- 1Department of Pediatrics, Seoul National University Children’s Hospital, Seoul, Republic of Korea
- 2Department of Pediatrics, Seoul National University College of Medicine, Seoul, Republic of Korea
- 3Department of Internal Medicine, Seoul National University Hospital, Seoul, Republic of Korea
- 4Department of Internal Medicine, Seoul National University Hospital College of Medicine, Seoul, Republic of Korea
- 5Section of Environmental Health, Department of Public Health, University of Copenhagen, Copenhagen, Denmark
- 6Institute of Environmental Medicine, Seoul National University Medical Research Center, Seoul, Republic of Korea
- 7Environmental Health Center, Seoul National University College of Medicine, Seoul, Republic of Korea
- 8Division of Children and Adolescent Psychiatry, Department of Psychiatry, Seoul National University Hospital, Seoul, Republic of Korea
- 9Department of Psychiatry, Hanyang University Medical Center, Seoul, Republic of Korea
- 10Department of Preventive Medicine, Seoul National University College of Medicine, Seoul, Republic of Korea
Background: Adequate iodine intake is essential for growing children, as both deficient and excessive iodine status can result in thyroid dysfunction. We investigated the iodine status and its association with thyroid function in 6-year-old children from South Korea.
Methods: A total of 439 children aged 6 (231 boys and 208 girls) were investigated from the Environment and Development of Children cohort study. The thyroid function test included free thyroxine (FT4), total triiodothyronine (T3), and thyroid-stimulating hormone (TSH). Urine iodine status was evaluated using urine iodine concentration (UIC) in morning spot urine and categorized into iodine deficient (< 100 μg/L), adequate (100–199 μg/L), more than adequate (200–299 μg/L), mild excessive (300–999 μg/L), and severe excessive (≥ 1000 μg/L) groups. The estimated 24-hour urinary iodine excretion (24h-UIE) was also calculated.
Results: The median TSH level was 2.3 μIU/mL, with subclinical hypothyroidism detected in 4.3% of patients without sex differences. The median UIC was 606.2 μg/L, with higher levels in boys (684 μg/L vs. 545 μg/L, p = 0.021) than girls. Iodine status was categorized as deficient (n = 19, 4.3%), adequate (n = 42, 9.6%), more than adequate (n = 54, 12.3%), mild excessive (n = 170, 38.7%), or severe excessive (n = 154, 35.1%). After adjusting for age, sex, birth weight, gestational age, body mass index z-score, and family history, both the mild and severe excess groups showed lower FT4 (β = − 0.04, p = 0.032 for mild excess; β = − 0.04, p = 0.042 for severe excess) and T3 levels (β = − 8.12, p = 0.009 for mild excess; β = − 9.08, p = 0.004 for severe excess) compared to the adequate group. Log-transformed estimated 24h-UIE showed a positive association with log-transformed TSH levels (β = 0.04, p = 0.046).
Conclusion: Excess iodine was prevalent (73.8%) in 6-year-old Korean children. Excess iodine was associated with a decrease in FT4 or T3 levels and an increase in TSH levels. The longitudinal effects of iodine excess on later thyroid function and health outcomes require further investigation.
1 Introduction
Iodine is an essential micronutrient for the synthesis of thyroid hormone synthesis (1). Both iodine deficiency and excess can result in thyroid dysfunction, with a U-shaped association between iodine exposure and the risk of thyroid disease (2). Considering the critical role of thyroid function in childhood growth and development, children need an optimal iodine intake. The global health program has focused on intervention for iodine deficiency through salt iodization (3). However, with effective iodine fortification, concerns about iodine excess and its health impact have also been raised (4, 5). Iodine excess-induced hypothyroidism, resulting from failure to escape from the acute Wolff-Chaikoff effect in susceptible individuals has been reported (5) in addition to iodine-induced hyperthyroidism after iodine supplementation in iodine-deficient areas (6).
The association between iodine excess and thyroid dysfunction has been studied in the pediatric population (7–9), with some supporting results showing elevated thyroid stimulating hormone (TSH) levels in children exposed to iodine excess (8, 9). South Korea is an iodine-replete area (10) characterized by high seaweed consumption (11). According to the 2013–2015 Korean National Health and Nutrition Examination Survey, a high prevalence (64.9%) of iodine excess has been reported in Korean children and adolescents (8, 12).
In this study, we aimed to investigate the iodine status, using urine iodine measurements, and its association with thyroid function in 6-year-old Korean children.
2 Methods
2.1 Study population
This study was performed as a part of the Environment and Development of Children cohort study investigating the effects of early-life environmental risk factors on physical and neurobehavioral development (13). Among the 574 children who were examined at 6-years of age during 2015–2017 study period, 477 were evaluated for thyroid function and iodine status. Thirty-six children with multiple births and two diagnosed with thyroid disease (congenital hypothyroidism and Hashimoto’s thyroiditis, respectively) were excluded. Finally, 439 children were included in this study (Supplementary Figure 1). Informed consent was waived in accordance with the institutional review board of Seoul National University Hospital (IRB no. 1704-118-848).
2.2 Anthropometric assessments and questionnaires
Height, weight, and BMI z-scores were calculated using the 2007 Korean National Growth Charts, with overweight or obesity was defined as a body mass index (BMI) above the 85th percentile (14). Data on personal and parental medical history, socioeconomic status (monthly household income and parental educational status), dietary supplement intake, and dairy product consumption were collected using structured questionnaires.
2.3 Evaluation of iodine status
Single spot urine samples were collected in the morning, after 8-hour of fasting, to measure the levels of UIC (μg/L) and urinary creatinine (Cr, mg/dL). UIC was measured by inductively coupled plasma mass spectrometry (ICP-MS), using a 7900 ICP-MS apparatus (Agilent Technologies, Santa Clara, CA, USA), and urinary Cr levels were measured by the kinetic alkaline picrate method (Architect TBA-C16000, Abbott, Abbott Park, IL, USA). The range of intra- and inter-assay coefficients of variation (CV) for UIC was 1.6-2.0% and 1.6-1.9%, respectively. The iodine status of the participants was assessed using the UIC (μg/L), iodine/Cr ratio (μg/g), and estimated 24-hour urine iodine excretion (24 h-UIE, μg/day). Children were categorized into four iodine status groups using the UIC, based on World Health Organization (WHO) recommendations: iodine deficiency (UIC < 100 μg/L), adequate (UIC 100–199 μg/L), more than adequate (UIC 200–299 μg/L), and excessive (UIC ≥ 300 μg/L) (3). The excessive group was further divided into the mild excessive (UIC 300–999 μg/L) and severe excessive (UIC ≥ 1000 μg/L) groups. The estimated 24 h-UIE (μg/day) was calculated from the iodine/Cr ratio using the following equation: estimated 24 h-UIE (μg/day) = iodine/Cr (μg/g) × predicted 24 h-Cr excretion (g/day). The predicted 24 h-Cr excretion (g/day) was determined using age- and anthropometry-based reference values (15).
2.4 Measurement of thyroid function
Thyroid function tests, including assays for free thyroxine (FT4), total triiodothyronine (T3), and TSH, were performed using a chemiluminescent microparticle immune assay on an ARCHITECT i2000 System (Abbott Korea, Seoul, Korea). The normal range was defined as 0.70–1.48 ng/dL (9.01–19.05 pmol/L) for FT4, 58–159 ng/dL (0.89–2.44 nmol/L) for T3, and 0.38–4.94 μIU/mL for TSH, respectively. The range of CVs for FT4, T3, and TSH was 2.0-2.6%, 3.0-4.4%, and 2.6-2.9%, respectively. Subclinical hypothyroidism was defined as TSH levels between 4.94–10 μIU/mL with normal FT4 levels.
2.5 Statistical analysis
All continuous variables were tested for normality and expressed as mean ± standard deviation or median with interquartile range (IQR). Iodine parameters and TSH levels were log-transformed for analysis. The participants’ characteristics were analyzed using the Student’s t-test or Mann–Whitney U-test for continuous variables, and the chi-squared test was used for categorical variables. Clinical characteristics and thyroid function were compared among the four iodine status groups (each group vs. the iodine-adequate group as a reference group), and a Bonferroni-corrected p-value of 0.017 was applied when performing multiple comparisons. The linear trend in thyroid function in the UIC categories was evaluated. We used generalized additive models (GAMs) to test the nonlinear relationship between iodine measurements and thyroid hormone levels. Linear and logistic regression analyses were used to evaluate the relationship between iodine status, thyroid hormone levels, and subclinical hypothyroidism. A directed acyclic graph (DAG) was used to visualize the causal relationships among variables and to identify potential confounding variables (Supplementary Figure 2) (16). The following variables were included in the multivariate model: age, sex, gestational age, birth weight, BMI z-scores, and parental history of thyroid disease. The R Statistical Software package (version 3.6.0; R Foundation for Statistical Computing, Vienna, Austria) was used for the statistical analyses, and the statistical significance was determined as p < 0.05.
3 Results
3.1 Clinical characteristics
Table 1 shows the clinical characteristics of 439 children (231 boys) included in the analysis. The mean age was 5.9 ± 0.1 years and the mean BMI z-score was -0.1 ± 1.0. Twenty-one patients (4.8%) had a history of thyroid disease. Most parents (82.9% of mother and 85.2% of father) had high educational levels. The mean FT4 and T3 levels were 1.16 ± 0.11 ng/dL and 148.1 ± 18.5 ng/dL, respectively. The median TSH level was 2.29 μIU/mL (range, 0.53–8.59 μIU/mL). None of the patients showed overt hypothyroidism and 19 (4.3%) had subclinical hypothyroidism. No sex-related differences in thyroid function were observed. All children with subclinical hypothyroidism had a normal stature, with three children (15.8%) being overweight and two (10.5%) obese. None of these children showed clinical signs of hypothyroidism.
3.2 Comparison among iodine status categories
The median UIC was 606.2 μg/L (IQR, 292.4–1446.4 μg/L), with a between-sex difference (683.6 μg/L in boys vs. 554.8 μg/L in girls, p = 0.021, Table 1). The distribution of iodine status categories was as follows: iodine deficient (n = 19, 4.3%), adequate (n = 42, 9.6%), more than adequate (n = 54, 12.3%), or excessive (n = 324, 73.8%). The median iodine/Cr and estimated 24 h-UIE levels were 826.1 μg/g and 313.4 μg/day, respectively, with no between-sex difference. There were no differences in clinical characteristics among the four iodine categories (Supplementary Table 1). In addition, dietary supplement intake or proportion of high dairy product consumption (≥ 2 cups/day) did not differ among the four groups.
As shown in Table 2, we compared the three iodine status categories (iodine deficient, more than adequate, and excessive groups) with the reference category (iodine adequate group). The iodine excessive group showed lower T3 levels (147.2 vs. 155.1 mg/dL, p = 0.010), as revealed by the Bonferroni correction (p = 0.017). Interestingly, 17 (89.5%) of the 19 children with subclinical hypothyroidism had excessive iodine. However, FT4 and log-transformed TSH (log-TSH) levels and the risk of subclinical hypothyroidism did not differ among the four iodine categories.
3.3 Relationship between iodine status and thyroid function
Due to the high prevalence of iodine excess, iodine status was classified into five categories [deficient, adequate, more than adequate, mild excessive (UIC of 300–999 μg/L, n = 170, 38.7%), and severe excessive (UIC ≥ 1000 μg/L, n = 154, 35.1%)]. The results of the univariate analysis are described in Supplementary Tables 2, 3. After adjusting for age, sex, gestational age, birth weight, BMI z-scores, and parental history of thyroid disease, a multivariate-adjusted model was constructed to compare thyroid function among the five iodine categories. Compared to the reference category (iodine adequate group), the more than adequate group showed lower T3 levels (β = –8.62, p = 0.021, Figure 1B) and log-TSH levels (β = –0.19, p = 0.035, Figure 1C), and both mild and severe excessive groups showed lower FT4 (β = –0.04, p = 0.032; mild excessive group: β = –0.04, p = 0.042 for the severe excessive group; Figure 1A) and T3 levels (β = –8.12, p = 0.009, for the mild excessive group; β = –9.08, p = 0.004 for the severe excessive group; Figure 1B). In a sex-stratified analysis, these associations for iodine excess were significant in girls but not in boys (Figure 1; Table 3). In girls, FT4 and T3 levels were lower in the iodine-deficient group than in the iodine-adequate group with marginal significance. Due to the small number of children with adequate and more than adequate group, we performed a sensitivity analysis by combining the two groups as a reference category. The results showed the similar trends of lower FT4 and T3 and higher TSH levels among the iodine excess group compared to reference group (Supplementary Table 4).
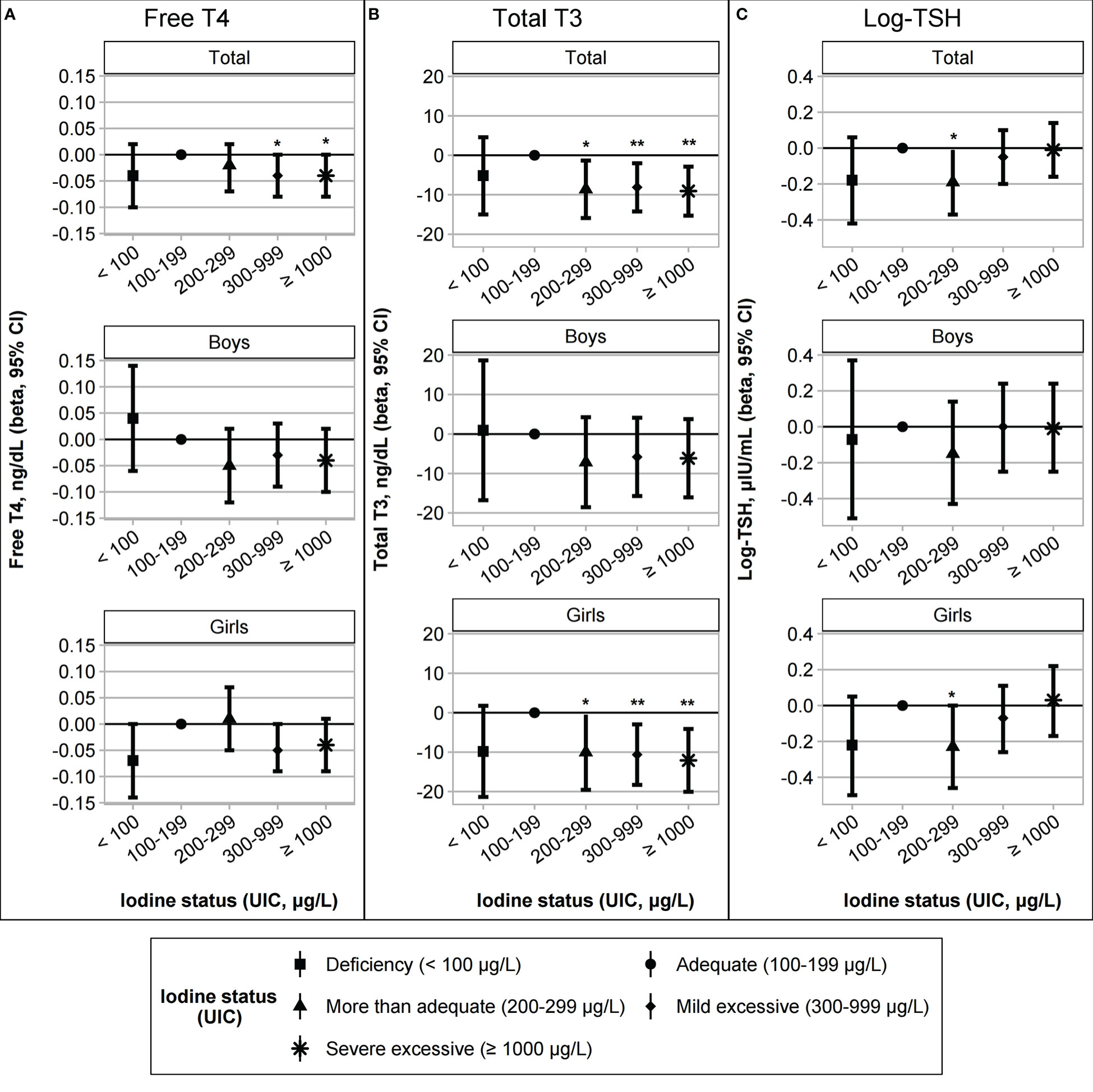
Figure 1 Association between iodine status and thyroid function (A) free T4, (B) total T3, and (C) log-transformed TSH levels. The iodine status groups were categorized as deficient (UIC < 100 μg/L, n = 19), adequate (UIC 100–199 μg/L, n = 42), more than adequate (UIC 200–299 μg/L, n = 54), mild excessive (UIC 300–999 μg/L, n = 170), or severe excessive (UIC ≥ 1000 μg/L, n = 154). Regression models for the total group were adjusted for age, sex, gestational age, birth weight, body mass index z-scores, and a parental history of thyroid disease. The sex-stratified models were adjusted for age, gestational age, birth weight, body mass index z-scores, and parental history of thyroid disease. *p < 0.05; **p < 0.01.
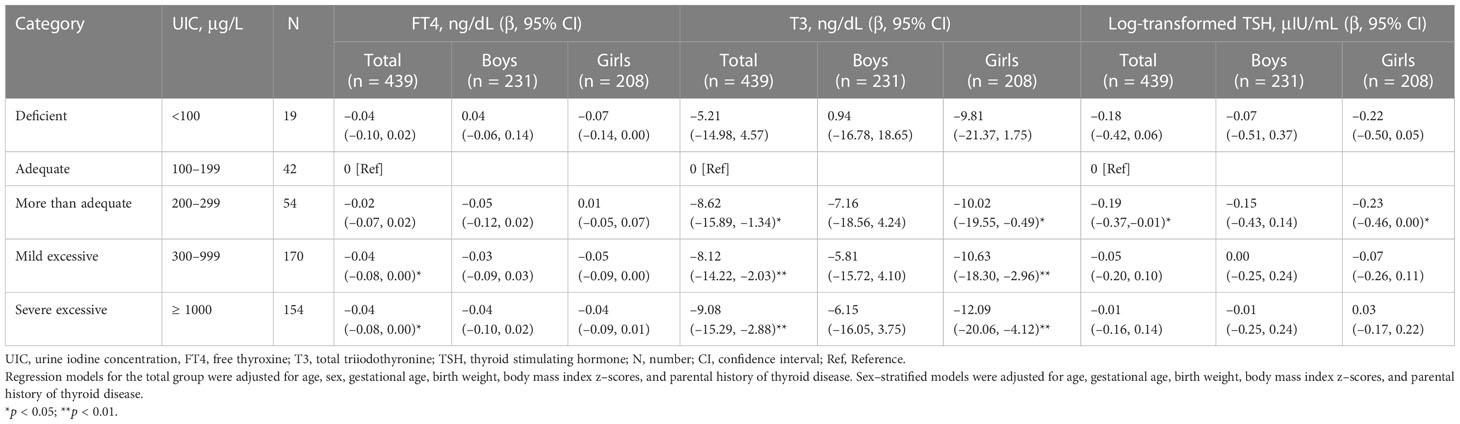
Table 3 Association between the iodine status and thyroid hormone levels after adjusting for covariates (multivariate models).
We investigated the nonlinear relationship between iodine measurements and thyroid hormone levels using GAM but did not reveal clear nonlinear relationships (Supplementary Figure 3). To focus on the association between iodine excess and thyroid function, we analyzed iodine-sufficient or-excessive children (n = 420) after excluding iodine-deficient children (n = 19). Results of the univariate analysis are reported in Supplementary Table 5, showing positive associations between continuous iodine variables and log-TSH levels in girls. After adjusting for covariates, log-transformed iodine/Cr (log-iodine/Cr) and log-transformed estimated 24 h-UIE (log-estimated 24 h-UIE) levels were significantly associated with log-TSH levels (β = 0.05, p = 0.035 for log-iodine/Cr; β = 0.04, p = 0.046 for log-estimated 24 h-UIE, Table 4). In a sex-stratified analysis, a positive relationship between log-iodine/Cr and log-estimated 24 h-UIE and log-TSH levels was found only in girls (p < 0.05, Table 4) but not in boys. FT4 and T3 levels were not associated with log-transformed UIC, iodine/Cr, nor estimated 24 h-UIE levels in either sex. The risk of subclinical hypothyroidism was not associated with continuous iodine variables (Supplementary Table 6).
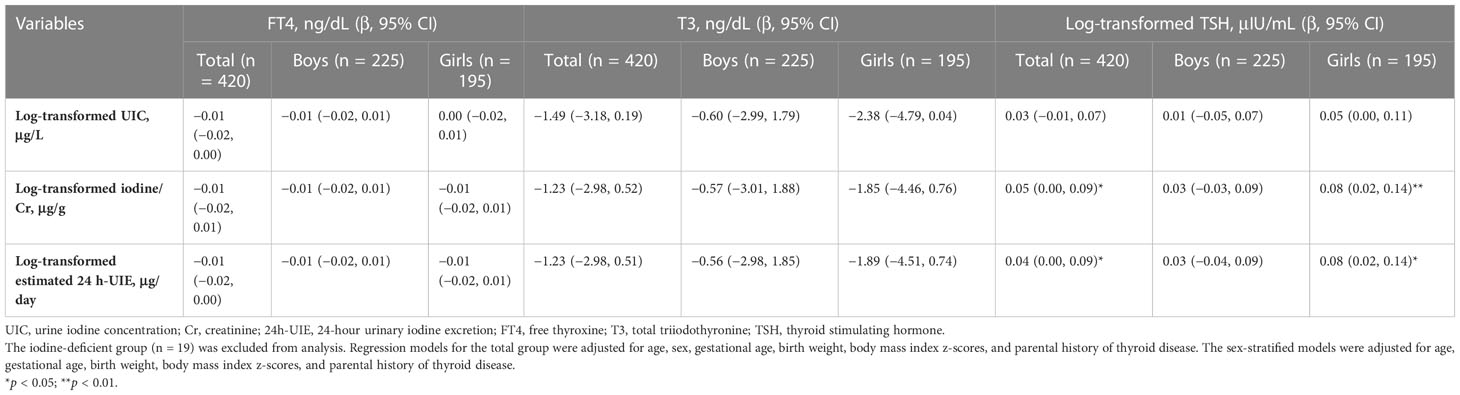
Table 4 Association between continuous iodine variables and thyroid hormone levels after adjusting for covariates.
4 Discussion
Among 6-year-old children living in South Korea, iodine excess was prevalent (73.8%), with a median UIC level of 606 μg/L. Only 9.6% of the children showed adequate iodine status, with a low frequency (4.3%) of iodine deficiency. Compared to the iodine adequate group, the iodine excessive group exhibited lower FT4 and T3 levels, and the log-estimated 24 h-UIE showed a positive association with log-TSH levels. In a sex-stratified analysis, this trend was only significant in girls. Although iodine excess was reported to be prevalent in Korean children, 6 to 19 years of age, this is the first study showing a decreasing trend of FT4 and T3 levels within the normal range in the iodine excess group and an increasing trend of log-TSH levels, with higher urine iodine excretion in Korean prepubertal children.
The high levels of the median UIC (606 μg/L (IQR, 292.4–1466.4]) and the high prevalence of iodine excess (73.8%) in 6-year-old children were comparable to previous pediatric reports in children 6 to 19 years of age (median UIC of 449 μg/L and 64.9% of iodine excess) (8). This was higher than the UIC of 274 ug/L in the adult population, according to the same database from the Korean National Health and Nutrition Examination Survey from 2013–2015 (17). Excessive iodine status has been reported in Korean children, ranging from 54.9% to 76.7% prevalence of iodine excess and 328 to 458 μg/L median UIC (18–20). Common dietary sources of iodine in Korea include seaweeds, such as sea mustard, laver, kelp, as well as pickled vegetables, milk, and dairy products (11). However, there were no significant differences in dairy product consumption among iodine status groups in this study. Higher UIC levels in boys than in girls may result from higher consumption of iodine-rich food and higher energy intake in boys (21). Considering the absence of sex differences in Cr-adjusted iodine variables, sex differences in UIC may be due to differences in urine volume or hydration status (12, 17).
The decreasing trend in FT4 and T3 levels with increasing TSH levels in the iodine excess group suggests a possible risk for iodine excess-associated hypothyroidism. Although the prevalence of subclinical hypothyroidism was not significantly different among the iodine groups (2.4% in the adequate and 5.3% in the excess group), 89.5% (17 of 19 children) of children with subclinical hypothyroidism had an iodine excess. Several pediatric studies have supported the effect of iodine excess on a shift in FT4 or T3 to lower levels (8), TSH towards higher levels (8, 9, 22), and an increased risk of subclinical hypothyroidism (23). However, some studies have reported no significant associations (7, 24). The prevalence of subclinical hypothyroidism among iodine groups also varied according to the geographic region and age group. A previous nationwide Korean study showed significantly different frequencies of subclinical hypothyroidism: 3.9% in the iodine adequate and 6.6% in the excess group (8). A Chinese study also reported similar results, with a higher prevalence of subclinical hypothyroidism in high iodine area (6.7%, median UIC of 1030 μg/L) than in adequate iodine area (0.7%, median UIC of 123 μg/L) (23). However, an international study performed in 12 countries showed similar rates of subclinical hypothyroidism in the iodine adequate (0.5%) and excess (0.6%) group (9). A meta-analysis found that chronic exposure to excess iodine increased the odds ratio for subclinical and overt hypothyroidism in adults but not children, possibly due to the high heterogeneity of the included pediatric studies (4). Previous pediatric studies were limited by reporting the crude differences in thyroid hormone levels among UIC groups (8, 9, 22, 24). Only a few pediatric studies have performed the adjusted relationship between iodine status and thyroid function (7, 23). The use of multivariate-adjusted regression models strengthened the findings in our study.
We found lower FT4 levels in girls in the iodine-deficient group than in the adequate group. However, our results were limited by the small number of participants in iodine-deficient groups. Iodine deficiency is associated with hypothyroidism and goiter in children (25). Thus, both iodine deficiency and excess can lead to impaired thyroid hormone production, suggesting a U-shaped correlation (2). A recent nationwide study in Korean children and adolescents (10–19 years of age) also showed a U-shape and an inverted U-shape correlation between serum TSH and FT4 levels and UIC, respectively (8).
The mechanisms underlying iodine-induced thyroid dysfunction include failure to escape Wolff-Chaikoff effects or iodine-induced thyroid autoimmunity (5), although there is a possibility of additional mechanisms. Acute iodine excess can cause a transient decrease in thyroid hormone production by inhibiting thyroid peroxidase activity or intrathyroidal deiodinase activity, called the Wolff-Chaikoff effect (5). Healthy individuals can maintain normal thyroid function by escaping the Wolff-Chaikoff effect within several days, through a decrease in sodium iodine symporter expression. However, failure of this adaptation can lead to iodine-induced hypothyroidism in susceptible populations, such as infants, the elderly, or those with thyroid diseases (5, 26, 27). In addition, high iodine levels may induce thyroid autoimmunity, leading to elevated TSH levels and hypothyroidism (26). As this study included healthy children, iodine-induced thyroid dysfunction was not definite in our study population. However, a decreasing trend in thyroid hormone levels was observed in the iodine-excessive group.
Only girls showed a significant association between iodine status and thyroid function in this study. A Korean adult study reported that the correlation between iodine status and the thyroid disease was only significant in women, suggesting that women may be more susceptible to excessive iodine exposure (28). Estrogen, directly and indirectly, affects thyroid gland proliferation and function, including iodine uptake through the sodium-iodine symporter and thyroid peroxidase activity (29). However, because this study included prepubertal children, the mechanisms underlying sex differences remain unclear.
In this study, a higher BMI z-score and lower gestational age were associated with higher serum T3 levels in our sample of 6-year-old children. With regard to childhood obesity, the positive relationship between BMI and T3 levels can be explained by an adaptation process to increase resting energy expenditure or elevated deiodinase activity (30–32). The relationship between prematurity and childhood thyroid function has previously been reported, showing trends towards decrease in free T4 and an increase in T3 and TSH levels (33, 34), although the underlying mechanisms remains to be determined.
We used UIC, iodine/Cr ratio, and the estimated 24h-UIE as iodine variables in this study. Although measurement of 24h-UIE is the most reliable method to evaluate iodine status, it is difficult to apply in field studies (35, 36). UIC from spot urine has been the most widely used biomarker for iodine status in populations as >90% of dietary iodine is excreted by urine, although UIC is influenced by urine volume and hydration status (35). UIC related to urinary Cr has been used to overcome this limitation to determine iodine status. In children, anthropometry-based 24-h urinary Cr reference values can be used to estimate the 24h-UIE from spot urine (15). Several pediatric studies have reported that the estimated 24h-UIE better reflects measured 24h-UIE than UIC, providing a valid and reliable alternative to measured 24h-UIE (37, 38). In this study, we used the estimated 24h-UIE to complement UIC and identified a significant positive association between the estimated 24h-UIE and TSH levels.
The limitations of our study need to be acknowledged in the interpretation of findings to practice. Foremost is the cross-sectional design and relatively small sample size, including the small proportion of participants in the non-iodine excess group, which did not allow us to evaluate the relationship between iodine deficiency and thyroid function. Further studies using a prospective design and focusing on environmental sources of iodine excess are needed to generalize the adverse effects of iodine excess on health outcomes and to suggest preventive strategies for iodine excess. Second, the single-spot urine collection used in this study to assess UIC for iodine status could not reflect within-day and day-to-day variations in urine iodine excretion. Although we could not collect 24 h urine samples or repeatedly collect spot urine samples, an ideal way to provide a valid estimate of iodine status (39), spot urine samples collected in the morning after overnight fasting can eliminate within-day variations. In addition, we obtained similar results regardless of which iodine variables (UIC or urinary Cr-adjusted iodine status) used to examine the association between iodine status and thyroid function (35). Third, we could not evaluate thyroid autoantibodies in all study populations. However, thyroid autoantibodies were not detected in our children with subclinical hypothyroidism. Considering the low prevalence of positive thyroid autoantibodies (2.3%) in Korean children and adolescents (8), the modifying effect of thyroid autoimmunity is insignificant in our 6-year-old children. Fourth, potential confounders, such as exposure to endocrine disrupting chemicals (EDCs) or heavy metals that can affect thyroid dysfunction, were not considered in this study. The mixed effects of various thyroid disrupting chemicals, including both iodine and other EDCs, need further investigation.
In conclusion, iodine was deficient in 4.3%, adequate in 21.9%, and excessive in 73.8% of 6-year-old children living in South Korea during 2015–2017. Furthermore, excess iodine was associated with decreased FT4 or T3 levels and increased TSH levels, particularly in girls. The long-term health effects of iodine excess remain to be determined, considering the high prevalence of iodine excess in Korean children. Further studies to determine the optimal iodine intake are required.
Data availability statement
The datasets presented in this article are not readily available because the fundamental results of the study are reported in the paper and supporting information. The raw data cannot be shared publicly due to ethical restrictions because they contain potentially sensitive information. The data are available upon reasonable request from the Scientific Research Committee. Requests to access the datasets should be directed to YJL, yjlee103@naver.com.
Ethics statement
The studies involving human participants were reviewed and approved by Institutional Review Board of Seoul National University Hospital (IRB no. 1704-118-848). Written informed consent from the participants’ legal guardian/next of kin was not required to participate in this study in accordance with the national legislation and the institutional requirements.
Author contributions
YJL carried out the initial analyses, drafted the initial manuscript, and reviewed and revised the manuscript. SWC, Y-HL, B-NK, JIK, Y-CH, YJP, and CHS conceptualized and designed the study, coordinated and supervised data collection, and critically reviewed the manuscript for important intellectual content. YAL conceptualized and designed the study, collected data, and reviewed and revised the manuscript. All authors contributed to the article and approved the submitted version.
Funding
The EDC study was initially supported by grants from the Environmental Health Center funded by the Korean Ministry of Environment, a grant from the Ministry of Food and Drug Safety in 2018 (18162MFDS121), and the Center for Environmental Health through the Ministry of Environment. This study was supported by a National Research Foundation of Korea (NRF) grant funded by the Ministry of Education, Science, and Technology (MEST) of the Korean government (No. 2021R1A2C1011241). This research was supported by the Research Program 2017, funded by the Seoul National University College of Medicine Research Foundation (No. 800-20170140). This study was supported by a grant from the SNUH Research Fund (no. 04-2016-3020).
Acknowledgments
The authors thank Kyung-shin Lee, Jin-A Park, Ji-Young Lee, and Yumi Choi for their assistance with data collection. The Biospecimens and data used in this study were provided by the Biobank of Seoul National Univrsity Hospital, a member of the Korea Biobank Network.
Conflict of interest
The authors declare that the research was conducted in the absence of any commercial or financial relationships that could be construed as a potential conflict of interest.
Publisher’s note
All claims expressed in this article are solely those of the authors and do not necessarily represent those of their affiliated organizations, or those of the publisher, the editors and the reviewers. Any product that may be evaluated in this article, or claim that may be made by its manufacturer, is not guaranteed or endorsed by the publisher.
Supplementary material
The Supplementary Material for this article can be found online at: https://www.frontiersin.org/articles/10.3389/fendo.2023.1099824/full#supplementary-material
References
1. Chung HR. Iodine and thyroid function. Ann Pediatr Endocrinol Metab (2014) 19(1):8–12. doi: 10.6065/apem.2014.19.1.8
2. Laurberg P, Pedersen IB, Carlé A, Knudsen N, Andersen S, Ovesen L, et al. The U-shaped curve of iodine intake and thyroid disorders. In: Preedy VR, Burrow GN, Watson R, editors. Comprehensive handbook of iodine. San Diego: Elsevier (2009). p. 449–55.
3. World Health Organization. Assessment of iodine deficiency disorders and monitoring their elimination: a guide for programme managers ([amp]]lrm;2007). World Health Organization. Available at: https://apps.who.int/iris/handle/10665/43781 (Accessed May 15, 2022).
4. Katagiri R, Yuan X, Kobayashi S, Sasaki S. Effect of excess iodine intake on thyroid diseases in different populations: A systematic review and meta-analyses including observational studies. PloS One (2017) 12(3):e0173722. doi: 10.1371/journal.pone.0173722
5. Leung AM, Braverman LE. Consequences of excess iodine. Nat Rev Endocrinol (2014) 10(3):136–42. doi: 10.1038/nrendo.2013.251
6. Stanbury JB, Ermans AE, Bourdoux P, Todd C, Oken E, Tonglet R, et al. Iodine-induced hyperthyroidism: Occurrence and epidemiology. Thyroid (1998) 8(1):83–100. doi: 10.1089/thy.1998.8.83
7. Chen W, Zhang Y, Hao Y, Wang W, Tan L, Bian J, et al. Adverse effects on thyroid of Chinese children exposed to long-term iodine excess: Optimal and safe tolerable upper intake levels of iodine for 7- to 14-y-old children. Am J Clin Nutr (2018) 107(5):780–8. doi: 10.1093/ajcn/nqy011
8. Kang MJ, Hwang IT, Chung HR. Excessive iodine intake and subclinical hypothyroidism in children and adolescents aged 6-19 years: Results of the sixth Korean national health and nutrition examination survey, 2013-2015. Thyroid (2018) 28(6):773–9. doi: 10.1089/thy.2017.0507
9. Zimmermann MB, Aeberli I, Andersson M, Assey V, Yorg JA, Jooste P, et al. Thyroglobulin is a sensitive measure of both deficient and excess iodine intakes in children and indicates no adverse effects on thyroid function in the UIC range of 100-299 mug/L: a UNICEF/ICCIDD study group report. J Clin Endocrinol Metab (2013) 98(3):1271–80. doi: 10.1210/jc.2012-3952
10. Iodine Global Network. Global scorecard of iodine nutrition in 2017 in the general population and in pregnant women (PW) (2017). Iodine Global Network. Available at: https://www.ign.org/cm_data/IGN_Global_Scorecard_AllPop_and_PW_May2017.pdf (Accessed May 15, 2022).
11. Choi JY, Ju DL, Song YJ. Revision of an iodine database for Korean foods and evaluation of dietary iodine and urinary iodine in Korean adults using 2013-2015 Korea national health and nutrition examination survey. J Nutr Health (2020) 53(3):271–87. doi: 10.4163/jnh.2020.53.3.271
12. Choi YC, Cheong JI, Chueh HW, Yoo JH. Iodine status and characteristics of Korean adolescents and their parents based on urinary iodine concentration: A nationwide cross-sectional study. Ann Pediatr Endocrinol Metab (2019) 24(2):108–15. doi: 10.6065/apem.2019.24.2.108
13. Kim KN, Lim YH, Shin CH, Lee YA, Kim BN, Kim JI, et al. Cohort profile: The environment and development of children (EDC) study: A prospective children’s cohort. Int J Epidemiol (2018) 47(4):1049–50f. doi: 10.1093/ije/dyy070
14. Moon JS, Lee SY, Nam CM, Choi JM, Choi BK, Seo JW, et al. 2007 Korean National growth chart: review of developmental process and an outlook. Korean J Pediatr (2008) 51(1):1–25. doi: 10.3345/kjp.2008.51.1.1
15. Remer T, Neubert A, Maser-Gluth C. Anthropometry-based reference values for 24-h urinary creatinine excretion during growth and their use in endocrine and nutritional research. Am J Clin Nutr (2002) 75(3):561–9. doi: 10.1093/ajcn/75.3.561
16. Textor J, van der Zander B, Gilthorpe MS, Liskiewicz M, Ellison GT. Robust causal inference using directed acyclic graphs: The r package ‘dagitty’. Int J Epidemiol (2016) 45(6):1887–94. doi: 10.1093/ije/dyw341
17. Kim HI, Oh HK, Park SY, Jang HW, Shin MH, Kim SW, et al. Urinary iodine concentration and thyroid hormones: Korea national health and nutrition examination survey 2013-2015. Eur J Nutr (2019) 58(1):233–40. doi: 10.1007/s00394-017-1587-8
18. Choi YS, Ock S, Kwon S, Jung SB, Seok KH, Kim YJ, et al. Excessive iodine status among school-age children in Korea: A first report. Endocrinol Metab (Seoul) (2017) 32(3):370–4. doi: 10.3803/EnM.2017.32.3.370
19. Lee J, Kim JH, Lee SY, Lee JH. Iodine status in Korean preschool children as determined by urinary iodine excretion. Eur J Nutr (2014) 53(2):683–8. doi: 10.1007/s00394-013-0558-y
20. Lee JH. Analysis of urine iodine concentration by inductively coupled plasma-mass spectrometry method in normally developed children aged less than 7 years in masan city (Korea). J Korean Child Neurol Soc (2011) 19(3):199–207.
21. Vila L, Donnay S, Arena J, Arrizabalaga JJ, Pineda J, Garcia-Fuentes E, et al. Iodine status and thyroid function among Spanish schoolchildren aged 6-7 years: the tirokid study. Br J Nutr (2016) 115(9):1623–31. doi: 10.1017/S0007114516000660
22. Medani AM, Elnour AA, Saeed AM. Excessive iodine intake, water chemicals and endemic goitre in a Sudanese coastal area. Public Health Nutr (2013) 16(9):1586–92. doi: 10.1017/S1368980012004685
23. Sang Z, Chen W, Shen J, Tan L, Zhao N, Liu H, et al. Long-term exposure to excessive iodine from water is associated with thyroid dysfunction in children. J Nutr (2013) 143(12):2038–43. doi: 10.3945/jn.113.179135
24. Nepal AK, Suwal R, Gautam S, Shah GS, Baral N, Andersson M, et al. Subclinical hypothyroidism and elevated thyroglobulin in infants with chronic excess iodine intake. Thyroid (2015) 25(7):851–9. doi: 10.1089/thy.2015.0153
25. Zimmermann MB, Boelaert K. Iodine deficiency and thyroid disorders. Lancet Diabetes Endocrinol (2015) 3(4):286–95. doi: 10.1016/s2213-8587(14)70225-6
26. Laurberg P, Cerqueira C, Ovesen L, Rasmussen LB, Perrild H, Andersen S, et al. Iodine intake as a determinant of thyroid disorders in populations. Best Pract Res Clin Endocrinol Metab (2010) 24(1):13–27. doi: 10.1016/j.beem.2009.08.013
27. Farebrother J, Zimmermann MB, Andersson M. Excess iodine intake: Sources, assessment, and effects on thyroid function. Ann N Y Acad Sci (2019) 1446(1):44–65. doi: 10.1111/nyas.14041
28. Kim S, Kwon YS, Kim JY, Hong KH, Park YK. Association between iodine nutrition status and thyroid disease-related hormone in Korean adults: Korean national health and nutrition examination survey VI (2013-2015). Nutrients (2019) 11(11):2757. doi: 10.3390/nu11112757
29. Santin AP, Furlanetto TW. Role of estrogen in thyroid function and growth regulation. J Thyroid Res (2011) 2011:875125. doi: 10.4061/2011/875125
30. Metwalley KA, Farghaly HS. Subclinical hypothyroidism in children: updates for pediatricians. Ann Pediatr Endocrinol Metab (2021) 26(2):80–5. doi: 10.6065/apem.2040242.121
31. Reinehr T. Thyroid function in the nutritionally obese child and adolescent. Curr Opin Pediatr (2011) 23(4):415–20. doi: 10.1097/MOP.0b013e328344c393
32. Marras V, Casini MR, Pilia S, Carta D, Civolani P, Porcu M, et al. Thyroid function in obese children and adolescents. Horm Res Paediatr (2010) 73(3):193–7. doi: 10.1159/000284361
33. Radetti G, Fanolla A, Pappalardo L, Gottardi E. Prematurity may be a risk factor for thyroid dysfunction in childhood. J Clin Endocrinol Metab (2007) 92(1):155–9. doi: 10.1210/jc.2006-1219
34. Posod A, Odri Komazec I, Pupp Peglow U, Meraner D, Griesmaier E, Kiechl-Kohlendorfer U. Former very preterm infants show alterations in thyroid function at a preschool age. BioMed Res Int (2017) 2017:3805370. doi: 10.1155/2017/3805370
35. Rohner F, Zimmermann M, Jooste P, Pandav C, Caldwell K, Raghavan R, et al. Biomarkers of nutrition for development–iodine review. J Nutr (2014) 144(8):1322S–42S. doi: 10.3945/jn.113.181974
36. Vejbjerg P, Knudsen N, Perrild H, Laurberg P, Andersen S, Rasmussen LB, et al. Estimation of iodine intake from various urinary iodine measurements in population studies. Thyroid (2009) 19(11):1281–6. doi: 10.1089/thy.2009.0094
37. Montenegro-Bethancourt G, Johner SA, Stehle P, Neubert A, Remer T. Iodine status assessment in children: Spot urine iodine concentration reasonably reflects true twenty-four-hour iodine excretion only when scaled to creatinine. Thyroid (2015) 25(6):688–97. doi: 10.1089/thy.2015.0006
38. Chen W, Li X, Guo X, Shen J, Tan L, Lin L, et al. Urinary iodine excretion (UIE) estimated by iodine/creatinine ratio from spot urine in Chinese school-age children. Clin Endocrinol (Oxf) (2017) 86(4):628–33. doi: 10.1111/cen.13282
Keywords: iodine, thyroid hormone, urine, thyroid function test, child, Republic of Korea
Citation: Lee YJ, Cho SW, Lim Y-H, Kim B-N, Kim JI, Hong Y-C, Park YJ, Shin CH and Lee YA (2023) Relationship of iodine excess with thyroid function in 6-year-old children living in an iodine-replete area. Front. Endocrinol. 14:1099824. doi: 10.3389/fendo.2023.1099824
Received: 16 November 2022; Accepted: 01 February 2023;
Published: 13 February 2023.
Edited by:
Laurent M. Sachs, Muséum National d’Histoire Naturelle, FranceReviewed by:
Patricia Rannaud-bartaire, Muséum National d’Histoire Naturelle, FranceWenxing Guo, Tianjin Medical University, China
Copyright © 2023 Lee, Cho, Lim, Kim, Kim, Hong, Park, Shin and Lee. This is an open-access article distributed under the terms of the Creative Commons Attribution License (CC BY). The use, distribution or reproduction in other forums is permitted, provided the original author(s) and the copyright owner(s) are credited and that the original publication in this journal is cited, in accordance with accepted academic practice. No use, distribution or reproduction is permitted which does not comply with these terms.
*Correspondence: Young Ah Lee, nina337@snu.ac.kr