- 1Institut de Recerca de l’Hospital de la santa Creu i Sant Pau, Sant Quintí, Barcelona, Spain
- 2Biomedical Research Institute Sant Pau (IIB Sant Pau), Sant Quintí, Barcelona, Spain
- 3Institute of Biomedical Research of Barcelona (IIBB), Spanish National Research Council (CSIC), Barcelona, Spain
- 4Universitat Autònoma de Barcelona, Barcelona, Spain
- 5Endocrinology and Nutrition Service, Hospital de la Santa Creu i Sant Pau, Sant Quintí, Barcelona, Spain
- 6Centro de Investigación Biomédica en Red de Diabetes y Enfermedades Metabólicas (CIBERDEM), Instituto de Salud Carlos III, Madrid, Spain
- 7Cardiology Department, Santa Creu i Sant Pau University Hospital, Barcelona, Spain
- 8Cardiac Imaging Unit, Cardiology Department, Santa Creu i Sant Pau University Hospital, Barcelona, Spain
- 9Centro de Investigación Biomédica en Red de Enfermedades Cardiovasculares (CIBERCV), Institute of Health Carlos III, Madrid, Spain
Background: Low-density lipoprotein receptor-related protein 1 (LRP1) negatively modulates circulating atrial natriuretic peptide (ANP) levels. Both molecules are involved in the regulation of cardiometabolism.
Objectives: To evaluate soluble LRP1 (sLRP1) and ANP levels in people with newly diagnosed type 2 diabetes mellitus (T2DM) and determine the effects of metabolic optimization.
Methods: This single-center longitudinal observational study recruited patients with newly diagnosed T2DM (n = 29, HbA1c > 8.5%), and 12 healthy control, age- and sex-matched volunteers. sLRP1 and ANP levels were measured by immunoassays at T2DM onset and at one year after optimization of glycemic control (HbA1c ≤ 6.5%).
Results: T2DM had higher sLRP1 levels than the control group (p = 0.014) and lower ANP levels (p =0.002). At 12 months, 23 T2DM patients reached the target of HbA1c ≤ 6.5%. These patients significantly reduced sLRP1 and increased ANP levels. Patients who did not achieve HbA1c < 6.5% failed to normalize sLRP1 and ANP levels. There was an inverse correlation in the changes in sLRP1 and ANP (p = 0.031). The extent of sLRP1 changes over 12 months of metabolic control positively correlated with those of total cholesterol, LDL cholesterol, TG, TG/HDLc, and apolipoprotein B.
Conclusions: Newly diagnosed T2DM patients have an increased sLRP1/ANP ratio, and increased sLRP1 and decreased ANP levels are normalized in the T2DM patients that reached an strict glycemic and metabolic control. sLRP1/ANP ratio could be a reliable marker of cardiometabolic function.
Introduction
Patients with type 2 diabetes mellitus (T2DM) show higher incidence of heart failure and higher cardiovascular disease (CVD) mortality risk (1). Achieving optimal glycemic control is a clinical goal in persons with T2DM to reduce CVD risk, especially at early stages of T2DM (2, 3). However, new biomarkers connecting cardiovascular and metabolic alterations need to be developed for helping to prevent CVD in T2DM.
Low-density lipoprotein receptor-related protein 1 (LRP1) plays a crucial role in atherosclerosis progression (4, 5). Its soluble form (sLRP1) comprises the α chain (515 kDa) and a fragment of the β chain (55 kDa) of the cellular receptor. sLRP1 can be detected in the circulation after either constitutive or induced cleavage (6). Previous studies from our group have shown that atherogenic lipoproteins promote sLRP1 release from vascular smooth muscle cells of human atherosclerotic plaque explants, and that circulating levels of sLRP1 are associated with carotid atherosclerosis in familial hypercholesterolemic patients (7) and with carotid plaque inflammation measured by 18F-FDG PET in patients with a recent ischemic stroke (8). In line with this, we further demonstrated that circulating sLRP1 levels are also associated with coronary artery disease risk, independently of potential confounding factors (9).
LRP1 is overexpressed in advanced human atherosclerotic lesions enriched in lipids (10), in the vascular wall of hypercholesterolemic rabbits and pigs (5, 11, 12) and in myocardium of in vivo models of diabetic rats (13).
In the context of cardiometabolic diseases, epicardial fat expresses and releases an altered pattern of adipokines and other molecules that contribute to vascular and cardiac dysfunction (14, 15). As LRP1 is overexpressed in epicardial fat of T2DM patients (16), it could be hypothesized that dysfunctional epicardial fat contributes to increased circulating sLRP1 levels. Supporting this, sLRP1 levels directly correlate with epicardial fat volume in type 1 diabetes mellitus (T1DM) patients and in the general population (17, 18). Together, these results suggest that sLRP1 could be a dual biomarker of cardiac and metabolic alterations in T2DM.
Atrial natriuretic peptide (ANP) is secreted by cardiomyocytes (19), and its circulating concentration decrease under insulin resistance (20, 21). Our group has previously shown in a murine model that the circulating levels of ANP are modulated by cardiac LRP1 levels, and that the reduction of cardiomyocyte LRP1 levels increases the release of ANP that coordinately inhibits hepatic fatty acid (FA) synthesis and activates FA uptake and oxidation, limiting weight gain and enhancing whole-body energy consumption (22).
LRP1 levels in heart inversely modulate ANP levels (22), which is essential in the control of metabolism. In light of this, the sLRP1/ANP ratio could be used as an indicator that integrates information about metabolic and cardiovascular alterations. Our hypothesis is that the inverse relation between the circulating levels of sLRP1 and ANP in humans are favorably influenced by the optimization of the glycemic control. The aims of this study were i) to evaluate sLRP1 and ANP levels in newly diagnosed T2DM patients as compared to healthy controls, and ii) to determine the effect of achieving metabolic optimization on these circulating biomarkers.
Methods
Patients
This single-center, observational longitudinal study included 29 patients with T2DM (mean age 56 ± 9 years) who had been referred to the Endocrinology and Nutrition Department of Hospital de la Santa Creu i Sant Pau, Barcelona (Spain), as well as 12 age- and sex-matched control subjects. The study was approved by the Ethics Committee of the Hospital de Sant Pau (reference number of the protocol IIBSP-REL-2017-27, data of approval 07/26/2017). Written informed consent was obtained from all participants. This study was performed in full compliance with the Declaration of Helsinki. T2DM patients were newly diagnosed without previous hypoglycemic, lipid-lowering or anti-inflammatory drugs. Control group were normolipemic and normoglycemic subjects, with no personal or family history of premature coronary disease, major cardiovascular risk factors, or infectious or inflammatory disease.
All T2DM patients (n = 29) were studied at onset and at 12 months after treatment started. Patients were subjected to an intensive intervention carried out to achieve recommended targets of cardiovascular risk factors that includes diet, physical activity and pharmacotherapy, in accordance with clinical practice guidelines. Lipid profile, glucose, CRP and HbA1c levels were determined by routine procedures. All T2DM patients participated in a comprehensive diabetes self-management education program, which includes individualized instruction on nutrition, physical activity and optimizing metabolic control. According to our protocol for the management of severe hyperglycemia, the initial therapy included triple therapy with metformin, dipeptidyl peptidase inhibitors and basal insulin in 90% of patients. Basal insulin was suspended after 1–2 weeks, and non-insulin drugs were modified at the discretion of the responsible physician according to the characteristics of each patient.
Immunoassays
Blood samples were obtained at disease onset and 12 months of treatment start by venipuncture in EDTA-containing or additive-free Vacutainer tubes to obtain plasma or serum, respectively. Tubes were centrifuged for 15 min at 1500g at room temperature for a maximum period of 30 min. The resulting plasma or serum was aliquoted and stored at –80 until use. sLRP1 levels were measured in plasma using an ELISA kit from Cloud-Clone corp (SEB010Hu), and ANP levels were measured in serum using an ELISA kit from LSBio kit (LS-F57269).
Statistical analysis
In order to justify the validity of the kits used, we have revised the ELISA data in terms of sensitivity limits and reliability of results. The Intra-Assay CV for LRP1 ELISA Kit was <10% and for ANP ELISA Kit <6.9%. The coefficients of variation Inter-Assay was CV<12% for LRP1 ELISA kit and CV<8.7% for ANP ELISA Kit. These intra-assay and inter-assay coefficient of variation (CV) support the quality and feasibility of these assays to be used as evaluation tools. The power was between 0.78 and 0.96 with N of 29 patients and 8 controls for the variables sLRP1, ANP and sLRP1/ANP. The normality of numerical data distribution was verified using the Shapiro-Wilk test, and the homoscedasticity, with the Levene’s test. The categorical variables are presented as frequencies and percentages, and the quantitative variables are presented as mean ± SD. A bivariate analysis was used for paired data, and a Chi-square analysis was used for categorical data. Relationships between different Δparameters and Δbiomarkers were assessed using Spearman correlation analysis. To determine the possible confounding factors between the associations of different variables, a simple linear regression test was used. Due to the small sample size, the analysis was validated using a non-parametric approach. All statistical analyses were performed with SPSS software, version 27. A two-sided p-value < 0.05 was considered statistically significant.
Results
Clinical characteristics
Table 1 summarizes the clinical and metabolic variables of newly diagnosed T2DM patients as compared to healthy controls. There were significant differences in weight (p = 0.031), body mass index (BMI) (p = 0.005), HbA1c (p < 0.001), blood glucose (p < 0.001), C-reactive protein (CRP) (p < 0.001), triglycerides (TG) (p < 0.001), high-density lipoprotein cholesterol (HDLc) (p = 0.002), TG/HDL index (p < 0.001) and ApoB (p = 0.044) between newly diagnosed T2DM patients and control subjects. All variables, except HDLc, were higher in the T2DM group. Age and sex distribution between groups was similar.
Glycemic control optimization normalizes the main metabolic and lipidic variables at a 12-month follow-up
As summarized in Table 1, the main variables that were optimized after 12 months of T2DM metabolic control were weight, BMI, HbA1c levels, glucose, HDLc and CRP.
From the total 29 T2DM patients, 23 reached a strict glycemic control (HbA1c ≤ 6.5) while only 6 remained in less strict glycemic control (HbA1c > 6.5). As shown in Table 1, most metabolic variables including weight, BMI, glucose, CRP, TC, LDLc, ApoB, TG/HDLc index and HDLc only significantly improved in the group reaching a strict glycemic control after 1 year.
Newly diagnosed T2DM patients showed increased sLRP1 and decreased ANP plasma levels compared to controls
As shown in Figure 1, circulating sLRP1 concentrations were significantly higher in the T2DM group at onset than in the control group (p = 0.014), while those of ANP were lower (p = 0.002). Therefore, the sLRP1/ANP ratio was much higher in new-onset T2DM patients than in healthy controls (p < 0.001). Possible correlations between sLRP1, ANP, sLRP1/ANP and clinical and metabolic variables was analyzed in T2DM patients. sLRP1 directly correlated with circulating TGs (r2 = 0.475; p = 0.009), TG/HDLc index (r2 = 0.445; p = 0.015) and ApoB (r2 = 0.374; p = 0.046). ANP inversely correlated with circulating ApoB (r2=-0.374; p = 0.046), CT (r2 = -0.378; p = 0.043) and LDLc (r2 = -0.367; p = 0.05). The ratio sLRP1/ANP directly correlated with ApoB (r2 = 0.506; p = 0.005), TG/HDLc index (r2 = 0.424; p = 0.022), LDLc (r2 = 0.375; p = 0.045), TGs (r2 = 0.392; p = 0.035), and TC (r2 = 0.400; p = 0.032).
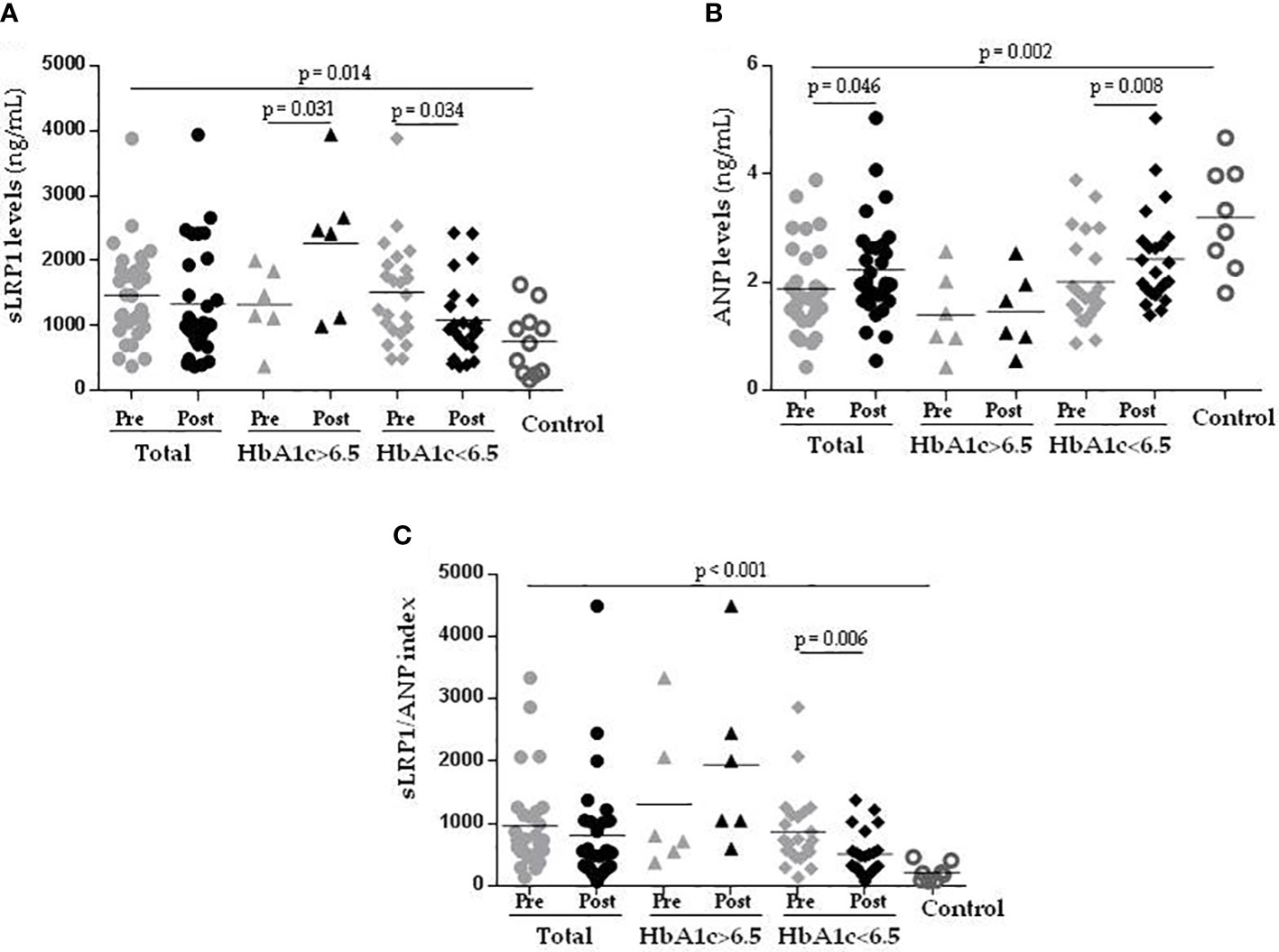
Figure 1 Newly diagnosed T2DM patients have increased sLRP1 and reduced ANP plasma levels that are normalized after 1 year of optimal glycemic control. Graph showing circulating levels of sLRP1 (A), ANP (B) and sLRP1/ANP ratio (C) in control subjects (n = 12), Total (n = 27) (Pre =T2DM onset), (Post = T2DM-1year), T2DM-1year HbA1c>6.5 (n = 6) (Pre =T2DM onset), (Post = T2DM-1year), and T2DM-1year HbA1c ≤ 6.5 (n = 21) (Pre =T2DM onset), (Post = T2DM-1year). The horizontal line gives the mean of values from the same group. Comparisons between groups were analyzed with the Wilcoxon-test. T2DM, type 2 diabetes mellitus.
Metabolic optimization normalizes circulating levels of sLRP1 and ANP, and sLRP1/ANP ratio at a 12-month follow-up
To analyze the evolution of circulating levels of sLRP1 and ANP, and of the sLRP1/ANP ratio according to the glycemic control, the changes in these variables from T2DM onset (Pre) to T2DM-1-year (Post) were analyzed in strict glycemic control (HbA1c ≤ 6.5%) versus less strict glycemic control (HbA1c > 6.5%) patients (Figure 1).
After 1 year of treatment, sLRP1 levels were significantly downregulated in T2DM patients with a strict glycemic control, but upregulated in those with less strict glycemic control (Figure 1A). ANP levels were upregulated after 1 year of treatment in patients reaching a strict glycemic control but not in those that maintained HbA1c > 6.5% over 1 year of treatment, in which ANP levels did not change (Figure 1B). Coherently, sLRP1/ANP ratio was significantly downregulated after one year of glycemic control only in those patients that reached a strict glycemic control (Figure 1C).
Correlations between classical and new variables changes over 1 year were analyzed by Spearman correlation analysis. ΔsLRP1 directly correlated with changes in metabolic variables such as ΔWeight (r2 = 0.643; p < 0.001), ΔBMI (r2 = 0.636; p < 0.001), ΔTG (r2 = 0.668; p < 0.001), ΔGlucose (r2 = 0.481; p = 0.011), ΔTG/HDL (r2 = 0.621; p = 0.001) and inversely with the change in ΔANP (r2 = –0.402; p = 0.031). In addition, the reduction in ΔsLRP1 also directly correlated with reductions in circulating lipids including ΔTC (r2 = 0.673; p < 0.001), ΔLDLc (r2 = 0.534; p = 0.004), and ApoB (r2 = 0.736; p < 0.001).
Furthermore, we analyzed whether the correlation between ΔsLRP1 and other variables, like ΔTG, ΔTC, ΔLDL, ΔApoB and ΔTG/HDL, was maintained by considering possible confounding factors, such as weight or BMI (by simple linear regression). The sLRP1 correlation adjusted for weight was significant with TG (p < 0.000), TC (p = 0.001), LDL (p = 0.002), TG/HDL (p < 0.000) and ApoB (p < 0.000). The sLRP1 correlation adjusted by BMI was also significant for TG (p = 0.001), TC (p = 0.002), LDL (p = 0.004), TG/HDL (p < 0.000) and ApoB (p = 0.001). Therefore, the correlation between the reduction in sLRP1 and lipid variables was independent of the reduction in weight or BMI.
Discussion
This study revealed for the first time that blood sLRP1 levels, which predict cardiovascular risk (9), are upregulated, while ANP levels, which inversely predict metabolic risk (21–23), are downregulated in T2DM patients at disease onset as compared to healthy controls. In addition, we showed that sLRP1 and ANP inversely evolved after 1 year of treatment.
The elevated circulating sLRP1 levels in T2DM patients found here are in line with the documented increased LRP1 levels in the epicardial fat of T2DM patients (16) and in the myocardium of diabetic rats (13), and also with the close association between blood sLRP1 levels and the extension of epicardial fat in patients with type 1 diabetes and in the general population (17, 18). On the other hand, the low levels of ANP found in newly diagnosed T2DM patients are in line with the potential of low ANP levels to predict the development of diabetes in humans (23–27).
Previous studies from our group conducted in an experimental murine model evidenced that reduced levels of cardiac LRP1 promote increased circulating ANP levels, while increased levels of LRP1 favor decreased circulating ANP levels (22). Through the control of circulating ANP levels, cardiac LRP1 modulates not only fatty acid metabolism in the liver but also whole-body metabolism (22). Results from this experimental murine model highlight the presence of a functional LRP1-ANP link between heart, liver and adipose tissue. In the present study, a crucial point is that baseline sLRP1 levels are increased, while ANP are decreased, in newly diagnosed T2DM patients (without previous treatment) as compared to control subjects. In addition, if glycemic optimization is achieved after 1 year of treatment, there is a tight and inverse correlation between decreased sLRP1 levels and increased ANP levels. Taken together, these results suggest that the link between sLRP1 and ANP, previously described by our group in an in vivo model, is likely present in T2DM patients. In addition, our results support the concept that sLRP1 and ANP, which are key mediators of cardiometabolic mechanisms, are connected in humans and can play a key role in the interplay between cardiac and metabolic alterations.
Results from the present study show that strict glycemic control (HbA1c ≤ 6.5%) is highly efficient in reducing sLRP1 levels and increasing ANP levels in T2DM patients to the same levels found in the control group. These results suggest that an optimal glycemic control of T2DM patients may exert beneficial effects on parameters associated to cardiovascular risk, such as LRP1 (9). Currently, there is an intense debate about the potential and mechanisms of lowering HbA1c to provide protection against cardiovascular complications of T2DM (28, 29). Over the last decade, one of the proposed markers of glycemic control has been TG/HDLc (30–32). Results from the present study evidenced a positive correlation of sLRP1 with TG and with the TG/HDLc ratio in line with previous studies by our group showing the association of circulating TGs with epicardial LRP1 levels in T2DM patients (16). Here, we also showed that the decline in sLRP1 over 1-year caused by achieving a strict glycemic control correlated with the decrease in TGs and TG/HDL. This association between sLRP1 and TG remained after adjusting by weight and BMI. At this moment, the mechanisms underlying this association remain unclear. TG/HDLc has been proposed to be associated with CVD in the context of metabolic conditions (30–32), and sLRP1 has been reported to be predictive of cardiovascular risk in a case-cohort study (9). Therefore, it seems important to ascertain the mechanisms that determine the close association between sLRP1 and TG/HDLc in order to elucidate new mechanisms that are potentially involved in increasing the cardiovascular risk of patients with diabetes.
Here, we also observed that the reduction in sLRP1 after reaching optimal glycemic control correlated with the changes in other lipid parameters, such as TC, LDL-C and ApoB100. Such correlations were previously observed in different populations, in which sLRP1 is associated with cardiovascular risk (7–9). Further studies will be required to know whether sLRP1 reduction is associated to a decrease of atherosclerosis in T2DM patients. In addition, further studies with increased numbers of participants and long-term follow-up are required to validate the potential of sLRP1 and sLRP1/ANP to predict the beneficial effects of lipidic/glycemic control in cardiac and metabolic alterations
Strengths and limitations
The main limitation of this study is the reduced number of participants due to the difficulty to recruit newly diagnosed untreated T2DM patients. One remarkable strength is the homogeneity of the newly diagnosed T2DM patients included in this study in terms of lack of previous hypoglycemic, lipid lowering or anti-inflammatory treatments.
Conclusion
Results from the present study show that the cardiac LRP1-ANP axis previously reported by our group in an experimental murine model of prediabetes is likely working in T2DM patients. The high levels of sLRP1 in the cardiovascular system of T2DM patients could lead to low circulating levels of ANP, explaining the high sLRP1/ANP ratio found in these patients as compared to healthy controls (summarized in Figure 2). Altered sLRP1 and ANP levels are normalized in the T2DM group that reached an optimal metabolic control. Therefore, we propose that sLRP1/ANP can be a potential marker of the cardiovascular benefits of glycemic control in T2DM patients. Further studies are required to know whether sLRP1/ANP index might improve the predictive value of other biomarkers in terms of cardiovascular and metabolic outcomes in T2DM patients.
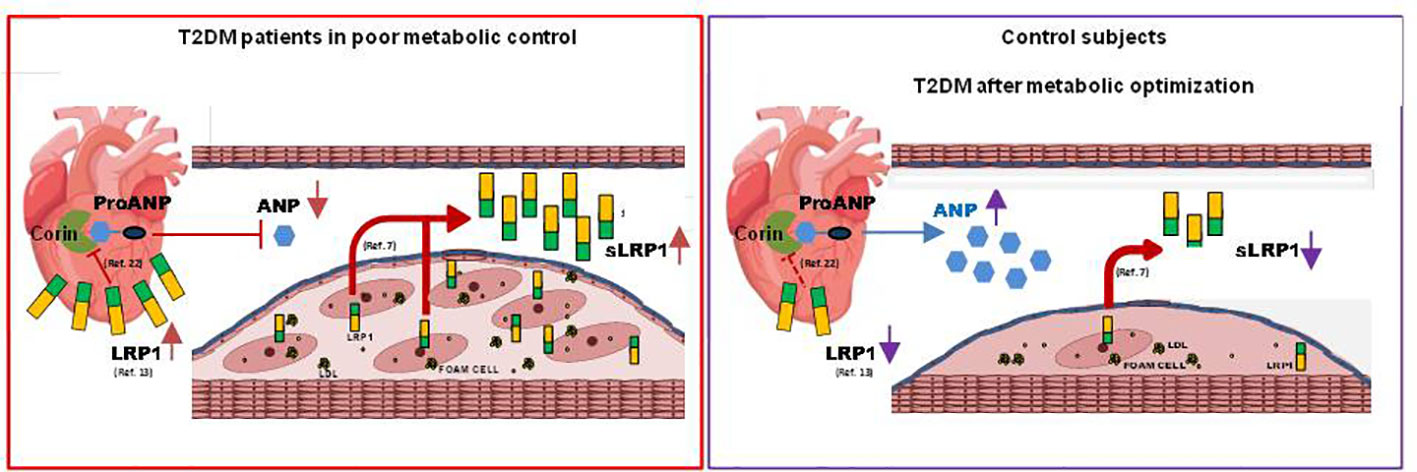
Figure 2 This graphical abstract combines previous findings from our group in a murine model of prediabetes with results obtained in the current study in T2DM patients. In the murine model, the reduction of cardiomyocyte LRP1 levels causes the increase of corin enzyme activity and ANP release (22). In addition, LRP1 levels are increased in the myocardium of diabetic hearts (13) and circulating sLRP1 levels correlate with atherosclerosis in humans (7). The main findings of the present study are that circulating levels of sLRP1 are increased while those of ANP are decreased in newly diagnosed type 2 diabetic patients (T2DM), and that altered sLRP1 and ANP levels are normalized in the T2DM group that reached an optimal metabolic control.
Data availability statement
The raw data supporting the conclusions of this article will be made available by the authors, without undue reservation.
Ethics statement
The studies involving human participants were reviewed and approved by Ethics Committee of the Hospital de SantPau (protocol IIBSP-REL-2017-27). The patients/participants provided their written informed consent to participate in this study.
Author contributions
EG and PG performed ELISA assays, organized the database and did the statistical analysis. IM, JR and PG researched clinical data. AB-A collected data and contributed to the discussion. CR contributed to generation of the database. LC performed ELISA assays and collected data. JJ, SB and JS-Q designed the study and contributed to the generation of data and to discussion. XG-M and DV contributed to discussion of data. AP designed the study and wrote the manuscript. VLl-C designed the study, researched laboratory data and wrote the manuscript. All authors contributed to the article and approved the submitted version.
Funding
The economic support to develop this project was received from: Fundació MARATÓ TV3 with the grant 201521-10 (to VLl-C). Instituto de Salud Carlos III (ISCIII) and co-financed with ERDFs with the grantsPI16-00471 (to JS-Q), PI17-00232 (to JJ), PI20-00334 (to JLS-Q), FIS PI18/01584 and FIS PI21/01523 (to VLl-C). Fundación BBVA Ayudas a equipos de investigación 2019 (“Translational Molecular Imaging for Detection of Cholesterol Entrapment in the Vasculature with 68Ga-labeled LRP1-derived Peptides”) (to VLl-C). AB-A is a predoctoral fellow (FI19/00205) granted by the Programme_Contratos predoctorales de formación de investigación en salud_ from the Instituto de Salud Carlos III (ISCIII) and co-financed with ERDFs. The team is part of CIBER Enfermedades Cardiovasculares (CIBERCV; CB16/11/00276 to DV, XG-M and VLl-C), and CIBER Diabetes y Enfermedades Metabólicas Asociadas (CIBERDEM; CB07/08/0016 to JJ, JS-Q, and AP) run by the Instituto de Salud Carlos III. VLl-C and JJ are members of Redes de investigación (Enfermedades Metabólicas y Cáncer RED2018-102799-T), a project run by MINECO. VLl-C, AB-A and DV are members of the Quality Research Group from Generalitat de Catalunya 2017 SGR 946 and JJ, SB and JS-Q of the 2017-SGR-1149group. VLl-C, EG, AB-A, SB and JS-Q are members of the Spanish Atherosclerosis Society Vascular Biology Group. IR-SANTPAU is a centre of CERCA Programme/Generalitat de Catalunya.
Acknowledgments
We are grateful to the professionals who participated in data collection and to the nursing team of the Hospital for their help during this work. We thank Ignasi Gich for his work with the statistical analysis, and Veronica A. Raker for manuscript editing.
Conflict of interest
The authors declare that the research was conducted in the absence of any commercial or financial relationships that could be construed as a potential conflict of interest.
Publisher’s note
All claims expressed in this article are solely those of the authors and do not necessarily represent those of their affiliated organizations, or those of the publisher, the editors and the reviewers. Any product that may be evaluated in this article, or claim that may be made by its manufacturer, is not guaranteed or endorsed by the publisher.
Abbreviations
Apo, apolipoprotein; ANP, atrial natriuretic peptide; BMI, body mass index; CRP, C reactive protein; HDLc, high-density lipoprotein cholesterol; LDLc, low-density lipoprotein cholesterol; sLRP1, soluble low-density lipoprotein receptor-related protein 1; TC, total cholesterol; TG, triglycerides; T2DM, type 2 diabetes mellitus.
References
1. Glovaci D, Fan W, Wong ND. Epidemiology of diabetes mellitus and cardiovascular disease. Curr Cardiol Rep (2019) 21:21. doi: 10.1007/s11886-019-1107-y
2. Llamazares Iglesias O, Sastre Marcos J, Peña Cortés V, Luque Pazos A, Cánovas Gaillemin B, Vicente Delgado A, et al. Metabolic and cardiovascular risk factor control in a diabetic cohort. Four-year results. Endocrinol Nutr (2012) 59:117–24. doi: 10.1016/j.endonu.2011.11.004
3. Wright AK, Suarez-Ortegon MF, Read SH, Kontopantelis E, Buchan I, Emsley R, et al. Risk factor control and cardiovascular event risk in people with type 2 diabetes in primary and secondary prevention settings. Circulation (2020) 142:1925–36. doi: 10.1161/CIRCULATIONAHA.120.046783
4. Llorente-Cortés V, Otero-Viñas M, Camino-López S, Llampayas O, Badimon L. Aggregated low-density lipoprotein uptake induces membrane tissue factor procoagulant activity and microparticle release in human vascular smooth muscle cells. Circulation (2004) 110:452–9. doi: 10.1161/01.CIR.0000136032.40666.3D
5. Bornachea O, Benitez-Amaro A, Vea A, Nasarre L, de Gonzalo-Calvo D, Escola-Gil JC, et al. Immunization with the Gly1127-Cys1140 amino acid sequence of the LRP1 receptor reduces atherosclerosis in rabbits. Molecular, immunohistochemical and nuclear imaging studies. Theranostics (2020) 10:3263–80. doi: 10.7150/thno.37305
6. Quinn KA, Pye VJ, Dai YP, Chesterman CN, Owensby DA. Characterization of the soluble form of the low-density lipoprotein receptor-related protein (LRP). Exp Cell Res (1999) 251:433–41. doi: 10.1006/excr.1999.4590
7. de Gonzalo-Calvo D, Cenarro A, Martínez-Bujidos M, Badimon L, Bayes-Genis A, Ordonez-Llanos J, et al. Circulating soluble low-density lipoprotein receptor-related protein 1 (sLRP1) concentration is associated with hypercholesterolemia: A new potential biomarker for atherosclerosis. Int J Cardiol (2015) 201:20–9. doi: 10.1016/j.ijcard.2015.07.085
8. Garcia E, Camps−Renom P, Puig N, Fernandez−Leon A, Aguilera−Simon A, Benitez−Amaro A, et al. Soluble low−density lipoprotein receptor−related protein 1 as a surrogate marker of carotid plaque inflammation assessed by 18F−FDG PET in patients with a recent ischemic stroke. J Trans Med (2023) 21:131. doi: 10.1186/s12967-022-03867-w
9. de Gonzalo-Calvo D, Elosua R, Vea A, SubIrana I, Sayols-Baixeras S, Marrugat J, et al. Soluble low-density lipoprotein receptor-related protein 1 as a biomarker of coronary risk: Predictive capacity and association with clinical events. Atherosclerosis (2019) 287:93–9. doi: 10.1016/j.atherosclerosis.2019.06.904
10. Llorente-Cortés V, Otero-Viñas M, Berrozpe M, Badimon L. Intracellular lipid accumulation, low-density lipoprotein receptor-related protein expression, and cell survival in vascular smooth muscle cells derived from normal and atherosclerotic human coronaries. Eur J Clin Invest (2004) 34:182–90. doi: 10.1111/j.1365-2362.2004.01316.x
11. Llorente-Cortes V, Casani L, Cal R, Llenas A, Juan-Babot O, Camino-López S, et al. Cholesterol-lowering strategies reduce vascular LRP1 overexpression induced by hypercholesterolaemia. Eur J Clin Invest (2011) 41:1087–97. doi: 10.1111/j.1365-2362.2011.02513.x
12. Llorente-Cortés V, Otero-Viñas M, Sánchez S, Rodríguez C, Badimon L. Low-density lipoprotein upregulates low-density lipoprotein receptor-related protein expression in vascular smooth muscle cells: possible involvement of sterol regulatory element binding protein-2-dependent mechanism. Circulation (2002) 106:3104–10. doi: 10.1161/01.cir.0000041434.28573.0b
13. Samouillan V, Revuelta-López E, Dandurand J, Nasarre L, Badimon L, Lacabanne C, et al. Cardiomyocyte intracellular cholesteryl ester accumulation promotes tropoelastin physical alteration and degradation: Role of LRP1 and cathepsin S. Int J Biochem Cell Biol (2014) 55:209–19. doi: 10.1016/j.biocel.2014.09.005
14. Iacobellis G, Leonetti F. Epicardial adipose tissue and insulin resistance in obese subjects. J Clin Endocrinol Metab (2005) 90:6300–2. doi: 10.1210/jc.2005-1087
15. Patel VB, Shah S, Verma S, Oudit GY. Epicardial adipose tissue as a metabolic transducer: role in heart failure and coronary artery disease. Heart Fail Rev (2017) 22:889–902. doi: 10.1007/s10741-017-9644-1
16. Nasarre L, Juan-Babot O, Gastelurrutia P, Llucia-Valldeperas A, Badimon L, Bayes-Genis A, et al. Low density lipoprotein receptor-related protein 1 is upregulated in epicardial fat from type 2 diabetes mellitus patients and correlates with glucose and triglyceride plasma levels. Acta Diabetol (2014) 51:23–30. doi: 10.1007/s00592-012-0436-8
17. de Gonzalo-Calvo D, Vilades D, Nasarre L, Carreras F, Leta R, Garcia-Moll X, et al. Circulating levels of soluble low-density lipoprotein receptor-related protein 1 (sLRP1) as novel biomarker of epicardial adipose tissue. Int J Cardiol (2016) 223:371–3. doi: 10.1016/j.ijcard.2016.08.149
18. de Gonzalo-Calvo D, Colom C, Vilades D, Rivas-Urbina A, Moustafa AH, Pérez-Cuellar M, et al. Soluble LRP1 is an independent biomarker of epicardial fat volume in patients with type 1 diabetes mellitus. Sci Rep (2018) 8:16715. doi: 10.1038/s41598-018-19230-3
19. Goetze JP, Bruneau BG, Ramos HR, Ogawa T, de Bold MK, de Bold AJ. Cardiac natriuretic peptides. Nat Rev Cardiol (2020) 17:698–717. doi: 10.1038/s41569-020-0381-0
20. Rao S, Pena C, Shurmur S, Nugent K. Atrial natriuretic peptide: Structure, function, and physiological effects: A narrative review. Curr Cardiol Rev (2021) 17:e051121191003. doi: 10.2174/1573403X17666210202102210
21. Verboven K, Hansen D, Jocken JWE, Blaak EE. Natriuretic peptides in the control of lipid metabolism and insulin sensitivity. Obes Rev (2017) 18:1243–59. doi: 10.1111/obr.12598
22. Benitez-Amaro A, Revuelta-López E, Bornachea O, Cedó L, Vea À, Herrero L, et al. Low-density lipoprotein receptor-related protein 1 deficiency in cardiomyocytes reduces susceptibility to insulin resistance and obesity. Metabolism (2020) 106:154–91. doi: 10.1016/j.metabol.2020.154191
23. Magnusson M, Jujic A, Hedblad B, Engström G, Persson M, Struck J, et al. Low plasma level of atrial natriuretic peptide predicts development of diabetes: the prospective Malmo Diet and Cancer study. J Clin Endocrinol Metab (2012) 97:638–45. doi: 10.1210/jc.2011-2425
24. Gruden G, Landi A, Bruno G. Natriuretic peptides, heart, and adipose tissue: new findings and future developments for diabetes research. Diabetes Care (2014) 37:2899–908. doi: 10.2337/dc14-0669
25. Moro C. Targeting cardiac natriuretic peptides in the therapy of diabetes and obesity. Expert Opin Ther Targets (2016) 20:1445–52. doi: 10.1080/14728222.2016.1254198
26. Schlueter N, de Sterke A, Willmes DM, Spranger J, Jordan J, Birkenfeld AL. Metabolic actions of natriuretic peptides and therapeutic potential in the metabolic syndrome. Pharmacol Ther (2014) 144:12–27. doi: 10.1016/j.pharmthera.2014.04.007
27. Neeland IJ, Winders BR, Ayers CR, Das SR, Chang AY, Berry JD, et al. Higher natriuretic peptide levels associate with a favorable adipose tissue distribution profile. J Am Coll Cardiol (2013) 62:752–60. doi: 10.1016/j.jacc.2013.03.038
28. Taylor SI, Yazdi ZS, Beitelshees AL. Pharmacological treatment of hyperglycemia in type 2 diabetes. J Clin Invest (2021) 131:e142243. doi: 10.1172/JCI142243
29. Tian J, Ohkuma T, Cooper M, Harrap S, Mancia G, Poulter N, et al. Effects of intensive glycemic control on clinical outcomes among patients with type 2 diabetes with different levels of cardiovascular risk and hemoglobin A1c in the ADVANCE trial. Diabetes Care (2020) 43:1293–9. doi: 10.2337/dc19-1817
30. Esan O, Wierzbicki AS. Triglycerides and cardiovascular disease. Curr Opin Cardiol (2021) 36:469–77. doi: 10.1097/HCO.0000000000000862
31. Scicali R, Giral P, D'Erasmo L, Cluzel P, Redheuil A, Di Pino A, et al. High TG to HDL ratio plays a significant role on atherosclerosis extension in prediabetes and newly diagnosed type 2 diabetes subjects. Diabetes Metab Res Rev (2021) 37:e3367. doi: 10.1002/dmrr.3367
Keywords: sLRP1, ANP, metabolic control, T2DM, hypoglycemic treatments
Citation: García E, Gil P, Miñambres I, Benitez-Amaro A, Rodríguez C, Claudi L, Julve J, Benitez S, Sánchez-Quesada JL, Rives J, Garcia-Moll X, Vilades D, Perez A and Llorente-Cortes V (2023) Increased sLRP1 and decreased atrial natriuretic peptide plasma levels in newly diagnosed T2DM patients are normalized after optimization of glycemic control. Front. Endocrinol. 14:1236487. doi: 10.3389/fendo.2023.1236487
Received: 07 June 2023; Accepted: 20 July 2023;
Published: 10 August 2023.
Edited by:
Aleksandra Klisic, Primary Health Care Center Podgorica, MontenegroReviewed by:
Elettra Mancuso, University Magna Graecia of Catanzaro, ItalyJoaquim Barreto, State University of Campinas, Brazil
Copyright © 2023 García, Gil, Miñambres, Benitez-Amaro, Rodríguez, Claudi, Julve, Benitez, Sánchez-Quesada, Rives, Garcia-Moll, Vilades, Perez and Llorente-Cortes. This is an open-access article distributed under the terms of the Creative Commons Attribution License (CC BY). The use, distribution or reproduction in other forums is permitted, provided the original author(s) and the copyright owner(s) are credited and that the original publication in this journal is cited, in accordance with accepted academic practice. No use, distribution or reproduction is permitted which does not comply with these terms.
*Correspondence: Vicenta Llorente-Cortes, dmljZW50YS5sbG9yZW50ZUBpaWJiLmNzaWMuZXM=; Y2xsb3JlbnRlQHNhbnRwYXUuY2F0; Antonio Perez, QXBlcmV6QHNhbnRwYXUuY2F0
†These authors have contributed equally to this work
‡ORCID: Antonio Perez, orcid.org/0000-0001-5528-1143
Vicenta Llorente-Cortes, orcid.org/0000-0002-0067-7201