- 1College of Medicine, King Saud bin Abdulaziz University for Health Sciences, Ministry of National Guard Health Affairs, Riyadh, Saudi Arabia
- 2Department of Pediatrics, King Abdullah Specialized Children’s Hospital, King Abdulaziz Medical City, Ministry of National Guard Health Affairs, Riyadh, Saudi Arabia
- 3King Abdullah International Medical Research Center, Ministry of National Guard Health Affairs, Riyadh, Saudi Arabia
- 4Department of Pediatrics, McMaster University, Hamilton, ON, Canada
- 5Division of Pediatric Endocrinology, McMaster Children’s Hospital, Hamilton, ON, Canada
- 6Department of Health Research Methods, Evidence and Impact, McMaster University, Hamilton, ON, Canada
- 7Michael G. De Groote School of Medicine, McMaster University, Hamilton, ON, Canada
Type 2 diabetes (T2D) rates in children and adolescents are rising globally. T2D is a complex and aggressive disease in children with several comorbidities, high treatment failure rates, and insulin needs within a few years from diagnosis. While myriads of pharmacotherapies are licensed to treat adults with T2D, treatments accessible to children and adolescents have been limited until recently. Metformin is an old drug with multiple beneficial metabolic health effects beyond glycemic control. This review discusses Metformin’s origins, its mechanisms of action, and evidence for its use in the pediatric population to treat and prevent T2D. We also explore the evidence for its use as an obesity therapy, which is the primary driver of T2D, and T2D-driven comorbidities. While emerging therapies create new horizons for managing pediatric T2D, Metformin remains an inexpensive and safe part of the treatment plans of many T2D children globally for its beneficial metabolic effects.
Introduction
Pediatric type 2 diabetes (T2D) has become a global public health concern driven by childhood obesity (1–4). Multiple biosocial drivers contribute to the intertwining of both diseases (1, 5). Over the past three decades, T2D has increased steadily in children (6), with a 7.1% annual increase in incidence rates (3) and a 95% increase in prevalence in the USA over almost two decades (7). Recent global data suggest incidence rates of up to 31-94 per 100,000 per year and prevalence of up to 160-5,300 per 100,000 in high-risk populations, such as Indigenous and African youth (8). While initially described as a disease of Indigenous children, current data reveal a surge in T2D across different ethnic groups (9, 10). There are currently no population-based screening programs for T2D in children; the disease remains uncommon in children, with most cases of new-onset diabetes being type 1 diabetes. This pattern is coupled with the 30% reproducibility of one of the primary screening tests in children–the oral glucose tolerance test (11). The use of glycated hemoglobin A1c (HbA1c) has been purposed for monitoring glycemic control in these children rather than establishing the diagnosis alone (12, 13). The more reproducible test, fasting glucose, is not very practical in children for obvious reasons and does miss those who become hyperglycemic after a carbohydrate challenge.
As up to 50% of children with T2D are asymptomatic at presentation and are mostly diagnosed when screened because they have obesity, the current prevalence and incidence rates for T2D likely underestimate the true scale of the disease in children (9, 14).
T2D presents as an aggressive disease with multiple comorbidities, and patients have a progressive failure of β-cell function and progress to insulin dependence within 3-5 years post-diagnosis. This rapid deterioration of β-cell function is more rapid in children when compared to adults with T2D (15–19).
Several conditions are associated with T2D including obesity, dyslipidemia, hypertension, obstructive sleep apnea, polycystic ovary syndrome, and non-alcoholic fatty liver disease (20–24). Microvascular complications manifest as nephropathy, neuropathy, and retinopathy and present much earlier than those in children with type 1 diabetes (25).
T2D impacts the children’s longevity and quality of life, and the premature mortality seen in this population is a significant concern (26).
While initial approaches to treatment involved using lifestyle to manage diabetes, it has become clear that this approach needs supplementation with pharmacotherapy to establish and maintain glycemic control (27). So far, pharmacotherapies used to treat T2D include metformin, insulin, sodium-glucose cotransporter-2 (SGLT-2) inhibitors, and glucagon-like peptide-1 (GLP-1) receptor agonists (28–31). This review focuses on the use of Metformin in the management of T2D patients.
Metformin: an ancient drug
Metformin is the most widely prescribed pharmacotherapeutic agent to treat T2D globally (32–35). Metformin is a biguanide derived from a guanidine, galegine, from the French lilac or goat’s rue (Galega officinalis), which was used for centuries to treat diabetes (36). Metformin hydrochloride (HCl) is hydrophilic and has a molecular weight of 165.63 kDa (37). The molecular formula of Metformin HCl, the compound used in clinical preparations, is C4H11N5 • HCl (37). Metformin is hydrophilic and has a molecular weight of 129.16 kDa (38). The molecular formula of Metformin is C4H11N5 (38). For the remainder of the paper, the use of Metformin assumes the use of Metformin HCl.
Advances in chemistry and new drug manufacturing methods pioneered almost a century ago led to the synthesis of Metformin in 1922 as dimethylbiguanide (39). However, it did not come into broad use to treat diabetes initially. One of the most critical biomedical discoveries of the 20th century–insulin in 1921, overshadowed the use of other medications to treat diabetes (40). In 1957, Metformin was studied by Dr. Jean Sterne, who investigated its antihyperglycemic properties and called it Glucophage (glucose eater), a name that still resonates today as a brand name for metformin (41). Although the higher potency of other biguanides, such as phenformin, led to their prioritization in clinical trials (42), the development of lactic acidosis led to early discontinuation of the trials and the interest in Metformin was reignited (43, 44). While less potent than other guanidines, Metformin did possess a better safety profile (45). Furthermore, the U.K. Prospective Diabetes Study further supported the use of Metformin in T2D, with improved glycemic outcomes and decreased morbidity and mortality (46). These discoveries elevated Metformin to become the first-line therapy for adults with T2D (46, 47).
Metformin HCl was licensed in the United Kingdom in 1958 and in 1972 in Canada (48). The U.S. Food and Drug Administration (FDA) only approved the use of Metformin for T2D treatment in adults in December of 1994 and in children ≥10 years old with T2D in December of 2000 (49). Extended-release metformin formulations were subsequently approved (50), and the first extended-release liquid formulation followed more recently (51, 52). The availability of liquid formulations offers an alternative to Metformin tablets that may be large or hard to swallow for pediatric patients, leading to decreased adherence. Additionally, when evaluating the taste of liquid Metformin formulations, they were preferred compared to crushed Metformin tablets (53). Therefore, liquid Metformin may improve adherence to treatment in the pediatric population. However, the liquid form may not be readily available in all parts of the world.
Pharmacokinetics and pharmacodynamics
Metformin is taken orally. The typical doses to treat diabetes for immediate-release preparations are 500-2,000 mg/day. The leading site of metformin absorption is the small intestine in the duodenum and jejunum via the action of plasma membrane monoamine transporter (PMAT) in intestinal cells, with a minor contribution of the colon (54, 55).
Taken orally, Metformin has a bioavailability of up to 60%, and it is absorbed within 6 hours (56). After absorption, Metformin does not bind to plasma proteins and is distributed to the liver (57–59). The non-absorbed Metformin accumulates at the distal small intestine, and it is then eliminated in the feces (60).
Metformin has a mean elimination plasma half-life of around 5 hours. Its onset of action is at around 1.5 hours, and the total duration of action is 16-20 hours (61). The therapeutic concentration of Metformin has been debated, and there are limitations in the literature about the levels and their methods of measurement at clinically relevant doses. However, a widely accepted circulating level at therapeutic doses (20 mg/kg/day) is 10-40 μmol/L (62, 63). Metformin is excreted unchanged via renal tubular excretion as the major route for elimination (64).
The extended-release formulation is based on the combination of Metformin with polymer delivery systems that allow the tablet to absorb water and expand to 150% of its original size within 15 minutes of ingestion, anchoring it in the stomach (65, 66). The pill slowly releases Metformin into the small intestine over 8-9 hours. When the Metformin is released, the tablet’s excipients disintegrate and are excreted in the feces at around 15 hours post-ingestion. The main advantage of extended-release Metformin is the potential for improved adherence to treatment in children and reduced gastrointestinal side effects. This metformin formulation is administered once daily, and the typical dose of these preparations is 1000-2000 mg/day, with a half-life of 6.5 hours and actions on plasma glucose for 24 hours (65, 67) (68).
Mechanisms of action
Metformin exerts its glucose-lowering effect through various mechanisms in different organs (Figure 1).
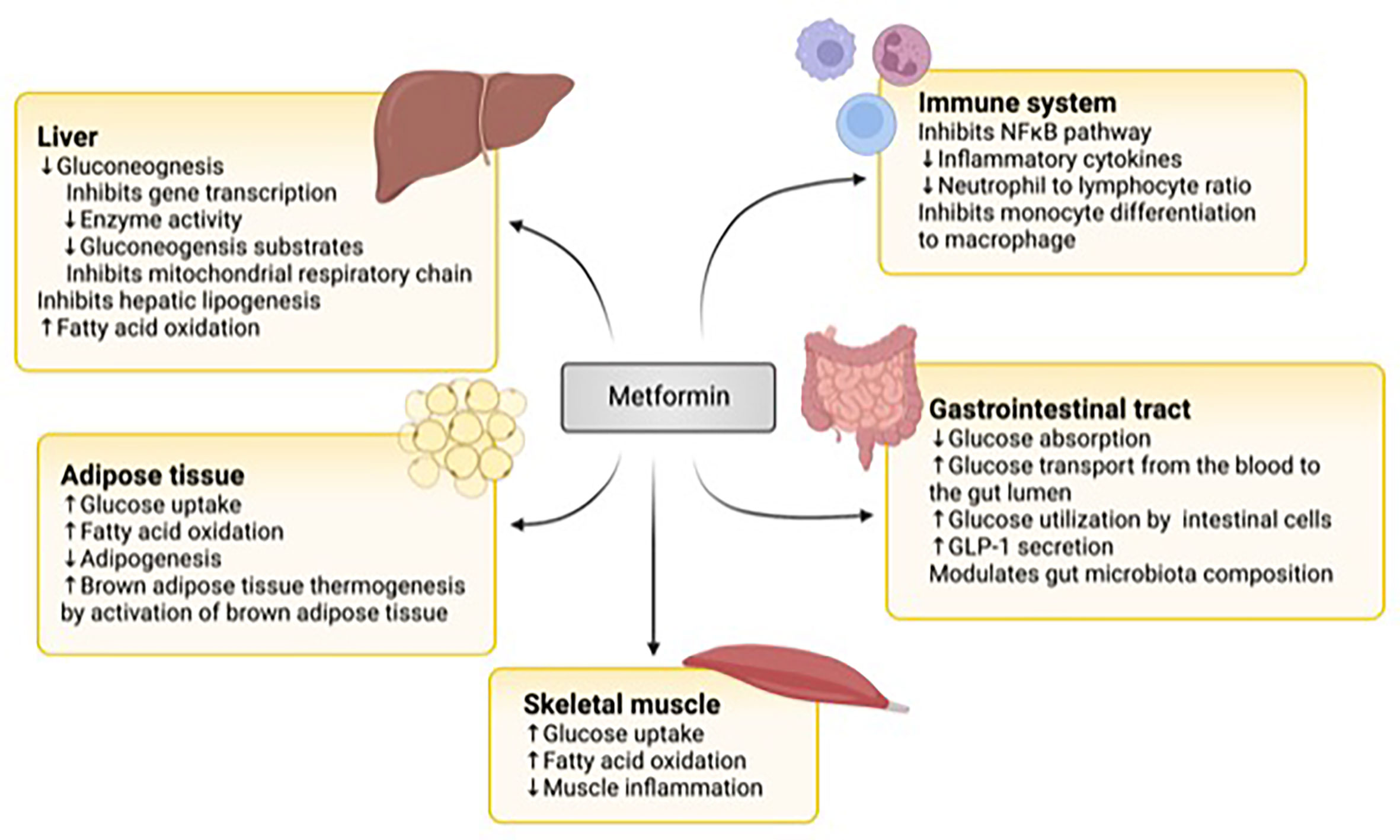
Figure 1 The mechanisms of action of metformin. In the liver, metformin downregulates gluconeogenesis by inhibiting gene transcription, gluconeogenic enzyme activity and substrates, and the mitochondrial respiratory chain. It also inhibits lipogenesis and upregulates fatty acid oxidation. In the adipose tissue, it increases glucose uptake and fatty acid oxidation and brown adipose tissue while downregulating adipogenesis. In skeletal muscle, metformin stimulates glucose uptake and fatty acid oxidation. Metformin inhibits the nuclear factor KB (NFKB) pathway in immune cells and the differentiation of monocytes to macrophages. It also lowers inflammatory cytokine production and the neutrophil to lymphocyte chain. In the gastrointestinal tract, it modulates the gut microbiome, decreases glucose absorption from the intestin, It also increases glucose transport from the blood to the gut lumen, glucose utilization by intestinal cells, and glucagon peptide-1 (GLP-1) secretion. Figure created with BioRender.com.
The liver is one of the important target organs of metformin actions. Metformin reduces hepatic glucose output by inhibiting gluconeogenesis (69, 70). While Metformin inhibits complex 1 of the respiratory chain in the mitochondria leading to an increase in adenosine monophosphate (AMP) and activation of AMP-activated protein kinase (AMPK), which in turn inhibits hepatic gluconeogenesis, the concentration of Metformin needed to achieve such effects in humans is supra-therapeutic (71, 72).
Additionally, Metformin inhibits hepatic gluconeogenesis by AMPK-independent mechanisms (73). These mechanisms include inhibiting gene transcription (73) and activity of enzymes in the gluconeogenic pathway (74), in addition to decreasing substrates available for gluconeogenesis such as lactate and glycerol (75, 76). Metformin also inhibits hepatic lipogenesis and increases fatty acid oxidation (77, 78).
Metformin’s additional actions include increasing glucose uptake and fatty acid oxidation in skeletal muscle and adipose tissue (78–82). It also reduces adipogenesis and activates brown adipose tissue leading to enhanced thermogenesis (83).
More recently, the effect of Metformin on the gut has been recognized (84). Metformin decreases the absorption of glucose from the intestine. It increases glucose transport from the blood into the lumen of the intestine (85–87), in addition to increasing glucose utilization by intestinal cells (88). Metformin also promotes the secretion of Glucagon-Like Peptide-1 (GLP-1) from the upper intestine, augmenting glucose-dependent insulin secretion (89, 90). Furthermore, Metformin influences the gut microbiome by promoting the growth of beneficial species that degrade mucin and generate short-chain fatty acids (91, 92). Animal studies have shown that mice given a metformin-modulated gut microbiome have improved glucose tolerance (92).
Metformin downregulates inflammation by inhibiting the NFϰB pathway and decreasing the secretion of inflammatory cytokines (93, 94). It also affects the proportion of white blood cells by reducing the ratio of neutrophils to lymphocytes (93) and inhibits the differentiation of monocytes to macrophages (95).
Treating type 2 diabetes with metformin
Lifestyle interventions encompassing healthy eating and physical activity recommendations need to be provided to children with T2D in a culturally and developmentally appropriate manner (96). While the exact endpoints of lifestyle interventions may not be easily achievable and are not solely focused on glycemic control, a suggested target is at least a 7% weight loss, presumed to improve metabolic markers such as HbA1c and C-peptide levels (97), and may help reduce the risk of microvascular complications (98). However, the aggressive nature of T2D and the high treatment failure rates have put a particular urgency on efforts to identify adjunct therapies to lifestyle interventions that may improve outcomes.
Currently, Metformin is the first pharmacotherapeutic choice for children with T2D with metabolic stability, including being asymptomatic and with an HbA1c <8.5% and blood glucose levels <250 mg/dL (<13.9 mmol/L) (96) (Tables 1, 2). In the presence of symptomatic hyperglycemia with polyuria, polydipsia, and weight loss, with biochemical deterioration and HbA1c >8.5% and blood glucose >250 mg/dL (≥14.0 mmol/L) and no acidosis, both Metformin and basal insulin are initiated (96). In general, Metformin treatment reduces HbA1c by 1-2% (99, 102).
A minority of patients with T2D present in diabetic ketoacidosis (DKA) at diagnosis (103). In these patients, conventional DKA management with insulin is initiated to reverse the acidosis; then Metformin is started in addition to subcutaneous insulin therapy for a few weeks and then a decision is made as to whether insulin can be weaned or stopped with continued Metformin treatment (96).
Because of its gastrointestinal side effects, Metformin should be started at a low dose with gradual titration, depending on tolerance. The typical metformin regimen involves a starting dose of 250-500 mg/day, with the weekly escalation of the dose up to a maximum of 1,000 mg twice daily or 850 mg three times per day (96, 104, 105). Extended-release formulations can be prescribed to reduce gastrointestinal side effects, or Metformin can be taken with meals.
Even though Metformin has been approved for use in pediatric T2D for decades, prospective studies in this population are limited, and trials of new medications have used them as add-ons to Metformin with or without insulin.
A randomized controlled trial of 16 weeks compared Metformin to a placebo in youth aged 10-16 with T2D. Metformin lowered HbA1c and fasting plasma glucose when compared to placebo (99).
Another study from Japan included youth with T2D and found that Metformin decreased HbA1c by 0.81% at 24 weeks and 0.46% at 52 weeks (101). While gastrointestinal side effects were widespread in almost all patients, no serious adverse events necessitated Metformin’s discontinuation over the 52-week study period (101).
Compared to glimepiride, a sulfonylurea, Metformin achieved similar reductions in HbA1c in those aged 8-17 years with T2D. While the incidence of hypoglycemia was similar in both groups, Metformin led to less weight gain compared to glimepiride (100).
Significant insights into the role of Metformin in pediatric T2D management were gleaned from the seminal TODAY study, a randomized controlled clinical trial that included 699 children with T2D. The trial compared Metformin with or without lifestyle interventions and Metformin plus rosiglitazone. The primary outcome was the need to use insulin due to failure in achieving glycemic control, defined as an HbA1c <8% for at least six months (106).
At a median of two months of metformin therapy during the run-in period, Metformin led to 91% of participants achieving an HbA1c <8%, with 78% achieving an HbA1c level less than 7% and 46% having an HbA1c level less than 6% (107). The benefits of Metformin waned over time, with only 49.3% for Metformin alone and 53.4% for the Metformin plus lifestyle intervention achieving durable glycemic control after four years (27).
Importantly, an HbA1c of <6.3% at randomization was associated with durable glycemic control (108). In participants with deteriorating glycemic control, a decline in β-cell function rather than reduced insulin sensitivity was noted (18, 109). Data from the Pediatric Diabetes Consortium T2D Registry confirmed this association of lower HbA1c at diagnosis with maintained glycemic control ≥2 years post-diagnosis (110).
Importantly, in youth with recent-onset T2D or impaired glucose tolerance, neither insulin glargine followed by metformin nor metformin alone preserved β-cell function (111). However, discontinuation of therapy resulted in worsening glycemic control, reflected by HbA1c, fasting and 2-hour post prandial glucose, as well as a decline in β-cell function after a 9-month washout period (112).
Future research efforts must concentrate on understanding Metformin’s glycemic effects and potential benefits in preventing or decreasing diabetes-related complications and cardiovascular events.
Metformin therapy of type 2 diabetes-related comorbidities
Obesity
Obesity is a significant risk factor for T2D in children, and the prevalence of overweight and obesity is close to 75% in youth with T2D (113). Metformin has been shown to reduce weight, BMI, and waist circumference (114–126). The weight loss associated with Metformin is usually modest and results from its action on the gastrointestinal tract leading to decreased appetite. Additionally, Metformin has a positive effect on markers of insulin resistance, such as fasting plasma glucose, fasting insulin levels, and homeostasis model assessment-estimated insulin resistance (HOMA-IR) (114, 116–118, 121, 122, 125). Interestingly, Metformin may improve weight, BMI, waist circumference, total adiposity, and abdominal adiposity in prepubertal children born small for gestational age (127).
Metformin reduces BMI by around 1.4 kg/m2 (125, 126, 128–131), and most clinical trials were relatively short, following participants for 6-12 months (114–116, 119–122). Most weight loss occurs in the first six months of treatment, tends to slow down, and is not necessarily sustained after treatment cessation (128–132). In an 18-month multicenter randomized controlled trial that included 42 participants (66% females) with a median age of 13, BMI improved in the metformin group at 6 and 9 months. Yet, it returned to baseline at the end of the 18- month study period (133). Additionally, an open-label 18- month extension of the study confirmed the lack of a sustainable effect of Metformin on BMI in adolescents with longer-term follow up (134). Interestingly, while the effect of Metformin on weight and BMI may not be sustained, youth with obesity treated with Metformin had less weight and BMI gain when compared to those who did not receive Metformin (135, 136).
While most studies demonstrate improvement in weight or BMI on treatment (114–122), trials of longer duration that may need to be combined with co-interventions are needed to address its long-term effects on body mass and metabolic health.
In the TODAY study, BMI at two years of treatment increased by 1.6 and 1.4 kg/m2 in the metformin and metformin plus lifestyle intervention groups, respectively (97). However, a subgroup of patients, representing about one-third in each of the metformin and the metformin plus lifestyle intervention groups, achieved a weight loss of ≥7% at 12 months and 24 months. This weight loss was associated with improved cardiometabolic health markers such as HbA1c, systolic blood pressure and lipid profile (97). These results suggest that a subgroup of youth with T2D may benefit from treatment with weight reduction and improved glycemic control and cardiometabolic markers. Defining this subgroup in detail is critical to personalize care and improve outcomes in pediatric T2D (Table 2).
Polycystic ovary syndrome
Polycystic ovary syndrome (PCOS) is characterized by menstrual irregularities with anovulation or oligo-ovulation with clinical and biochemical hyperandrogenism (137–141). The prevalence of PCOS in girls with T2D is close to 20% (21), compared with up to 11% in the general pediatric population (142). Hyperinsulinism and insulin resistance are essential drivers in the pathophysiology of PCOS (143–145), and this may be related to elevated insulin levels promoting luteinizing hormone secretion from the pituitary gland, with the combined effect of insulin and luteinizing hormone stimulation of the ovarian theca cells to produce androgens (146–148). Although obesity is a significant risk factor for PCOS, a proportion of adolescent girls with PCOS have a normal BMI and evidence of insulin resistance (145, 149, 150).
Metformin has been considered as a treatment option for PCOS. Small randomized controlled studies demonstrated that Metformin promoted a decrease in weight and BMI (151–153) and improved insulin sensitivity (152, 153). Metformin also had favorable effects on clinical and biochemical hyperandrogenism, with decreased free testosterone levels (151, 152, 154, 155) and improved hirsutism (153, 155) and menstrual irregularity (152, 154). Compared to combined oral contraceptive pills (OCPs), one of the main strategies employed for treating PCOS, Metformin is superior to OCPs in reducing BMI and improving insulin sensitivity (156). On the other hand, OCPs resulted in a more significant improvement in menstrual irregularity (156). Metformin and OCPs had an equivalent positive effect on reducing testosterone levels and improving hirsutism (156).
Given the high prevalence of PCOS in girls with T2D, using Metformin in this population may have the added benefit of improving the clinical and biochemical features of PCOS in addition to reducing weight, improving insulin sensitivity, and achieving glycemic targets (Table 2).
Non-alcoholic fatty liver disease
Non-alcoholic fatty liver disease is a spectrum of hepatic disorders that range from steatosis to steatohepatitis and can ultimately progress to cirrhosis. The steatosis is caused by abnormal hepatic lipid metabolism, with insulin resistance and hyperinsulinemia playing a central role (157–160). Fat mass expansion and insulin resistance in obesity lead to the mobilization of free fatty acids from the adipose tissue, which eventually enter the liver, exceeding its oxidation capacity (157–161). Additionally, hyperinsulinemia promotes hepatic lipogenesis (157–160). This combination of increased fatty acid influx, decreased fatty acid oxidation, and increased hepatic triglyceride synthesis leads to hepatic steatosis (157–160). About 50% of youth with T2D have NAFLD, and the degree of dysglycemia correlates with the severity of NAFLD (162, 163).
The current mainstay of therapy for NAFLD is lifestyle intervention, which has been shown to improve both radiologic and biochemical evidence of steatosis (164). Adding Metformin to lifestyle change, compared to placebo or vitamin E supplementation, did not significantly improve liver enzymes (165–167). However, while Metformin does not impact liver enzyme levels, including alanine transaminase (ALT) and aspartate transaminase (AST), it may help lessen histologic findings such as lobular inflammation and hepatocellular ballooning (165, 167), as well as reduce fatty deposits in the liver on ultrasound (166, 168) (Table 2). Although current evidence does not support the use of Metformin as a treatment for NAFLD, the stable liver enzymes profile with its use is reassuring (165–167).
Off-label use of metformin in children younger than 10 years of age and pre-adolescents
There is limited evidence that suggests that Metformin is safe and effective in improving weight or BMI as well as other metabolic markers of obesity in children younger than 10 years of age.
A randomized trial evaluating the use of Metformin to treat obesity in prepubertal children recruited 80 participants, who were randomized to either Metformin or placebo (169). At 6-months, the Metformin arm had a lower BMI z-score, as well as an improved quantitative insulin sensitivity check index (QUICKI) and a higher leptin-to-adiponectin ratio compared to the placebo arm, with no serious adverse events or cases of lactic acidosis (169). Similar results were reported by another study that included 18 prepubertal children, with improvement in weight and BMI SDS as well as the fat mass after 24 months of therapy (170). However, due to the small sample size, the study was underpowered to detect a statistically significant difference (170). In obese pre-adolescents with hyperinsulinemia, metformin reduced BMI, BMI SDS, weight, HOMA-IR, fasting insulin, and fasting glucose (122). Yet, there was no significant change in first-phase insulin secretion or insulin sensitivity by clamp studies (122).
Metformin may also have a positive effect on the body composition and metabolic profile of children with a history of being small for gestational age (SGA), given their risk of obesity and insulin resistance (127). A small pilot study that recruited 23 children 6-9 years of age with a history of SGA and catch-up growth with increased visceral fat were randomized to Metformin (n=6) and placebo (n=17) (127). After 24 months, the Metformin arm participants had lower weight and BMI SDS as well as lower total and abdominal fat compared to the control arm (127). Additionally, HOMA-IR improved in the Metformin group (127). There is a need for adequately powered randomized clinical trials to address the role of metformin in managing obesity in this age group.
Side effects and contraindications
Metformin has a favorable safety profile. The most common metformin-related side effects are gastrointestinal including diarrhea, abdominal pain, bloating, nausea, and loss of appetite. These side effects occurred in around 50% of participants in the TODAY study (27). Gastrointestinal side effects are typically temporary and tend to improve with time (27, 129, 131). These symptoms can be mitigated by gradual dose escalation, ingestion of Metformin alongside meals, or the administration of extended-release preparations.
Metformin has been linked with vitamin B12 deficiency, most likely due to its effect on the gastrointestinal tract (171–173). The most plausible mechanism is the alteration in the calcium-dependent binding of the intrinsic factor and vitamin B12 complex with the receptors in the terminal ileum (174, 175). However, small short-term studies that included pediatric patients on Metformin for various indications did not demonstrate an association between metformin and vitamin B12 deficiency (176, 177).
Serious adverse events related to Metformin, namely hypoglycemia and lactic acidosis, are rare. Metformin’s risk of hypoglycemia is very low, when used as monotherapy and when patients consume carbohydrates regularly (105). Hypoglycemia in metformin therapy typically occurs when insulin or other hypoglycemic medications are also used.
Lactic acidosis is a concerning side effect associated with Metformin in adults and occurs in the presence of hypoperfusion or hypoxemia (178). The risk of lactic acidosis with Metformin is negligible in children and adolescents on Metformin in obesity (129, 131). In the TODAY study, there was only one case of lactic acidosis that was deemed unrelated to metformin use (27). Additionally, there were no clinically significant cases of lactic acidosis reported in two other trials that included youth with T2D on metformin (99, 101). Therefore, Metformin does not seem to cause lactic acidosis in pediatric T2D patients. However, it can certainly occur with a concurrent illness or dehydration that may drive lactic acid build-up.
Contraindications to Metformin include significant cardiac impairment resulting in hypoperfusion and hypoxia (179). Additionally, significant liver impairment is another contraindication (37).
Metformin can be used safely in youth with elevated liver enzymes in the context of NAFLD, provided liver function is preserved (165–167). Until recently, Metformin was also contraindicated for renal impairment. However, the FDA has updated its labelling to indicate that Metformin can be used safely in mild and some cases of moderate renal impairment (180). Metformin is contraindicated when eGFR is less than 30 ml/min/1.73 m2 and is not recommended when eGFR is between 30 and 45 ml/min/1.73 m2 (37, 180). Metformin should be stopped in cases of acute illness if there is a risk of dehydration, poor fluid intake, and hypoxemia, such as sepsis (179). Additionally, Metformin should be withheld before surgery and restarted once oral intake has resumed (37, 179). Metformin should also be discontinued before administration of radiographic contrast and for 48 hours afterwards until renal function is re-evaluated (37, 179).
Metformin treatment to prevent T2D
Since T2D is a severe and aggressive metabolic disorder in children, prevention efforts have targeted the upstream events in its development, including pre-diabetes (181) (Table 2).
The current worldwide pooled prevalence of pre-diabetes is 8.84% (182). Up to 8% of children and adolescents with pre-diabetes progress to T2D, with increased body mass index (BMI)-based measures being essential predictors of progression (183–185). While adult studies have shown that Metformin can help prevent the progression to T2D (186–188), most pediatric studies have focused on markers of insulin sensitivity rather than T2D development as an outcome.
The effect of Metformin on markers of glycemic control or insulin resistance, including fasting plasma glucose, HbA1c, fasting insulin, and HOMA-IR, has been variable. Trials that treated youth with obesity and clinical or biochemical signs of insulin resistance with Metformin provided mixed results. Some studies demonstrated a reduction in fasting plasma glucose and insulin levels with an improvement in insulin sensitivity; yet, there were no differences in insulin levels or HOMA-IR in other studies (115, 116, 189–191). In a small, randomized controlled trial that included children and youth with impaired glucose tolerance regardless of weight, Metformin treatment for three months reduced HbA1c and HOMA-IR (192). However, Metformin may not have a clear beneficial effect on β-cell preservation during puberty in children and adolescents with obesity and normoglycemia. A randomized controlled study that followed 44 normoglycemic children and adolescents with obesity from early puberty to puberty completion demonstrated that, although Metformin improved anthropometric parameters such as BMI z-score, waist circumference and percent body fat, it did not have a beneficial effect on insulin sensitivity or β-cell function compared to placebo (193).
The Metformin in Obese Children and Adolescents (MOCA) trial included children and adolescents with obesity and impaired glucose tolerance or hyperinsulinemia that were randomized to Metformin versus placebo. There was minimal improvement in fasting glucose levels at three months, and that trend was not persistent at six months (119). Similarly, in another randomized controlled trial that included children and adolescents with obesity and insulin resistance based on HOMA-IR, there was no significant difference in HbA1c or HOMA-IR when comparing Metformin to placebo over 18 months of therapy (133). Moreover, an open label 18-month extension of this study found that there was a progressive increase in both BMI and HOMA-IR in participants who continued Metformin (134).
In summary, while Metformin helps reduce the progression to T2D in adults and may positively influence markers of glycemic control and insulin sensitivity in children and adolescents, current evidence on the role of Metformin in preventing progression from pre-diabetes to T2D in youth is lacking. Adequately powered trials with long-term follow-up focusing on T2D prevention as a primary outcome in youth are required.
Conclusion
Metformin is considered first-line therapy in newly diagnosed pediatric patients with T2D, with or without insulin. It is generally considered a well-tolerated and safe medication. Even though Metformin has been approved for use in children for almost three decades, long-term prospective studies in this population are lacking. They are needed to better understand Metformin’s role in reducing T2D complications and preventing progression from pre-diabetes to diabetes. In addition to improving glycemic control and insulin sensitivity, Metformin may support BMI reduction which is important in improving insulin sensitivity.
Author contributions
MCS is the guarantor. The paper topics, layout, writing, editing, and final approval was done by both authors. All authors contributed to the article and approved the submitted version.
Acknowledgments
We would like to thank Ms. Subin Park for her editorial assistance and help in designing the figure.
Conflict of interest
The authors declare that the research was conducted in the absence of any commercial or financial relationships that could be construed as a potential conflict of interest.
Publisher’s note
All claims expressed in this article are solely those of the authors and do not necessarily represent those of their affiliated organizations, or those of the publisher, the editors and the reviewers. Any product that may be evaluated in this article, or claim that may be made by its manufacturer, is not guaranteed or endorsed by the publisher.
References
1. Candler TP, Mahmoud O, Lynn RM, Majbar AA, Barrett TG, Shield JPH. Continuing rise of type 2 diabetes incidence in children and young people in the UK. Diabetic Med (2018) 35(6):737–44. doi: 10.1111/dme.13609
2. Dabelea D, Mayer-Davis EJ, Saydah S, et al. Prevalence of type 1 and type 2 diabetes among children and adolescents from 2001 to 2009. JAMA (2014) 311(17):1778–86. doi: 10.1001/jama.2014.3201
3. Mayer-Davis EJ, Dabelea D, Lawrence JM. Incidence trends of type 1 and type 2 diabetes among youths, 2002-2012. N Engl J Med (2017) 377(3):301. doi: 10.1056/NEJMc1706291
4. Swinburn BA, Sacks G, Hall KD, McPherson K, Finegood DT, Moodie ML, et al. The global obesity pandemic: shaped by global drivers and local environments. Lancet (2011) 378(9793):804–14. doi: 10.1016/S0140-6736(11)60813-1
5. Carino M, Elia Y, Sellers E, Curtis J, McGavock J, Scholey J, et al. Comparison of clinical and social characteristics of Canadian youth living with type 1 and type 2 diabetes. Can J Diabet (2021) 45(5):428–35. doi: 10.1016/j.jcjd.2021.01.008
6. Amed S, Islam N, Sutherland J, Reimer K. Incidence and prevalence trends of youth-onset type 2 diabetes in a cohort of Canadian youth: 2002-2013. Pediatr Diabetes (2018) 19(4):630–6. doi: 10.1111/pedi.12631
7. Lawrence JM, Divers J, Isom S, Saydah S, Imperatore G, Pihoker C, et al. Trends in prevalence of type 1 and type 2 diabetes in children and adolescents in the US, 2001-2017. JAMA (2021) 326(8):717–27. doi: 10.1001/jama.2021.11165
8. International Diabetes Federation. IDF diabetes atlas. Available at: https://www.diabetesatlas.org (Accessed 08-03-2022).
9. Amed S, Dean HJ, Panagiotopoulos C, Sellers EA, Hadjiyannakis S, Laubscher TA, et al. Type 2 diabetes, medication-induced diabetes, and monogenic diabetes in Canadian children: a prospective national surveillance study. research support, non-U.S. gov't. Diabetes Care (2010) 33(4):786–91. doi: 10.2337/dc09-1013
10. Sellers EA, Moore K, Dean HJ. Clinical management of type 2 diabetes in indigenous youth. Review Pediatr Clin North Am (2009) 56(6):1441–59. doi: 10.1016/j.pcl.2009.09.013
11. Libman IM, Barinas-Mitchell E, Bartucci A, Robertson R, Arslanian S. Reproducibility of the oral glucose tolerance test in overweight children. J Clin Endocrinol Metab (2008) 93(11):4231–7. doi: 10.1210/jc.2008-0801
12. Lee JM, Wu EL, Tarini B, Herman WH, Yoon E. Diagnosis of diabetes using hemoglobin A1c: Should recommendations in adults be extrapolated to adolescents? J Pediatr (2011) 158(6):947–952 e1-3. doi: 10.1016/j.jpeds.2010.11.026
13. Nowicka P, Santoro N, Liu H, Lartaud D, Shaw MM, Goldberg R, et al. Utility of hemoglobin A(1c) for diagnosing prediabetes and diabetes in obese children and adolescents. Diabetes Care (2011) 34(6):1306–11. doi: 10.2337/dc10-1984
14. Mayer-Davis EJ, Beyer J, Bell RA, Dabelea D, D'Agostino R Jr, Imperatore G, et al. Diabetes in African American youth: Prevalence, incidence, and clinical characteristics: The SEARCH for diabetes in youth study. Diabetes Care (2009) 32 Suppl 2:S112–22. doi: 10.2337/dc09-S203
15. Elder DA, Hornung LN, Herbers PM, Prigeon R, Woo JG, D'Alessio DA. Rapid deterioration of insulin secretion in obese adolescents preceding the onset of type 2 diabetes. J Pediatr (2015) 166(3):672–8. doi: 10.1016/j.jpeds.2014.11.029
16. Elder DA, Hornung LN, Khoury JC, D'Alessio DA. Beta-cell function over time in adolescents with new type 2 diabetes and obese adolescents without diabetes. J Adolesc Health (2017) 61(6):703–8. doi: 10.1016/j.jadohealth.2017.06.007
17. Gungor N, Bacha F, Saad R, Janosky J, Arslanian S. Youth type 2 diabetes: Insulin resistance, beta-cell failure, or both? Diabetes Care (2005) 28(3):638–44. doi: 10.2337/diacare.28.3.638
18. Narasimhan S, Weinstock RS. Youth-onset type 2 diabetes mellitus: Lessons learned from the TODAY study. Mayo Clin Proc (2014) 89(6):806–16. doi: 10.1016/j.mayocp.2014.01.009
19. Rise Consortium, Investigators RC. Effects of treatment of impaired glucose tolerance or recently diagnosed type 2 diabetes with metformin alone or in combination with insulin glargine on beta-cell function: Comparison of responses in youth and adults. Diabetes (2019) 68(8):1670–80. doi: 10.2337/db19-0299
20. Cioana M, Deng J, Hou M, Nadarajah A, Qiu Y, Chen SSJ, et al. Prevalence of hypertension and albuminuria in pediatric type 2 diabetes: A systematic review and meta-analysis. JAMA Netw Open (2021) 4(4):e216069. doi: 10.1001/jamanetworkopen.2021.6069
21. Cioana M, Deng J, Nadarajah A, Hou M, Qiu Y, Chen SSJ, et al. Prevalence of polycystic ovary syndrome in patients with pediatric type 2 diabetes: A systematic review and meta-analysis. JAMA Netw Open (2022) 5(2):e2147454–e2147454. doi: 10.1001/jamanetworkopen.2021.47454
22. Copeland KC, Zeitler P, Geffner M, Guandalini C, Higgins J, Hirst K, et al. Characteristics of adolescents and youth with recent-onset type 2 diabetes: the TODAY cohort at baseline. J Clin Endocrinol Metab (2011) 96(1):159–67. doi: 10.1210/jc.2010-1642
23. Newfield RS, Graves CL, Newbury RO, Schwimmer JB, Proudfoot JA, Say DS, et al. Non-alcoholic fatty liver disease in pediatric type 2 diabetes: Metabolic and histologic characteristics in 38 subjects. Pediatr Diabet Feb (2019) 20(1):41–7. doi: 10.1111/pedi.12798
24. Sellers EA, Yung G, Dean HJ. Dyslipidemia and other cardiovascular risk factors in a Canadian first nation pediatric population with type 2 diabetes mellitus. Pediatr Diabet (2007) 8(6):384–90. doi: 10.1111/j.1399-5448.2007.00284.x
25. Dabelea D, Stafford JM, Mayer-Davis EJ, D'Agostino R Jr, Dolan L, Imperatore G, et al. Association of type 1 diabetes vs type 2 diabetes diagnosed during childhood and adolescence with complications during teenage years and young adulthood. JAMA (2017) 317(8):825–35. doi: 10.1001/jama.2017.0686
26. Constantino MI, Molyneaux L, Limacher-Gisler F, Al-Saeed A, Luo C, Wu T, et al. Long-term complications and mortality in young-onset diabetes: type 2 diabetes is more hazardous and lethal than type 1 diabetes. Diabetes Care (2013) 36(12):3863–9. doi: 10.2337/dc12-2455
27. Today Study Group, Zeitler P, Hirst K, Pyle L, Linder B, Copeland K, et al. A clinical trial to maintain glycemic control in youth with type 2 diabetes. N Engl J Med (2012) 366(24):2247–56. doi: 10.1056/NEJMoa1109333
28. Arslanian SA, Hannon T, Zeitler P, Chao LC, Boucher-Berry C, Barrientos-Perez M, et al. Once-weekly dulaglutide for the treatment of youths with type 2 diabetes. N Engl J Med (2022) 387(5):433–43. doi: 10.1056/NEJMoa2204601
29. Tamborlane WV, Barrientos-Perez M, Fainberg U, Frimer-Larsen H, Hafez M, Hale PM, et al. Liraglutide in children and adolescents with type 2 diabetes. N Engl J Med (2019) 381(7):637–46. doi: 10.1056/NEJMoa1903822
30. Tamborlane WV, Bishai R, Geller D, Shehadeh N, Al-Abdulrazzaq D, Vazquez EM, et al. Once-weekly exenatide in youth with type 2 diabetes. Diabetes Care (2022) 45(8):1833–40. doi: 10.2337/dc21-2275
31. Tamborlane WV, Laffel LM, Shehadeh N, Isganaitis E, Van Name M, Ratnayake J, et al. Efficacy and safety of dapagliflozin in children and young adults with type 2 diabetes: A prospective, multicentre, randomised, parallel group, phase 3 study. Lancet Diabetes Endocrinol (2022) 10(5):341–50. doi: 10.1016/S2213-8587(22)00052-3
32. Allyhiani M, Kurdi A, Abdulaziz A, Faqeh S, Alhajjaji A, Alansari S, et al. Prescribing patterns of antidiabetics in type 2 diabetes and factors affecting them. Saudi Pharm J (2022) 30(2):112–9. doi: 10.1016/j.jsps.2021.12.019
33. Campbell DJT, Campbell DB, Ogundeji Y, Au F, Beall R, Ronksley PE, et al. First-line pharmacotherapy for incident type 2 diabetes: Prescription patterns, adherence and associated costs. Diabetes Med (2021) 38(9):e14622. doi: 10.1111/dme.14622
34. Vajravelu ME, Hitt TA, Amaral S, Levitt Katz LE, Lee JM, Kelly A. Real-world treatment escalation from metformin monotherapy in youth-onset type 2 diabetes mellitus: A retrospective cohort study. Pediatr Diabet (2021) 22(6):861–71. doi: 10.1111/pedi.13232
35. van den Heuvel JM, Farzan N, van Hoek M, Maitland-van der Zee AH, Ahmadizar F. Mining treatment patterns of glucose-lowering medications for type 2 diabetes in the Netherlands. BMJ Open Diabetes Res Care (2020) 8(1):1–7. doi: 10.1136/bmjdrc-2019-000767
36. Bailey CJ, Day C. Traditional plant medicines as treatments for diabetes. Diabetes Care (1989) 12(8):553–64. doi: 10.2337/diacare.12.8.553
37. FDALabel. Glucophage (metformin hydrochloride) tablets . Available at: https://www.accessdata.fda.gov/drugsatfda_docs/label/2017/020357s037s039,021202s021s023lbl.pdf (Accessed Sep. 4, 2022).
38. National Center for Biotechnology Information. PubChem compound summary for CID 4091, metformin . Available at: https://pubchem.ncbi.nlm.nih.gov/compound/Metformin (Accessed Sep. 4, 2022).
39. Werner EA, Bell J. The preparation of methylguanidine, and of ββ-dimethylguanidine by the interaction of dicyanodiamide, and methylammonium and dimethylammonium chlorides respectively. J Chem Soc Trans (1922) 121:1790–4. doi: 10.1039/CT9222101790
40. Banting FG, Best CH, Collip JB, Campbell WR, Fletcher AA. Pancreatic extracts in the treatment of diabetes mellitus. Can Med Assoc J (1922) 2:141–6.
41. Sterne J. Blood sugar-lowering effect of 1,1-dimethylbiguanide. Therapie (1958) 13(4):650–9. L'action hypoglycemiante de la N-N-dimethyl-guanyl guanidine.
42. Mc KJ, Kuwayti K, Rado PP. Clinical experience with DBI (phenformin) in the management of diabetes. Can Med Assoc J (1959) 80(10):773–8.
43. Luft D, Schmulling RM, Eggstein M. Lactic acidosis in biguanide-treated diabetics: a review of 330 cases. Diabetologia (1978) 14(2):75–87. doi: 10.1007/BF01263444
44. Walker RS, Linton AL. Phenethyldiguanide: A dangerous side-effect. Br Med J (1959) 2(5158):1005–6. doi: 10.1136/bmj.2.5158.1005
45. Sterne J. Report on 5-years' experience with dimethylbiguanide (metformin, glucophage) in diabetic therapy. Wien Med Wochenschr (1963) 113:599–602.
46. King P, Peacock I, Donnelly R. The UK prospective diabetes study (UKPDS): clinical and therapeutic implications for type 2 diabetes. Br J Clin Pharmacol (1999) 48(5):643–8. doi: 10.1046/j.1365-2125.1999.00092.x
47. American Diabetes A. 9. pharmacologic approaches to glycemic treatment: Standards of medical care in diabetes-2021. Diabetes Care (2021) 44(Suppl 1):S111–24. doi: 10.2337/dc21-S009
48. Bailey CJ. Metformin: Historical overview. Diabetologia (2017) 60(9):1566–76. doi: 10.1007/s00125-017-4318-z
49. Buck ML. Use of metformin in pediatric patients. Available at: https://med.virginia.edu/pediatrics/wp-content/uploads/sites/237/2015/12/200407.pdf (Accessed Sep. 4, 2022).
50. Drug Approval Package. Glucophage XR (Metformin hydrochloride) extended release tablets . Available at: https://www.accessdata.fda.gov/drugsatfda_docs/nda/2000/21-202_Glucophage.cfm (Accessed Sep. 4, 2022).
51. Sun pharma launches RIOMET ER oral suspension in the U.S . Available at: http://www.riometer.com/RIOMET_ER_Press_Release_2020.pdf (Accessed Sep. 4, 2022).
52. . Available at: https://www.fda.gov/media/131715/download (Accessed Sep. 4, 2022).
53. Marshall AC, Damstra M, Tuley M, Schifando EL. Assessment of taste and grittiness of Riomet((R)) ER strawberry, Riomet((R)) ER grape, Riomet((R)) cherry, and metformin immediate-release tablets in healthy subjects. Drugs R D (2019) 19(1):57–66. doi: 10.1007/s40268-018-0260-x
54. Graham GG, Punt J, Arora M, Day RO, Doogue MP, Duong JK, et al. Clinical pharmacokinetics of metformin. Clin Pharmacokinet (2011) 50(2):81–98. doi: 10.2165/11534750-000000000-00000
55. Zhou M, Xia L, Wang J. Metformin transport by a newly cloned proton-stimulated organic cation transporter (plasma membrane monoamine transporter) expressed in human intestine. Drug Metab Dispos (2007) 35(10):1956–62. doi: 10.1124/dmd.107.015495
56. Scheen AJ. Clinical pharmacokinetics of metformin. Clin Pharmacokinet (1996) 30(5):359–71. doi: 10.2165/00003088-199630050-00003
57. Wilcock C, Wyre ND, Bailey CJ. Subcellular distribution of metformin in rat liver. J Pharm Pharmacol (1991) 43(6):442–4. doi: 10.1111/j.2042-7158.1991.tb03507.x
58. Tucker GT, Casey C, Phillips PJ, Connor H, Ward JD, Woods HF. Metformin kinetics in healthy subjects and in patients with diabetes mellitus. Br J Clin Pharmacol (1981) 12(2):235–46. doi: 10.1111/j.1365-2125.1981.tb01206.x
59. Beckmann R. Absorption, distribution in the organism and elimination of metformin. Diabetologia (1969) 5(5):318–24. doi: 10.1007/BF00452906. Resorption, Verteilung im Organismus und Ausscheidung von Metformin.
60. Bailey CJ, Wilcock C, Scarpello JH. Metformin and the intestine. Diabetologia (2008) 51(8):1552–3. doi: 10.1007/s00125-008-1053-5
61. Castelino RL, Peterson G. Prescribing of metformin based on estimated GFR rather than serum creatinine expands the eligible population and is likely safe. Evidence-Based Med (2016) 21(2):68. doi: 10.1136/ebmed-2015-110351
62. Kajbaf F, De Broe ME, Lalau JD. Therapeutic concentrations of metformin: A systematic review. Clin Pharmacokinet (2016) 55(4):439–59. doi: 10.1007/s40262-015-0323-x
63. He L, Wondisford FE. Metformin action: Concentrations matter. Cell Metab (2015) 21(2):159–62. doi: 10.1016/j.cmet.2015.01.003
64. Pentikainen PJ, Neuvonen PJ, Penttila A. Pharmacokinetics of metformin after intravenous and oral administration to man. Eur J Clin Pharmacol (1979) 16(3):195–202. doi: 10.1007/BF00562061
65. Timmins P, Donahue S, Meeker J, Marathe P. Steady-state pharmacokinetics of a novel extended-release metformin formulation. Clin Pharmacokinet (2005) 44(7):721–9. doi: 10.2165/00003088-200544070-00004
66. Gusler G, Gorsline J, Levy G, Zhang SZ, Weston IE, Naret D, et al. Pharmacokinetics of metformin gastric-retentive tablets in healthy volunteers. J Clin Pharmacol (2001) 41(6):655–61. doi: 10.1177/00912700122010546
67. Cullen E, Liao J, Lukacsko P, Niecestro R, Friedhoff L. Pharmacokinetics and dose proportionality of extended-release metformin following administration of 1000, 1500, 2000 and 2500 mg in healthy volunteers. Biopharm Drug Dispos (2004) 25(6):261–3. doi: 10.1002/bdd.407
68. FDA. Available at: https://www.accessdata.fda.gov/drugsatfda_docs/label/2009/021748s005lbl.pdf (Accessed October 16, 2022).
69. Hundal RS, Krssak M, Dufour S, Laurent D, Lebon V, Chandramouli V, et al. Mechanism by which metformin reduces glucose production in type 2 diabetes. Diabetes (2000) 49(12):2063–9. doi: 10.2337/diabetes.49.12.2063
70. Inzucchi SE, Maggs DG, Spollett GR, Page SL, Rife FS, Walton V, et al. Efficacy and metabolic effects of metformin and troglitazone in type II diabetes mellitus. N Engl J Med (1998) 338(13):867–72. doi: 10.1056/NEJM199803263381303
71. Owen MR, Doran E, Halestrap AP. Evidence that metformin exerts its anti-diabetic effects through inhibition of complex 1 of the mitochondrial respiratory chain. Biochem J (2000) 348 Pt 3:607–14. doi: 10.1042/bj3480607
72. Stephenne X, Foretz M, Taleux N, van der Zon GC, Sokal E, Hue L, et al. Metformin activates AMP-activated protein kinase in primary human hepatocytes by decreasing cellular energy status. Diabetologia (2011) 54(12):3101–10. doi: 10.1007/s00125-011-2311-5
73. Foretz M, Hebrard S, Leclerc J, Zarrinpashneh E, Soty M, Mithieux G, et al. Metformin inhibits hepatic gluconeogenesis in mice independently of the LKB1/AMPK pathway via a decrease in hepatic energy state. J Clin Invest (2010) 120(7):2355–69. doi: 10.1172/JCI40671
74. Hunter RW, Hughey CC, Lantier L, Sundelin EI, Peggie M, Zeqiraj E, et al. Metformin reduces liver glucose production by inhibition of fructose-1-6-bisphosphatase. Nat Med (2018) 24(9):1395–406. doi: 10.1038/s41591-018-0159-7
75. Madiraju AK, Qiu Y, Perry RJ, Rahimi Y, Zhang XM, Zhang D, et al. Metformin inhibits gluconeogenesis via a redox-dependent mechanism in vivo. Nat Med (2018) 24(9):1384–94. doi: 10.1038/s41591-018-0125-4
76. Radziuk J, Zhang Z, Wiernsperger N, Pye S. Effects of metformin on lactate uptake and gluconeogenesis in the perfused rat liver. Diabetes (1997) 46(9):1406–13. doi: 10.2337/diab.46.9.1406
77. Boudaba N, Marion A, Huet C, Pierre R, Viollet B, Foretz M. AMPK re-activation suppresses hepatic steatosis but its downregulation does not promote fatty liver development. EBioMedicine (2018) 28:194–209. doi: 10.1016/j.ebiom.2018.01.008
78. Tokubuchi I, Tajiri Y, Iwata S, Hara K, Wada N, Hashinaga T, et al. Beneficial effects of metformin on energy metabolism and visceral fat volume through a possible mechanism of fatty acid oxidation in human subjects and rats. PloS One (2017) 12(2):e0171293. doi: 10.1371/journal.pone.0171293
79. Anedda A, Rial E, Gonzalez-Barroso MM. Metformin induces oxidative stress in white adipocytes and raises uncoupling protein 2 levels. J Endocrinol (2008) 199(1):33–40. doi: 10.1677/JOE-08-0278
80. Chen SC, Brooks R, Houskeeper J, Bremner SK, Dunlop J, Viollet B, et al. Metformin suppresses adipogenesis through both AMP-activated protein kinase (AMPK)-dependent and AMPK-independent mechanisms. Mol Cell Endocrinol (2017) 440:57–68. doi: 10.1016/j.mce.2016.11.011
81. Cokorinos EC, Delmore J, Reyes AR, Albuquerque B, Kjobsted R, Jorgensen NO, et al. Activation of skeletal muscle AMPK promotes glucose disposal and glucose lowering in non-human primates and mice. Cell Metab (2017) 25(5):1147–1159 e10. doi: 10.1016/j.cmet.2017.04.010
82. Grisouard J, Timper K, Radimerski TM, Frey DM, Peterli R, Kola B, et al. Mechanisms of metformin action on glucose transport and metabolism in human adipocytes. Biochem Pharmacol (2010) 80(11):1736–45. doi: 10.1016/j.bcp.2010.08.021
83. Geerling JJ, Boon MR, van der Zon GC, van den Berg SA, van den Hoek AM, Lombes M, et al. Metformin lowers plasma triglycerides by promoting VLDL-triglyceride clearance by brown adipose tissue in mice. Diabetes (2014) 63(3):880–91. doi: 10.2337/db13-0194
84. Buse JB, DeFronzo RA, Rosenstock J, Kim T, Burns C, Skare S, et al. The primary glucose-lowering effect of metformin resides in the gut, not the circulation: Results from short-term pharmacokinetic and 12-week dose-ranging studies. Diabetes Care (2016) 39(2):198–205. doi: 10.2337/dc15-0488
85. Ito J, Nogami M, Morita Y, Sakaguchi K, Komada H, Hirota Y, et al. Dose-dependent accumulation of glucose in the intestinal wall and lumen induced by metformin as revealed by (18) f-labelled fluorodeoxyglucose positron emission tomography-MRI. Diabetes Obes Metab (2021) 23(3):692–9. doi: 10.1111/dom.14262
86. Koffert JP, Mikkola K, Virtanen KA, Andersson AD, Faxius L, Hallsten K, et al. Metformin treatment significantly enhances intestinal glucose uptake in patients with type 2 diabetes: Results from a randomized clinical trial. Diabetes Res Clin Pract (2017) 131:208–16. doi: 10.1016/j.diabres.2017.07.015
87. Morita Y, Nogami M, Sakaguchi K, Okada Y, Hirota Y, Sugawara K, et al. Enhanced release of glucose into the intraluminal space of the intestine associated with metformin treatment as revealed by [(18)F]Fluorodeoxyglucose PET-MRI. Diabetes Care (2020) 43(8):1796–802. doi: 10.2337/dc20-0093
88. Bailey CJ, Mynett KJ, Page T. Importance of the intestine as a site of metformin-stimulated glucose utilization. Br J Pharmacol (1994) 112(2):671–5. doi: 10.1111/j.1476-5381.1994.tb13128.x
89. Bahne E, Sun EWL, Young RL, Hansen M, Sonne DP, Hansen JS, et al. Metformin-induced glucagon-like peptide-1 secretion contributes to the actions of metformin in type 2 diabetes. JCI Insight (2018) 3(23):1–15. doi: 10.1172/jci.insight.93936
90. Mannucci E, Tesi F, Bardini G, Ognibene A, Petracca MG, Ciani S, et al. Effects of metformin on glucagon-like peptide-1 levels in obese patients with and without type 2 diabetes. Diabetes Nutr Metab (2004) 17(6):336–42.
91. de la Cuesta-Zuluaga J, Mueller NT, Corrales-Agudelo V, Velasquez-Mejia EP, Carmona JA, Abad JM, et al. Metformin is associated with higher relative abundance of mucin-degrading akkermansia muciniphila and several short-chain fatty acid-producing microbiota in the gut. Diabetes Care (2017) 40(1):54–62. doi: 10.2337/dc16-1324
92. Wu H, Esteve E, Tremaroli V, Khan MT, Caesar R, Manneras-Holm L, et al. Metformin alters the gut microbiome of individuals with treatment-naive type 2 diabetes, contributing to the therapeutic effects of the drug. Nat Med (2017) 23(7):850–8. doi: 10.1038/nm.4345
93. Cameron AR, Morrison VL, Levin D, Mohan M, Forteath C, Beall C, et al. Anti-inflammatory effects of metformin irrespective of diabetes status. Circ Res (2016) 119(5):652–65. doi: 10.1161/CIRCRESAHA.116.308445
94. Li Y, Gappy S, Liu X, Sassalos T, Zhou T, Hsu A, et al. Metformin suppresses pro-inflammatory cytokines in vitreous of diabetes patients and human retinal vascular endothelium. PloS One (2022) 17(7):e0268451. doi: 10.1371/journal.pone.0268451
95. Vasamsetti SB, Karnewar S, Kanugula AK, Thatipalli AR, Kumar JM, Kotamraju S. Metformin inhibits monocyte-to-macrophage differentiation via AMPK-mediated inhibition of STAT3 activation: Potential role in atherosclerosis. Diabetes (2015) 64(6):2028–41. doi: 10.2337/db14-1225
96. American Diabetes Association Professional Practice C, Draznin B, Aroda VR, Bakris G, Benson G, Brown FM, et al. Children and adolescents: Standards of medical care in diabetes-2022. Diabetes Care (2022) 45(Suppl 1):S208–31. doi: 10.2337/dc22-S014
97. Marcus MD, Wilfley DE, El Ghormli L, Zeitler P, Linder B, Hirst K, et al. Weight change in the management of youth-onset type 2 diabetes: The TODAY clinical trial experience. Pediatr Obes (2017) 12(4):337–45. doi: 10.1111/ijpo.12148
98. Today Study Group, Bjornstad P, Drews KL, Caprio S, Gubitosi-Klug R, Nathan DM, et al. Long-term complications in youth-onset type 2 diabetes. N Engl J Med (2021) 385(5):416–26. doi: 10.1056/NEJMoa2100165
99. Jones KL, Arslanian S, Peterokova VA, Park JS, Tomlinson MJ. Effect of metformin in pediatric patients with type 2 diabetes: A randomized controlled trial. Diabetes Care (2002) 25(1):89–94. doi: 10.2337/diacare.25.1.89
100. Gottschalk M, Danne T, Vlajnic A, Cara JF. Glimepiride versus metformin as monotherapy in pediatric patients with type 2 diabetes: A randomized, single-blind comparative study. Diabetes Care Apr (2007) 30(4):790–4. doi: 10.2337/dc06-1554
101. Matsuura N, Amemiya S, Sugihara S, Urakami T, Kikuchi N, Kato H, et al. Metformin monotherapy in children and adolescents with type 2 diabetes mellitus in Japan. Diabetol Int (2019) 10(1):51–7. doi: 10.1007/s13340-018-0361-3
102. Garber AJ, Duncan TG, Goodman AM, Mills DJ, Rohlf JL. Efficacy of metformin in type II diabetes: results of a double-blind, placebo-controlled, dose-response trial. Am J Med (1997) 103(6):491–7. doi: 10.1016/s0002-9343(97)00254-4
103. Dabelea D, Rewers A, Stafford JM, Standiford DA, Lawrence JM, Saydah S, et al. Trends in the prevalence of ketoacidosis at diabetes diagnosis: The SEARCH for diabetes in youth study. Pediatrics (2014) 133(4):e938–45. doi: 10.1542/peds.2013-2795
104. Diabetes Canada Clinical Practice Guidelines Expert Committee, Panagiotopoulos C, Hadjiyannakis S, Henderson M. Type 2 diabetes in children and adolescents. Can J Diabet (2018) 42 Suppl 1:S247–54. doi: 10.1016/j.jcjd.2017.10.037
105. Zeitler P, Arslanian S, Fu J, Pinhas-Hamiel O, Reinehr T, Tandon N, et al. ISPAD clinical practice consensus guidelines 2018: Type 2 diabetes mellitus in youth. Pediatr Diabet (2018) 19 Suppl 27:28–46. doi: 10.1111/pedi.12719
106. Today Study Group, Zeitler P, Epstein L, Grey M, Hirst K, Kaufman F, et al. Treatment options for type 2 diabetes in adolescents and youth: A study of the comparative efficacy of metformin alone or in combination with rosiglitazone or lifestyle intervention in adolescents with type 2 diabetes. Pediatr Diabet (2007) 8(2):74–87. doi: 10.1111/j.1399-5448.2007.00237.x
107. Laffel L, Chang N, Grey M, Hale D, Higgins L, Hirst K, et al. Metformin monotherapy in youth with recent onset type 2 diabetes: Experience from the prerandomization run-in phase of the TODAY study. Pediatr Diabet (2012) 13(5):369–75. doi: 10.1111/j.1399-5448.2011.00846.x
108. Zeitler P, Hirst K, Copeland KC, El Ghormli L, Levitt Katz L, Levitsky LL, et al. HbA1c after a short period of monotherapy with metformin identifies durable glycemic control among adolescents with type 2 diabetes. Diabetes Care (2015) 38(12):2285–92. doi: 10.2337/dc15-0848
109. Today Study Group. Effects of metformin, metformin plus rosiglitazone, and metformin plus lifestyle on insulin sensitivity and beta-cell function in TODAY. Diabetes Care (2013) 36(6):1749–57. doi: 10.2337/dc12-2393
110. Bacha F, Cheng P, Gal RL, Kollman C, Tamborlane WV, Klingensmith GJ, et al. Initial presentation of type 2 diabetes in adolescents predicts durability of successful treatment with metformin monotherapy: Insights from the pediatric diabetes consortium T2D registry. Horm Res Paediatr (2018) 89(1):47–55. doi: 10.1159/000481687
111. Rise Consortium. Impact of insulin and metformin versus metformin alone on beta-cell function in youth with impaired glucose tolerance or recently diagnosed type 2 diabetes. Diabetes Care (2018) 41(8):1717–25. doi: 10.2337/dc18-0787
112. Hannon TS, Edelstein SL, Arslanian SA, Caprio S, Zeitler PS, Buchanan TA, et al. Withdrawal of medications leads to worsening of OGTT parameters in youth with impaired glucose tolerance or recently-diagnosed type 2 diabetes. Pediatr Diabet (2020) 21(8):1437–46. doi: 10.1111/pedi.13129
113. Cioana M, Deng J, Nadarajah A, Hou M, Qiu Y, Chen SSJ, et al. The Prevalence of Obesity Among Children With Type 2 Diabetes: A Systematic Review and Meta-analysis. JAMA Netw Open (2022) 5(12):1–18. doi: 10.1001/jamanetworkopen.2022.47186
114. Atabek ME, Pirgon O. Use of metformin in obese adolescents with hyperinsulinemia: A 6-month, randomized, double-blind, placebo-controlled clinical trial. J Pediatr Endocrinol Metab (2008) 21(4):339–48. doi: 10.1515/jpem.2008.21.4.339
115. Clarson CL, Mahmud FH, Baker JE, Clark HE, McKay WM, Schauteet VD, et al. Metformin in combination with structured lifestyle intervention improved body mass index in obese adolescents, but did not improve insulin resistance. Endocrine (2009) 36(1):141–6. doi: 10.1007/s12020-009-9196-9
116. Freemark M, Bursey D. The effects of metformin on body mass index and glucose tolerance in obese adolescents with fasting hyperinsulinemia and a family history of type 2 diabetes. Pediatrics (2001) 107(4):E55. doi: 10.1542/peds.107.4.e55
117. Fu JF, Liang L, Zou CC, Hong F, Wang CL, Wang XM, et al. Prevalence of the metabolic syndrome in zhejiang Chinese obese children and adolescents and the effect of metformin combined with lifestyle intervention. Int J Obes (Lond) (2007) 31(1):15–22. doi: 10.1038/sj.ijo.0803453
118. Kay JP, Alemzadeh R, Langley G, D'Angelo L, Smith P, Holshouser S. Beneficial effects of metformin in normoglycemic morbidly obese adolescents. Metabolism (2001) 50(12):1457–61. doi: 10.1053/meta.2001.28078
119. Kendall D, Vail A, Amin R, Barrett T, Dimitri P, Ivison F, et al. Metformin in obese children and adolescents: The MOCA trial. J Clin Endocrinol Metab (2013) 98(1):322–9. doi: 10.1210/jc.2012-2710
120. Mauras N, DelGiorno C, Hossain J, Bird K, Killen K, Merinbaum D, et al. Metformin use in children with obesity and normal glucose tolerance–effects on cardiovascular markers and intrahepatic fat. J Pediatr Endocrinol Metab (2012) 25(1-2):33–40. doi: 10.1515/jpem-2011-0450
121. Srinivasan S, Ambler GR, Baur LA, Garnett SP, Tepsa M, Yap F, et al. Randomized, controlled trial of metformin for obesity and insulin resistance in children and adolescents: Improvement in body composition and fasting insulin. J Clin Endocrinol Metab (2006) 91(6):2074–80. doi: 10.1210/jc.2006-0241
122. Yanovski JA, Krakoff J, Salaita CG, McDuffie JR, Kozlosky M, Sebring NG, et al. Effects of metformin on body weight and body composition in obese insulin-resistant children: A randomized clinical trial. Diabetes (2011) 60(2):477–85. doi: 10.2337/db10-1185
123. Luong DQ, Oster R, Ashraf AP. Metformin treatment improves weight and dyslipidemia in children with metabolic syndrome. J Pediatr Endocrinol Metab (2015) 28(5-6):649–55. doi: 10.1515/jpem-2014-0201
124. Warnakulasuriya LS, Fernando MMA, Adikaram AVN, Thawfeek ARM, Anurasiri WL, Silva RR, et al. Metformin in the management of childhood obesity: A randomized control trial. Child Obes (2018) 14(8):553–65. doi: 10.1089/chi.2018.0043
125. Masarwa R, Brunetti VC, Aloe S, Henderson M, Platt RW, Filion KB. Efficacy and safety of metformin for obesity: A systematic review. Pediatrics (2021) 147(3):1–13. doi: 10.1542/peds.2020-1610
126. Sadeghi A, Mousavi SM, Mokhtari T, Parohan M, Milajerdi A. Metformin therapy reduces obesity indices in children and adolescents: A systematic review and meta-analysis of randomized clinical trials. Child Obes (2020) 16(3):174–91. doi: 10.1089/chi.2019.0040
127. Diaz M, Bassols J, Lopez-Bermejo A, de Zegher F, Ibanez L. Metformin treatment to reduce central adiposity after prenatal growth restraint: A placebo-controlled pilot study in prepubertal children. Pediatr Diabet (2015) 16(7):538–45. doi: 10.1111/pedi.12220
128. Lentferink YE, Knibbe CAJ, van der Vorst MMJ. Efficacy of metformin treatment with respect to weight reduction in children and adults with obesity: A systematic review. Drugs (2018) 78(18):1887–901. doi: 10.1007/s40265-018-1025-0
129. McDonagh MS, Selph S, Ozpinar A, Foley C. Systematic review of the benefits and risks of metformin in treating obesity in children aged 18 years and younger. JAMA Pediatr (2014) 168(2):178–84. doi: 10.1001/jamapediatrics.2013.4200
130. Brufani C, Crino A, Fintini D, Patera PI, Cappa M, Manco M. Systematic review of metformin use in obese nondiabetic children and adolescents. Horm Res Paediatr (2013) 80(2):78–85. doi: 10.1159/000353760
131. Bouza C, Lopez-Cuadrado T, Gutierrez-Torres LF, Amate J. Efficacy and safety of metformin for treatment of overweight and obesity in adolescents: An updated systematic review and meta-analysis. Obes Facts (2012) 5(5):753–65. doi: 10.1159/000345023
132. Wilson DM, Abrams SH, Aye T, Lee PD, Lenders C, Lustig RH, et al. Metformin extended release treatment of adolescent obesity: A 48-week randomized, double-blind, placebo-controlled trial with 48-week follow-up. Arch Pediatr Adolesc Med (2010) 164(2):116–23. doi: 10.1001/archpediatrics.2009.264
133. van der Aa MP, Elst MA, van de Garde EM, van Mil EG, Knibbe CA, van der Vorst MM. Long-term treatment with metformin in obese, insulin-resistant adolescents: Results of a randomized double-blinded placebo-controlled trial. Nutr Diabet (2016) 6(8):e228. doi: 10.1038/nutd.2016.37
134. Lentferink YE, van der Aa MP, van Mill E, Knibbe CAJ, van der Vorst MMJ. Long-term metformin treatment in adolescents with obesity and insulin resistance, results of an open label extension study. Nutr Diabet (2018) 8(1):47. doi: 10.1038/s41387-018-0057-6
135. Concepcion JQ, Tucker LY, Huang K. Metformin for pediatric obesity and insulin resistance: A retrospective study within an integrated health care system. Obes (Silver Spring) (2021) 29(9):1526–37. doi: 10.1002/oby.23207
136. Kyler KE, Kadakia RB, Palac HL, Kwon S, Ariza AJ, Binns HJ. Use of metformin for weight management in children and adolescents with obesity in the clinical setting. Clin Pediatr (Phila) (2018) 57(14):1677–85. doi: 10.1177/0009922818803404
137. Fauser BC, Tarlatzis BC, Rebar RW, Legro RS, Balen AH, Lobo R, et al. Consensus on women's health aspects of polycystic ovary syndrome (PCOS): the Amsterdam ESHRE/ASRM-sponsored 3rd PCOS consensus workshop group. Fertil Steril (2012) 97(1):28–38 e25. doi: 10.1016/j.fertnstert.2011.09.024
138. Ibáñez L, Oberfield SE, Witchel S, Auchus RJ, Chang RJ, Codner E, et al. An international consortium update: Pathophysiology, diagnosis, and treatment of polycystic ovarian syndrome in adolescence. Horm Res Paediatr (2017) 88(6):371–95. doi: 10.1159/000479371
139. Legro RS, Arslanian SA, Ehrmann DA, Hoeger KM, Murad MH, Pasquali R, et al. Diagnosis and treatment of polycystic ovary syndrome: An endocrine society clinical practice guideline. J Clin Endocrinol Metab (2013) 98(12):4565–92. doi: 10.1210/jc.2013-2350
140. Pena AS, Witchel SF, Hoeger KM, Oberfield SE, Vogiatzi MG, Misso M, et al. Adolescent polycystic ovary syndrome according to the international evidence-based guideline. BMC Med (2020) 18(1):72. doi: 10.1186/s12916-020-01516-x
141. Witchel SF, Oberfield S, Rosenfield RL, Codner E, Bonny A, Ibanez L, et al. The diagnosis of polycystic ovary syndrome during adolescence. Horm Res Paediatr (2015). doi: 10.1159/000375530
142. Naz MSG, Tehrani FR, Majd HA, Ahmadi F, Ozgoli G, Fakari FR, et al. The prevalence of polycystic ovary syndrome in adolescents: A systematic review and meta-analysis. Int J Reprod Biomed (2019) 17(8):533–42. doi: 10.18502/ijrm.v17i8.4818
143. Arslanian SA, Lewy VD, Danadian K. Glucose intolerance in obese adolescents with polycystic ovary syndrome: Roles of insulin resistance and beta-cell dysfunction and risk of cardiovascular disease. J Clin Endocrinol Metab (2001) 86(1):66–71. doi: 10.1210/jcem.86.1.7123
144. Cree-Green M, Rahat H, Newcomer BR, Bergman BC, Brown MS, Coe GV, et al. Insulin resistance, hyperinsulinemia, and mitochondria dysfunction in nonobese girls with polycystic ovarian syndrome. J Endocr Soc (2017) 1(7):931–44. doi: 10.1210/js.2017-00192
145. Lewy VD, Danadian K, Witchel SF, Arslanian S. Early metabolic abnormalities in adolescent girls with polycystic ovarian syndrome. J Pediatr (2001) 138(1):38–44. doi: 10.1067/mpd.2001.109603
146. Diamanti-Kandarakis E, Dunaif A. Insulin resistance and the polycystic ovary syndrome revisited: An update on mechanisms and implications. Endocr Rev (2012) 33(6):981–1030. doi: 10.1210/er.2011-1034
147. Rosenfield RL, Ehrmann DA. The pathogenesis of polycystic ovary syndrome (PCOS): The hypothesis of PCOS as functional ovarian hyperandrogenism revisited. Endocr Rev (2016) 37(5):467–520. doi: 10.1210/er.2015-1104
148. Soldani R, Cagnacci A, Yen SS. Insulin, insulin-like growth factor I (IGF-I) and IGF-II enhance basal and gonadotrophin-releasing hormone-stimulated luteinizing hormone release from rat anterior pituitary cells in vitro. Eur J Endocrinol (1994) 131(6):641–5. doi: 10.1530/eje.0.1310641
149. Dunaif A, Finegood DT. Beta-cell dysfunction independent of obesity and glucose intolerance in the polycystic ovary syndrome. J Clin Endocrinol Metab (1996) 81(3):942–7. doi: 10.1210/jcem.81.3.8772555
150. Dunaif A, Segal KR, Futterweit W, Dobrjansky A. Profound peripheral insulin resistance, independent of obesity, in polycystic ovary syndrome. Diabetes (1989) 38(9):1165–74. doi: 10.2337/diab.38.9.1165
151. Al-Zubeidi H, Klein KO. Randomized clinical trial evaluating metformin versus oral contraceptive pills in the treatment of adolescents with polycystic ovarian syndrome. J Pediatr Endocrinol Metab (2015) 28(7-8):853–8. doi: 10.1515/jpem-2014-0283
152. Allen HF, Mazzoni C, Heptulla RA, Murray MA, Miller N, Koenigs L, et al. Randomized controlled trial evaluating response to metformin versus standard therapy in the treatment of adolescents with polycystic ovary syndrome. J Pediatr Endocrinol Metab (2005) 18(8):761–8. doi: 10.1515/jpem.2005.18.8.761
153. El Maghraby HA, Nafee T, Guiziry D, Elnashar A. Randomized controlled trial of the effects of metformin versus combined oral contraceptives in adolescent PCOS women through a 24 month follow up period. Middle East Fertil Soc J (2015) 20(3):131–7. doi: 10.1016/j.mefs.2014.10.003
154. Bridger T, MacDonald S, Baltzer F, Rodd C. Randomized placebo-controlled trial of metformin for adolescents with polycystic ovary syndrome. Arch Pediatr Adolesc Med (2006) 160(3):241–6. doi: 10.1001/archpedi.160.3.241
155. Hoeger K, Davidson K, Kochman L, Cherry T, Kopin L, Guzick DS. The impact of metformin, oral contraceptives, and lifestyle modification on polycystic ovary syndrome in obese adolescent women in two randomized, placebo-controlled clinical trials. J Clin Endocrinol Metab (2008) 93(11):4299–306. doi: 10.1210/jc.2008-0461
156. Al Khalifah RA, Florez ID, Dennis B, Thabane L, Bassilious E. Metformin or oral contraceptives for adolescents with polycystic ovarian syndrome: A meta-analysis. Pediatrics (2016) 137(5). doi: 10.1542/peds.2015-4089
157. Fabbrini E, Sullivan S, Klein S. Obesity and nonalcoholic fatty liver disease: Biochemical, metabolic, and clinical implications. Hepatology (2010) 51(2):679–89. doi: 10.1002/hep.23280
158. Hwang JH, Stein DT, Barzilai N, Cui MH, Tonelli J, Kishore P, et al. Increased intrahepatic triglyceride is associated with peripheral insulin resistance: In vivo MR imaging and spectroscopy studies. Am J Physiol Endocrinol Metab (2007) 293(6):E1663–9. doi: 10.1152/ajpendo.00590.2006
159. Ipsen DH, Lykkesfeldt J, Tveden-Nyborg P. Molecular mechanisms of hepatic lipid accumulation in non-alcoholic fatty liver disease. Cell Mol Life Sci (2018) 75(18):3313–27. doi: 10.1007/s00018-018-2860-6
160. Koo SH. Nonalcoholic fatty liver disease: Molecular mechanisms for the hepatic steatosis. Clin Mol Hepatol (2013) 19(3):210–5. doi: 10.3350/cmh.2013.19.3.210
161. Mittendorfer B, Magkos F, Fabbrini E, Mohammed BS, Klein S. Relationship between body fat mass and free fatty acid kinetics in men and women. Obes (Silver Spring) (2009) 17(10):1872–7. doi: 10.1038/oby.2009.224
162. Nadeau KJ, Klingensmith G, Zeitler P. Type 2 diabetes in children is frequently associated with elevated alanine aminotransferase. J Pediatr Gastroenterol Nutr (2005) 41(1):94–8. doi: 10.1097/01.MPG.0000164698.03164.E5
163. Portillo-Sanchez P, Bril F, Maximos M, Lomonaco R, Biernacki D, Orsak B, et al. High prevalence of nonalcoholic fatty liver disease in patients with type 2 diabetes mellitus and normal plasma aminotransferase levels. J Clin Endocrinol Metab (2015) 100(6):2231–8. doi: 10.1210/jc.2015-1966
164. Utz-Melere M, Targa-Ferreira C, Lessa-Horta B, Epifanio M, Mouzaki M, Mattos AA. Non-alcoholic fatty liver disease in children and adolescents: Lifestyle change - a systematic review and meta-analysis. Ann Hepatol (2018) 17(3):345–54. doi: 10.5604/01.3001.0011.7380
165. Lavine JE, Schwimmer JB, Van Natta ML, Molleston JP, Murray KF, Rosenthal P, et al. Effect of vitamin e or metformin for treatment of nonalcoholic fatty liver disease in children and adolescents: the TONIC randomized controlled trial. JAMA (2011) 305(16):1659–68. doi: 10.1001/jama.2011.520
166. Nadeau KJ, Ehlers LB, Zeitler PS, Love-Osborne K. Treatment of non-alcoholic fatty liver disease with metformin versus lifestyle intervention in insulin-resistant adolescents. Pediatr Diabet (2009) 10(1):5–13. doi: 10.1111/j.1399-5448.2008.00450.x
167. Nobili V, Manco M, Ciampalini P, Alisi A, Devito R, Bugianesi E, et al. Metformin use in children with nonalcoholic fatty liver disease: An open-label, 24-month, observational pilot study. Clin Ther (2008) 30(6):1168–76. doi: 10.1016/j.clinthera.2008.06.012
168. Shiasi Arani K, Taghavi Ardakani A, Moazami Goudarzi R, Talari HR, Hami K, Akbari H, et al. Effect of vitamin e and metformin on fatty liver disease in obese children- randomized clinical trial. Iran J Public Health (2014) 43(10):1417–23.
169. Pastor-Villaescusa B, Canete MD, Caballero-Villarraso J, Hoyos R, Latorre M, Vazquez-Cobela R, et al. Metformin for obesity in prepubertal and pubertal children: A randomized controlled trial. Pediatrics (2017) 140(1). doi: 10.1542/peds.2016-4285
170. Bassols J, Martinez-Calcerrada JM, Osiniri I, Diaz-Roldan F, Xargay-Torrent S, Mas-Pares B, et al. Effects of metformin administration on endocrine-metabolic parameters, visceral adiposity and cardiovascular risk factors in children with obesity and risk markers for metabolic syndrome: A pilot study. PloS One (2019) 14(12):e0226303. doi: 10.1371/journal.pone.0226303
171. Aroda VR, Edelstein SL, Goldberg RB, Knowler WC, Marcovina SM, Orchard TJ, et al. Long-term metformin use and vitamin B12 deficiency in the diabetes prevention program outcomes study. J Clin Endocrinol Metab (2016) 101(4):1754–61. doi: 10.1210/jc.2015-3754
172. Niafar M, Hai F, Porhomayon J, Nader ND. The role of metformin on vitamin B12 deficiency: A meta-analysis review. Intern Emerg Med (2015) 10(1):93–102. doi: 10.1007/s11739-014-1157-5
173. Khattab R, Albannawi M, Alhajjmohammed D, Alkubaish Z, Althani R, Altheeb L, et al. Metformin-induced vitamin B12 deficiency among type 2 diabetes mellitus' patients: A systematic review. Curr Diabetes Rev (2022). doi: 10.2174/1573399818666220418080959
174. Infante M, Leoni M, Caprio M, Fabbri A. Long-term metformin therapy and vitamin B12 deficiency: An association to bear in mind. World J Diabet (2021) 12(7):916–31. doi: 10.4239/wjd.v12.i7.916
175. Bauman WA, Shaw S, Jayatilleke E, Spungen AM, Herbert V. Increased intake of calcium reverses vitamin B12 malabsorption induced by metformin. Diabetes Care (2000) 23(9):1227–31. doi: 10.2337/diacare.23.9.1227
176. Yu YM, So SKC, Khallouq BB. The effect of metformin on vitamin B12 levels in pediatric patients. Ann Pediatr Endocrinol Metab (2022) 27(3):223–8. doi: 10.6065/apem.2142210.105
177. Tas O, Kontbay T, Dogan O, Kose E, Berberoglu M, Siklar Z, et al. Does metformin treatment in pediatric population cause vitamin B12 deficiency? Klin Padiatr (2022) 234(4):221–7. doi: 10.1055/a-1702-2614. Verursacht die Metformin-Behandlung bei Kindern und Jugendlichen einen Vitamin-B12-Mangel?
178. DeFronzo RA, Goodman AM. Efficacy of metformin in patients with non-insulin-dependent diabetes mellitus. the multicenter metformin study group. N Engl J Med (1995) 333(9):541–9. doi: 10.1056/NEJM199508313330902
179. Crowley MJ, Diamantidis CJ, McDuffie JR, Cameron B, Stanifer J, Mock CK, et al. Metformin Use in Patients with Historical Contraindications or Precautions [Internet]. Washington (DC): Department of Veterans Affairs (US) (2016) .
180. FDA Drug Safety Communication. FDA Revises warnings regarding use of the diabetes medicine metformin in certain patients with reduced kidney function . Available at: https://www.fda.gov/drugs/drug-safety-and-availability/fda-drug-safety-communication-fda-revises-warnings-regarding-use-diabetes-medicine-metformin-certain (Accessed Sep. 4, 2022).
181. American Diabetes Association Professional Practice Committee. 2. Classification and diagnosis of diabetes: Standards of medical care in diabetes-2022. Diabetes Care (2022) 45(Suppl 1):S17–38. doi: 10.2337/dc22-S002
182. Han C, Song Q, Ren Y, Chen X, Jiang X, Hu D. Global prevalence of prediabetes in children and adolescents: A systematic review and meta-analysis. J Diabet (2022) 14(7):434–41. doi: 10.1111/1753-0407.13291
183. Galderisi A, Giannini C, Weiss R, Kim G, Shabanova V, Santoro N, et al. Trajectories of changes in glucose tolerance in a multiethnic cohort of obese youths: An observational prospective analysis. Lancet Child Adolesc Health (2018) 2(10):726–35. doi: 10.1016/S2352-4642(18)30235-9
184. Kleber M, deSousa G, Papcke S, Wabitsch M, Reinehr T. Impaired glucose tolerance in obese white children and adolescents: Three to five year follow-up in untreated patients. Exp Clin Endocrinol Diabet (2011) 119(3):172–6. doi: 10.1055/s-0030-1263150
185. Love-Osborne KA, Sheeder JL, Nadeau KJ, Zeitler P. Longitudinal follow up of dysglycemia in overweight and obese pediatric patients. Pediatr Diabet (2018) 19(2):199–204. doi: 10.1111/pedi.12570
186. Diabetes Prevention Program Research Group, Knowler WC, Fowler SE, Hamman RF, Christophi CA, Hoffman HJ, et al. 10-year follow-up of diabetes incidence and weight loss in the diabetes prevention program outcomes study. Lancet (2009) 374(9702):1677–86. doi: 10.1016/S0140-6736(09)61457-4
187. Diabetes Prevention Program Research Group. Long-term effects of lifestyle intervention or metformin on diabetes development and microvascular complications over 15-year follow-up: The diabetes prevention program outcomes study. Lancet Diabetes Endocrinol (2015) 3(11):866–75. doi: 10.1016/S2213-8587(15)00291-0
188. Ramachandran A, Snehalatha C, Mary S, Mukesh B, Bhaskar AD, Vijay V, et al. The Indian diabetes prevention programme shows that lifestyle modification and metformin prevent type 2 diabetes in Asian Indian subjects with impaired glucose tolerance (IDPP-1). Diabetologia (2006) 49(2):289–97. doi: 10.1007/s00125-005-0097-z
189. Love-Osborne K, Sheeder J, Zeitler P. Addition of metformin to a lifestyle modification program in adolescents with insulin resistance. J Pediatr (2008) 152(6):817–22. doi: 10.1016/j.jpeds.2008.01.018
190. Wiegand S, l'Allemand D, Hubel H, Krude H, Burmann M, Martus P, et al. Metformin and placebo therapy both improve weight management and fasting insulin in obese insulin-resistant adolescents: a prospective, placebo-controlled, randomized study. Eur J Endocrinol (2010) 163(4):585–92. doi: 10.1530/EJE-10-0570
191. Sun J, Wang Y, Zhang X, He H. The effects of metformin on insulin resistance in overweight or obese children and adolescents: A PRISMA-compliant systematic review and meta-analysis of randomized controlled trials. Med (Baltimore) (2019) 98(4):e14249. doi: 10.1097/MD.0000000000014249
192. Gomez-Diaz RA, Talavera JO, Pool EC, Ortiz-Navarrete FV, Solorzano-Santos F, Mondragon-Gonzalez R, et al. Metformin decreases plasma resistin concentrations in pediatric patients with impaired glucose tolerance: A placebo-controlled randomized clinical trial. Metabolism (2012) 61(9):1247–55. doi: 10.1016/j.metabol.2012.02.003
Keywords: metformin, type 2 diabetes, youth, management, pediatric type 2 diabetes
Citation: Alfaraidi H and Samaan MC (2023) Metformin therapy in pediatric type 2 diabetes mellitus and its comorbidities: A review. Front. Endocrinol. 13:1072879. doi: 10.3389/fendo.2022.1072879
Received: 18 October 2022; Accepted: 12 December 2022;
Published: 06 February 2023.
Edited by:
Chengchen Zhang, University of Maryland, Baltimore, United StatesReviewed by:
Barbara Glowinska-Olszewska, Medical University of Bialystok, PolandXiumin Wang, Shanghai Children’s Medical Center, China
Copyright © 2023 Alfaraidi and Samaan. This is an open-access article distributed under the terms of the Creative Commons Attribution License (CC BY). The use, distribution or reproduction in other forums is permitted, provided the original author(s) and the copyright owner(s) are credited and that the original publication in this journal is cited, in accordance with accepted academic practice. No use, distribution or reproduction is permitted which does not comply with these terms.
*Correspondence: M. Constantine Samaan, samaanc@mcmaster.ca