- 1Unidad de Investigación, Hospital Universitario Nuestra Señora de Candelaria (HUNSC), Santa Cruz de Tenerife, Spain
- 2GEENDIAB (Grupo Español para el estudio de la Nefropatía Diabética), Sociedad Española de Nefrología, Santander, Spain
- 3Instituto de Tecnologías Biomédicas, Universidad de La Laguna, Santa Cruz de Tenerife, Spain
- 4RICORS2040 (Red de Investigación Renal-RD21/0005/0013), Instituto de Salud Carlos III, Madrid, Spain
- 5Área de Medicina Preventiva y Salud Pública, Universidad de La Laguna, San Cristóbal de La Laguna, Spain
- 6Escuela de Doctorado y Estudios de Posgrado, Universidad de La Laguna, San Cristóbal de La Laguna, Spain
- 7Vascular Surgery Service, HUNSC, Santa Cruz de Tenerife, Spain
- 8Servicio de Anatomía Patológica, HUNSC, Santa Cruz de Tenerife, Spain
- 9Departamento de Anatomía e Histología Humana, Universidad de Salamanca, Salamanca, Spain
- 10Servicio de Análisis Clínicos, HUNSC, Santa Cruz de Tenerife, Spain
- 11Servicio de Nefrología, HUNSC, Santa Cruz de Tenerife, Spain
Introduction: Klotho protein is predominantly expressed in the kidneys and has also been detected in vascular tissue and peripheral blood circulating cells to a lesser extent. Carotid artery intima-media thickness (CIMT) burden, a marker of subclinical atherosclerosis, has been associated with reductions in circulating Klotho levels in chronic kidney disease patients, who show reduced levels of this protein at all stages of the disease. However, the contribution of serum Klotho and its expression levels in peripheral blood circulating cells and in the carotid artery wall on the CIMT in the absence of kidney impairment has not yet been evaluated.
Methods: We conducted a single-center study in 35 atherosclerotic patients with preserved kidney function (eGFR≥60 mL/min/1.73m2) subjected to elective carotid surgery. Serum levels of Klotho and cytokines TNFa, IL6 and IL10 were determined by ELISA and transcripts encoding for Klotho (KL), TNF, IL6 and IL10 from vascular segments were measured by qRT-PCR. Klotho protein expression in the intima-media and adventitia areas was analyzed using immunohistochemistry.
Results: APatients with higher values of CIMT showed reduced Klotho levels in serum (430.8 [357.7-592.9] vs. 667.8 [632.5-712.9] pg/mL; p<0.001), mRNA expression in blood circulating cells and carotid artery wall (2.92 [2.06-4.8] vs. 3.69 [2.42-7.13] log.a.u., p=0.015; 0.41 [0.16-0.59] vs. 0.79 [0.37-1.4] log.a.u., p=0.013, respectively) and immunoreactivity in the intimal-medial area of the carotids (4.23 [4.15-4.27] vs. 4.49 [4.28-4.63] log µm2 p=0.008). CIMT was inversely related with Klotho levels in serum (r= -0.717, p<0.001), blood mRNA expression (r=-0.426, p=0.011), and with carotid artery mRNA and immunoreactivity levels (r= -0.45, p=0.07; r= -0.455, p= 0.006, respectively). Multivariate analysis showed that serum Klotho, together with the gene expression levels of tumor necrosis factor TNFa in blood circulating cells, were independent determinants of CIMT values (adjusted R2 = 0.593, p<0.001).
Discussion: The results of this study in subjects with eGFR≥60mL/min/1.73m2 show that patients with carotid artery atherosclerosis and higher values of CIMT present reduced soluble Klotho levels, as well as decreased KL mRNA expression in peripheral blood circulating cells and Klotho protein levels in the intima-media of the carotid artery wall.
Introduction
Atherosclerosis is the underlying process of most cardiovascular events, the main cause of death in middle-aged and elderly people (1). This process is thought to be a chronic inflammatory disease initiated and perpetuated by a variety of cardiovascular risk factors (2). Klotho protein, an important regulator of mineral metabolism (3), has been reported to exert a protective anti-inflammatory role in the pathogenesis of atherosclerosis in both clinical and preclinical studies (4–12).Klotho is a single-pass transmembrane protein predominantly expressed in the kidneys and, at lesser extent, it has also been detected in the vasculature, and in peripheral blood circulating cells (PBCCs) (13–15). A circulating soluble form of Klotho is also generated by proteolytic cleavage of the extracellular domain of the membranous protein. This shedding is operated by 2 metalloproteinases of the A disintegrin and metalloproteinase domain containing protein (ADAM) family (ADAM10 and ADAM17) (16). ADAM17 also plays a key role in the proteolytic release from cell membranes of some cytokines including tumor necrosis factor (TNF) α, which is produced by various cell types such as macrophages, monocytes, and T cells, thus playing a central role in the regulation of the immune system and in a variety of inflammatory responses (17). Defects in Klotho expression in mice result in a syndrome that is similar to human aging, including Mönckeberg-type arteriosclerosis from the aorta to small arterioles (13), impairments in angiogenesis and vasculogenesis (6) and endothelial dysfunction (7). Furthermore, in vivo gene delivery or upregulation of Klotho expression protect against endothelial dysfunction in experimental models of atherosclerosis (8) and ameliorates vascular calcification in an adenine-induced chronic renal failure model (18, 19). Similarly, endothelial dysfunction in heterozygous Klotho-deficient mice is prevented by parabiosis with wild-type mice (7). Also in vitro, Klotho protects vascular smooth muscle and endothelial cells against various stressors (20, 21).
In the clinical scenario, the reduction in circulating Klotho has been proposed as a predictor of atherosclerosis (10) being related with carotid artery intima-media thickness (CIMT) burden, a marker of subclinical atherosclerosis (10, 22), as well as with an increased incidence of cardiovascular disease (CVD) (23). These associations with vascular damage and CVD are particularly relevant in patients with renal disease since Klotho levels are reduced in all stages of the disease (9, 24, 25). Thus, both the reduction of soluble Klotho in blood and the decrease of gene expression in PBCCs have been associated with increased CIMT in patients with moderate to severe chronic kidney disease (CKD) (9, 26, 27). However, the contribution of Klotho expression both in PBCCs and in the carotid artery wall on the CIMT burden in patients with preserved values of estimated glomerular filtration rate (eGFR) has not been assessed yet. Moreover, the relationship of circulating Klotho with the CIMT in patients with preserved kidney function has been refuted by some recent reports (28–30). For all these reasons, we conducted a single-center study in a group of atherosclerotic patients with GFR ≥60mL/min/1.73m2 subjected to elective carotid surgery. In this group, we performed different measurements of Klotho protein levels in serum and carotid artery, and of Klotho gene expression in PBCCs and carotids, and analyzed their relationship with CIMT values. We also determined the levels of ADAM-17 and of inflammatory cytokines involved in the atherosclerotic process including tumor necrosis factor α (TNFα) and the interleukins (IL) 6 and 10.
Patients and methods
Study design and participants
This cross-sectional single-center proof-of-concept study recruited patients from the Vascular Surgery Service of the University Hospital Nuestra Señora de Candelaria (Santa Cruz de Tenerife, Spain). The study was conducted in accordance with the tenets of the Declaration of Helsinki, and approval was obtained from the local ethics committee. Written informed consent was provided by all participants. Exclusion criteria were estimated eGFR lower than 60 mL/min/1.73m2; hemodynamic instability during the surgical procedure (systolic blood pressure (SBP)<90 mmHg and/or administration of inotropes or vasopressors); chronic or acute inflammatory status including: immunologic disease, tumoral disease, hepatitis B, hepatitis C, or HIV, infectious intercurrent episodes in the previous month; receipt of immunotherapy or immunosuppressive therapies; institutionalization; and inability or unwillingness to provide informed consent.
After an initial evaluation of 62 patients, only 35 subjects met the inclusion and exclusion criteria and were finally included in the study. Thus, the study group consisted in 35 patients older than 18 years who underwent elective endarterectomies due to clinical atherosclerotic vascular disease.
At the time of surgery, sections of the affected carotid artery were retrieved. Whole blood samples (3 mL) were also collected in PAXgene Blood RNA tubes (BD, Franklin Lakes, NJ) and in routine blood-collection tubes (BD serum separation transport tube—BD, Franklin Lakes, NJ). Serum fractions were isolated, aliquoted and immediately frozen at -80°C.
Measurements of CIMT were performed by two qualified radiologists who were blinded to the study participant´s details. Ultrasonographies were made with a high-resolution ultrasound (Philips ATL 5000 HDI, Royal Philips Electronics, Amsterdam, The Netherlands).
Biochemical determinations
The Clinical Analysis Service determined biochemical and hematological parameters by employing standardized tests. Serum levels of Klotho and inflammatory cytokines were measured using commercial ELISA assay kits according to manufacturer’s instructions. For serum Klotho, a solid phase sandwich ELISA (Immuno-Biological Laboratories, Gunma, Japan) – sensitivity: 6.15 pg/mL; intra- and inter-assay coefficients of variation: 2.7–3.5% and 2.9–11.4%, respectively- was employed. Serum levels of cytokines TNFα, IL6 and IL10 were determined with Quantikine® ELISA kits (R&D Systems, Abingdon, UK) -sensitivity: 0.10 pg/mL, 0.70 pg/mL, and 0.09 pg/mL, respectively; intra- and inter-assay coefficients of variability were < 10.8%-.
Gene expression analysis
Total RNA was extracted from homogenized vascular segments by employing liquid nitrogen with a pestle and mortar and RNAzol RT (Sigma Aldrich, MO, USA). Blood total RNA was isolated using PAXgene Blood RNA Kit (PreAnalytiX, Switzerland). RNA samples were stored at −80°C until being retrotranscribed to cDNA using a High Capacity RNA-to-cDNA kit (Applied Biosystems, CA, USA) for further use in quantitative RT-PCR (qRT-PCR).
Transcripts encoding for Klotho (KL), TNF, IL6, IL10, and glyceraldehyde-3-phosphate dehydrogenase (GAPDH) genes were measured by TaqMan qRT-PCR employing the following probes: Hs00183100_m1 [KL], Hs00174128 m1 [TNF], Hs00985639_ml [IL6], Hs00961622_m1 [IL10], and Hs99999905_m1 [GAPDH]. target mRNA was estimated by relative quantification using the 2-ΔΔCt method and GAPDH as housekeeping gene. Samples were tested in triplicate and values were expressed as arbitrary units (a.u.).
Histology and immunohistochemistry
Blood vessels sections were fixed in 4% buffered formalin for 24 h, dehydrated in ascending concentrations of ethanol, cleared in xylene and embedded in paraffin blocks. Trimmed sections of 3µm were processed for histology and immunohistochemistry. Hematoxylin and eosin (HE) staining was performed. For immunohistochemistry, the carotid sections were boiled in sodium citrate solution for 30 min in a Decloaking Chamber™ NxGen (Biocare Medicals, CA, USA) for antigen retrieval and then returned to room temperature. After blocking with 10% Bovine Serum Albumin (BSA) for 30 min. The sections were incubated with 1:100 dilution of rabbit monoclonal anti-Klotho primary antibody (Abcam, ab181373). After washing with PBS, the sections were incubated with the secondary antibody anti-rabbit IgG for 30 min. Following a PBS rinse, sections were developed with 3, 3-diaminobenzidine tetrahydrochloride (DAB) (Sigma-Aldrich, St. Louis, MO, USA) to produce a brown color. For immunoreactivity quantification analysis, images of each slide including intima- media and adventitia layers were captured and processed with a high-resolution video camera (Sony, DF-W-X710, Kōnan, Japan) connected to a light microscope (Nikon Eclipse 50i). Stained brown areas were quantified using ImageJ software (Rasband, W.S., ImageJ, National Institutes of Health, Bethesda, MD, USA). Briefly, Klotho protein expression in the intima-media and adventitia areas was analyzed separately and expressed as square microns (μm2) of staining. The immunoreactivity values represent the mean of the quantification values obtained in 5 microscopic fields with the same dimensions that were chosen at random in each stained carotid section.
Statistical analysis
Normal distribution of continuous variables was checked using the Kolmogorov–Smirnov’s test. Normally distributed variables were expressed as the mean ± SD and non-normally distributed as the median (interquartile range). Categorical data were expressed as number and percent frequency. Differences between groups were analyzed using Chi-square test, Student’s t-test or the Mann–Whitney U-test as appropriate. The Spearman rank correlation test was used to determine bivariate correlations. Backward stepwise multiple regression analysis was performed to determine potential predictor variables (age, sex, body mass index (BMI), hypertension (HT), type 2 diabetes mellitus (DM2), smoking, dyslipidemia, serum uric acid, eGFR, urinary albumin excretion (UAE), phosphorus, serum C-reactive protein (PCR), neutrophil-to-lymphocyte ratio (NLR), serum Klotho, PBCCs and carotid mRNA expression levels of KL, TNF, IL6, and IL10, and immunoreactivity for Klotho protein) of CIMT. Collinearity was excluded analyzing the tolerance and variance inflation factor. A value of P < 0.05 was considered to be statistically significant. All analyses were performed using SPSS software version 25 (IBM Corp. Armonk, NY, USA).
Results
Demographic, clinical and biochemical characteristics of the study population are presented in Table 1. A total of 35 patients (27 males), with a mean age of 65 ± 6.9 years, were included in this study. The median and IQR values for CIMT and eGFR were 1.15 (1.0-1.62) mm and 93.05 (82.4-98.2) mL/min/1.73m2, respectively. Main comorbidities included DM2 (25.7%), HT (82.9%), smoking (48.6%), and dyslipidemia (45.7%).
The study population was divided into two subgroups according to the median CIMT value: high CIMT (≥ 1.15 mm; HCIMT, n=17) and low CIMT (< 1.15 mm; LCIMT, n=18). The median serum Klotho levels in the HCIMT group were significantly lower than in the LCIMT group (430.8 [357.7-592.9] vs. 667.8 [632.5-712.9] pg/mL; p<0.001) (Table 1, Figure 1). Patients included in the HCIMT group also presented a higher incidence of DM2 (41.2 vs. 11.11; p=0.049) and higher levels of serum TNFα (1.04 [0.81-1.32] vs. 0.84 [0.53-0.93] pg/mL; p=0.045) (Table 1). No differences between groups were observed in the use of antiaggregant, beta-blockers, angiotensin-converting enzyme inhibitor or angiotensin receptor antagonist, calcium channel blockers, or statins.
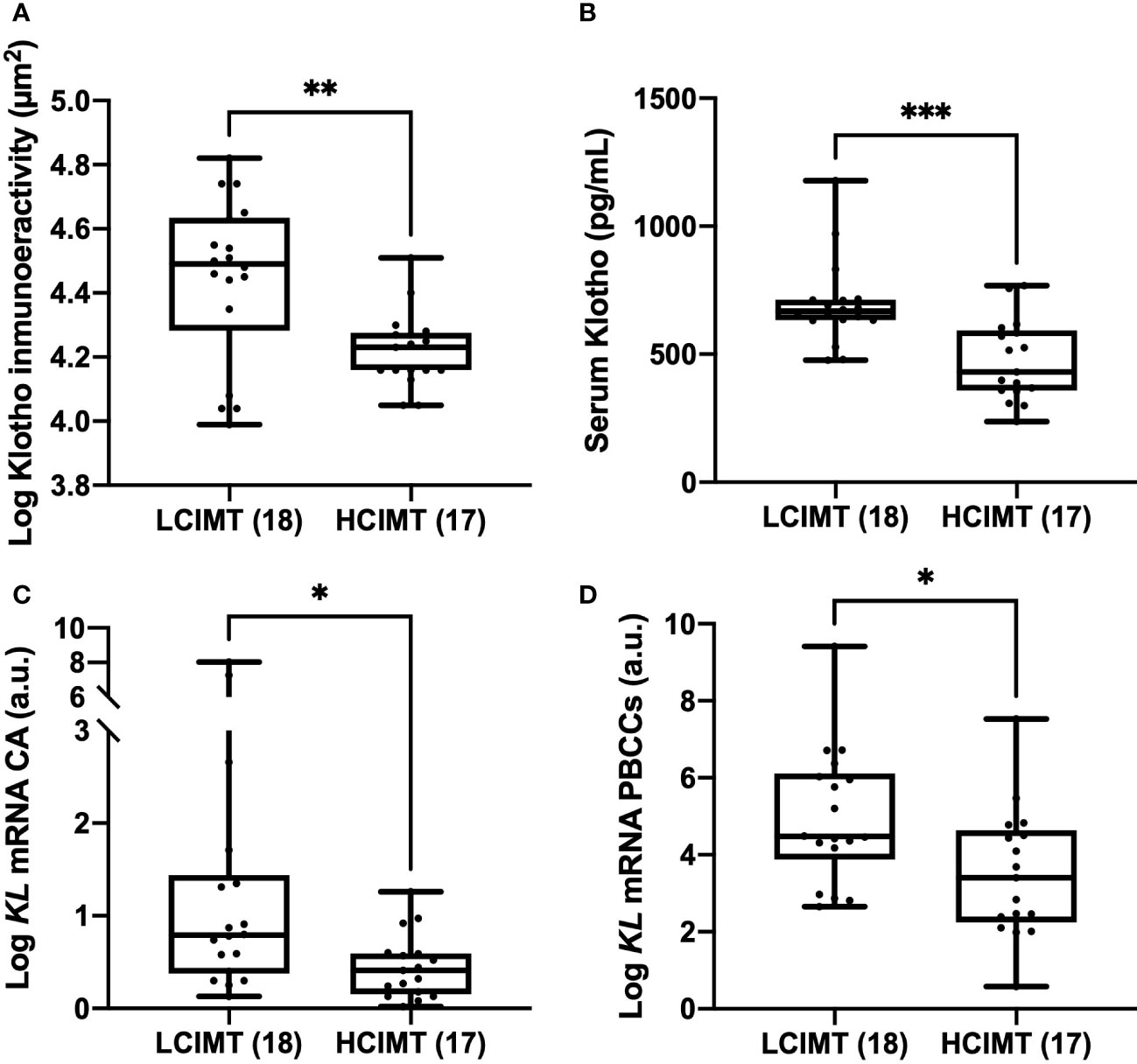
Figure 1 Differences in Klotho vascular immunoreactivity (A), serum levels (B), and mRNA gene expression levels in CA (C), and PBCCs (D) according to LCIMT and HCIMT groups. The line within the box represents the median value. The lower and the upper border of the box represent the first and third quartile, respectively. The whiskers indicate maximum and minimum values. HCIMT, high carotid-intima thickness; LCIMT, low carotid-intima thickness; CA, carotid artery; KL, Klotho gene; PBCCs, peripheral blood circulating cells. HCIMT, n=17; LCIMT, n=18. *p<0.05; ** p<0.01; ***p<0.001.
Regarding gene expression, we found reduced mRNA levels of KL in the HCIMT group either in PBCCs (2.92 [2.06-4.8] vs. 3.69 [2.42-7.13] log a.u., p=0.015) and in carotid artery fragments (0.41 [0.16-0.59] vs. 0.79 [0.37-1.4] log a.u., p=0.013) (Table 2, Figure 1). Klotho protein was detected both in the intimal-medial area and in the adventitia of the carotid sections of all the patients included in the study. No differences were observed in the immunoreactivity of the adventitia according to the subgroups (HCIMT: 3.62 [2.8-4.1] vs. LCIMT: 3.71 [2.9-4.3] log µm2, p=0.131). However, and similarly to what is observed with KL mRNA levels, the immunoreactivity for Klotho protein in the intima-media was reduced in patients included in the HCIMT group (4.23 [4.15-4.27] vs. 4.49 [4.28-4.63] log µm2, p=0.008) (Figure 2). Gene expression of ADAM17 was also reduced in carotids of HCIMT patients (0.84 [0.61-1.31] vs. 1.08 [0.9-2.3] log a.u., p=0.012). Concerning inflammatory cytokines, we only found differences in the expression levels for TNF mRNA in PBCCs, which was increased in the HCIMT group (3.73 [1.94-4.12] vs. 2.14 [1.89-2.82] log a.u., p=0.019) (Table 2).
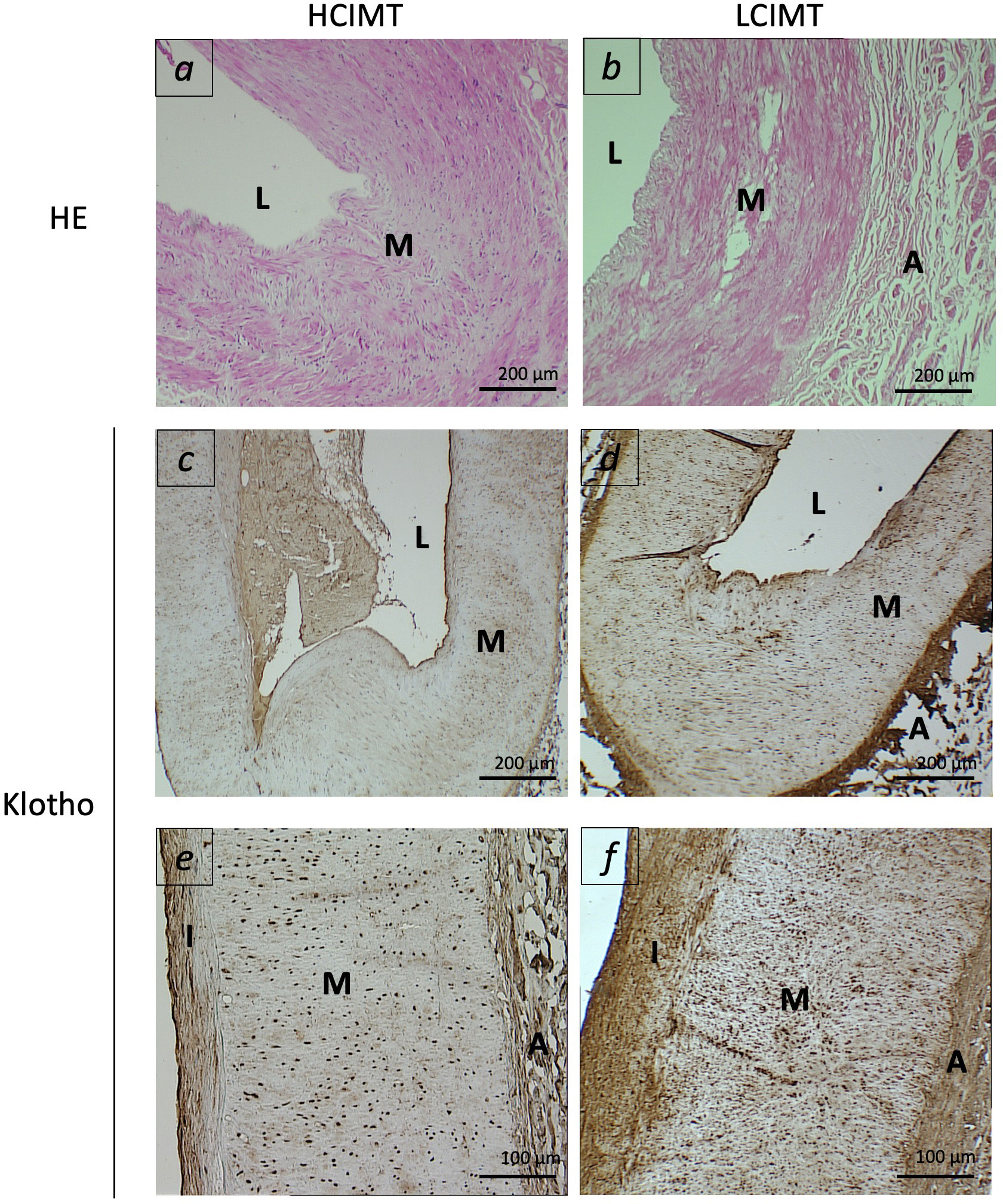
Figure 2 Representative images of carotid arterial sections from patients included in the HCIMT (A, C, E) and LCIMT (B, D, F) groups. Hematoxylin & eosin (HE) staining (A, B) and immunohistochemical staining for Klotho (C–F). HCIMT, n=17; LCIMT, n=18. Original magnification 10x (A–D) and 20x (E, F). L, lumen; I, tunica intima; M, tunica media; A, adventitia.
Correlation analysis showed that CIMT were inversely correlated with the levels of serum Klotho (r= -0.717, p<0.001), the values of immunoreactivity for Klotho protein in the intima-media area (r= -0.455, p= 0.006), and with KL mRNA expression levels both in PBCCs (r=-0.426, p=0.011) and in carotid arteries (r= -0.45, p=0.07) (Table 3, Figure 3). We also found a direct correlation of CIMT values with UAE (r=0.386, p=0.022), and with the PBCCs mRNA expression of ADAM17 (r=0.419, p=0.012).
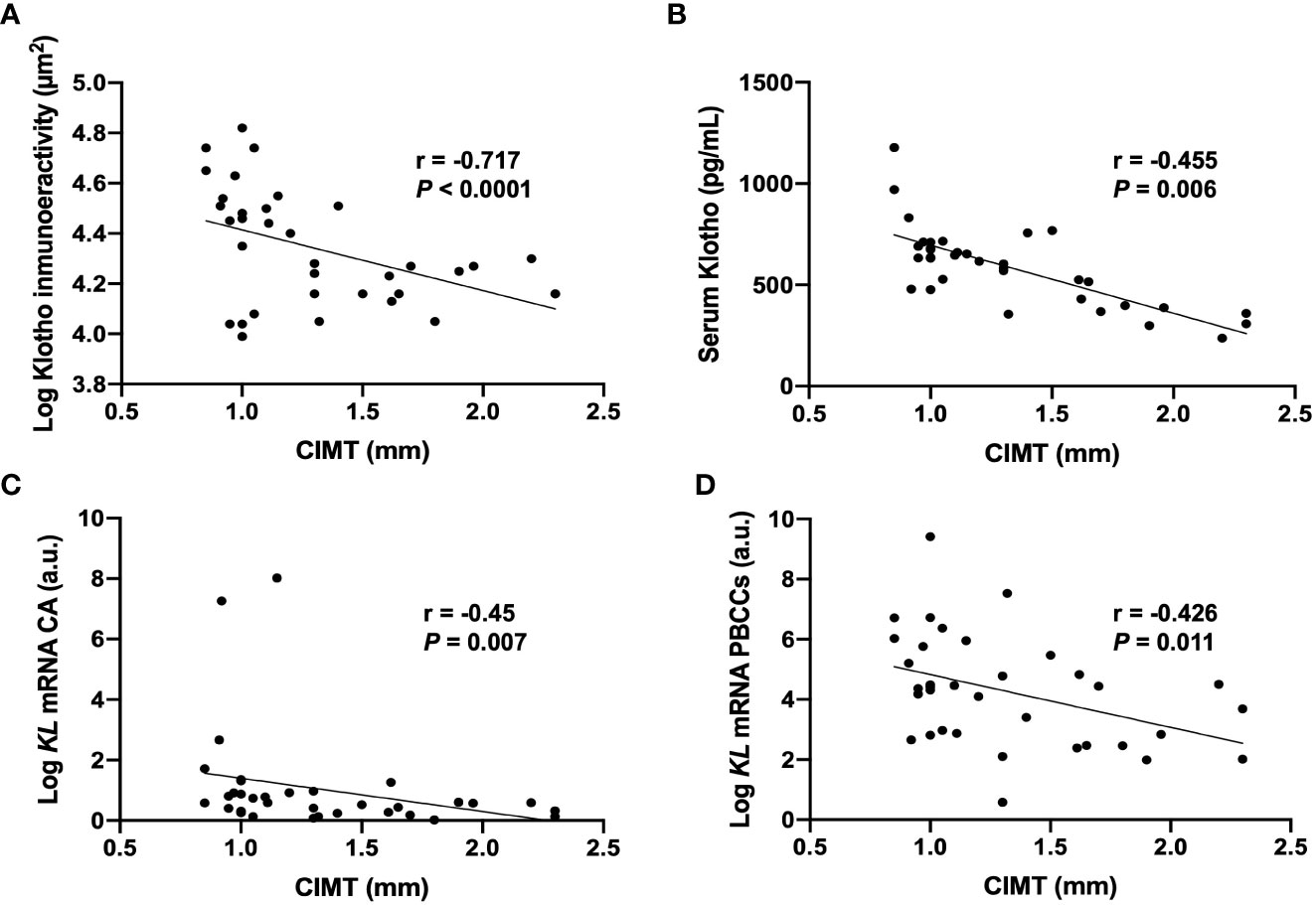
Figure 3 Correlations between CIMT values and Klotho vascular immunoreactivity (A), serum levels (B), and mRNA gene expression levels in CA (C) and PBCCs (D). CA, carotid artery; KL, Klotho gene; PBCCs, peripheral blood circulating cells. N=35.
Finally, to test the independent association between Klotho and the CIMT values, we performed a backward stepwise multiple regression analysis. This analysis included CIMT as the dependent variable and diverse potential predictors including, among others, UAE, serum Klotho, PBCCs mRNA expression of ADAM17, PBCCs and carotid mRNA expression levels of KL, TNF, IL6, and IL10, and immunoreactivity for Klotho protein as covariates. The results showed that only serum Klotho concentrations and mRNA TNF expression levels in PBCCs were related with the values of CIMT (adjusted R2 = 0.593, p<0.001) (Table 4). Tolerance and VIF values were higher than 0.60 and lower than 1.5 for all variables in any of the analysis. Therefore, collinearity was excluded.
Discussion
The results of this study in subjects with eGFR≥60mL/min/1.73m2 show that patients with carotid artery atherosclerosis and higher values of CIMT present reduced soluble Klotho levels, as well as decreased KL mRNA expression in PBCCs and Klotho protein levels in the intima-media of the carotid artery wall. In multivariate analysis, only serum Klotho was found to be an independent determinant of CIMT value, together with TNF expression in PBCCs. Several clinical and preclinical studies have suggested that Klotho exerts strong vascular protective effects. For instance, higher soluble Klotho levels have been related to a lower mortality and CVD morbidity (23, 31, 32). Conversely, in patients with CKD, a condition characterized by reductions of Klotho protein from early stages, low circulating Klotho concentrations have been associated with increased arterial stiffness and CIMT (9, 26). However, studies about the relationship between Klotho and CIMT in patients with atherosclerosis and preserved kidney function are scarce and none of them had previously considered the expression levels of Klotho in PBCCs and atherosclerotic vascular tissue.
Circulating Klotho may participate in vascular health by protecting against endothelial dysfunction (7, 8) which plays an important role in the development of atherosclerosis (33). But beyond its soluble form, potential benefits of Klotho in vascular homeostasis may also come from its expression in vascular tissue and in PBCCs. Klotho is endogenously expressed in human vascular smooth muscle cells (14, 32) and although its exact role in the vasculature remains to be elucidated, inhibition of Klotho expression in these cells results in accelerated calcification (32). Moreover, its reduction is associated with coronary artery disease (31) and is inversely related with inflammation in atherosclerosis (11). Bivariate analyses presented in this work show inverse relationships of CIMT with vascular Klotho, both at mRNA and protein levels. However, after adjusting for age, gender, blood pressure, smoking, DM2, dyslipidemia, albuminuria and other cardiovascular risk factors and inflammatory markers, only the inverse association of serum Klotho with CIMT remained as statistically significant.
The association between low Klotho and CVD has been recently extended to PBCCs, in which reductions in Klotho expression have been related with aging process and with the development of pathologies with an inflammatory component, including atherosclerosis (26, 34–36). Macrophages, monocytes, lymphocytes, and other PBCCs play an important role in development of the inflammatory response associated with atherogenesis (37, 38). Upregulation of Klotho expression in PBCC could have beneficial anti-inflammatory actions leading to better progression of atherosclerotic lesions. Thus, Klotho expression in PBCCs has been related with the attenuation of acute inflammation induced by lipopolysaccharides, with the inactivation of NF-kB signaling and with the promotion of M2 polarization in macrophages (39). Likewise, the stimulation of Klotho expression in these cells has also been related with the suppression of the stress response of the Golgi apparatus and endoplasmic reticulum, the reduction of oxidative stress and pro-inflammatory cytokines production, and with an increased expression of anti-inflammatory cytokines as well as with the preservation of the immune function in senescent monocytes (40, 41). Moreover, we recently reported an association between Klotho expression in PBCCs and vascular and systemic inflammation in atherosclerotic vascular disease (15) and with subclinical atherosclerosis in CKD patients (26).
Besides transmembrane Klotho shedding, ADAM17 is responsible of proteolytical cleavage of several cell surface proteins highly expressed in leukocytes which are involved in the inflammatory response, such as TNFα, TNF-RI, TNF-RII, IL-6R, RANKL, INF-γ or CD40 (42). As shown in Tables 1 and 2, patients in the HCIMT group showed a steepen pro-inflammatory state marked by a tendency to have higher values of neutrophil-to-lymphocyte ratio and significant higher levels of serum TNF-α or TNF gene expression. So, a higher expression of ADAM17 in PBCCs of these patients could respond to the need to exert a pro-inflammatory response by leukocytes in the context of atherosclerotic damage. In the case of the vascular wall, several cytokines, receptors, adhesion molecules or growth factors expressed in this tissue, and directly related to the setting of atherosclerosis, are substrates of ADAM17. Actually, ADAM17 is considered a pro-inflammatory molecule in the context of atherosclerotic damage, since it is capable to mediate endothelial damage, vascular inflammation, and to promote plaque vulnerability. In fact, several studies indicate that overexpression of ADAM17 is linked to exacerbation of the atherosclerotic process (43–45). Surprisingly, we observed that the expression of this metalloproteinase in the HCIMT group in CA is reduced compared to individuals with LCIMT in our cohort, which is an unexpected finding that might be related to the low sample size of the study.
Although this work provides novel information about the relationship between Klotho and atherosclerosis, we acknowledge several limitations. This is a proof-of-concept study, where the main limitation is the small sample size and the cross-sectional design, which only allowed us to show associations without definitive inferences about their direction or causality. Moreover, our results only show relationships in an already developed clinical scenario and do not provide information about the independent implication of reductions in Klotho levels with the development and progression of the carotid artery atherosclerotic lesion. In addition, we did not measure ADAM10 expression values neither in PBCCs nor in arterial tissue. This alpha-secretase is also responsible of the shedding of Klotho and, similarly to ADAM17, is known to cleave inflammatory mediators, including TNFα. Moreover, we did not analyze the effect of confounders other than serum Klotho levels as potential predictors of atherosclerosis Specifically, UAE has been previously related with subclinical atherosclerosis (46), so we cannot exclude the contribution of albuminuria to CIMT values in our study.
Moreover, the expression of Klotho is also present in the adventitia, probably in the fibroblasts (47). Although we did not find differences between groups in the immunoreactivity for Klotho in this layer, the results of the gene expression study depicted in this work did not differentiate between intima-media and adventitia, so we cannot exclude that the differences in mRNA expression observed might be due to differential expression of this protein in the different layers of the carotid artery.
Given the nature of this study, carried out in normal clinical practice conditions and with patients undergoing elective surgery, we were unable to have a control group. In order to partially solve this limitation, and although it is not the ideal situation, we have used the group of patients with low CIMT in the comparative analysis. Finally, the small sample size that could make that some of our results did not reach statistical significance and, consequently, the ability to describe the association between some variables including vascular and PBCCs expression of Klotho might be limited. Also, this limitation could make that the findings made in this group not be transferable to larger populations.
Nevertheless, our study presents some strengths. Together with serum, we have also determined Klotho expression levels in PBCCs and in vascular tissue, which have a clear implication with the progression of atherosclerosis. Moreover, data included conventional and CVD-related cardiovascular risk factors, including the serum, PBCCs and vascular expression of ADAM-17 and of inflammatory cytokines involved in the atherosclerotic process.
In conclusion, we found that lower Klotho levels are inversely correlated with CIMT values in a group of patients with atherosclerosis and preserved kidney function. Our results are in line with previous findings in the literature that point to soluble Klotho as a protective factor against cardiovascular disorders. Further experimental studies are needed to delve into the interactions between Klotho and the progression of the atherosclerosis burden. In particular, in vitro studies are needed to determine the underlying mechanisms by which Klotho is able to maintain endothelial integrity and prevent vascular smooth muscle cell differentiation into proatherogenic phenotypes. These effects may be mediated by reducing oxidative stress and inflammation, and by maintaining endothelial nitric oxide production.
Data availability statement
The raw data supporting the conclusions of this article will be made available by the authors, without undue reservation.
Ethics statement
The studies involving human participants were reviewed and approved by Comité Ético de Investigación con Medicamentos (CEIm). The patients/participants provided their written informed consent to participate in this study.
Author contributions
JD-C: funding acquisition, writing—original draft preparation, acquisition, analysis, and interpretation of data, manuscript submission. EM-N: patient inclusion, acquisition, analysis, and interpretation of data. AM-O and VT: acquisition, analysis, and interpretation of data. CF: acquisition of data; ÁL-C, AD-M, VL-T, MA-G, NP-D, and AG-L acquisition of data and revising the manuscript. CM-F and JN-G: funding acquisition, design and planning of the work, patient inclusion, acquisition, analysis, and interpretation of data, and drafting the work. All authors have read and agreed to the published version of the manuscript.
Funding
This study was supported by Instituto de Salud Carlos III (ISCIII) -PI13/01726, PI16/00024, PI19/00035, PI21/01037 and RD16/0009/0022-, Fundación Canaria Instituto de Investigación Sanitaria de Canarias (FIISC) -PIFIISC21/08-, and Fundación Canaria Mapfre-Guanarteme (FCMG) -OA22/107-. EM-N was funded by a research contract from REDinREN-ISCIII (RD16/0009/0022). CF is recipient of a fellowship from Agencia Canaria de Investigación, Innovación y Sociedad de la Información of Consejería de Economía, Industria, Comercio y Conocimiento, Gobierno de Canarias (ACIISI) TESIS2018010110). JD-C is recipient of a contract from Miguel Servet Programme-ISCIII (CP20/00122). AG-L is recipient of a PFIS contract from ISCIII (FI22/00213).
Conflict of interest
The authors declare that the research was conducted in the absence of any commercial or financial relationships that could be construed as a potential conflict of interest.
Publisher’s note
All claims expressed in this article are solely those of the authors and do not necessarily represent those of their affiliated organizations, or those of the publisher, the editors and the reviewers. Any product that may be evaluated in this article, or claim that may be made by its manufacturer, is not guaranteed or endorsed by the publisher.
References
1. Global health estimates 2020: deaths by cause, age, sex, by country and by region, 2000-2019. Geneva World Health Organ.
2. Ross R. Atherosclerosis – an inflammatory disease. N Engl J Med (1999) 340:115–26. doi: 10.1056/NEJM199901143400207
4. Ohta J, Rakugi H, Ishikawa K, Yang J, Ikushima M, Chihara Y, et al. Klotho gene delivery suppresses oxidative stress in vivo. Geriat Gerontol Int (2007) 7:293–9. doi: 10.1111/j.1447-0594.2007.00406.x
5. Maekawa Y, Ishikawa K, Yasuda O, Oguro R, Hanasaki H, Kida I, et al. Klotho suppresses TNF-alpha-induced expression of adhesion molecules in the endothelium and attenuates NF-kappaB activation. Endocrine (2009) 35:341–6. doi: 10.1007/s12020-009-9181-3
6. Shimada T, Takeshita Y, Murohara T, Sasaki K, Egami K, Shintani S, et al. Angiogenesis and vasculogenesis are impaired in the precocious-aging klotho mouse. Circulation (2004) 110:1148–55. doi: 10.1161/01.CIR.0000139854.74847.99
7. Saito Y, Yamagishi T, Nakamura T, Ohyama Y, Aizawa H, Suga T, et al. Klotho protein protects against endothelial dysfunction. Biochem Biophys Res Commun (1998) 248:324–9. doi: 10.1006/bbrc.1998.8943
8. Saito Y, Nakamura T, Ohyama Y, Suzuki T, Iida A, Shiraki-Iida T, et al. In vivo klotho gene delivery protects against endothelial dysfunction in multiple risk factor syndrome. Biochem Biophys Res Commun (2000) 276:767–72. doi: 10.1006/bbrc.2000.3470
9. Kitagawa M, Sugiyama H, Morinaga H, Inoue T, Takiue K, Ogawa A, et al. A decreased level of serum soluble klotho is an independent biomarker associated with arterial stiffness in patients with chronic kidney disease. LloS One (2013) 8(2):e56695. doi: 10.1371/journal.pone.0056695
10. Keles N, Caliskan M, Dogan B, Keles NN, Kalcik M, Aksu F, et al. Low serum level of klotho is an early predictor of atherosclerosis. Tohoku J Exp Med (2015) 237(1):17–23. doi: 10.1620/tjem.237.17
11. Martín-Núñez E, Donate-Correa J, López-Castillo Á, Delgado-Molinos A, Ferri C, Rodríguez-Ramos S, et al. Soluble levels and endogenous vascular gene expression of KLOTHO are related to inflammation in human atherosclerotic disease. Clin Sci (2017) 131(21):2601–9. doi: 10.1042/CS20171242
12. Pan H-C, Chou K-M, Lee C-C, Yang N-I, Sun C-Y. Circulating klotho levels can predict long-term macrovascular outcomes in type 2 diabetic patients. Atherosclerosis (2018) 276:83–90. doi: 10.1016/j.atherosclerosis.2018.07.006
13. Kuro-o M, Matsumura Y, Aizawa H, Kawaguchi H, Suga T, Utsugi T, et al. Mutation of the mouse klotho gene leads to a syndrome resembling ageing. Nature (1997) 390:45–51. doi: 10.1038/36285
14. Donate-Correa J, Mora-Fernández C, Martínez-Sanz R, Muros-de-Fuentes M, Pérez H, Meneses-Pérez B, et al. Expression of FGF23/KLOTHO system in human vascular tissue. Int J Cardiol (2013) 165:179–83. doi: 10.1016/j.ijcard.2011.08.850
15. Martín-Núñez E, Pérez-Castro A, Tagua VG, Hernández-Carballo C, Ferri C, Pérez-Delgado N, et al. Klotho expression in peripheral blood circulating cells is associated with vascular and systemic inflammation in atherosclerotic vascular disease. Sci Rep (2022) 12(1):8422. doi: 10.1038/s41598-022-12548-z
16. Chen CD, Podvin S, Gillespie E, Leeman SE, Abraham CR. Insulin stimulates the cleavage and release of the extracellular domain of klotho by ADAM10 and ADAM17. Proc Natl Acad Sci USA (2007) 104:19796e19801. doi: 10.1073/pnas.0709805104
17. Solomon KA, Pesti N, Wu G, Pesti N, Wu G, Newton RC. Cutting edge: a dominant negative form of TNF-alpha converting enzyme inhibits proTNF and TNFRII secretion. J Immunol (1999) 163:4105–8. doi: 10.4049/jimmunol.163.8.4105
18. Zhao Y, Zhao MM, Cai Y, Zheng MF, Sun WL, Zhang SY, et al. Mammalian target of rapamycin signaling inhibition ameliorates vascular calcification via klotho upregulation. Kidney Int (2015) 88(4):711–21. doi: 10.1038/ki.2015.160
19. Chang JR, Guo J, Wang Y, Hou YL, Lu WW, Zhang JS, et al. Intermedin1–53 attenuates vascular calcification in rats with chronic kidney disease by upregulation of α-klotho. Kidney Int (2016) 89(3):586–600. doi: 10.1016/j.kint.2015.12.029
20. Six I, Okazaki H, Gross P, Cagnard J, Boudot C, Maizel J, et al. Direct, acute effects of klotho and FGF23 on vascular smooth muscle and endothelium. PLoS One (2014) 9(4):e93423. doi: 10.1371/journal.pone.0093423
21. Kusaba T, Okigaki M, Matui A, Murakami M, Ishikawa K, Kimura T, et al. Klotho is associated with VEGF receptor-2 and the transient receptor potential canonical-1 Ca2+ channel to maintain endothelial integrity. Proc Natl Acad Sci (2010) 107(45):19308–13. doi: 10.1073/pnas.1008544107
22. Willeit P, Tschiderer L, Allara E, Reuber K, Seekircher L, Gao L, et al. Carotid intima-media thickness progression as surrogate marker for cardiovascular risk: meta-analysis of 119 clinical trials involving 100 667 patients. Circulation. (2020) 142(7):621–42. doi: 10.1161/CIRCULATIONAHA.120.046361
23. Semba RD, Cappola AR, Sun K, Bandinelli S, Dalal M, Crasto C, et al. Plasma klotho and cardiovascular disease in adults. J Am Geriatr. Soc (2011) 59:1596–160. doi: 10.1111/j.1532-5415.2011.03558.x
24. Kim HR, Nam BY, Kim DW, Kang MW, Han JH, Lee MJ, et al. Circulating α-klotho levels in CKD and relationship to progression. Am J Kidney Dis (2013) 61:899–909. doi: 10.1053/j.ajkd.2013.01.024
25. Zheng S, Zheng Y, Jin L, Zhou Z, Li Z. Relationship between serum soluble klotho protein and coronary artery calcification and prognosis in patients on maintenance hemodialysis. Iran J Public Health (2018) 47:510–8.
26. Donate-Correa J, Ferri CM, Martín-Núñez E, Pérez-Delgado N, González-Luis A, Mora-Fernández C, et al. Klotho as a biomarker of subclinical atherosclerosis in patients with moderate to severe chronic kidney disease. Sci Rep (2021) 11(1):15877. doi: 10.1038/s41598-021-95488-4
27. Abdallah E, Mosbah O, Khalifa G, Metwaly A, El-Bendary O. Assessment of the relationship between serum soluble klotho and carotid intima-media thickness and left ventricular dysfunction in hemodialysis patients kidney res clin pract. Res Clin Pract (2016) 35:42e49. doi: 10.1016/j.krcp.2015.12.006
28. Seiler S, Rogacev KS, Roth HJ, Shafein P, Emrich I, Neuhaus S, et al. Associations of FGF-23 and sKlotho with cardiovascular outcomes among patients with CKD stages 2–4. Clin J Am Soc Nephrol. (2014) 9(6):1049–58. doi: 10.2215/CJN.07870713
29. Inci A, Sari F, Olmaz R, Coban M, Dolu S, Sarikaya M, et al. Soluble klotho levels in diabetic nephropathy: relationship with arterial stiffness. Eur Rev Med Pharmacol Sci (2016) 20(15):3230–7.
30. Castelblanco E, Hernández M, Alonso N, Ribes-Betriu A, Real J, Granado-Casas M, et al. Association of α-klotho with subclinical carotid atherosclerosis in subjects with type 1 diabetes mellitus. Cardiovasc Diabetol (2022) 21(1):207. doi: 10.1186/s12933-022-01640-3
31. Navarro-Gonzalez JF, Donate-Correa J, Muros de Fuentes M, Perez-Hernandez H, Martinez-Sanz R, Mora-Fernandez C. Reduced klotho is associated with the presence and severity of coronary artery disease. Heart (2014) 100:34e40. doi: 10.1136/heartjnl-2013-304746
32. Lim K, Lu TS, Molostvov G, Lee C, Lam FT, Zehnder D, et al. Vascular klotho deficiency potentiates the development of human artery calcification and mediates resistance to fibroblast growth factor 23. Circulation (2012) 125:2243e2255. doi: 10.1161/CIRCULATIONAHA.111.053405
33. Celermajer DS, Sorensen KE, Gooch VM, Spiegelhalter DJ, Miller OI, Sullivan ID, et al. Non-invasive detection of endothelial dysfunction in children and adults at risk of atherosclerosis. Lancet (1992) 340:1111–11153. doi: 10.1016/0140-6736(92)93147-F
34. Li L, Wang Y, Gao W, Yuan C, Zhang S, Zhou H, et al. Klotho reduction in alveolar macrophages contributes to cigarette smoke extract-induced inflammation in chronic obstructive pulmonary disease. J Biol Chem (2015) 290:27890–900. doi: 10.1074/jbc.M115.655431
35. Witkowski JM, Soroczyńska-Cybula M, Bryl E, Smoleńska Z, Jóźwik AJ. Klotho: a common link in physiological and rheumatoid arthritis-related aging of human CD4+ lymphocytes. J Immunol (2007) 178:771–7. doi: 10.4049/jimmunol.178.2.771
36. Karami M, Mehrabi F, Allameh A, Pahlevan Kakhki M, Amiri M, Emami Aleagha MS. Klotho gene expression decreases in peripheral blood mononuclear cells (PBMCs) of patients with relapsingremitting multiple sclerosis. J Neurol Sci (2017) 381:305–7. doi: 10.1016/j.jns.2017.09.012
37. Chávez-Sánchez L, Espinosa-Luna JE, Chávez-Rueda K, Legorreta-Haquet MV, Montoya-Díaz E, Blanco-Favela F. Innate immune system cells in atherosclerosis. Arch Med Res (2014) 45:1–14. doi: 10.1016/j.arcmed.2013.11.007
38. Tabas I, Bornfeldt KE. Macrophage phenotype and function in different stages of atherosclerosis. Circ Res (2016) 118:653–67. doi: 10.1161/CIRCRESAHA.115.306256
39. Lv J, Chen J, Wang M, Yan F. Klotho alleviates indoxyl sulfate-induced heart failure and kidney damage by promoting M2 macrophage polarization. Aging (Albany N Y.) (2020) 12:9139–50. doi: 10.18632/aging.103183
40. Mytych J, Sołek P, Będzińska A, Rusinek K, Warzybok A, Tabęcka-Łonczyńska A, et al. Towards age-related anti-inflammatory therapy: klotho suppresses activation of ER and golgi stress response in senescent monocytes. Cells (2020) 9:261. doi: 10.3390/cells9020261
41. Mytych J, Wos I, Solek P, Koziorowski M. Protective role of klotho protein on epithelial cells upon co-culture with activated or senescent monocytes. Exp Cell Res (2017) 350:358–67. doi: 10.1016/j.yexcr.2016.12.013
42. Zunke F, Rose-John S. The shedding protease ADAM17: physiology and pathophysiology. Biochim Biophys Acta Mol Cell Res (2017) 1864(11 Pt B):2059–70. doi: 10.1016/j.bbamcr.2017.07.001
43. Rizza S, Copetti M, Cardellini M, Menghini R, Pecchioli C, Luzi A, et al. A score including ADAM17 substrates correlates to recurring cardiovascular event in subjects with atherosclerosis. Atherosclerosis (2015) 239(2):459–64. doi: 10.1016/j.atherosclerosis.2015.01.029
44. Sakamuri SSVP, Higashi Y, Sukhanov S, Siddesha JM, Delafontaine P, Siebenlist U, et al. TRAF3IP2 mediates atherosclerotic plaque development and vulnerability in ApoE(-/-) mice. Atherosclerosis (2016) 252:153–60. doi: 10.1016/j.atherosclerosis.2016.05.029
45. Janssen EM, Dy SM, Meara AS, Kneuertz PJ, Presley CJ, Bridges JFP, et al. Analysis of patient preferences in lung cancer – estimating acceptable tradeoffs between treatment benefit and side effects. Patient Prefer Adherence (2020) 14:927–37. doi: 10.1186/1475-2840-11-112
46. Kweon SS, Shin MH, Lee YH, Choi JS, Nam HS, Park KS, et al. Higher normal ranges of urine albumin-to-creatinine ratio are independently associated with carotid intima-media thickness. Cardiovasc Diabetol (2012) 11:112. doi: 10.1186/1475-2840-11-112
Keywords: Klotho, carotid, intima and media thickness, gene expression data, serum, immunohistochemistry
Citation: Donate-Correa J, Martín-Núñez E, Martin-Olivera A, Mora-Fernández C, Tagua VG, Ferri CM, López-Castillo Á, Delgado-Molinos A, López-Tarruella VC, Arévalo-Gómez MA, Pérez-Delgado N, González-Luis A and Navarro-González JF (2023) Klotho inversely relates with carotid intima- media thickness in atherosclerotic patients with normal renal function (eGFR ≥60 mL/min/1.73m2): a proof-of-concept study. Front. Endocrinol. 14:1146012. doi: 10.3389/fendo.2023.1146012
Received: 16 January 2023; Accepted: 26 April 2023;
Published: 19 May 2023.
Edited by:
Jochen Georg Schneider, University of Luxembourg, LuxembourgReviewed by:
Nan Zhu, Shanghai General Hospital, ChinaMaaike Schilperoort, Columbia University, United States
Farzaneh Rostamzadeh, Kerman Medical University, Iran
Copyright © 2023 Donate-Correa, Martín-Núñez, Martin-Olivera, Mora-Fernández, Tagua, Ferri, López-Castillo, Delgado-Molinos, López-Tarruella, Arévalo-Gómez, Pérez-Delgado, González-Luis and Navarro-González. This is an open-access article distributed under the terms of the Creative Commons Attribution License (CC BY). The use, distribution or reproduction in other forums is permitted, provided the original author(s) and the copyright owner(s) are credited and that the original publication in this journal is cited, in accordance with accepted academic practice. No use, distribution or reproduction is permitted which does not comply with these terms.
*Correspondence: Javier Donate-Correa, jdonatecorrea@gmail.com; Juan F. Navarro-González, jnavgon@gobiernodecanarias.org
†These authors have contributed equally to this work
‡These authors share senior authorship