- 1Department of Pharmacy, Nanjing Drum Tower Hospital, Affiliated Hospital of Medical School, Nanjing University, Nanjing, Jiangsu, China
- 2Nanjing Drum Tower Hospital Clinical College of Nanjing University of Chinese Medicine, Nanjing, Jiangsu, China
- 3Nanjing Qlife Medical Technology Co., Ltd, Nanjing, Jiangsu, China
Background: Abnormally changed steroid hormones during pregnancy are closely related to the pathological process of gestational diabetes mellitus (GDM). Our aim was to systematically profile the metabolic alteration of circulating steroid hormones in GDM women and screen for risk factors.
Methods: This study was a case-control study with data measured from 40 GDM women and 70 healthy pregnant women during their 24-28 gestational weeks. 36 kinds of steroid hormones, including 3 kinds of corticosteroids, 2 kinds of progestins, 5 kinds of androgens and 26 kinds of downstream estrogens in serum were systematically measured using a combined sensitive UPLC-MS/MS method. The flux of different metabolic pathways of steroid hormones was analyzed. Logistic regression and ROC curve model analyses were performed to identify potential steroid markers closely associated with GDM development.
Results: Serum corticosteroids, progestins and almost all the estrogen metabolites via 16-pathway from parent estrogens were higher in GDM women compared with healthy controls. Most of the estrogen metabolites via 4-pathway and more than half of the metabolites via 2-pathway were not significantly different. 16α-hydroxyestrone (16OHE1), estrone-glucuronide/sulfate (E1-G/S) and the ratio of total 2-pathway estrogens to total estrogens were screened as three indicators closely related to the risk of GDM development. The adjusted odds ratios of GDM for the highest quartile compared with the lowest were 72.22 (95% CI 11.27-462.71, Ptrend <0.001) for 16OHE1 and 6.28 (95% CI 1.74-22.71, Ptrend <0.05) for E1-G/S. The ratio of 2-pathway estrogens to total estrogens was negatively associated with the risk of GDM.
Conclusion: The whole metabolic flux from cholesterol to downstream steroid hormones increased in GDM condition. The most significant changes were observed in the 16-pathway metabolism of estrogens, rather than the 2- or 4-pathway or other types of steroid hormones. 16OHE1 may be a strong marker associated with the risk for GDM.
1 Introduction
Gestational diabetes mellitus (GDM) is an idiopathic complication during pregnancy, characterized primarily by hyperglycemia first diagnosed during pregnancy. It is estimated that GDM affects more than 20 million live births worldwide (1). The prevalence of GDM varies from 1% to 28% in different countries and regions. Compared with European pregnant women, Asian pregnant women were found to have a higher incidence of GDM (2, 3). However, since the lack of uniformity in screening method and diagnosis criteria for GDM, the comparison on the prevalence of GDM between and within countries needs to be further discussed (4).
In terms of short-term effects, GDM can cause high blood pressure during pregnancy, high fetal weight and premature delivery (5). In the later long-term follow-up of women with GDM, they are at higher risk of developing type 2 diabetes (T2D) (6, 7), hypertension (8) and other cardiovascular events (9–12) than those without GDM. Moreover, emerging evidence tends to suggest that GDM also has profound influences on the health of offspring, whether short or long term. The newborns of GDM mothers may develop macrosomia, hypoglycemia, respiratory distress syndrome and some other severe complications (13). Henceforth, they may also risk long-term health problems including insulin resistance (IR), subsequent obesity, T2D, and increased neuropsychiatric morbidity (14).
At present, the pathogenesis of GDM is not very clear. IR, genetic susceptibility, metabolic disorders, and the interaction of complex factors are proved to be closely associated with GDM. In general, IR is considered to underlie the pathophysiology of GDM (15). IR may occur during pregnancy due to impairment of pancreatic islet β-cell function, which makes it fail to compensate for insulin. Current studies suggested other potential factors related to IR in pregnant women, including levels of steroid hormones, placental hormones, and inflammatory mediators, which function as antagonists to insulin (16).
As we all know, steroid hormones exert strong biological activities in our bodies via specific receptors. They could be divided into four classes according to the structures: estrogens, progestins, androgens and corticosteroids. These steroid hormones are closely linked in a metabolic network originated from cholesterol. Steroid hormones could affect insulin function in diverse aspects. For instance, progesterone, cortisol and estrogens were found to affect β-cell function or the sensitivity of peripheral tissues to insulin (17). CAR-mediated signaling pathway was confirmed to be involved in the influence of estrogens and progestins on IR (18). Progesterone may exert a toxic effect on pancreatic β-cells through an oxidative-stress-dependent mechanism that induces apoptosis (19). Hence, the abnormal changes of steroid hormone levels (e.g. testosterone, estradiol and progesterone, etc.) observed in women with GDM (19–24) are correlated with the occurrence and development of IR. Remarkably, most existing studies focused on several or several types of precursor steroid hormones, which are confirmed to have high biological activities and could be easily measured. However, in the metabolic network of steroid hormones, there are dozens of structural analogues with similar or opposite physiological activity (Figure 1). Changes of steroid levels are not only related to the activities of upstream or downstream enzymes but also to the levels of intermediates in the pathways. Focusing only on a few steroid hormones makes it difficult to trace the cause of metabolic changes or to reveal the potential mechanism of GDM pathogenesis. Precursor steroid hormones could be metabolized through reduction, oxidation, methylation, glucuronidation or other metabolic pathways in vivo (25). Despite the relative low levels of some metabolites, they are proved to be involved in some physiological processes, such as fat disposition regulation and muscle mass promotion (26, 27). At present, there is still a lack of research on the overall metabolic network of steroid hormones in GDM pregnant women. The relationship between steroid metabolites and GDM is still unclear.
This study aims to systematically profile the changes of steroid metabolic network in pregnancy with GDM by employing a combined sensitive ultra-performance liquid chromatography-tandem mass spectrometry (UPLC-MS/MS). For the first time, the overall metabolic characteristics of steroid hormones in pregnant women with GDM were analyzed and summarized through quantifying 36 kinds of steroid hormones, including 3 kinds of corticosteroids, 2 kinds of progestins, 5 kinds of androgens and 26 kinds of downstream estrogens and their metabolites. This work contributes to further disclosing the correlation between the metabolic disorders of steroid hormones and the pathogenesis of GDM.
2 Materials and methods
2.1 Chemicals and materials
Estradiol (E2), estrone (E1) and estriol (E3) were purchased from China National Institutes for Food and Drug Control (Beijing, China). 2-Hydroxyestrone (2OHE1), 2-hydroxyestradiol (2OHE2), 2-methoxyestrone (2MeOE1), 2-methoxyestradiol (2MeOE2), 4-methoxyestrone (4MeOE1), 4-methoxyestradiol (4MeOE2), 16α-hydroxyestrone (16OHE1), 16-epiestriol (16EpiE3), 17-epiestriol (17EpiE3), aldosterone (ALD) and internal standards (deuterated steroid hormones) were purchased from TRC (Toronto, Canada). Progesterone (P4), 17α-hydroxyprogesterone (17α-OHP4), testosterone (T), dihydrotestosterone (DHT), androstenedione (AD), corticosterone (CORT) and cortisol (F) were purchased from Dr. Ehrenstorfer (Augsburg, Germany). Dehydroepiandrosterone (DHEA) was purchased from Cerilliant (Round Rock, TX, USA). Dehydroepiandrosterone sulfate (DHEA-S) was purchased from ISOscience (Ambler, PA, USA). 4-Hydroxyestrone (4OHE1), dansyl chloride, L-ascorbic acid, and β-glucuronidase/arylsulfatase were obtained from Sigma-Aldrich (St. Louis, MO). Methanol (HPLC grade) and formic acid were purchased from Merck (Darmstadt, Germany) or Fisher (Waltham, MA, USA). Deionized water was prepared using a Milli-Q system (Millipore, Milford, MA, USA). Acetone was obtained from Nanjing Chemical Reagent CO., LTD.
2.2 Study population and sample collection
This study was approved by the ethical committee of Drum Tower Hospital Affiliated to Medical School of Nanjing University (No.2021-021-01) and was registered to ChiCTR (identifier: ChiCTR2100048675). Written consent has been obtained from each patient or subject after full explanation of the purpose and nature of all procedures used. All women in this study had singleton pregnancies and underwent routine 75-g oral glucose tolerance test (OGTT) screening at 24-28 gestational weeks. GDM was diagnosed based on IADPSG guideline to meet one or more of the following diagnostic criteria (28): (1) fasting blood glucose≥5.1 mmol/L; (2) 1 h≥10.0 mmol/L; (3) 2 h≥8.5 mmol/L. The final study population was determined after excluding pregnant women with any of the following characteristics: (1) <18 years of age; (2) severe maternal or fetal illness (malignancy, decompensated liver disease, heart disease, hypertension, congenital anomaly, etc.); (3) pre-existing diabetes mellitus or overt diabetes; (4) polycystic ovarian syndrome; (5) presence of drug use that interferes with steroid metabolism (progestin drugs); (6) non-singleton pregnancies; (7) newborns diagnosed with congenital anomaly (heart disease, neurological disease, metabolic diseases, etc.) or missing health assessment information for the newborns. Finally, a total of 40 GDM women and 70 healthy pregnant women aged 26–42 were recruited for analysis.
2.3 Anthropometric and biochemical measurements
A detailed medical history including age, gestational age, height and other basic information was recorded for each participant. Maternal weight at 24-28 weeks of gestation was measured by the standard method. The pre-pregnancy weight was recorded according to patient statements. BMI value was calculated by dividing the weight (kilograms) by the square of height (meter2). Each participant underwent a 75-g OGTT after an overnight fast of 10–12 hours. Blood lipids (triglycerides (TG), total cholesterol (TC), high-density lipoprotein (HDL), and low-density lipoprotein (LDL) and liver enzymes, including aminotransferase (ALT), aspartate aminotransferase (AST), g-glutamyl transferase (GGT) and plasma glucose were measured by using a biochemical autoanalyzer (Beckman, CA, USA).
2.4 Quantification of the serum steroid hormones based on UPLC-MS/MS method
Steroid hormones in serum samples collected at the at 24-28 weeks of gestation were determined using a Waters UPLC I-Class system interfaced with a Xevo TQ-S triple quadrupole mass spectrometer (Waters Corp., Milford, MA, USA). This method validation was performed according to the Guidelines for Bio-analytical Method Validation. For the determination of estrogens, mass spectrometry was performed in positive mode and multiple reaction monitoring (MRM). Liquid chromatography separation was achieved on a Waters CORTECS C18 column (2.1 × 150 mm, 1.6 μm) at 40°C. The flow rate was 0.3 mL/min. The mobile phase consisted of solvent A, deionized water (containing 0.05% formic acid and 2 mM ammonium acetate) and solvent B, methanol. The elution gradient was as follows: 0.0-4.1min, isocratic 78% B; 4.1-4.2 min, linear gradient 78-81% B; 4.2-8.0 min, isocratic 81% B; 8.0-8.01min, linear gradient 81-78% B; 8.01-10.0min, isocratic 78% B. The total run time was 10.0 min. For the determination of corticosteroids, progestins and androgens, mass spectrometry was performed in a positive and negative ion switching mode and MRM. Liquid chromatography separation was achieved on a Phenomenex Kinetex XB-C18 (3.0 × 50 mm, 2.6 µm) at 40°C. The flow rate was 0.3 mL/min. The mobile phase consisted of solvent A, deionized water (containing 100 μM ammonium fluoride) and solvent B, methanol. The elution gradient was as follows: 40% B increased to 98% B from 0.0 min to 3.0 min, maintained for 0.5 min, retuned to initial conditions, and then re-equilibrated for 2.5 min. The total run time was 6.0 min.
Sample preparation procedure was performed as previously described (29, 30). Briefly, charcoal-stripped human serum with no detectable levels of any steroid hormones was used for preparation of calibration standards and quality control samples. Free estrogens, conjugated (-glucuronide/sulfate, -G/S) estrogen metabolites and other steroid hormones were directly or indirectly measured through preparation procedures with or without β-glucuronidase/sulfatase hydrolysis step. Serum samples and enzymatically hydrolyzed serum samples underwent liquid-liquid extraction with methyl tert-butyl ether. After extraction, the organic solvent portion was transferred and evaporated to dryness at 60°C under nitrogen gas. For the determination of estrogens, the dried samples then underwent the derivatization process using dansyl chloride. All residue samples were reconstituted with methanol and analyzed by UPLC-MS/MS.
2.5 Statistical analysis
The normality of values was analyzed by Shapiro-Wilk test. Values with normal distribution were summarized as the mean ± standard deviation (SD), and the corresponding significance was examined by using the Student’s t-test. Values with non-normality were summarized as the median (interquartile range, IQR), and significance was examined by Mann-Whitney U test. P < 0.05 was considered significant. Volcano graph was drawn by GraphPad Prism 8.0 to screen for the obvious changes of serum steroid hormones in GDM women compared to healthy pregnant women. A mixed-effects Least Absolute Shrinkage Selection Operator (LASSO) logistic regression, implemented by STATA/MP version 16.0, was used for multicollinearity elimination and variable selection. Receiver operating characteristic (ROC) curves were plotted to classify the performance of biomarkers. The most relevant steroid hormones are grouped into quartiles to determine the linear trend relationship between the independent variables and the prevalence of GDM.
3 Results
3.1 Establishment and method validation of UPLC–MS/MS analysis
A combined sensitive and rapid UPLC-MS/MS method was developed to profile the steroid network in GDM women. 23 kinds of steroid hormones, including 5 kinds of upstream C21 steroid hormones (corticosteroids and progestins), 5 kinds of C19 steroid hormones (androgens) and 13 kinds of downstream C18 steroid hormones (estrogens), were directly measured through the UPLC-MS/MS method. 13 kinds of conjugated Phase II metabolites were indirectly measured by the β-glucuronidase/sulfatase hydrolysis method. As shown in Figure 2, we optimized the elution gradient of chromatographic mobile phases to achieve rapid separation of multiple isomers. The peaks for each steroid appeared sharp and symmetrical. The total running time kept within 6 mins and 10 mins. This method validation was performed according to the guidelines for Bio-analytical Method Validation published by the US FDA and fulfilled the acceptance criteria, as shown in Supplementary Tables S1–4. The linear range of the measured steroid hormones was as follows: estrogens (0.001-2 ng/mL), T (0.05-10 ng/mL), DHT (0.025-5 ng/mL), 17α-OHP4(0.05-10 ng/mL), P4 (0.05-10 ng/mL), CORT (0.1-20 ng/mL), DHEA (0.1-20 ng/mL), AD (0.05-10 ng/mL), F (1-200 ng/mL), ALD (0.01-2 ng/mL), DHEA-S (50-10000 ng/mL).
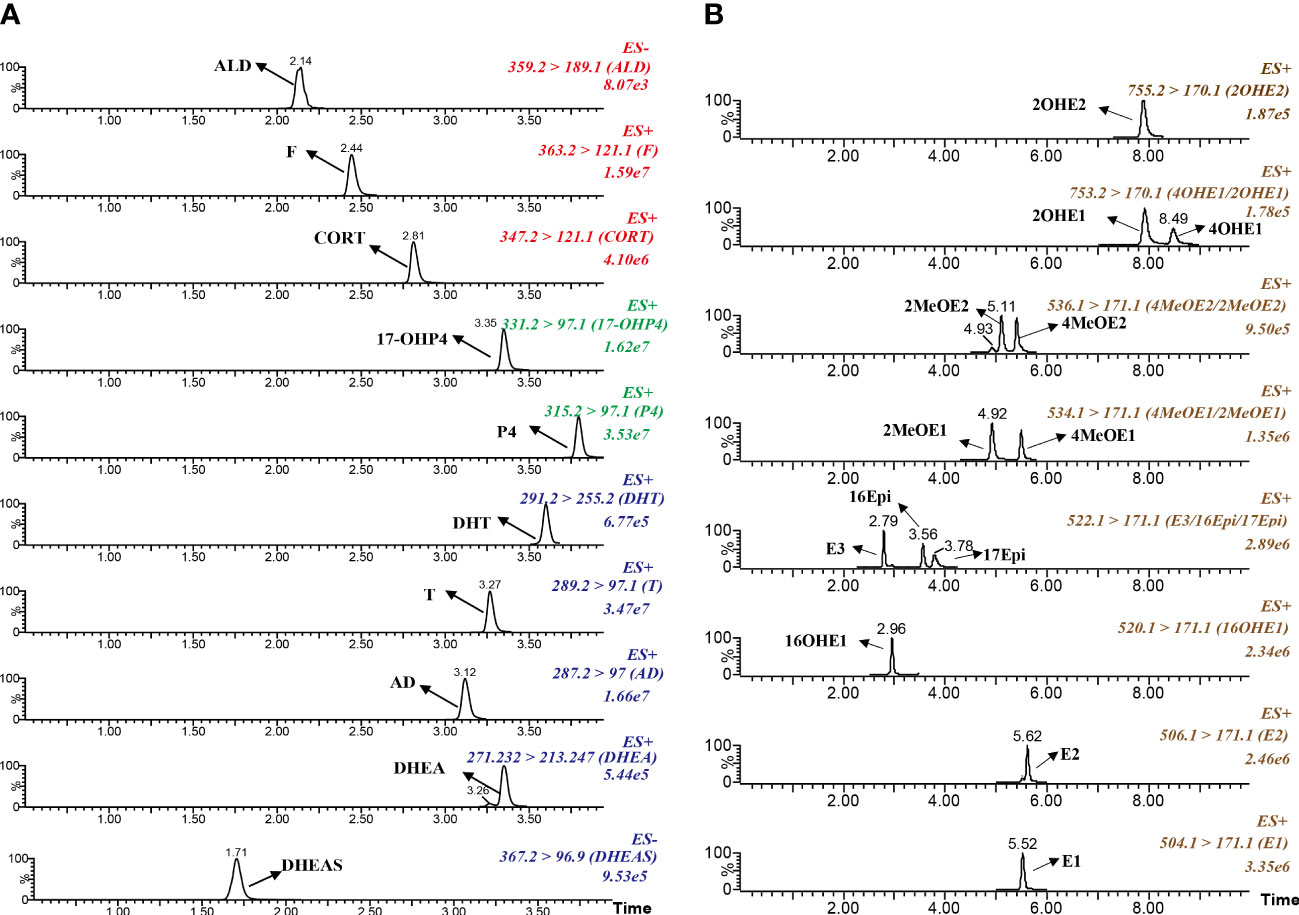
Figure 2 Typical chromatograms of multiple reaction monitoring for target steroid hormones. (A) Corticosteroids, progestins and androgens. (B) Estrogens and the metabolites.
3.2 Monitoring parameters
A total of 110 pregnant women participated in this study, including 40 GDM women and 70 healthy pregnant women. The baseline characteristics of the GDM group and corresponding controls are shown in Table 1. There was no statistical significance in age and weight between GDM and healthy pregnant women. GDM women had higher BMI values and higher levels of serum fasting and post-load glucose, TG and HDL compared with healthy pregnant women. The liver function index and other serum lipids were comparable between the two groups.
3.3 Profiling of serum steroid hormones in women with GDM
The levels of 36 steroid hormones measured by UPLC-MS/MS are presented in Table 2. Briefly, in addition to androgens, there was a statistically significant increase in serum corticosteroid, progestin and some downstream estrogen levels of GDM women, compared with the healthy. The levels of serum androgens are roughly comparable. Changes in serum concentrations of progestins and corticosteroids were relatively mild between GDM and healthy women, except for 17-OHP4 (p<0.05, FC 1.6), an active intermediate derived from P4 via 17-hydroxylase.
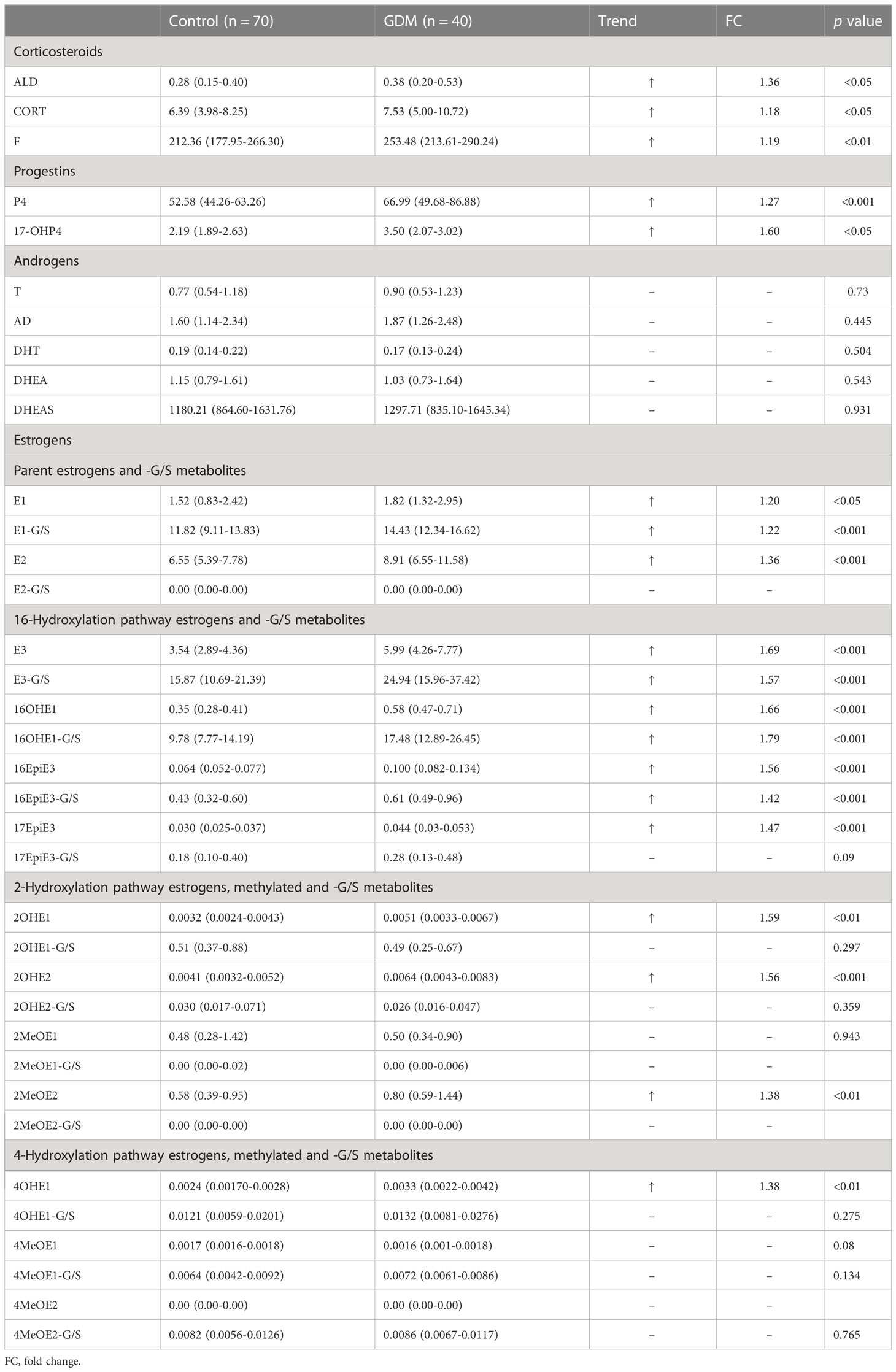
Table 2 The concentrations of serum steroids and metabolites in GDM women and healthy pregnant women.
Among all changed steroid hormones, estrogens showed relatively large changes. Among all kinds of estrogens measured, 3 kinds of conjugated metabolites, including E1-G/S, E3-G/S and 16OHE1-G/S, have absolute advantages in serum concentrations. The median concentrations of serum parent estrogens (E1, p<0.05; E2, p<0.001) and the conjugate metabolite (E1-G/S, p<0.001) in GDM women were higher than those in healthy women. E1-G/S was nearly 10 times higher in serum than its precursor E1. However, E2-G/S was not detected in serum samples. Interestingly, almost all metabolites via the 16-hydroxylation pathway from parent estrogens were higher in GDM women. Among these 16-hydroxylated metabolites, the median concentrations of 5 metabolites, including E3, E3-G/S, 16OHE1, 16OHE1-G/S, and 16EpiE3 (p<0.001), changed more than 1.5 times in the serum of GDM women compared with healthy women. In contrast, most estrogen metabolites via 4-hydroxylation pathway and more than half of the metabolites via 2-hydroxylation pathway were not significantly different in serum between the two groups. Besides, 2MeOE2-G/S and 4MeOE2 were not detected in all serum samples. 2MeOE1-G/S was not detected in more than 80% of serum samples.
From the perspective of metabolic flux, there was a significantly higher flux of hydroxylation metabolism of estrogens (p<0.001, FC 1.55) in GDM women, and this elevation of hydroxylation was mainly contributed by 16- hydroxylation pathway, as shown in Table 3. No significant difference was observed in the total calculated concentration of metabolites via 2- or 4-hydroxylation pathways. In addition, the metabolic ratios of 2- and 4-pathways to total estrogens decreased significantly in GDM women.
3.4 Correlation analysis reveal potential steroid markers associated with GDM
As shown in Figures 3, 4, a total of 23 kinds of steroid indicators were screened out according to Student’s t-test results and fold change values (p<0.05, FC>1.2). ROC analysis was further employed to preliminarily evaluate the diagnostic performance of serum metabolites in the discrimination of GDM from controls. The area under the curve (AUC) values of 9 directly measured steroid hormones (16OHE1, 16OHE1-G/S, E3, E1-G/S, E2, 16-EpiE3-G/S, 17-EpiE3, 16-epiE3 and 2OHE2) and 4 indirectly calculated indicators (total estrogens, conjugated estrogens, total hydroxylated estrogens, and total 16-hydroxylated estrogens) achieved good AUC values (>0.7), which indicated good diagnostic efficacy and close correlation. Among these indicators, 16OHE1 achieved the highest AUC value of 0.85. Based on all quantitative results, a combined model was established through LASSO logistic regression considering the basic biochemical characteristics, directly measured and indirectly calculated steroid indicators to eliminate the multicollinearity and find the specific markers associated with GDM. With parameter lambda of 0.05 obtained by cross-validation, we identified a diagnostic panel of 5 elements, including BMI, TG, 16OHE1, E1-G/S and the ratio of total 2-pathway estrogens to total estrogens. The equation Logit (P) = 0.172*BMI+0.438*TG+0.104*E1-G/S+7.381*16OHE1-29.131* ratio (2-pathway: total)-10.172. The AUC-ROC of the model was 0.89, which indicated that the screened markers are closely related to the development of GDM.
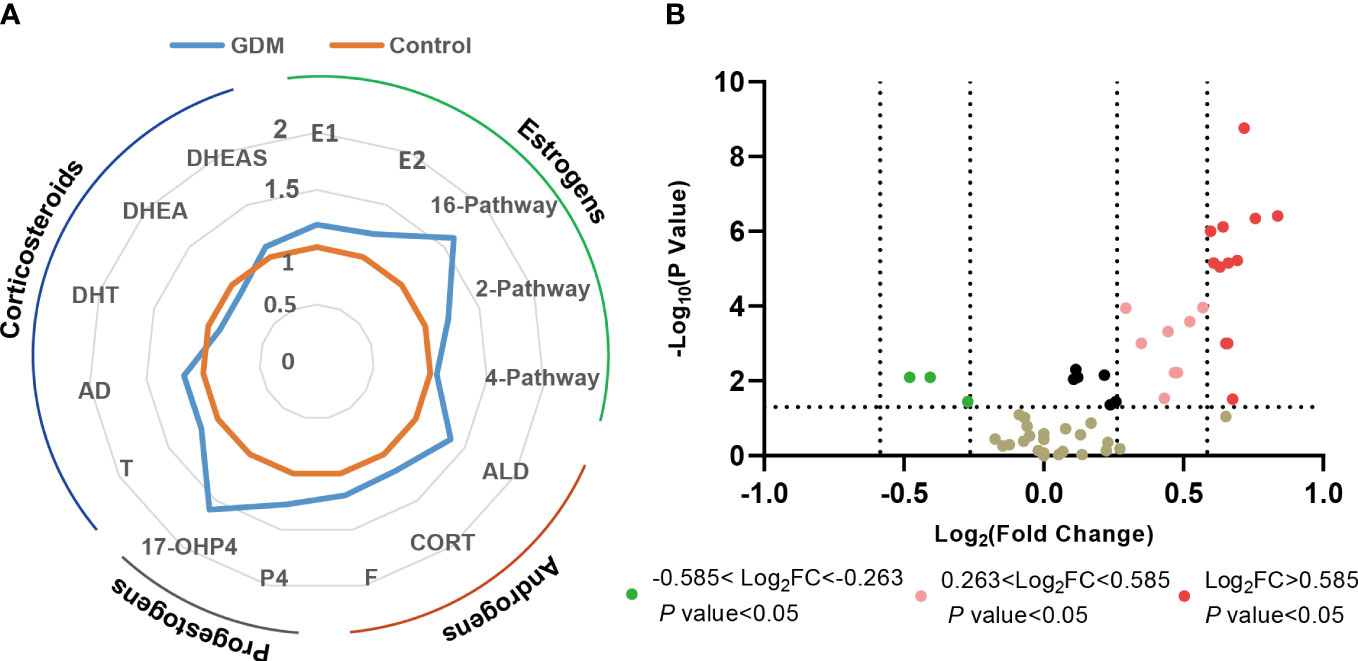
Figure 3 Changes of steroid metabolism in GDM women. (A) Phase diagrams showed the changes in different types of serum steroid hormones in GDM women compared with the health controls. (B) Volcano plot of serum steroid hormones in GDM women compared with the health controls. FC, fold change.
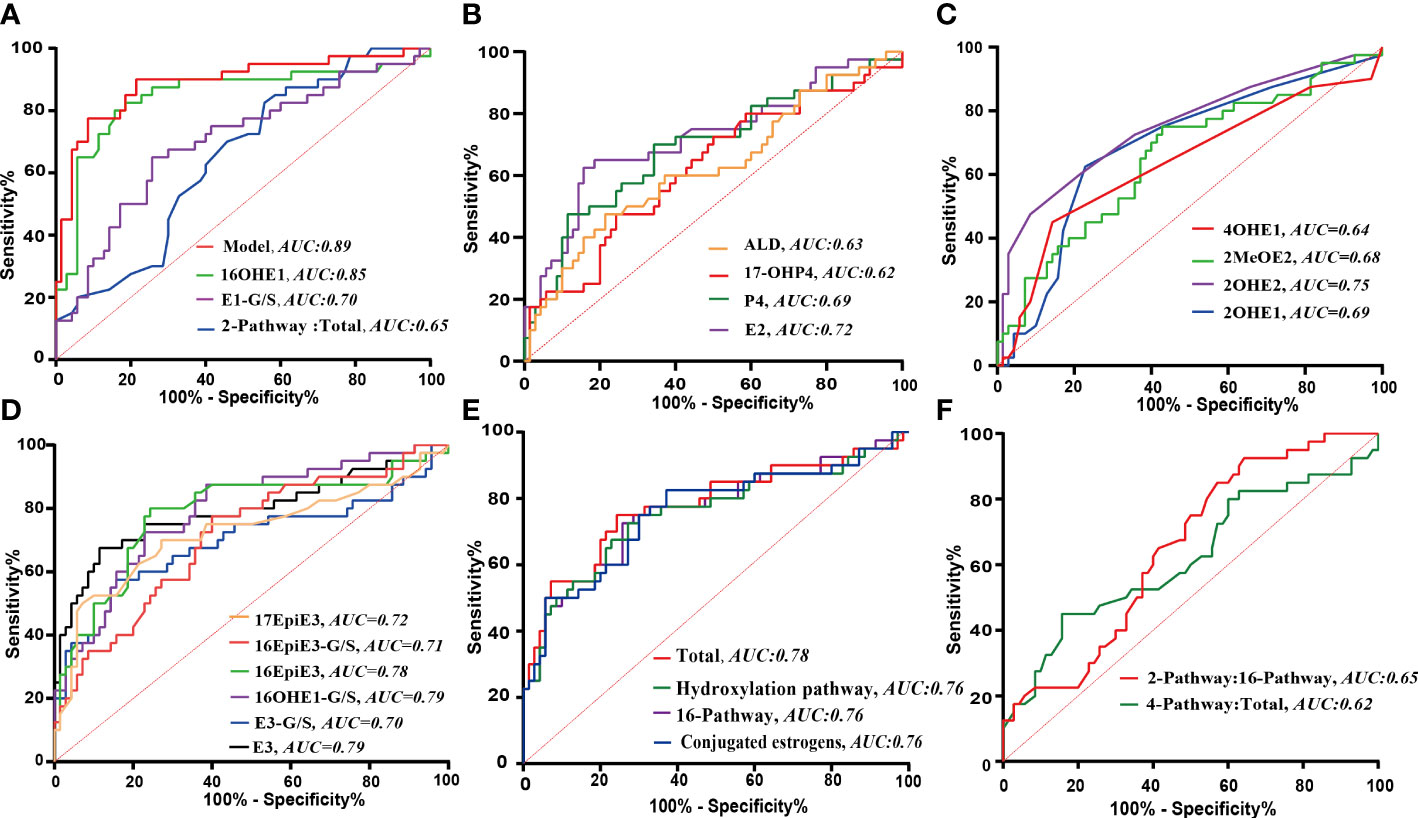
Figure 4 The ROC analyses of significantly changed indicators. (A) ROC curves of the combination model and the screened individual elements. (B–F) ROC curves of the steroid indicators with statistically significant change in GDM. B: Progestins, androgens and parent estrogens; C: Estrogens in 2- or 4- pathway; D: Estrogens in 16- pathway; E: Calculated pathway flux; F: Calculated pathway ratio.
To further explore the correlation between the screened steroid hormones and the risk of GDM, the concentrations of serum 16OHE1, E1-G/S and the ratio of 2-pathway metabolites to total estrogens were stratified into quartiles to perform binary logistic regression analysis, as shown in Table 4. It was observed that 16OHE1 and E1-G/S were significantly positively correlated with GDM risk. This association was also enhanced in the age, BMI and TG–adjusted models. The age, BMI and TG adjusted odds ratios (ORs) of GDM for the highest quartile compared with the lowest were 72.22 (95% CI 11.27-462.71, Ptrend <0.001) for 16OHE1 and 6.28 (95% CI 1.74-22.71, Ptrend< 0.05) for E1-G/S. Besides, the ratio of total 2-pathway estrogens to total estrogens was negatively associated with the risk for GDM, whether with or without adjustment for potential confounders. After adjustment by potential confounders, the negative association between the ratio of total 2-pathway estrogens to total estrogens and the risk for GDM was not significant (adjusted OR for the highest versus the lowest quartile, 0.65; Ptrend= 0.108).
4 Discussion
So far, various factors related to the pathogenesis and development of GDM have been investigated. Although the occurrence of IR in pregnant women may be closely related to the levels of steroid hormones, there is no data available on the alteration of the whole steroid metabolic network during the pregnancy process of GDM. Limited studies reported several kinds of serum steroid hormones in GDM patients based on immunoassay with lower specificity. Due to the different commercial kits, the results vary across laboratories (31–33) and the types of analyzed steroid hormones are limited. Hence, some potentially important steroid hormones have not been fully explored.
Generally, large amounts of progestogens and estrogens are produced in the mother’s body to adapt to the homeostasis during pregnancy (34). Claudio Villarroel et al. (24) studied 24 GDM cases and 24 control women during the second half of pregnancy and found that GDM pregnant women had lower E1 and E2 serum levels but a higher T level. Junguk Hur et al. (35) showed that an early second trimester serum unconjugated E3>95th percentile of the values generated from the overall screen population was associated with an increased risk for GDM (OR 2.05, p<0.001). However, conjugated E3 was not detected in the study. As a supplement, our results indicated that both conjugated and unconjugated E3 levels in GDM women were significantly higher than those in the healthy pregnant controls. Consistent with the previous findings that GDM women had higher P4 levels (36), our results demonstrated that GDM women also had significantly higher levels of 17-OHP4 (an active metabolite of P4) compared to the healthy pregnancy controls.
Abnormal levels of steroid hormones have been reported to modulate pancreatic function and susceptibility to develop IR (37). Vejrazkova D et al. (38) proposed that the effect of progesterone was probably related to the increase of IR due to a reduction of GLUT4 expression. Another study provided new insights that progesterone can be toxic to β-cells through oxidative stress mechanism that induces apoptosis (19). Besides, estradiol was proved to exert effects on carbohydrate metabolism and showed bidirectional regulation effects in many studies. Estradiol was found to prevent IR in female mice (39). However, estradiol at a high level could suppress GLUT4 gene expression (40), which may contribute to the process of IR. For a long time, the research on the relationship between estrogens and IR is limited and inconclusive.
Our results suggested that the risk for GDM seemed to be significantly elevated with increased 16-pathway metabolism and decreased 2-pathway metabolism. It is worth mentioning that 16OHE1 may be a strong marker associated with the risk for GDM (adjusted OR for the highest versus the lowest quartile, 72.22; Ptrend < 0.001). 16OHE1 is a metabolite derived from 16-hydroxylase metabolism of estrone and could exert potent biological estrogenic effects. Most studies focused on exploring the relationship between 16OHE1 and breast cancer or other gynecological cancer (41). The ratio of 2OHE1 to 16OHE1 is inversely correlated with the risk for breast and cervical cancer. Hence, 16OHE1 is traditionally considered to be a harmful estrogen metabolite, while 2-hydroxylation of estrogen is contrary. The potential mechanisms include that high level of 16OHE1 could exert a strong biological estrogenic effect, which may promote cytotoxicity and cause cell changes or apoptosis (42). To some extent, this viewpoint is consistent with our results. However, further studies are needed to clarify the relationship between 16OHE1 and the pathological process of GDM or the destruction of β cell function. Besides, the gene polymorphisms of CYP1A1 and CYP1B1 were reported to be involved in regulating the ratio of 2OHE1 to 16OHE1 in vivo (43, 44), and their correlation with the pathogenesis of GDM still needs further clarification.
As our results indicated, E1-G/S is closely related to the risk of GDM. E1-G/S represents the metabolites of glucuronidation and sulfation of E1. Previous studies revealed that UGT1A10 and SULT1E1 are key enzymes involved in E1 glucuronidation and sulfation, respectively (45, 46). UGT1A10 is an extrahepatic enzyme and is exclusively expressed in gastrointestinal tract, kidneys, etc. SULT1E1 has the highest affinity for E1, E2 and catechol estrogens and is expressed in lung, liver and kidneys. The expression of SULT1E1 is highly related to breast and endometrial cancers since it could inactivate estrogens in vivo. E1-S was supposed to give a better indication of the extent of aromatase inhibition than E1 or E2 (47). Besides, genetic polymorphisms of SULT1E1 and UGT1A10 (e.g., SULT1E1 rs11569705, rs11569705; UGT1A10 rs2741049) have been validated to be associated with a significant change in enzyme activity or protein level (48, 49). So far, however, no research has been performed on analyzing the expressions, activities, and gene polymorphisms of UGT1A10 and SULT1E1 in GDM condition. Whether the elevation of serum E1-G/S concentration is related to the expressions or activities of the metabolic enzymes needs to be further confirmed, and the specific structures of the glucuronidation and sulfation metabolites changed in GDM need to be clarified. However, this study also has limitations, it is of great clinical significance to further confirm whether the steroid hormone indicators including 16OHE1 and E1-G/S exhibit similar changes in the first trimester of pregnancy, which may make the early prediction of GDM possible.
In summary, for the first time, the overall metabolic characteristics of steroid hormones in GDM women at second trimester during pregnancy were analyzed and summarized through quantifying 36 kinds of steroid hormones. Serum corticosteroids, progestins and especially the estrogen metabolites via 16-pathway from parent estrogens were higher in GDM women compared with healthy pregnant controls. Three estrogen-related markers, especially 16OHE1, may be closely associated with the risk for GDM. It is suggested that more attention should be paid to estrogen metabolism during pregnancy. Further research on the role of estrogen metabolites in the pathological process of GDM should be implemented.
Data availability statement
The original contributions presented in the study are included in the article/Supplementary Material. Further inquiries can be directed to the corresponding authors.
Ethics statement
The studies involving human participants were reviewed and approved by the ethical committee of Drum Tower Hospital Affiliated to Medical School of Nanjing University. The patients/participants provided their written informed consent to participate in this study.
Author contributions
All authors participated in the interpretation of the study results and in the drafting, critical revision, and approval of the final version of the manuscript. NY, CJ, and HZ were involved in the study design and data analyses. JG and XZ were involved in subject recruitment and sample collection. JH, ML, and WZ were responsible for sample processing, data analyses and plotting. TZ, MW, and CJ were responsible for study monitoring. All authors contributed to the article.
Funding
The project was supported by the National Natural Science Foundation of China (No.82204501, 82204846), Jiangsu Research Hospital Association for Precision Medication (No. JY202109), Nanjing Pharmaceutical Association-Changzhou SiYao Hospital Pharmaceutical Research Fund (2021YX001), Jiangsu Commission of Health Research Fund (No. Z2020053).
Acknowledgments
We acknowledge the Nanjing Medical Center for Clinical Pharmacy for their support.
Conflict of interest
Author WZ and ML are employed by Nanjing Qlife Medical Technology Co., Ltd.
The remaining authors declare that the research was conducted in the absence of any commercial or financial relationships that could be construed as a potential conflict of interest.
Publisher’s note
All claims expressed in this article are solely those of the authors and do not necessarily represent those of their affiliated organizations, or those of the publisher, the editors and the reviewers. Any product that may be evaluated in this article, or claim that may be made by its manufacturer, is not guaranteed or endorsed by the publisher.
Supplementary material
The Supplementary Material for this article can be found online at: https://www.frontiersin.org/articles/10.3389/fendo.2023.1196935/full#supplementary-material
Abbreviations
16EpiE3, 16-epiestriol; 16OHE1, 16α-hydroxyestrone; 16OHE1, 16α-hydroxyestrone; 17EpiE3, 17-epiestriol; 17α-OHP4, 17α-hydroxyprogesterone; 2MeOE1, 2-methoxyestrone; 2MeOE2, 2-methoxyestradiol; 2OHE1, 2-Hydroxyestrone; 2OHE2, 2-hydroxyestradiol; 4MeOE1, 4-methoxyestrone; 4MeOE2, 4-methoxyestradiol; 4OHE1, 4-Hydroxyestrone; AD, androstenedione; ALD, aldosterone; ALT, aminotransferase; AST, aspartate aminotransferase; CORT, corticosterone; DHEA, Dehydroepiandrosterone; DHEA-S, Dehydroepiandrosterone sulfate; DHT, dihydrotestosterone; E1, estrone; E1-G/S; estrone-glucuronide/sulfate; E2, estradiol; E3, estriol; F, cortisol; GDM, gestational diabetes mellitus; GGT, g-glutamyl transferase; HDL: high-density lipoprotein; IQR, interquartile range; IR, insulin resistance; LASSO, Least Absolute Shrinkage Selection Operator; LDL, low-density lipoprotein; MRM, multiple reaction monitoring; OGTT, oral glucose tolerance test; P4, progesterone; ROC, Receiver operating characteristic; SD, standard deviation; T, testosterone; T2D, type 2 diabetes; TC, total cholesterol; TG, triglycerides; UPLC-MS/MS, ultra-performance liquid chromatography-tandem mass spectrometry.
References
1. Saravanan P. Gestational diabetes: opportunities for improving maternal and child health. Lancet Diabetes Endocrinol (2020) 8:793–800. doi: 10.1016/S2213-8587(20)30161-3
2. Nguyen CL, Pham NM, Binns CW, Duong DV, Lee AH. Prevalence of gestational diabetes mellitus in Eastern and southeastern Asia: a systematic review and meta-analysis. J Diabetes Res (2018) 2018:6536974. doi: 10.1155/2018/6536974
3. Eades CE, Cameron DM, Evans JMM. Prevalence of gestational diabetes mellitus in Europe: a meta-analysis. Diabetes Res Clin Pract (2017) 129:173–81. doi: 10.1016/j.diabres.2017.03.030
4. Kuo CH, Li HY. Diagnostic strategies for gestational diabetes mellitus: review of current evidence. Curr Diabetes Rep (2019) 19:155. doi: 10.1007/s11892-019-1271-x
5. Sferruzzi-Perri AN, Lopez-Tello J, Napso T, Yong HEJ. Exploring the causes and consequences of maternal metabolic maladaptations during pregnancy: lessons from animal models. Placenta (2020) 98:43–51. doi: 10.1016/j.placenta.2020.01.015
6. Napoli A, Sciacca L, Pintaudi B, Tumminia A, Dalfrà MG, Festa C, et al. Et al: screening of postpartum diabetes in women with gestational diabetes: high-risk subgroups and areas for improvements-the STRONG observational study. Acta Diabetol (2021) 58:1187–97. doi: 10.1007/s00592-021-01707-9
7. Bellamy L, Casas JP, Hingorani AD, Williams D. Type 2 diabetes mellitus after gestational diabetes: a systematic review and meta-analysis. Lancet (2009) 373:1773–9. doi: 10.1016/S0140-6736(09)60731-5
8. Tobias DK, Hu FB, Forman JP, Chavarro J, Zhang C. Increased risk of hypertension after gestational diabetes mellitus: findings from a large prospective cohort study. Diabetes Care (2011) 34:1582–4. doi: 10.2337/dc11-0268
9. Tobias DK, Stuart JJ, Li S, Chavarro J, Rimm EB, Rich-Edwards J, et al. Association of history of gestational diabetes with long-term cardiovascular disease risk in a Large prospective cohort of US women. JAMA Internal Med (2017) 177:1735–42. doi: 10.1001/jamainternmed.2017.2790
10. Daly B, Toulis KA, Thomas N, Gokhale K, Martin J, Webber J, et al. Correction: increased risk of ischemic heart disease, hypertension, and type 2 diabetes in women with previous gestational diabetes mellitus, a target group in general practice for preventive interventions: a population-based cohort study. PloS Med (2019) 16:e1002881. doi: 10.1371/journal.pmed.1002881
11. Kramer CK, Campbell S, Retnakaran R. Gestational diabetes and the risk of cardiovascular disease in women: a systematic review and meta-analysis. Diabetologia (2019) 62:905–14. doi: 10.1007/s00125-019-4840-2
12. Lowe WL Jr., Lowe LP, Kuang A, Catalano PM, Nodzenski M, Talbot O, et al. Et al: maternal glucose levels during pregnancy and childhood adiposity in the hyperglycemia and adverse pregnancy outcome follow-up study. Diabetologia (2019) 62:598–610. doi: 10.1007/s00125-018-4809-6
13. Al-Khalifah R, Al-Subaihin A, Al-Kharfi T, Al-Alaiyan S, Alfaleh KM. Neonatal short-term outcomes of gestational diabetes mellitus in saudi mothers: a retrospective cohort study. J Clin Neonatol (2012) 1:29–33. doi: 10.4103/2249-4847.92241
14. Farahvar S, Walfisch A, Sheiner E. Gestational diabetes risk factors and long-term consequences for both mother and offspring: a literature review. Expert Rev Endocrinol Metab (2019) 14:63–74. doi: 10.1080/17446651.2018.1476135
15. Ma Y, Wang N, Gu L, Wei X, Ren Q, Huang Q, et al. Postpartum assessment of the beta cell function and insulin resistance for Chinese women with previous gestational diabetes mellitus. Gynecol Endocrinol (2019) 35:174–8. doi: 10.1080/09513590.2018.1512094
16. Plows JF, Stanley JL, Baker PN, Reynolds CM, Vickers MH. The pathophysiology of gestational diabetes mellitus. Int J Mol Sci (2018) 19:3342. doi: 10.3390/ijms19113342
17. Kühl C. Etiology and pathogenesis of gestational diabetes. Diabetes Care (1998) 21 Suppl 2:B19–26.
18. Masuyama H, Hiramatsu Y. Potential role of estradiol and progesterone in insulin resistance through constitutive androstane receptor. J Mol Endocrinol (2011) 47:229–39. doi: 10.1530/JME-11-0046
19. Nunes VA, Portioli-Sanches EP, Rosim MP, Araujo MS, Praxedes-Garcia P, Valle MM, et al. Progesterone induces apoptosis of insulin-secreting cells: insights into the molecular mechanism. J Endocrinol (2014) 221:273–84. doi: 10.1530/JOE-13-0202
20. Uzelac PS, Li X, Lin J, Neese LD, Lin L, Nakajima ST, et al. Dysregulation of leptin and testosterone production and their receptor expression in the human placenta with gestational diabetes mellitus. Placenta (2010) 31:581–8. doi: 10.1016/j.placenta.2010.04.002
21. Nadal A, Alonso-Magdalena P, Soriano S, Ropero AB, Quesada I. The role of oestrogens in the adaptation of islets to insulin resistance. J Physiol (2009) 587:5031–7. doi: 10.1113/jphysiol.2009.177188
22. Root-Bernstein R, Podufaly A, Dillon PF. Estradiol binds to insulin and insulin receptor decreasing insulin binding in vitro. Front Endocrinol (Lausanne) (2014) 5:118. doi: 10.3389/fendo.2014.00118
23. Villarroel C, Salinas A, López P, Kohen P, Rencoret G, Devoto L, et al. Anti-müllerian hormone in type 2 and gestational diabetes during the second half of pregnancy: relationship with sexual steroid levels and metabolic parameters. Gynecol Endocrinol (2018) 34:120–4. doi: 10.1080/09513590.2017.1359824
24. Villarroel C, Salinas A, López P, Kohen P, Rencoret G, Devoto L, et al. Pregestational type 2 diabetes and gestational diabetes exhibit different sexual steroid profiles during pregnancy. Gynecol Endocrinol (2017) 33:212–7. doi: 10.1080/09513590.2016.1248933
25. Schiffer L, Barnard L, Baranowski ES, Gilligan LC, Taylor AE, Arlt W, et al. Human steroid biosynthesis, metabolism and excretion are differentially reflected by serum and urine steroid metabolomes: a comprehensive review. J Steroid Biochem Mol Biol (2019) 194:105439. doi: 10.1016/j.jsbmb.2019.105439
26. Wang P, Zhu BT. Unique effect of 4-hydroxyestradiol and its methylation metabolites on lipid and cholesterol profiles in ovariectomized female rats. Eur J Pharmacol (2017) 800:107–17. doi: 10.1016/j.ejphar.2017.02.032
27. Ikeda K, Horie-Inoue K, Inoue S. Functions of estrogen and estrogen receptor signaling on skeletal muscle. J Steroid Biochem Mol Biol (2019) 191:105375. doi: 10.1016/j.jsbmb.2019.105375
28. Metzger BE, Gabbe SG, Persson B, Buchanan TA, Catalano PA, Damm P, et al. Et al: international association of diabetes and pregnancy study groups recommendations on the diagnosis and classification of hyperglycemia in pregnancy. Diabetes Care (2010) 33:676–82. doi: 10.2337/dc10-0719
29. Xu X, Roman JM, Issaq HJ, Keefer LK, Veenstra TD, Ziegler RG. Quantitative measurement of endogenous estrogens and estrogen metabolites in human serum by liquid chromatography-tandem mass spectrometry. Anal Chem (2007) 79:7813–21. doi: 10.1021/ac070494j
30. Ponzetto F, Mehl F, Boccard J, Baume N, Rudaz S, Saugy M, et al. Longitudinal monitoring of endogenous steroids in human serum by UHPLC-MS/MS as a tool to detect testosterone abuse in sports. Anal Bioanal Chem (2016) 408:705–19. doi: 10.1007/s00216-015-9185-1
31. Goh HH, Lim LS, Wong PC, Ratnam SS. Plasma oestriol in pregnancies complicated by diabetes mellitus. Aust J Exp Biol Med Sci (1982) 60(Pt 5):529–40. doi: 10.1038/icb.1982.57
32. Levitz M, Selinger M. Plasma estriol levels in class d diabetes of pregnancy. Am J Obstetrics Gynecol (1970) 108:82–4. doi: 10.1016/0002-9378(70)90209-7
33. Svendsen R, Sorensen B. The concentration of unconjugated oestrone and 17 beta-oestradiol in plasma during pregnancy. Acta Endocrinol (Copenh) (1964) 47:237–44. doi: 10.1530/acta.0.0470237
34. Kampmann U, Knorr S, Fuglsang J, Ovesen P. Determinants of maternal insulin resistance during pregnancy: an updated overview. J Diabetes Res (2019) 2019:5320156. doi: 10.1155/2019/5320156
35. Hur J, Cho EH, Baek KH, Lee KJ. Prediction of gestational diabetes mellitus by unconjugated estriol levels in maternal serum. Int J Mol Sci (2017) 14:123–7. doi: 10.7150/ijms.17321
36. Zhang Z, Kang X, Guo Y, Zhang J, Xie J, Shao S, et al. Association of circulating galectin-3 with gestational diabetes mellitus, progesterone, and insulin resistance. J Diabetes (2021) 13:54–62. doi: 10.1111/1753-0407.13088
37. Morimoto S, Jiménez-Trejo F, Cerbón M. Sex steroids effects in normal endocrine pancreatic function and diabetes. Curr Top Med Chem (2011) 11:1728–35. doi: 10.2174/156802611796117540
38. Vejrazkova D, Vcelak J, Vankova M, Lukasova P, Bradnova O, Halkova T, et al. Steroids and insulin resistance in pregnancy. J Steroid Biochem Mol Biol (2014) 139:122–9. doi: 10.1016/j.jsbmb.2012.11.007
39. Stubbins RE, Najjar K, Holcomb VB, Hong J, Núñez NP. Oestrogen alters adipocyte biology and protects female mice from adipocyte inflammation and insulin resistance. Diabetes Obes Metab (2012) 14:58–66. doi: 10.1111/j.1463-1326.2011.01488.x
40. Barros RP, Morani A, Moriscot A, Machado UF. Insulin resistance of pregnancy involves estrogen-induced repression of muscle GLUT4. Mol Cell Endocrinol (2008) 295:24–31. doi: 10.1016/j.mce.2008.07.008
41. Thomson CA, Chow HHS, Wertheim BC, Roe DJ, Stopeck A, Maskarinec G, et al. Et al: a randomized, placebo-controlled trial of diindolylmethane for breast cancer biomarker modulation in patients taking tamoxifen. Breast Cancer Res Treat (2017) 165:97–107. doi: 10.1007/s10549-017-4292-7
42. Lustig RH, Mobbs CV, Bradlow HL, McEwen BS, Pfaff DW. Differential effects of estradiol and 16 alpha-hydroxyestrone on pituitary and preoptic estrogen receptor regulation. Endocrinology (1989) 125:2701–9. doi: 10.1210/endo-125-5-2701
43. Paracchini V, Pedotti P, Raimondi S, Garte S, Bradlow HL, Sepkovic DW, et al. A common CYP1B1 polymorphism is associated with 2-OHE1/16-OHE1 urinary estrone ratio. Clin Chem Lab Med (2005) 43:702–6. doi: 10.1515/CCLM.2005.119
44. Sowers MR, Wilson AL, Kardia SR, Chu J, McConnell DS. CYP1A1 and CYP1B1 polymorphisms and their association with estradiol and estrogen metabolites in women who are premenopausal and perimenopausal. Am J Med (2006) 119:S44–51. doi: 10.1016/j.amjmed.2006.07.006
45. Secky L, Svoboda M, Klameth L, Bajna E, Hamilton G, Zeillinger R, et al. The sulfatase pathway for estrogen formation: targets for the treatment and diagnosis of hormone-associated tumors. J Drug Deliv (2013) 2013:957605. doi: 10.1155/2013/957605
46. Kallionpää RA, Järvinen E, Finel M. Glucuronidation of estrone and 16α-hydroxyestrone by human UGT enzymes: the key roles of UGT1A10 and UGT2B7. J Steroid Biochem Mol Biol (2015) 154:104–11. doi: 10.1016/j.jsbmb.2015.07.013
47. Naitoh K, Honjo H, Yamamoto T, Urabe M, Ogino Y, Yasumura T, et al. Estrone sulfate and sulfatase activity in human breast cancer and endometrial cancer. J Steroid Biochem Mol Biol (1989) 33:1049–54. doi: 10.1016/0022-4731(89)90408-1
48. Yi M, Negishi M, Lee SJ. Estrogen sulfotransferase (SULT1E1): its molecular regulation, polymorphisms, and clinical perspectives. J Pers Med (2021) 11:194. doi: 10.3390/jpm11030194
Keywords: steroid hormones, gestational diabetes, UPLC-MS/MS, estrogens, corticosteroids, progestins
Citation: Yang N, Zhang W, Ji C, Ge J, Zhang X, Li M, Wang M, Zhang T, He J and Zhu H (2023) Metabolic alteration of circulating steroid hormones in women with gestational diabetes mellitus and the related risk factors. Front. Endocrinol. 14:1196935. doi: 10.3389/fendo.2023.1196935
Received: 30 March 2023; Accepted: 02 June 2023;
Published: 15 June 2023.
Edited by:
Mitra Tavakoli, University of Exeter, United KingdomReviewed by:
Maria Luisa Lazo De La Vega Monroy, University of Guanajuato, MexicoJuan J. Chillaron, Parc de Salut Mar, Spain
Copyright © 2023 Yang, Zhang, Ji, Ge, Zhang, Li, Wang, Zhang, He and Zhu. This is an open-access article distributed under the terms of the Creative Commons Attribution License (CC BY). The use, distribution or reproduction in other forums is permitted, provided the original author(s) and the copyright owner(s) are credited and that the original publication in this journal is cited, in accordance with accepted academic practice. No use, distribution or reproduction is permitted which does not comply with these terms.
*Correspondence: Jun He, aGVqQG5qdWNtLmVkdS5jbg==; Huaijun Zhu, aHVhaWp1bi56aHVAZ21haWwuY29t
†These authors have contributed equally to this work and share first authorship