BC), closeness centrality (CC), degree centrality (DC), Eigenvector centrality (EC), local average connectivity-based method (LAC), and network centrality (NC), which were greater than or equal to the median value (32).
Functional Enrichment Analyses
The GO and KEGG functional enrichment analyses were performed through R software to obtain the core biological processes and signaling pathways. The top 10 items of GO analysis and 30 items of KEGG analysis were mapped as bubble plots. Finally, R software was utilized to generate the key signaling pathways related to OP (33).
Chemicals and Reagents
Primary antibodies against total (t)-Akt1, Bcl-2, Bax, Parp were purchased from Ruiying Biological Co. (Jiangsu, China). Primary antibodies against Caspase-3, p-Akt1(S473), PI3K were obtained from Hua’an Biological Co. (Hangzhou, China). Primary antibodies against alkaline phosphatase (Alp), Osterix (Osx) were from CUSABIO Biological Co. (Wuhan, China). And primary antibody against Runx2 was supplied by Abcam Company Ltd. (Cambridge, MA, USA). Alexa Fluor® 488 rabbit anti-goat IgG (H+L) secondary antibody (green) was obtained from Zhongshan Jinqiao Biotech Co. (Beijing, China). TUNEL Bright Green Apoptosis Detection Kit was supplied by Vazyme Biotech Co. (Nanjing, China). The remaining chemicals were from Sigma-Aldrich (St. Louis, Mo, USA) unless otherwise stated.
Preparation of ZGBSF
Ten raw herbs of ZGBSF were provided from the pharmacy department of the first affiliated hospital of Zhejiang Chinese Medical University (Hangzhou, China). A voucher of the specimen was stored at the first affiliated hospital of Zhejiang Chinese Medical University (Table 1). Epimedium, Dipsaci Radix, Paeoniae Radix Alba, Chuanxiong Rhizoma, Hedysarum Multijugum Maxim., Rehmanniae Radix Praeparata, Eucommiae Cortex, Achyranthis Bidentatae Radix, Carthami Flos, Angelicae Sinensis Radix were mixed as a proportion of 2:1.5:1.5:1:1.5:2:1.5:1.5:1:1.5 (w/w), and then soaked in 12 volumes of distilled water (v/m) for 1 h, decocted for 2 times, and 1.5 h each time. Finally, the water-based decoction was concentrated to 1 g/mL and stored at -20°C prior to use.
Animals and Experimental Grouping
Twenty-four C57BL/6J female mice (8-week-old, 20 ± 1 g) were provided by the animal center of Zhejiang Chinese Medical University (Hangzhou, China) and housed at the Animal Care Facility of Zhejiang Chinese Medical University according to the institutional guidelines for laboratory animals. All proposals concerning animals were approved by the Ethics Committee of Zhejiang Chinese Medical University (20211220–02), and all laboratory procedures were performed following the Regulations for the Administration of Affairs Concerning Experimental Animals approved by the State Council of the People’s Republic of China.
OVX Modeling and Treatments
All mice were randomly divided into 3 groups: Sham group, Ovariectomy (OVX) group, ZGBSF group (n = 8 in each group). After anesthesia with 1.5% isoflurane (R510-22-16, RWD Life Science, Shenzhen, China) in 100% oxygen flowing at 1.0 L/min, two ovaries of OVX and ZGBSF mice were removed, while the ovaries of Sham mice were preserved, and only part of the fat tissue around the ovaries was removed. Two days after OVX surgery, mice in the ZGBSF group were orally administered with ZGBSF (0.2 mL/10 g body weight), once a day for 8 consecutive weeks. Mice in the other two groups were given the same dosage of normal saline. All mice were euthanized 8 weeks after intragastric administration, and the femurs were collected for further analysis.
Micro-CT Analysis
After fixation with paraformaldehyde, the femurs were scanned using micro-CT (μCT) equipment (Skyscan 1176, Bruker μCT N.V., Kontich, Belgium) at a voltage of 50 kV with a current of 500 μA and a resolution of 9 μm per pixel. The NRecon v1.6 and CTAn v1.15 software were utilized to reconstruct the three-dimensional (3D) structure of the distal femoral metaphysis as we previously described (34).
Histological, Immunohistochemistry, and Immunofluorescence Analyses
After μCT analysis, the femurs were decalcified with 14% EDTA (pH 7.4) for 3 weeks, then dehydrated, embedded in paraffin, and processed into 5-μm-thick coronal-oriented sections. Then sections were stained with hematoxylin-eosin (H&E) and tartrate-resistant acid phosphatase (TRAP) as we previously described (35, 36). For immunohistochemistry (IHC) or immunofluorescence (IF) analysis, sections were incubated with primary antibodies of Runx2 (diluted 1:300), Alp (diluted 1:200), Osx (diluted 1:300), t-Akt1 (diluted 1:300), p-Akt1(S473) (diluted 1:50), PI3K (diluted 1:50), Bcl-2 (diluted 1:300), Bax (diluted 1:300), Caspase-3 (diluted 1:300), Parp (diluted 1:300) at 4°C overnight. Secondary biotinylated goat anti-rabbit antibody (diluted 1:1000) (Invitrogen) was added for 30 min the following day, and a diaminobenzidine solution (Invitrogen) was used to detect IHC staining. For IF analysis, secondary goat anti-rabbit antibody conjugated with fluorescence (diluted 1:1000) was added for 30 min the next day. The quantification of positive cells was estimated in a blinded manner with Image-Pro Plus 6.0 (Media Cybernetics, Silver Spring, MD, USA).
TUNEL Assay
To further evaluate apoptosis of osteoblasts in femoral tissues, the DNA damage, another hallmark of aopotosis, was determined by the TUNEL Bright Green Apoptosis Detection Kit (Vazyme Biotech, Nanjing, China). All operations were performed according to the manufacturer’s instructions. The Image-Pro Plus 6.0 (Media Cybernetics, Silver Spring, MD, USA) was used to calculate the number of positive cells by using three sections from each sample in three randomly selected fields of view with blind method. And the total number of cells was calculated by DAPI staining.
Statistical Analysis
All numerical data were expressed as mean ± standard error of mean (SEM). One-way analysis of variance (ANOVA) with a Student-Newman-Keuls (SNK) post hoc test was performed using GraphPad Prism 6.0 (GraphPad Software Inc., La Jolla, CA). When P < 0.05, the differences were considered to be statistically significant.
Results
Active Compounds in ZGBSF and Targets Prediction
A total of 145 active compounds of ZGBSF were screened, including 23 from Yin Yang Huo, 8 from Xu Duan, 13 from Bai Shao, 7 from Chuan Xiong, 20 from Huang Qi, 2 from Shu Di Huang, 28 from Du Zhong, 20 from Niu Xi, 22 from Hong Hua, and 2 from Dang Gui. And 2729 related targets of ZGBSF were obtained from the TCMSP database, containing 511 types in Yin Yang Huo, 63 types in Xu Duan, 123 types in Bai Shao, 42 types in Chuan Xiong, 462 types in Huang Qi, and 34 types in Shu Di Huang, 532 types in Du Zhong, 444 types in Niu Xi, 449 types in Hong Hua, and 69 types in Dang Gui. After excluding duplicates, a total of 86 compounds and 233 targets were selected for the following research.
Potential Targets Prediction of OP
To identify potential targets of OP, we searched 5 databases for OP-related research reports. A total of 4725 targets of OP were screen out, of which 384 were from the DrugBank database, 4273 from the GeneCards database, 31 from the TTD database, 3 from the PharmGkb database, 34 from the OMIM database. After expurgating duplicate targets, 4392 targets related to OP were screened out (Figure 2A).
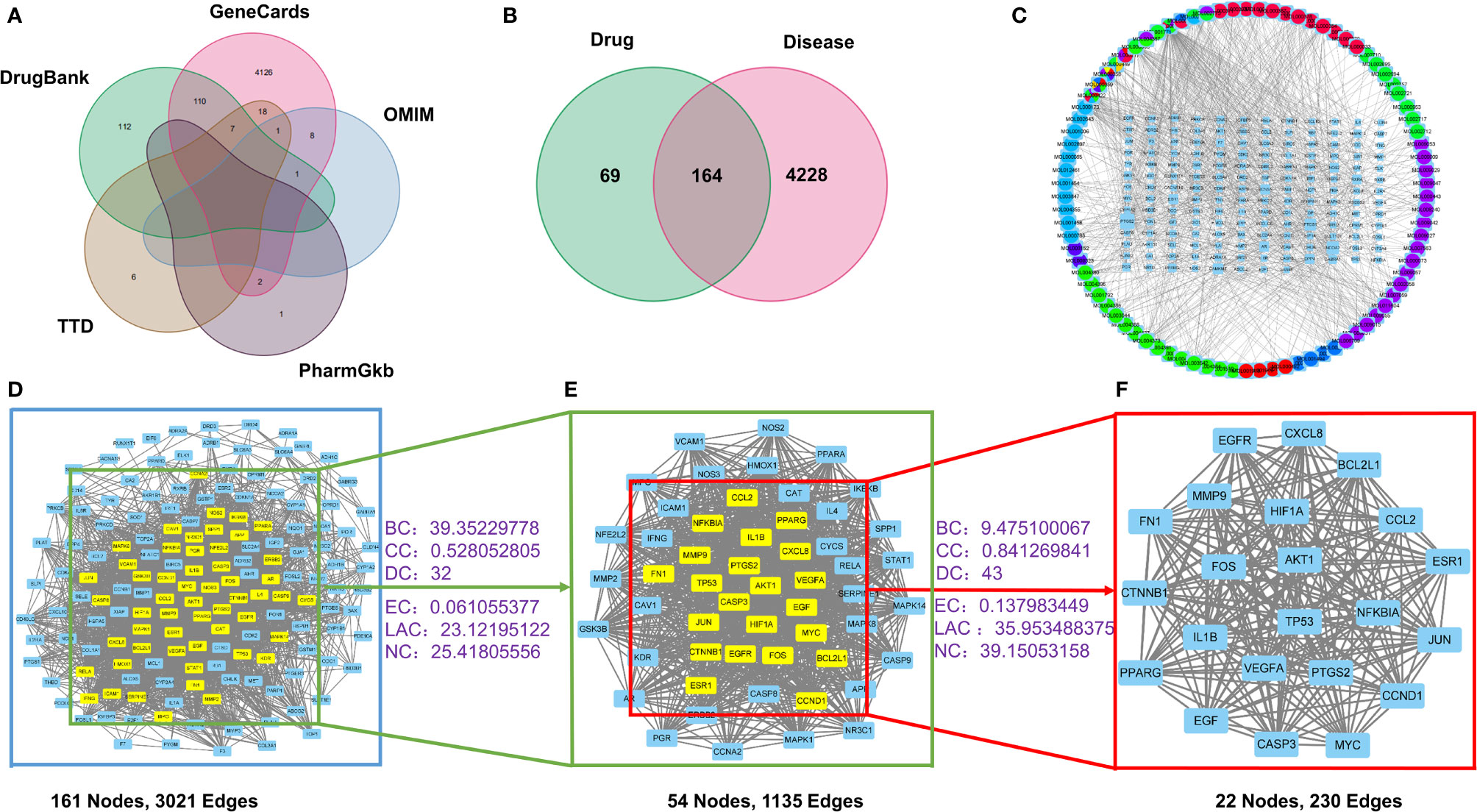
Figure 2 The drug-target-disease network and PPI network construction. (A) The collection of predictive targets for OP. (B) The intersection targets of ZGBSF-related targets and OP-related targets. (C) The network of drug-target-disease includes 10 kinds of herbs, 86 active components, and 164 target genes. Circle represents active components and rectangle represents related targets. (D) PPI network of predicted targets of ZGBSF against OP. (E) The significant proteins of the PPI network were extracted from (D). (F) Screening 22 key proteins of ZGBSF in the treatment of OP were extracted from (E).
Drug-Target-Disease Network Construction
To obtain the intersection of ZGBSF target genes and OP target genes, the Venn diagram analysis was performed. The results showed that a total of 164 target genes were identified (Figure 2B), which matched with the related targets of 86 active compounds. Then, to clarify the relationship among active compounds, targets, and OP, a drug-target-disease network, including 250 nodes and 847 edges, was constructed by using Cytoscape 3.8 software (Figure 2C). The top 10 core compounds of ZGBSF against OP were listed in Table 2.
PPI Network Construction and Key Targets
To obtain the pivotal proteins of ZGBSF in treating OP, after removing 3 isolates, a PPI network with 161 nodes and 3021 edges was constructed by using a String database (Figure 2D). Then, the thresholds of BC, CC, DC, EC, LAC, and NC were set in two steps according to the topological parameters calculated by CytoNCA. And a total of 22 pivotal proteins were filtered out, including Caspase-3, BCL2L1, TP53, IL-1β, Akt1, ESR1, FN1, CCND1, VEGFA, HIF1A, EGFR, CXCL8, CCL2, CTNNB1, JUN, PPARG, FOS, NFKBIA, PTGS2, MYC, MMP9, EGF, which were strongly linked to OP (Figures 2E, F).
Functional Enrichment Analysis
To explore the crucial biological processes of ZGBSF in the treatment of OP, the GO functional enrichment analyses were performed. The results of GO analysis revealed that the 164 genes were enriched in 2709 GO entries, consisting of 2401 biological progress (BP), 92 cellular components (CC), and 216 molecular functions (MF) (P < 0.05), and the top 10 entries of BP, CC and MF were shown in Figure 3A. BP analysis revealed that related targets were mainly centered on cellular response to chemical stress, drug, oxidative stress, etc. CC analysis indicated that related targets were mainly centered on membrane microdomain, membrane raft, etc. Moreover, MF analysis showed that potential targets were primarily focused on nuclear receptor activity, transcription factor activity, RNA polymerase II−specific DNA−binding transcription factor binding, etc.
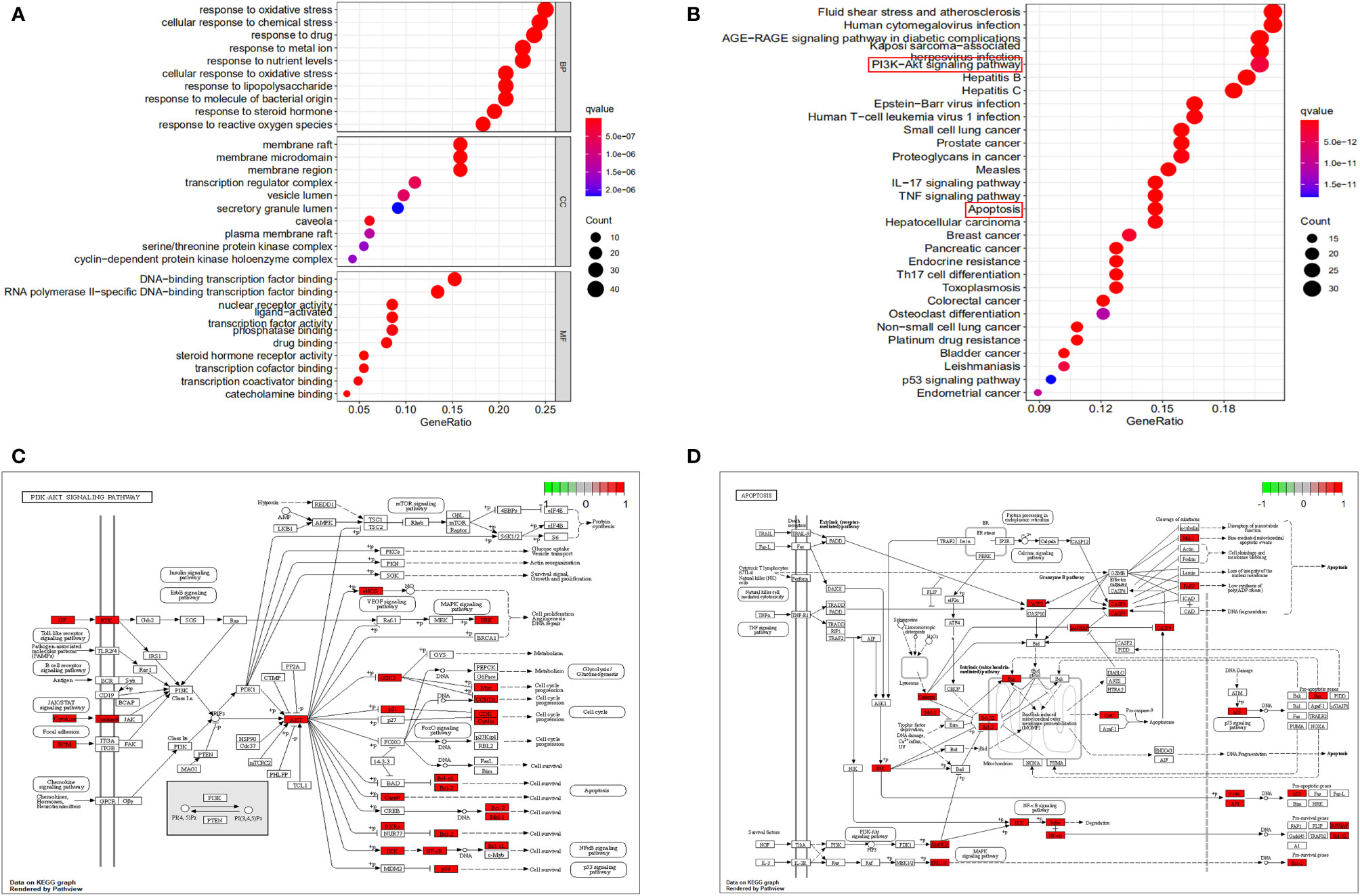
Figure 3 GO and KEGG functional analysis. (A) The top 10 of GO enrichment analysis in Biological processes (BP), cellular components (CC) and molecular function (MF). (B) The top 30 signaling pathways were analyzed by KEGG. (C, D) The PI3K-Akt signaling pathway (C) and apoptosis (D) were described in detail. The larger the nodes, the darker the color, and the more genes were enriched.
To investigate the representative signaling pathways associated with the key targets, the KEGG enrichment analysis was performed. And the results showed that 160 significantly enriched signaling pathways were retrieved (P < 0.05). The top 30 significantly enriched signaling pathways closely correlated with OP were shown in Figure 3B, including apoptosis, PI3K-Akt, and TNF signaling pathways, etc. Furthermore, we found that apoptosis and PI3K-Akt signaling pathways were most closely related to OP in these enriched pathways, and the predicted targets corresponding to these two signaling pathways were shown in Figures 3C, D.
ZGBSF Ameliorates Bone Loss and the Progression of OP in OVX Mice
To verify the core pharmacological mechanism of ZGBSF in treating OP predicted by the above-mentioned network pharmacology analysis, OVX model mice were established and treated intragastrically with ZGBSF for 8 weeks. The femurs of OVX mice were harvested and radiographic alterations were assessed by μCT analysis. The results of μCT analysis showed that compared with sham mice, the bone mass of OVX mice significantly reduced, whereas ZGBSF intervention significantly restored the bone mass (Figure 4A). Moreover, the results of bone morphological parameter analysis showed that ZGBSF enhanced the decrease of bone mineral density (BMD), bone volume fraction (BV/TV), trabecular thickness (Tb.Th), trabecular number (Tb.N) in OVX mice. However, it had no significant recovery effect for the widened trabecular separation (Tb.Sp) (Figures 4B–F). In parallel, the histological changes of the femur were examined using H&E staining. The results showed that the femoral tissues of OVX mice exhibited less trabecular bone, and a large number of lipid droplets were present instead. ZGBSF administration significantly reversed these alterations (Figures 4G–I). All these findings showed that ZGBSF effectively attenuated bone loss and the progression of OP.
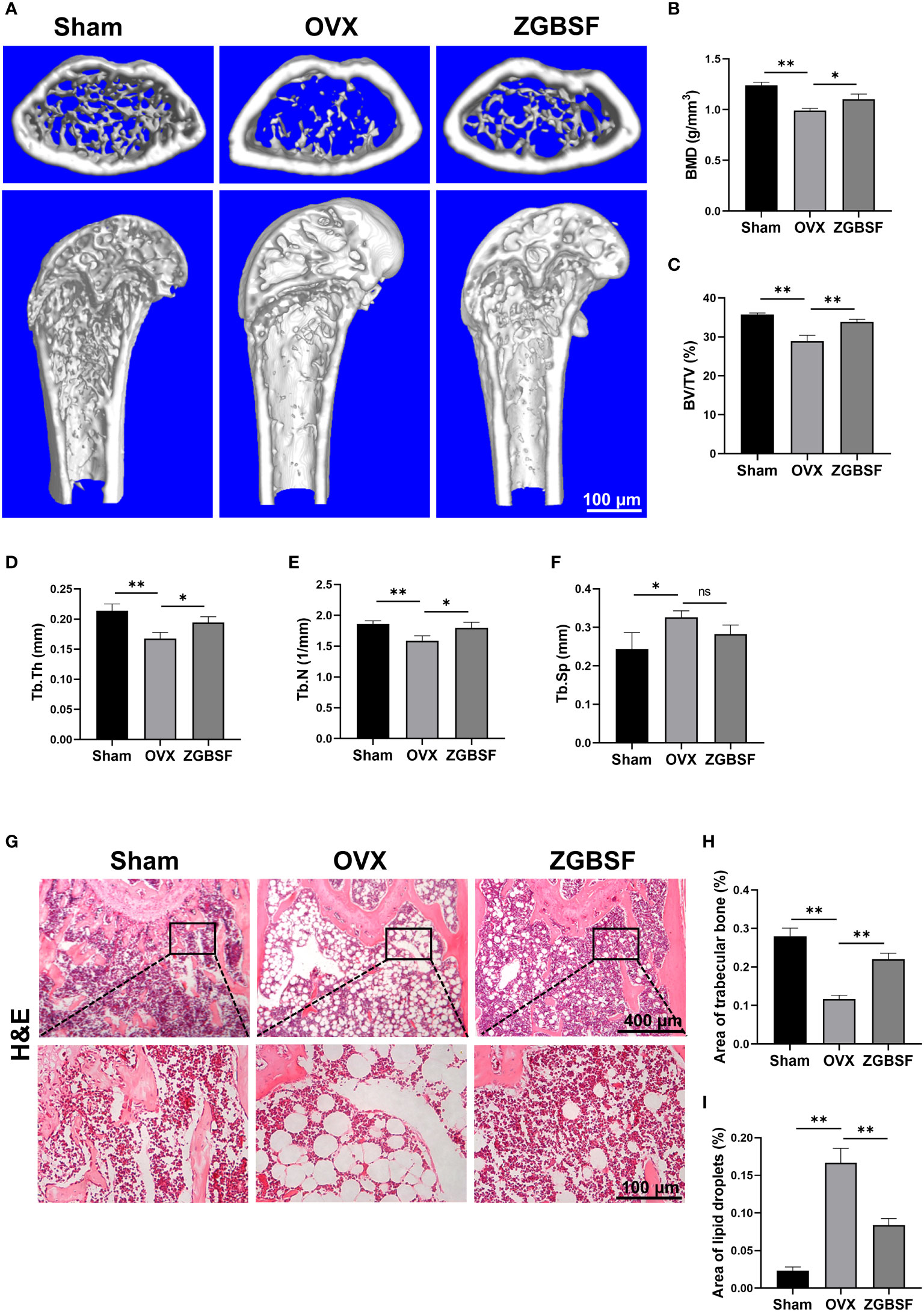
Figure 4 ZGBSF ameliorates bone loss in OVX mice. (A) Representative μCT images of distal femoral. Quantification of bone mineral density (BMD) (B), bone volume fraction (BV/TV) (C), trabecular thickness (Tb.Th) (D), trabecular number (Tb.N) (E) and trabecular separation (Tb.Sp) (F). (G) Hematoxylin-eosin staining of the distal femur. (H) The area of trabecular bone (%). (I) The area of lipid droplets (%). Data were presented as mean ± SEM of three independent experiments. *P < 0.05, **P < 0.01, NS indicated no significant difference.
ZGBSF Promotes Osteogenesis and Fails to Inhibit the Formation of Osteoclasts
Bone homeostasis and remodelling is regulated by a balance between osteogenesis of osteoblast and osteoclastogenesis of osteoclast (37). To examine the effects of ZGBSF on osteogenesis, protein levels of Runx2, Alp, and Osx were determined by IHC analysis. The results of IHC analysis revealed that Runx2, Alp, and Osx were significantly decreased in OVX mice. The treatment of ZGBSF significantly increased the expression of Runx2, Alp, and Osx (Figures 5A–D). Subsequently, to explore the effect of ZGBSF on osteoclast formation, the number of osteoclasts was evaluated by TRAP staining. Compared with sham mice, the number of osteoclasts in the distal femurs of OVX mice was markedly increased. Unexpectedly, ZGBSF intervention had no significant effect on the increase of osteoclast number in OVX mice (Figures 5E, F).
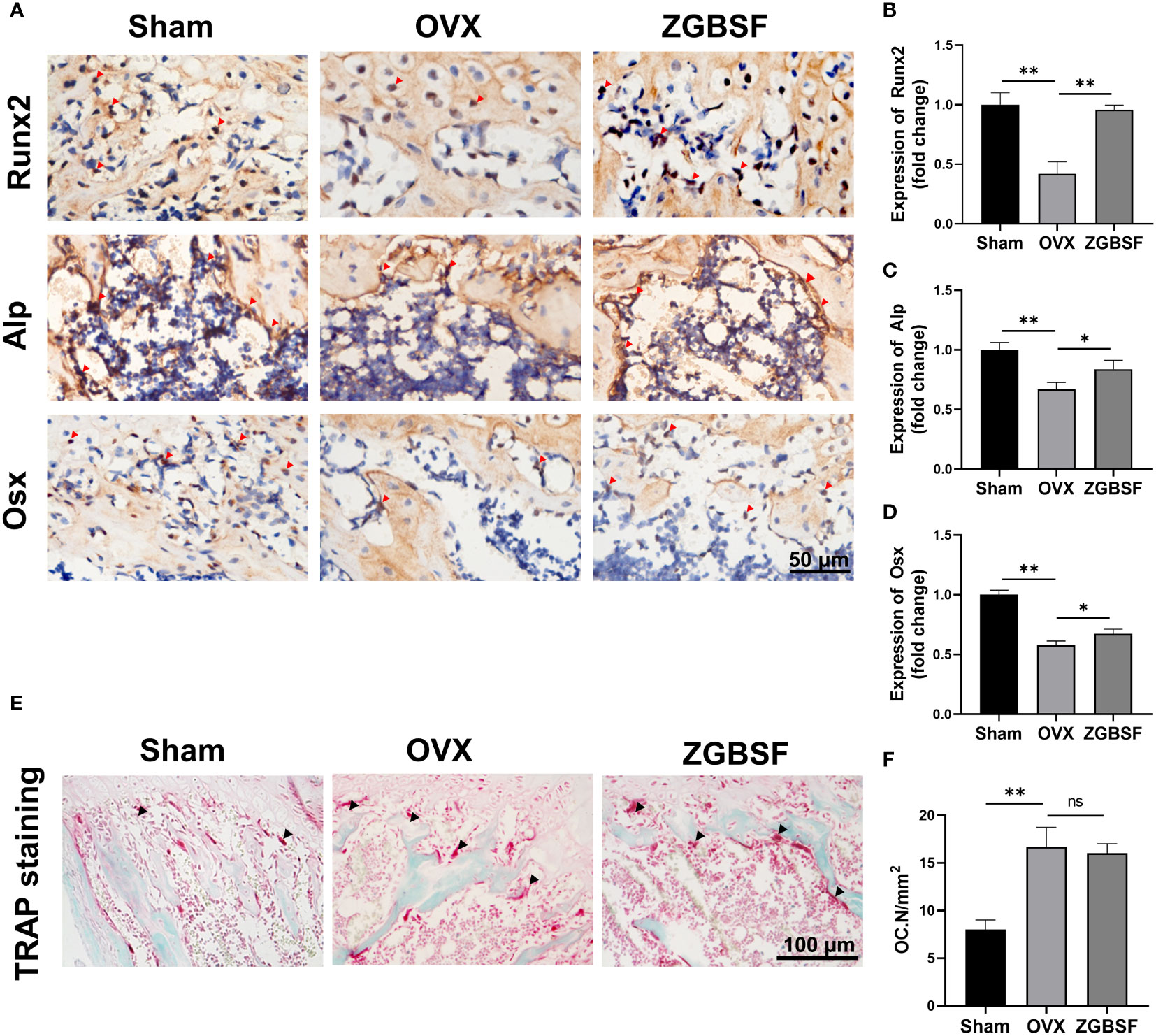
Figure 5 ZGBSF promotes osteogenesis and fails to inhibit osteoclastogenesis. (A) Immunohistochemistry of Runx2, Alp, and Osx of the distal femur. Red arrows indicated the high expression of Runx2, Alp, and Osx. (B–D) The quantification of Runx2, Alp, and Osx expression. (E) TRAP staining of the distal femur. (F) The number of osteoclasts (OC.N/mm2). The arrows represented the positive expression. Data were presented as mean ± SEM of three independent experiments. *P < 0.05, **P < 0.01, NS indicated no significant difference.
ZGBSF Suppresses Apoptosis and Activates the PI3K-Akt Signaling Pathway of Osteoblasts
The prediction results from our network pharmacology analysis revealed that the apoptosis and PI3K-Akt signaling pathways were pivotal biological events in ZGBSF against OP. Therefore, we first determined the expression of Bcl-2, Bax, Caspase-3, and Parp, indicators of apoptosis, by IF analysis. The IF results showed that Bcl-2 was markedly decreased in the distal femur of OVX mice, while Bax, Caspase-3 and Parp were significantly increased. ZGBSF significantly reversed these alterations (Figures 6A–E). In parallel, the anti-apoptosis effect of ZGBSF was further confirmed by TUNEL staining. The results of TUNEL staining revealed that ZGBSF significantly inhibited the increased apoptosis ratio of osteoblasts in femoral tissues of OVX mice (Figures 6F, G). All these findings showed that ZGBSF suppressed apoptosis of osteoblasts and contributed to osteogenesis, thus ameliorating bone loss of OVX mice.
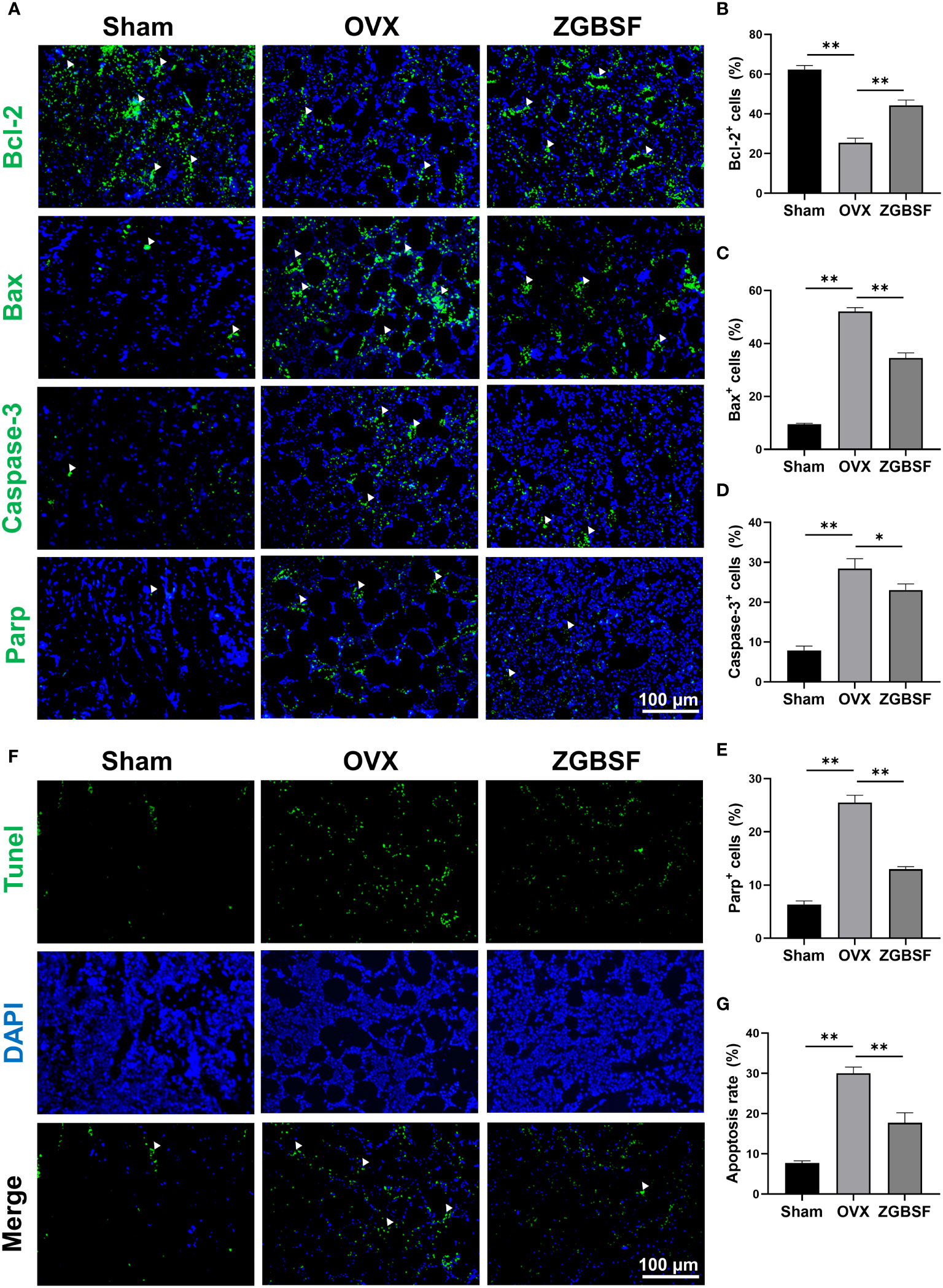
Figure 6 ZGBSF suppresses apoptosis of osteoblasts to ameliorate OP in OVX mice. (A) Immunofluorescence of Bcl-2, Bax, Caspase-3 and Parp in distal femur. (B–E) The quantification of Bcl-2, Bax, Caspase-3 and Parp positive cells. (F) Tunel staining was performed to examine the apoptosis status of osteoblasts. (G) The quantification of TUNEL positive rates in the distal femur. White arrows indicated the positive expression. Data were presented as mean ± SEM of three independent experiments. *P < 0.05, **P < 0.01.
Next, to verify the effect of ZGBSF on PI3K−Akt signaling pathway predicted by KEGG enrichment analysis, the phosphorylation status of Akt1, and expression of PI3K protein were examined by IHC analysis of t-Akt1, p-Akt1, and PI3K. The IHC results showed that the levels of p-Akt1 and PI3K protein in distal femoral tissue of OVX mice were lower than those in sham mice. ZGBSF treatment significantly upregulated the expression of p-Akt1 and PI3K protein in ZGBSF mice (Figures 7A–C).
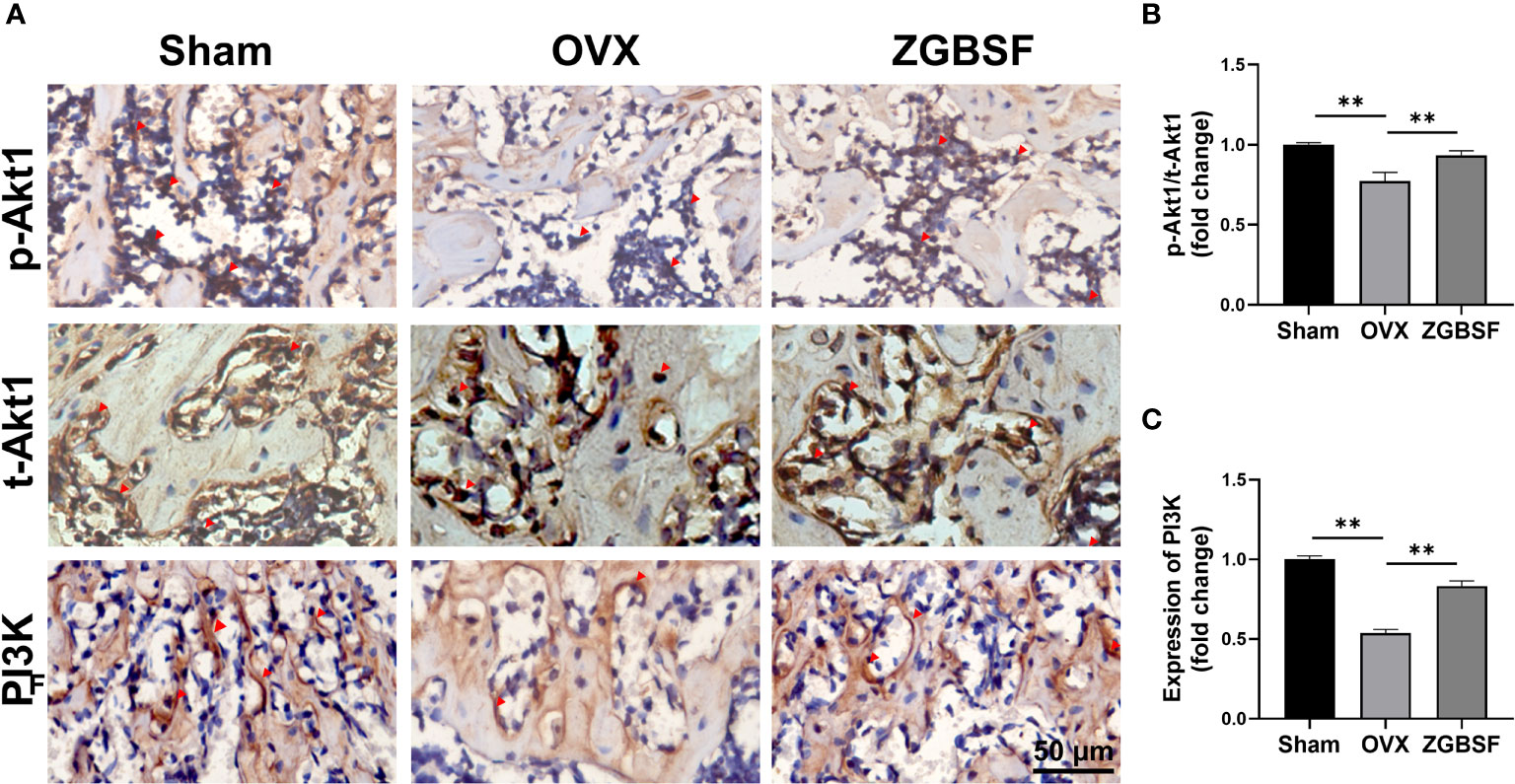
Figure 7 ZGBSF activates the PI3K-Akt signaling pathway of femoral tissue. (A) Immunohistochemistry of t-Akt1, Akt1(S473), and PI3K of distal femur. (B, C) The quantification of Akt1(S473)/t-Akt1, and PI3K expression. Red arrows indicated the positive expression. Data were presented as mean ± SEM of three independent experiments. **P < 0.01.
Discussion
OP is a common chronic skeletal disease worldwide, characterized by bone loss and increased fracture risk. Fractures caused by OP are highly susceptible to disability and mortality in the elderly (38, 39). ZGBSF is a novel TCM formula, which has been proved to be an effective drug for treating OP in clinic. However, due to the diversity of components in ZGBSF, the pharmacological mechanism of its anti-OP effect is still elusive, which greatly hinders its popularization and application. Network pharmacology, a newly developed cross-discipline, has unique advantages in deciphering the molecular mechanisms of TCM on diseases with multiple components, targets, and pathways (40, 41). In this study, we deciphered the underlying molecular mechanisms of ZGBSF in the treatment of OP via network pharmacology analysis and in vivo experimental validation. We found that ZGBSF contributed to osteogenesis by inhibiting osteoblast apoptosis and activating PI3K-Akt signaling pathways, thereby attenuating bone loss and the progression of OP.
Based on network pharmacology analysis, the core compounds of TCM have made great contributions to the therapeutic effect of treating diseases (42). In the present study, we firstly discovered that the top active compounds in ZGBSF consist of quercetin, luteolin, kaempferol, baicalein, etc. Previous studies have shown that quercetin could inhibit oxidative stress and inflammatory response as well as the apoptosis of osteoblasts, thereby promoting osteogenesis (43). In vivo and in vitro experiments also showed that luteolin and kaempferol ameliorated OP by regulating oxidative stress and osteogenesis (44, 45). In addition, baicalein contributed to the expression of OP-related proteins and extracellular matrix mineralization (46). Therefore, all these findings showed that multiple components of ZGBSF had a good therapeutic effect on OP.
To explore the underlying mechanisms of ZGBSF in the treatment of OP, the OP-related targets of core components of ZGBSF were identified by the PPI network. We found that the top targets of ZGBSF for OP included Caspase-3, BCL2L1, and TP53, which were closely related to the process of apoptosis. Caspase-3 is a critical regulator of apoptosis, which has been proven to impair bone formation (47). A recent study reported that lowering the expression of Caspase-3 by quercetin and vitamin E inhibited osteoblast apoptosis, thus alleviating OP (48). BCL2L1 is a vital apoptosis regulator gene encoding splicing variants of anti-apoptosis [Bcl-x(L)] and pro-apoptosis [Bcl-x(S)] (49). Overexpression of BCL-x(L) in osteoblasts suppressed the apoptosis of osteoblasts and increased the bone mass of trabecular bone and cortical bone (50). In addition, as a pro-apoptotic protein, TP53 is closely related to OP (51). An in vitro experiment revealed that downregulating the expression of p53 observably increased the expression of osteogenesis-related proteins, such as Alp and Runx2 (52). All these findings showed that Caspase-3, BCL2L1, and P53 played an important role in the progression of OP.
Previous studies have shown that the excessive apoptosis of osteoblasts may exacerbate the progression of osteoporosis (53). Inhibiting the apoptosis of osteoblasts significantly enhanced osteogenic differentiation, thereby exerting anti-OP effects (54). A recent study on OP model of rats showed that quercetin improved ovariectomy-induced osteoporosis via regulation of apoptosis (48). Consistent with the above studies, our KEGG enrichment analysis showed that apoptosis was involved in the intervention of ZGBSF for OP, and in vivo mouse OVX model evidence revealed that ZGBSF treatment significantly overturned the decreased Bcl-2 protein and increased levels of Bax, Caspase-3, Parp as well as increased DNA damage in OVX mice. The results showed that ZGBSF attenuated the apoptosis of osteoblasts.
Increasing studies revealed that the PI3K-Akt signaling pathway played an important role in the physiological and pathological processes of OP (55, 56). Activation of the PI3K-Akt signaling pathway resulted in enhanced osteogenic differentiation of human bone marrow mesenchymal stem cells (15). Similarly, another work discovered that quercetin, one of the top active compounds in ZGBSF, promoted osteogenic differentiation and mineralization via PI3K-Akt signaling pathway (57). Consistent with those studies, our results uncovered that the activity of the PI3K-Akt signaling pathway was decreased in OVX mice, whereas ZGBSF treatment significantly activated the activity of the PI3K-Akt signaling pathway. And ZGBSF significantly enhanced the expression of decreased osteogenic markers (Runx2, Alp, and Osx) in OVX mice. However, ZGBSF administration had no significant effect on the increase of osteoclasts number in OVX mice. All these findings revealed that the treatment of ZGBSF dramatically promoted osteogenesis by activating the PI3K-Akt signaling pathway.
In total, ZGBSF could ameliorate the progression of OP via multi-ingredients, multi-targets, multi-pathways mode, which was different from a single drug with few targets. Network target and signaling pathway could perfectly decipher sophisticated interactions between diseases with chemical ingredients in TCM. However, there were some limitations in this study. First, only core targets and pathways of ZGBSF were verified, which may result in a slight deviation of results. Further validation of other relevant targets and signaling pathways predicted by network pharmacology is needed in future experiments. Second, our in vivo animal experiment was limited to mouse OVX model, so human-derived osteoblasts were used to make the experiment more clinically relevant in a follow-up experiment.
Conclusion
In summary, to decipher the molecular mechanisms of ZGBSF in the treatment of OP, network pharmacology analysis coupled with experimental validation were performed. The network pharmacology analysis revealed that ZGBSF exerted anti-OP effects via multi-ingredients, multi-targets, and multi-pathways, and the experimental results validated that ZGBSF promoted osteogenesis via inhibition of osteoblast apoptosis and activation of PI3K-Akt signaling pathway, thus ameliorating bone loss of OP. This study could provide an optimized method to elucidate the pharmacological mechanisms of ZGBSF and supply a novel candidate for the treatment of OP.
Data Availability Statement
The original contributions presented in the study are included in the article/supplementary material. Further inquiries can be directed to the corresponding authors.
Ethics Statement
The animal study was reviewed and approved by The Ethics Committee of Zhejiang Chinese Medical University.
Author Contributions
HR, PT, XL, CW contributed to the concept and design of this study. HZ, CZ, ZZ performed the computational analyses and performed the in vivo experiments. SaY, YB, FF, HL, YL, SxY, YG, YC, KZ, MY, WD, KT, HJ performed the experimental analysis. HZ drafted the manuscript. All the authors read, revised, and approved the final manuscript.
Funding
This study was financially supported by National Natural Science Foundation of China (No.: 82174140, 82174401, 82104164, 81973870, 81973881, 81904053, 81804121), China Postdoctoral Science Foundation (No.: 2018M632154), Natural Science Foundation of Zhejiang Province (No.: LY22H270003, LBY22H270008, LY19H270006, and LQ19H080001), Traditional Chinese Medical Administration of Zhejiang Province (No.: 2022ZX005, 2022ZB119, 2021ZB090), Zhejiang medical and health science and technology project (No.: 2021KY222), Research Project of Zhejiang Chinese Medical University Scientific (No.: 2021JKZDZC02, 2021JKZKTS036A, 2021JKJNTZ022B, 2019ZG25, 2018ZR06), National Undergraduate Innovation and Entrepreneurship Training Program (202110344005, 202110344025, S202110344007, 202010344004), General Research Project of Zhejiang Provincial Education Department “Special Project for the Reform of Cultivation Mode of Professional Degree Graduate Students in Higher Education Institutions” (No.: Y202145932), Postgraduate Science Research Fund of Zhejiang Chinese Medical University (No.: 2021YKJ02, 2020YKJ07).
Conflict of Interest
The authors declare that the research was conducted in the absence of any commercial or financial relationships that could be construed as a potential conflict of interest.
Publisher’s Note
All claims expressed in this article are solely those of the authors and do not necessarily represent those of their affiliated organizations, or those of the publisher, the editors and the reviewers. Any product that may be evaluated in this article, or claim that may be made by its manufacturer, is not guaranteed or endorsed by the publisher.
References
1. Cotts KG, Cifu AS. Treatment of Osteoporosis. Jama (2018) 319(10):1040–1. doi: 10.1001/jama.2017.21995
2. Compston JE, McClung MR, Leslie WD. Osteoporosis. Lancet (London England) (2019) 393(10169):364–76. doi: 10.1016/s0140-6736(18)32112-3
3. Macías I, Alcorta-Sevillano N, Rodríguez CI, Infante A. Osteoporosis and the Potential of Cell-Based Therapeutic Strategies. Int J Mol Sci (2020) 21(5):1653. doi: 10.3390/ijms21051653
4. Lin Z, Zheng J, Chen J, Chen M, Dong S. Antiosteoporosis Effect and Possible Mechanisms of the Ingredients of Fructus Psoraleae in Animal Models of Osteoporosis: A Preclinical Systematic Review and Meta-Analysis. Oxid Med Cell Longev (2021) 2021:2098820. doi: 10.1155/2021/2098820
5. Chen M, Han H, Zhou S, Wen Y, Chen L. Morusin Induces Osteogenic Differentiation of Bone Marrow Mesenchymal Stem Cells by Canonical Wnt/beta-Catenin Pathway and Prevents Bone Loss in an Ovariectomized Rat Model. Stem Cell Res Ther (2021) 12(1):173. doi: 10.1186/s13287-021-02239-3
6. Song N, Zhao Z, Ma X, Sun X, Ma J, Li F, et al. Naringin Promotes Fracture Healing Through Stimulation of Angiogenesis by Regulating the VEGF/VEGFR-2 Signaling Pathway in Osteoporotic Rats. Chem-Biol Interact (2017) 261:11–7. doi: 10.1016/j.cbi.2016.10.020
7. Cui J, Li X, Wang S, Su Y, Chen X, Cao L, et al. Triptolide Prevents Bone Loss via Suppressing Osteoclastogenesis Through Inhibiting PI3K-AKT-NFATc1 Pathway. J Cell Mol Med (2020) 24(11):6149–61. doi: 10.1111/jcmm.15229
8. Ukon Y, Makino T, Kodama J, Tsukazaki H, Tateiwa D, Yoshikawa H, et al. Molecular-Based Treatment Strategies for Osteoporosis: A Literature Review. Int J Mol Sci (2019) 20(10):2557. doi: 10.3390/ijms20102557
9. Chen LR, Ko NY, Chen KH. Medical Treatment for Osteoporosis: From Molecular to Clinical Opinions. Int J Mol Sci (2019) 20(9):2213. doi: 10.3390/ijms20092213
10. Rachner TD, Khosla S, Hofbauer LC. Osteoporosis: Now and the Future. Lancet (London England) (2011) 377(9773):1276–87. doi: 10.1016/s0140-6736(10)62349-5
11. Che CT, Wong MS, Lam CW. Natural Products From Chinese Medicines With Potential Benefits to Bone Health. Molecules (Basel Switzerland) (2016) 21(3):239. doi: 10.3390/molecules21030239
12. Zhu B, Zhang QL, Hua JW, Cheng WL, Qin LP. The Traditional Uses, Phytochemistry, and Pharmacology of Atractylodes Macrocephala Koidz.: A Review. J Ethnopharmacol (2018) 226:143–67. doi: 10.1016/j.jep.2018.08.023
13. Liu C, Ma R, Wang L, Zhu R, Liu H, Guo Y, et al. Rehmanniae Radix in Osteoporosis: A Review of Traditional Chinese Medicinal Uses, Phytochemistry, Pharmacokinetics and Pharmacology. J Ethnopharmacol (2017) 198:351–62. doi: 10.1016/j.jep.2017.01.021
14. Xu R, Zeng Q, Xia C, Chen J, Wang P, Zhao S, et al. Fractions of Shen-Sui-Tong-Zhi Formula Enhance Osteogenesis Via Activation of β-Catenin Signaling in Growth Plate Chondrocytes. Front Pharmacol (2021) 12:711004. doi: 10.3389/fphar.2021.711004
15. Ye C, Zhang W, Hang K, Chen M, Hou W, Chen J, et al. Extracellular IL-37 Promotes Osteogenic Differentiation of Human Bone Marrow Mesenchymal Stem Cells via Activation of the PI3K/AKT Signaling Pathway. Cell Death Dis (2019) 10(10):753. doi: 10.1038/s41419-019-1904-7
16. Hussain T, Tan B, Liu G, Oladele OA, Rahu N, Tossou MC, et al. Health-Promoting Properties of Eucommia Ulmoides: A Review. Evid Based complementary Altern Med eCAM (2016) 2016:5202908. doi: 10.1155/2016/5202908
17. Zhang W, Xue K, Gao Y, Huai Y, Wang W, Miao Z, et al. Systems Pharmacology Dissection of Action Mechanisms of Dipsaci Radix for Osteoporosis. Life Sci (2019) 235:116820. doi: 10.1016/j.lfs.2019.116820
18. Yang L, Fan L, Wang K, Chen Y, Liang L, Qin X, et al. Analysis of Molecular Mechanism of Erxian Decoction in Treating Osteoporosis Based on Formula Optimization Model. Oxid Med Cell Longev (2021) 2021:6641838. doi: 10.1155/2021/6641838
19. An L, Lin Y, Li L, Kong M, Lou Y, Wu J, et al. Integrating Network Pharmacology and Experimental Validation to Investigate the Effects and Mechanism of Astragalus Flavonoids Against Hepatic Fibrosis. Front Pharmacol (2020) 11:618262. doi: 10.3389/fphar.2020.618262
20. Guo C, Kang X, Cao F, Yang J, Xu Y, Liu X, et al. Network Pharmacology and Molecular Docking on the Molecular Mechanism of Luo-Hua-Zi-Zhu (LHZZ) Granule in the Prevention and Treatment of Bowel Precancerous Lesions. Front Pharmacol (2021) 12:629021. doi: 10.3389/fphar.2021.629021
21. Zhang H, Yao S, Zhang Z, Zhou C, Fu F, Bian Y, et al. Network Pharmacology and Experimental Validation to Reveal the Pharmacological Mechanisms of Liuwei Dihuang Decoction Against Intervertebral Disc Degeneration. Drug Design Dev Ther (2021) 15:4911–24. doi: 10.2147/dddt.s338439
22. Feng W, Ao H, Yue S, Peng C. Systems Pharmacology Reveals the Unique Mechanism Features of Shenzhu Capsule for Treatment of Ulcerative Colitis in Comparison With Synthetic Drugs. Sci Rep (2018) 8(1):16160. doi: 10.1038/s41598-018-34509-1
23. Ru J, Li P, Wang J, Zhou W, Li B, Huang C, et al. TCMSP: A Database of Systems Pharmacology for Drug Discovery From Herbal Medicines. J Cheminform (2014) 6:13. doi: 10.1186/1758-2946-6-13
24. UniProt C. UniProt: A Worldwide Hub of Protein Knowledge. Nucleic Acids Res (2019) 47(D1):D506–D15. doi: 10.1093/nar/gky1049
25. Wishart DS, Feunang YD, Guo AC, Lo EJ, Marcu A, Grant JR, et al. DrugBank 5.0: A Major Update to the DrugBank Database for 2018. Nucleic Acids Res (2018) 46(D1):D1074–D82. doi: 10.1093/nar/gkx1037
26. Stelzer G, Dalah I, Stein TI, Satanower Y, Rosen N, Nativ N, et al. In-Silico Human Genomics With GeneCards. Hum Genomics (2011) 5(6):709–17. doi: 10.1186/1479-7364-5-6-709
27. Li YH, Yu CY, Li XX, Zhang P, Tang J, Yang Q, et al. Therapeutic Target Database Update 2018: Enriched Resource for Facilitating Bench-to-Clinic Research of Targeted Therapeutics. Nucleic Acids Res (2018) 46(D1):D1121–7. doi: 10.1093/nar/gkx1076
28. Barbarino JM, Whirl-Carrillo M, Altman RB, Klein TE. PharmGKB: A Worldwide Resource for Pharmacogenomic Information. Wiley Interdiscip Rev Syst Biol Med (2018) 10(4):e1417. doi: 10.1002/wsbm.1417
29. Amberger JS, Bocchini CA, Schiettecatte F, Scott AF, Hamosh A. OMIM.org: Online Mendelian Inheritance in Man (OMIM(R)), an Online Catalog of Human Genes and Genetic Disorders. Nucleic Acids Res (2015) 43(Database issue):D789–98. doi: 10.1093/nar/gku1205
30. He D, Huang JH, Zhang ZY, Du Q, Peng WJ, Yu R, et al. A Network Pharmacology-Based Strategy For Predicting Active Ingredients And Potential Targets Of LiuWei DiHuang Pill In Treating Type 2 Diabetes Mellitus. Drug Des Devel Ther (2019) 13:3989–4005. doi: 10.2147/DDDT.S216644
31. Du A, Xie Y, Ouyang H, Lu B, Jia W, Xu H, et al. Si-Miao-Yong-An Decoction for Diabetic Retinopathy: A Combined Network Pharmacological and. In Vivo Approach Front Pharmacol (2021) 12:763163. doi: 10.3389/fphar.2021.763163
32. Shi H, Dong C, Wang M, Liu R, Wang Y, Kan Z, et al. Exploring the Mechanism of Yizhi Tongmai Decoction in the Treatment of Vascular Dementia Through Network Pharmacology and Molecular Docking. Ann Transl Med (2021) 9(2):164. doi: 10.21037/atm-20-8165
33. Li Q, Hu S, Huang L, Zhang J, Cao G. Evaluating the Therapeutic Mechanisms of Selected Active Compounds in Cornus Officinalis and Paeonia Lactiflora in Rheumatoid Arthritis via Network Pharmacology Analysis. Front Pharmacol (2021) 12:648037. doi: 10.3389/fphar.2021.648037
34. Xu R, Zeng Q, Xia C, Chen J, Wang P, Zhao S, et al. Fractions of Shen-Sui-Tong-Zhi Formula Enhance Osteogenesis Via Activation of Beta-Catenin Signaling in Growth Plate Chondrocytes. Front Pharmacol (2021) 12:711004. doi: 10.3389/fphar.2021.711004
35. Fu F, Bao R, Yao S, Zhou C, Luo H, Zhang Z, et al. Aberrant Spinal Mechanical Loading Stress Triggers Intervertebral Disc Degeneration by Inducing Pyroptosis and Nerve Ingrowth. Sci Rep (2021) 11(1):772. doi: 10.1038/s41598-020-80756-6
36. Xia C, Zou Z, Fang L, Ge Q, Zhang P, Xu H, et al. Bushenhuoxue Formula Promotes Osteogenic Differentiation of Growth Plate Chondrocytes Through Beta-Catenin-Dependent Manner During Osteoporosis. BioMed Pharmacother (2020) 127:110170. doi: 10.1016/j.biopha.2020.110170
37. Zhu Y, Li Z, Zhang Y, Lan F, He J, Wu Y. The Essential Role of Osteoclast-Derived Exosomes in Magnetic Nanoparticle-Infiltrated Hydroxyapatite Scaffold Modulated Osteoblast Proliferation in an Osteoporosis Model. Nanoscale (2020) 12(16):8720–6. doi: 10.1039/d0nr00867b
38. Johnston CB, Dagar M. Osteoporosis in Older Adults. Med Clinics North Am (2020) 104(5):873–84. doi: 10.1016/j.mcna.2020.06.004
39. Vandenbroucke A, Luyten FP, Flamaing J, Gielen E. Pharmacological Treatment of Osteoporosis in the Oldest Old. Clin Interv Aging (2017) 12:1065–77. doi: 10.2147/cia.s131023
40. Wang X, Wang ZY, Zheng JH, Li S. TCM Network Pharmacology: A New Trend Towards Combining Computational, Experimental and Clinical Approaches. Chin J Natural Med (2021) 19(1):1–11. doi: 10.1016/s1875-5364(21)60001-8
41. Gao L, Cao M, Li JQ, Qin XM, Fang J. Traditional Chinese Medicine Network Pharmacology in Cardiovascular Precision Medicine. Curr Pharm design (2021) 27(26):2925–33. doi: 10.2174/1381612826666201112142408
42. Xia H, Liu J, Yang W, Liu M, Luo Y, Yang Z, et al. Integrated Strategy of Network Pharmacological Prediction and Experimental Validation Elucidate Possible Mechanism of Bu-Yang Herbs in Treating Postmenopausal Osteoporosis via ESR1. Front Pharmacol (2021) 12:654714. doi: 10.3389/fphar.2021.654714
43. Wong SK, Chin KY, Ima-Nirwana S. Quercetin as an Agent for Protecting the Bone: A Review of the Current Evidence. Int J Mol Sci (2020) 21(17):6448. doi: 10.3390/ijms21176448
44. Jing Z, Wang C, Yang Q, Wei X, Jin Y, Meng Q, et al. Luteolin Attenuates Glucocorticoid-Induced Osteoporosis by Regulating ERK/Lrp-5/GSK-3β Signaling Pathway In Vivo and In Vitro. J Cell Physiol (2019) 234(4):4472–90. doi: 10.1002/jcp.27252
45. Liu H, Yi X, Tu S, Cheng C, Luo J. Kaempferol Promotes BMSC Osteogenic Differentiation and Improves Osteoporosis by Downregulating miR-10a-3p and Upregulating CXCL12. Mol Cell Endocrinol (2021) 520:111074. doi: 10.1016/j.mce.2020.111074
46. Cai P, Lu Y, Yin Z, Wang X, Zhou X, Li Z. Baicalein Ameliorates Osteoporosis via AKT/FOXO1 Signaling. Aging (2021) 13(13):17370–9. doi: 10.18632/aging.203227
47. Liang J, Chen H, Pan W, Xu C. Puerarin Inhibits Caspase-3 Expression in Osteoblasts of Diabetic Rats. Mol Med Rep (2012) 5(6):1419–22. doi: 10.3892/mmr.2012.854
48. Vakili S, Zal F, Mostafavi-Pour Z, Savardashtaki A, Koohpeyma F. Quercetin and Vitamin E Alleviate Ovariectomy-Induced Osteoporosis by Modulating Autophagy and Apoptosis in Rat Bone Cells. J Cell Physiol (2021) 236(5):3495–509. doi: 10.1002/jcp.30087
49. Sillars-Hardebol AH, Carvalho B, Beliën JA, de Wit M, Delis-van Diemen PM, Tijssen M, et al. BCL2L1 has a Functional Role in Colorectal Cancer and its Protein Expression is Associated With Chromosome 20q Gain. J Pathol (2012) 226(3):442–50. doi: 10.1002/path.2983
50. Moriishi T, Fukuyama R, Miyazaki T, Furuichi T, Ito M, Komori T. Overexpression of BCLXL in Osteoblasts Inhibits Osteoblast Apoptosis and Increases Bone Volume and Strength. J Bone Mineral Res Off J Am Soc Bone Mineral Res (2016) 31(7):1366–80. doi: 10.1002/jbmr.2808
51. Yu T, You X, Zhou H, Kang A, He W, Li Z, et al. P53 Plays a Central Role in the Development of Osteoporosis. Aging (2020) 12(11):10473–87. doi: 10.18632/aging.103271
52. Yu T, Wu Q, You X, Zhou H, Xu S, He W, et al. Tomatidine Alleviates Osteoporosis by Downregulation of P53. Med Sci Monitor Int Med J Exp Clin Res (2020) 26:e923996. doi: 10.12659/msm.923996
53. Xiao L, Lin J, Chen R, Huang Y, Liu Y, Bai J, et al. Sustained Release of Melatonin From GelMA Liposomes Reduced Osteoblast Apoptosis and Improved Implant Osseointegration in Osteoporosis. Oxid Med Cell Longevity (2020) 2020:6797154. doi: 10.1155/2020/6797154
54. Wang Y, Liu J, Pang Q, Tao D. Alpinumisoflavone Protects Against Glucocorticoid-Induced Osteoporosis Through Suppressing the Apoptosis of Osteoblastic and Osteocytic Cells. BioMed Pharmacother (2017) 96:993–9. doi: 10.1016/j.biopha.2017.11.136
55. Kong Y, Nie ZK, Li F, Guo HM, Yang XL, Ding SF. MiR-320a was Highly Expressed in Postmenopausal Osteoporosis and Acts as a Negative Regulator in MC3T3E1 Cells by Reducing MAP9 and Inhibiting PI3K/AKT Signaling Pathway. Exp Mol Pathol (2019) 110:104282. doi: 10.1016/j.yexmp.2019.104282
56. Zhang Y, Cao X, Li P, Fan Y, Zhang L, Li W, et al. PSMC6 Promotes Osteoblast Apoptosis Through Inhibiting PI3K/AKT Signaling Pathway Activation in Ovariectomy-Induced Osteoporosis Mouse Model. J Cell Physiol (2020) 235(7-8):5511–24. doi: 10.1002/jcp.29261
Keywords: osteoporosis, Zhuanggu Busui formula, network pharmacology, pharmacological mechanisms, apoptosis, PI3K-Akt signalling
Citation: Zhang H, Zhou C, Zhang Z, Yao S, Bian Y, Fu F, Luo H, Li Y, Yan S, Ge Y, Chen Y, Zhan K, Yue M, Du W, Tian K, Jin H, Li X, Tong P, Ruan H and Wu C (2022) Integration of Network Pharmacology and Experimental Validation to Explore the Pharmacological Mechanisms of Zhuanggu Busui Formula Against Osteoporosis. Front. Endocrinol. 12:841668. doi: 10.3389/fendo.2021.841668
Received: 22 December 2021; Accepted: 30 December 2021;
Published: 28 January 2022.
Edited by:
Jun Liu, Guangdong Provincial Academy of Chinese Medical Sciences, ChinaReviewed by:
Xing Ji, Children’s Hospital of Philadelphia, United StatesYan Wu, Zhejiang Provincial Key Laboratory of Diagnosis and Treatment of Sports System Diseases, China
Yong Ma, Nanjing University of Chinese Medicine, China
Copyright © 2022 Zhang, Zhou, Zhang, Yao, Bian, Fu, Luo, Li, Yan, Ge, Chen, Zhan, Yue, Du, Tian, Jin, Li, Tong, Ruan and Wu. This is an open-access article distributed under the terms of the Creative Commons Attribution License (CC BY). The use, distribution or reproduction in other forums is permitted, provided the original author(s) and the copyright owner(s) are credited and that the original publication in this journal is cited, in accordance with accepted academic practice. No use, distribution or reproduction is permitted which does not comply with these terms.
*Correspondence: Hongfeng Ruan, cmhmQHpjbXUuZWR1LmNu; Peijian Tong, cGVpamlhbnRvbmd6anRjbUAxNjMuY29t; Xiaofeng Li, bGl4aWFvZmVuZzA0MDlAMTYzLmNvbQ==
†These authors have contributed equally to this work