- Division of Endocrinology, Department of Pediatrics, Zucker School of Medicine at Hofstra/Northwell, New Hyde Park, NY, United States
Despite advances in the characterization of partial clinical remission (PR) of type 1 diabetes, an accurate definition of PR remains problematic. Two recent studies in children with new-onset T1D demonstrated serious limitations of the present gold standard definition of PR, a stimulated C-peptide (SCP) concentration of >300 pmol/L. The first study employed the concept of insulin sensitivity score (ISS) to show that 55% of subjects with new-onset T1D and a detectable SCP level of >300 pmol/L had low insulin sensitivity (IS) and thus might not be in remission when assessed by insulin-dose adjusted A1c (IDAA1c), an acceptable clinical marker of PR. The second study, a randomized controlled trial of vitamin D (ergocalciferol) administration in children and adolescents with new-onset T1D, demonstrated no significant difference in SCP between the ergocalciferol and placebo groups, but showed a significant blunting of the temporal trend in both A1c and IDAA1c in the ergocalciferol group. These two recent studies indicate the poor specificity and sensitivity of SCP to adequately characterize PR and thus call for a re-examination of current approaches to the definition of PR. They demonstrate the limited sensitivity of SCP, a static biochemical test, to detect the complex physiological changes that occur during PR such as changes in insulin sensitivity, insulin requirements, body weight, and physical activity. These shortcomings call for a broader definition of PR using a combination of functional markers such as IDAA1c and ISS to provide a valid assessment of PR that reaches beyond the static changes in SCP alone.
LITERATURE SEARCH CRITERIA
A literature search was conducted to identify publications addressing the definitions of partial clinical remission (PR) in children and adults. Medline, EMBASE, and Ovid were searched using the following search terms: clinical remission, partial remission, partial clinical remission, honeymoon phase, C-peptide, type 1 diabetes, children, pediatric type 1 diabetes, and paediatric type 1 diabetes.
Introduction
The definition of partial clinical remission (PR) remains problematic (1). Type 1 diabetes (T1D) is associated with reduced insulin sensitivity (IS) (2), but the adoption of the measurement of serum C-peptide (SCP) concentration as a primary endpoint in clinical studies on PR (3–7) does not accurately capture this reduced IS state (8). Furthermore, SCP has several physiological, biochemical, and pharmacokinetic limitations that affect its specificity and sensitivity (9, 10). Despite these shortcomings, the American Diabetes Association recommends SCP as the gold standard test for the assessment of PR in clinical trials (11). In contrast, the International Society for Pediatric and Adolescent Diabetes (ISPAD) recommends the insulin-dose adjusted A1c (IDAA1c) as its gold standard test for PR (12, 13). However, both SCP and IDAA1c do not directly assess insulin resistance or sensitivity (1) which limits the ability of each formula to fully characterize the heterogeneity of T1D and its multiple endotypes (14).
Diabetes mellitus affects 34.2 million, or 10.5% of the US population (15). T1D is a syndrome of persistent hyperglycemia secondary to insulinopenia resulting from autoimmune destruction of pancreatic β-cells (16). At the time of diagnosis of T1D, approximately 50% of residual β-cell function (RBCF) may remain, and this RBCF may persist for months or years (17–19). Prolonging the partial clinical remission (PR), also known as the ‘honeymoon’ phase of T1D improves glycemic control and reduces long-term complications (1, 20). Interventions to prevent immune-mediated destruction of β-cells with immunosuppressive and immunomodulatory agents have yielded promising trends, but insufficient protection (3–6). The primary outcome in these interventions is the SCP level (21). However, T1D is a heterogeneous disease with multiple endotypes (14), and recent data on the shortcomings of SCP as a primary endpoint in clinical studies on PR suggest that the negative results from some prior trials may be due to the limitations of SCP as a marker of PR, and not necessarily on the shortcomings of the study designs. Recent research suggests that functional parameters could provide a more robust characterization of PR(1, 13) (12) in a manner similar to the superiority of the measurement of plasma renin activity, a dynamic test, to plasma renin, a static test, in the assessment of the renin-angiotensin system (22).
Newer Discoveries in the Field: A Role for the Synergistic Impact of Insulin-Sensitivity Scores (ISS) and Insulin-Dose Adjusted A1c
Despite its approval by the American Diabetes Association (11), there is accumulating evidence in the literature that the current gold standard definition of PR, the SCP, is imprecise and underestimates PR, and in most cases fails to detect PR when present (8, 19, 23). Two recent studies suggest that the use of a combination of IDAA1c and ISS to confirm PR is more precise and specific (8, 23). Clinically, PR is defined by IDAA1c, a two-dimensional marker of PR that correlates insulin dose and measured HbA1c to residual β-cell function (24). IDAA1c has good agreement with stimulated C-peptide level of >300 pmol/L (25) which is currently considered the gold standard definition of PR, but IDAA1c has more utilitarian value as it also reflects changes in insulin requirements, body weight, and conditions that impact insulin requirements such as exercise. The IDAA1c is calculated by:
where PR is defined as IDAA1c of ≤9 (24).
In a recent pivotal longitudinal study, Mork et al (8) used the formula for insulin sensitivity score (ISS) developed by Dabelea et al (26) to determine the differences in ISS among youth in PR as defined by stimulated C-peptide level of >300 pmol/L (>2 ng/mL). The formula is:
[to convert triglycerides (TG) values from mmol/L to mg/dL, divide by 0.0113)] (26). Using the formula to generate ISS for each subject, Mork reported that 55% of their participants with detectable SCP of >300 pmol/L had low ISS and thus might not be in PR when defined by a functional marker of PR, the IDAA1c, suggesting that the presence of detectable SCP of >300 pmol/L (>2 ng/mL) does not equate to PR. Therefore, isolated use of SCP could have overestimated the number of subjects in PR in their cohort.
The discordance between SCP and PR was recently characterized by Nwosu et al (23) who randomized 36 subjects of 10-21 years with newly-diagnosed T1D in a 12-month randomized controlled trial (RCT) of ergocalciferol versus placebo to determine the impact of vitamin D on residual β-cell function (RBCF) and PR in youth with newly-diagnosed T1D (Figure 1). The trial’s hypothesis was that adjunctive ergocalciferol would increase RBCF and prolong PR. The primary aim was to determine the effect of ergocalciferol on RBCF and PR in children and adolescents with T1D, while the primary outcome was the longitudinal change in RBCF as marked by SCP and IDAA1c.
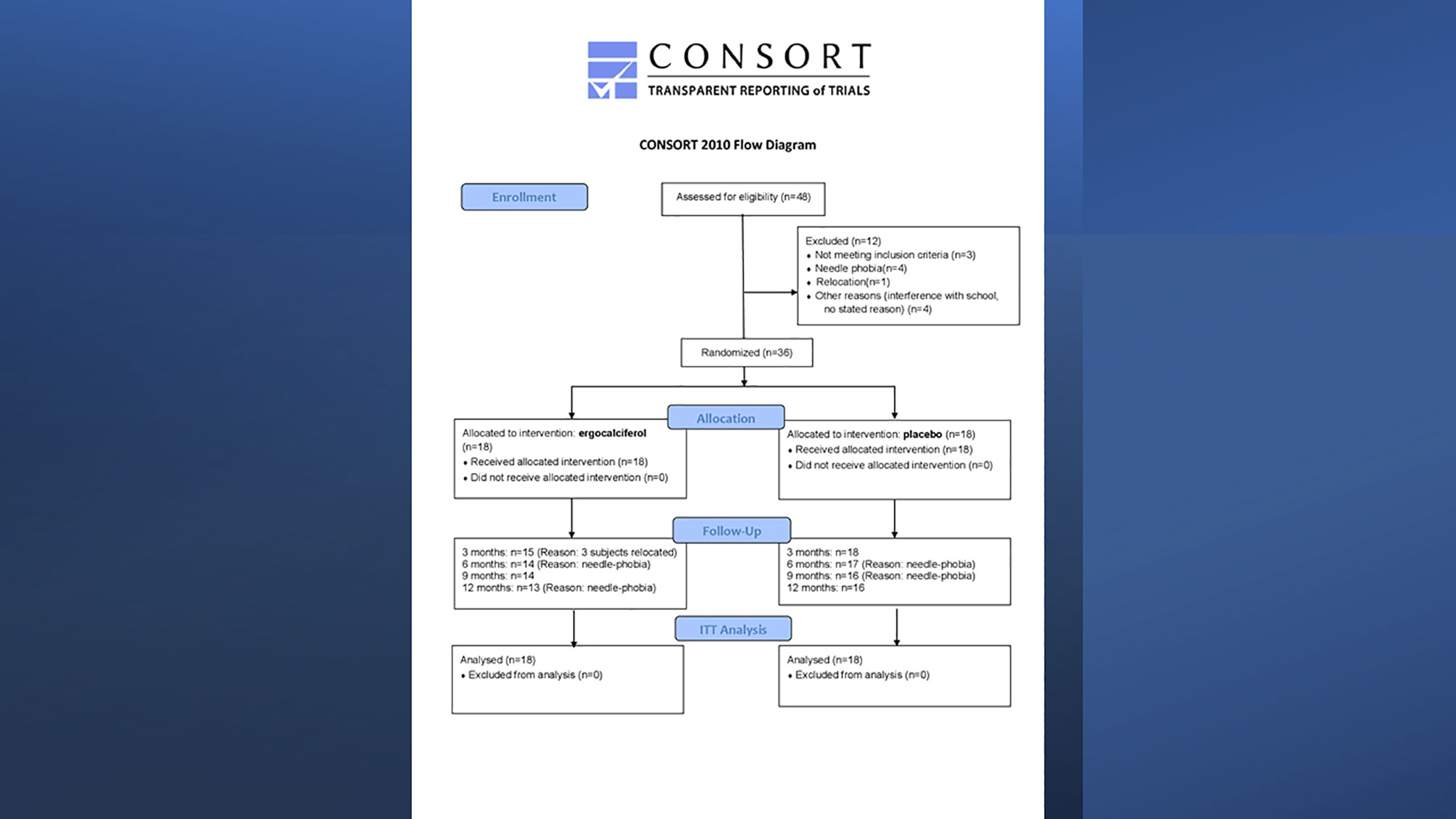
Figure 1 Consort Flow Diagram of randomized controlled trial of ergocalciferol in children and adolescents with newly diagnosed type 1 diabetes.
There were no differences in basal and SCP between the groups for the duration of the trial (Figures 2A, B). In contrast, there was a significant blunting of the temporal rise in both IDAA1c and A1c in the ergocalciferol group compared to the placebo group (Figures 2C, D). Specifically, the placebo group showed a faster rate of increase in HbA1c at a mean rate of change of 0.46% every 3 months, compared to the slower rate of change in the ergocalciferol group, mean rate of change of 0.14% every 3 months, (p=0.044). There was equally a faster rate of rise in IDAA1c in the placebo group at a mean rate of change of 0.77 every 3 months, whereas the rate of rise in the ergocalciferol group was significantly blunted, at a mean rate of change of 0.30 every 3 months (p=0.015).
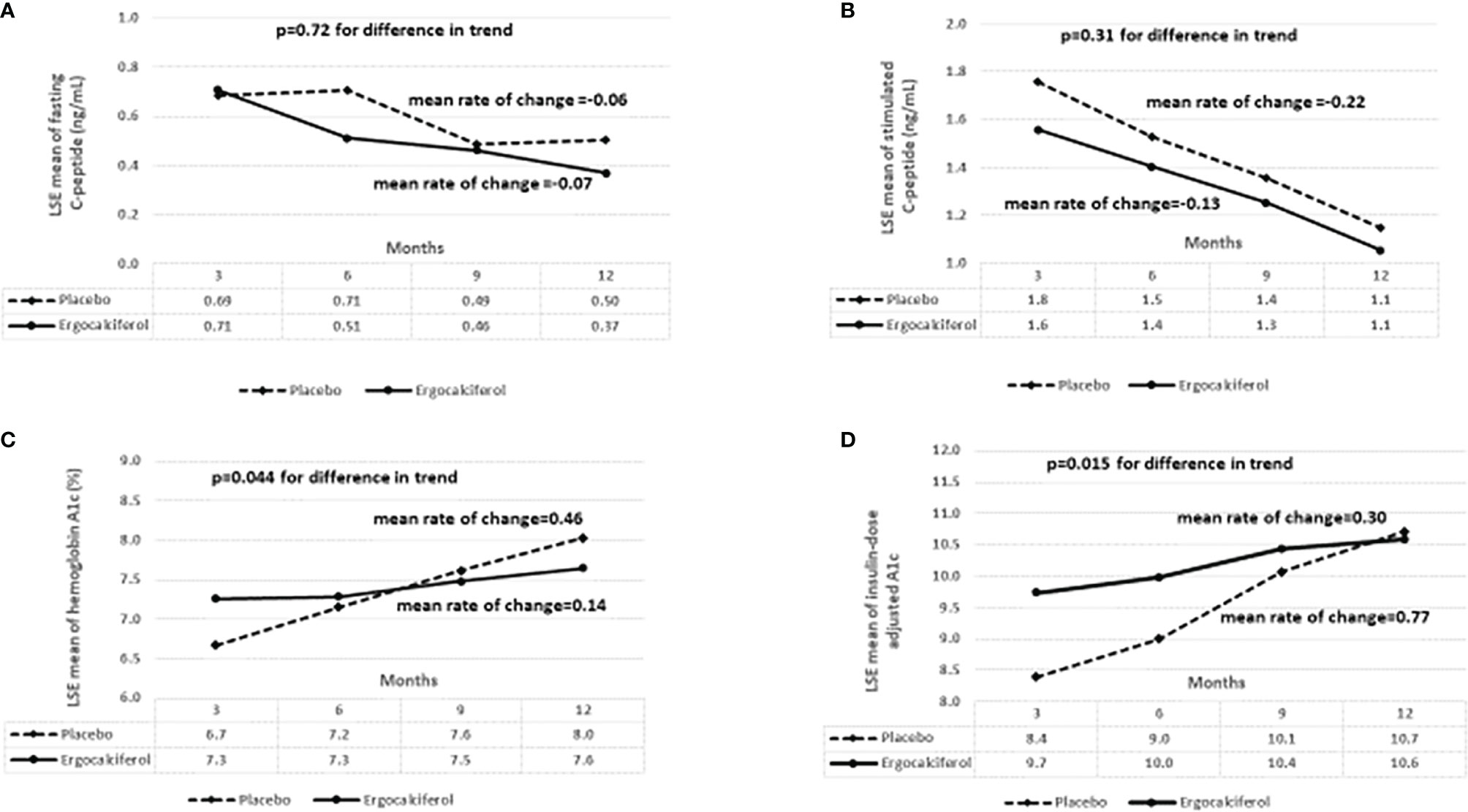
Figure 2 (A) shows the trend analysis of the least square estimates (LSE) of the means for fasting C-peptide. There was no significant difference in fasting C-peptide concentration between the ergocalciferol and placebo groups during the 12-month trial, (p=0.72). (B) There was no significant difference in the change in stimulated C-peptide concentration at 90 minutes between the ergocalciferol- and placebo-treated patients with type 1 diabetes during the 12-month trial, (p=0.31). (C) Temporal trend analysis showed a faster rate of rise in hemoglobin A1c (HbA1c) values in the placebo group compared to the vitamin group (p=0.044). (D) Temporal trend analysis showed a significantly faster rise in insulin dose adjusted A1c (IDAA1c) in the placebo group compared to the ergocalciferol group (p=0.015). This suggests beta-cell protection by ergocalciferol.
In contrast to the study by Mork et al, a 2016 observational study of 407 patients of 3-45 years by Hao et al (7) recommended that investigators use SCP as the primary endpoint in PR studies because IDAA1c significantly underestimated the number of subjects with SCP of ≥0.2 nmol/L, especially in children. This provided support for the 2004 decision by the ADA to adopt SCP as the gold-standard marker for assessing PR in clinical studies (11). However, in a landmark 2019 study of 1019 individuals with T1D for more than 50 years, who were prospectively followed for 4 years, Yu et al (19) reported that only 32% of the subjects had documented measurable SCP after using a combination of MMTT and hyperglycemic clamp with arginine infusion to estimate SCP; even though all 68 studied pancreases of their cohort were positive for insulin-containing β-cells. This discordance in premortem SCP and postmortem pancreatic histology, despite rigorous evaluation of pancreatic C-peptide secretory ability, led the authors to conclude that residual β-cells were present in individuals with T1D regardless of disease duration or measurable SCP levels. Interestingly, the average A1c for this cohort was 7.1%, suggesting that if the investigators had calculated the participants’ IDAA1c values, the mean value would have been mostly ≤9, suggesting that IDAA1c is more sensitive than SCP for PR detection. This is in line with the decision by ISPAD to designate IDAA1c as the gold-standard marker for PR (12).
A recent review article by Oram et al (27) reported a wide gap in the range of SCP positivity of 11% to 80% despite the fact that these studies used C-peptide assays of improved sensitivity and specificity in heterogenous groups of patients with long-standing T1D (18, 28–31). The detected C-peptide levels from these studies were mostly at very low concentrations. For example, the study by Wang et al (29), which used an ultrasensitive C-peptide assay, only detected SCP in 10% of the subjects, while the T1D Exchange study by Davis et al (30) detected only 29% SCP positivity using both basal and stimulated C-peptide measurements. The authors suggested that variations in SCP in these studies most likely resulted from cohort selection criteria, cohort ages and duration of T1D, differing assay sensitivities, and sample storage conditions. This wide discrepancy in SCP detection in various studies, and the range of factors that undergird its detectable concentration, limit its usefulness as a primary marker of PR as detailed below.
Limitations of Serum C-Peptide as a Primary Marker of Partial Clinical Remission of Type 1 Diabetes
The gold standard definition of PR is the detection of a stimulated C-peptide level (SCP) of >300 pmol/L following a mixed meal tolerance test (23, 32). Recent publications indicate that this one-dimensional definition may be insufficient to adequately address the components of a complex, multifaceted process such as PR[1, 13 (14)]. SCP is a biochemical marker with several limitations that diminish its use as a gold standard criterion for PR (27). Some of these limitations are physiological, anatomical, biochemical, pharmacokinetic, and glycemic related as detailed below.
Physiological Limitations
The major detriment to the use of SCP concentration to confirm PR is that its peak level depends on several physiological processes which may be deranged in patients with T1D. The are 2 primary provocative tests for SCP: the less popular glucagon stimulation test (GST) directly stimulates the β-cells to release C-peptide (33) while the more popular test, the mixed meal tolerance test (MMTT) stimulates insulin secretion from β-cells following the ingestion of a meal consisting of proteins, fats, and carbohydrates (34). A recent study (32) in healthy subjects without diabetes mellitus that compared GST and MMTT found that while GST directly stimulates the β-cells to release C-peptide, MMTT relies on an intact incretin axis that consists of operative gastric inhibitory peptide (GIP) and glucagon-like peptide-1 (GLP-1) systems. However, the responsiveness of the GIP/GLP-1 system is reduced in patients with recent onset T1D (9). Thus, the results of MMTT depends on the robustness and the combined action of the GIP and GLP-1 (34), and the type of meal used for the study (35). The reduced responsiveness of the GIP/GLP-1 systems in patients with recently diagnosed T1D suggests that the use of SCP as the primary outcome in these patients may underestimate their residual β-cell function (23). However, no study has investigated the impact of an altered incretin axis on β-cell response during MMTT (32) to validate the results obtained in trials relying on MMTT for SCP, as well as the adjustments needed for peak SCP concentrations in situations of suspected poor incretin response. This will prevent false negative results, and false negative conclusions in clinical trials.
The physiological significance of different concentrations of peak SCP following MMTT were recently examined by Rickels et al (36) who studied 63 adult patients who were stratified into 4 groups by their peak SCP concentration from MMTT. Using both hyperinsulinemic euglycemic clamp technique and hyperinsulinemic hypoglycemic clamping, the investigators showed that the group with the highest peak C-peptide concentration of >0.4 pmol/mL demonstrated robust β-cell responsiveness to hyperglycemia, as well as a robust α-cell responsiveness to hypoglycemia. This physiological adaption was absent in the groups with either negative or low peak C-peptide concentration. This α and β cell responsiveness to high peak C-peptide levels in patients with T1D may explain the significantly better glycemic control in remitters versus nonremitters. Thus, the transition from preclinical to clinical T1D is marked by progressive loss of β-cells with attendant inability of β-cells to suppress glucagon-mediated hyperglycemia. This study showed that residual C-peptide concentration of >0.4 pmol/mL following MMTT is a threshold of physiologic importance for both α-cell responsiveness to hypoglycemia and β-cell responsiveness to hyperglycemia. However, this responsiveness to hyperglycemia is incomplete as no amount of residual C-peptide can fully suppress glucagon once a diagnosis of T1D is made. Furthermore, sub-threshold residual C-peptide concentrations appear to be physiologically inactive.
Anatomical Limitations
T1D is a heterogenous disease with multiple endotypes (14). Recent reports of proinsulin production and secretion in most patients with long-standing T1D, including those without measurable C-peptide, suggest the presence of anatomic defect at the tissue level (27). This defect is associated with markedly reduced insulin production, abnormal hormone processing, with attendant proinsulin accumulation in the pancreas and general circulation (37, 38). This phenomenon suggests the existence of β-cells that can start the process of hormone production but are incapable of releasing mature insulin and C-peptide (27). Interestingly, proinsulin to C-peptide ratio, a marker of β-cells endoplasmic reticulum stress, is increased in children and adolescents with new-onset T1D (39). Thus, this could represent a significant limitation to the use of serum C-peptide to characterize PR in children. The existence of this subpopulation of β-cells is supported by recent reports that T1D is a heterogenous disease (14) marked by histopathological heterogeneity (40). Thus, a simple static test such as SCP may not adequately reflect the anatomic heterogeneity of the surviving β-cells.
Biochemical Limitations
The biochemical limitation of SCP assay were reported in a study (10) that examined the stability of C-peptide among 6 different assays. This study concluded that C-peptide stability varies significantly with different assay methods, and the stability for all assays is < 1 month, even when LC/MS/MS is used (41). It further reported that C-peptide is more stable in plasma than in serum, and that adding aprotinin to serum or plasma does not significantly improve the stability of C-peptide for any of the methods. Additionally, serum C-peptide degrades easily on storage (42) such that batched samples could easily give spuriously low readings leading to false negative results of SCP assay, which otherwise could be easily verified as inaccurate by the inclusion of the functional markers, IDAA1c and ISS.
Oram et al (27) reported that even under standardized protocols for pre-analytic handling of samples, the results of SCP could show wide inter-study variability. For example, whereas Mork (8), Rickels (36), and Mortensen (25) showed adequate C-peptide response in their observational studies, the RCT by Nwosu et al (23) did not detect any significant difference in SCP between the placebo and the experimental groups despite the detection of significantly lower temporal trends in the rise in both IDAA1c and A1c in the experimental group. Additionally, Yu et al (19) in their landmark study found no correlation in SCP detection by MMTT and hyperglycemic clamp with arginine infusion. In that study, the lack of correlation between premortem SCP levels and postmortem pancreatic morphological examination in the same patients in their cohort was so discrepant that the investigators concluded that regardless of measurable C-peptide levels or disease duration, residual β-cells were present in individuals with T1D.
Pharmacokinetic Properties
Earlier studies had highlighted the controversies surrounding the use of C-peptide to quantify insulin secretion because the kinetics of C-peptide under different conditions are not clearly defined (43). C-peptide is co-secreted with insulin and its serum concentration is dependent on the rate of production, volume of distribution, and renal clearance (44, 45). Therefore, factors that impair its production, volume of distribution, and clearance could easily lead to inaccurate results.
Glycemic and Related Limitations
Finally, SCP on its own is unable to detect changes in insulin resistance (IR), insulin sensitivity (IS) (8), insulin requirements, or changes in body weight. This contrasts with the functional markers, IDAA1c and ISS whose values change with conditions that alter insulin sensitivity and body weight. Though IDAA1c does not directly measure IS, the formular for ISS assesses IS directly.
Implications for Studies in the Field of Partial Clinical Remission
There are several implications for studies in the field. The first is the recognition that T1D is a heterogenous, complex disease with several endotypes, and over-reliance on SCP, a marker with numerous limitations, as the gold-standard definition of PR that could easily lead to negative study results in situations where functional definitions of PR could easily show a positive signal or result. Isolated use of SCP could easily under-estimate the proportion of subjects undergoing PR, a scenario that would be easily clarified using a combination of functional markers. The over-reliance on SCP fails to address the differences in IS status that is intrinsic to T1D and thus could lead to an over-estimation of PR in a population. In light of conclusions from recent studies (1, 13) (14), the overreliance on SCP as a study endpoint may be too simplistic and may not provide the full picture of the changes in insulin requirements, IS, and body weight, which are captured by the functional markers. SCP could vary by assay technique and thus may be subject to error. Serum C-peptide degrades easily and thus needs to be run within one week of collection. Therefore, batched study samples could easily lead to spuriously low results and under-estimation of PR.
Conclusions and Recommendations
This review recognizes that T1D is a heterogenous disease with multiple endotypes and recommends that studies on PR should use a combination of IDAA1c and ISS to first establish PR, and the changes in IS during PR to provide a comprehensive picture of the pathophysiology of PR. Using this approach, SCP will serve as a secondary marker of PR. This paradigm will enable future clinical trials on PR to employ multi-faceted approaches to the definition of PR and not rely solely on SCP. Therefore, clinical trials will focus more on the impact of interventions on functional parameters such as IDAA1c and IS, and secondarily on SCP. This will help obviate the imprecise results of clinical trials arising from the shortcomings of SCP.
Future Directions
A change in the characterization of PR with emphasis on functional markers as gold standard tests might reveal that some of the earlier studies that showed no significant difference in SCP between the treatment and placebo arms might demonstrate positive results when assessed using functional markers. For example, some of the studies examining the impact of immunomodulatory agents on PR could use a combination of IDAA1c and ISS as their endpoints. This change in the definition of PR will improve sensitivity and specificity and accelerate the pace of research in the field of partial clinical remission and its clinical applications. This will move the field forward.
Author Contributions
The author confirms being the sole contributor of this work and has approved it for publication.
Funding
This study was funded in part by an investigator-initiated research grant, Grant ID: 1 R21 DK113353-03, to BN from NIDDK, NIH.
Conflict of Interest
The author declares that the research was conducted in the absence of any commercial or financial relationships that could be construed as a potential conflict of interest.
Publisher’s Note
All claims expressed in this article are solely those of the authors and do not necessarily represent those of their affiliated organizations, or those of the publisher, the editors and the reviewers. Any product that may be evaluated in this article, or claim that may be made by its manufacturer, is not guaranteed or endorsed by the publisher.
References
1. Nwosu BU. Partial Clinical Remission of Type 1 Diabetes Mellitus in Children: Clinical Applications and Challenges With Its Definitions. Eur Med J Diabetes (2019) 4:89–98.
2. Nadeau KJ, Regensteiner JG, Bauer TA, Brown MS, Dorosz JL, Hull A, et al. Insulin Resistance in Adolescents With Type 1 Diabetes and Its Relationship to Cardiovascular Function. J Clin Endocrinol Metab (2010) 95:513–21. doi: 10.1210/jc.2009-1756
3. Sherry N, Hagopian W, Ludvigsson J, Jain SM, Wahlen J, Ferry RJ Jr., et al. Teplizumab for Treatment of Type 1 Diabetes (Protege Study): 1-Year Results From a Randomised, Placebo-Controlled Trial. Lancet (2011) 378:487–97. doi: 10.1016/S0140-6736(11)60931-8
4. Rapini N, Schiaffini R, Fierabracci A. Immunotherapy Strategies for the Prevention and Treatment of Distinct Stages of Type 1 Diabetes: An Overview. Int J Mol Sci (2020) 21. doi: 10.3390/ijms21062103
5. Rachid O, Osman A, Abdi R, Haik Y. CTLA4-Ig (Abatacept): A Promising Investigational Drug for Use in Type 1 Diabetes. Expert Opin Investig Drugs (2020) 29:221–36. doi: 10.1080/13543784.2020.1727885
6. Ouyang H, Wen J, Song K. Decreased Interleukin-35 Levels and CD4(+)EBI3(+) T Cells in Patients With Type 1 Diabetes and the Effects of the Antibody Against CD20 (Rituximab). Arch Med Sci: AMS (2021) 17:258–61. doi: 10.5114/aoms.2020.101510
7. Hao W, Gitelman S, DiMeglio LA, Boulware D, Greenbaum CJ, G. Type 1 Diabetes TrialNet Study. Fall in C-Peptide During First 4 Years From Diagnosis of Type 1 Diabetes: Variable Relation to Age, HbA1c, and Insulin Dose. Diabetes Care (2016) 39:1664–70. doi: 10.2337/dc16-0360
8. Mork FCB, Madsen JOB, Jensen AK, Hall GV, Pilgaard KA, Pociot F, et al. Differences in Insulin Sensitivity in the Partial Remission Phase of Childhood Type 1 Diabetes; a Longitudinal Cohort Study. Diabet. Med (2021), e14702.
9. Kaas A, Andersen ML, Fredheim S, Hougaard P, Buschard K, Petersen JS, et al. Proinsulin, GLP-1, and Glucagon Are Associated With Partial Remission in Children and Adolescents With Newly Diagnosed Type 1 Diabetes. Pediatr Diabetes (2012) 13:51–8. doi: 10.1111/j.1399-5448.2011.00812.x
10. Wiedmeyer HM. The Stability of C-Peptide in Storage, 64th Scientific Sessions (2004) of the American Diabetes Association. (2004).
11. Palmer JP, Fleming GA, Greenbaum CJ, Herold KC, Jansa LD, Kolb H, et al. C-Peptide Is the Appropriate Outcome Measure for Type 1 Diabetes Clinical Trials to Preserve Beta-Cell Function: Report of an ADA Workshop, 21-22 October 2001. Diabetes (2004) 53:250–64. doi: 10.2337/diabetes.53.1.250
12. Couper JJ, Haller MJ, Greenbaum CJ, Ziegler AG, Wherrett DK, Knip M, et al. ISPAD Clinical Practice Consensus Guidelines 2018: Stages of Type 1 Diabetes in Children and Adolescents. Pediatr Diabetes (2018) 19(Suppl 27):20–7. doi: 10.1111/pedi.12734
13. Fonolleda M, Murillo M, Vazquez F, Bel J, Vives-Pi M. Remission Phase in Paediatric Type 1 Diabetes: New Understanding and Emerging Biomarkers. Horm. Res Paediatr (2017) 88:307–15. doi: 10.1159/000479030
14. Battaglia M, Ahmed S, Anderson MS, Atkinson MA, Becker D, Bingley PJ, et al. Introducing the Endotype Concept to Address the Challenge of Disease Heterogeneity in Type 1 Diabetes. Diabetes Care (2020) 43:5–12. doi: 10.2337/dc19-0880
15. A. American Diabetes. Cardiovascular Disease and Risk Management: Standards of Medical Care in Diabetes-2020. Diabetes Care (2020) 43:S111–34.
17. Sherry NA, Tsai EB, Herold KC. Natural History of Beta-Cell Function in Type 1 Diabetes. Diabetes (2005) 54 Suppl 2:S32–9. doi: 10.2337/diabetes.54.suppl_2.s32
18. Oram RA, Jones AG, Besser RE, Knight BA, Shields BM, Brown RJ, et al. The Majority of Patients With Long-Duration Type 1 Diabetes Are Insulin Microsecretors and Have Functioning Beta Cells. Diabetologia (2014) 57:187–91. doi: 10.1007/s00125-013-3067-x
19. Yu MG, Keenan HA, Shah HS, Frodsham SG, Pober D, He Z, et al. Residual Beta Cell Function and Monogenic Variants in Long-Duration Type 1 Diabetes Patients. J Clin Invest (2019) 129:3252–63. doi: 10.1172/JCI127397
20. Steffes MW, Sibley S, Jackson M, Thomas W. Beta-Cell Function and the Development of Diabetes-Related Complications in the Diabetes Control and Complications Trial. Diabetes Care (2003) 26:832–6. doi: 10.2337/diacare.26.3.832
21. Robertson RP. Estimation of Beta-Cell Mass by Metabolic Tests: Necessary, But How Sufficient? Diabetes (2007) 56:2420–4. doi: 10.2337/db07-0742
23. Nwosu BU, Parajuli S, Jasmin G, Fleshman J, Sharma RB, Alonso LC, et al. Ergocalciferol in New-Onset Type 1 Diabetes: A Randomized Controlled Trial. J Endocr Soc (2022) 6:bvab179. doi: 10.1210/jendso/bvab179
24. Max Andersen ML, Hougaard P, Porksen S, Nielsen LB, Fredheim S, Svensson J, et al. Partial Remission Definition: Validation Based on the Insulin Dose-Adjusted HbA1c (IDAA1C) in 129 Danish Children With New-Onset Type 1 Diabetes. Pediatr Diabetes (2014) 15:469–76. doi: 10.1111/pedi.12208
25. Mortensen HB, Hougaard P, Swift P, Hansen L, Holl RW, Hoey H, et al. New Definition for the Partial Remission Period in Children and Adolescents With Type 1 Diabetes. Diabetes Care (2009) 32:1384–90. doi: 10.2337/dc08-1987
26. Dabelea D, D’Agostino RB Jr., Mason CC, West N, Hamman RF, Mayer-Davis EJ, et al. Development, Validation and Use of an Insulin Sensitivity Score in Youths With Diabetes: The SEARCH for Diabetes in Youth Study. Diabetologia (2011) 54:78–86. doi: 10.1007/s00125-010-1911-9
27. Oram RA, Sims EK, Evans-Molina C. Beta Cells in Type 1 Diabetes: Mass and Function; Sleeping or Dead? Diabetologia (2019) 62:567–77. doi: 10.1007/s00125-019-4822-4
28. Oram RA, McDonald TJ, Shields BM, Hudson MM, Shepherd MH, Hammersley S, et al. Most People With Long-Duration Type 1 Diabetes in a Large Population-Based Study Are Insulin Microsecretors. Diabetes Care (2015) 38:323–8. doi: 10.2337/dc14-0871
29. Wang L, Lovejoy NF, Faustman DL. Persistence of Prolonged C-Peptide Production in Type 1 Diabetes as Measured With an Ultrasensitive C-Peptide Assay. Diabetes Care (2012) 35:465–70. doi: 10.2337/dc11-1236
30. Davis AK, DuBose SN, Haller MJ, Miller KM, DiMeglio LA, Bethin KE, et al. Prevalence of Detectable C-Peptide According to Age at Diagnosis and Duration of Type 1 Diabetes. Diabetes Care (2015) 38:476–81. doi: 10.2337/dc14-1952
31. McGee P, Steffes M, Nowicki M, Bayless M, Gubitosi-Klug R, Cleary P, et al. Insulin Secretion Measured by Stimulated C-Peptide in Long-Established Type 1 Diabetes in the Diabetes Control and Complications Trial (DCCT)/Epidemiology of Diabetes Interventions and Complications (EDIC) Cohort: A Pilot Study. Diabet. Med (2014) 31:1264–8.
32. Guglielmi C, Del Toro R, Lauria A, Maurizi AR, Fallucca S, Cappelli A, et al. Effect of GLP-1 and GIP on C-Peptide Secretion After Glucagon or Mixed Meal Tests: Significance in Assessing B-Cell Function in Diabetes. Diabetes Metab Res Rev (2017) 33. doi: 10.1002/dmrr.2899
33. Cobelli C, Toffolo GM, Dalla Man C, Campioni M, Denti P, Caumo A, et al. Assessment of Beta-Cell Function in Humans, Simultaneously With Insulin Sensitivity and Hepatic Extraction, From Intravenous and Oral Glucose Tests. Am J Physiol Endocrinol Metab (2007) 293:E1–E15.
34. Besser RE, Shields BM, Casas R, Hattersley AT, Ludvigsson J. Lessons From the Mixed-Meal Tolerance Test: Use of 90-Minute and Fasting C-Peptide in Pediatric Diabetes. Diabetes Care (2013) 36:195–201. doi: 10.2337/dc12-0836
35. Sosenko JM, Palmer JP, Rafkin LE, Krischer JP, Cuthbertson D, Greenbaum CJ, et al. Trends of Earlier and Later Responses of C-Peptide to Oral Glucose Challenges With Progression to Type 1 Diabetes in Diabetes Prevention Trial-Type 1 Participants. Diabetes Care (2010) 33:620–5. doi: 10.2337/dc09-1770
36. Rickels MR, Evans-Molina C, Bahnson HT, Ylescupidez A, Nadeau KJ, Hao W, et al. High Residual C-Peptide Likely Contributes to Glycemic Control in Type 1 Diabetes. J Clin Invest (2020) 130:1850–62. doi: 10.1172/JCI134057
37. Wasserfall C, Nick HS, Campbell-Thompson M, Beachy D, Haataja L, Kusmartseva I, et al. Persistence of Pancreatic Insulin mRNA Expression and Proinsulin Protein in Type 1 Diabetes Pancreata. Cell Metab (2017) 26:568–575 e3. doi: 10.1016/j.cmet.2017.08.013
38. Rodriguez-Calvo T, Zapardiel-Gonzalo J, Amirian N, Castillo E, Lajevardi Y, Krogvold L, et al. Increase in Pancreatic Proinsulin and Preservation of Beta-Cell Mass in Autoantibody-Positive Donors Prior to Type 1 Diabetes Onset. Diabetes (2017) 66:1334–45. doi: 10.2337/db16-1343
39. Freese J, Al-Rawi R, Choat H, Martin A, Lunsford A, Tse H, et al. Proinsulin to C-Peptide Ratio in the First Year After Diagnosis of Type 1 Diabetes. J Clin Endocrinol Metab (2021) 106:e4318–26. doi: 10.1210/clinem/dgab463
40. Rowe PA, Campbell-Thompson ML, Schatz DA, Atkinson MA. The Pancreas in Human Type 1 Diabetes. Semin Immunopathol (2011) 33:29–43. doi: 10.1007/s00281-010-0208-x
41. Clevaland Heartlab. Insulin Resistance. Panel With Score (2018). Available at: https://www.clevelandheartlab.com/tests/insulin-resistance-panel-with-score/.
42. McDonald TJ, Perry MH, Peake RW, Pullan NJ, O’Connor J, Shields BM, et al. EDTA Improves Stability of Whole Blood C-Peptide and Insulin to Over 24 Hours at Room Temperature. PloS One (2012) 7:e42084. doi: 10.1371/journal.pone.0042084
43. Polonsky KS, Rubenstein AH. C-Peptide as a Measure of the Secretion and Hepatic Extraction of Insulin. Pitfalls Limit Diabetes (1984) 33:486–94. doi: 10.2337/diab.33.5.486
44. Polonsky K, Frank B, Pugh W, Addis A, Karrison T, Meier P, et al. The Limitations to and Valid Use of C-Peptide as a Marker of the Secretion of Insulin. Diabetes (1986) 35:379–86. doi: 10.2337/diab.35.4.379
Keywords: type 1 diabetes, Honeymoon phase, partial clinical remission, insulin dose adjusted A1c, insulin sensitivity
Citation: Nwosu BU (2022) Partial Clinical Remission of Type 1 Diabetes: The Need for an Integrated Functional Definition Based on Insulin-Dose Adjusted A1c and Insulin Sensitivity Score. Front. Endocrinol. 13:884219. doi: 10.3389/fendo.2022.884219
Received: 25 February 2022; Accepted: 23 March 2022;
Published: 03 May 2022.
Edited by:
Alok Raghav, Ganesh Shankar Vidyarthi Memorial Medical College, IndiaReviewed by:
Philippe Lysy, Cliniques Universitaires Saint-Luc, BelgiumJamal Ahmad, Aligarh Muslim University, India
Copyright © 2022 Nwosu. This is an open-access article distributed under the terms of the Creative Commons Attribution License (CC BY). The use, distribution or reproduction in other forums is permitted, provided the original author(s) and the copyright owner(s) are credited and that the original publication in this journal is cited, in accordance with accepted academic practice. No use, distribution or reproduction is permitted which does not comply with these terms.
*Correspondence: Benjamin Udoka Nwosu, Ym53b3N1MUBub3J0aHdlbGwuZWR1