- 1Institute of Biophysics Carlos Chagas Filho, Universidade Federal do Rio de Janeiro, Rio de Janeiro, Brazil
- 2Postgraduate Program in Nutritional Sciences, Department of Nutrition, Center for Health Sciences, Universidade Federal da Paraíba, João Pessoa, Brazil
- 3Campus Duque de Caxias Professor Geraldo Cidade, Universidade Federal do Rio de Janeiro, Rio de Janeiro, Brazil
- 4Department of Biorregulation, Health Sciences Institute, Universidade Federal da Bahia, Salvador, Brazil
Coronavirus disease 2019 (COVID-19) was characterized as a pandemic in March, 2020 by the World Health Organization. COVID-19 is a respiratory syndrome that can progress to acute respiratory distress syndrome, multiorgan dysfunction, and eventually death. Despite being considered a respiratory disease, it is known that other organs and systems can be affected in COVID-19, including the thyroid gland. Thyroid gland, as well as hypothalamus and pituitary, which regulate the functioning of most endocrine glands, express angiotensin-converting enzyme 2 (ACE2), the main protein that functions as a receptor to which SARS-CoV-2 binds to enter host cells. In addition, thyroid gland is extremely sensitive to changes in body homeostasis and metabolism. Immune system cells are targets for thyroid hormones and T3 and T4 modulate specific immune responses, including cell-mediated immunity, natural killer cell activity, the antiviral action of interferon (IFN) and proliferation of T- and B-lymphocytes. However, studies show that patients with controlled hypothyroidism and hyperthyroidism do not have a higher prevalence of COVID-19, nor do they have a worse prognosis when infected with the virus. On the other hand, retrospective observational studies, prospective studies, and case reports published in the last two years reported abnormal thyroid function related to acute SARS-CoV-2 infection or even several weeks after its resolution. Indeed, a variety of thyroid disorders have been documented in COVID-19 patients, including non-thyroidal illness syndrome (NTIS), subacute thyroiditis and thyrotoxicosis. In addition, thyroid disease has already been reported as a consequence of the administration of vaccines against SARS-CoV-2. Overall, the data revealed that abnormal thyroid function may occur during and in the convalescence post-COVID condition phase. Although the cellular and molecular mechanisms are not completely understood, the evidence suggests that the “cytokine storm” is an important mediator in this context. Thus, future studies are needed to better investigate the pathophysiology of thyroid dysfunction induced by COVID-19 at both molecular and clinical levels.
1. Introduction
In December, 2019, a pneumonia of unknown origin emerged in Wuhan, China. On January, the virus responsible for the pneumonia was identified as a new coronavirus, later named severe acute respiratory syndrome coronavirus 2, SARS-CoV-2, and the disease was named coronavirus disease 2019 (COVID-19) (1). The genetic material of the virus was rapidly sequenced (2), and the transmission could not be prevented. The viruses rapidly spread across China and then all over the world, so on March 11th 2020, World Health Organization (WHO) declared that COVID-19 reached pandemic levels. Since then, life on earth was totally affected by the pandemic, with changes in the way humans interact with each other, millions of deaths, ills and a great economic impact. On August 20th, 2022, according to WHO, the number of confirmed cases was higher than 595 million and confirmed deaths surpassed 6.4 million.
Most coronavirus strains that infect humans cause a mild respiratory disease; however, SARS-CoV-2 causes a serious illness, which can lead to severe respiratory syndrome and eventually to death. The most frequent symptoms include fever, dyspnea, sore throat, anosmia, dysgeusia, fatigue, and can progress to pneumonia, acute respiratory distress syndrome, and multiorgan dysfunction. SARS-CoV-2 genetic material is a positive-sense single-stranded RNA and the virus consists of a spherical particle, enveloped, with a diameter of approximately 120 nm (3). The origin of SARS-CoV-2 is controversial and the Huanan seafood wholesale market in Wuhan was suggested to be the place where the virus jumped to humans, with bat (Rhinolophus affinis) and pangolin (Manis javanica) being most probably the natural and intermediate hosts, respectively (4).
2. COVID-19 and Thyroid gland: General concepts
Despite being initially described as a respiratory disease, in the course of time it was observed that other organs and systems could be affected by COVID-19, such as cardiovascular system (5), central nervous system (6), kidney (7), liver (8), among others. Endocrine system is also affected by COVID-19, including pancreas, adrenal, testicle, reproductive tract, parathyroid gland and the thyroid gland (9–14) (Figure 1). Moreover, endocrine-metabolic disturbances, such as diabetes mellitus and obesity, are highly associated to severe illness (15, 16).
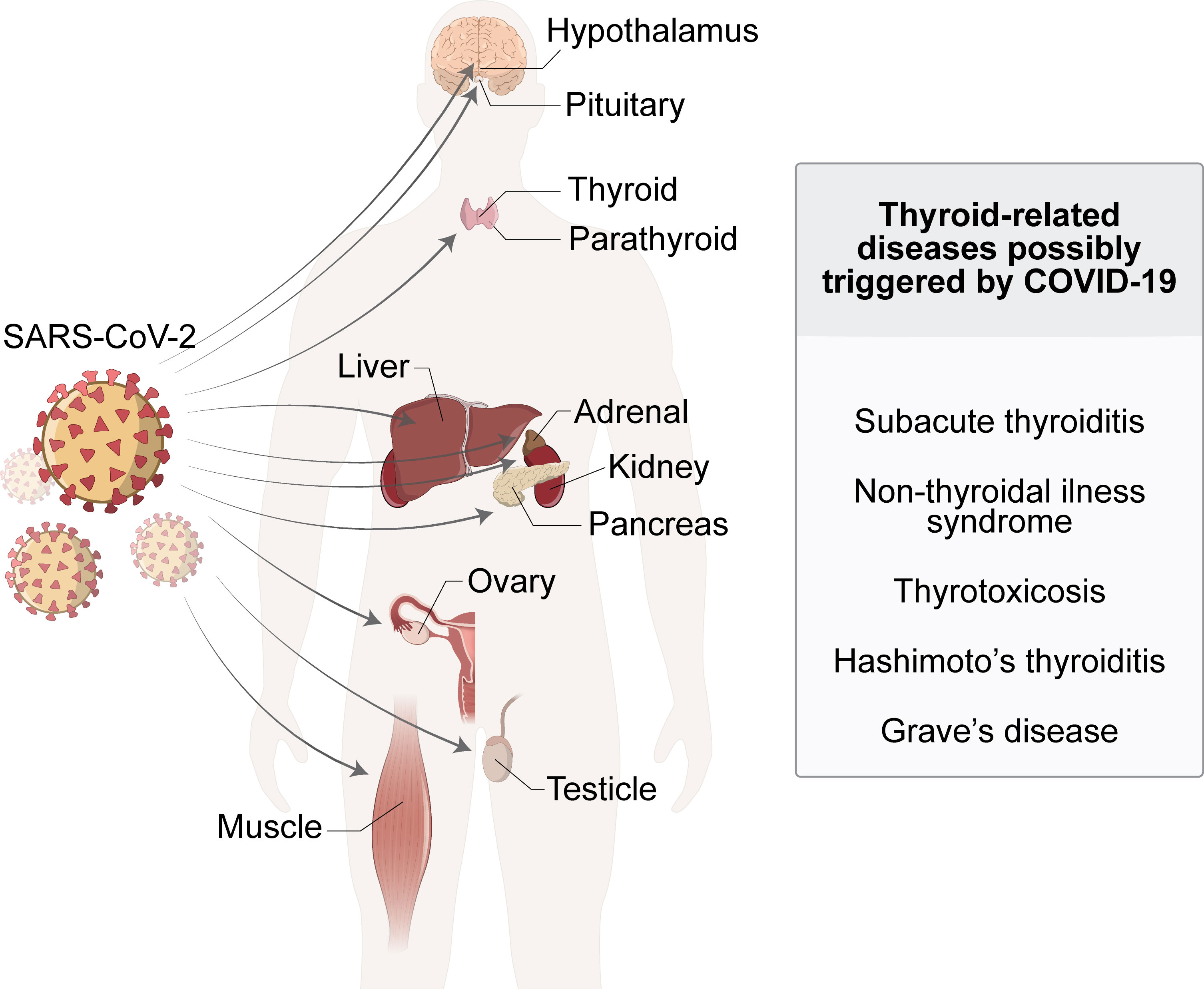
Figure 1 SARS-Cov-2 infection affects and damages a wide-ranging of human organs and systems. The manifestation of endocrine disorders, including thyroid-related pathologies, have been diagnosed in COVID-19 patients, suggesting a possible causal relationship between these conditions.
It is not surprising that the endocrine system can be affected by SARS-CoV-2 since both hypothalamus and pituitary, which regulate the functioning of most endocrine glands, express angiotensin-converting enzyme 2 (ACE2), the main protein to which SARS-CoV-2 binds to enter host cells (17, 18). ACE2 is a transmembrane protein with carboxypeptidase activity, which cleaves angiotensin I to angiotensin 1-9 and angiotensin II to angiotensin 1-7. The viral envelope contains a spike glycoprotein, which interacts with ACE2 with high specificity and affinity, which contributes to the high transmissibility and infectivity of SARS-CoV-2. Then, virus particle enters the cell by endocytosis or by fusion of the viral envelope with the cell membrane. The spike protein is not cleaved, thus the enzymatic activity of ACE2 is not relevant for the virus entry in host cell (19).
Besides hypothalamus and pituitary, thyroid gland also express ACE2 and may be directly affected by COVID-19 (20, 21). Thyroid gland is responsible for the production of thyroid hormones: the prohormone tetraiodothyronine (T4) and the active hormone triiodothyronine (T3). In fact, around 90% of the circulating T3 in humans is produced by the peripheral conversion of T4 to T3, by enzymes called deiodinases. T3 and some of its metabolites are the main regulators of basal metabolic rate, with effects on central nervous system, cardiovascular system, respiratory system, skeletal muscles, among others.
Thyroid hormones regulate to some extent the immune system (22). It has already been shown that one of the targets of thyroid hormones are immune system cells and that THs modulate specific immune responses, including cell-mediated immunity, natural killer cell activity, the antiviral action of interferon (IFN) and proliferation of T- and B-lymphocytes (22–24). In healthy subjects, there is a positive correlation between serum thyroid hormone levels and inflammatory markers, monocyte-activated IL-6 expression, the percentage of memory T cells, the quantity of natural killer T cells and the quantities of CD3+/CD4+/CD45RO+ memory T helper cells. On the other hand, serum thyroid hormones are negatively correlated with lymphocyte death and to the ratio of naïve: cytotoxic CD3+/CD8+/CD45RO+ memory T cells (25). These data suggest that THs stimulate the immune system to strongly react to infection.
Even though it has already been shown that hypothyroidism and hyperthyroidism have opposite effects on some parameters of the immune response, there is no evidence that patients with poorly controlled thyroid disorders are more susceptible to contract viral infections. However, considering the role of thyroid hormones on the immune system, it is plausible that patients with uncontrolled thyroid dysfunction may be at higher risk of complications due to these infections (26). Contrasting results have been reported for other immune functions, and so it is difficult to establish a clear correlation between immune function and hyper- or hypothyroid conditions (22). Overall, hypothyroidism tends to impair the activation of the immune system while hyperthyroidism results in the activation of the immune response (27).
Therefore, thyroid function could have an impact in the prognosis of COVID-19 and conversely COVID-19 could have an impact on thyroid function. In fact, abnormal thyroid function has been reported during SARS-CoV-2 infection or even several weeks after its resolution. Indeed, a variety of thyroid disorders have been documented in COVID-19 patients including non-thyroidal illness syndrome (NTIS), subacute thyroiditis (SAT), thyrotoxicosis and hypothyroidism in retrospective observational studies and case-reports (Figure 1). Although a cause-effect association between the infection and the onset of thyroid dysfunction has not yet been demonstrated from a mechanistic point-of-view, these reports raise the concern about whether thyroid function should or not receive a special attention in COVID-19 patients. Herein, we review important aspects of the relationship between COVID-19 and thyroid, including the interaction of thyroid hormones with immune system, the effect of infectious agents on the incidence of thyroid disorders and recent data regarding the relationship between COVID-19 and thyroid.
3. Pre-existing thyroid dysfunction and COVID-19
3.1. Hypothyroidism
Hypothyroidism is the insufficient production of thyroid hormones. This disease can be congenital, due to mutations in proteins that are essential for thyroid hormones synthesis pathway and defects in thyroid gland formation, or even the absence of the gland. Hypothyroidism can also be acquired, due to iodide deficiency, tumors or infections in the thyroid gland or in the pituitary, and autoimmunity. Hashimoto’s thyroiditis is an idiopathic thyroid atrophy due to a chronic autoimmune inflammatory reaction, being the most common form of hypothyroidism in humans (28). Hashimoto’s autoimmune thyroid disease is characterized by the production of autoantibodies against thyroglobulin (Tg-Ab) and thyroperoxidase (Tpo-Ab) that are essential for hormonal synthesis. Hypothyroid patients have lower metabolic rate, decreased thermogenesis, bradycardia, lethargy and drowsiness (29).
Animal models have shown that the experimental induction of hypothyroidism leads to an involution of the spleen and lymph nodes as well as a decrease in the humoral and cell-mediated immune response (30, 31). Hypothyroidism induced by chronic restraint stress seems to be related to the reduction of T-cell lymphoproliferative response, since T4 replacement reversed it. Besides, in these chronic stress mice bearing tumors, T4 reversed the alteration of lymphoma growth, interleukin-2 production and specific cytotoxic response against tumor cells (32). Furthermore, B-lymphocytes can also be regulated by thyroid hormones. In mouse strains deficient in the production of anterior pituitary-derived hormones, and consequently secondary hypothyroid, the frequency and absolute number of pro-B- and B-lymphocytes are lower, showing that THs can regulate the proliferative potential of T- and B-lymphocytes (33–35). Clinically, patients with severe hypothyroidism due to autoimmune thyroiditis experience a dramatic decrease in lymphocyte function, which is restored when T4 is normalized by exogenous hormone administration (36).
Considering that hypothyroidism leads to immune system dysfunctions, and that ACE2 is expressed in thyroid gland, one could speculate that hypothyroidism might impact the outcomes in COVID-19 patients. A retrospective study conducted in the New York City health system evaluated a cohort of 3703 COVID-19 patients, of which 251 patients (6.8%) had pre-existing hypothyroidism. The authors found that hypothyroidism was not associated with increased risk of hospitalization or an increased risk of mechanical ventilation or death (37). Other studies have also shown that the prevalence of hypothyroidism appears similar in COVID-19 patients compared to the general population, which indicates that hypothyroidism does not increase the chance of COVID-19 infection, and also that hypothyroidism is not associated with a greater COVID-19 death risk (38, 39). Despite this, previous studies show that, although well-managed hypothyroidism is not associated with increased infection risk, poorly controlled hypothyroidism may increase the susceptibility to infections (36, 40). Therefore, it is important that patients with thyroid disorders maintain their treatment during the COVID-19 pandemic.
3.2. Hyperthyroidism
Hyperthyroidism is characterized by higher levels of circulating thyroid hormone. It is mostly an acquired condition, which is most frequently caused by Graves’ disease, toxic multinodular goiter or toxic adenoma (41). Graves’ disease is an autoimmune disorder, in which thyroid-stimulating antibodies activate the thyroid-stimulating hormone (TSH) receptors, triggering increased thyroid hormone synthesis. The clinical condition observed in Graves’ disease is thyrotoxicosis, resulting from excessive amounts of thyroid hormones in the tissues and blood. In those patients, whole-body metabolism is activated leading to body weight loss, sweating, heat intolerance, increased heart rate, overactive bowel movement, tremor, nervousness, and exophthalmos (42).
Hyperthyroidism is associated with unbalanced immune responses, including abnormal antibody production (either increased or decreased) (43), increased migration of polymorphonuclear leukocytes (44), increased lymphocyte proliferation (45) and increased macrophages reactive oxygen species (ROS) production (27, 46). Compared to healthy controls, hyperthyroid patients present higher levels of serum immunoglobulins M and G (IgM, IgG) and higher levels of p65 and p-IκBα in B-lymphocytes, which are indicators of NF-κB activation. In addition, these patients have higher serum oxidative stress levels (47). These results suggest that hyperthyroidism increases ROS production activating the NF-κB pathway that, in turn, enhances the production of Ig’s by B-lymphocyte.
Due to this hyper-responsiveness of the immune system during hyperthyroidism, it is plausible that uncontrolled hyperthyroid patients, especially with thyrotoxicosis, may be at higher risk of complications from any infection (42). On the other hand, angiotensin converting enzyme activity and the counter-regulatory components of the RAS (renin-angiotensin system) is increased in patients with hyperthyroidism (48, 49). A study conducted in China shows that COVID-19 patients with thyroid disease had a significantly higher fatality rate (20% vs 0%) and they were more likely to stay in the hospital for more than 28 days than were those without thyroid disease (80% vs 56.52%) (50). However, the authors defined thyroid disease as an abnormal thyroid function test result, and included patients with overt thyrotoxicosis, overt hypothyroidism, subclinical hypothyroidism, subclinical hyperthyroidism, and euthyroid sick syndrome. Therefore, it is not possible to estimate the contribution of each thyroid disease to a worse prognosis of COVID-19.
In general, previously published data show that patients with controlled hyperthyroidism are not considered to be at higher risk of contracting COVID-19 (26, 51, 52), but there are two exceptions. It is known that patients taking antithyroid drugs present higher risk of developing neutropenia or agranulocytosis, which occurs in 0.2-0.5% of patients taking these medications (42, 53). Neutropenia is associated with increased risk of infections. Therefore, patients with neutropenia caused by the administration of antithyroid drugs may be more prone to complications during COVID-19 infection due to reduced immune response. Likewise, patients with Graves’ ophthalmopathy who are undergoing immunosuppressive agents, like glucocorticoids, may also be considered to be more vulnerable to COVID-19 infection (26, 51). Additionally, since thyroid hormones regulate vascular tonus and multi-organ dysfunction is associated to hypoxia, T3 treatment has been suggested to be potentially useful in the treatment of severe COVID-19 (54).
4. Thyroid dysfunction during COVID-19
4.1. Non-thyroidal illness syndrome (NTIS) and COVID-19
The first reports of abnormal serum thyroid hormone concentrations after severe illness or starvation in patients with no history of thyroid disease has been made nearly 60 years ago (55, 56). In mild-to-moderate illness, the most typical laboratory finding is a reduction in serum T3 and, remarkably, no concomitant increase in TSH. Accordingly, this condition has been named “low T3 syndrome”, “euthyroid sick syndrome” or “non-thyroidal illness syndrome”. Elevated reverse T3 (rT3) has long been considered another hallmark of NTIS, but now some authors argue that its levels may be normal or even reduced in some patients with NTIS (57). Reduced levels of rT3 have already been observed in AIDS patients that present NTIS, and this phenomenon has also been observed in COVID-19 and NTIS patients (58–60). Additionally, a decline in T4 and TSH may also be observed in critically ill patients. Thus, NTIS is a complex condition with no unique phenotype, which greatly depends on disease severity. Pro-inflammatory cytokines, like interleukin-6 and IL-1β, are recognized as major players in the pathogenesis of NTIS since the 90’s, when an inverse correlation between serum T3 and IL-6 in hospitalized patients was first observed (61). Moreover, the chronic treatment of rodents with these cytokines recapitulated several hallmarks of human NTIS, including low T3, T4 and hypothalamic TRH mRNA expression (62, 63).
The entire hypothalamus-pituitary-thyroid (HPT) axis is profoundly affected by severe illness (Figure 2). At the hypothalamic level, TRH decrease is largely mediated by a T3-induced feedback mechanism in illness. The TH transporters MCT10 and OATP1C1 are upregulated in the hypothalamus of prolonged ill rabbits, which may further contribute to the negative feedback (64). Several subsequent animal studies using different illnesses models revealed a consistent upregulation of iodothyronine deiodinase 2 (D2) both in the PVN and in tanycytes, suggesting an increase in local T3 production, which helped explain the abnormal negative feedback resulting in TRH downregulation besides low levels of serum T3 (65, 66). Deiodinase 3 (D3) is negatively regulated by LPS in the PVN of mice and in cultured neuroblastoma cells, leading to increased intracellular T3 levels in these cells (67, 68). Although logical, the increase in intra-hypothalamic levels of T3 still lacks direct experimental evidence in vivo. At the pituitary level, illness leads to an impaired TSH response to low levels of circulating T3 and T4 (64) and lack of pulsatile secretion (69). This disturbed TSH response can be partially attributed to diminished TRH stimulus and to a direct effect of cytokines like IL-1β and TNF-α (70, 71). The contribution of pituitary’s D2 and/or D1 activity to these responses is still disputable.
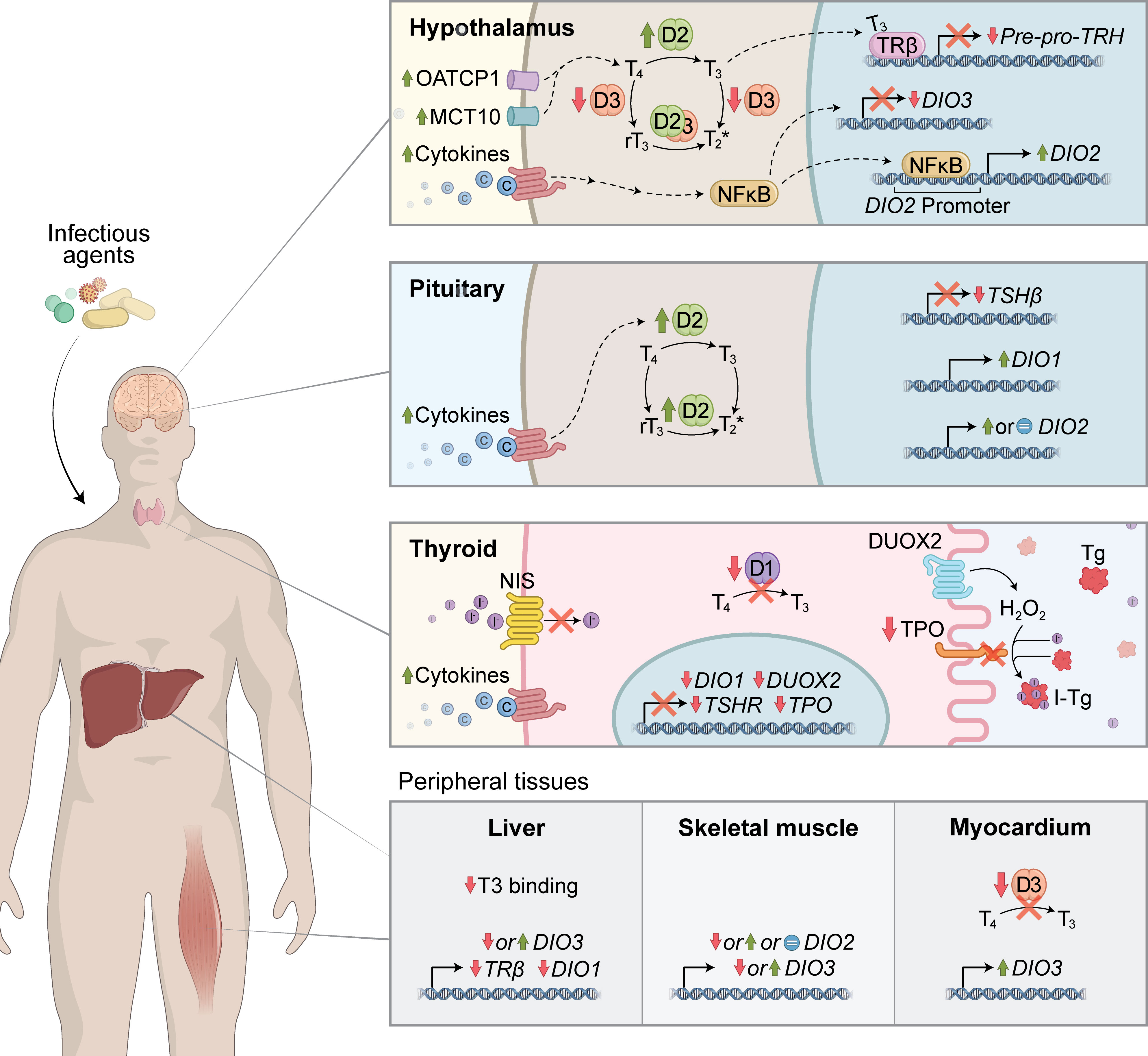
Figure 2 Mechanistic insights into COVID-19-induced Non-thyroidal illness syndrome (NTIS). Multiple mechanisms might be involved in the pathogenesis of NTIS. During severe illness, the hypothalamus-pituitary-thyroid (HPT) axis is profoundly affected, which is primarily mediated by pro-inflammatory cytokines. In the hypothalamus, illness promotes TRH downregulation due to abnormal T3-induced negative feedback. Potential mechanisms involved include increased local T3 production by D2 and increased expression in TH transporters (MCT10 and OATP1C1). At the pituitary level, illness impairs TSH response to low levels of circulating T3 and T4, which can be secondary to reduced TRH levels or the direct effect of pro-inflammatory cytokines. Modulation of deiodinase activity might be additionally involved. Cytokines also inhibit multiple steps of TH biosynthesis and D1 activity in the thyroid gland, decreasing hormonal synthesis and secretion. In other peripheral tissues, suppressed D1 and increased D3 expression/activity could contribute to low T3 and high rT3 levels observed in sick individuals.
The thyroid gland is also directly influenced by illness (Figure 2). Data from a vast collection of in vivo and in vitro models report that cytokines (e.g., IL-1α, IL-1β, TNF-α, IFNγ) can inhibit several steps of the TH synthesis machinery, including TSH receptor expression (72), iodide incorporation by NIS (73–75), iodide organification by TPO (76–78), DUOX expression (77), thyroglobulin production (79) and deiodinase 1 expression and activity (80–82). Collectively, these effects lead to a decrease in the synthesis and secretion of TH by the thyroid gland.Yet, most of the circulating T3 is not produced by the gland itself but through deiodination of T4 in peripheral tissues, such as liver, kidney and muscle (83, 84). During illness, alterations in the expression and function of D1 (85, 86), D2 (85, 87–89) and D3 (85, 86) might also contribute to serum TH abnormal levels (Figure 2).
There is still limited data on the literature outlining NTIS during viral infectious diseases. Although the few reports involve different populations and different viruses, they congregate in terms of clinical manifestations. In a cohort of HIV+ and AIDS patients, fT3 levels were lower than in healthy controls while calculated TBG capacity was increased (59). A subsequent study from Nigeria showed that, among 108 HIV-1+ individuals, 52% had abnormalities in thyroid function. Of these, 8.5% had subclinical hypothyroidism and 45.5% had NTIS. The HIV−1+ individuals had significantly lower TSH, T3 and T4 when compared with HIV-1- controls (90).
Viruses that attack the respiratory tract have been also linked to the development of NTIS. The influenza A virus subtype H7N9 (A/H7N9) is a bird flu strain of the Influenza virus A (avian influenza) that infected humans in China in 2013. Of patients infected with H7N9, 70.6% presented abnormally low total T3 levels, 58.8% had low free T3 and TSH levels and 29.4% had abnormally low total and free T4 levels (below the lower limit of the reference ranges for each hormone) (91).
At the time of this publication, thyroid function tests of more than 2,000 COVID-19 patients have been reported in the literature. Collectively, they make clear that low serum fT3 and NTIS at admission strongly predict poor outcomes in these patients, although the utility of measuring fT3 at admission is still disputable in terms of cost-effectiveness since other biochemical indicators provide similar predictive value. Here we present some of the relevant clinical data regarding NTIS in COVID-19 patients.
Only three months after the beginning of the outbreak the first report of altered thyroid hormones in COVID-19 patients was presented in a retrospective analysis of 274 COVID-19 cases in the region of Wuhan. The authors revealed that serum levels of fT3 and TSH were significantly diminished in deceased patients compared to recovered patients, even though the decrease in TSH was still within the normal range (92). Similar observations were consistently reported in several other papers, although the incidence of decreased levels of fT3 and NTIS varied greatly, probably as a result of the huge discrepancies in the disease severity of the cohort included in each study.
In a study with 50 patients confirmed with moderate to critical COVID-19 with no history of thyroid disease, Chen et al. reported altered thyroid function in more than 60% of patients (93). Low TSH with or without lower-than-normal levels of tT3 were the most frequent alterations found in these patients. Interestingly, TSH and tT3 levels were lower in patients with COVID-19 when compared with healthy patients and non-COVID-19 pneumonia patients, suggesting that these clinical observations could be characteristic of SARS-CoV-2 infection. However, it is important to highlight that in this study thyroid function was evaluated while most of COVID-19 patients were under glucocorticoid treatment, which may affect thyroid function. In another cohort, Zhang and collaborators identified thyroid disorders in 28% of 71 COVID-19 patients, which included mainly NTIS (48%) and subclinical hypothyroidism (28%) (50). In agreement, NTIS was also found in more than 25% of COVID-19 in another Chinese cohort and its occurrence was associated with inflammation and disease severity (94).
A retrospective study published in October of 2020 conducted in Changsha (China) analyzed clinical and laboratory data of 149 patients with mild COVID-19 infection within the first 3 months of the pandemic and detected that 28% had NTIS, characterized by fT3<2.3 pg/mL and low or normal TSH. Compared to non-NTIS patients, NTIS-patients had lower tT3 (0.66 vs. 0.96 ng/mL, p<0.0001), tT4 (8.3 vs. 9.5 µg/dL, p<0.0001), and non-statistically significant lower TSH (1.36 vs. 1.74 µIU/mL, p = 0.06). Also, NTIS patients had a higher ESR, CRP and lymphopenia. NTIS was identified as an independent risk factor for disease severity by Cox-regression model (HR = 2.5 [95%CI 1.05-6.02]) and receiver operating characteristic (ROC) analysis (AUC = 0.81) (94). Another study searched for predictors of mortality in 121 ICU-admitted severe COVID-19 patients and detected that fT3 was the second-best predictor of death (AUC from ROC = 0.86), only after Sequential Organ Failure Assessment (SOFA) (AUC from ROC = 0.96) (95). Similar results were obtained in a cohort of moderately severe COVID-19 patients in which only 31.5% were admitted to the ICU (AUC from ROC = 0.84) (96).
Likewise, Gao et al. followed 100 COVID-19 patients (66% of then were severely or critically ill) and showed that fT3, TSH and fT3/fT4 ratio decreased with clinical deterioration and were lower in non-survivors. Moreover, the reduction in fT3 levels was independently associated with all-cause mortality (97). The prospective analysis of 115 COVID-19 patients in Italy, in which 18% had NTIS also observed that low fT3 was associated with mortality and inflammation. The following of these patients thought the hospitalization revealed that, not unexpectedly, the number of patients with decreased levels of fT3 and TSH increased during hospitalization. However, most of them were under corticosteroids therapy, emphasizing the need for cautious interpretation of TSH and TH after ICU admission (98).
A recent prospective analysis of 245 hospitalized patients with moderate or critical (ICU-admitted) COVID-19 patients in Brazil corroborated previous findings that fT3 is negatively associated with survival. Similar to previous findings, only 6.5% of patients had NTIS (characterized as fT3<2.0 pg/mL and low or normal TSH) but this diagnosis significantly increased the risk of death (OR = 7.05). This was the first study to measure rT3 levels in COVID-19 patients and it revealed that they were elevated (>0.35 ng/mL) in 63% of the patients and were significantly higher in critical patients compared to non-critical patients. Interestingly, although rT3 is frequently considered a hallmark of NTIS and NTIS increases the risk of death by COVID-19, serum rT3 levels were not associated to death risk, but the opposite. Patients with simultaneously fT3<2.6 pg/mL and rT3<0.38 ng/mL had a significantly worse clinical outcome (36% mortality rate) than patients with only fT3<2.6 pg/mL (17%), only rT3<0.38 ng/mL (20%), or none of these alterations (5%). Moreover, the authors showed that the value obtained from the mathematical product of T3 × rT3 had the strongest predictive value for mortality amongst all analyzed parameters (AUC from ROC = 0.7). Remarkably, the OR for death in patients with T3×rT3<1.29 was 8.08 (60).
These results shed light on a possible underappreciated protective role of rT3 in critically ill patients. Rastogi et al. (2018) evaluated the efficacy of intravenous rT3 administration as a neuroprotective agent in rat model of middle cerebral artery occlusion induced cerebral ischemia-reperfusion and in an in vitro model of oxygen glucose deprivation/reoxygenation. The authors demonstrated that the administration of rT3 significantly reduced markers of neuronal injury, oxidative stress [levels of malondialdehyde, glutathione and reactive oxygen species (ROS)], infarct size and neurological deficit after ischemic insult (99). The authors’ explanation would be the inhibition of rT3-induced DIO2 synthesis, with a decrease in T3 action at the brain level, reducing O2 consumption and oxidative stress. Interestingly, this finding contrasts with the prognostic value of rT3 in other conditions such as acute myocardial infarction, in which rT3 > 0.27 ng/mL increases the risk of 1-year mortality (HR = 3.0) (100). Of note, a rapid decrease in rT3 has also been observed in animal models of acute illness by turpentine-induced sterile abscess (67) and in critically ill HIV+ patients with or without secondary infections (59).
Consistent data has been obtained about the prognostic value of thyroid hormones abnormalities during COVID-19 illness and their impact on patient outcome. However, no advances have been obtained yet regarding the mechanisms accountable for fT3 reduction. More specifically, the contribution of thyroid hormone production and peripheral tissue TH metabolism (both through deiodination and minor metabolization routes) and also the role of TH metabolites like rT3. Increased rT3 is often observed in critically ill patients and is mostly attributed to decreased liver D1 activity and, with less certainty, to increased liver D3 (85). However, the finding by Beltrão et al. (2021) that decreased levels of rT3 leads to less favorable outcomes in COVID-19 raises the question of whether and how disturbances in TH metabolism in peripheral tissues may impact the course of the disease (60).
Recently, Beltrão et al. (2022) showed a protective role of the polymorphic variant DIO2 (Thr92Ala) heterozygous state in COVID-19 mortality. In his study, heterozygous in-hospital patients were protected by 47-62% of risk mortality. The protective role of Thr92Ala’s heterozygous advantage was supported in a meta-analysis of 21 studies on thousands of cases with different diseases, such as ischemic stroke, myocardial infarction, and left ventricular hypertrophy. This protection could be explained by the gene Thr92Ala-DIO2 expression association with endoplasmic reticulum stress, inflammation, oxidative stress, apoptosis, and mitochondrial dysfunction that are mechanisms also related to the pathophysiology of COVID-19 (101).
It must also be highlighted that even mild decreases in fT3 that remain within the normal range can indicate a poor clinical outcome. Schwarz et al. (2021) divided a small cohort of COVID-19 patients into fT3 tertiles and observed that the bottom tertile (that included patients below the normal range and in the lower part of the normal range) had a mortality rate 6-times higher than the two greater tertiles (96). Other studies also proposed fT3 cut-off values above the bottom limit of the normal reference range that efficiently indicated increased risk of death in COVID-19 (60, 95).
Several studies were published about COVID-19 patients and poor outcomes related to thyroid dysfunction during hospitalization. However, it is still unclear if the relation is accurate. To answer this question, we did a systematic review. We only selected studies with a sample of adults and greater than 50 subjects, excluding studies with children and pregnant women. After applying the eligibility criteria, we found 27 studies on the subject, counting 4554 patients. Our research focused on two main types of studies: (i) retrospective studies (Table 1) and prospective studies (Table 2). Most studies were retrospective (18 studies). The number of patients evaluated in the studies ranged from 50 to 506. Most patients were critical and had several comorbidities (mainly hypertension and DM), and the mortality rate ranged from 0 to 29%. In thyroid function assessment in-hospital, most studies evaluated the levels of TSH, fT4, and fT3 in their patients, while only two studies evaluated rT3 and thyroglobulin. Some patients were diagnosed with NTIS and thyrotoxicosis during hospitalization, and the prevalence ranged from 1.7-66.3% and 0-28%, respectively (Tables 1, 2).
The abnormalities in TH and TSH in COVID-19 seem to be, as for other critical illnesses, transient. Khoo et al. (124) compared the levels of T4 and TSH at admission and after COVID-19 recovery with the patient-matched baseline level assayed in 2019 (i.e., before the pandemic) and confirmed that after recovery serum hormone levels returned to baseline. The lack of knowledge about the precise role of thyroid hormone fluctuations during illness makes it challenging to estimate the potential of a TH replacement therapy in COVID-19. An ongoing randomized placebo-controlled clinical trial (NCT04348513) aims to investigate whether the administration of T3 (liothyronine, 0.8g/kg i.v.) to ICU-admitted COVID-19 patients alleviates their need for cardiorespiratory support (54).
Importantly, most of the above-mentioned studies presented clinical data of patients admitted throughout the first 6 months of 2020, when SARS-CoV-2 variants of concern (VOC) were still not widespread. Whether the clinical course of patients infected by VOC will differ from the course of the disease caused by the original strain is still to be elucidated.
4.2. Subacute thyroiditis
Subacute Thyroiditis (SAT), also known as De Quervein’s thyroiditis or subacute granulomatous thyroiditis, is a self-limited inflammatory disorder of the thyroid gland that usually disappears in a few months. Patients usually show neck pain and enlarged thyroid and tenderness upon palpation. Symptoms as low fever, fatigue, malaise and myalgia are common (125). The development of subacute thyroiditis has been linked to viral infection or post inflammatory reaction to several different viruses, including mumps virus, measles virus, rubella virus, adenovirus, cytomegalovirus, enterovirus, Coxsackie virus, HIV, influenza virus and dengue fever virus (126). The inflammatory process causes damage and rupture of follicular cells, which leads to the release of T3 and T4 in circulation, inducing thyrotoxicosis symptoms in the first weeks of disease (Figure 3).
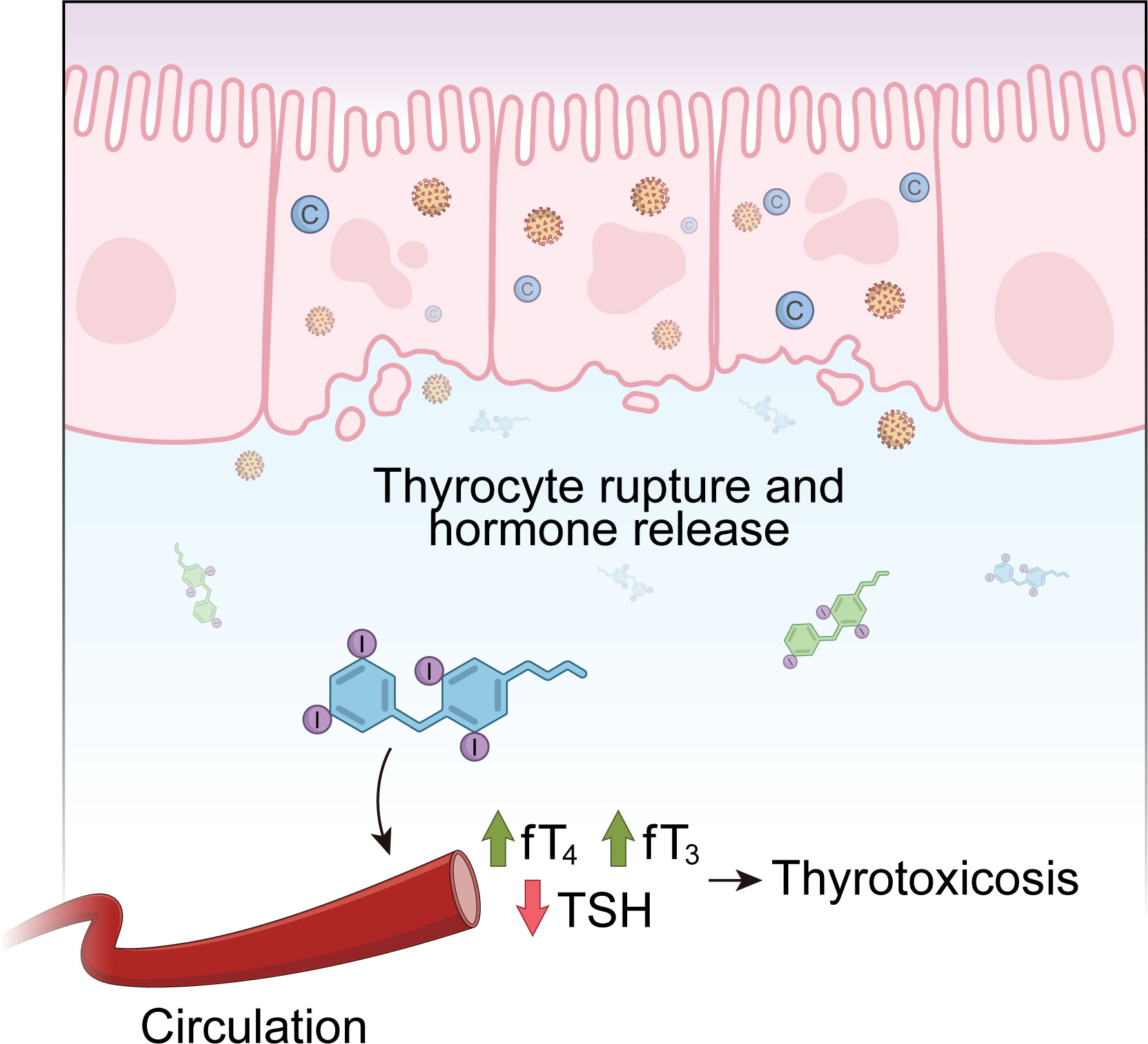
Figure 3 The course of Subacute Thyroiditis (SAT). Subacute Thyroiditis is a self-limited inflammatory disorder of the thyroid gland that often follows a viral infection. The damage and rupture of follicular cells, caused by the inflammatory process, results in the release of T3 and T4 in circulation, inducing thyrotoxicosis symptoms in the first weeks of disease. When extensive follicular destruction occurs, subacute thyroiditis can progress to hypothyroidism before returning to the euthyroid state.
A study published in 1967 demonstrated that 45% of patients with subacute thyroiditis presented an increase of at least four times in viral antibodies during their thyroid disease (127). Curiously, clusters of subacute thyroiditis have been reported during outbreaks of viral infection (126) and a higher prevalence of this disease has been reported during the summer, which is the season of the highest incidence of enteroviruses and enterobacteria (128). It is well known that infections can cause direct effects as tissue damage, and also non-infectious consequences, such as malignancies, immunodeficiency syndrome, peptic ulcer and autoimmune diseases. It has been demonstrated that several infectious agents are involved in the development of autoimmune diseases, such as rheumatic fever, lupus erythematosus, insulin-dependent diabetes, among others (129). In this context, thyroid diseases thought to be of infectious etiology (e.g. subacute thyroiditis) have been shown to be associated with thyroid autoimmune phenomena. When follicular destruction is extensive, subacute thyroiditis can progress and cause hypothyroidism (Hashimoto’s thyroiditis) (Figure 3). In fact, thyroid autoantibodies (anti-thyroglobulin and anti-thyroid peroxidase) have been found in 40-60% of patients with subacute thyroiditis (127, 130). Around 30% of those patients will experience subsequent hypothyroidism before returning to euthyroid state (125). There are also reports of the sequential occurrence of Graves’ disease and subacute thyroiditis (131, 132).
Several mechanisms have been proposed for induction of thyroid autoimmunity by viral agents including: (1) viral induction of changes in self antigen expression, or exposure of cryptic epitopes; (2) induction of local inflammation (e.g. by cytokine release), resulting in activation of autoreactive T-cells (bystander mechanism); (3) molecular mimicry between viral antigens and thyroidal antigens; (4) induction of heat shock proteins in the thyroid; and (5) induction of aberrant expression of MHC class II molecules on thyroid cells (129). However, these autoimmune phenomena seem to represent a non-specific and transient response to the inflammatory release of thyroid antigens and classical autoimmune thyroid disease is only rarely triggered by viral infections (128).
Viruses that attack the respiratory tract have been linked to the development of thyroiditis. Cases of De Quervain thyroiditis, with low TSH levels and high levels of free T3 and T4, were described in the course of H1N1 influenza infection (133, 134). The H1N1 virus has also been linked to the occurrence of thyroid storm, which is a potentially fatal intensification of thyrotoxicosis, and is characterized by hyperthermia, tachycardia, severe agitation and altered mental status (135, 136). In 2009, a 31-year-old female diagnosed with community-acquired bronchopneumonia with possible influenza A (H1N1) viral pneumonia, presented tachycardia and T3 levels 16 times higher than the threshold. The diagnosis of thyroid storm was made, but despite treatment with propylthiouracil (PTU), the patient progressed from multiorgan failure to brain death (135). A recent case report also presented the occurrence of a thyroid storm associated with Influenza A infection in a 10-year old girl (137).
A prospective study followed 61 survivors of the 2002 SARS epidemy (with no pre-existing endocrine diseases) 3 months after recovery and observed that 6.7% of them became biochemically hypothyroid (with one case of primary and three of central hypothyroidism) and 39% had hypocortisolism (two of hypocortisolic patients had also transient subclinical thyrotoxicosis). The authors speculated that these effects might be due to SARS-induced reversible hypophysitis or a direct effect of the virus on the hypothalamus (138). In addition, in subjects who died of SARS, follicular epithelial damage in the thyroid gland were found during the autopsy, with large numbers of cells exfoliated into the follicle and undergoing apoptosis (139). A subsequent study also observed in autopsies that the adenohypophysis of SARS patients had profound alterations including lower positive-cell count and staining intensity for TSH, GH and ACTH (140).
In May of 2020, an Italian case-report provided the first case of subacute thyroiditis potentially associated with a prior mild COVID-19 infection (141). An 18-year-old female patient reported neck pain radiated to the jaw, fever and palpitation 15 days after a positive RT-PCR for SARS-CoV-2. The patient showed painful and enlarged thyroid to palpation and laboratory findings typical of acute phase of destructive thyroiditis, including elevated fT3 and fT4, undetectable TSH, detectable thyroglobulin (Tg) and anti-Tg antibodies. Antibodies against TPO and TSH receptor were absent, and the inflammatory markers CRP, ESR and white blood count were elevated. Neck ultrasound revealed diffuse hypoechoic areas. The patient was diagnosed with SAT and treated with prednisone. Forty days after diagnosis thyroid function and inflammatory markers were normalized. Subsequent studies also reported additional isolated cases of painful symptomatic SAT developed 16 to 42 days after COVID-19 infection (142, 143) and also cases during active COVID-19 disease (144–147), reinforcing a possible association between SARS-CoV-2 infection and SAT (Table 1).
The THYRCOV study retrospectively evaluated the thyroid function in a cohort of 287 patients hospitalized for COVID-19 in non-intensive care units and found that thyrotoxicosis was the thyroid disorder with higher prevalence (104). In this cohort, around 55 patients (20.2%) showed low TSH (≤0.10 mU/L), from whom 31 patients presented overt thyrotoxicosis. From those 31 patients, 10 had atrial fibrillation. Fifteen patients (5.2%) were diagnosed with high TSH (>4.80 mU/L), and overt hypothyroidism was present in two of those patients (104). Thyrotoxicosis was significantly associated with higher serum IL-6 levels. Thyroid function was spontaneously improved during the follow-up, which raised the hypothesis that the thyrotoxic state could be related to destructive thyroiditis, but it was not properly investigated in this cohort.
Corroborating this findings Muller and colleagues found a thyrotoxicosis’ (TSH <0.28 mU/L and/or fT4>1.7 ng/dL) prevalence of 15% (13 in 85 patients) in patients with COVID-19 (HICU-20) admitted in high intensity of care units (HICUs), compared to 2% (2 in 41 patients) in those admitted in low intensity of care units (LICU-20) and only 1% (1 of 78 patients) in patients admitted in HICUs in 2019 for non-COVID-19 related reasons (HICU-19). Free T3 equally low in all groups. TSH levels were lower in HICU-20 than other groups, while fT4 was higher in HICU-20 only when compared to LICU-20 (117). However, it is important to mention that fT4 and fT3 were only measured in patients whose TSH levels were less than 0.45 mIU/L. Since a greater proportion of HICU-20 group (24,7%) showed low TSH levels (TSH <0.45 mIU/L) when compared to HICU-19 (17,7%) and LICU-20 (9,8% groups), TH levels were more frequently measured in HICU-20, which might be a bias. CRP levels were higher in COVID-19 patients than the non-COVID-19 group. From the 6 patients with thyrotoxicosis that were followed post-discharge, all had normal thyroid function 1.5-2 months later and were negative to thyroid autoantibodies and 3 of them had ultrasound and CT scans suggestive of subacute thyroiditis. However, since no patient reported neck pain, TSH levels were not extremely low, fT4 was not very high and lymphopenia and not leukocytosis were present, it was probably not a typical case of subacute thyroiditis. The authors suggested a routine evaluation of thyroid function in patients in ICU due to increased risk of thyrotoxicosis.
A Chinese cohort of 367 patients with predominantly mild to moderate COVID-19 detected abnormal thyroid function in 62 patients (16,9%) (120). Twenty-seven patients (7,4%) had non-thyroidal illness syndrome (NTIS) and 30 patients (8,2%) had biochemical alterations that were suggestive of distinct phases of thyroiditis such as: isolated low TSH and high-normal fT4, isolated slightly elevated fT3, high-normal Ft4 or isolated low fT4. None had overt thyrotoxicosis. Of these 30 patients with subnormal TSH, 5 presented anti-TPO or anti-TSHR autoantibodies, suggesting an autoimmune component in these cases. Pre-existing autoimmune thyroid disorder was present in 5 patients.
In contrast with the previous studies, Khoo and collaborators, did not found any case of overt thyrotoxicosis in a cohort 334 patients admitted with COVID-19 in intensive therapy unit, either during the disease or follow-up (124). Most COVID-19 patients (86.6%) were euthyroid but 5.7% present subclinical hyperthyroidism and a small proportion present overt hypothyroidism (0,6%), which did not differ from non-COVID patients. A small significant reduction in TSH and fT4 was observed in patients with COVID-19 when compared with non-COVID-19 patients which might be compatible with a nonthyroidal illness syndrome and did not justify any treatment. A negative correlation between TSH and Cortisol or CRP was observed.
Of note, since the beginning of COVID-19 vaccination campaigns, some studies show the development of thyroid disease, especially thyroiditis, after the vaccines administration. Currently, more than 30 articles reporting data of SAT onset after COVID-19 vaccine. This relation seems more prevalent in women and the main symptoms are neck pain, palpitations, fatigue, fever and weight loss (148, 149). The patients almost always presented with thyrotoxicosis and elevated serum inflammatory markers (148, 150). Two cases of vaccine-induced Graves’ disease have been reported in two female health-care workers of 28 and 40 years of age. The patients presented typical symptoms of thyrotoxicosis 2-3 days after receiving the first dose of the Pfizer-BioNTech vaccine (151). A sistematic review concluded that the thyroid disease onset occurred an average of 11 days after the administration of the vaccine (150). Cases of SAT had already been observed after the administration of the H1N1 vaccine (152, 153). The first mechanism suggested to explain the relation between COVID-19 vaccination and thyroid disease is the autoimmune/inflammatory syndrome induced by adjuvants (ASIA) (148, 151). But it is also already shown that antibodies against SARS-CoV-2 proteins could cross-react with tissue antigens, including thyroid peroxidase (TPO) (154). Despite that, considering that billions of vaccines against COVID-19 have already been administered around the world, the development of thyroid disorders following SARS-CoV-2 vaccination is a very rare side effect.
4.3. Thyroid features in COVID-19 patients
In 2002, the Severe Acute Respiratory Syndrome (SARS) caused by SARS-CoV, a member of the Coronaviridae family, became an epidemic and rapidly spread to 26 countries (155). Extensive follicular damage, with large numbers of cells exfoliated in the follicle, was observed in the thyroid glands obtained from five SARS patients. The follicular architecture was prominently affected, showing follicular distortion and collapse (139).
In the first report of subacute thyroiditis (SAT) associated with COVID-19 infection, diffuse hypoechoic areas in the thyroid ultrasound were reported, in addition to the alterations in FT3, FT4, TSH, and the presence of TgAb (142). Subsequently, other studies found alterations in the thyroid ultrasonography of COVID-19 patients that developed SAT, including bilateral hypoechoic areas (145–147, 156), heterogeneity in the parenchyma (144), a relative diffuse decrease of vascularity (144, 146, 147) and increased vascularity (145) and inflammation (104). Thoracic computed tomography also showed that COVID-19 patients present altered thyroid tissue density during their infective states compared to prior infection. In these patients, the iodine content in thyroid tissue decreased, suggesting thyroiditis (157). Likewise, a case report of SARS-CoV-2-associated thyroid storm also detected ultrasound changes in the thyroid. The patient, a 25-year-old woman, presented exophthalmos, tachycardia, diffusely enlarged goiter with a bruit, and fine tremor. Laboratory results demonstrated very low TSH levels (TSH<0.01 mIU/L) and high levels of FT4 (5.34 ng/dL) and TT3 (654 ng/dL). Thyroid ultrasound revealed heterogeneous echotexture with increased vascularity (158).
As discussed before, viral infections are considered a major factor in the pathogenesis of autoimmune thyroid diseases, and a link between SARS-CoV-2 infection and Hashimoto thyroiditis and Graves’ disease has already been reported. In a patient who started to present hyperthyroidism symptoms (fatigue, shortness of breath, palpitations, and weight loss) nearly 3 weeks after a mild SARS-CoV-2 infection, the ultrasound showed a diffusely heterogeneous and irregular thyroid and a nodular image below the sternal notch. Thyroid scintigraphy confirmed Graves’ disease pattern (159). Not many studies have evaluated the post-mortem thyroid of patients who died of COVID-19. Of these, some found no significant damage of follicular thyroid cells (102), while others reported chronic inflammation of the thyroid, follicular epithelial cell disruption, or interstitial lymphocytic infiltration (160, 161).
Importantly, thyroid morphological changes persist even after COVID-19 resolution. A study conducted in China evaluated the thyroid of 79 COVID-19 survivors approximately one month after acute COVID-19 infection. At this time, all patients presented normal T4, T3 and TSH levels. Interestingly, higher SARS-CoV-2 viral load on presentation was associated with smaller thyroid volumes among the male survivors. The authors also observed that 13.9% of the COVID-19 survivors had ultrasonographic features suggestive of thyroiditis (5 had heterogeneous echogenicity, 6 had abnormal vascularity and 3 had micro-nodulation) (162). A similar result was also found in patients whose thyroid was evaluated 6 months after COVID-19 infection. In the Turkish cohort, the mean thyroid gland volume was significantly lower in COVID-19 survivors (10.3 ± 3.4 mL) than in non-COVID patients (14 ± 5.3 mL). There were no differences in thyroid gland volume between males and females (163). These findings encourage longitudinal follow-up to clarify a possible direct viral effect of thyroid atrophy.
5. COVID-19 and thyroid: State-of-art
Herein, we present an overview of the current knowledge regarding the relationship between thyroid dysfunction and SARS-CoV-2 infection. Overall, these data revealed that abnormal thyroid function may occur during and in the convalescence post-COVID condition phase. Although the cellular and molecular mechanisms are not completely understood, the evidence suggests that the “cytokine storm” is an important mediator in this context. It is very likely that indirect mechanisms (e.g., increased serum cytokines and immune cells) are responsible for most of the effects observed in the whole HPT axis. On the other hand, some authors have also proposed that the thyroid cells could be directly infected by SARS-CoV-2. It has been consistently demonstrated in multiple datasets that ACE2 mRNA is expressed in both human thyroid tissues and primary cultured cells, suggesting that the thyroid could be vulnerable to direct viral infection and its cytopathic effects (21, 164). However, stringent immunohistochemistry analysis from the The Human Protein Atlas performed with 7 different antibodies against human ACE2 reveals that thyrocytes do not have ACE2 protein. Indeed, ACE2 protein has been detected in endothelial cells within the thyroid gland, which may explain the detection of ACE2 mRNA in whole tissue extracts. Hence, thyroid function alteration during COVID-19 is more likely a result of pro-inflammatory signals and impaired central control than a direct infection of follicular cells by SARS-CoV-2.
However, it is important to highlight that the studies found in the literature have limitations. First of all, they were retrospective and, in most of them, thyroid function tests were performed only at admission and/or days after resolution, which did not allow the observation of dynamic alterations in thyroid function during disease progression. Some studies did not assess thyroid function on all cohort, while others measured only TSH or limited fT3 or fT4 measurements only to patients with abnormal TSH. Moreover, only one study measured rT3 levels. Thus, future studies are needed to better investigate the pathophysiology of thyroid dysfunction induced by COVID 19 at both molecular and clinical levels. Furthermore, future prospective studies are crucial to clarify the prevalence of thyroid function alterations in COVID-19 patients, as well as to provide more clinical data to elucidate how it could impact the disease outcome.
Author contributions
The attributions the authors had in the production of the manuscript were: Literature review and article writing: CR, JC and FH; Text review and interpretation of data for the work: AF, RF, DC and HR; Figure creation: FH; Data collection: FB, Text review: FB, DC and HR and research coordinator and text review: DC. All authors contributed to the article and approved the submitted version.
Funding
This work was supported by grants from Fundação Carlos Chagas Filho de Amparo à Pesquisa do Estado do Rio de Janeiro (FAPERJ), Coordenação de Aperfeiçoamento de Pessoal de Nível Superior (CAPES) and Conselho Nacional de Desenvolvimento Científico e Tecnológico (CNPq).
Conflict of interest
The authors declare that the research was conducted in the absence of any commercial or financial relationships that could be construed as a potential conflict of interest.
Publisher’s note
All claims expressed in this article are solely those of the authors and do not necessarily represent those of their affiliated organizations, or those of the publisher, the editors and the reviewers. Any product that may be evaluated in this article, or claim that may be made by its manufacturer, is not guaranteed or endorsed by the publisher.
Abbreviations
- ACE2, angiotensin-converting enzyme 2; ACTH, adrenocorticotrophic hormone; AUC, area under the curve; COVID-19, Coronavirus disease 19; CRP, C-reactive protein; D1, iodothyronine deiodinase 1; D2, iodothyronine deiodinase 2; D3, iodothyronine deiodinase 3; ESR, erythrocyte sedimentation rate; GH, growth hormone; HPT, hypothalamus-pituitary-thyroid axis; ICU, Intensive Care Unit; IFN, Interferon; IL, Interleukin; IG, immunoglobulins; NO, nitric oxide; NOS, nitric oxide synthase; NTIS, non-thyroidal illness syndrome; PVN, paraventricular nucleus; OR, Odds ratio; RAS, renin-angiotensin system; ROC, receiver operating characteristic; ROS, reactive oxygen species; RT-PCR, real-time reverse-transcriptase-polymerase chain reaction; SARS-CoV-2, severe acute respiratory syndrome coronavirus 2; SAT, subacute thyroiditis; T3, triiodothyronine; T4, thyroxine; TBG, thyroxine-binding protein; TG, thyroglobulin; THs, thyroid hormones; TNF-α, tumor necrosis factor-alpha; TPO, thyroperoxidase; TRH, thyroid-releasing hormone; TSH, thyroid-stimulating hormone or thyrotropin; WHO, World Health Organization.
References
1. Zhu N, Zhang D, Wang W, Li X, Yang B, Song J, et al. A novel coronavirus from patients with pneumonia in China, 2019. N Engl J Med (2020) 382:727–33. doi: 10.1056/nejmoa2001017
2. Wu F, Zhao S, Yu B, Chen YM, Wang W, Song ZG, et al. A new coronavirus associated with human respiratory disease in China. Nature (2020) 579:265–9. doi: 10.1038/s41586-020-2008-3
3. Liu Z, Xiao X, Wei X, Li J, Yang J, Tan H, et al. Composition and divergence of coronavirus spike proteins and host ACE2 receptors predict potential intermediate hosts of SARS-CoV-2. J Med Virol (2020) 92:595–601. doi: 10.1002/jmv.25726
4. Malaiyan J, Arumugam S, Mohan K, Gomathi Radhakrishnan G. An update on the origin of SARS-CoV-2: Despite closest identity, bat (RaTG13) and pangolin derived coronaviruses varied in the critical binding site and O-linked glycan residues. J Med Virol (2021) 93:499–505. doi: 10.1002/jmv.26261
5. Mottola F, Verde N, Ricciolino R, Di Mauro M, Migliaccio M, Carfora V, et al. Cardiovascular system in COVID-19: Simply a viewer or a leading actor? Life (2020) 10:165. doi: 10.3390/life10090165
6. Daneshi SA, Taheri M, Fattahi A. SARS coronavirus 2 and central nervous system manifestations: causation, relation, or coexistence? a case series study and literature review. Br J Neurosurg (2020) 0:1–6. doi: 10.1080/02688697.2020.1861433
7. Izzedine H, Brocheriou I, Arzouk N, Seilhean D, Couvert P, Cluzel P, et al. COVID-19-associated collapsing glomerulopathy: a report of two cases and literature review. Intern Med J (2020) 50:1551–8. doi: 10.1111/imj.15041
8. Nasa P, Alexander G. COVID-19 and the liver: What do we know so far? World J Hepatol (2021) 13:522–32. doi: 10.4254/wjh.v13.i5.522
9. Georgakopoulou V, Avramopoulos P, Papalexis P, Bitsani A, Damaskos C, Garmpi A, et al. COVID−19 induced hypoparathyroidism: A case report. Exp Ther Med (2022) 23:1–5. doi: 10.3892/etm.2022.11276
10. Stanley KE, Thomas E, Leaver M, Wells D. Coronavirus disease-19 and fertility: viral host entry protein expression in male and female reproductive tissues. Fertil Steril (2020) 114:33–43. doi: 10.1016/j.fertnstert.2020.05.001
11. Scappaticcio L, Pitoia F, Esposito K, Piccardo A, Trimboli P. Impact of COVID-19 on the thyroid gland: an update. Rev Endocr Metab Disord (2021) 22:803–15. doi: 10.1007/s11154-020-09615-z
12. Arlt W, Baldeweg SE, Pearce SHS, Simpson HL. ENDOCRINOLOGY in the TIME of COVID-19: Management of adrenal insufficiency. Eur J Endocrinol (2020) 183:G25–32. doi: 10.1530/EJE-20-0361
13. Kanczkowski W, Evert K, Stadtmüller M, Haberecker M, Laks L, Chen L-S, et al. COVID-19 targets human adrenal glands. Lancet Diabetes Endocrinol (2022) 10:13–6. doi: 10.1016/S2213-8587(21)00291-6
14. Steenblock C, Richter S, Berger I, Barovic M, Schmid J, Schubert U, et al. Viral infiltration of pancreatic islets in patients with COVID-19. Nat Commun (2021) 12:3534. doi: 10.1038/s41467-021-23886-3
15. Yaribeygi H, Sathyapalan T, Jamialahmadi T, Sahebkar A. The impact of diabetes mellitus in COVID-19: A mechanistic review of molecular interactions. J Diabetes Res (2020) 2020. doi: 10.1155/2020/5436832
16. Vassilopoulou E, Bumbacea RS, Pappa AK. Obesity and Infection : What have we learned from the COVID-19 pandemic. Frontiers in Nutrition (2022) 9:1–10. doi: 10.3389/fnut.2022.931313
17. Dai S-Y, Zhang Y-P, Peng W, Shen Y, He J-J. Central infusion of angiotensin II type 2 receptor agonist compound 21 attenuates DOCA/NaCl-induced hypertension in female rats. Oxid Med Cell Longev (2016) 2016:1–9. doi: 10.1155/2016/3981790
18. Wang K, Xu Y, Yang W, Zhang Y. Insufficient hypothalamic angiotensin-converting enzyme 2 is associated with hypertension in SHR rats. Oncotarget (2017) 8:20244–51. doi: 10.18632/oncotarget.15666
19. Bakhshandeh B, Jahanafrooz Z, Abbasi A, Goli MB, Sadeghi M, Mottaqi MS, et al. Mutations in SARS-CoV-2; consequences in structure, function, and pathogenicity of the virus. Microb Pathog (2021) 154:104831. doi: 10.1016/j.micpath.2021.104831
20. Li M-Y, Li L, Zhang Y, Wang X. Expression of the SARS-CoV-2 cell receptor gene ACE2 in a wide variety of human tissues. Infect Dis Poverty (2020) 9:45. doi: 10.1186/s40249-020-00662-x
21. Lazartigues E, Qadir MMF, Mauvais-Jarvis F. Endocrine significance of SARS-CoV-2’s reliance on ACE2. Endocrinology (2020) 161:1–16. doi: 10.1210/endocr/bqaa108
22. De Vito P, Incerpi S, Pedersen JZ, Luly P, Davis FB, Davis PJ. Thyroid hormones as modulators of immune activities at the cellular level. Thyroid (2011) 21:879–90. doi: 10.1089/thy.2010.0429
23. Mancini A, Di Segni C, Raimondo S, Olivieri G, Silvestrini A, Meucci E, et al. Thyroid hormones, oxidative stress, and inflammation. Mediators Inflammation (2016) 2016. doi: 10.1155/2016/6757154
24. van der Spek AH, Fliers E, Boelen A. Thyroid hormone metabolism in innate immune cells. J Endocrinol (2017) 232:R67–81. doi: 10.1530/JOE-16-0462
25. Hodkinson CF, Simpson EEA, Beattie JH, O’Connor JM, Campbell DJ, Strain JJ, et al. Preliminary evidence of immune function modulation by thyroid hormones in healthy men and women aged 55-70 years. J Endocrinol (2009) 202:55–63. doi: 10.1677/JOE-08-0488
26. Boelaert K, Visser WE, Taylor PN, Moran C, Léger J, Persani L. Endocrinology in the time of COVID-19: Management of hyperthyroidism and hypothyroidism. Eur J Endocrinol (2020) 183:G33–9. doi: 10.1530/EJE-20-0445
27. Rubingh J, Spek A, Fliers E, Boelen A. The role of thyroid hormone in the innate and adaptive immune response during infection. Compr Physiol Wiley (2020), 10(4):1277–87. doi: 10.1002/cphy.c200003
28. Radetti G. Clinical aspects of hashimoto’s thyroiditis. Endocr Dev (2014) 26:158–70. doi: 10.1159/000363162
29. Chaker L, Bianco AC, Jonklaas J, Peeters RP. Hypothyroidism. Lancet (2017) 390:1550–62. doi: 10.1016/S0140-6736(17)30703-1
30. Klecha AJ, Genaro AM, Lysionek AE, Caro RA, Coluccia AG, Cremaschi GA. Experimental evidence pointing to the bidirectional interaction between the immune system and the thyroid axis. Int J Immunopharm (2000) 22:491–500. doi: 10.1016/S0192-0561(00)00012-6
31. Klecha AJ, Genaro AM, Gorelik G, Barreiro Arcos ML, Silberman DM, Schuman M, et al. Integrative study of hypothalamus-pituitary-thyroid-immune system interaction: Thyroid hormone-mediated modulation of lymphocyte activity through the protein kinase c signaling pathway. J Endocrinol (2006) 189:44–55. doi: 10.1677/joe.1.06137
32. Frick LR, Rapanelli M, Bussmann UA, Klecha AJ, Arcos MLB, Genaro AM, et al. Involvement of thyroid hormones in the alterations of T-cell immunity and tumor progression induced by chronic stress. Biol Psychiatry (2009) 65:935–42. doi: 10.1016/j.biopsych.2008.12.013
33. Montecino-Rodriguez E, Clark RG, Powell-Braxton L, Dorshkind K. Primary b cell development is impaired in mice with defects of the pituitary/thyroid axis. J Immunol (1997) 159:2712–9.
34. Montecino-Rodriguez E, Clark R, Johnson A, Collins L, Dorshkind K. Defective b cell development in Snell dwarf (dw/dw) mice can be corrected by thyroxine treatment. J Immunol (1996) 157:3334–40.
35. Horseman ND, Zhao W, Montecino-Rodriguez E, Tanaka M, Nakashima K, Engle SJ, et al. Defective mammopoiesis, but normal hematopoiesis, in mice with a targeted disruption of the prolactin gene. EMBO J (1997) 16:6926–35. doi: 10.1093/emboj/16.23.6926
36. Schoenfeld PS, Myers JW, Myers L, LaRocque JC. Suppression of cell-mediated immunity in hypothyroidism. South Med J (1995) 88:347–9. doi: 10.1097/00007611-199503000-00019
37. van Gerwen M, Alsen M, Little C, Barlow J, Naymagon L, Tremblay D, et al. Outcomes of patients with hypothyroidism and COVID-19: A retrospective cohort study. Front Endocrinol (Lausanne) (2020) 11:565. doi: 10.3389/fendo.2020.00565
38. Daraei M, Hasibi M, Abdollahi H, Mirabdolhagh Hazaveh M, Zebaradst J, Hajinoori M, et al. Possible role of hypothyroidism in the prognosis of COVID-19. Intern Med J (2020) 50:1410–2. doi: 10.1111/imj.15000
39. Guan W, Liang W, Zhao Y, Liang H, Chen Z, Li Y, et al. Comorbidity and its impact on 1590 patients with COVID-19 in China: a nationwide analysis. Eur Respir J (2020) 55:2000547. doi: 10.1183/13993003.00547-2020
40. Mayer D, Miller-Hänisch B, Böckh A, Hehrmann R. Fulminant meningococcal meningitis and sepsis associated with severe hypothyroidism caused by autoimmune (Hashimoto) thyreoiditis. Exp Clin Endocrinol Diabetes (2009) 105:80–0. doi: 10.1055/s-0029-1211942
42. De Leo S, Lee SY, Braverman LE, Unit E, Sciences C. Hyperthyroidism: Lancet review. Lancet (2016) 388:906–18. doi: 10.1016/S0140-6736(16)00278-6.Hyperthyroidism
43. Marino F, Guasti L, Cosentino M, De Piazza D, Simoni C, Piantanida E, et al. Thyroid hormone regulation of cell migration and oxidative metabolism in polymorphonuclear leukocytes: Clinical evidence in thyroidectomized subjects on thyroxine replacement therapy. Life Sci (2006) 78:1071–7. doi: 10.1016/j.lfs.2005.06.016
44. Wolach B, Lebanon B, Jedeikin A, Shapiro MS, Shenkman L. Neutrophil chemotaxis, random migration, and adherence in patients with hyperthyroidism. Acta Endocrinol (Copenh) (1989) 121:817–20. doi: 10.1530/acta.0.1210817
45. Duarte CG, Azzolini AECS, Assis-Pandochi AI. Effect of the period of treatment with a single dose of propylthiouracil on the antibody response in rats. Int Immunopharm (2003) 3:1419–27. doi: 10.1016/S1567-5769(03)00139-5
46. Szabó J, Fóris G, Mezösi E, Nagy E, Paragh G, Sztojka I, et al. Parameters of respiratory burst and arachidonic acid metabolism in polymorphonuclear granulocytes from patients with various thyroid diseases. Exp Clin Endocrinol Diabetes (2009) 104:172–6. doi: 10.1055/s-0029-1211440
47. Nandakumar DN, Koner BC, Vinayagamoorthi R, Nanda N, Negi VS, Goswami K, et al. Activation of NF-κB in lymphocytes and increase in serum immunoglobulin in hyperthyroidism: Possible role of oxidative stress. Immunobiology (2008) 213:409–15. doi: 10.1016/j.imbio.2007.10.005
48. Yotsumoto H. Increased levels of serum angiotensin-converting enzyme activity in hyperthyroidism. Ann Intern Med (1982) 96:326. doi: 10.7326/0003-4819-96-3-326
49. Diniz GP, Senger N, Carneiro-Ramos MS, Santos RAS, Barreto-Chaves MLM. Cardiac ACE2/angiotensin 1–7/Mas receptor axis is activated in thyroid hormone-induced cardiac hypertrophy. Ther Adv Cardiovasc Dis (2016) 10:192–202. doi: 10.1177/1753944715623228
50. Zhang Y, Lin F, Tu W, Zhang J, Choudhry AA, Ahmed O, et al. Thyroid dysfunction may be associated with poor outcomes in patients with COVID-19. Mol Cell Endocrinol (2021) 521. doi: 10.1016/j.mce.2020.111097
51. Duntas LH, Jonklaas J. COVID-19 and thyroid diseases: A bidirectional impact. J Endocr Soc (2021) 5:1–11. doi: 10.1210/jendso/bvab076
52. Caron P. Thyroid disorders and SARS-CoV-2 infection: From pathophysiological mechanism to patient management. Ann Endocrinol (Paris) (2020) 81:507–10. doi: 10.1016/j.ando.2020.09.001
53. Burch HB, Cooper DS. Anniversary review: Antithyroid drug therapy: 70 years later. Eur J Endocrinol (2018) 179:R261–74. doi: 10.1530/EJE-18-0678
54. Pantos C, Kostopanagiotou G, Armaganidis A, Trikas A, Tseti I, Mourouzis I. Triiodothyronine for the treatment of critically ill patients with COVID-19 infection: A structured summary of a study protocol for a randomised controlled trial. Trials (2020) 21:21–3. doi: 10.1186/s13063-020-04474-0
55. Oppenheimer JH, Squee R, Surks MI, Hauer H. Binding of thyroxine by serum proteins evaluated by equilibrum dialysis and electrophoretic techniques Alterations in nonthyroidal illness. J Clin Invest (1963) 42:1769–82. doi: 10.1172/JCI104862
56. Wartofsky L, Burman KDAlterations in thyroid function in patients with systemic illness: The “Euthyroid sick syndrome.”Endocr Rev (1982) 3:164–217 doi: 10.1210/edrv-3-2-164
57. Fliers E, Boelen A. An update on non-thyroidal illness syndrome. J Endocrinol Invest (2021) 44:1597–607. doi: 10.1007/s40618-020-01482-4
58. LoPresti JS, Fried JC, Spencer CA, Nicoloff JT. Unique alterations of thyroid hormone indices in the acquired immunodeficiency syndrome (AIDS). Ann Intern Med (1989) 110:970–5. doi: 10.7326/0003-4819-110-12-970
59. Grunfeld C, Pang M, Doerrler W, Jensen P, Shimizu L, Feingold KR, et al. Indices of thyroid function and weight loss in human immunodeficiency virus infection and the acquired immunodeficiency syndrome. Metabolism (1993) 42:1270–6. doi: 10.1016/0026-0495(93)90124-7
60. Beltrão FEDL, Beltrão DCDA, Carvalhal G, Beltrão FEDL, Brito ADS, Da Capistrano KHR, et al. Thyroid hormone levels during hospital admission inform disease severity and mortality in COVID-19 patients. Thyroid (2021) 31:1639–49. doi: 10.1089/thy.2021.0225
61. Boelen A, Wiersinga W, Schiphorst P. Association between serum interleukin-6 in nonthyroidal. J Clin Endocrinol Metab (1993) 77:1695–9. doi: 10.1210/jcem.77.6.8263160
62. van Haasteren GA, van der Meer MJ, Hermus AR, Linkels E, Klootwijk W, Kaptein E, et al. Different effects of continuous infusion of interleukin-1 and interleukin-6 on the hypothalamic-hypophysial-thyroid axis. Endocrinology (1994) 135:1336–45. doi: 10.1210/endo.135.4.7925094
63. Boelen A, Maas MAW, Lowik CWGM, Platvoet MC, Wiersinga WM. Induced illness in interleukin-6 (IL-6) knock-out mice: a causal role of IL-6 in the development of the low 3,5,3’-triiodothyronine syndrome. Endocrinology (1996) 137:5250–4. doi: 10.1210/endo.137.12.8940342
64. Mebis L, Paletta D, Debaveye Y, Ellger B, Langouche L, D’Hoore A, et al. Expression of thyroid hormone transporters during critical illness. Eur J Endocrinol (2009) 161:243–50. doi: 10.1530/EJE-09-0290
65. Boelen A, Kwakkel J, Thijssen-Timmer DC, Alkemade A, Fliers E, Wiersinga WM. Simultaneous changes in central and peripheral components of the hypothalamus-pituitary-thyroid axis in lipopolysaccharide-induced acute illness in mice. J Endocrinol (2004) 182:315–23. doi: 10.1677/joe.0.1820315
66. De Vries EM, Kwakkel J, Eggels L, Kalsbeek A, Barrett P, Fliers E, et al. NFκB signaling is essential for the lipopolysaccharide-induced increase of type 2 deiodinase in tanycytes. Endocrinology (2014) 155:2000–8. doi: 10.1210/en.2013-2018
67. Boelen A, Kwakkel J, Wiersinga WM, Fliers E. Chronic local inflammation in mice results in decreased TRH and type 3 deiodinase mRNA expression in the hypothalamic paraventricular nucleus independently of diminished food intake. J Endocrinol (2006) 191:707–14. doi: 10.1677/joe.1.07056
68. De Vries EM, Surovtseva O, Vos WG, Kunst RF, Van Beeren M, Kwakkel J, et al. Downregulation of type 3 deiodinase in the hypothalamus during inflammation. Thyroid (2019) 29:1336–43. doi: 10.1089/thy.2019.0201
69. Van den Berghe G, Wouters P, Weekers F, Mohan S, Baxter RC, Veldhuis JD, et al. Reactivation of pituitary hormone release and metabolic improvement by infusion of growth hormone-releasing peptide and thyrotropin-releasing hormone in patients with protracted critical Illness1. J Clin Endocrinol Metab (1999) 84:1311–23. doi: 10.1210/jcem.84.4.5636
70. Harel G, Shamoun DS, Kane JP, Magner JA, Szabo M. Prolonged effects of tumor necrosis factor-α on anterior pituitary hormone release. Peptides (1995) 16:641–5. doi: 10.1016/0196-9781(95)00019-G
71. Wassen FW, Moerings EP, Van Toor H, De Vrey EA, Hennemann G, Everts ME. Effects of interleukin-1 beta on thyrotropin secretion and thyroid hormone uptake in cultured rat anterior pituitary cells. Endocrinology (1996) 137:1591–8. doi: 10.1210/endo.137.5.8612490
72. Nishikawa T, Yamashita S, Namba H, Usa T, Tominaga T, Kimura H, et al. Interferon-gamma inhibition of human thyrotropin receptor gene expression. J Clin Endocrinol Metab (1993) 77:1084–9. doi: 10.1210/jcem.77.4.8408457
73. Nolte A, Bechtner G, Rafferzeder M, Gartner R. Interleukin-1 beta (IL-1β) binds to intact porcine thyroid follicles, decreases iodide uptake but has no effect on cAMP formation or proliferation. Horm Metab Res (1994) 26:413–8. doi: 10.1055/s-2007-1001720
74. Ajjan RA, Watson PF, Findlay C, Metcalfe RA, Crisp M, Ludgate M, et al. The sodium iodide symporter gene and its regulation by cytokines found in autoimmmunity. J Endocrinol (1998) 158:351–8. doi: 10.1677/joe.0.1580351
75. Sato K, Satoh T, Shizume K, Ozawa M, Han DC, Imamura H, et al. Inhibition of 125 I organification and thyroid hormone release by interleukin-1, tumor necrosis factor- α , and interferon- γ in human thyrocytes in suspension culture*. J Clin Endocrinol Metab (1990) 70:1735–43. doi: 10.1210/jcem-70-6-1735
76. Asakawa H, Miyagawa J, Kobayashi T, Hanafusa T, Kuwajima M, Matsuzawa Y. Effects of interleukin-1β on scanning electron microscopic appearance and thyroid peroxidase content of human thyrocytes in monolayer culture. Immunopharmacol (1996) 34:131–7. doi: 10.1016/0162-3109(96)00126-9
77. Gérard AC, Boucquey M, Van Den Hove MF, Colin IM. Expression of TPO and ThOXs in human thyrocytes is downregulated by IL-1α/IFN-γ, an effect partially mediated by nitric oxide. Am J Physiol - Endocrinol Metab (2006) 291:242–53. doi: 10.1152/ajpendo.00439.2005
78. Ashizawa K, Yamashita S, Nagayama Y, Kimura H, Hirayu H, Izumi M, et al. Interferon- y inhibits thyrotropin-induced thyroidal peroxidase gene expression in cultured human thyrocytes. J Clin Endocrinol Metab (1989) 69:475–7. doi: 10.1210/jcem-69-2-475
79. Rasmussen AK, Kayser L, Feldt-Rasmussen U, Bendtzen K. Influence of tumour necrosis factor-α, tumour necrosis factor-β and interferon-γ, separately and added together with interleukin-1β, on the function of cultured human thyroid cells. J Endocrinol (1994) 143:359–65. doi: 10.1677/joe.0.1430359
80. Pekary AE, Berg L, Santini F, Chopra I, Hershman JM. Cytokines modulate type I iodothyronine deiodinase mRNA levels and enzyme activity in FRTL-5 rat thyroid cells. Mol Cell Endocrinol (1994) 101:R31–R35. doi: 10.1016/0303-7207(94)90256-9
81. Hashimoto H, Igarashi N, Miyawaki T, Sato T. Effects of tumor necrosis factor-α, interleukin-1β, and interleukin-6 on type I iodothyronine 5′-deiodination in rat thyroid cell line, FRTL-5. J Interf Cytokine Res (1995) 15:367–75. doi: 10.1089/jir.1995.15.367
82. Tang KT, Braverman LE, DeVito WJ. Tumor necrosis factor-alpha and interferon-gamma modulate gene expression of type I 5’-deiodinase, thyroid peroxidase, and thyroglobulin in FRTL-5 rat thyroid cells. Endocrinology (1995) 136:881–8. doi: 10.1210/endo.136.3.7867596
83. Maia AL, Kim BW, Huang SA, Harney JW, Larsen PR. Type 2 iodothyronine deiodinase is the major source of plasma T3 in euthyroid humans. J Clin Invest (2005) 115:2524–33. doi: 10.1172/JCI25083
84. Luongo C, Dentice M, Salvatore D. Deiodinases and their intricate role in thyroid hormone homeostasis. Nat Rev Endocrinol (2019) 15:479–88. doi: 10.1038/s41574-019-0218-2
85. Peeters RP, Wouters PJ, Kaptein E, Van Toor H, Visser TJ, Van Den Berghe G. Reduced activation and increased inactivation of thyroid hormone in tissues of critically ill patients. J Clin Endocrinol Metab (2003) 88:3202–11. doi: 10.1210/jc.2002-022013
86. Peeters RP, Van Der Geyten S, Wouters PJ, Darras VM, Van Toor H, Kaptein E, et al. Tissue thyroid hormone levels in critical illness. J Clin Endocrinol Metab (2005) 90:6498–507. doi: 10.1210/jc.2005-1013
87. Mebis L, Langouche L, Visser TJ, Van Den Berghe G. Brief report: The type II iodothyronine deiodinase is up-regulated in skeletal muscle during prolonged critical illness. J Clin Endocrinol Metab (2007) 92:3330–3. doi: 10.1210/jc.2007-0510
88. Kwakkel J, Van Beeren HC, Ackermans MT, Platvoet-ter Schiphorst MC, Fliers E, Wiersinga WM, et al. Skeletal muscle deiodinase type 2 regulation during illness in mice. J Endocrinol (2009) 203:263–70. doi: 10.1677/JOE-09-0118
89. Rodriguez-Perez A, Palos-Paz F, Kaptein E, Visser TJ, Dominguez-Gerpe L, Alvarez-Escudero J, et al. Identification of molecular mechanisms related to nonthyroidal illness syndrome in skeletal muscle and adipose tissue from patients with septic shock. Clin Endocrinol (Oxf) (2008) 68:821–7. doi: 10.1111/j.1365-2265.2007.03102.x
90. Emokpae MA, Akinnuoye IM. Asymptomatic thyroid dysfunction in human immunodeficiency virus-1-infected subjects. J Lab Physic (2018) 10:130–4. doi: 10.4103/jlp.jlp_172_16
91. Zhang J, Chen Y. Patients infected with avian influenza a H7N9 virus have abnormally low thyroid hormone levels. Clin Lab (2014) 60:1241–1243. doi: 10.7754/Clin.Lab.2013.130742
92. Chen T, Wu D, Chen H, Yan W, Yang D, Chen G, et al. Clinical characteristics of 113 deceased patients with coronavirus disease 2019: retrospective study. BMJ (2020) 368:m1091. doi: 10.1136/bmj.m1091
93. Chen M, Zhou W, Xu W. Thyroid function analysis in 50 patients with COVID-19: A retrospective study. Thyroid (2020) 00:1–4. doi: 10.1089/thy.2020.0363
94. Zou R, Wu C, Zhang S, Wang G, Zhang Q, Yu B, et al. Euthyroid sick syndrome in patients with COVID-19. Front Endocrinol (Lausanne) (2020) 11:566439. doi: 10.3389/fendo.2020.566439
95. Guo W, Ran L, Zhu J, Ge Q, Du Z, Wang F, et al. Identifying critically ill patients at risk of death from coronavirus disease. World J Emerg Med (2021) 12:18. doi: 10.5847/wjem.j.1920-8642.2021.01.003
96. Schwarz Y, Percik R, Oberman B, Yaffe D, Zimlichman E, Tirosh A. Sick euthyroid syndrome on presentation of patients with COVID-19: A potential marker for disease severity. Endocr Pract (2021) 27:101–9. doi: 10.1016/j.eprac.2021.01.001
97. Gao W, Guo W, Guo Y, Shi M, Dong G, Wang G, et al. Thyroid hormone concentrations in severely or critically ill patients with COVID-19. J Endocrinol Invest (2021) 44:1031–40. doi: 10.1007/s40618-020-01460-w
98. Campi I, Bulgarelli I, Dubini A, Perego GB, Tortorici E, Torlasco C, et al. The spectrum of thyroid function tests during hospitalization for SARS COV-2 infection. Eur J Endocrinol (2021) 184:699–709. doi: 10.1530/EJE-20-1391
99. Rastogi L, Godbole MM, Sinha RA, Pradhan S. Reverse triiodothyronine (rT3) attenuates ischemia-reperfusion injury. Biochem Biophys Res Commun (2018) 506:597–603. doi: 10.1016/j.bbrc.2018.10.031
100. Friberg L, Drvota V, Bjelak AH, Eggertsen G, Ahnve S. Association between increased levels of reverse triiodothyronine and mortality after acute myocardial infarction. Am J Med (2001) 111:699–703. doi: 10.1016/S0002-9343(01)00980-9
101. de Lima Beltrão FE, de Almeida Beltrão DC, Carvalhal G, de Lima Beltrão FE, de Souza Braga Filho J, de Brito Oliveira J, et al. Heterozygote advantage of the type II deiodinase Thr92Ala polymorphism on intrahospital mortality of COVID-19. J Clin Endocrinol Metab (2022) 107:e2488–501. doi: 10.1210/clinem/dgac075
102. Wang W, Su X, Ding Y, Fan W, Zhou W, Su J, et al. Thyroid function abnormalities in COVID-19 patients. Front Endocrinol (Lausanne) (2021) 11:623792. doi: 10.3389/fendo.2020.623792
103. Sun L, Song F, Shi N, Liu F, Li S, Li P, et al. Combination of four clinical indicators predicts the severe/critical symptom of patients infected COVID-19. J Clin Virol (2020) 128:104431. doi: 10.1016/j.jcv.2020.104431
104. Lania A, Sandri MT, Cellini M, Mirani M, Lavezzi E, Mazziotti G. Thyrotoxicosis in patients with COVID-19: The THYRCOV study. Eur J Endocrinol (2020) 183:381–7. doi: 10.1530/EJE-20-0335
105. Sen K, Chakraborty S, Sinha A, Sen S, Alam M. Thyroid function test in COVID-19 patients: A cross-sectional study in a tertiary care hospital. Indian J Endocrinol Metab (2020) 24:532. doi: 10.4103/ijem.IJEM_779_20
106. Chen M, Zhou W, Xu W. Thyroid function analysis in 50 patients with COVID-19: A retrospective study. Thyroid (2021) 31:8–11. doi: 10.1089/thy.2020.0363
107. Vassiliadi DA, Ilias I, Pratikaki M, Jahaj E, Vassiliou AG, Detsika M, et al. Thyroid hormone alterations in critically and non-critically ill patients with SARS-CoV-2 infection. Endocr Connect (2021) 10:646–55. doi: 10.1530/EC-21-0029
108. Dincer Yazan C, Ilgin C, Elbasan O, Apaydin T, Dashdamirova S, Yigit T, et al. The association of thyroid hormone changes with inflammatory status and prognosis in COVID-19. Int J Endocrinol (2021) 2021:1–11. doi: 10.1155/2021/2395212
109. Ahn J, Lee MK, Lee JH, Sohn SY. Thyroid hormone profile and its prognostic impact on the coronavirus disease 2019 in Korean patients. Endocrinol Metab (2021) 36:769–77. doi: 10.3803/EnM.2021.1109
110. Clausen CL, Rasmussen ÅK, Johannsen TH, Hilsted LM, Skakkebæk NE, Szecsi PB, et al. Thyroid function in COVID-19 and the association with cytokine levels and mortality. Endocr Connect (2021) 10:1234–42. doi: 10.1530/EC-21-0301
111. Okwor CJ, Meka IA, Akinwande KS, Edem VF, Okwor VC. Assessment of thyroid function of newly diagnosed SARS-CoV-2 infected patients in Nigeria. Pan Afr Med J (2021) 40. doi: 10.11604/pamj.2021.40.9.26358
112. Dutta A, Jevalikar G, Sharma R, Farooqui KJ, Mahendru S, Dewan A, et al. Low FT3 is an independent marker of disease severity in patients hospitalized for COVID-19. Endocr Connect (2021) 10:1455–62. doi: 10.1530/EC-21-0362
113. Lang S, Liu Y, Qu X, Lu R, Fu W, Zhang W, et al. Association between thyroid function and prognosis of COVID-19: A retrospective observational study. Endocr Res (2021) 46:170–7. doi: 10.1080/07435800.2021.1924770
114. Zheng J, Cui Z, Shi N, Tian S, Chen T, Zhong X, et al. Suppression of the hypothalamic-pituitary-thyroid axis is associated with the severity of prognosis in hospitalized patients with COVID-19. BMC Endocr Disord (2021) 21:228. doi: 10.1186/s12902-021-00896-2
115. Sethi Y, Uniyal N, Maheshwari S, Sinha R, Goel A. Thyroid function abnormalities in the acute phase of COVID-19: A cross-sectional hospital-based study from north India. Cureus (2022) 2. doi: 10.7759/cureus.24942
116. Okoye C, Niccolai F, Rogani S, Lemmi B, Peta U, Del Vecchio S, et al. Is non-thyroidal illness syndrome (NTIS) a clinical predictor of COVID-19 mortality in critically ill oldest old patients? J Endocrinol Invest (2022) 45:1689–92. doi: 10.1007/s40618-022-01806-6
117. Muller I, Cannavaro D, Dazzi D, Covelli D, Mantovani G, Muscatello A, et al. SARS-CoV-2-related atypical thyroiditis. Lancet Diabetes Endocrinol (2020) 8:739–41. doi: 10.1016/S2213-8587(20)30266-7
118. Khoo B, Tan T, Clarke SA, Mills EG, Patel B, Modi M, et al. Thyroid function before, during, and after COVID-19. J Clin Endocrinol Metab (2021) 106:E803–11. doi: 10.1210/clinem/dgaa830
119. Güven M, Gültekin H. The prognostic impact of thyroid disorders on the clinical severity of COVID-19: Results of single-centre pandemic hospital. Int J Clin Pract (2021) 75:1–9. doi: 10.1111/ijcp.14129
120. Lui DTW, Lee CH, Chow WS, Lee ACH, Tam AR, Fong CHY, et al. Role of non-thyroidal illness syndrome in predicting adverse outcomes in COVID-19 patients predominantly of mild-to-moderate severity. Clin Endocrinol (2021) 95:469–77. doi: 10.1111/cen.14476
121. Ballesteros Vizoso MA, Castilla AF, Barceló A, Raurich JM, Argente del Castillo P, Morell-García D, et al. Thyroid disfunction in critically ill COVID-19 patients Relationship with in-hospital mortality. J Clin Med (2021) 10:5057. doi: 10.3390/jcm10215057
122. Ilera V, Delfino LC, Zunino A, Glikman P, Drnovsek M, Reyes A, et al. Correlation between inflammatory parameters and pituitary–thyroid axis in patients with COVID-19. Endocrine (2021) 74:455–60. doi: 10.1007/s12020-021-02863-2
123. Sparano C, Zago E, Morettini A, Nozzoli C, Yannas D, Adornato V, et al. Euthyroid sick syndrome as an early surrogate marker of poor outcome in mild SARS-CoV-2 disease. J Endocrinol Invest (2022) 45:837–47. doi: 10.1007/s40618-021-01714-1
124. Khoo B, Tan T, Clarke SA, Mills EG, Patel B, Modi M, et al. Thyroid function before, during and after COVID-19. J Clin Endocrinol Metab (2021). 106(2):e803–e811. doi: 10.1210/clinem/dgaa830
125. Alfadda AA, Sallam RM, Elawad GE, Aldhukair H, Alyahya MM. Subacute thyroiditis: Clinical presentation and long term outcome. Int J Endocrinol (2014) 2014. doi: 10.1155/2014/794943
126. Desailloud R, Hober D. Viruses and thyroiditis: An update. Virol J (2009) 6. doi: 10.1186/1743-422X-6-5
127. Volpé R, Row VV, Ezrin C. Circulating viral and thyroid antibodies in subacute thyroiditis. J Clin Endocrinol Metab (1967) 27:1275–84. doi: 10.1210/jcem-27-9-1275
128. Tomer Y, Davies TF. Infections and autoimmune endocrine disease. Baillieres Clin Endocrinol Metab (1995) 9:47–70. doi: 10.1016/S0950-351X(95)80819-1
129. Tomer Y, Davies TF. Infection, thyroid disease, and autoimmunity. Endocr Rev (1993) 14:107–20. doi: 10.1210/edrv-14-1-107
130. Lio S, Pontecorvi A, Caruso M, Monaco F, D’Armiento M. Transitory subclinical and permanent hypothyroidism in the course of subacute thyroiditis (de quervain). Acta Endocrinol (Copenh) (1984) 106:67–70. doi: 10.1530/acta.0.1060067
131. Werner SC. Graves’ disease following acute (Subacute) thyroiditis. Arch Intern Med (1979) 139:1313. doi: 10.1001/archinte.1979.03630480085027
132. Wartofsky L, Schaaf M. Graves’ disease with thyrotoxicosis following subacute thyroiditis. Am J Med (1987) 83:761–4. doi: 10.1016/0002-9343(87)90910-7
133. Dimos G, Pappas G, Akritidis N. Subacute thyroiditis in the course of novel H1N1 influenza infection. Endocrine (2010) 37:440–1. doi: 10.1007/s12020-010-9327-3
134. Michas G, Alevetsovitis G, Andrikou I, Tsimiklis S, Vryonis E. De quervain thyroiditis in the course of H1N1 influenza infection. Hippokratia (2014) 18:86–7.
135. Baharoon SA. H1N1 infection-induced thyroid storm. Ann Thorac Med (2010) 5:110–2. doi: 10.4103/1817-1737.62475
136. Chiha M, Samarasinghe S, Kabaker AS. Thyroid storm: An updated review. J Intensive Care Med (2015) 30:131–40. doi: 10.1177/0885066613498053
137. Higaki T, Tsukahara K, Obara T, Nojima T, Yamamoto H, Osako T, et al. Pediatric airway compromise due to thyroid storm associated with influenza a infection: A case report. Respir Med Case Rep (2020) 31:101182. doi: 10.1016/j.rmcr.2020.101182
138. Leow MKS, Kwek DSK, Ng AWK, Ong KC, Kaw GJL, Lee LSU. Hypocortisolism in survivors of severe acute respiratory syndrome (SARS). Clin Endocrinol (Oxf) (2005) 63:197–202. doi: 10.1111/j.1365-2265.2005.02325.x
139. Wei L, Sun S, hong X, Zhang J, Xu Y, Zhu H, et al. Pathology of the thyroid in severe acute respiratory syndrome. Hum Pathol (2007) 38:95–102. doi: 10.1016/j.humpath.2006.06.011
140. Wei L, Sun S, Zhang J, Zhu H, Xu Y, Ma Q, et al. Endocrine cells of the adenohypophysis in severe acute respiratory syndrome (SARS). Biochem Cell Biol (2010) 88:723–30. doi: 10.1139/O10-022
141. Brancatella A, Ricci D, Cappellani D, Viola N, Sgrò D, Santini F, et al. Is subacute thyroiditis an underestimated manifestation of SARS-CoV-2 infection? insights from a case series. J Clin Endocrinol Metab (2020) 105:e3742–6. doi: 10.1210/clinem/dgaa537
142. Brancatella A, Ricci D, Viola N, Sgrò D, Santini F, Latrofa F. Subacute thyroiditis after sars-COV-2 infection. J Clin Endocrinol Metab (2020) 105:2367–70. doi: 10.1210/clinem/dgaa276
143. Ruggeri RM, Campennì A, Siracusa M, Frazzetto G, Gullo D. Subacute thyroiditis in a patient infected with SARS-COV-2: an endocrine complication linked to the COVID-19 pandemic. Hormones (2021) 20:219–21. doi: 10.1007/s42000-020-00230-w
144. Asfuroglu Kalkan E, Ates I. A case of subacute thyroiditis associated with covid-19 infection. J Endocrinol Invest (2020) 43:1173–4. doi: 10.1007/s40618-020-01316-3
145. Chakraborty U, Ghosh S, Chandra A, Ray AK. Subacute thyroiditis as a presenting manifestation of COVID-19: A report of an exceedingly rare clinical entity. BMJ Case Rep (2020) 13. doi: 10.1136/bcr-2020-239953
146. Ippolito S, Dentali F, Tanda ML. SARS-CoV-2: a potential trigger for subacute thyroiditis? insights from a case report. J Endocrinol Invest (2020) 43:1171–2. doi: 10.1007/s40618-020-01312-7
147. Mattar SAM, Koh SJQ, Rama Chandran S, Cherng BPZ. Subacute thyroiditis associated with COVID-19. BMJ Case Rep (2020) 13. doi: 10.1136/bcr-2020-237336
148. Ippolito S, Gallo D, Rossini A, Patera B, Lanzo N, Fazzino GFM, et al. SARS-CoV-2 vaccine-associated subacute thyroiditis: insights from a systematic review. J Endocrinol Invest (2022) 45:1189–200. doi: 10.1007/s40618-022-01747-0
149. Ruggeri RM, Giovanellla L, Campennì A. SARS-CoV-2 vaccine may trigger thyroid autoimmunity: real-life experience and review of the literature. J Endocrinol Invest (2022) 2:2283–2289. doi: 10.1007/s40618-022-01863-x
150. Caironi V, Pitoia F, Trimboli P. Thyroid inconveniences with vaccination against SARS-CoV-2: The size of the matter A systematic review. Front Endocrinol (Lausanne) (2022) 13:900964. doi: 10.3389/fendo.2022.900964
151. Vera-Lastra O, Navarro AO, Domiguez MPC, Medina G, Valadez TIS, Jara LJ. Two cases of graves’ disease following SARS-CoV-2 vaccination: An autoimmune/inflammatory syndrome induced by adjuvants. Thyroid (2021) 31:1436–9. doi: 10.1089/thy.2021.0142
152. Hsiao JY, Hsin SC, Hsieh MC, Hsia PJ, Shin SJ. Subacute thyroiditis following influenza vaccine (Vaxigrip®) in a young female. Kaohsiung J Med Sci (2006) 22:297–300. doi: 10.1016/S1607-551X(09)70315-8
153. Girgis CM, Russo RR, Benson K. Subacute thyroiditis following the H1N1 vaccine. J Endocrinol Invest (2010) 33:506. doi: 10.1007/BF03346633
154. Vojdani A, Kharrazian D. Potential antigenic cross-reactivity between SARS-CoV-2 and human tissue with a possible link to an increase in autoimmune diseases. Clin Immunol (2020) 217:108480. doi: 10.1016/j.clim.2020.108480
155. Guo Y, Korteweg C, McNutt MA, Gu J. Pathogenetic mechanisms of severe acute respiratory syndrome. Virus Res (2008) 133:4–12. doi: 10.1016/j.virusres.2007.01.022
156. Sohrabpour S, Heidari F, Karimi E, Ansari R, Tajdini A, Heidari F. Subacute thyroiditis in COVID-19 patients. Eur Thyroid J (2020) 9:321–3. doi: 10.1159/000511707
157. Akkoyunlu Y, Kalaycioglu B, Yurtsever I, Eren A, Bayram M, Mert A, et al. Analysis of the involvement of the thyroid gland using computed tomography in patients with suspected SARS-CoV-2 infection: A retrospective study. Eur Rev Med Pharmacol Sci (2021) 25:4149–55. doi: 10.26355/eurrev_202106_26058
158. Rao AN, Al-Ward RY, Gaba R. Thyroid storm in a patient with COVID-19. AACE Clin Case Rep (2021) 7:360–2. doi: 10.1016/j.aace.2021.06.011
159. Sousa B, Pestana Santos C, Gonçalves Ferreira A, Judas T. Graves’ disease caused by SARS-CoV-2 infection. Eur J Case Rep Intern Med (2022), 9(7):10–2. doi: 10.12890/2022_003470
160. Poma AM, Basolo A, Bonuccelli D, Proietti A, Macerola E, Ugolini C, et al. Activation of type I and type II interferon signaling in SARS-CoV-2-Positive thyroid tissue of patients dying from COVID-19. Thyroid (2021) 31:1766–75. doi: 10.1089/thy.2021.0345
161. Ren L, Liu Q, Wang R, Chen R, Ao Q, Wang X, et al. Clinicopathologic features of COVID-19: A case report and value of forensic autopsy in studying SARS-CoV-2 infection. Am J Forensic Med Pathol (2021) 42:164–9. doi: 10.1097/PAF.0000000000000644
162. Lui DTW, Fung MMH, Chiu KWH, Lee CH, Chow WS, Lee ACH, et al. Higher SARS-CoV-2 viral loads correlated with smaller thyroid volumes on ultrasound among male COVID-19 survivors. Endocrine (2021) 74:205–14. doi: 10.1007/s12020-021-02855-2
163. Urhan E, Karaca Z, Kara CS, Yuce ZT, Unluhizarci K. The potential impact of COVID-19 on thyroid gland volumes among COVID-19 survivors. Endocrine (2022) 76:635–41. doi: 10.1007/s12020-022-03019-6
Keywords: COVID-19, SARS-CoV-2, subacute thyroiditis, non-thyroidal illness syndrome, NTIS, hypothyroidism, hyperthyroidism
Citation: Rossetti CL, Cazarin J, Hecht F, Beltrão FEL, Ferreira ACF, Fortunato RS, Ramos HE and de Carvalho DP (2022) COVID-19 and thyroid function: What do we know so far? Front. Endocrinol. 13:1041676. doi: 10.3389/fendo.2022.1041676
Received: 11 September 2022; Accepted: 05 December 2022;
Published: 19 December 2022.
Edited by:
Gabriela Brenta, Dr. César Milstein Care Unit, ArgentinaReviewed by:
Anthony Martin Gerdes, New York Institute of Technology, United StatesKursad Unluhizarci, Erciyes University, Turkey
Copyright © 2022 Rossetti, Cazarin, Hecht, Beltrão, Ferreira, Fortunato, Ramos and de Carvalho. This is an open-access article distributed under the terms of the Creative Commons Attribution License (CC BY). The use, distribution or reproduction in other forums is permitted, provided the original author(s) and the copyright owner(s) are credited and that the original publication in this journal is cited, in accordance with accepted academic practice. No use, distribution or reproduction is permitted which does not comply with these terms.
*Correspondence: Helton Estrela Ramos, cmFtb3NoZWx0b25AZ21haWwuY29t
†These authors have contributed equally to this work and share first authorship