- 1College of Animal Science and Technology, Hunan Agricultural University, Changsha, Hunan, China
- 2Animal Sciences Division, Nuclear Institute for Agriculture and Biology College (NIAB-C), Pakistan Institute of Engineering and Applied Sciences (PIEAS), Faisalabad, Pakistan
- 3Department of Biomedical Sciences, College of Veterinary Medicine, King Faisal University, Al-Hofuf, Al-Ahsa, Saudi Arabia
- 4Department of Pharmacology, Faculty of Veterinary Medicine, Kafrelshikh University, Kafrelshikh, Egypt
- 5Department of Cytology and Histology, Faculty of Veterinary Medicine, Suez Canal University, Ismailia, Egypt
- 6Department of Animal Reproduction, Faculty of Animal Husbandry and Veterinary Sciences, Sindh Agriculture University, Tandojam, Sindh, Pakistan
- 7Department of Veterinary Microbiology, Faculty of Animal Husbandry and Veterinary Sciences, Sindh Agriculture University, Tandojam, Sindh, Pakistan
- 8Institute of Subtropical Agriculture, Chinese Academy of Sciences, Changsha, Hunan, China
- 9Department of Zoology, Minhaj University, Lahore, Pakistan
- 10Food Engineering and Bioprocess Technology, Asian Institute of Technology, Bangkok, Thailand
Male infertility is a widely debated issue that affects males globally. There are several mechanisms involved. Oxidative stress is accepted to be the main contributing factor, with sperm quality and quantity affected by the overproduction of free radicals. Excess reactive oxygen species (ROS) cannot be controlled by the antioxidant system and, thus, potentially impact male fertility and hamper sperm quality parameters. Mitochondria are the driving force of sperm motility; irregularities in their function may lead to apoptosis, alterations to signaling pathway function, and, ultimately, compromised fertility. Moreover, it has been observed that the prevalence of inflammation may arrest sperm function and the production of cytokines triggered by the overproduction of ROS. Further, oxidative stress interacts with seminal plasma proteomes that influence male fertility. Enhanced ROS production disturbs the cellular constituents, particularly DNA, and sperms are unable to impregnate the ovum. Here, we review the latest information to better understand the relationship between oxidative stress and male infertility, the role of mitochondria, the cellular response, inflammation and fertility, and the interaction of seminal plasma proteomes with oxidative stress, as well as highlight the influence of oxidative stress on hormones; collectively, all of these factors are assumed to be important for the regulation of male infertility. This article may help improve our understanding of male infertility and the strategies to prevent it.
Introduction
Male infertility is a fertility-related disorder in which a male cannot impregnate a female to achieve a successful pregnancy (1). It is a worldwide issue and contributes to 50% of infertility cases (2) and may occur for multifaceted reasons, such as disruption to the hypothalamus or pituitary function or obstruction or inflammation in the testicles, which subsequently lead to infertility. Moreover, some other conditions, such as hypogonadism, erectile dysfunction, epididymitis, congenital bilateral absence of the vas deferens, and Sertoli cell syndrome, are known to be contributing factors for male infertility (3). Most male infertility factors are idiopathic (2). All of these factors are believed to be directly or indirectly involved in the production of oxidative stress. Reactive oxygen species (ROS) are the active oxidative metabolites that are responsible for producing oxidative stress and are also a prominent cause of male infertility (4, 5). Overwhelming oxidative stress may influence the reproductive system, as well as aspects of the semen, such as sperm concentration, motility, and morphology, thus causing a deterioration in semen quality, resulting in a poor conception rate (6). It has been noted that oxidative stress is involved in diseases that affect male fertility status (7).
The sperm plasma membrane contains polyunsaturated fatty acids, which make it more delicate and vulnerable to oxidative damage, and eventually spermatozoa lose the capacity to fertilize. Moreover, fragmented DNA may impair the paternal genetic ability to develop embryos (5). ROS consist of one or more unpaired electrons, which are capable of damaging lipids, carbohydrates, DNA, and amino acids (8). Interestingly, ROS exist in three forms: primary, secondary, and tertiary. Not all ROS are free radicals (5, 9); however, the physiological concentration of ROS plays a pivotal role in sperm capacitation, hyperactivation, and other acrosomal changes (10). Evidence has indicated that 30–80% of male-related fertility issues are a result of ROS-triggered sperm damage (11–13).
Advanced proteomic tools allow the characterization of semen profiles by applying mechanistic approaches and are helpful for detecting proteins and their underlying molecular mechanisms, which can predict the significance of male fertility-related hindrances (13). Increasing knowledge in this area permits easy understanding of the seminal plasma and sperm proteins and allows the identification of differences between fertile and infertile men (14). Previous literature revealed the relationship between oxidative stress-potentiated male infertility and the sperm and seminal plasma protein profile; alterations to the expression and function of proteins may be evident at sperm maturation. Further studies are needed to identify the pathologies linked to male infertility on molecular and proteomic levels. The impact of oxidative stress has well been documented in male infertility, although limited literature exists about the relationship between oxidative stress and the proteomic profile of human ejaculation. The current literature regarding human infertility reveals the association between oxidative stress and the proteomic profile (15–17). Additionally, further studies based on proteomic profiles have documented poor semen quality, which is influenced by oxidative stress (18, 19).
Male infertility cases can be diagnosed through the evaluation of basic semen characteristics, such as liquefaction time, sperm count, motility, morphological features, and sperm viability. However, the WHO has set some guidelines or reference values for sperm abnormality, alteration of sperm concentration, motility, and morphology by which the fertility status of humans, as well as animals, can be assessed (20, 21). Numerous advanced tools can be applied to figure out the possible causes of infertility, based on the detection of free radicals, antioxidant capacity analysis, sperm DNA oxidation, DNA compaction, apoptosis, the presence of anti-sperm antibodies, and genetic testing (22). Elevated concentrations of ROS have been reported in infertile human patients with DNA damage and unstable chromatin packing (5). Sperm DNA damage is a biomarker for the loss of cellular integrity, which is associated with a decline in semen quality, and is thus regarded as a cause of infertility in many humans (23, 24). Assisted reproductive biotechnology using spermatozoa with fragmented DNA is more susceptible to lower fertilization and pregnancy rates, abnormal embryonic development, and an increased risk of miscarriage, congenital defects, and other anomalies that occur during childhood (25, 26). The amount of DNA fragmentation is a viable indicator of assisted reproductive outcomes in idiopathic infertile couples. However, increased sperm DNA fragmentation has been associated with lower birth weight after IVF treatment (24). Our main purpose when designing this review was to elaborate on the role of mitochondria, the cellular response in fertility-related problems, and the interaction of seminal plasma proteomes with oxidative stress and highlight the influence of oxidative stress on hormones.
ROS and mitochondria function
A higher level of ROS induces oxidative stress caused by oxidants in germ cells. Mitochondria are key organelles; at low levels, ROS maintain redox balance. Excessive levels of ROS potentiate lipid peroxidation events, which inhibit small molecules of aldehydes, such as acrolein, malondialdehyde, and 4-hydroxynonenal (4-HNE). These molecules bind with protein sites at susceptible histidine, lysine, and cysteine residues on targeted proteins (27). The activity of these proteins impairs electron flow towards the mitochondrial electron transport chain and generates free radicals that are responsible for the production of more aldehyde products (27). Any factor that influences germ cells through the production of oxidants by way of oxidative phosphorylation can cause oxidant cascades. Oxidative stress occurs for several reasons, such as a lack of antioxidants, ionization radiation, leukocytes, obesity, smoking, reproductive tract infections, and pesticides. A positive relationship between spermatozoa consisting of polyunsaturated fatty acids and free radicals has been well established.
Mitochondrial ROS production is an essential process for inducing intrinsic apoptosis. A huge number of spermatozoa eventually undergo apoptosis, while the limited number that remain are pivotal for the successful continuation of the fertilization process. Lipopolysaccharide (LPS), a bacterial endotoxin, is known to induce apoptosis (28) in a large number of spermatozoa. Apoptosis is an essential process for the continuation of life as macrophages and neutrophils rarely exert phagocytosis to eliminate dead spermatozoa. It has been noted that the apoptotic process can be completed irrespective of the activation of inflammation, cytokines, and ROS production. However, leukocyte infiltration causes a damaging effect due to the occurrence of an inflammatory response that repeats after a vasectomy or during a sexual act. These sperms induce a response, which may be reversed in the presence of phosphatidylserine (an apoptosis marker), that subjects gametes to phagocytosis. The ROS and RNS mechanism that is crucial for the basic development correction and functional activity of spermatozoa is displayed in Figure 1.
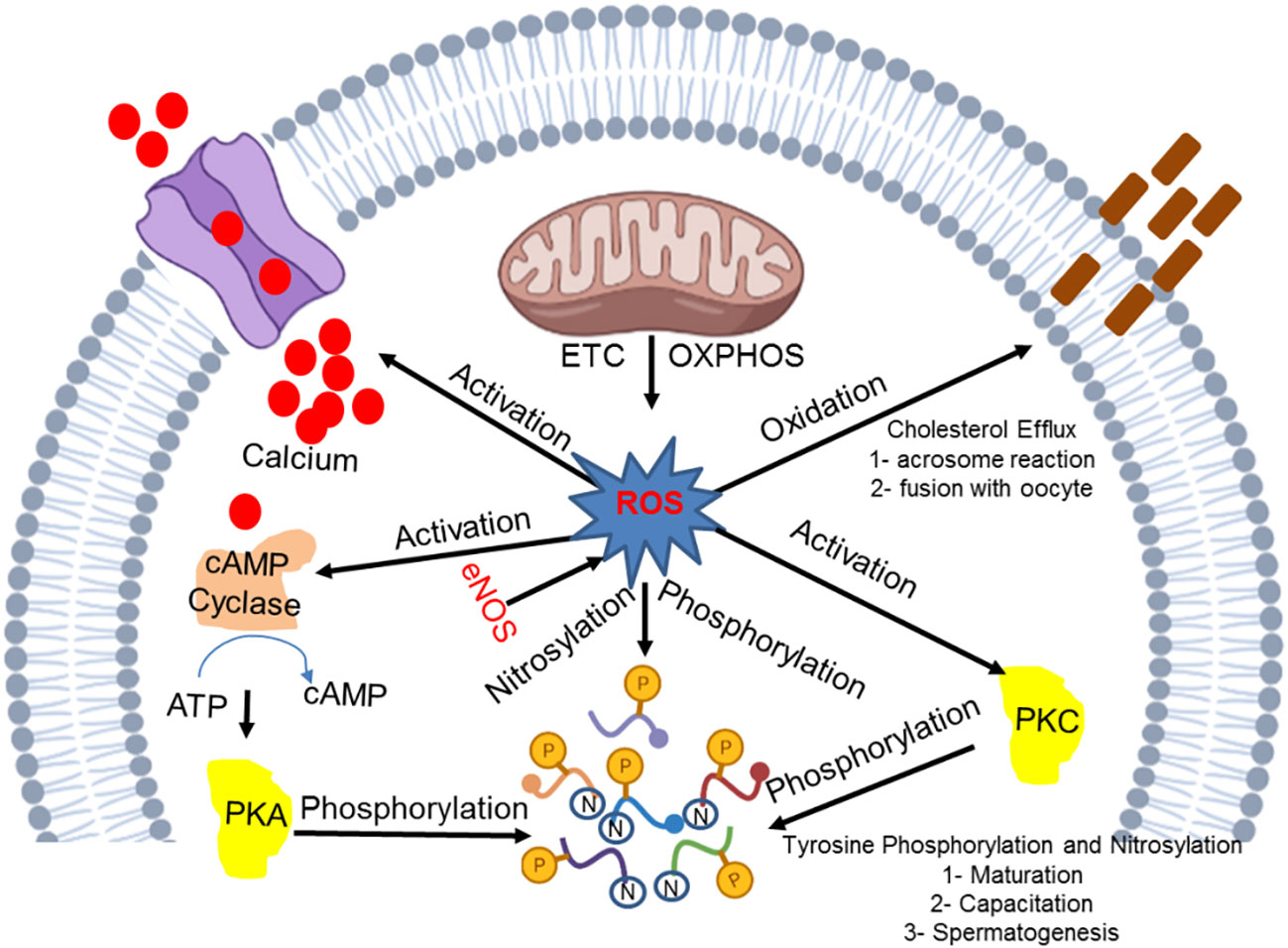
Figure 1 Molecular insight into the ROS and RNS mechanism in the development and functional integrity of spermatozoa.
Realistically, spermatozoa undergo apoptosis due to the activation of an enzyme that cells utilize for their survival, known as phosphatidylinositol-4,5-bisphosphate 3-kinase (PI3K) (29). As the activation of the PI3K signaling pathway takes place, it phosphorylates downstream kinases, such as AKT (Protein kinase B), thus stimulation of these pathways makes gametes active and viable. AKT targets downstream proteins, such as BCL2-related death promoter (BAD), which, once dephosphorylated, form pro-apoptotic pores in the mitochondrial membrane and foster pore formation with BAK/BAX (30). Once the basic mechanism of sperm apoptotic origin is understood, it is necessary to understand the underlying mechanism that promotes PI3K activity. Of note, spermatozoa contain several pro-survival hormonal receptors, such as prolactin (31) and insulin, once they are stimulated by their respective ligands, which makes their continuous survival sustainable. By contrast, if the PI3K inhibitor wortmanin is used then gametes promptly promote mitochondrial ROS generation, rendering cells more susceptible to apoptosis (29).
ROS and the capacitation process
ROS are stimulated by several mechanisms that are based on the activation of adenylyl cyclase activity (32), which in turn stimulates protein kinase A (33, 34). H202 plays the key role during capacitation of mediating the processes of phosphorylation and capacitation, and this has been well reported in suspensions of hamster, bovine, and human sperm (32). Likewise, exposure of the spermatozoa to synthetic oxidized conditions induces the extracellular generation of ROS through glucose oxidase or xanthine oxidase systems and the initiation of the capacitation process. Tyrosine phosphorylation can be attenuated by the induction of catalase in several species (32). However, ROS-generated leucocytes contribute to human sperm capacitation and may reverse in the presence of seminal plasma antioxidants (35). The profound function of H202 is illustrated by catalase, which restores the spontaneous induction of tyrosine phosphorylation in capacitating mammalian spermatozoa, and hence, reduces functions, such as hyperactivation, acrosomal exocytosis, and sperm-egg fusion; all of these steps are achieved following capacitation (36). A variety of ROS sources have been used for the activation of capacitation processes, such as superoxide anion, nitric oxide, and peroxynitrite (37). It has been noted that a huge interconversion of ROS occurs during sperm capacitation and any ROS can take part in it. If the potential of oxidative metabolites displays a crucial role in capacitation then the regulators will be H2O2 and peroxynitriate. Peroxynitriate is responsible for producing a variety of capacitating spermatozoa features, e.g., suppression of tyrosine phosphate activity (38).
The positive impact of ROS generation and capacitation has been reported in the female reproductive tract. Spermatozoa can only produce excessive ROS once they are released by the oviductal epithelium, immediately before the site of fertilization. In this scenario, every spermatozoon is briefly exposed to ROS and prepares itself for fertilization. In case fertilization by spermatozoa does not occur, spontaneous free radical production leads to overcapacitation and ultimately induces oxidative stress. Eventually, this leads to the production of lipid aldehydes, which initiate ROS-mediated peroxidation and subsequently trigger apoptosis (39, 40). Sperm capacitation towards apoptosis may assist in the long-term storage of spermatozoa to sustain sperm capacitation for a longer period. The reality is that several domestic species of spermatozoa undergo capacitation-like changes that lead to oxidative and stress-related cryopreservation, and this may be a potential factor facilitating the longevity of these gametes prior to insemination (41). The best way to ameliorate oxidative stress-induced cryopreserved spermatozoa is through the addition of antioxidants, such as lycopene, cysteamine, melatonin, vitamin E, and resveratrol (42), which are widely used due to their significant impact. Molecular insights into the spermatozoa capacitation process and apoptosis are depicted in Figure 2.
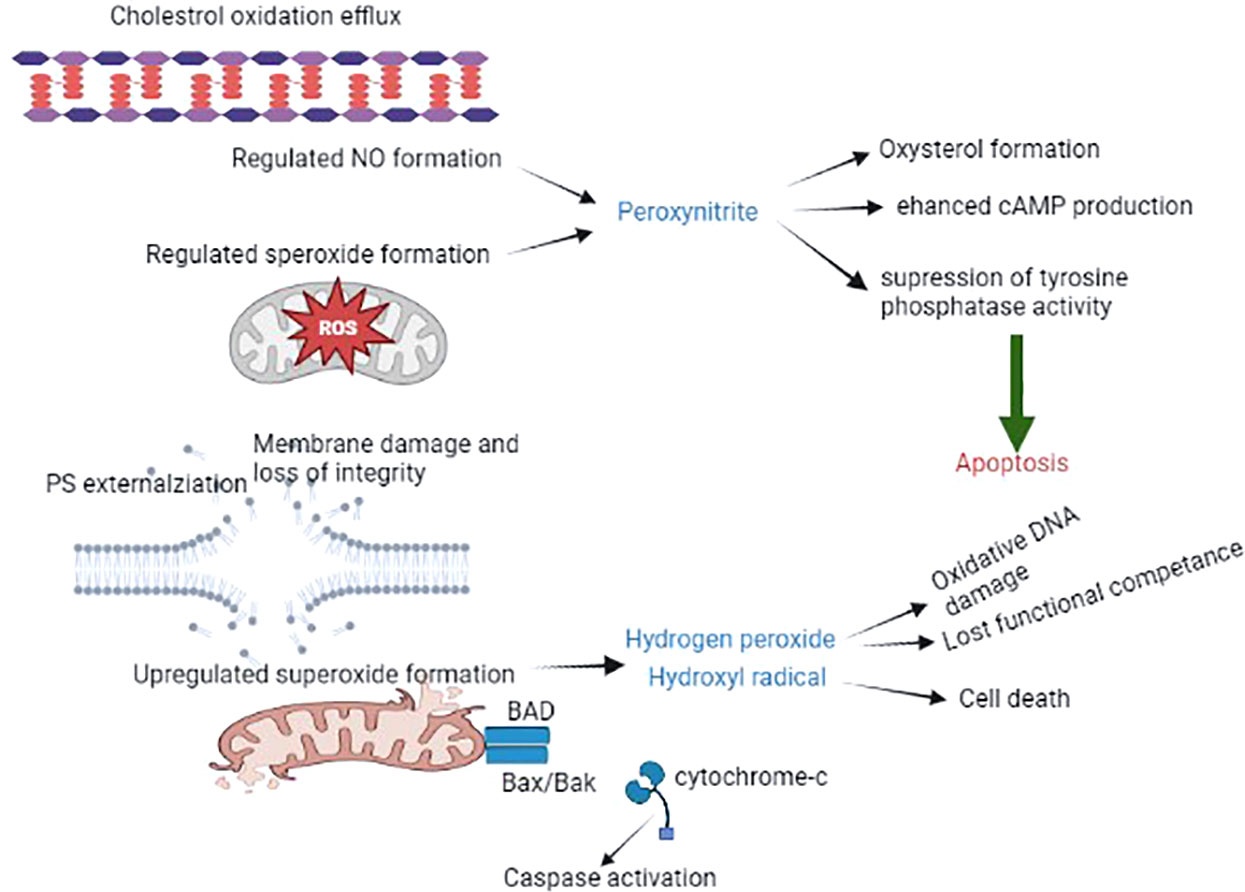
Figure 2 The spermatozoa capacitation process and apoptosis. The schematic diagram illustrates the accelerating production of ROS (mainly ONOO), resulting in the generation of oxysterol, which helps to eliminate cholesterol from the plasma membrane, leading to the promotion of membrane fluidity and other alterations, such as tyrosine phosphatase suppression and enhanced cAMP activity. This process eventually leads to capacitated spermatozoa. The absence of fertilization results in the generation of oxysterol and lipid aldehydes, which trigger apoptosis, resulting in increased mitochondrial superoxide production, lipid peroxidation, cytochrome c release, caspase activation, phosphatidylserine exposure, oxidative DNA fragmentation, and ultimately death.
Oxidative insult and male hormones in reproduction
The occurrence of oxidative stress depends on either the overproduction of ROS or depletion of antioxidants, which may result in lipid peroxidation in Leydig cells and germ cells and is detrimental to lipoproteins, protein aggregation and fragmentation, and steroidogenic enzyme inhibition (43). The prevalence of OS in the testicles results in declining testosterone production due to injury of the Leydig cells or other endocrine structures, such as the anterior pituitary (44, 45). It is notable that the physiological production of hormones also produces ROS that are mainly derived from mitochondrial respiration and catalytic reactions of the steroidogenic cytochrome P450 enzymes (46). In this way, the production of ROS suppresses the substantial production of steroids and is deleterious to the mitochondrial membranes of the spermatozoa (47). OS is associated with a higher number of immature spermatozoa through an indirect effect on male hormone production, which is associated with spermatogenesis (48, 49).
It has been noted that hormones, such as follicle stimulating hormone (FSH), luteinizing hormone (LH), testosterone, estrogen (E2) and prolactin (PRL), may regulate seminal total antioxidant capacity (TAC) (50, 51). An association between PRL or free thyroxine T4 (fT4) and a negative correlation of gonadotropins or gonadal steroids with TAC have also been observed (52). It is believed that some hormones, such as testosterone and melatonin (MLT), may increase antioxidant capacity to defend sperm and other testicular cells from the detrimental effects of ROS (53, 54). Other hormonal metabolites, such as dehydroepiandrosterone (DHEA), increase cellular antioxidants through an exact mechanism that remains elusive (55). In infertile men, direct and indirect connections between testosterone and antioxidant levels and between testosterone and zinc have been documented (51, 56). Coenzyme Q10 (CoQ10) may reduce the concentrations of FSH and LH (57). The negative association has been exhibited in serum concentrations of testosterone, E2, fT4, and sperm DNA fragmentation (58, 59). The suppression of antioxidants might influence triiodothyronine (T3), thyroxine (T4), and neurotransmitter noradrenaline and elevate sperm DNA fragmentation (60). The administration of highly purified FSH to idiopathic infertile men reduces ROS production (61) and sperm DNA damage (62). However, it has been found that testosterone may trigger DNA fragmentation and germ cell caspase activities in Sertoli cells (63), and a longer antioxidant effect may modulate FSH, testosterone, and inhibin B concentration (64).
As discussed above, excessive ROS influences the hypothalamic-pituitary adrenal axis (HPA) and in turn releases corticosterone and cortisol in animals and humans, which induces stress. The release of stress hormones impairs crosstalk between HPG and HPA axes, disrupting LH production by the anterior pituitary gland. Reduced levels of LH are unable to activate Leydig cells, which produce testosterone. Reduced FSH weakens the production of androgen-binding protein (ABP) by the Sertoli cells, and hence, collectively reduces the level of circulatory testosterone due to abundant OS. ROS influences the HPT axis to diminish T3 and T4 secretion. Reduced T3 levels of steroidogenic acute regulatory protein (StAR) mRNA, protein in Leydig cells, and testosterone production occur (65). The overproduction of OS reduces insulin production by the pancreas, which exerts a detrimental effect on T3 release by the thyroid gland, and therefore, testosterone biosynthesis occurs. During OS production, testicular E2 and inhibin are largely secreted, which suppress testosterone production. After OS production, aromatase activity is enhanced, which leads to higher E2 production. Further, exposure to ROS promotes PRL release by the anterior pituitary gland, which results in a reduction in the release of GnRH. In summary, it has been observed that OS disrupt hormonal communication in different ways. Hormones play a key role in the functionality of the male reproductive system. ROS affect testosterone production, which in turn influences spermatogenesis. Moreover, OS also affects male reproductive behavior by interfering with testosterone production, eventually causing infertility.
Cellular response against inflammation and infertility
Inflammation is a natural defense against foreign invaders that causes cellular injury and subsequently results in the restoration of tissue function (66, 67). It has been noted that an inflammatory response is established due to the excessive production of prostaglandin E2, cytokines, and nitric oxide (NO) by macrophages and other inflammatory cells (68). Some evidence suggests that inflammation may affect steroidogenesis and spermatogenesis. A sudden decline of blood testosterone and luteinizing hormones have also been noted with inflammation (69). A study was conducted in which lipopolysaccharide (LPS) was used to stimulate an inflammatory response; a considerable reduction in testosterone was observed. However, a lower response of steroidogenesis was reported and known to be called steroid acute regulatory StAR proteins (70). Evidence has revealed that inflammation increases spermatogenic arrest and inhibits the sperm maturation process (71). The epididymis is another target of inflammation due to testicular attacks. Importantly, inflammation is triggered by leukocytes that infiltrate semen and secrete anti-sperm antibodies. The inflammation reaction promotes rigidity of the sperm flagella membrane by reducing the lipid content of the membrane. Thus, the inhibition of sperm motility may result in sperm agglutination and asthenospermia. Additionally, it causes defects in acrosome reaction, which prevent sperm penetrating the oolemma. Moreover, it suppresses DNA integrity due to the increased number of apoptotic sperm cells (5).
Previous literature indicate a relationship between oxidative stress and inflammation. The inflammatory response has been documented in semen due to elevated levels of ROS in infertile men (72). Moreover, invading bacteria generate ROS by themselves, whereas leukocytes are considered to be the essential player of seminal ROS (8). Leukocytes increase ROS production in two ways, one direct and one indirect; the indirect source involves the release of inflammatory cytokines, which increase the level of ROS. A direct increase in ROS is achieved through the activation of phagocytosis. These oxidants harm the spermatozoon membrane, resulting in an oxidative burst in which the oxidant/antioxidant ratio is severely disrupted. This scenario even occurs when pathogens are successfully executed (69).
Cytokines are polypeptide proteins that are attributed to immune response, cellular growth and differentiation, inflammation, etc. In the male reproductive tract, cytokines are secreted by the testes and are responsible for germ cell proliferation and the differentiation of mesenchymal cells and take part in steroid anabolism (8, 73). A growing number of studies have revealed that ROS and cytokines interplay with each other in a complex manner. ROS increase cytokine production, while a few cytokines regulate the pro-oxidant and antioxidant system and ROS production (5, 74, 75). Many studies have described the relationship between cytokines and ROS. For example, increased concentrations of IL-6 and IL-8 trigger the peroxidation process, influencing sperm functionality and eventually causing infertility during male reproductive tract inflammation (5). The limited concentration of cytokines plays an essential role in the male gonad’s function and seems to be present in seminal plasma (76). A vast network of cytokines, chemokines, and growth factors, along with their soluble receptors and antagonists and other factors, were investigated in human semen (76, 77). Human semen also secretes tumor necrosis factor α (TNF-α), interleukins, IFN-γ, and some of their soluble receptors, which are present in immune cells, mesenchymal cells, Sertoli cells, and spermatogonia. The physiological concentration of cytokines (IL-6, IL-8, and TNF-α) has been reported in human semen (76, 77). Cytokines are not directly involved in apoptosis, but TNF-α, TGF-β2, and TGF-β3, in addition to testosterone, are capable of regulating spermatogenesis (78, 79). However, TGF-β plays various roles in cellular functions, including the secretary function of Leydig cells, Sertoli cells, the biological development of testes, and spermatogenesis intensity (80). As discussed earlier, sperm consists of an array of cytokines and immune factors, although their effect on semen quality and sperm function parameters needs to be debated (81, 82).
Oxidative insults, cellular defense, and male reproduction
It has been reported that increased oxidative stress can be implicated in various pathogenic conditions, such as inflammation, ischemia, and heat stress, which suggests it plays an important role in male infertility (5, 83). Spermatozoa are easily targeted by oxidation. Spermatogenic cells eliminate oxidative DNA by apoptosis through p53-dependent and -independent mechanisms (84), showing that higher activity may lead to male infertility (85). However, redox-sensitive proteins are the most susceptible to ROS and are regarded as ROS potent targets under oxidative stress.
The cellular antioxidant defense neutralizes ROS (superoxide anion radicals), such as superoxide dismutase (SOD) and glutathione peroxidase (GPX). Further, a detailed review of an antioxidant enzymatic system in male reproduction has been discussed (86, 87); only the ameliorative effects of ROS in male reproductive anomalies have been described in this Review. SOD is an enzyme that converts superoxide radicals into hydrogen peroxide, which halts the deleterious effects of a radical chain reaction at the initiation stage (88). Copper-zinc sodium dismutase encoded by SOD1 is mainly present in the cytoplasm, and partly in mitochondria. Although SOD1-deficient females are infertile, SOD1 is not associated with anomalies that hamper male fertility (89). Moreover, SOD1 enzyme deficiency may induce testicular atrophy and confer proneness to heat stress (90). Sperm numbers following higher incidences of lipid peroxidation products were reduced in aged SOD1-deficient mice compared with wild-type mice (91), while the fertilizing ability linkage was not found in mice. Manganese sodium dismutase, a mitochondrial isoform, works under the influence of oxidative stress and inflammation. The deficiency of this enzyme is lethal once the fetus/infant is born (92). Moreover, transgenic mice may exhibit a higher expression of SOD2 and are infertile; the underlying mechanism of this condition is unknown (93). SOD3 is an extracellular SOD that is present at high levels in epididymis fluid and at low levels in spermatogenic cells (94). SOD3 knockout mice do not show any prominent phenotypic alteration in male reproduction, although the presence of SOD3 in the penis has been associated with increased erectile function in aged mice (95). The superoxide radical rapidly reacts with nitric oxide to form peroxinitrite and higher levels of SOD3 in blood plasma enhance the half-life of nitric oxide and ultimately promote erectile functions. Conversely, excessive SOD activity has been linked with human sperm movement anomalies (96) that may eliminate superoxide, suggesting that SOD plays an important role in sperm movement. As a result, both the source and the underlying mechanism determine whether superoxide has a beneficial or detrimental effect on reproductive function.
It has long been known that a variety of sources of hydrogen peroxide production via enzymatic and non-enzymatic reactions exist and their successful elimination is carried out by glutathione peroxidase (GPX), catalase, and peroxiredoxin (PRDX). GPX demonstrates this by catalyzing the reduction of different peroxidases through the transfer of electrons from glutathione (97), while the functions of each member and gene family are different and complex (98). Peroxiredoxins (PRDXs) catalyze the reductive removal of hydrogen peroxide with the help of thioredoxin (Trx), not glutathione, as it donates an electron (99) and also has multifaceted functions in redox reactions consisting of ROS signaling.
Oxidative stress in seminal plasma and sperm
Free radicals or ROS are oxygen-based centered radicals with one or more unpaired electrons (100). Examples of free radicals and non-radicals are hydroxyl, superoxide, peroxyl, and lipid peroxyl, while the non-radicals consists of singlet oxygen, hydrogen peroxides, hypochloric acid, lipid peroxide, and ozone (101). The most important ROS from sperm are hydroxyl radicals, superoxide anion, and hydrogen peroxide. Owing to their high reactivity in nature, they possess very short half-lives, i.e., nanoseconds (10−9 s) for hydroxyl radicals and milliseconds (10−3 s) for superoxide anion; therefore, they react at their generation site (102, 103). Moreover, when the dismutation of superoxide anion takes place, it causes the formation of hydrogen peroxide, which is almost a weak free radical (104, 105). Once the superoxide and hydrogen peroxide are produced, they undergo different cellular reactions and then transform into highly powerful hydroxyl radical free radicals via the Fenton and Haber–Weiss reaction (106). In addition to that, superoxide anion interrelates with nitric oxide and forms a peroxynitrite. Nitric oxide is also regarded as a reactive free radical with an odd number of electrons (105, 107). The most critical sources of ROS production in males are leukocytes and immature spermatozoa (85). Leukocytes, especially neutrophils and macrophages, have been linked with excessive ROS formation, which causes sperm dysfunction (101, 108).
Oxidants and semen parameters
It is well known that limited concentrations of ROS are essential for spermatogenesis. ROS generation in the context of antioxidants is necessary for spermatogenic processes to occur. Antioxidants, such as hydrogen peroxide, contribute to the sperm capacitation process and thus help spermatozoa bind to the zona pellucida and fertilize the egg (9, 83). Notably, catalase causes the decomposition of H202 and also maintains sperm motility (5). This relationship between H202 and catalase balances redox status and abrogates oxidative stress. Free radicals are the byproducts of oxidative metabolism in mitochondria. In response to these reactions, oxygen reduction occurs in mitochondria (5). Normally, mitochondria are located in the midpiece of the sperm, and studies have indicated that mitochondrial DNA is more vulnerable to mutation than nuclear DNA; therefore, it elevates ROS production (109). Enhanced ROS production has been associated with the stimulation of cytochrome-c, a protein involved in apoptosis (programmed cell death), and is reported in males with infertility problems (110). Previous studies have highlighted that the generation of mitochondrial-mediated ROS production is deeply involved in DNA damage (111). DNA damage by ROS may subsequently lead to poor in vitro blastocyst formation (112). Moreover, the sperm membrane mainly consists of polyunsaturated fatty acids, which play a key role in membrane fusion (9). However, seminal fluid is an essential source of antioxidants in semen, as the spermatozoa’s lack of cytoplasm and DNA compaction mean there is little space for antioxidant enzymic translations (113). As aforementioned, the fragile structure of the sperm membrane can be easily targeted by ROS, which ultimately affects sperm motility (114). The positive and negative effect of ROS on male infertility is illustrated in Figure 3.
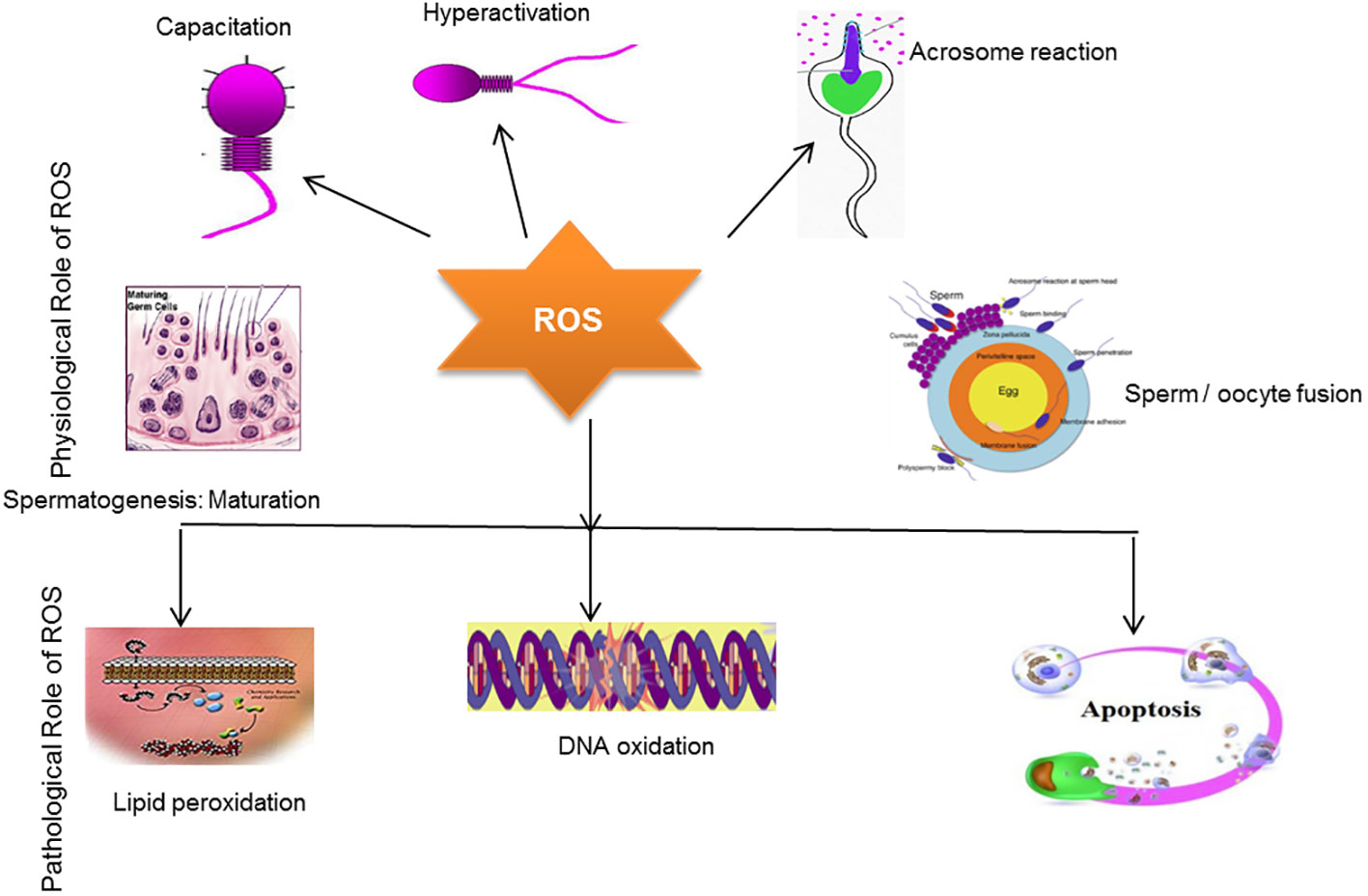
Figure 3 The positive and negative effect of ROS in male infertility. The schematic diagram demonstrates that a limited concentration of ROS plays a key role in sperm capacitation, sperm hyperactivation, acrosomal reaction, sperm maturation, and sperm/oocyte fusion processes. Conversely, overproduction of ROS induces oxidative stress, which ultimately damages the sperm membrane, causing lipid peroxidation and DNA oxidation, which eventually induce apoptosis, resulting in infertility.
Seminal plasma proteomes and oxidative stress
A variety of proteins have been proposed to be the possible markers of oxidative stress (OS) damage. Wang and colleagues suggest that the decline of DJ-1 protein contributes to the suppression of OS triggered by endocrine disruptors, a proposed marker of OS in asthenozoospermic patients (19). Herwig and colleagues observed that tubulin folding cofactor β and higher levels of α-1 chymotrypsin and aldose reductase are associated with OS in patients with idiopathic oligo-astheno-teratozoospermia (18). Another study indicated the increased expression of prolactin-triggered protein, which is related to OS damage and poor sperm quality (16). Intasqui and colleagues showed that overexpression of mucin 5B in normozoospermicmen correlated with increased seminal lipid peroxidation levels, suggesting that this protein contributes to sperm transport alteration in both sexes; therefore, it could be a marker of lipid peroxidation resulting from OS (115). Of note, OS-augmented modulation of the seminal plasma proteome does not normally occur on large scales in infertile patients, but it also prevails in fertile subjects. In a current study, fertile patients with enhanced OS exhibited overexpression of proteins attributed to stress response, such as haptoglobin (HP), peroxiredoxin 4 (PRDX4), and protein S100 calcium-binding protein A9 (S100A9). Specifically, HP and PRDX4 exert antioxidant properties, thus their overexpression is known to be involved in the scavenging effect against the overproduction of ROS. The S100A9 protein possesses pro-inflammatory activity; its overexpression along with C3 complement shows the inflammatory state caused by OS (116). The overexpression of seminal plasma proteome due to oxidative stress in patients is presented in Table 1.
Lifestyle and diseases may trigger seminal plasma OS. Obesity, alcohol abuse, cigarette smoking, and heavy metals have been firmly linked with OS. Moreover, environmental factors, such as heavy metals, also contribute to excessive ROS. Additionally, ROS occur as a result of various diseases, such as accessory gland infection/inflammation (MAGI) and varicocele (117). At present, irrespective of varicocele, few studies have reported SP proteome modulation in patients. Moreover, there is no literature about the differential expression of proteins with and without increased ROS, and few proteins have been suggested as disease markers. However, intelectin 1 overexpression has been observed in asthenozoospermic patients with OS, revealing the possible existence of genital tract infection. Likewise, a study reported that alcohol dehydrogenase overexpression contributed to alcohol metabolism and aminolevulenic acid dehydratase overexpression indicated exposure to lead. Thus, it proves that lifestyle and environmental factors have a detrimental effect on sperm quality due to the overproduction of free radicals (19). Further detailed information regarding the involvement of male infertility problems is well illustrated by (118).
Evidence of sperm transcriptomic profile and oxidative stress
Transcriptionally sperm cells are energetic; RNAs are presumed to be involved in spermatogenic events (119). It is thought that sperm RNAs are linked to several functions, including fertilization (119, 120). Further, RNAs in sperm are known to be indicators of sperm quality index (121–123) and fertility (120, 124, 125). Interestingly, sperm consists of coding and non-coding RNAs (123, 126) that potentially might have an effect on sperm activeness. A DNA microarray revealed that 559 transcripts in low-fertile bulls are dysregulated. Notably, transcripts such as PRDX6, NOS3, SOD, BAK, and BCL2L11 have been associated with fertility and are linked to the oxidation reduction process, the mediation of MMP, and apoptosis. This study provides a pathway to develop male-fertility-related markers (127).
The bull transcriptomic profile revealed that non-coding RNAs (ncRNAs) are involved in the regulation of sperm motility (128). The ncRNAs are the main regulators of spermatogenesis and male fertility but literature on lncRNAs in human oligozoospermia is scant. The sequencing data of lncRNA and mRNA from 12 human normozoospermia and oligozoospermia samples revealed the altered expression of lncRNAs (DE lncRNAs) and mRNAs (DE mRNAs) in male infertility. The Gaussian graphical model, gene ontology, and Encyclopedia of Genes and Genomes pathways were applied to identify them and investigate their possible functions. The transcriptome data showed that DE lncRNAs and DE mRNAs and their target genes were involved in the accretion of unfolded proteins in sperm ER, PERK-EIF2 pathway-induced ER stress, oxidative stress, and apoptotic sperms in individual oligozoospermia subjects. This suggests that these lncRNAs and pathways could be utilized as a therapeutic target for infertility. There is less evidence about the semen transcriptomic profile in terms of interactions with oxidative stress. More studies are required to determine whether oxidative stress is involved in male infertility problems (129).
Several RNA-seq studies have attempted to characterize the transcriptome of ejaculated spermatozoa in terms of sperm quality and fertility. Semen quality varies according to season. A total of 4,436 coding genes of moderate to high abundance have been identified in sperm RNA. The fragmentation of the transcript increased in genes associated with spermatogenesis, chromatin compaction, and fertility. The summer and winter ejaculates had different transcriptomic profiles, with 34 coding genes and 7 microRNAs showing a significantly distinct distribution. These genes were linked to oxidative stress, DNA damage, and autophagy. The annotation of the boar sperm transcriptomic profile was used to identify sperm quality markers in pigs (130). Table 2 shows the involvement of transcriptomic factors on male infertility.
Oxidative stress and DNA fragmentation
The overproduction of ROS may influence male infertility by interacting with different cellular components, resulting in sperm damage (138, 139). This process involves lipid peroxidation and protein oxidation through the utilization of numerous molecular mechanisms. In particular, OS induces the production of oxidized DNA adducts, such as 8-hydroxy-2’-deoxyguanosine (8OHdG) within the DNA, resulting in single- or double-strand breaks (140). Moreover, ROS stimulates caspases and nucleases that contribute to apoptotic pathways; therefore, they cause indirect damage to the sperm DNA through abortive apoptosis (141).
Presently, research measuring oxidative stress relies on estimations of intracellular ROS (using a chemiluminescence assay) (142), total antioxidant capacity (TAC) (143), malondialdehyde (144), or DNA damage (8-OHdG) (145), which have been identified as markers of OS and significant sperm damage in infertile patients (146–148). Further, sperm DNA damage impairs sperm fertility capacity and embryo development during natural conception and has been linked with assisted reproductive tools (149–151). Intriguingly, it has been noted that measurement of oxidative stress might be important for infertile subjects who can benefit from antioxidant supplementation or an alteration in lifestyle (152). These considerations show that there is a dire need to understand the correlation between seminal plasma oxidative stress and sperm DNA damage and for the development of new diagnostic methods. More recently, a novel galvanostat-based technique was used to measure OS. This technique determines the balance between oxidants and reductants in semen, which is known as the oxidation-reduction potential (ORP) (153).
Spermatozoa possess a one-base excision repair (BER) enzyme upstream during their development, which is helpful for DNA repair. This enzyme is known as 8-oxoguanine DNA glycosylase 1 (OGG1), and it assists in the release of adducts into the extracellular space through the excision of DNA base adducts (154, 155). Spermatozoa do not possess BER enzymes, such as apurinic endonuclease 1 (APE1) and x-ray repair cross-complementing protein 1 (XRCC1). For that reason, the DNA repair ability of spermatozoa is delicate, resulting in the repair of oxidized DNA base adducts, such as 8-OHdG (155). Moreover, it has been found that 8-OHdG triggers germline mutations, indirectly causing DNA damage in human spermatozoa (156).
Male infertility preventive strategies
Male infertility is a highly concerning issue that has not received much focus in terms of better understanding its magnitude and prevalence. Several factors of male infertility are idiopathic in nature. As such, there is an emerging need to address the problem and investigate preventive strategies (157).
The following approaches should be considered for preventing male infertility problems:
1. Oxidative stress is the main cause of male infertility induction and attempts should be made to limit the production of oxidative stress. However, it should be kept in mind that some ROS production is needed to maintain male fertility.
2. The cellular mechanism involved in male infertility may provide new pathways for drug development from antioxidant compounds that are safe and secure and exert less toxic effects than commercially available classical drugs.
3. Nanoparticle-based approaches could be useful for the targeted delivery of polyphenol-derived drugs.
4. The integration of knowledge and computer science through machine learning algorithms should be adopted in male infertility diagnostic approaches, as well as in searches for targeted therapies (158).
5. An integrated AI system should assist the assessment of computerized semen analysis; AI-based applications can estimate environmental conditions and lifestyle to improve semen quality forecasts.
6. The cause of idiopathic male infertility is unknown but AI-based technology can improve the classification of fertile/infertile couples using biological and clinical signatures.
7. An attempt should be made to break down barriers linked to religious and cultural beliefs that prevent individuals from speaking openly about their infertility issues.
8. There is need of create awareness among populations so that male infertility problems can be discussed more frequently.
9. Excessive weight has been linked with reduced sperm production. Therefore, diet and daily exercise need to planned appropriately.
10. Addiction tends to influence physiological function. Addictive behavior needs to be avoided and monitored.
11. Tightly fitting clothing influences blood circulation to the genital organs and raises testicular temperature, thus disturbing semen production and decreasing fertility. Therefore, tight clothes need to be avoided.
12. Electronic gadgets that produce low levels of radiation eventually disturb sperm production. Therefore, it is better to minimize the use of these gadgets.
13. Deficiency of nutrients, particularly zinc and vitamin C, may disturb sperm production. Therefore, it is important to have a healthy and balanced diet. Supplementation can be used if the diet lacks the required nutrition.
14. Infection and inflammation may severely influence sperm production. Proper treatment following the doctor’s instructions and daily exercise boost the immune system and normalize the situation.
Conclusions
In conclusion, we have reviewed the relationship between oxidative stress and male infertility and the involvement of proteomic studies in male infertility. We have compared the values of differential protein profiles in seminal plasma in both oxidative and physiological conditions. The proteomic profile of seminal plasma may play an important role in preventing oxidative stress, and it has been recognized as a putative marker/indicator of the prevalence of oxidative stress. With the literature in mind, the pathway analysis indicates the contribution of proteins to stress, cellular, metabolic, and regulatory pathways. The compiled studies in this Review will contribute to the exploration of the prominent causes of idiopathic male infertility. It is hoped that if male infertility is recognized at a molecular level, its diagnosis, treatment, and prevention can be improved. It was difficult to enumerate which mechanism should be targeted In normozoospermic conditions. However, this scenario is still incomplete and further research is needed to develop diagnostic assays based on methylated patterns, such as RNA and phosphorylation profiles. We further highlighted the attractiveness of sperm DNA integrity as a biomarker for unexplained infertility. In the coming years, it is expected that idiopathic fertility can be diagnosed using omics technologies.
Author contributions
TH: conceptualization, writing—original draft preparation, MK and EM: methodology, illustration of figures and. GM and DHK, editing of manuscript, BT, funding acquisition and visualization, editing of the manuscript, YY, MIC, AF, AY, MSK editing of the manuscript. All authors contributed and approved the submitted version of manuscript.
Funding
This work was supported by the National Key R&D Program (2021YFD1300401), the National Natural Science Foundation of China (U20A2054 and 32130099), and the earmarked fund for CARS (CARS-35).
Conflict of interest
The authors declare that the research was conducted in the absence of any commercial or financial relationships that could be construed as a potential conflict of interest.
Publisher’s note
All claims expressed in this article are solely those of the authors and do not necessarily represent those of their affiliated organizations, or those of the publisher, the editors and the reviewers. Any product that may be evaluated in this article, or claim that may be made by its manufacturer, is not guaranteed or endorsed by the publisher.
References
1. Medicine PCotASfR. Definitions of infertility and recurrent pregnancy loss: a committee opinion. Fertil Steril (2013) 99(1):63. doi: 10.1016/j.fertnstert.2012.09.023
3. Parekattil SJ, Esteves SC, Agarwal A. Male Infertility: Contemporary clinical approaches, andrology, ART & antioxidants. Springer (2012).
4. Hamada AJ, Montgomery B, Agarwal A. Male Infertility: A critical review of pharmacologic management. Expert Opin Pharmacother (2012) 13(17):2511–31. doi: 10.1517/14656566.2012.740011
5. Tremellen K. Oxidative stress and male infertility–a clinical perspective. Hum Reprod Update (2008) 14(3):243–58. doi: 10.1093/humupd/dmn004
6. Khosrowbeygi A, Zarghami N. Levels of oxidative stress biomarkers in seminal plasma and their relationship with seminal parameters. BMC Clin Pathol (2007) 7(1):1–6. doi: 10.1186/1472-6890-7-6
7. Pasqualotto FF, Sharma RK, Nelson DR, Thomas AJ Jr., Agarwal A. Relationship between oxidative stress, semen characteristics, and clinical diagnosis in men undergoing infertility investigation. Fertil Steril (2000) 73(3):459–64. doi: 10.1016/S0015-0282(99)00567-1
8. Ochsendorf F. Infections in the male genital tract and reactive oxygen species. Hum Reprod Update (1999) 5(5):399–420. doi: 10.1093/humupd/5.5.399
9. Hwang K, Lamb DJ. Molecular mechanisms of antioxidants in male infertility. Male infertility: Springer (2012), 45–54. doi: 10.1007/978-1-4614-3335-4_5
10. Agarwal A, Allamaneni SS, Said TM. Chemiluminescence technique for measuring reactive oxygen species. Reprod BioMed Online (2004) 9(4):466–8. doi: 10.1016/S1472-6483(10)61284-9
11. Iwasaki A, Gagnon C. Formation of reactive oxygen species in spermatozoa of infertile patients. Fertil Steril (1992) 57(2):409–16. doi: 10.1016/S0015-0282(16)54855-9
12. Ochsendorf F, Thiele J, Fuchs J, Schüttau H, Freisleben H, Buslau M, et al. Chemiluminescence in semen of infertile men. Andrologia (1994) 26(5):289–93. doi: 10.1111/j.1439-0272.1994.tb00804.x
13. Du Plessis SS, Kashou AH, Benjamin DJ, Yadav SP, Agarwal A. Proteomics: a subcellular look at spermatozoa. Reprod Biol Endocrinol (2011) 9(1):1–12. doi: 10.1186/1477-7827-9-36
14. Mitulović G, Mechtler K. HPLC techniques for proteomics analysis–a short overview of latest developments. Brief Funct Genomics (2006) 5(4):249–60. doi: 10.1093/bfgp/ell034
15. Hamada A, Sharma R, Du Plessis SS, Willard B, Yadav SP, Sabanegh E, et al. Two-dimensional differential in-gel electrophoresis–based proteomics of male gametes in relation to oxidative stress. Fertil Steril (2013) 99(5):1216–26. e2. doi: 10.1016/j.fertnstert.2012.11.046
16. Sharma R, Agarwal A, Mohanty G, Du Plessis SS, Gopalan B, Willard B, et al. Proteomic analysis of seminal fluid from men exhibiting oxidative stress. Reprod Biol Endocrinol (2013) 11(1):1–15. doi: 10.1186/1477-7827-11-85
17. Sharma R, Agarwal A, Mohanty G, Hamada AJ, Gopalan B, Willard B, et al. Proteomic analysis of human spermatozoa proteins with oxidative stress. Reprod Biol Endocrinol (2013) 11(1):1–18. doi: 10.1186/1477-7827-11-48
18. Herwig R, Knoll C, Planyavsky M, Pourbiabany A, Greilberger J, Bennett KL. Proteomic analysis of seminal plasma from infertile patients with oligoasthenoteratozoospermia due to oxidative stress and comparison with fertile volunteers. Fertil Steril (2013) 100(2):355–66.e2. doi: 10.1016/j.fertnstert.2013.03.048
19. Wang J, Wang J, Zhang H-R, Shi H-J, Ma D, Zhao H-X, et al. Proteomic analysis of seminal plasma from asthenozoospermia patients reveals proteins that affect oxidative stress responses and semen quality. AJA (2009) 11(4):484. doi: 10.1038/aja.2009.26
20. Organisation WH. WHO laboratory manual for the examination of human semen and sperm-cervical mucus interaction. Camb Univ Press (1999).
21. Lu J-C, Huang Y-F, Lü N-Q. WHO laboratory manual for the examination and processing of human semen: its applicability to andrology laboratories in China. Zhonghua nan ke xue Nat J Androl (2010) 16(10):867–71.
22. Kovac JR, Pastuszak AW, Lamb DJ. The use of genomics, proteomics, and metabolomics in identifying biomarkers of male infertility. Fertil Steril (2013) 99(4):998–1007. doi: 10.1016/j.fertnstert.2013.01.111
23. Lewis SE, Aitken RJ, Conner SJ, De Iuliis G, Evenson DP, Henkel R, et al. The impact of sperm DNA damage in assisted conception and beyond: Recent advances in diagnosis and treatment. Reprod BioMed Online (2013) 27(4):325–37. doi: 10.1016/j.rbmo.2013.06.014
24. Simon L, Proutski I, Stevenson M, Jennings D, McManus J, Lutton D, et al. Sperm DNA damage has a negative association with live-birth rates after IVF. Reprod BioMed Online (2013) 26(1):68–78. doi: 10.1016/j.rbmo.2012.09.019
25. Aitken R, Bronson R, Smith T, De Iuliis G. The source and significance of DNA damage in human spermatozoa; a commentary on diagnostic strategies and straw man fallacies. MHR: Basic Sci Reprod Med (2013) 19(8):475–85. doi: 10.1093/molehr/gat025
26. Lewis SE. Sperm DNA fragmentation and base oxidation. Gen Dam Hum Spermat (2014), 103–16. doi: 10.1007/978-1-4614-7783-9_7
27. Aitken RJ, Whiting S, De Iuliis GN, McClymont S, Mitchell LA, Baker MA. Electrophilic aldehydes generated by sperm metabolism activate mitochondrial reactive oxygen species generation and apoptosis by targeting succinate dehydrogenase. JBC (2012) 287(39):33048–60. doi: 10.1074/jbc.M112.366690
28. Fujita Y, Mihara T, Okazaki T, Shitanaka M, Kushino R, Ikeda C, et al. Toll-like receptors (TLR) 2 and 4 on human sperm recognize bacterial endotoxins and mediate apoptosis. Hum Reprod (2011) 26(10):2799–806. doi: 10.1093/humrep/der234
29. Koppers AJ, Mitchell LA, Wang P, Lin M, Aitken RJ. Phosphoinositide 3-kinase signalling pathway involvement in a truncated apoptotic cascade associated with motility loss and oxidative DNA damage in human spermatozoa. Biochem (2011) 436(3):687–98. doi: 10.1042/BJ20110114
30. Polzien L, Baljuls A, Rennefahrt UE, Fischer A, Schmitz W, Zahedi RP, et al. Identification of novel in vivo phosphorylation sites of the human proapoptotic protein BAD: pore-forming activity of BAD is regulated by phosphorylation. JBC (2009) 284(41):28004–20. doi: 10.1074/jbc.M109.010702
31. Pujianto DA, Curry BJ, Aitken RJ. Prolactin exerts a prosurvival effect on human spermatozoa via mechanisms that involve the stimulation of akt phosphorylation and suppression of caspase activation and capacitation. Endocrinology (2010) 151(3):1269–79. doi: 10.1210/en.2009-0964
32. Rivlin J, Mendel J, Rubinstein S, Etkovitz N, Breitbart H. Role of hydrogen peroxide in sperm capacitation and acrosome reaction. Biol Reprod (2004) 70(2):518–22. doi: 10.1095/biolreprod.103.020487
33. O’Flaherty C, de Lamirande E, Gagnon C. Reactive oxygen species modulate independent protein phosphorylation pathways during human sperm capacitation. Free Radic Biol Med (2006) 40(6):1045–55. doi: 10.1016/j.freeradbiomed.2005.10.055
34. O’Flaherty C, de Lamirande E, Gagnon C. Positive role of reactive oxygen species in mammalian sperm capacitation: Triggering and modulation of phosphorylation events. Free Radic Biol Med (2006) 41(4):528–40. doi: 10.1016/j.freeradbiomed.2006.04.027
35. Villegas J, Kehr K, Soto L, Henkel R, Miska W, Sanchez R. Reactive oxygen species induce reversible capacitation in human spermatozoa. Andrologia (2003) 35(4):227–32. doi: 10.1046/j.1439-0272.2003.00564.x
36. Aitken R, Paterson M, Fisher H, Buckingham D, Van Duin M. Redox regulation of tyrosine phosphorylation in human spermatozoa and its role in the control of human sperm function. J Cell Sci (1995) 108(5):2017–25. doi: 10.1242/jcs.108.5.2017
37. Rodriguez P, Beconi M. Peroxynitrite participates in mechanisms involved in capacitation of cryopreserved cattle. Anim Reprod Sci (2009) 110(1-2):96–107. doi: 10.1016/j.anireprosci.2007.12.017
38. Takakura K, Beckman JS, MacMillan-Crow LA, Crow JP. Rapid and irreversible inactivation of protein tyrosine phosphatases PTP1B, CD45, and LAR by peroxynitrite. Arch Biochem Biophy (1999) 369(2):197–207. doi: 10.1006/abbi.1999.1374
39. Aitken RJ. The capacitation-apoptosis highway: Oxysterols and mammalian sperm function. Biol Reprod (2011) 85(1):9–12. doi: 10.1095/biolreprod.111.092528
40. Aitken RJ, Baker MA, Nixon B. Are sperm capacitation and apoptosis the opposite ends of a continuum driven by oxidative stress? AJA (2015) 17(4):633. doi: 10.4103/1008-682X.153850
41. Cormier N, Ma S, Bailey Jl Premature capacitation of bovine spermatozoa is initiated by cryopreservation. J Androl (1997) 18(4):461–8. doi: 10.1002/j.1939-4640.1997.tb01953.x
42. Ashrafi I, Kohram H, Ardabili FF. Antioxidative effects of melatonin on kinetics, microscopic and oxidative parameters of cryopreserved bull spermatozoa. Anim Reprod Sci (2013) 139(1-4):25–30. doi: 10.1016/j.anireprosci.2013.03.016
43. Darbandi S, Darbandi M. Lifestyle modifications on further reproductive problems. Cresco J Reprod Sci (2016) 1(1):1–2.
44. Zirkin BR, Chen H. Regulation of leydig cell steroidogenic function during aging. Biol Reprod (2000) 63(4):977–81. doi: 10.1095/biolreprod63.4.977
45. Turner TT, Bang HJ, Lysiak JJ. Experimental testicular torsion: Reperfusion blood flow and subsequent testicular venous plasma testosterone concentrations. Urology (2005) 65(2):390–4. doi: 10.1016/j.urology.2004.09.033
46. Hanukoglu I. Antioxidant protective mechanisms against reactive oxygen species (ROS) generated by mitochondrial P450 systems in steroidogenic cells. Drug Metab Rev (2006) 38(1-2):171–96. doi: 10.1080/03602530600570040
47. Luo L, Chen H, Trush MA, Show MD, Anway MD, Zirkin BR. Aging and the brown Norway rat leydig cell antioxidant defense system. J Androl (2006) 27(2):240–7. doi: 10.2164/jandrol.05075
48. Aitken RJ, Baker MA, Sawyer D. Oxidative stress in the male germ line and its role in the aetiology of male infertility and genetic disease. Reprod BioMed Online (2003) 7(1):65–70. doi: 10.1016/S1472-6483(10)61730-0
49. Agarwal A, Said TM. Role of sperm chromatin abnormalities and DNA damage in male infertility. Hum Reprod Update (2003) 9(4):331–45. doi: 10.1093/humupd/dmg027
50. Meucci E, Milardi D, Mordente A, Martorana GE, Giacchi E, De Marinis L, et al. Total antioxidant capacity in patients with varicoceles. Fertil Steril (2003) 79:1577–83. doi: 10.1016/S0015-0282(03)00404-7
51. Mancini A, Leone E, Festa R, Grande G, Silvestrini A, De Marinis L, et al. Effects of testosterone on antioxidant systems in male secondary hypogonadism. J Androl (2008) 29(6):622–9. doi: 10.2164/jandrol.107.004838
52. Mancini A, Festa R, Silvestrini A, Nicolotti N, Di Donna V, La Torre G, et al. Hormonal regulation of total antioxidant capacity in seminal plasma. J Androl (2009) 30(5):534–40. doi: 10.2164/jandrol.108.006148
53. Chainy G, Samantaray S, Samanta L. Testosterone-induced changes in testicular antioxidant system. Andrologia (1997) 29(6):343–9. doi: 10.1111/j.1439-0272.1997.tb00328.x
54. Shang X, Huang Y, Ye Z, Yu X, Gu W. Protection of melatonin against damage of sperm mitochondrial function induced by reactive oxygen species. Zhonghua nan ke xue= Natl J Androl (2004) 10(8):604–7.
55. Lakpour N, Mahfouz RZ, Akhondi MM, Agarwal A, Kharrazi H, Zeraati H, et al. Relationship of seminal plasma antioxidants and serum male hormones with sperm chromatin status in male factor infertility. Syst Biol Reprod Med (2012) 58(5):236–44. doi: 10.3109/19396368.2012.689923
56. Oluboyo A, Adijeh R, Onyenekwe C, Oluboyo B, Mbaeri T, Odiegwu C, et al. Relationship between serum levels of testosterone, zinc and selenium in infertile males attending fertility clinic in nnewi, south east Nigeria. AJMMS (2012) 41:51–4.
57. Safarinejad MR. Efficacy of coenzyme Q10 on semen parameters, sperm function and reproductive hormones in infertile men. J Urol (2009) 182(1):237–48. doi: 10.1016/j.juro.2009.02.121
58. Richthoff J, Spano M, Giwercman Y, Frohm B, Jepson K, Malm J, et al. The impact of testicular and accessory sex gland function on sperm chromatin integrity as assessed by the sperm chromatin structure assay (SCSA). Hum Reprod (2002) 17(12):3162–9. doi: 10.1093/humrep/17.12.3162
59. Meeker JD, Singh NP, Hauser R. Serum concentrations of estradiol and free T4 are inversely correlated with sperm DNA damage in men from an infertility clinic. J Androl (2008) 29(4):379–88. doi: 10.2164/jandrol.107.004416
60. Dobrzyńska MM, Baumgartner A, Anderson D. Antioxidants modulate thyroid hormone-and noradrenaline-induced DNA damage in human sperm. Mutagenesis (2004) 19(4):325–30. doi: 10.1093/mutage/geh037
61. Palomba S, Falbo A, Espinola S, Rocca M, Capasso S, Cappiello F, et al. Effects of highly purified follicle-stimulating hormone on sperm DNA damage in men with male idiopathic subfertility: a pilot study. J Endocrinol Invest (2011) 34(10):747–52. doi: 10.3275/7745
62. Colacurci N, Monti MG, Fornaro F, Izzo G, Izzo P, Trotta C, et al. Recombinant human FSH reduces sperm DNA fragmentation in men with idiopathic oligoasthenoteratozoospermia. J Androl (2012) 33(4):588–93. doi: 10.2164/jandrol.111.013326
63. Tesarik J, Martinez F, Rienzi L, Iacobelli M, Ubaldi F, Mendoza C, et al. In-vitro effects of FSH and testosterone withdrawal on caspase activation and DNA fragmentation in different cell types of human seminiferous epithelium. Hum Reprod (2002) 17(7):1811–9. doi: 10.1093/humrep/17.7.1811
64. Nematollahi-Mahani S, Azizollahi G, Baneshi M, Safari Z, Azizollahi S. Effect of folic acid and zinc sulphate on endocrine parameters and seminal antioxidant level after varicocelectomy. Andrologia (2014) 46(3):240–5. doi: 10.1111/and.12067
65. Manna PR, Tena-Sempere M, Huhtaniemi IT. Molecular mechanisms of thyroid hormone-stimulated steroidogenesis in mouse leydig tumor cells: Involvement of the steroidogenic acute regulatory (StAR) protein. JBC (1999) 274(9):5909–18. doi: 10.1074/jbc.274.9.5909
66. Kalyanaraman B, Darley-Usmar V, Davies KJ, Dennery PA, Forman HJ, Grisham MB, et al. Measuring reactive oxygen and nitrogen species with fluorescent probes: challenges and limitations. Free Radic Biol Med (2012) 52(1):1–6. doi: 10.1016/j.freeradbiomed.2011.09.030
67. Adewoyin M, Mohsin SMN, Arulselvan P, Hussein MZ, Fakurazi S. Enhanced anti-inflammatory potential of cinnamate-zinc layered hydroxide in lipopolysaccharide-stimulated RAW 264.7 macrophages. Drug Des Dev Ther (2015) 9:2475. doi: 10.2147/DDDT.S72716
68. O’Bryan MK, Schlatt S, Gerdprasert O, Phillips DJ, de Kretser DM, Hedger MP. Inducible nitric oxide synthase in the rat testis: Evidence for potential roles in both normal function and inflammation-mediated infertility. Biol Reprod (2000) 63(5):1285–93. doi: 10.1095/biolreprod63.5.1285
69. Wang J, Wang W, Li S, Han Y, Zhang P, Meng G, et al. Hydrogen sulfide as a potential target in preventing spermatogenic failure and testicular dysfunction. ARS (2018) 28(16):1447–62. doi: 10.1089/ars.2016.6968
70. Hales DB. Interleukin-1 inhibits leydig cell steroidogenesis primarily by decreasing 17 alpha-hydroxylase/C17-20 lyase cytochrome P450 expression. Endocrinology (1992) 131(5):2165–72. doi: 10.1210/endo.131.5.1425417
71. Liew SH, Meachem SJ, Hedger MP. A stereological analysis of the response of spermatogenesis to an acute inflammatory episode in adult rats. J Androl (2007) 28(1):176–85. doi: 10.2164/jandrol.106.000752
72. D’agata R, Vicari E, Moncada M, Sidoti G, Calogero A, Fornito M, et al. Generation of reactive oxygen species in subgroups of infertile men. Int J Androl (1990) 13(5):344–51. doi: 10.1111/j.1365-2605.1990.tb01042.x
73. Diemer T, Hales D, Weidner W. Immune–endocrine interactions and leydig cell function: the role of cytokines. Andrologia (2003) 35(1):55–63. doi: 10.1046/j.1439-0272.2003.00537.x
74. Moretti E, Collodel G, Mazzi L, Campagna M, Iacoponi F, Figura N. Resistin, interleukin-6, tumor necrosis factor-alpha, and human semen parameters in the presence of leukocytospermia, smoking habit, and varicocele. Fertil Steril (2014) 102(2):354–60. doi: 10.1016/j.fertnstert.2014.04.017
75. Lazaros LA, Xita NV, Chatzikyriakidou AL, Kaponis AI, Grigoriadis NG, Hatzi EG, et al. Association of TNFα, TNFR1, and TNFR2 polymorphisms with sperm concentration and motility. J Androl (2012) 33(1):74–80. doi: 10.2164/jandrol.110.011486
76. Maegawa M, Kamada M, Irahara M, Yamamoto S, Yoshikawa S, Kasai Y, et al A repertoire of cytokines in human seminal plasma. J Reprod Immunol (2002) 54(1-2):33–42. doi: 10.1016/S0165-0378(01)00063-8
77. Politch JA, Tucker L, Bowman FP, Anderson DJ. Concentrations and significance of cytokines and other immunologic factors in semen of healthy fertile men. Hum Reprod (2007) 22(11):2928–35. doi: 10.1093/humrep/dem281
78. Cheng CY, Mruk DD. A local autocrine axis in the testes that regulates spermatogenesis. Nat Rev Endocrinol (2010) 6(7):380–95. doi: 10.1038/nrendo.2010.71
79. Li MW, Mruk DD, Lee WM, Cheng CY. Cytokines and junction restructuring events during spermatogenesis in the testis: An emerging concept of regulation. Cytokine Growth Factor Rev (2009) 20(4):329–38. doi: 10.1016/j.cytogfr.2009.07.007
80. Gonzalez CR, Matzkin ME, Frungieri MB, Terradas C, Ponzio R, Puigdomenech E, et al. Expression of the TGF-beta1 system in human testicular pathologies. Reprod Biol Endocrinol (2010) 8(1):1–11. doi: 10.1186/1477-7827-8-148
81. Camejo M, Segnini A, Proverbio F. Interleukin-6 (IL-6) in seminal plasma of infertile men, and lipid peroxidation of their sperm. Arch Androl (2001) 47(2):97–101. doi: 10.1080/014850101316901280
82. Vera O, Vásqucz LA, Muñoz MG. Semen quality and presence of cytokines in seminal fluid of bull ejaculates. Theriogenology (2003) 60(3):553–8. doi: 10.1016/S0093-691X(03)00031-1
83. Aitken RJ, Buckingham DW, Brindle J, Gomez E, Baker HG, Irvine DS. Andrology: Analysis of sperm movement in relation to the oxidative stress created by leukocytes in washed sperm preparations and seminal plasma. Hum Reprod (1995) 10(8):2061–71. doi: 10.1093/oxfordjournals.humrep.a136237
84. Yin Y, DeWolf WC, Morgentaler A. Experimental cryptorchidism induces testicular germ cell apoptosis by p53-dependent and-independent pathways in mice. Biol Reprod (1998) 58(2):492–6. doi: 10.1095/biolreprod58.2.492
85. Garrido N, Meseguer M, Simon C, Pellicer A, Remohi J. Pro-oxidative and anti-oxidative imbalance in human semen and its relation with male fertility. Asian J Androl (2004) 6(1):59–66.
86. Bauché F, Fouchard M-H, Jégou B. Antioxidant system in rat testicular cells. FEBS letters. (1994) 349(3):392–6. doi: 10.1016/0014-5793(94)00709-8
87. Fujii J, Iuchi Y, Matsuki S, Ishii T. Cooperative function of antioxidant and redox systems against oxidative stress in male reproductive tissues. Asian J Androl (2003) 5(3):231–42.
88. Fridovich I. Superoxide radical and superoxide dismutases. Annu Rev Biochem (1995) 64(1):97–112. doi: 10.1146/annurev.bi.64.070195.000525
89. Ho Y-S, Gargano M, Cao J, Bronson RT, Heimler I, Hutz RJ. Reduced fertility in female mice lacking copper-zinc superoxide dismutase. JBC (1998) 273(13):7765–9. doi: 10.1074/jbc.273.13.7765
90. Ishii T, Matsuki S, Iuchi Y, Okada F, Toyosaki S, Tomita Y, et al. Accelerated impairment of spermatogenic cells in SOD1-knockout mice under heat stress. Free Rad Res (2005) 39(7):697–705. doi: 10.1080/10715760500130517
91. Tsunoda S, Kawano N, Miyado K, Kimura N, Fujii J. Impaired fertilizing ability of superoxide dismutase 1-deficient mouse sperm during in vitro fertilization. Biol Reprod (2012) 87(5):121. doi: 10.1095/biolreprod.112.102129
92. Li Y, Huang T-T, Carlson EJ, Melov S, Ursell PC, Olson JL, et al. Dilated cardiomyopathy and neonatal lethality in mutant mice lacking manganese superoxide dismutase. Nat Genet (1995) 11(4):376–81. doi: 10.1038/ng1295-376
93. Raineri I, Carlson EJ, Gacayan R, Carra S, Oberley TD, Huang T-T, et al. Strain-dependent high-level expression of a transgene for manganese superoxide dismutase is associated with growth retardation and decreased fertility. Free Radic Biol Med (2001) 31(8):1018–30. doi: 10.1016/S0891-5849(01)00686-4
94. Ookawara T, Kizaki T, Takayama E, Imazeki N, Matsubara O, Ikeda Y, et al. Nuclear translocation of extracellular superoxide dismutase. BBRC (2002) 296(1):54–61. doi: 10.1016/S0006-291X(02)00804-5
95. Bivalacqua TJ, Armstrong JS, Biggerstaff J, Abdel-Mageed AB, Kadowitz PJ, Hellstrom WJ, et al. Gene transfer of extracellular SOD to the penis reduces O 2–• and improves erectile function in aged rats. Am J Physiol Heart Circ Physiol (2003) 284(4):H1408–H21. doi: 10.1152/ajpheart.00770.2002
96. Aitken J, Fisher H. Reactive oxygen species generation and human spermatozoa: the balance of benefit and risk. Bioessays (1994) 16(4):259–67. doi: 10.1002/bies.950160409
97. Imai H, Nakagawa Y. Biological significance of phospholipid hydroperoxide glutathione peroxidase (PHGPx, GPx4) in mammalian cells. Free Rad Biol Med (2003) 34(2):145–69. doi: 10.1016/S0891-5849(02)01197-8
98. Flohé L. Selenium in mammalian spermiogenesis. Biological Chemistry (2007) 388(10):987–95. doi: 10.1515/BC.2007.112
99. Rhee SG, Woo HA, Kil IS, Bae SH. Peroxiredoxin functions as a peroxidase and a regulator and sensor of local peroxides. JBC (2012) 287(7):4403–10. doi: 10.1074/jbc.R111.283432
100. Chen S-j, Allam J-P, Duan Y-g, Haidl G. Influence of reactive oxygen species on human sperm functions and fertilizing capacity including therapeutical approaches. Arch Gynecol Obstet (2013) 288(1):191–9. doi: 10.1007/s00404-013-2801-4
101. Bansal AK, Bilaspuri G. Impacts of oxidative stress and antioxidants on semen functions. Vet Med Int (2011) 2011:1–8. doi: 10.4061/2011/686137
102. Henkel RR. Leukocytes and oxidative stress: Dilemma for sperm function and male fertility. AJA (2011) 13(1):43. doi: 10.1038/aja.2010.76
103. Miranda-Vilela AL, Alves PCZ, Akimoto AK, Pereira LCS, NazaréKlautau-Guimarães Md, Grisolia CK. The effect of hydrogen peroxide-induced oxidative stress on leukocytes depends on age and physical training in healthy human subjects carrying the same genotypes of antioxidant enzymes’ gene polymorphisms. Am J Hum Biol (2010) 22(6):807–12. doi: 10.1002/ajhb.21086
104. Halliwell B, Clement MV, Long LH. Hydrogen peroxide in the human body. FEBS letters. (2000) 486(1):10–3. doi: 10.1016/S0014-5793(00)02197-9
105. Du Plessis SS, Agarwal A, Halabi J, Tvrda E. Contemporary evidence on the physiological role of reactive oxygen species in human sperm function. J Assist Reprod Genet (2015) 32(4):509–20. doi: 10.1007/s10815-014-0425-7
106. Koppenol WH. The haber-Weiss cycle–70 years later. Redox Rep (2001) 6(4):229–34. doi: 10.1179/135100001101536373
107. Kelm M. Nitric oxide metabolism and breakdown. BBA (1999) 1411(2-3):273–89. doi: 10.1016/S0005-2728(99)00020-1
108. Saleh RA, Agarwal A, Kandirali E, Sharma RK, Thomas AJ Jr., Nada EA, et al. Leukocytospermia is associated with increased reactive oxygen species production by human spermatozoa. Fertil Steril (2002) 78(6):1215–24. doi: 10.1016/S0015-0282(02)04237-1
109. Liu C-Y, Lee C-F, Hong C-H, Wei Y-H. Mitochondrial DNA mutation and depletion increase the susceptibility of human cells to apoptosis. Mitochondrial Pathogenesis: Springer (2004) p:133–45. doi: 10.1007/978-3-662-41088-2_14
110. Wang X, Sharma RK, Gupta A, George V, Thomas AJ Jr., Falcone T, et al. Alterations in mitochondria membrane potential and oxidative stress in infertile men: a prospective observational study. Fertil Steril (2003) 80:844–50. doi: 10.1016/S0015-0282(03)00983-X
111. Henkel R, Kierspel E, Stalf T, Mehnert C, Menkveld R, Tinneberg H-R, et al. Effect of reactive oxygen species produced by spermatozoa and leukocytes on sperm functions in non-leukocytospermic patients. Fertil Steril (2005) 83(3):635–42. doi: 10.1016/j.fertnstert.2004.11.022
112. Meseguer M, Martinez-Conejero J, O’Connor JE, Pellicer A, Remohí J, Garrido N. The significance of sperm DNA oxidation in embryo development and reproductive outcome in an oocyte donation program: A new model to study a male infertility prognostic factor. Fertil Steril (2008) 89(5):1191–9. doi: 10.1016/j.fertnstert.2007.05.005
113. Jeulin C, Soufir J, Weber P, Laval-Martin D, Calvayrac R. Catalase activity in human spermatozoa and seminal plasma. Gamet Res (1989) 24(2):185–96. doi: 10.1002/mrd.1120240206
114. Agarwal A, Makker K, Sharma R. Clinical relevance of oxidative stress in male factor infertility: an update. Am J Reprod Immunol (2008) 59(1):2–11. doi: 10.1111/j.1600-0897.2007.00559.x
115. Intasqui P, Antoniassi MP, Camargo M, Nichi M, Carvalho VM, Cardozo KHM, et al. Differences in the seminal plasma proteome are associated with oxidative stress levels in men with normal semen parameters. Fertil Steril (2015) 104(2):292–301. doi: 10.1016/j.fertnstert.2015.04.037
116. Dias TR, Samanta L, Agarwal A, Pushparaj PN, Panner Selvam MK, Sharma R. Proteomic signatures reveal differences in stress response, antioxidant defense and proteasomal activity in fertile men with high seminal ROS levels. Int J Mol Sci (2019) 20(1):203. doi: 10.3390/ijms20010203
117. Agarwal A, Durairajanayagam D, Halabi J, Peng J, Vazquez-Levin M. Proteomics, oxidative stress and male infertility. Reprod BioMed Online (2014) 29(1):32–58. doi: 10.1016/j.rbmo.2014.02.013
118. Cannarella R, Crafa A, Barbagallo F, Mongioì LM, Condorelli RA, Aversa A, et al. Seminal plasma proteomic biomarkers of oxidative stress. Int J Mol Sci (2020) 21(23):9113. doi: 10.3390/ijms21239113
119. Gòdia M, Swanson G, Krawetz SA. A history of why fathers’ RNA matters. Biol Reprod (2018) 99(1):147–59. doi: 10.1093/biolre/ioy007
120. Jodar M, Sendler E, Moskovtsev SI, Librach CL, Goodrich R, Swanson S, et al. Absence of sperm RNA elements correlates with idiopathic male infertility. Sci Transl Med (2015) 7(295):295re6–re6. doi: 10.1126/scitranslmed.aab1287
121. Bissonnette N, Lévesque-Sergerie J-P, Thibault C, Boissonneault G. Spermatozoal transcriptome profiling for bull sperm motility: A potential tool to evaluate semen quality. Reproduction (2009) 138(1):65. doi: 10.1530/REP-08-0503
122. Ablondi M, Gòdia M, Rodriguez-Gil JE, Sánchez A, Clop A. Characterisation of sperm piRNAs and their correlation with semen quality traits in swine. Anim Genet (2021) 52(1):114–20. doi: 10.1111/age.13022
123. Gòdia M, Castelló A, Rocco M, Cabrera B, Rodríguez-Gil JE, Balasch S, et al. Identification of circular RNAs in porcine sperm and evaluation of their relation to sperm motility. Sci Rep (2020) 10(1):1–11. doi: 10.1038/s41598-020-64711-z
124. Feugang J, Rodriguez-Osorio N, Kaya A, Wang H, Page G, Ostermeier G, et al. Transcriptome analysis of bull spermatozoa: Implications for male fertility. Reprod BioMed Online (2010) 21(3):312–24. doi: 10.1016/j.rbmo.2010.06.022
125. Govindaraju A, Uzun A, Robertson L, Atli MO, Kaya A, Topper E, et al. Dynamics of microRNAs in bull spermatozoa. Reprod Biol Endocrinol (2012) 10(1):1–10. doi: 10.1186/1477-7827-10-82
126. Krawetz SA, Kruger A, Lalancette C, Tagett R, Anton E, Draghici S, et al. A survey of small RNAs in human sperm. Hum Reprod (2011) 26(12):3401–12. doi: 10.1093/humrep/der329
127. Saraf KK, Kumaresan A, Sinha MK, Datta TK. Spermatozoal transcripts associated with oxidative stress and mitochondrial membrane potential differ between high-and low-fertile crossbred bulls. Andrologia (2021) 53(5):e14029. doi: 10.1111/and.14029
128. Wang X, Yang C, Guo F, Zhang Y, Ju Z, Jiang Q, et al. Integrated analysis of mRNAs and long noncoding RNAs in the semen from Holstein bulls with high and low sperm motility. Sci Rep (2019) 9(1):1–9. doi: 10.1038/s41598-018-38462-x
129. Sun T-C, Zhang Y, Yu K, Li Y, Yu H, Zhou S-J, et al. LncRNAs induce oxidative stress and spermatogenesis by regulating endoplasmic reticulum genes and pathways. Aging (Albany NY) (2021) 13(10):13764. doi: 10.18632/aging.202971
130. Gòdia M, Estill M, Castelló A, Balasch S, Rodríguez-Gil JE, Krawetz SA, et al. A RNA-seq analysis to describe the boar sperm transcriptome and its seasonal changes. Front Genet (2019) 10:299. doi: 10.3389/fgene.2019.00299
131. Lu J, Gu H, Tang Q, Wu W, Yuan B, Guo D, et al. Common SNP in hsa-miR-196a-2 increases hsa-miR-196a-5p expression and predisposes to idiopathic male infertility in Chinese han population. Sci Rep (2016) 6(1):1–8. doi: 10.1038/srep19825
132. Wu W, Qin Y, Li Z, Dong J, Dai J, Lu C, et al. Genome-wide microRNA expression profiling in idiopathic non-obstructive azoospermia: Significant up-regulation of miR-141, miR-429 and miR-7-1-3p. Hum Reprod (2013) 28(7):1827–36. doi: 10.1093/humrep/det099
133. Che Q, Wang W, Duan P, Fang F, Liu C, Zhou T, et al. Downregulation of miR-322 promotes apoptosis of GC-2 cell by targeting Ddx3x. Reprod Boil Endocrinol (2019) 17(1):1–9. doi: 10.1186/s12958-019-0506-7
134. Radtke A, Dieckmann KP, Grobelny F, Salzbrunn A, Oing C, Schulze W, et al. Expression of miRNA-371a-3p in seminal plasma and ejaculate is associated with sperm concentration. Andrology (2019) 7(4):469–74. doi: 10.1111/andr.12664
135. Hong Y, Wang C, Fu Z, Liang H, Zhang S, Lu M, et al. Systematic characterization of seminal plasma piRNAs as molecular biomarkers for male infertility. Sci Rep (2016) 6(1):1–10. doi: 10.1038/srep24229
136. Wu W, Hu Z, Qin Y, Dong J, Dai J, Lu C, et al. Seminal plasma microRNAs: Potential biomarkers for spermatogenesis status. Mol Hum Reprod (2012) 18(10):489–97. doi: 10.1093/molehr/gas022
137. Zhi E-L, Liang G-Q, Li P, Chen H-X, Tian R-H, Xu P, et al. Seminal plasma miR-192a: a biomarker predicting successful resolution of nonobstructive azoospermia following varicocele repair. AJA (2018) 20(4):396. doi: 10.4103/aja.aja_8_18
138. Agarwal A, Saleh RA, Bedaiwy MA. Role of reactive oxygen species in the pathophysiology of human reproduction. Fertil Steril (2003) 79(4):829–43. doi: 10.1016/S0015-0282(02)04948-8
139. Aitken RJ. Reactive oxygen species as mediators of sperm capacitation and pathological damage. Mol Reprod Dev (2017) 84(10):1039–52. doi: 10.1002/mrd.22871
140. Bui A, Sharma R, Henkel R, Agarwal A. Reactive oxygen species impact on sperm DNA and its role in male infertility. Andrologia (2018) 50(8):e13012. doi: 10.1111/and.13012
141. Sinha K, Das J, Pal PB, Sil PC. Oxidative stress: the mitochondria-dependent and mitochondria-independent pathways of apoptosis. Arch Toxicol (2013) 87(7):1157–80. doi: 10.1007/s00204-013-1034-4
142. Vessey W, Perez-Miranda A, Macfarquhar R, Agarwal A, Homa S. Reactive oxygen species in human semen: validation and qualification of a chemiluminescence assay. Fertil Steril (2014) 102(6):1576–83.e4. doi: 10.1016/j.fertnstert.2014.09.009
143. Roychoudhury S, Sharma R, Sikka S, Agarwal A. Diagnostic application of total antioxidant capacity in seminal plasma to assess oxidative stress in male factor infertility. J Assist Reprod Genet (2016) 33(5):627–35. doi: 10.1007/s10815-016-0677-5
144. Gomes E, Irvine D, Aitken R. Evaluation of a spectrophotometric assay for the measurement of malondialdehyde and 4-hydroxyalkenals in human spermatozoa: Relashionships with semen quality and sperm function. Int J Androl sL. (1998) 21:81–94. doi: 10.1046/j.1365-2605.1998.00106.x
145. Cambi M, Tamburrino L, Marchiani S, Olivito B, Azzari C, Forti G, et al. Development of a specific method to evaluate 8-hydroxy, 2-deoxyguanosine in sperm nuclei: relationship with semen quality in a cohort of 94 subjects. Reproduction (2013) 145(3):227–35. doi: 10.1530/REP-12-0404
146. Aitken RJ, De Iuliis GN, Finnie JM, Hedges A, McLachlan RI. Analysis of the relationships between oxidative stress, DNA damage and sperm vitality in a patient population: Development of diagnostic criteria. Hum Reprod (2010) 25(10):2415–26. doi: 10.1093/humrep/deq214
147. Aktan G, Doğru-Abbasoğlu S, Küçükgergin C, Kadıoğlu A, Özdemirler-Erata G, Koçak-Toker N. Mystery of idiopathic male infertility: Is oxidative stress an actual risk? Fertil Steril (2013) 99(5):1211–5. doi: 10.1016/j.fertnstert.2012.11.045
148. Homa ST, Vassiliou AM, Stone J, Killeen AP, Dawkins A, Xie J, et al. A comparison between two assays for measuring seminal oxidative stress and their relationship with sperm DNA fragmentation and semen parameters. Genes (2019) 10(3):236. doi: 10.3390/genes10030236
149. Sakkas D, Alvarez JG. Sperm DNA fragmentation: Mechanisms of origin, impact on reproductive outcome, and analysis. Fertil Steril (2010) 93(4):1027–36. doi: 10.1016/j.fertnstert.2009.10.046
150. Kamkar N, Ramezanali F, Sabbaghian M. The relationship between sperm DNA fragmentation, free radicals and antioxidant capacity with idiopathic repeated pregnancy loss. Reprod Biol (2018) 18(4):330–5. doi: 10.1016/j.repbio.2018.11.002
151. Deng C, Li T, Xie Y, Guo Y, Qy Y, Liang X, et al. Sperm DNA fragmentation index influences assisted reproductive technology outcome: A systematic review and meta-analysis combined with a retrospective cohort study. Andrologia (2019) 51(6):e13263. doi: 10.1111/and.13263
152. Majzoub A, Agarwal A. Systematic review of antioxidant types and doses in male infertility: Benefits on semen parameters, advanced sperm function, assisted reproduction and live-birth rate. Arab J Urol (2018) 16(1):113–24. doi: 10.1016/j.aju.2017.11.013
153. Agarwal A, Sharma R, Roychoudhury S, Du Plessis S, Sabanegh E. MiOXSYS: a novel method of measuring oxidation reduction potential in semen and seminal plasma. Fertil Steril (2016) 106(3):566–73.e10. doi: 10.1016/j.fertnstert.2016.05.013
154. Bauer NC, Corbett AH, Doetsch PW. The current state of eukaryotic DNA base damage and repair. Nucleic Acids Res (2015) 43(21):10083–101. doi: 10.1093/nar/gkv1136
155. Smith TB, Dun MD, Smith ND, Curry BJ, Connaughton HS, Aitken RJ. The presence of a truncated base excision repair pathway in human spermatozoa that is mediated by OGG1. JCS (2013) 126(6):1488–97. doi: 10.1242/jcs.121657
156. Ohno M, Sakumi K, Fukumura R, Furuichi M, Iwasaki Y, Hokama M, et al. 8-oxoguanine causes spontaneous de novo germline mutations in mice. Sci Rep (2014) 4(1):1–9. doi: 10.1038/srep04689
157. Kumar N, Singh AK. Trends of male factor infertility, an important cause of infertility: A review of literature. J Hum Reprod Sci (2015) 8(4):191. doi: 10.4103/0974-1208.170370
158. Medenica S, Zivanovic D, Batkoska L, Marinelli S, Basile G, Perino A, et al. The future is coming: Artificial intelligence in the treatment of infertility could improve assisted reproduction outcomes–the value of regulatory frameworks. Diagnostics (2022) 12(12):2979. doi: 10.3390/diagnostics12122979
Keywords: male infertility, ROS, oxidative stress, semen quality, steroidogenesis
Citation: Hussain T, Kandeel M, Metwally E, Murtaza G, Kalhoro DH, Yin Y, Tan B, Chughtai MI, Yaseen A, Afzal A and Kalhoro MS (2023) Unraveling the harmful effect of oxidative stress on male fertility: A mechanistic insight. Front. Endocrinol. 14:1070692. doi: 10.3389/fendo.2023.1070692
Received: 15 October 2022; Accepted: 02 January 2023;
Published: 13 February 2023.
Edited by:
Sara Marchiani, University of Florence, ItalyReviewed by:
Marianna Santonastaso, Università degli Studi della Campania “Luigi Vanvitelli”, ItalyZia Ur Rehman, University of Agriculture, Peshawar, Pakistan
Hao Xiao, Guangdong Academy of Agricultural Sciences, China
Recep Hakki Koca, Bingöl University, Turkey
Copyright © 2023 Hussain, Kandeel, Metwally, Murtaza, Kalhoro, Yin, Tan, Chughtai, Yaseen, Afzal and Kalhoro. This is an open-access article distributed under the terms of the Creative Commons Attribution License (CC BY). The use, distribution or reproduction in other forums is permitted, provided the original author(s) and the copyright owner(s) are credited and that the original publication in this journal is cited, in accordance with accepted academic practice. No use, distribution or reproduction is permitted which does not comply with these terms.
*Correspondence: Tarique Hussain, ZHJ0YXJpcXVlcmFob29AZ21haWwuY29t; Bie Tan, YmlldGFuQGh1bmF1LmVkdS5jbg==