- 1Institute of Translational Medicine, College of Life Science and Agronomy, Zhoukou Normal University, Zhoukou, Henan, China
- 2Department of Nutrition and Food Hygiene, College of Public Health, Xinxiang Medical University, Xinxiang, Henan, China
- 3Key Laboratory of Animal Ecology and Conservation Biology, Institute of Zoology, Chinese Academy of Sciences, Beijing, China
- 4School of Life Science and Technology, ShanghaiTech University, Shanghai, China
Overweight and obesity have become a world-wide problem. However, effective intervention approaches are limited. Brown adipose tissue, which helps maintain body temperature and contributes to thermogenesis, is dependent on uncoupling protein1. Over the last decade, an in-creasing number of studies have found that activating brown adipose tissue and browning of white adipose tissue can protect against obesity and obesity-related metabolic disease. Brown adipose tissue has gradually become an appealing therapeutic target for the prevention and re-versal of obesity. However, some important issues remain unresolved. It is not certain whether increasing brown adipose tissue activity is the cause or effect of body weight loss or what the risks might be for sympathetic nervous system-dependent non-shivering thermogenesis. In this review, we comprehensively summarize approaches to activating brown adipose tissue and/or browning white adipose tissue, such as cold exposure, exercise, and small-molecule treatment. We highlight the functional mechanisms of small-molecule treatment and brown adipose tissue transplantation using batokine, sympathetic nervous system and/or gut microbiome. Finally, we discuss the causality between body weight loss induced by bariatric surgery, exercise, and brown adipose tissue activity.
1. General overview of obesity and BAT
The increasing overweight and obesity pandemic has received significant attention worldwide. A total of 108 million children and 604 million adults are currently considered obese all by the year 2015 (1). The body mass index report of 31.5 million adults worldwide from 1975 to 2016 shows that the prevalence of overweight increased from 26.6% to 39%, and the prevalence of obesity increased from 7% to 12.5% (2). Obesity is associated with a shortened lifespan as well as with various types and degree of risks, such as insulin resistance and type 2 diabetes mellitus (T2DM), hyperlipidemia, stroke, and cardiovascular disease (3). Keaver et al. reported that on current trends, the proportion of overweight or obese people in Ireland could reach 85% by 2030. Obesity-related diseases such as cardiovascular disease increased by 97%, cancer by 61% and type 2 diabetes by 21% (4). The rapidly increasing prevalence and disease burden of elevated BMI highlights the need for a continued focus on ways to fight obesity. Obesity develops from excessive food intake or inadequate total energy expenditure, including basic energy expenditure, activity-based energy expenditure, diet-induced thermogenesis, and energy expenditure from thermoregulation. For this reason, caloric restriction and increased exercise are the most common ways that most people lose weight over a long period of time. Although these are effective, dieting and exercise must be maintained for a long time, and the risk remains that body weight will return. Bariatric surgery and drugs have also been used to treat obesity (5). However, bariatric surgery carries a high risk of considerable morbidity and potential mortality (6). As of 2020, the following were FDA-approved anti-obesity drugs: Orlistat, Lorcaserin, Phentermine/Topiramate, Naltrexone/Bupropion, and Liraglutide (7). Over the last few decades, several anti-obesity drugs have been withdrawn from the market due to their side effects. Sibutramine, for example, increases the risk for heart attack and stroke. The use of 2,4-dinitrophenol increases the risk for neurological diseases and cataracts, and when used in high doses, it will lead to irregular respiratory uncoupling of all cells, resulting in high temperature and death (8, 9). Sibutramine have been withdrawn owing to hepatic injury, Orlistat has some unacceptable side effects (10). Lorcaserin increases the risk of breast cancer (11).
Obesity develops form excessive energy intake exceeds energy expenditure over a long period. In addition to white adipose tissue (WAT), which has the function of storing excess energy intake, the body also has an energy-consuming adipose tissue, brown adipose tissue (BAT), which heats the body and stores thermal energy. Compared with white adipocytes, brown adipocytes have more lipid droplets but smaller size, and have more mitochondria (12). In the early days of the study, brown fat was believed to be widely present in many species during infancy, including humans. With the development of technology and concept, in 2009, people intuitively observed the existence of active brown fat in adults through 18 fluoro-deoxy-glucose positron emission tomography coupled with computed tomography (18F-FDG-PET/CT) (13). As a necessary thermoregulator in early life, BAT are present in a large number in infants and children. In adults, the number of BAT at the scapula basically disappears, and there are a few active BAT at the clavicle, pericarotid artery and pericardium (13–15). With the increase of age, the probability of BAT detection in human body decreases. Using PET/CT scans of subjects’ BAT, results showed that BAT detection rates were three times higher in subjects under 50 than in subjects under 64 (16). In addition to age, the distribution of BAT in the body of men and women is also different. PET/CT scan results show that the detection rate and content of BAT in the body of females are higher than that of males (16).
The thermogenesis of BAT depends on uncoupling protein1 (UCP1), a fatty acid anion transporter present in the mitochondrial inner membrane. UCP1 uncouples the oxidative respiratory chain of mitochondria that prevents ADP from synthesizing ATP, resulting in the release of energy in the form of thermal energy, which is directly governed by sympathetic nerve fibers (Figure 1). In mature adipocytes, noradrenaline (NE) released from sympathetic nervous system (SNS) binds to β3-adrenergic receptor (β3-AR) to promote guanylate binding protein (Gs) activates adenylate cyclase (AC). Activated AC can convert intracellular ATP into cyclic adenosine monophosphate (cAMP), increase intracellular cAMP concentration, and activate cAMP dependent protein kinase A (PKA), which activates hormone-sensitive triglyceride lipase (HSL) and accelerates the hydrolysis of triglycerides (TG) to glycerol and free fatty acids (FFA). At the same time, PKA can also activate p38 mitogen activated protein kinase (p38MAPK), and then activate the downstream substrate PPARγ coactivator-1α (PGC1-α) and activating transcription factor-2 (ATF-2). On the one hand, activated p38MAPK promote the phosphorylation of PGC1-α, and activated PGC1-α binds to the UCP1 promoter through the coactivator peroxisome proliferator-activated receptor (PPAR) to jointly promote the transcription of UCP1. On the other hand, activated p38MAPK phosphorylates ATF-2, and activated ATF-2 promotes the transcription of UCP1 through cAMP response element binding protein (CREB). PKA can also directly phosphorylate CREB. After activated CREB binds with cAMP response element (CRE), it can directly induce the expression of UCP1 (17, 18) (Figure 2). Males have a higher active BAT ratio than females, and its activity gradually decreases with age. In addition, the activity of brown fat is also significantly reduced with the development of obesity (19–23). It is estimated that when BAT is fully activated, only 50 g BAT can consume 20% of the body’s basal metabolic energy (20). Studies found that under cold or β-adrenergic agonist stimulation, brown-like adipocytes, known as beige cells, appeared in the subcutaneous WAT of mice. Beige adipocytes have multilocular fat droplets, high mitochondrial content and expression of UCP1. Beige adipocytes also have a thermogenic function, which can promote energy consumption. Its role in anti-obesity has attracted more and more attention. Trying to efficiently use brown or beige adipocytes in the human body to burn energy to reduce body fat content has been become one of the breakthrough points worth looking forward to in the current anti-obesity field (24).
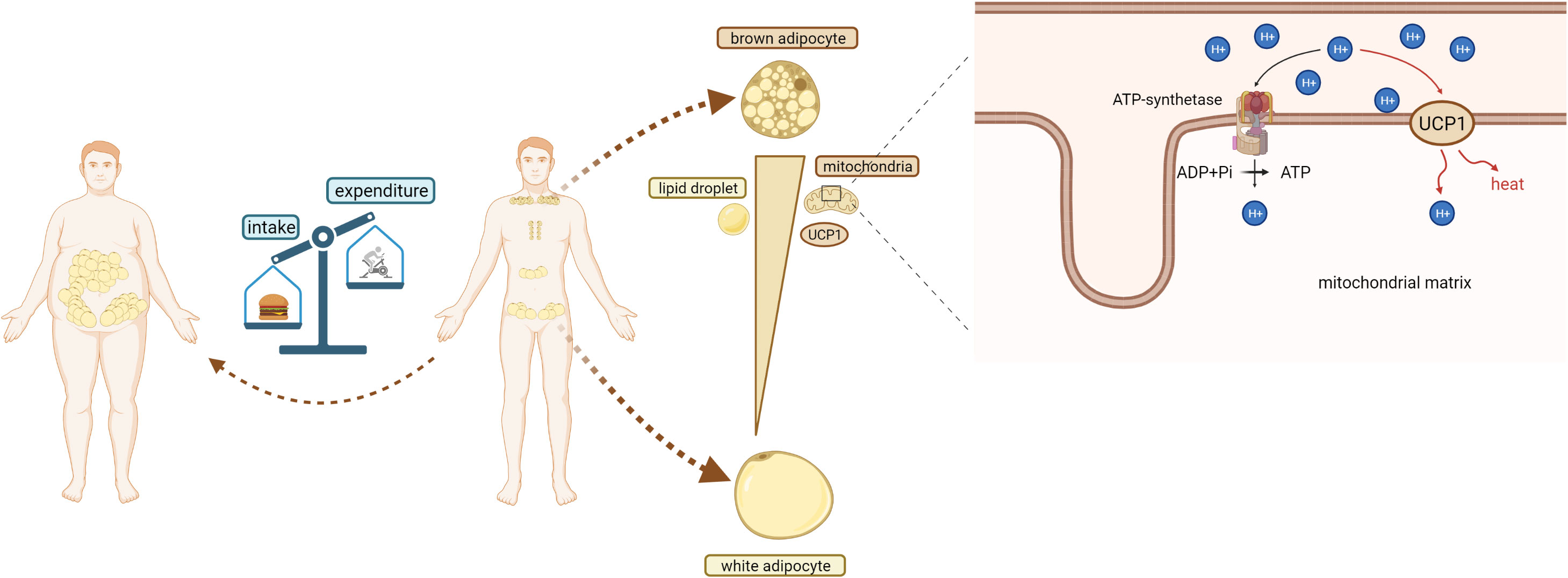
Figure 1 Obesity and BAT.Obesity occurs when the body’s energy intake is greater than its energy consumption. In adults, BAT is found in the back scapula and clavicle area, around the heart and kidneys, and WAT is located around the viscera and groin. Compared with white adipocytes, brown adipocytes contain a large number of mitochondria and high expression of UCP1. Brown adipocytes also has a small volume and a large number of lipid droplets. In mitochondria of brown adipocytes, UCP1 mediates protons to pass through the inner membrane of mitochondria, dissipating proton (H+) gradient and generating heat.
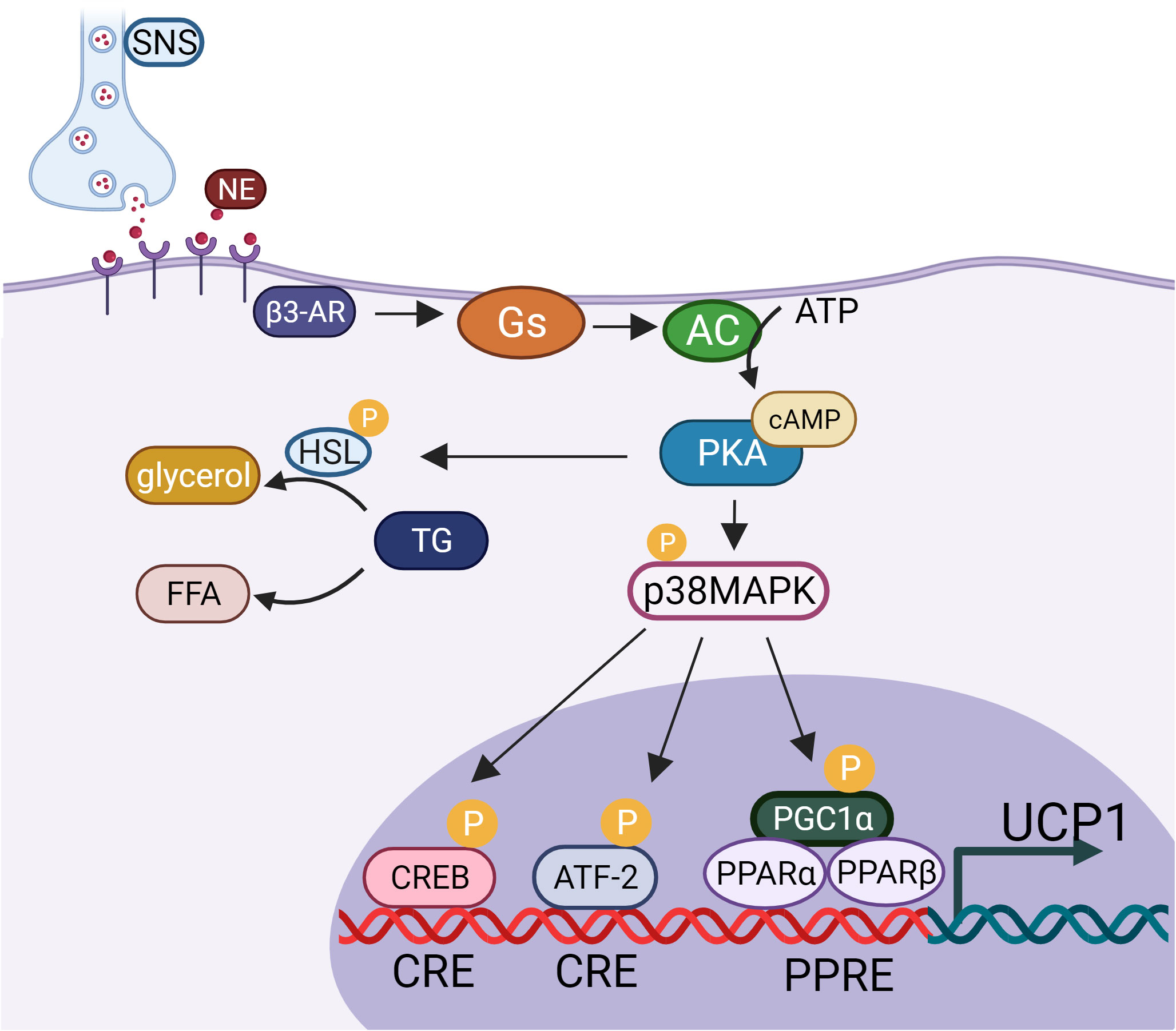
Figure 2 UCP1 activation pathway.SNS, sympathetic nervous system; NE, noradrenaline; β3-AR, β3- adrenergic receptor; Gs, guanylate binding protein; AC, adenylate cyclase; cAMP, cyclic adenosine monophosphate; PKA, protein kinase A; HSL, hormone-sensitive triglyceride lipase; TG, triglycerides; FFA, free fatty acids; p38MAPK, p38 mitogen activated protein kinase; CREB, cAMP response element binding protein; CRE, cAMP response element; PGC1-α, PPARγ coactivator-1α; ATF-2, activating transcription factor- 2; PPAR, proliferator-activated receptor; UCP1, uncoupling protein1.
2. Anti-obesity function of BAT
Based on the powerful energy-expenditure function of adaptive thermogenesis in the human body, BAT can be used as a target to reverse obesity and obesity-related metabolic diseases. The hope of identifying the anti-obesity function of BAT and establishing ways of activating it has aroused intense enthusiasm among scientists (25, 26). In recent decades, a large number of studies in different model organisms have highlighted the importance of the anti-obesity function of BAT through increasing energy expenditure (27–29). In addition to its thermogenic functions, BAT can also regulate energy metabolism of the whole body in model animals by secreting batokine through the autocrine and paracrine methods (30, 31). This review summarizes recent advances in methods of activating BAT and WAT browning in the past decade, such as cold exposure, exercise, diet, small-molecule treatment, and BAT transplantation. To illustrate the potential efficiency of these reagents in activating BAT or in WAT browning to thermogenesis, related molecular mechanisms are also elucidated. We also investigate the relationship between BAT activity and weight loss and examine means of optimizing the pathway of BAT activation, as well as reporting a few advances.
3. Cold-exposure: The most classic way to activate BAT
For humans and rodents, BAT is a thermogenic tissue whose main function is heat production via non-shivering thermogenesis (NST) when activated by cold exposure (26–28). In response to this exposure, NE released from the SNS regulates brown adipocytes at multiple levels, and it binds to β3-AR on brown and beige adipocytes. Subsequently, UCP1 becomes highly expressed and activated, thereby promoting lipid β-oxidation and heat production (32). These steps promote the proliferation and differentiation of brown preadipocytes to increase the level of NST (33–35). It is worth noting that increased sympathetic excitability can cause an accelerated heartbeat. Interestingly, other mechanisms also exist that regulate this. Li (36) found that gut microbes play a key role in BAT activation with cold exposure. A short-chain fatty acid (butyrate) produced by gut microbes appears to be an important component in the activation of BAT during cold exposure. In addition, low ambient temperatures can also induce WAT browning in a UCP1-dependent manner (37). The researchers found that the products of 12-lipoxygenase in mouse serum increased under cold stimulation, and 12-lipoxygenase in brown adipocytes produced related lipoxygenase and promoted the uptake and utilization of glucose by fat and muscle, while the products of 12-lipoxygenase in obese individuals decreased significantly (38). At present, studies of BAT activation have focused mainly on exposing rodents to severe cold (typically 4–10°C) (34, 39). Obviously, this kind of cold exposure is not practical for use against human obesity. In fact, the temperatures (21–22°C) found in standard animal facilities in these studies are already much lower than rodent’s thermal neutral zone of 30°C, which profoundly impacts their basal metabolic rate, and some scientists predict that this temperature might already prompt BAT to consume energy (37). Interestingly, this view has been confirmed by some recent findings that show mild cold (20°C or 22°C) exposure also significantly increases the thermogenic function of BAT (40, 41). These provides us with a new strategy for anti-obesity possibilities of human BAT, namely, that mild cold exposure can be used in place of severe cold exposure. In addition, it is important to recognize that atherosclerosis patients can lose weight and increase their risk for cardiovascular events by activating brown fat. The faster the heartbeat, which is directly related to the sympathetic nerve, the greater the cardiovascular mortality (42). Traditionally, beige fat is considered to have browning potential in cold environment, which can promote heat production and energy consumption be in cold or cold weather (43). Recently, scholars have found that in addition to cold exposure, beige fat can also sense local mild thermal effects (local hyperthermia therapy, LHT) and activate thermogenesis through heat shock transcription factor 1 (HSF1). Rather than the traditional view of cold activation of beige fat, LHT achieved using a hydrogel-based photothermal therapy activated beige fat, it is worth noting that the weight loss caused by local hyperthermia therapy will not be accompanied by the change of NE in blood (44).
4. Exercise-induced browning of WAT
In 1991, Stallknecht et al. first indicated that swimming training increases the mitochondrial enzyme activity of WAT in rats and gives a mitochondria-enriched fraction of WAT a browner appearance (45). However, the specific mechanism of the browning of WAT has not been identified. In 2012, Boström et al. identified a new myokine, irisin, which is released into the circulation during exercise and triggers the transformation of white fat cells into brown-in-white cells in mice (46). However, Norheim et al. found that, after 12 weeks of training in humans, skeletal muscle mRNA for PGC1-α and fibronectin type III domain-containing protein 5 (FNDC5) mRNA levels increased, and surprisingly, circulating irisin was reduced. UCP1 mRNA did not correlate with FNDC5 expression in subcutaneous adipose tissue or skeletal muscle (47). Except for irisin, other exercise-induced circulating factors such as Catecholamines (48), Interleukin-6 (IL-6) (49), Meteorin-like protein (50), fibroblast growth factor 21 (FGF21) (51) also have connection with exercise-induced browning of WAT (52). The development and maintenance of the brown phenotype in adipose tissue is maintained by SNS and noradrenergic post-ganglionic neurons. A massive activation of the SNS was observed during exercise, in addition to swimming training, running training can counteract obesity by an adrenergic-regulated brown recruitment of adipocytes, and increases adipose progenitor cell population in BAT to ameliorate high-fat diet-induced metabolic and vascular dysfunction (53, 54). These findings, as well as others (46, 54, 55), have demonstrated that exercise training such as swimming, voluntary wheel running and treadmill running leads to the browning of rodents WAT in a variety of ways. After moderate exercise, the sympathetic nerve activity increased and the expression levels of β3-AR and UCP1 were up-regulated in BAT of early overfed male wistar rats, then resulting in increased thermogenic function of BAT and higher energy consumption (56). However, exercise training consistently fails to induce browning in humans even though exercise brings other benefits to human’s health (47, 57, 58). Surprisingly, a recent randomized and controlled study found that after combined training, the thermogenic activity of BAT was significantly increased in overweight or T2DM patients, and the expression of genes related to thermogenic profile (TMEM26, EPSTI1) in subcutaneous fat was significantly increased (59). However, some experimental evidence in rats suggests otherwise. Sedentary rats exposed to cold had higher amounts of total protein and DNA in brown adipose tissue than those in the exercise group. It may be that exercise leads to the heat production of muscles, while reducing the heat production of BAT (60). Although a large body of evidence shows that exercise increases browning of WAT, whether it increases heat production is still debatable.
5. Dietary patterns
Previous studies have shown that food intake can effectively activate the thermogenesis of BAT in humans and rodents (61, 62). Researchers found that post-prandial glucose surge and increased insulin affect the transcription of clock gene, BAT activity exhibits glucose-dependent circadian rhythm (62), this concept retains for quite a while. To our surprise, another group of researchers has also recently identified that only insulin, not glucose, can rapidly induce the expression of PERIOD, which is a clock gene, and that insulin and IGF-1 are primary signals of the cellular clock feeding time (63).
In addition, certain special dietary patterns can induce the browning of WAT and BAT thermogenesis through different mechanisms. CDC-like kinase 2 (CLK2) responds to high fat diet (HFD) and is expressed in BAT, is upregulated upon refeeding, and then enhances CREB-dependent UCP1 expression (64). Moreover, every-other-day fasting (EODF) (one day feeding-one day fasting) for 15 cycles can selectively upregulate monocarboxylate transporter 1 expression in beige cells by shaping the gut microbiota. EODF stimulates beige fat development within WAT and dramatically ameliorates obesity, insulin resistance, and hepatic steatosis (65). Intermittent fasting (IF) (one day fasting-two days refeeding) for 12 weeks can activate BAT through pregnancy zone protein (PZP) secreted by the liver, so as to promote diet induced heat generation, and finally have the effect of anti-obesity (66). In addition, WAT depots are smaller and denser in 60% calorie-restricted diet fed mice. caloric restriction (CR) leads to browning of WAT, promotes the development of functional beige fat, and enhances the type 2 immune response and silent information regulator type 1 (SIRT1) expression (67). These dietary patterns seem to be more appropriate for anti-obesity treatment than stimulation of the SNS, such as through the action of the gut microbiota, and E. faecalis and its metabolite MA can reduce adiposity through BAT activation and beige fat formation (28). In summary, changing dietary patterns may be a relatively safe and feasible method of browning WAT as an anti-obesity measure. Considering the synergy between different dietary restrictions, we speculate that the insulin rhythm caused by the feeding behavior of mice is consistent with the observation that the circadian rhythm of BAT can activate BAT.
6. Small molecules
Modern studies have highlighted the potential function that some small molecules in fruits and vegetables have in browning of WAT, activating BAT, and preventing and treating obesity (Table 1). Long-term consumption of certain plant extracts, such as capsaicin (68, 90) and its esters, ephedrine (75, 91), and green tea (92) can directly activate brown fat, thereby increasing energy consumption and achieving weight control (75). The Ephedrine in ephedra sinica Stapf can increase the energy metabolism of BAT (93), and the active ingredient in red peppers, capsaicin may enhance the metabolism by directly or indirectly activating the β-adrenergic pathway (94, 95). Capsaicin has a strong initial oral effect, and its local administration is irritating, which limits its clinical application. Urolithin A is a major microbial metabolite derived from polyphenolics of berries and pomegranate fruits (96), it has also been shown to inhibit HFD-induced by activating the ability of brown and beige fat to produce heat in mice, which is dependent on the thyroid hormone pathway (97). Resveratrol, a polyphenolic compound rich in a variety of plant species, has been found to affect the expression of SIRT1, PGC1-α, and AMPK to improve mitochondrial function and promote BAT production (73, 98, 99). Recently, it has also been found that it can also regulate bile acid metabolism through gut microbiota remodeling, so as to activate BAT and promote WAT browning (100). The mulberry grows widely, and its extracts anthocyanin 3-O-glucoside (C3G) and rutin (Rut) are widely used in daily life (31, 87). C3G activates AKT, ERK, and p-38 signaling pathways in subcutaneous fat and epididymal fat. At the same time, Rut upregulates the ERK signaling pathway in subcutaneous fat and the AKT signaling pathway in muscle to improve insulin sensitivity (31, 87). Hypericin (HPF) extracted from Hypericum perforatum can directly target dihydrolipoamide S-acetyltransferase to regulate AMPK-PGC1-α signal pathway, and finally upregulate the expression of UCP1, promote adipose tissue heat production (88). Large-leaf yellow tea extract can prevent the reduction of these genes that is induced by HFD (UCP1, PGC1-α) in subcutaneous adipose tissue (SAT) and BAT, promote the beige of SAT and activate the thermogenic function of BAT (89). Ephedrine may cause side effects, such as increased heart rate and blood pressure, and may increase the number of metabolites in the circulatory system, but it does not activate brown fat in adults (101). There have been few reports on effects of resveratrol on body weight in human trials (102–104). Although the possibility that rutin regulates BAT metabolic activity through stimulating the SNS cannot be excluded (105), cellular experiments have indicated that rutin directly activates BAT oxidation in vitro. Several dietary compounds have been shown to activate brown fat activity at clinical levels (Table 2), and increasing evidence has revealed that thermogenic regulators have therapeutic effects against obesity through increasing BAT mass and/or activity, and some products have been involved in phase 1, 2, 3, and 4 clinical trials, such as β3-AR agonist and fluvastatin (112, 113). Endogenous molecules, such as irisin and FGF21, might be potential targets for certain molecules. However, the risks of these molecules to the central nervous system and sympathetic nerve activation should be considered (114).
7. BAT transplantation and batokine
With the rediscovery of BAT in adult humans, study on increasing the thermogenic function of BAT has reached an exciting boom. Many studies have focused on increasing the amount of BAT. This can be accomplished by transplanting extrinsic BAT, which is an effective way to reduce body weight gain in mice and to enhance whole-body energy metabolism; unexpectedly, however, transplanted BAT almost totally loses thermogenic function but activates the thermogenesis of endogenous BAT (27, 115). Indeed, Stanford et al. transplanted adult BAT into the visceral cavity of diabetic mice and found that IL-6 levels became elevated, which in turn induced an increase in FGF21 expression and improved glucose tolerance (29).
BAT transplantation has been performed in diabetic mouse models of dietary and obesity-related pathology. In most of these studies, BAT transplantation has shown beneficial effects on the metabolic health of recipient mice (transBATation) were fed a HFD (Table 3), and it has great potential as an anti-obesity strategy. BAT transplantation enhances systemic energy metabolism in a mouse model of diet-induced obesity (27). Many studies have shown that BAT transplantation can affect the action of batokine. In one study, the transplantation of embryonic BAT into a streptozotocin-induced mouse model of type 1 diabetes reversed the symptoms of diabetes, reduced inflammation, and elevated adiponectin levels. It is worth emphasizing that these effects were independent of insulin, and the level of IGF-1 was significantly elevated in BAT-transplanted mice (32). A few years later, similar results were achieved using a non-obese diabetes (NOD) model, showing that BAT transplantation combined the action of multiple adipokines to establish a new equilibrium in the animal to control chronic glycemia (118). In 2012, Harvard researchers transplanted adult rat BAT into the visceral cavity of diabetic mice and found that IL-6 levels became elevated in the mice, which in turn induced an increase in FGF21 expression. When the BAT used for transplantation was obtained from IL-6-knockout mice, the improved metabolic profile was lost, but glucose homeostasis improved. This result indicates that BAT-derived IL-6 is required for the effects of BAT transplantation on glucose homeostasis and insulin sensitivity (29).
These findings indicate the role of some potential protein factors in BAT function. In ob/ob mice with BAT loss, the expression of IL-6 and FGF21 in mice does not increase while the level of adiponectin increases significantly (27). This may be caused by different locations of transplantations in mice and different receptors. BAT transplantation improves whole-body energy metabolism and ameliorates polycystic ovary syndrome (PCOS), and the transplantation of BAT into PCOS rats significantly stabilizes menstrual irregularity and improves systemic insulin sensitivity up to a normal level. It also activates endogenous BAT and thereby increases the circulating level of adiponectin, which plays a prominent role in whole-body energy metabolism and ovarian physiology (115, 117). These results demonstrate that BAT transplantation may reduce obesity and related diseases by activating endogenous BAT. In addition, they show that transplanted mice confer resistance to HFD-induced obesity via increases in whole-body sympathetic activity (119). However, in view of the differences of Batokine between human and mouse, it is necessary to discuss BAT plasticity from the perspective of human physiology (14). For example, in rodents, FGF21 is secreted from BAT in response to thermogenic activation, while in human brown adipocytes, FGF21 is nearly undetectable (121).
8. Remaining questions
8.1. BAT activity and body weight loss: Which is the cause, and which is the effect?
As described above, there are various ways that BAT activity or the browning of WAT can be improved (Table 4). These approaches are often associated with body weight gain and loss (124, 125). Body weight loss can lead to increased activity of BAT. For example, in a recent study on bariatric surgery, which is an effective way to lose weight in obese individuals, it increased BAT activity, such that 1 year after surgery, the positive rate of BAT activity increased to 50% (125). This study partially demonstrated that, under the studied circumstances, BAT activity enhancement may be the result of body weight loss. However, some experimental evidence in rats suggests that bariatric surgery reduces volume, oxidative metabolism, and thermogenic gene expression in interscapular brown adipose tissue in rats (126). Researchers have shown that exercise can promote BAT recruitment, regulate the expression of UCP1, and enhance the function of mitochondria in mice and rats; meanwhile, increased BAT activity is accompanied by reduced body weight gain (127, 128). In fact, without body weight loss, long-term training has little effect on the browning of human subcutaneous adipose tissue, and it does not enhance the levels of UCP1 and PGC1-α (47). The same question concerning the small molecules that activate BAT remains. For instance, in one study, after treatment with a mixture of ephedrine and methylxanthine, MSG-induced obese mice increased their energy consumption by 20%, weight loss by 25%, and fat loss by 75% (129). When resveratrol reduced body weight and promoted BAT function, the researchers also observed that resveratrol could activate the expression of SIRT1, AMPK, and PGC1-α (99).
These findings raise the question: Between BAT activity and body weight loss, which is the cause and which is the effect?
Some possible answers present themselves. For instance, in one study, 4 h of cold exposure significantly enhanced BAT activity (130, 131). Hanssen et al. found that short-term (10-day) cold acclimation improved insulin sensitivity in patients with type 2 diabetes mellitus without body weight change. PET-CT results demonstrated that 10 days of cold acclimation induced thermogenesis of BAT in the scapula (132). Li et al. showed that, with 6 days of EODF treatment, mice WAT was greatly browned when the body weight showed no change (123).
At the same time, in vitro studies can be an adjunct method in some cases. Ephedrine can stimulate BAT respiration through adrenergic receptors in vitro (133), rutin directly activates BAT oxidation in vitro (31). In addition, in vivo studies on the absence of non-shivering (UCP1-dependent) thermogenesis should be performed to ensure if BAT and/or browning WAT are essential for the anti-obesity effects of these treatments. Kalinovich et al. observed the effects of C12TPP on high-fat-diet-induced obese mice. After C12TPP treatment, the body weights were significantly reduced. When UCP1-KO mice were treated with C12TPP, this effect disappeared, and the effects of C12TPP on the body weight of obese mice did not depend on UCP1 (134). All in all, many aspects of this exciting subject remain largely unknown and clearly merit further investigation.
8.2. SNS-dependent non-shivering thermogenesis
Stimulation of sympathetic nerves can cause abdominal visceral cardiovascular contraction and heartbeat enhancement and acceleration. Many treatments to stimulate the thermogenesis of fat can work through the SNS. Cold exposure stimulates sympathetic nervous (SN) excitability to promote the browning process (32, 135), and exercise can significantly activate the SN (53, 54). In addition, many small molecules can also enhance the excitability of the SN (105). At the same time, considering that SNS plays vital role in multiple physiological metabolic processes, these methods of stimulating or enhancing sympathetic excitability may have negative effects on the body. For example, cold stimulation can increase the thermogenesis of BAT through the gut microbiota (36), warm environments inhibit brown fat heat production, which is controlled by the nervous system (136). But cold stimulation also activates sympathetic nerves and increases systolic blood pressure, leads to rapid heartbeat and cardiac strain, which ultimately increases the risk of cardiovascular disease (137, 138). Cold stimulation is not a good anti-obesity strategy for people with cardiovascular disease. Many regulators target GPCRs, TRP, or nuclear receptors, and some have undergone clinical trials in different phases (112, 113). Transient receptor potential (TRP) ion channels are transmembrane ion channels that allow cations to pass through the cell membrane non selectively. They can mediate calcium influx and high intracellular calcium level activates the expression of PGC1α/UCP1 and increases BAT thermogenesis (139, 140). TRP channels can be activated by a variety of stimuli. The capsaicin receptor TRPV1 is expressed in brown adipose tissue and its expression level is elevated during the differentiation of pre-brown adipocytes (141).. TRPV2 was also highly expressed in brown fat, and the expression of heat genes in BAT decreased after TRPV2 knockout (142). Menthol acts as a ligand for TRPM8, activating TRPM8 in brown and white fat and improving glucose tolerance (143). The factors that promote the browning of WAT can increase thermogenesis in different ways, such as by increasing the expression of UCP1 (82) and stimulating the melanocortin/corticotropin system (144). Calcium supplementation can increase the gene expression of UCP1 and increase the protein expression of brown adipocytes marker genes, such as PGC1α, PDH, and Cyto C in inguinal WAT (145) (140). Plant extracts also have great potential, and rutin and mulberry can enhance the thermogenesis of fat by increasing the number of mitochondria (31). Resveratrol increases BAT thermogenesis by increasing SIRT1 (73, 98, 99). These methods are more direct and targeted. BAT transplantation is also a positive approach to anti-obesity (27, 115). It promotes the secretion of certain factors to activate endogenous BAT, and may not only reverse obesity and type 2 diabetes but may also ameliorate PCOS (117). However, clinical applications would require much more research.
9. Perspective
Over the last decade, increased BAT activity has garnered great interest, as numerous studies have established an association between BAT activity and metabolic health. Activating BAT or promoting browning can improve glycolipid metabolism and glucose homeostasis (27–29), and transplanting BAT can also improve PCOS (117). Unfortunately, most of the experimental studies are carried out on model animals. Based on the current technology and ethics, it is not realistic to carry out brown fat transplantation surgery on humans. We need to pay more attention to methods that can increase the secretion of brown fat in the body, which seems more practical. BAT activity and body metabolism can be influenced in many ways and through different pathways (Table 4). Yet, further study is needed to understand what can effectively, accurately, and safely increase the activity of BAT. The SN, as part of the vegetative nerve, maintains the balance of the body, along with the parasympathetic nerve. When the body is in a state of tension, sympathetic activity plays a major role. The stimulation of sympathetic nerves can cause abdominal visceral cardiovascular contraction as well as heartbeat enhancement and acceleration. Increased sympathetic excitability can cause heartbeat acceleration, and the faster the heartbeat associated with the sympathetic nerve, the higher the cardiovascular mortality (42, 144, 146, 147). Many methods of stimulating the thermogenesis of fat can function through the SNS. In increasing BAT activity, exercise and cold stimulation can significantly activate the SNS (53, 148–150), but much additional research is needed to understand what type of exercise is needed and of what intensity, as well as long it would take to effectively increase BAT activity. Thus, exercise and cold stimulation may not be a good approach for people with cardiovascular disease. Each method can improve BAT activity along a variety of pathways, so it is necessary to evaluate what methods are most suitable for different groups of people. By contrast, it appears to be more versatile and safer to improve BAT activity through the promotion of batokine secretion or through the action of gut microbiota. In addition, studies have shown that these approaches can lead to phenotypes that improve metabolism, anti-obesity, and increased BAT activity, but few studies have demonstrated the causality between these phenotypes, and direct evidence was required to clarify the targets of these methods. Validation with UCP1-KO mice or direct targeting of brown fat would increase the persuasiveness of this perspective. Some views hold that the change of UCP1 mRNA level does not generate heat (151), and it requires activation of β-adrenergic receptors to become active (152). In addition, the feeding environment in animal experiments is basically 22-26°C, which is not a thermoneutrality, which makes the sympathetic nerve of experimental animals may be in a chronic activation state for a long time, thus affecting energy consumption. In view of this, some scholars recently raised mice at 30°C(thermoneutrality) when exploring the impact of time-restricted feeding on energy consumption of mice. This temperature condition is defined as the minimum energy consumption of mice when maintaining body temperature (133). Considering that the amount of active BAT in the human body gradually decreases with age (16), this makes it fundamentally difficult to activate BAT in adults or older people. A large number of studies have shown that the browning of WAT can improve glucose and lipid metabolism and insulin resistance in obese and type 2 diabetes patients (153, 154). The role of browning of WAT is even more important for the prevention and treatment of obesity in human (155). This review highlights the targets of different activation methods and other effects they bring. Regardless, in many methods of activating BAT or promoting browning, we need to choose an efficient, reliable, and safe approach.
Author contributions
XL provided the idea of this manuscript, ZZ wrote the content, YS was responsible for the collection of materials, HX and MD were responsible for the revision of the article. All authors contributed to the article and approved the submitted version.
Funding
This work is supported by the Starting Research Fund from the Xin Xiang Medical University (XYBSKYZZ202135), Science and technology innovation talent Program of Henan Province (19HASTIT015).
Conflict of interest
The authors declare that the research was conducted in the absence of any commercial or financial relationships that could be construed as a potential conflict of interest.
Publisher’s note
All claims expressed in this article are solely those of the authors and do not necessarily represent those of their affiliated organizations, or those of the publisher, the editors and the reviewers. Any product that may be evaluated in this article, or claim that may be made by its manufacturer, is not guaranteed or endorsed by the publisher.
Abbreviations
BMI, body mass index; T2DM, type 2 diabetes mellitus; WAT, white adipose tissue; BAT, brown adipose tissue; 18F-FDG-PET/CT, 18 fluoro-deoxy-glucose positron emission tomography coupled with computed tomography; UCP1, uncoupling protein1; NE, noradrenaline; SNS, sympathetic nervous system; β3-AR, β3-adrenergic receptor; Gs, guanylate binding protein; AC, adenylate cyclase; cAMP, cyclic adenosine monophosphate; PKA, protein kinase A; HSL, hormone-sensitive triglyceride lipase; TG, triglycerides; FFA, free fatty acids; p38MAPK, p38 mitogen activated protein kinase; PGC1-α, PPARγ coactivator-1α; ATF-2, activating transcription factor-2; PPAR, proliferator-activated receptor; CREB, cAMP response element binding protein; CRE, cAMP response element; NST, non-shivering thermogenesis; HSF1, heat shock transcription factor 1; FNDC5, fibronectin type III domain-containing protein 5; IL-6, interleukin 6; FGF21, fibroblast growth factor 21; CLK2, CDC-like kinase 2; HFD, high fat diet; EODF, every-other-day fasting; IF, intermittent fasting; PZP, pregnancy zone protein; CR, caloric restriction; SIRT1, silent information regulator type 1; C3G, anthocyanin 3-O-glucoside; Rut, rutin; HPF, hypericin; SAT, subcutaneous adipose tissue; NOD, non-obese diabetes; PCOS, polycystic ovary syndrome; SN, sympathetic nervous.
References
1. GBD 2015 Obesity Collaborators, Afshin A, Forouzanfar MH, Reitsma MB, Sur P, Estep K, et al. Health effects of overweight and obesity in 195 countries over 25 years. N Engl J Med (2017) 377(1):13–27. doi: 10.1056/NEJMoa1614362
2. Hitziger M, Berger Gonzalez M, Gharzouzi E, Ochaíta Santizo D, Solis Miranda R, Aguilar Ferro AI, et al. Patient-centered boundary mechanisms to foster intercultural partnerships in health care: a case study in Guatemala. J Ethnobiol Ethnomed (2017) 13(1):44. doi: 10.1186/s13002-017-0170-y
3. collaborators, N.C.D.C. NCD countdown 2030: worldwide trends in non-communicable disease mortality and progress towards sustainable development goal target 3.4. Lancet (2018) 392(10152):1072–88. doi: 10.1016/S0140-6736(18)31992-5
4. Keaver L, Webber L, Dee A, Shiely F, Marsh T, Balanda K, et al. Application of the UK foresight obesity model in Ireland: the health and economic consequences of projected obesity trends in Ireland. PLoS One (2013) 8(11):e79827. doi: 10.1371/journal.pone.0079827
5. Gadde KM, Martin CK, Berthoud HR, Heymsfield SB. Obesity: Pathophysiology and management. J Am Coll Cardiol (2018) 71(1):69–84. doi: 10.1016/j.jacc.2017.11.011
6. Henley SJ, Ward EM, Scott S, Ma J, Anderson RN, Firth AU, et al. Annual report to the nation on the status of cancer, part I: National cancer statistics. Cancer (2020) 126(10):2225–49. doi: 10.1002/cncr.32802
7. Tak YJ, Lee SY. Long-term efficacy and safety of anti-obesity treatment: Where do we stand? Curr Obes Rep (2021) 10(1):14–30. doi: 10.1007/s13679-020-00422-w
8. Harms M, Seale P. Brown and beige fat: development, function and therapeutic potential. Nat Med (2013) 19(10):1252–63. doi: 10.1038/nm.3361
9. Daneschvar HL, Aronson MD, Smetana GW. FDA-Approved anti-obesity drugs in the united states. Am J Med (2016) 129(8):879.e1–6. doi: 10.1016/j.amjmed.2016.02.009
10. Wadden TA, Volger S, Sarwer DB, Vetter ML, Tsai AG, Berkowitz RI, et al. A two-year randomized trial of obesity treatment in primary care practice. N Engl J Med (2011) 365(21):1969–79. doi: 10.1056/NEJMoa1109220
11. Sharretts J, Galescu O, Gomatam S, Andraca-Carrera E, Hampp C, Yanoff L. Cancer risk associated with lorcaserin - the FDA's review of the CAMELLIA-TIMI 61 trial. N Engl J Med (2020) 383(11):1000–2. doi: 10.1056/NEJMp2003873
12. Young P, Arch JR, Ashwell M. Brown adipose tissue in the parametrial fat pad of the mouse. FEBS Lett (1984) 167(1):10–4. doi: 10.1016/0014-5793(84)80822-4
13. Cypess AM, Lehman S, Williams G, Tal I, Rodman D, Goldfine AB, et al. Identification and importance of brown adipose tissue in adult humans. N Engl J Med (2009) 360(15):1509–17. doi: 10.1056/NEJMoa0810780
14. Scheele C, Wolfrum C. Brown adipose crosstalk in tissue plasticity and human metabolism. Endocr Rev (2020) 41(1):53–65. doi: 10.1210/endrev/bnz007
15. Lidell ME. Brown adipose tissue in human infants. Handb Exp Pharmacol (2019) 251:107–23. doi: 10.1007/164_2018_118
16. Pfannenberg C, Werner MK, Ripkens S, Stef I, Deckert A, Schmadl M, et al. Impact of age on the relationships of brown adipose tissue with sex and adiposity in humans. Diabetes (2010) 59(7):1789–93. doi: 10.2337/db10-0004
17. Lowell BB, Flier JS. Brown adipose tissue, beta 3-adrenergic receptors, and obesity. Annu Rev Med (1997) 48:307–16. doi: 10.1146/annurev.med.48.1.307
18. Boss O, Hagen T, Lowell BB. Uncoupling proteins 2 and 3: potential regulators of mitochondrial energy metabolism. Diabetes (2000) 49(2):143–56. doi: 10.2337/diabetes.49.2.143
19. Vernochet C, Peres SB, Davis KE, McDonald ME, Qiang L, Wang H, et al. C/EBPalpha and the corepressors CtBP1 and CtBP2 regulate repression of select visceral white adipose genes during induction of the brown phenotype in white adipocytes by peroxisome proliferator-activated receptor gamma agonists. Mol Cell Biol (2009) 29(17):4714–28. doi: 10.1128/MCB.01899-08
20. Lowell BB, Spiegelman BM. Towards a molecular understanding of adaptive thermogenesis. Nature (2000) 404(6778):652–60. doi: 10.1038/35007527
21. van Marken Lichtenbelt WD, Vanhommerig JW, Smulders NM, Drossaerts JM, Kemerink GJ, Bouvy ND, et al. Cold-activated brown adipose tissue in healthy men. N Engl J Med (2009) 360(15):1500–8. doi: 10.1056/NEJMoa0808718
22. Saito M, Okamatsu-Ogura Y, Matsushita M, Watanabe K, Yoneshiro T, Nio-Kobayashi J, et al. High incidence of metabolically active brown adipose tissue in healthy adult humans: effects of cold exposure and adiposity. Diabetes (2009) 58(7):1526–31. doi: 10.2337/db09-0530
23. Kurylowicz A, Puzianowska-Kuznicka M. Induction of adipose tissue browning as a strategy to combat obesity. Int J Mol Sci (2020) 21(17):6241. doi: 10.3390/ijms21176241
24. Pfeifer A, Hoffmann LS. Brown, beige, and white: the new color code of fat and its pharmacological implications. Annu Rev Pharmacol Toxicol (2015) 55:207–27. doi: 10.1146/annurev-pharmtox-010814-124346
25. Townsend K, Tseng YH. Brown adipose tissue: Recent insights into development, metabolic function and therapeutic potential. Adipocyte (2012) 1(1):13–24. doi: 10.4161/adip.18951
26. Schulz TJ, Huang TL, Tran TT, Zhang H, Townsend KL, Shadrach JL, et al. Identification of inducible brown adipocyte progenitors residing in skeletal muscle and white fat. Proc Natl Acad Sci U.S.A. (2011) 108(1):143–8. doi: 10.1073/pnas.1010929108
27. Liu X, Wang S, You Y, Meng M, Zheng Z, Dong M, et al. Brown adipose tissue transplantation reverses obesity in Ob/Ob mice. Endocrinology (2015) 156(7):2461–9. doi: 10.1210/en.2014-1598
28. Quan LH, Zhang C, Dong M, Jiang J, Xu H, Yan C, et al. Myristoleic acid produced by enterococci reduces obesity through brown adipose tissue activation. Gut (2020) 69(7):1239–47. doi: 10.1136/gutjnl-2019-319114
29. Stanford KI, Middelbeek RJ, Townsend KL, An D, Nygaard EB, Hitchcox KM, et al. Brown adipose tissue regulates glucose homeostasis and insulin sensitivity. J Clin Invest (2013) 123(1):215–23. doi: 10.1172/JCI62308
30. Villarroya J, Cereijo R, Gavaldà-Navarro A, Peyrou M, Giralt M, Villarroya F, et al. New insights into the secretory functions of brown adipose tissue. J Endocrinol (2019) 243(2):R19–27. doi: 10.1530/JOE-19-0295
31. Yuan X, Wei G, You Y, Huang Y, Lee HJ, Dong M, et al. Rutin ameliorates obesity through brown fat activation. FASEB J (2017) 31(1):333–45. doi: 10.1096/fj.201600459rr
32. Cannon B, Nedergaard J. Brown adipose tissue: function and physiological significance. Physiol Rev (2004) 84(1):277–359. doi: 10.1152/physrev.00015.2003
33. Landsberg L, Krieger DR. Obesity, metabolism, and the sympathetic nervous system. Am J Hypertens (1989) 2(3 Pt 2):125S–32S. doi: 10.1093/ajh/2.3.125S
34. Lafontan M, Berlan M. Fat cell adrenergic receptors and the control of white and brown fat cell function. J Lipid Res (1993) 34(7):1057–91. doi: 10.1016/S0022-2275(20)37695-1
35. Tseng YH, Cypess AM, Kahn CR. Cellular bioenergetics as a target for obesity therapy. Nat Rev Drug Discovery (2010) 9(6):465–82. doi: 10.1038/nrd3138
36. Li B, Li L, Li M, Lam SM, Wang G, Wu Y, et al. Microbiota depletion impairs thermogenesis of brown adipose tissue and browning of white adipose tissue. Cell Rep (2019) 26(10):2720–37.e5. doi: 10.1016/j.celrep.2019.02.015
37. Dong M, Yang X, Lim S, Cao Z, Honek J, Lu H, et al. Cold exposure promotes atherosclerotic plaque growth and instability via UCP1-dependent lipolysis. Cell Metab (2013) 18(1):118–29. doi: 10.1016/j.cmet.2013.06.003
38. Leiria LO, Wang CH, Lynes MD, Yang K, Shamsi F, Sato M, et al. 12-lipoxygenase regulates cold adaptation and glucose metabolism by producing the omega-3 lipid 12-HEPE from brown fat. Cell Metab (2019) 30(4):768–83.e7. doi: 10.1016/j.cmet.2019.07.001
39. Cannon B, Nedergaard J. Nonshivering thermogenesis and its adequate measurement in metabolic studies. J Exp Biol (2011) 214(Pt 2):242–53. doi: 10.1242/jeb.050989
40. Lau J, Shi YC, Herzog H. Temperature dependence of the control of energy homeostasis requires CART signaling. Neuropeptides (2016) 59:97–109. doi: 10.1016/j.npep.2016.03.006
41. Winn NC, Acin-Perez R, Woodford ML, Hansen SA, Haney MM, Ayedun LA, et al. A thermogenic-like brown adipose tissue phenotype is dispensable for enhanced glucose tolerance in female mice. Diabetes (2019) 68(9):1717–29. doi: 10.2337/db18-1070
42. Hering D, Lachowska K, Schlaich M. Role of the sympathetic nervous system in stress-mediated cardiovascular disease. Curr Hypertens Rep (2015) 17(10):80. doi: 10.1007/s11906-015-0594-5
43. Shamsi F, Wang CH, Tseng YH. The evolving view of thermogenic adipocytes - ontogeny, niche and function. Nat Rev Endocrinol (2021) 17(12):726–44. doi: 10.1038/s41574-021-00562-6
44. Li Y, Wang D, Ping X, Zhang Y, Zhang T, Wang L, et al. Local hyperthermia therapy induces browning of white fat and treats obesity. Cell (2022) 185(6):949–66.e19. doi: 10.1016/j.cell.2022.02.004
45. Stallknecht B, Vinten J, Ploug T, Galbo H. Increased activities of mitochondrial enzymes in white adipose tissue in trained rats. Am J Physiol (1991) 261(3 Pt 1):E410–4. doi: 10.1152/ajpendo.1991.261.3.E410
46. Wu J, Boström P, Sparks LM, Ye L, Choi JH, Giang AH, et al. Beige adipocytes are a distinct type of thermogenic fat cell in mouse and human. Cell (2012) 150(2):366–76. doi: 10.1016/j.cell.2012.05.016
47. Norheim F, Langleite TM, Hjorth M, Holen T, Kielland A, Stadheim HK, et al. The effects of acute and chronic exercise on PGC-1alpha, irisin and browning of subcutaneous adipose tissue in humans. FEBS J (2014) 281(3):739–49. doi: 10.1111/febs.12619
48. Nguyen KD, Qiu Y, Cui X, Goh YP, Mwangi J, David T, et al. Alternatively activated macrophages produce catecholamines to sustain adaptive thermogenesis. Nature (2011) 480(7375):104–8. doi: 10.1038/nature10653
49. Knudsen JG, Murholm M, Carey AL, Biensø RS, Basse AL, Allen TL, et al. Role of IL-6 in exercise training- and cold-induced UCP1 expression in subcutaneous white adipose tissue. PLoS One (2014) 9(1):e84910. doi: 10.1371/journal.pone.0084910
50. Eaton M, Granata C, Barry J, Safdar A, Bishop D, Little JP. Impact of a single bout of high-intensity interval exercise and short-term interval training on interleukin-6, FNDC5, and METRNL mRNA expression in human skeletal muscle. J Sport Health Sci (2018) 7(2):191–6. doi: 10.1016/j.jshs.2017.01.003
51. Hansen JS, Clemmesen JO, Secher NH, Hoene M, Drescher A, Weigert C, et al. Glucagon-to-insulin ratio is pivotal for splanchnic regulation of FGF-21 in humans. Mol Metab (2015) 4(8):551–60. doi: 10.1016/j.molmet.2015.06.001
52. Townsend LK, Wright DC. Looking on the "brite" side exercise-induced browning of white adipose tissue. Pflugers Arch (2019) 471(3):455–65. doi: 10.1007/s00424-018-2177-1
53. De Matteis R, Lucertini F, Guescini M, Polidori E, Zeppa S, Stocchi V, et al. Exercise as a new physiological stimulus for brown adipose tissue activity. Nutr Metab Cardiovasc Dis (2013) 23(6):582–90. doi: 10.1016/j.numecd.2012.01.013
54. Xu X, Ying Z, Cai M, Xu Z, Li Y, Jiang SY, et al. Exercise ameliorates high-fat diet-induced metabolic and vascular dysfunction, and increases adipocyte progenitor cell population in brown adipose tissue. Am J Physiol Regul Integr Comp Physiol (2011) 300(5):R1115–25. doi: 10.1152/ajpregu.00806.2010
55. Rao RR, Long JZ, White JP, Svensson KJ, Lou J, Lokurkar I, et al. Meteorin-like is a hormone that regulates immune-adipose interactions to increase beige fat thermogenesis. Cell (2014) 157(6):1279–91. doi: 10.1016/j.cell.2014.03.065
56. Almeida DL, Moreira VM, Cardoso LE, Junior MDF, Pavanelo A, Ribeiro TA, et al. Lean in one way, in obesity another: effects of moderate exercise in brown adipose tissue of early overfed male wistar rats. Int J Obes (Lond) (2022) 46(1):137–43. doi: 10.1038/s41366-021-00969-1
57. Camera DM, Anderson MJ, Hawley JA, Carey AL. Short-term endurance training does not alter the oxidative capacity of human subcutaneous adipose tissue. Eur J Appl Physiol (2010) 109(2):307–16. doi: 10.1007/s00421-010-1356-3
58. Tsiloulis T, Carey AL, Bayliss J, Canny B, Meex RCR, Watt MJ, et al. No evidence of white adipocyte browning after endurance exercise training in obese men. Int J Obes (Lond) (2018) 42(4):721–7. doi: 10.1038/ijo.2017.295
59. Bonfante ILP, Monfort-Pires M, Duft RG, da Silva Mateus KC, de Lima Júnior JC, Dos Santos Trombeta JC, et al. Combined training increases thermogenic fat activity in patients with overweight and type 2 diabetes. Int J Obes (Lond) (2022) 46(6):1145–54. doi: 10.1038/s41366-022-01086-3
60. Arnold J, Richard D. Exercise during intermittent cold exposure prevents acclimation to cold rats. J Physiol (1987) 390:45–54. doi: 10.1113/jphysiol.1987.sp016685
61. Feldmann HM, Golozoubova V, Cannon B, Nedergaard J. UCP1 ablation induces obesity and abolishes diet-induced thermogenesis in mice exempt from thermal stress by living at thermoneutrality. Cell Metab (2009) 9(2):203–9. doi: 10.1016/j.cmet.2008.12.014
62. M.U D, Saari T, Raiko J, Kudomi N, Maurer SF, Lahesmaa M, et al. Postprandial oxidative metabolism of human brown fat indicates thermogenesis. Cell Metab (2018) 28(2):207–16.e3. doi: 10.1016/j.cmet.2018.05.020
63. Crosby P, Hamnett R, Putker M, Hoyle NP, Reed M, Karam CJ, et al. Insulin/IGF-1 drives PERIOD synthesis to entrain circadian rhythms with feeding time. Cell (2019) 177(4):896–909.e20. doi: 10.1016/j.cell.2019.02.017
64. Hatting M, Rines AK, Luo C, Tabata M, Sharabi K, Hall JA, et al. Adipose tissue CLK2 promotes energy expenditure during high-fat diet intermittent fasting. Cell Metab (2017) 25(2):428–37. doi: 10.1016/j.cmet.2016.12.007
65. Li G, Xie C, Lu S, Nichols RG, Tian Y, Li L, et al. Intermittent fasting promotes white adipose browning and decreases obesity by shaping the gut microbiota. Cell Metab (2017) 26(5):801. doi: 10.1016/j.cmet.2017.08.019
66. Lin J, Jiang X, Dong M, Liu X, Shen Q, Huang Y, et al. Hepatokine pregnancy zone protein governs the diet-induced thermogenesis through activating brown adipose tissue. Adv Sci (Weinh) (2021) 8(21):e2101991. doi: 10.1002/advs.202101991
67. Fabbiano S, Suárez-Zamorano N, Rigo D, Veyrat-Durebex C, Stevanovic Dokic A, Colin DJ, et al. Caloric restriction leads to browning of white adipose tissue through type 2 immune signaling. Cell Metab (2016) 24(3):434–46. doi: 10.1016/j.cmet.2016.07.023
68. Saito M, Yoneshiro T. Capsinoids and related food ingredients activating brown fat thermogenesis and reducing body fat in humans. Curr Opin Lipidol (2013) 24(1):71–7. doi: 10.1097/MOL.0b013e32835a4f40
69. Matsushita M, Yoneshiro T, Aita S, Kamiya T, Kusaba N, Yamaguchi K, et al. Kaempferia parviflora extract increases whole-body energy expenditure in humans: roles of brown adipose tissue. J Nutr Sci Vitaminol (Tokyo) (2015) 61(1):79–83. doi: 10.3177/jnsv.61.79
70. Choi JH, Kim SW, Yu R, Yun JW. Monoterpene phenolic compound thymol promotes browning of 3T3-L1 adipocytes. Eur J Nutr (2017) 56(7):2329–41. doi: 10.1007/s00394-016-1273-2
71. Choi JH, Yun JW. Chrysin induces brown fat-like phenotype and enhances lipid metabolism in 3T3-L1 adipocytes. Nutrition (2016) 32(9):1002–10. doi: 10.1016/j.nut.2016.02.007
72. Lone J, Choi JH, Kim SW, Yun JW. Curcumin induces brown fat-like phenotype in 3T3-L1 and primary white adipocytes. J Nutr Biochem (2016) 27:193–202. doi: 10.1016/j.jnutbio.2015.09.006
73. Andrade JM, Frade AC, Guimarães JB, Freitas KM, Lopes MT, Guimarães AL, et al. Resveratrol increases brown adipose tissue thermogenesis markers by increasing SIRT1 and energy expenditure and decreasing fat accumulation in adipose tissue of mice fed a standard diet. Eur J Nutr (2014) 53(7):1503–10. doi: 10.1007/s00394-014-0655-6
74. Alberdi G, Rodríguez VM, Miranda J, Macarulla MT, Churruca I, Portillo MP, et al. Thermogenesis is involved in the body-fat lowering effects of resveratrol in rats. Food Chem (2013) 141(2):1530–5. doi: 10.1016/j.foodchem.2013.03.085
75. Osuna-Prieto FJ, Martinez-Tellez B, Sanchez-Delgado G, Aguilera CM, Lozano-Sánchez J, Arráez-Román D, et al. Activation of human brown adipose tissue by capsinoids, catechins, ephedrine, and other dietary components: A systematic review. Adv Nutr (2019) 10(2):291–302. doi: 10.1093/advances/nmy067
76. Rossignoli CP, Dechandt CRP, Souza AO, Sampaio IH, Vicentini TM, Teodoro BG, et al. Effects of intermittent dietary supplementation with conjugated linoleic acid and fish oil (EPA/DHA) on body metabolism and mitochondrial energetics in mice. J Nutr Biochem (2018) 60:16–23. doi: 10.1016/j.jnutbio.2018.07.001
77. Zhang Z, Zhang H, Li B, Meng X, Wang J, Zhang Y, et al. Berberine activates thermogenesis in white and brown adipose tissue. Nat Commun (2014) 5:5493. doi: 10.1038/ncomms6493
78. Haratake A, Watase D, Setoguchi S, Terada K, Matsunaga K, Takata J, et al. Relationship between the acyl chain length of paradol analogues and their antiobesity activity following oral ingestion. J Agric Food Chem (2014) 62(26):6166–74. doi: 10.1021/jf500873a
79. Moon J, Do HJ, Kim OY, Shin MJ. Antiobesity effects of quercetin-rich onion peel extract on the differentiation of 3T3-L1 preadipocytes and the adipogenesis in high fat-fed rats. Food Chem Toxicol (2013) 58:347–54. doi: 10.1016/j.fct.2013.05.006
80. Velickovic K, Wayne D, Leija HAL, Bloor I, Morris DE, Law J, et al. Caffeine exposure induces browning features in adipose tissue in vitro and in vivo. Sci Rep (2019) 9(1):9104. doi: 10.1038/s41598-019-45540-1
81. Maeda H, Hosokawa M, Sashima T, Funayama K, Miyashita K. Fucoxanthin from edible seaweed, undaria pinnatifida, shows antiobesity effect through UCP1 expression in white adipose tissues. Biochem Biophys Res Commun (2005) 332(2):392–7. doi: 10.1016/j.bbrc.2005.05.002
82. Zhang C, He X, Sheng Y, Xu J, Yang C, Zheng S, et al. Allicin regulates energy homeostasis through brown adipose tissue. iScience (2020) 23(5):101113. doi: 10.1016/j.isci.2020.101113
83. Hong Y, Lin Y, Si Q, Yang L, Dong W, Gu X, et al. Ginsenoside Rb2 alleviates obesity by activation of brown fat and induction of browning of white fat. Front Endocrinol (Lausanne) (2019) 10:153. doi: 10.3389/fendo.2019.00153
84. Jeong MY, Kim HL, Park J, Jung Y, Youn DH, Lee JH, et al. Rubi fructus (Rubus coreanus) activates the expression of thermogenic genes in vivo and in vitro. Int J Obes (Lond) (2015) 39(3):456–64. doi: 10.1038/ijo.2014.155
85. Ma S, Yu H, Zhao Z, Luo Z, Chen J, Ni Y, et al. Activation of the cold-sensing TRPM8 channel triggers UCP1-dependent thermogenesis and prevents obesity. J Mol Cell Biol (2012) 4(2):88–96. doi: 10.1093/jmcb/mjs001
86. Chen LH, Chien YW, Liang CT, Chan CH, Fan MH, Huang HY, et al. Green tea extract induces genes related to browning of white adipose tissue and limits weight-gain in high energy diet-fed rat. Food Nutr Res (2017) 61(1):1347480. doi: 10.1080/16546628.2017.1347480
87. You Y, Yuan X, Liu X, Liang C, Meng M, Huang Y, et al. Cyanidin-3-glucoside increases whole body energy metabolism by upregulating brown adipose tissue mitochondrial function. Mol Nutr Food Res (2017) 61(11). doi: 10.1002/mnfr.201700261
88. Chen S, Liu X, Peng C, Tan C, Sun H, Liu H, et al. The phytochemical hyperforin triggers thermogenesis in adipose tissue via a dlat-AMPK signaling axis to curb obesity. Cell Metab (2021) 33(3):565–80.e7. doi: 10.1016/j.cmet.2021.02.007
89. Xu N, Chu J, Dong R, Lu F, Zhang X, Wang M, et al. Yellow tea stimulates thermogenesis in mice through heterogeneous browning of adipose tissues. Mol Nutr Food Res (2021) 65(2):e2000864. doi: 10.1002/mnfr.202000864
90. Kaur J, Kumar V, Kumar V, Shafi S, Khare P, Mahajan N, et al. Combination of TRP channel dietary agonists induces energy expending and glucose utilizing phenotype in HFD-fed mice. Int J Obes (Lond) (2022) 46(1):153–61. doi: 10.1038/s41366-021-00967-3
91. Astrup A, Bülow J, Madsen J, Christensen NJ. Contribution of BAT and skeletal muscle to thermogenesis induced by ephedrine in man. Am J Physiol (1985) 248(5 Pt 1):E507–15. doi: 10.1152/ajpendo.1985.248.5.E507
92. Yoneshiro T, Matsushita M, Hibi M, Tone H, Takeshita M, Yasunaga K, et al. Tea catechin and caffeine activate brown adipose tissue and increase cold-induced thermogenic capacity in humans. Am J Clin Nutr (2017) 105(4):873–81. doi: 10.3945/ajcn.116.144972
93. Baba S, Tatsumi M, Ishimori T, Lilien DL, Engles JM. Effect of nicotine and ephedrine on the accumulation of 18F-FDG in brown adipose tissue. J Nucl Med (2007) 48(6):981–6. doi: 10.2967/jnumed.106.039065
94. Diepvens K, Westerterp KR, Westerterp-Plantenga MS. Obesity and thermogenesis related to the consumption of caffeine, ephedrine, capsaicin, and green tea. Am J Physiol Regul Integr Comp Physiol (2007) 292(1):R77–85. doi: 10.1152/ajpregu.00832.2005
95. Watanabe T, Kawada T, Yamamoto M, Iwai K. Capsaicin, a pungent principle of hot red pepper, evokes catecholamine secretion from the adrenal medulla of anesthetized rats. Biochem Biophys Res Commun (1987) 142(1):259–64. doi: 10.1016/0006-291X(87)90479-7
96. Singh R, Chandrashekharappa S, Bodduluri 0053R , Baby BV, Hegde B, Kotla NG, et al. Enhancement of the gut barrier integrity by a microbial metabolite through the Nrf2 pathway. Nat Commun (2019) 10(1):89. doi: 10.1038/s41467-018-07859-7
97. Xia B, Shi XC, Xie BC, Zhu MQ, Chen Y, Chu XY, et al. Urolithin a exerts antiobesity effects through enhancing adipose tissue thermogenesis in mice. PLoS Biol (2020) 18(3):e3000688. doi: 10.1371/journal.pbio.3000688
98. Lagouge M, Argmann C, Gerhart-Hines Z, Meziane H, Lerin C, Daussin F, et al. Resveratrol improves mitochondrial function and protects against metabolic disease by activating SIRT1 and PGC-1alpha. Cell (2006) 127(6):1109–22. doi: 10.1016/j.cell.2006.11.013
99. Price NL, Gomes AP, Ling AJ, Duarte FV, Martin-Montalvo A, North BJ, et al. SIRT1 is required for AMPK activation and the beneficial effects of resveratrol on mitochondrial function. Cell Metab (2012) 15(5):675–90. doi: 10.1016/j.cmet.2012.04.003
100. Hui S, Liu Y, Huang L, Zheng L, Zhou M, Lang H, et al. Resveratrol enhances brown adipose tissue activity and white adipose tissue browning in part by regulating bile acid metabolism via gut microbiota remodeling. Int J Obes (Lond) (2020) 44(8):1678–90. doi: 10.1038/s41366-020-0566-y
101. Cypess AM, Chen YC, Sze C, Wang K, English J, Chan O, et al. Cold but not sympathomimetics activates human brown adipose tissue in vivo. Proc Natl Acad Sci U.S.A. (2012) 109(25):10001–5. doi: 10.1073/pnas.1207911109
102. Mongioi LM, La Vignera S, Cannarella R, Cimino L, Compagnone M, Condorelli RA, et al. The role of resveratrol administration in human obesity. Int J Mol Sci (2021) 22(9):4362. doi: 10.3390/ijms22094362
103. Yoshino J, Conte C, Fontana L, Mittendorfer B, Imai S, Schechtman KB, et al. Resveratrol supplementation does not improve metabolic function in nonobese women with normal glucose tolerance. Cell Metab (2012) 16(5):658–64. doi: 10.1016/j.cmet.2012.09.015
104. Poulsen MM, Vestergaard PF, Clasen BF, Radko Y, Christensen LP, Stødkilde-Jørgensen H, et al. High-dose resveratrol supplementation in obese men: an investigator-initiated, randomized, placebo-controlled clinical trial of substrate metabolism, insulin sensitivity, and body composition. Diabetes (2013) 62(4):1186–95. doi: 10.2337/db12-0975
105. Bartness TJ, Vaughan CH, Song CK. Sympathetic and sensory innervation of brown adipose tissue. Int J Obes (Lond) (2010) 34 Suppl 1:S36–42. doi: 10.1038/ijo.2010.182
106. Fuse S, Endo T, Tanaka R, Kuroiwa M, Ando A, Kume A, et al. Effects of capsinoid intake on brown adipose tissue vascular density and resting energy expenditure in healthy, middle-aged adults: A?randomized, double-blind, placebo-controlled study. Nutrients (2020) 12(9):2676. doi: 10.3390/nu12092676
107. Ang QY, Goh HJ, Cao Y, Li Y, Chan SP, Swain JL, et al. A new method of infrared thermography for quantification of brown adipose tissue activation in healthy adults (TACTICAL): a randomized trial. J Physiol Sci (2017) 67(3):395–406. doi: 10.1007/s12576-016-0472-1
108. de Ligt M, Bruls YMH, Hansen J, Habets MF, Havekes B, Nascimento EBM, et al. Resveratrol improves ex vivo mitochondrial function but does not affect insulin sensitivity or brown adipose tissue in first degree relatives of patients with type 2 diabetes. Mol Metab (2018) 12:39–47. doi: 10.1016/j.molmet.2018.04.004
109. Wu L, Xia M, Duan Y, Zhang L, Jiang H, Hu X, et al. Berberine promotes the recruitment and activation of brown adipose tissue in mice and humans. Cell Death Dis (2019) 10(6):468. doi: 10.1038/s41419-019-1706-y
110. Carey AL, Formosa MF, Van Every B, Bertovic D, Eikelis N, Lambert GW, et al. Ephedrine activates brown adipose tissue in lean but not obese humans. Diabetologia (2013) 56(1):147–55. doi: 10.1007/s00125-012-2748-1
111. Sugita J, Yoneshiro T, Hatano T, Aita S, Ikemoto T, Uchiwa H, et al. Grains of paradise (Aframomum melegueta) extract activates brown adipose tissue and increases whole-body energy expenditure in men. Br J Nutr (2013) 110(4):733–8. doi: 10.1017/S0007114512005715
112. Cypess AM, Weiner LS, Roberts-Toler C, Franquet Elía E, Kessler SH, Kahn PA, et al. Activation of human brown adipose tissue by a beta3-adrenergic receptor agonist. Cell Metab (2015) 21(1):33–8. doi: 10.1016/j.cmet.2014.12.009
113. Baskin AS, Linderman JD, Brychta RJ, McGehee S, Anflick-Chames E, Cero C, Johnson JW, et al, et al. Regulation of human adipose tissue activation, gallbladder size, and bile acid metabolism by a beta3-adrenergic receptor agonist. Diabetes (2018) 67(10):2113–25. doi: 10.2337/db18-0462
114. Liu J, Wang Y, Lin L. Small molecules for fat combustion: targeting obesity. Acta Pharm Sin B (2019) 9(2):220–36. doi: 10.1016/j.apsb.2018.09.007
115. Liu X, Zheng Z, Zhu X, Meng M, Li L, Shen Y, et al. Brown adipose tissue transplantation improves whole-body energy metabolism. Cell Res (2013) 23(6):851–4. doi: 10.1038/cr.2013.64
116. Gunawardana SC, Piston DW. Reversal of type 1 diabetes in mice by brown adipose tissue transplant. Diabetes (2012) 61(3):674–82. doi: 10.2337/db11-0510
117. Yuan X, Hu T, Zhao H, Huang Y, Ye R, Lin J, et al. Brown adipose tissue transplantation ameliorates polycystic ovary syndrome. Proc Natl Acad Sci U.S.A. (2016) 113(10):2708–13. doi: 10.1073/pnas.1523236113
118. Gunawardana SC, Piston DW. Insulin-independent reversal of type 1 diabetes in nonobese diabetic mice with brown adipose tissue transplant. Am J Physiol Endocrinol Metab (2015) 308(12):E1043–55. doi: 10.1152/ajpendo.00570.2014
119. Zhu Z, Spicer EG, Gavini CK, Goudjo-Ako AJ, Novak CM. Enhanced sympathetic activity in mice with brown adipose tissue transplantation (transBATation). Physiol Behav (2014) 125:21–9. doi: 10.1016/j.physbeh.2013.11.008
120. Oguri Y, Fujita Y, Abudukadier A, Ohashi A, Goto T, Furuya F, et al. Tetrahydrobiopterin activates brown adipose tissue and regulates systemic energy metabolism. JCI Insight (2017) 2(9):e91981. doi: 10.1172/jci.insight.91981
121. Deshmukh AS, Peijs L, Beaudry JL, Jespersen NZ, Nielsen CH, Ma T, et al. Proteomics-based comparative mapping of the secretomes of human brown and white adipocytes reveals EPDR1 as a novel batokine. Cell Metab (2019) 30(5):963–75.e7. doi: 10.1016/j.cmet.2019.10.001
122. Imai J, Katagiri H, Yamada T, Ishigaki Y, Ogihara T, Uno K, et al. Cold exposure suppresses serum adiponectin levels through sympathetic nerve activation in mice. Obes (Silver Spring) (2006) 14(7):1132–41. doi: 10.1038/oby.2006.130
123. Li G, Xie C, Lu S, Nichols RG, Tian Y, Li L, et al. Intermittent fasting promotes white adipose browning and decreases obesity by shaping the gut microbiota. Cell Metab (2017) 26(4):672–85.e4. doi: 10.1016/j.cmet.2017.08.019
124. Virtanen KA, Lidell ME, Orava J, Heglind M, Westergren R, Niemi T, et al. Functional brown adipose tissue in healthy adults. N Engl J Med (2009) 360(15):1518–25. doi: 10.1056/NEJMoa0808949
125. Vijgen GH, Bouvy ND, Teule GJ, Brans B, Hoeks J, Schrauwen P, et al. Increase in brown adipose tissue activity after weight loss in morbidly obese subjects. J Clin Endocrinol Metab (2012) 97(7):E1229–33. doi: 10.1210/jc.2012-1289
126. Baraboi ED, Li W, Labbé SM, Roy MC, Samson P, Hould FS, et al. Metabolic changes induced by the biliopancreatic diversion in diet-induced obesity in male rats: the contributions of sleeve gastrectomy and duodenal switch. Endocrinology (2015) 156(4):1316–29. doi: 10.1210/en.2014-1785
127. Atakan MM, Koşar ŞN, Güzel Y, Tin HT, Yan X., et al. The role of exercise, diet, and cytokines in preventing obesity and improving adipose tissue. Nutrients (2021) 13(5):1459. doi: 10.3390/nu13051459
128. de Las Heras N, Klett-Mingo M, Ballesteros S, Martín-Fernández B, Escribano Ó, Blanco-Rivero J, et al. Chronic exercise improves mitochondrial function and insulin sensitivity in brown adipose tissue. Front Physiol (2018) 9:1122. doi: 10.3389/fphys.2018.01122
129. Dulloo AG, Miller DS. The thermogenic properties of ephedrine/methylxanthine mixtures: animal studies. Am J Clin Nutr (1986) 43(3):388–94. doi: 10.1093/ajcn/43.3.388
130. Lu X, Solmonson A, Lodi A, Nowinski SM, Sentandreu E, Riley CL, et al. The early metabolomic response of adipose tissue during acute cold exposure in mice. Sci Rep (2017) 7(1):3455. doi: 10.1038/s41598-017-03108-x
131. Wang X, Wahl R. Responses of the insulin signaling pathways in the brown adipose tissue of rats following cold exposure. PLoS One (2014) 9(6):e99772. doi: 10.1371/journal.pone.0099772
132. Hanssen MJ, van der Lans AA, Brans B, Hoeks J, Jardon KM, Schaart G, et al. Short-term cold acclimation recruits brown adipose tissue in obese humans. Diabetes (2016) 65(5):1179–89. doi: 10.2337/db15-1372
133. Hepler C, Weidemann BJ, Waldeck NJ, Marcheva B, Cedernaes J, Thorne AK, et al. Time-restricted feeding mitigates obesity through adipocyte thermogenesis. Science (2022) 378(6617):276–84. doi: 10.1126/science.abl8007
134. Kalinovich AV, Mattsson CL, Youssef MR, Petrovic N, Ost M, Skulachev VP, et al. Mitochondria-targeted dodecyltriphenylphosphonium (C12TPP) combats high-fat-diet-induced obesity in mice. Int J Obes (Lond) (2016) 40(12):1864–74. doi: 10.1038/ijo.2016.146
135. Zeng W, Yang F, Shen WL, Zhan C, Zheng P, Hu J, et al. Interactions between central nervous system and peripheral metabolic organs. Sci China Life Sci (2022) 65(10):1929–58. doi: 10.1007/s11427-021-2103-5
136. Yang WZ, Du X, Zhang W, Gao C, Xie H, Xiao Y, et al. Parabrachial neuron types categorically encode thermoregulation variables during heat defense. Sci Adv (2020) 6(36):eabb9414. doi: 10.1126/sciadv.abb9414
137. Hintsala H, Kandelberg A, Herzig KH, Rintamäki H, Mäntysaari M, Rantala A, et al. Central aortic blood pressure of hypertensive men during short-term cold exposure. Am J Hypertens (2014) 27(5):656–64. doi: 10.1093/ajh/hpt136
138. Wang Q, Li C, Guo Y, Barnett AG, Tong S, Phung D, et al. Environmental ambient temperature and blood pressure in adults: A systematic review and meta-analysis. Sci Total Environ (2017) 575:276–86. doi: 10.1016/j.scitotenv.2016.10.019
139. Sun W, Luo Y, Zhang F, Tang S, Zhu T. Involvement of TRP channels in adipocyte thermogenesis: An update. Front Cell Dev Biol (2021) 9:686173. doi: 10.3389/fcell.2021.686173
140. Zhai M, Yang D, Yi W, Sun W. Involvement of calcium channels in the regulation of adipogenesis. Adipocyte (2020) 9(1):132–41. doi: 10.1080/21623945.2020.1738792
141. Kida R, Yoshida H, Murakami M, Shirai M, Hashimoto O, Kawada T, et al. Direct action of capsaicin in brown adipogenesis and activation of brown adipocytes. Cell Biochem Funct (2016) 34(1):34–41. doi: 10.1002/cbf.3162
142. Sun W, Uchida K, Suzuki Y, Zhou Y, Kim M, Takayama Y, et al. Lack of TRPV2 impairs thermogenesis in mouse brown adipose tissue. EMBO Rep (2016) 17(3):383–99. doi: 10.15252/embr.201540819
143. Jiang C, Zhai M, Yan D, Li D, Li C, Zhang Y, et al. Dietary menthol-induced TRPM8 activation enhances WAT "browning" and ameliorates diet-induced obesity. Oncotarget (2017) 8(43):75114–26. doi: 10.18632/oncotarget.20540
144. Haynes WG, Morgan DA, Djalali A, Sivitz WI, Mark AL. Interactions between the melanocortin system and leptin in control of sympathetic nerve traffic. Hypertension (1999) 33(1 Pt 2):542–7. doi: 10.1161/01.HYP.33.1.542
145. Zhang F, Su H, Song M, Zheng J, Liu F, Yuan C, et al. Calcium supplementation alleviates high-fat diet-induced estrous cycle irregularity and subfertility associated with concomitantly enhanced thermogenesis of brown adipose tissue and browning of white adipose tissue. J Agric Food Chem (2019) 67(25):7073–81. doi: 10.1021/acs.jafc.9b02663
146. Esler M. The sympathetic system and hypertension. Am J Hypertens (2000) 13(6 Pt 2):99S–105S. doi: 10.1016/S0895-7061(00)00225-9
147. Khan AA, Lip GYH, Shantsila A. Heart rate variability in atrial fibrillation: The balance between sympathetic and parasympathetic nervous system. Eur J Clin Invest (2019) 49(11):e13174. doi: 10.1111/eci.13174
148. Ouellet V, Labbé SM, Blondin DP, Phoenix S, Guérin B, Haman F, et al. Brown adipose tissue oxidative metabolism contributes to energy expenditure during acute cold exposure in humans. J Clin Invest (2012) 122(2):545–52. doi: 10.1172/JCI60433
149. Papanek PE, Wood CE, Fregly MJ. Role of the sympathetic nervous system in cold-induced hypertension in rats. J Appl Physiol (1985) 71(1):300–6. doi: 10.1152/jappl.1991.71.1.300
150. Notarius CF, Floras JS. Sympathetic neural responses in heart failure during exercise and after exercise training. Clin Sci (Lond) (2021) 135(4):651–69. doi: 10.1042/CS20201306
151. Nedergaard J, Cannon B. UCP1 mRNA does not produce heat. Biochim Biophys Acta (2013) 1831(5):943–9. doi: 10.1016/j.bbalip.2013.01.009
152. Shabalina IG, Ost M, Petrovic N, Vrbacky M, Nedergaard J, Cannon B, et al. Uncoupling protein-1 is not leaky. Biochim Biophys Acta (2010) 1797(6-7):773–84. doi: 10.1016/j.bbabio.2010.04.007
153. Bartelt A, Heeren J. Adipose tissue browning and metabolic health. Nat Rev Endocrinol (2014) 10(1):24–36. doi: 10.1038/nrendo.2013.204
154. Cheng L, Wang J, Dai H, Duan Y, An Y, Shi L, et al. Brown and beige adipose tissue: a novel therapeutic strategy for obesity and type 2 diabetes mellitus. Adipocyte (2021) 10(1):48–65. doi: 10.1080/21623945.2020.1870060
Keywords: obesity, brown adipose tissue (BAT), thermogenesis, uncoupling protein1 (UCP1), browning
Citation: Liu X, Zhang Z, Song Y, Xie H and Dong M (2023) An update on brown adipose tissue and obesity intervention: Function, regulation and therapeutic implications. Front. Endocrinol. 13:1065263. doi: 10.3389/fendo.2022.1065263
Received: 09 October 2022; Accepted: 19 December 2022;
Published: 11 January 2023.
Edited by:
Hendrik Lehnert, University of Salzburg, AustriaReviewed by:
Christopher J. Madden, Oregon Health and Science University, United StatesWuping Sun, Affiliated Nanshan Hospital of Shenzhen University Health Science Center, China
Copyright © 2023 Liu, Zhang, Song, Xie and Dong. This is an open-access article distributed under the terms of the Creative Commons Attribution License (CC BY). The use, distribution or reproduction in other forums is permitted, provided the original author(s) and the copyright owner(s) are credited and that the original publication in this journal is cited, in accordance with accepted academic practice. No use, distribution or reproduction is permitted which does not comply with these terms.
*Correspondence: Meng Dong, ZG9uZ21lbmdAaW96LmFjLmNu; Hengchang Xie, eGllaGNoQHNoYW5naGFpdGVjaC5lZHUuY24=
†These authors have contributed equally to this work