- 1College of Physical Education and Dance, Federal University of Goiás, Goiânia, Brazil
- 2Hypertension League, Federal University of Goiás, Goiânia, Brazil
- 3Instituto VIDA, Brasilia, Brazil
- 4Faculty of Medicine, Federal University of Goiás, Goiânia, Brazil
- 5Hospital das Clínicas, Federal University of Goiás, Goiânia, Brazil
- 6Banco de Olhos Foundation, Goiânia, Brazil
- 7Faculty of Nutrition, Federal University of Goiás, Goiânia, Brazil
Objective: To compare the effects of different aerobic training protocols on cardiometabolic variables in patients with type 2 diabetes mellitus (T2DM).
Methods: This study was a parallel clinical trial. Fifty-two men and women with T2DM (>40 years) were randomly allocated into three groups, and 44 (22 males/22 females) were included in the final analysis. Exercise intensity was based on the speed corresponding to the maximum oxygen consumption (vO2max). Moderate intensity continuous training (MICT) involved 14 minutes at 70% of vO2max; short interval high-intensity interval training (S-HIIT) consisted of 20 bouts of 30 seconds at 100% of V˙O2max with 30 seconds passive recovery; long interval high-intensity training (L-HIIT) consisted of 5 bouts of 2 minutes at 100% of vO2max with 2 minutes passive recovery. Training protocols were performed on a motorized treadmill two times per week for eight weeks. Glycated hemoglobin (Hb1Ac), total cholesterol, triglycerides, resting systolic blood pressure (SBP), resting diastolic blood pressure (DBP), resting heart rate (resting HR) and maximum oxygen consumption (V˙O2max) were measured before and after the exercise intervention. The study was registered on the Brazilian clinical trial records (ID: RBR45 4RJGC3).
Results: There was a significant difference between groups for changes on O2max. Greater increases on O2max were achieved for L-HIIT (p = 0.04) and S-HIIT (p = 0.01) in comparison to MICT group, with no significant difference between L-HIIT and S-HIIT (p = 0.9). Regarding comparison within groups, there were significant reductions on HbA1c and triglycerides levels only for L-HIIT (p< 0.05). O2max significantly increased for both L-HIIT (MD = 3.2 ± 1.7 ml/kg/min, p< 0.001) and S-HIIT (MD = 3.4 ± 1.7, p< 0.001). There was a significant reduction on resting SBP for L-HIIT group (MD = -12.07 ± 15.3 mmHg, p< 0.01), but not for S-HIIT and MICT. There were no significant changes from pre- to post-training on fasting glycemia, total cholesterol, HDL, LDL, resting HR and resting DBP for any group (p > 0.05).
Conclusion: Low-volume HIIT promoted greater improvements in cardiorespiratory capacity in comparison with low-volume MICT, independent of the protocols used. There were no other differences between groups. All protocols improved at least one of the variables analyzed; however, the most evident benefits were after the high-intensity protocols, especially L-HIIT.
1 Introduction
Type 2 diabetes mellitus (T2DM) is one of the leading causes of disability worldwide (1). T2DM is associated with increased mortality and reduced quality of life and might have serious complications, such as retinopathy, cardiovascular diseases and limb amputations (2). Therapeutic recommendations for T2DM aim to control glycemia, lipidemia, blood pressure (BP), body mass and promote lifestyle changes, such as increase physical activity levels (3). In this sense, aerobic training is considered an effective strategy for T2DM prevention and treatment (4, 5). Traditionally, performing at least 150 min/week of moderate (MICT) to vigorous intensity continuous training distributed at a minimum of three non-consecutive day during the week has been recommended to manage T2DM (6). However, there is evidence that high-intensity interval training (HIIT) might provide superior benefits on a variety of cardiometabolic risk factors in comparison to MICT (7, 8), leading physical activity guidelines to suggested the use of HIIT for managing T2DM (9).
HIIT is a type of aerobic training that consists of performing high intensity exercise bouts alternated with passive or active recovery periods (10). There are many different types HIIT, which might result in different physiological and perceptual responses (10–12). Among them, we can highlight the protocols involving shorter intervals (S-HIIT) and longer intervals (L-HIIT) (13).
Kilpatrick et al., (14) compared three work-matched HIIT protocols performed at the same intensity, but with different interval durations (120 s vs. 60 s vs. 30 s) in healthy young people. According to the results, longer intervals were associated with greater cardiovascular stress and higher discomfort (14). Similarly, Naves et al., (11) and Silva et al., (15) found that L-HIIT promoted greater cardiovascular stress in comparison with S-HIIT in healthy young men, and women with metabolic syndrome, respectively. Interestingly, most studies involving HIIT in T2DM used long intervals (≥2 minutes), which could be potentially dangerous due to the greater cardiovascular stress, especially if we consider that most T2DM patients have increased cardiovascular risks (16). However, while L-HIIT protocols may lead to increased cardiovascular stress, it also results in higher work performed, higher oxygen consumption and higher heart rate (HR), which can make them more efficient for promoting cardiometabolic adaptations (7, 17, 18).
In this context, it is important to determine whether the use of different HIIT protocols could affect cardiometabolic adaptations in T2DM in order to allow an adequate cost benefit analysis. Therefore, the aim of the present study was to investigate the effects of three different types of aerobic training protocols on cardiometabolic parameters in people with T2DM.
2 Material and methods
2.1 Study design
This study is a parallel randomized clinical trial that involved individuals with T2DM of both sex that performed different aerobic training protocols, two times per week for eight weeks. The study was performed at the Hospital of the Federal University of Goias (Goiania, Brazil), approved by the relevant Human Research Ethics Committee (Protocol No. 2,667,732, CAAE No. 54522016.6.0000.5083) and registered on the Brazilian clinical trial records (ID: RBR-4RJGC3). All participants gave written informed consent in accordance with the Declaration of Helsinki. Participants were assigned by randomization to one of three groups using a specialized website (www.random.org): L-HIIT, S-HIIT, or MICT.
Before and after the training period, participants were evaluated for anthropometric measures, resting BP, cardiopulmonary exercise test and blood markers. Anthropometric measures included weight and height for calculating body mass index (BMI), as well as waist and hip circumference. Weight was measured using a digital scale (BC 553, Tanita®, USA), and height was measured using a portable stadiometer (Personal Caprice Portatil, Sanny®, Brazil). BMI was calculated using the following formula [BMI = weight (kg)/height (m)²]. BP and HR were measured at sitting position after 10 minutes of rest according with Seventh Brazilian Arterial Hypertension Guideline using oscilometric method (Omron HEM-705) (19). Complementary results of the current experiment have been published previously (20).
All experimental procedures were carried out in the morning, with relative humidity between 40 and 60%, and temperature between 22 and 24°C, according to American College of Sports Medicine guidelines (ACSM) (21). Participants received orientation to not drink or eat products containing alcohol or caffeine 24 hours before and on the day of the tests, to not perform physical exercises or strenuous activities on the day before exercise, to have a light meal at least 2 hours before the tests, to wear clothes suitable for physical activity practice and to keep their usual diets habits during the intervention period.
2.2 Participants
Sixty participants with T2DM were recruited from 3rd Diabetes Task Force promoted by the Eye Bank Foundation of Goiás. To be included in the study, participants had to be 40 years old or more, diagnosed with T2DM, have a fasting glycemia above 126 mg/dL and/or glycated hemoglobin greater than 6.4%, and to not participate in other physical training program. Patients were excluded if they were active smokers or had myocardial revascularization, arrhythmias and frequent extrasystoles at rest or during physical exertion, unstable angina, obstructive pulmonary disease, neoplasm, renal or liver failure, orthopedic limitations, and uncontrolled hypothyroidism and cardiovascular diseases at moderate and high risk (classes C and D), according to the criteria American Heart Association (22). To be included in the final analyses, participants had to perform more than 85% of all training sessions.
After cardiopulmonary exercise tests, eight patients were excluded, including three with an exercise capacity<6 METS, two with uncontrolled arrhythmias during physical exertion, one with unstable angina, and two with a reduction in SBP during exercise to lower levels than resting SBP. Two patients were excluded from the final analyses because they presented irregular data for determined variables. The enrolment process until participant’s inclusion in final analyses is describe in Figure 1.
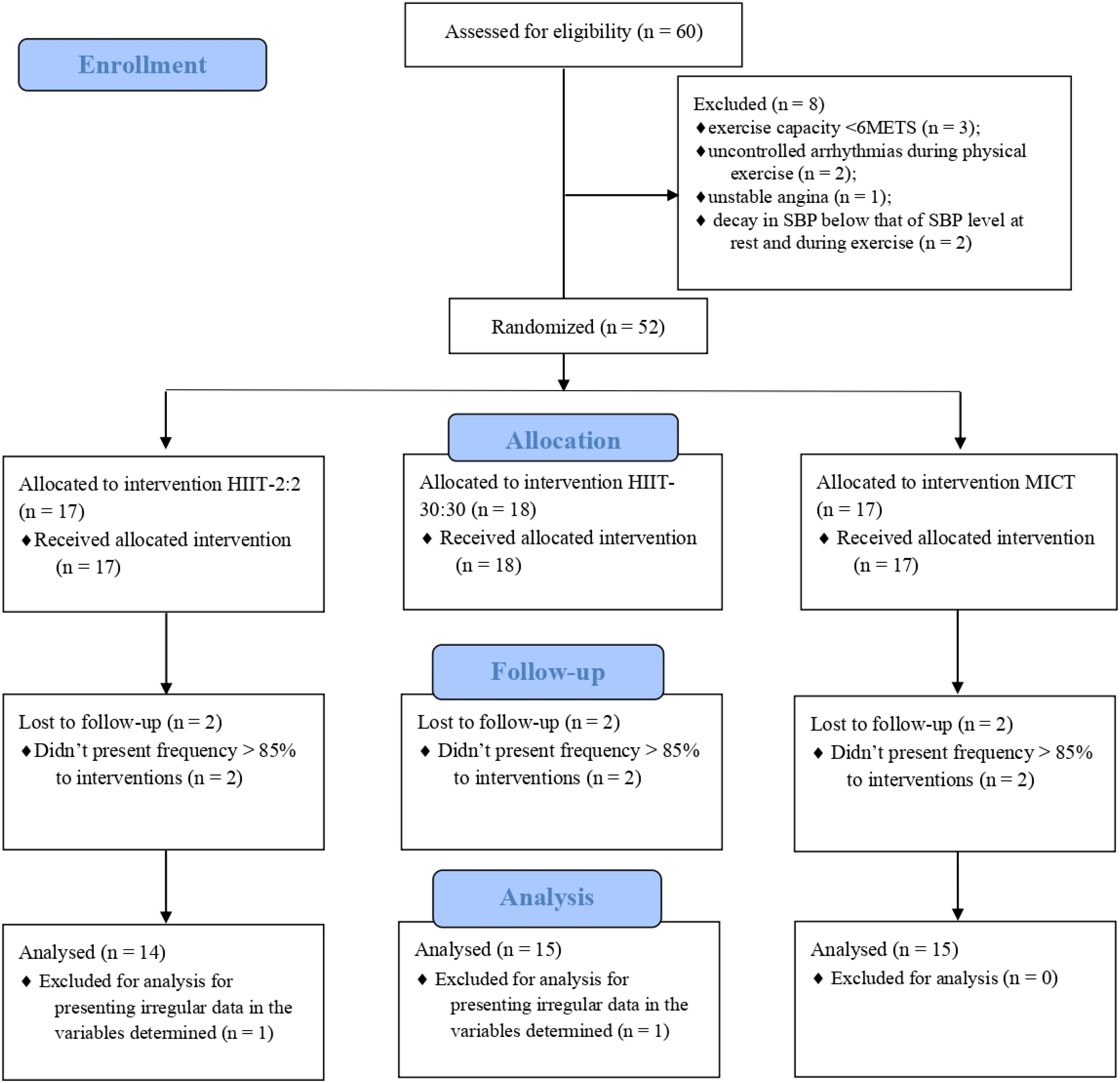
Figure 1 Study flow according to CONSORT recommendations. MICT, moderate intensity continuous training; HIIT, high-intensity interval training; SBP, systolic blood pressure.
The was no a priori definition of sample size. However, the total number of 60 participants was considered adequate because it provided a power > 0.9 to detect an effect size of 0.5 (G*Power 3.1, Germany).
2.3 Medication record
Medication use was self-reported according to Table 1 and there was no change in the medications of the patients during the intervention period.
2.4 Biochemical analyses
At the first visit to the laboratory, 4 mL of whole blood was collected by the vacuum method into EDTA tubes (Plastilab, Brazil) from the antecubital vein after approximately 12 h overnight fasting. Homogenized whole blood was used for preparation and processing of samples.
The following analyzes were performed for glucose metabolism: fasting glucose by enzymatic method using commercial kits (Labtest, Brazil), fasting insulin by chemiluminescence and HbA1c by immunoassay. This method is certified by the National Glycohemoglobin Standardization Program (23).
Lipid profile analysis involved total cholesterol by enzymatic system (reaction of the endpoint), HDL by System for direct homogeneous determination of HDL cholesterol in serum, and triglycerides by enzymatic system, by end point reaction, all using commercial kits (Labtest, Brazil).
2.5 Cardiopulmonary exercise test (CPET)
CPET involved an incremental ramp type protocol performed on a treadmill (Micromed®, Centurion 200, Brasília, Brazil) coupled to a computer. The test started with a two-minute warm-up with no inclination and speed correspondent to 50% of the initial values predicted for age and sex. Every 15 seconds of warm-up, speed increased by 0.5 km/h.
After warm-up, treadmill speed was set according to age and gender, following the recommendations of the Brazilian Society of Cardiology. The speed was then increased by 0.1 km/h every 10, 20 or 30 seconds until voluntary exhaustion, and participants were verbally encouraged to give their maximum effort. The test lasted between 8 to 12 minutes and ended when participants met the criteria for test interruption according to ACSM guideline (21).
Volunteers were instructed to wear comfortable clothes and avoid vigorous physical exercise (24 hours before the test), alcohol consumption (12 hours before the test) and caffeine (3 hours before the test). CPET was performed in the morning, to avoid the influence of the circadian rhythm. Ambient temperature (22°C - 24°C), relative humidity (40% - 60%) and lighting were controlled according to the preliminary conditions (23). Gas analysis was performed using a Cortex® analyzer (Metalyser II, Rome, Italy). Equipment calibration was performed for barometric pressure, ambient gas, gas mixture, flow and volume, as per the manufacturer’s recommendations.
HR, BP, rating of perceived exertion (RPE) and ventilatory parameters (peak, carbon dioxide production, respiratory oxygen equivalent, respiratory equivalent of the ventilatory threshold) were monitored and registered during the test. RPE was evaluated according to the Borg 0-10 (27). The instrument presents numerical classification from “0” to “10”, indicating low and maximum intensity effort, respectively. The values indicated by the volunteers were recorded at the end of each minute of the test (5th to 16th minute). Heart rate (HR) was measured by a cardiac monitor (Polar v800, Finland) during the test and up to 10 minutes of rest after its end, with the volunteer in the sitting position. Cardiac monitor was fixed in the chest and with simultaneous transmission to a watch. Data were later transferred and recorded in a specific software (Polar Flow, Finland) for proper analysis.
2.6 Exercise protocols
The protocols were customized with individualized monitored of HR, BP and v O2max achieved in the (CPET). Their respective exercise intensities were adapted from previous studies (11, 15), and were matched by the product (time * % v O2max).
Exercise session started with a 2 min warm-up and ended with a 2 min cooldown at 50% of v O2max. MICT consisted of continuous walking/running at 70% of v O2max for 14 minutes. L-HIIT consisted of 5 bouts of 2 minutes walking/running at 100% of v O2max interspersed by a passive recovery of 2 minutes. S-HIIT consisted of 20 bouts of 30 seconds walking/running at 100% of v O2max interspersed by a passive recovery for 30 seconds. All protocols were performed on a motorized treadmill (Micromed®, Centurion 200, Brasília, Brazil).
Exercise intensity was adjusted using perceived of exertion as previously suggested (24, 25).
2.7 Statistical analyses
Data normality and homogeneity were tested using the Shapiro-Wilk and Levene test, respectively. Post-training values were compared between groups using analysis of covariance (ANCOVA) with pre-training values as covariates. When a significant effect was identified, Bonferroni’s post hoc was used to identify were differences occurred. Paired T-test was used to compare within groups differences for HbA1c, fasting glycemia, total cholesterol, triglycerides, HDL, LDL, resting SBP, resting DBP, resting HR and O2max using pre- and post-training values.
All analyses were performed using SPSS statistical package (Statistical Package for Social Sciences Chicago, IL, USA) version 20.0 for Windows. Results are expressed as mean and standard deviation, and the accepted level of significance was (p< 0.05).
3 Results
The characteristics of participants are described in Table 2.
There were significant difference between groups for changes on O2max, with greater increases on O2max for L-HIIT (p = 0.04) and S-HIIT (p = 0.01) in comparison with MICT group. There was no significant difference between L-HIIT and S-HIIT (p = 0.9). Pre- and post-training values and comparisons between and within groups are presented in Table 3.
In comparison with pre-training values, there was a significant reduction on HbA1c levels for L-HIIT (mean difference [MD] = -1.8 ± 1.8%, p< 0.01), while no significant change was achieved for S-HIIT (MD = -0.92 ± 2.0%, p = 0.09) and MICT (MD = -0.59 ± 1.7%, p = 0.2). Triglycerides levels significantly reduced after L-HIIT (MD = -23.80 ± 39.6 mg/dL, p = 0.04), with no changes for S-HIIT (MD = -23.19 ± 49.0 mg/dL, p = 0.09) and MICT (MD = 5.36 ± 31.9 mg/dL, p = 0.5). There were no significant changes from pre- to post-training on fasting glycemia, total cholesterol, HDL, and LDL (p > 0.05).
There was a significant reduction on resting SBP for L-HIIT group (MD = -12.07 ± 15.3 mmHg, p< 0.01), but not for S-HIIT (MD = -7.33 ± 17.1 mmHg, p = 0.1) and MICT (MD = 4.43 ± 13.1 mmHg, p = 0.2). O2max significantly increased for both L-HIIT (MD = 3.2 ± 1.7 ml/kg/min, p< 0.001) and S-HIIT (MD = 3.4 ± 1.7, p< 0.001), but not for MICT (MD = 0.86 ± 3.8, p = 0.4). There were no significant changes from pre- to post-training for resting HR and resting DBP for any group (p > 0.05).
4 Discussion
The present study investigated the effects of three aerobic training protocols (L- HIIT, S-HIIT, and MICT) on cardiometabolic parameters of patients with T2DM. The recommendation of a minimum of 150 min per week of moderate intensity physical activities for blood glucose control and cardiovascular health is still predominant (26). However, our study demonstrated that the performance of 20 min high intensity exercise per week was sufficient to improve cardiorespiratory and metabolic fitness in patients with T2DM. These findings are particularly important since time-efficient exercise strategies emerge as promising alternatives to improve exercise adherence. Moreover, this is one of the first studies to compare HIIT protocols involving different interval lengths on metabolic parameters in T2DM patients. The present study found a significant reduction in HbA1c with large effect after L-HIIT, corroborating the idea that exercise intensity can play an important role in managing T2DM (27). Moreover, the use of HIIT protocols involving longer intervals might be preferred for blood glucose control in this population. These improvements in Hb1Ac can be explained by the increase in GLUT4 protein due to a higher concentration of calcium led by increased exercise intensity, resulting in protein translocation to the cell membrane and increasing glucose uptake by muscle cells (28–30). L-HIIT might also have led to a higher glycogen depletion, which is associated with improved glucose uptake (31, 32). A previous study demonstrated that PGC-1α increase (important protein for metabolic gene activation necessary for the use of substrate and mitochondrial biogenesis) occurs after HIIT, but not after MICT (33).
Previous studies have shown 15 to 20% reductions in cardiovascular events when HbA1c is reduced by 1% (34, 35). In addition, this reduction on HbA1c for L-HIIT group observed after 8 weeks of training is apparently higher than the observed after long-term (> 12 weeks) hypoglycemic drug treatment and insulin use (ranging from 0.6-0.8%) (45). In agreement with the current study, Winding et al., (36) found greater reductions on Hb1Ac after 11 weeks of HIIT (i.e., 10 bouts of 1 minute at 95% of peak workload interspersed by 1 minute of active rest) when compared to endurance training in individuals with T2DM. Moreover, HIIT has been shown to promote a rapid increase in skeletal muscle oxidative capacity, insulin sensitivity and glycemic control in adults with T2DM (7, 37, 38). When compared to MICT, HIIT programs have promoted greater improvements in HbA1c, fasting glycemia and other risk factors for T2DM (34, 35).
Although no significant difference was found for lipid profile between groups, there was a trend to decrease triglycerides and a large effect was found between pre and post intervention for both HIIT protocols [L-HIIT [-0.57 (wide)]; S-HIIT [-0.53 (wide)]. It is important to acknowledge that improvements in triglyceride and HDL levels are important due to the associations between dyslipidemia and cardiovascular diseases (39). The effects of HIIT on lipid profile in patients with T2DM have been inconsistent in the literature. There are studies showing improvements only in LDL cholesterol (40), only in HDL (41), or absence of alteration (42). The same studies also showed no effect of HIIT on triglycerides in patients with T2DM (40–42).
L-HIIT showed a greater reduction in resting SBP when compared to MICT (p = 0.018; d = 1.56 [large effect]). Although several studies have shown BP reductions through exercises (43), the reduction only in L-HIIT can be explained by the difference in baseline values (MICT 125 [25]mm/Hg; L-HIIT 147 [45]mm/Hg; S-HIIT 135 [20]mm/Hg), since greater reductions are seen in people with higher BP levels (44). Although there seems to be an agreement on the effectiveness of exercise to reduce BP (45), the effectiveness of aerobic exercise to reduce systolic and diastolic arterial pressure in patients with T2DM is still debated, with previous studies showing contradictory results (6, 46). Previous studies showed reductions in SBP after HIIT (~ 13 mmHg) (41) compatible with our results, revealing a potential for HIIT for controlling cardiovascular risks (47). O2max increases after HIIT is in accordance with the results of previous studies (48–50). Between group comparisons showed that the increases in O2max were larger in the L-HIIT and S-HIIT than MICT. This is in accordance with the suggestion that HIIT are more beneficial for the cardiorespiratory system. The absence of an increase O2max in the MICT was unexpected but could be associated with the changes in HbA1c since there are studies showing an association between O2max increase and in HbA1c reduction (R = −0.52, p<0.01). According to these findings, approximately 25% of the reductions in HbA1c may be related to the increase in O2max (51). O2max is an objective and independent indicator of cardiovascular risk and considered the most important physical conditioning variable (52, 53). Cardiorespiratory fitness is inversely associated with all-cause mortality and an increase of 1-2 METs (MET = 3.5ml O2/Kg/min) reduces 10% to 30% the risk of cardiovascular events (54). Although evidence for an optimal exercise intensity is still uncertain, it has been suggested that only exercise with intensity close to O2max allows the recruitment of large motor units (i.e., type II muscle fibers) (55, 56) and induces high cardiac output, which might be important for long term VO2max improvements (57).
One important limitation of the present study is the absence of dietary control. However, participants were oriented to not change their diet. We believe that these limitations do not prevent the conclusions of the study from being elaborated. In addition, due the lack of control group, it was not possible to know whether changes observed for exercising groups were different when compared to non-exercise conditions.
5 Conclusion
Low-volume HIIT promoted greater improvements in cardiorespiratory capacity in comparison with low-volume MICT, independent of the protocols used. There were no other difference between groups. All protocols improved at least one of the variables analyzed; however, the most evident benefits were after the high-intensity protocols, especially L-HIIT. Therefore, it will be up to the professional to analyze their patients individually to propose the best intervention for each case, within an appropriate cost-benefit perspective.
Data availability statement
The raw data supporting the conclusions of this article will be made available by the authors, under reasonable request.
Ethics statement
The studies involving human participants were reviewed and approved by Federal University of Goias Ethics Committee. The patients/participants provided their written informed consent to participate in this study.
Author contributions
PG conceived and designed the research. LS, JC, and JSC performed experiments. DS and PG analyzed data, interpreted results of experiments, and drafted manuscript. DS and AR edited and revised manuscript. All authors contributed to the article and approved the submitted version.
Acknowledgments
We would like to thank all participants who volunteered their time to participate in the study.
Conflict of interest
The authors declare that the research was conducted in the absence of any commercial or financial relationships that could be construed as a potential conflict of interest.
Publisher’s note
All claims expressed in this article are solely those of the authors and do not necessarily represent those of their affiliated organizations, or those of the publisher, the editors and the reviewers. Any product that may be evaluated in this article, or claim that may be made by its manufacturer, is not guaranteed or endorsed by the publisher.
References
1. Ogurtsova K, Guariguata L, Barengo NC, Ruiz PLD, Sacre JW, Karuranga S, et al. IDF diabetes atlas: Global estimates of undiagnosed diabetes in adults for 2021. Diabetes Res Clin Pract (2022) 183. doi: 10.1016/j.diabres.2021.109118
2. Cho NH, Shaw JE, Karuranga S, Huang Y, da Rocha Fernandes JD, Ohlrogge AW, et al. IDF diabetes atlas: Global estimates of diabetes prevalence for 2017 and projections for 2045. Diabetes Res Clin Pract (2018) 138:271–81. doi: 10.1016/j.diabres.2018.02.023
3. Lee I-M, Shiroma EJ, Lobelo F, Puska P, Blair SN, Katzmarzyk PT. Impact of physical inactivity on the world’s major non-communicable diseases. Lancet (2012) 380(9838):219–29. doi: 10.1016/S0140-6736(12)61031-9.Impact
4. Jeon CY, Lokken RP, Hu FB, van Dam RM. Physical activity of moderate intensity and risk of type 2 diabetes: a systematic review. Diabetes Care (2007) 30:744–52. doi: 10.2337/dc06-1842
5. De Rezende LFM, Rabacow FM, Viscondi JY, Luiz Odo C, Matsudo VK, Lee IM, et al. Effect of physical inactivity on major noncommunicable diseases and life expectancy in Brazil. J Phys Act. Heal (2015) 12:299–306. doi: 10.1123/jpah.2013-0241
6. Colberg SR, Sigal RJ, Fernhall B, Regensteiner JG, Blissmer BJ, Rubin RR. American College of sports medicine; American diabetes association. exercise and type 2 diabetes: the American college of sports medicine and the American diabetes association: joint position statement. Diabetes Care (2010) 33:147–67. doi: 10.2337/dc10-9990
7. Jelleyman C, Yates T, Donovan GO, Gray LJ, King JA, Khunti K, et al. Physical activity / metabolic effects the effects of high-intensity interval training on glucose regulation and insulin resistance : a meta-analysis. Obes Rev (2015) 16:942–61. doi: 10.1111/obr.12317
8. Liu J, Zhu L, Li P, and Xu N, Bing Y. Effectiveness of high-intensity interval training on glycemic control and cardiorespiratory fitness in patients with type 2 diabetes: a systematic review and meta-analysis. Aging Clin Exp Res (2019) 31(5):575–93. doi: 10.1007/s40520-018-1012-z
9. Colberg SR, Sigal RJ, Yardley JE, Riddell MC, Dunstan DW, Dempsey PC, et al. Physical activity/exercise and diabetes: A position statement of the American diabetes association. Diabetes Care (2016) 39(11):2065–79. doi: 10.2337/dc16-1728
10. Viana RB, de Lira CAB, Naves JPA, Coswig VS, Del Vecchio FB, Ramirez-Campillo R, et al. Can we draw general conclusions from interval training studies? Sport. Med (2018) 48(9):2001–9. doi: 10.1007/s40279-018-0925-1
11. Naves JPA, Rebelo ACS, Silva LRBE, Silva MS, Ramirez-Campillo R, Ramírez-Vélez R, et al. Cardiorespiratory and perceptual responses of two interval training and a continuous training protocol in healthy young men. Eur J Sport Sci (2019) 19(5):653–60. doi: 10.1080/17461391.2018.1548650
12. Souza D, Coswig V, de Lira CAB, Gentil P. H″IT″ting the barriers for exercising during social isolation. Biol (Basel). (2020) 9(9):245. doi: 10.3390/biology9090245
13. Tucker WJ, Sawyer BJ, Jarrett CL, Bhammar DM, Gaesser GA. Physiological responses to high-intensity interval exercise differing in interval duration. J Strength Cond. Res (2015) 1:3326–35. doi: 10.1519/JSC.0000000000001000
14. Kilpatrick MW, Martinez N, Little JP, Jung ME, Jones AM, Price NW, et al. Impact of high-intensity interval duration on perceived exertion. Med Sci Sport. Exerc (2015) 47(5):1038–45. doi: 10.1249/MSS.0000000000000495
15. Silva L.R.B.E.L.R.B.E., Zamunér ARAR, Gentil P, Alves FMFM, Leal A.G.F.A.G.F., Soares V, et al. Cardiac autonomic modulation and the kinetics of heart rate responses in the on- and off-transient during exercise in women with metabolic syndrome. Front Physiol (2017) 8:542(JUL). doi: 10.3389/fphys.2017.00542
16. Lehrke M, Marx N. Diabetes mellitus and heart failure. Am J Cardiol (2017) 120(1):S37–47. doi: 10.1016/j.amjcard.2017.05.014
17. Hwang C-L, Wu Y-T, Chou C-H. Effect of aerobic interval training on exercise capacity and metabolic risk factors in people with cardiometabolic disorders: a meta-analysis. J Cardiopulm. Rehabil. Prev (2011) 31(6):378–85. doi: 10.1097/HCR.0b013e31822f16cb
18. Liubaoerjijin Y, Terada T, Fletcher K, Boulé NG. Effect of aerobic exercise intensity on glycemic control in type 2 diabetes: a meta-analysis of head-to-head randomized trials. Acta Diabetol (2016) 53(5):769–81. doi: 10.1007/s00592-016-0870-0
19. Malachias M, Souza W, Plavnik FL, Rodrigues C, Brandão A. 7a diretriz brasileira de hipertensão arterial. Arq. Bras Cardiol (2016) 107(3). doi: 10.5935/abc.2013S010
20. Silva LRB, Gentil P, Seguro CS, de Oliveira JCM, Silva MS, Marques VA, et al. High-intensity interval training improves cardiac autonomic function in patients with type 2 diabetes: A randomized controlled trial. Biol (Basel). (2022) 11(1):66. doi: 10.3390/biology11010066
21. ACMS. Diretrizes do acsm para os testes de esforço e sua prescrição. Rio de Janeiro: Guanabara Koogan (2013).
22. Fletcher GF, Balady GJ, Amsterdam EA, Chaitman B, Eckel R, Fleg J. Exercise standards for testing and training: A statement for healthcare professionals from the American heart association. Circulation (2001) 104(14):1694–740. doi: 10.1161/hc3901.095960
24. Watt B, Grove R. Perceived exertion: Antecedents asd applications. Sport. Med (1993) 15(4):275–97. doi: 10.1016/B978-0-443-10260-8.50017-5
25. Mezzani A, Hamm LF, Jones AM, McBride PE, Moholdt T, Stone JA, et al. Aerobic exercise intensity assessment and prescription in cardiac rehabilitation: A joint position statement of the European association for cardiovascular prevention and rehabilitation, the American association of cardiovascular and pulmonary rehabilitat. Eur J Prev Cardiol (2013) 20(3):442–67. doi: 10.1177/2047487312460484
26. Whelton PK, Carey RM, Aronow WS, Casey DE, Collins KJ, Himmelfarb CD, et al. ACC/AHA/AAPA/ABC/ACPM/AGS/APhA/ASH/ASPC/NMA/PCNA guideline for the prevention, detection, evaluation and management of high blood pressure in adults: A report of the American college of Cardiology/American heart association task force on clinical practic. J Am Coll Cardiol (2018) 71(19):e127–248. doi: 10.1016/j.jacc.2017.11.006
27. Dudley GA, Abraham WM, Terjung RL. Influence of exercise intensity and duration on biochemical adaptations in skeletal muscle. J Appl Physiol Respir Environ Exerc. Physiol (1982) 53(4):844–50. doi: 10.1152/jappl.1982.53.4.844
28. Garvey WT, Maianu L, Huecksteadt TP, Birnbaum MJ, Molina JM, Ciaraldi TP. Pretranslational suppression of a glucose transporter protein causes insulin resistance in adipocytes from patients with non-insulin-dependent diabetes mellitus and obesity. J Clin Invest. (1991) 87(3):1072–81. doi: 10.1172/jci115068
29. Mavros Y, Simar D, Singh MAF. Glucose tranporter-4 expression in monocytes: A systematic review. Diabetes Res Clin Pract (2009) 84(2):123–31. doi: 10.1016/j.diabres.2009.02.014
30. Sticka KD, Schnurr TM, Jerome SP, Dajles A, Reynolds AJ, Duffy LK, et al. Exercise increases glucose transporter-4 levels on peripheral blood mononuclear cells. Med Sci Sports Exerc. (2018) 50(5):938–44. doi: 10.1249/MSS.0000000000001528
31. Kang J, Robertson RJ, Hagberg JM, Kelley DE, Goss FL, DaSilva SG, et al. Effect of exercise intensity on glucose and insulin metabolism in obese individuals and obese NIDDM patients. Diabetes Care (1996) 19(4):341–9. doi: 10.2337/DIACARE.19.4.341
32. Flemming D, Ingersen A, Andersen NB, Nielsen MB, Petersen HHH, Hansen CN, et al. Effects of one-legged high-intensity interval training on insulin-mediated skeletal muscle glucose homeostasis in patients with type 2 diabetes. Acta Physiol (Oxf) (2019) 226(2):e12345. doi: 10.1111/APHA.13245.
33. Schjerve IE, Tyldum G, Tjønna AE, Stølen T, Loennechen JP, Hansen HEM, et al. Both aerobic endurance and strength training programmes improve cardiovascular health in obese adults. Clin Sci (Lond). (2008) 115(9):283–93. doi: 10.1042/CS20070332
34. Stratton IM, Adler AI, Neil HA, Matthews DR, Manley SE, Cull CA, et al. Association of glycaemia with macrovascular and microvascular complications of type 2 diabetes (UKPDS 35): prospective observational study. B.M.J (2000) 321(7258):405–12. doi: 10.1136/bmj.321.7258.405
35. Selvin E, Marinopoulos S, Berkenblit G, Rami T, Brancati FL, Powe NR. Meta-analysis: glycosylated hemoglobin and cardiovascular disease in diabetes mellitus. Ann Intern Med (2004) 141(6):421–31. doi: 10.7326/0003-4819-141-6-200409210-00007
36. Winding KM, Munch GW, Iepsen UW, Van Hall G, Pedersen BK, Mortensen SP. The effect on glycaemic control of low-volume high-intensity interval training versus endurance training in individuals with type 2 diabetes. Diabetes Obes Metab (2018) 20(5):1131–9. doi: 10.1111/dom.13198
37. Umpierre D, Ribeiro PAB, Kramer CK, Leitão CB, Zucatti ATN, Azevedo MJ, et al. Physical activity advice only or structured exercise training and association with HbA1c levels in type 2 diabetes: A systematic review and meta-analysis. JAMA (2011) 305(17):1790–9. doi: 10.1001/jama.2011.576
38. Umpierre D, Ribeiro PAB, Schaan BD, Ribeiro JP. Volume of supervised exercise training impacts glycaemic control in patients with type 2 diabetes: A systematic review with meta-regression analysis. Diabetologia (2013) 56(2):242–51. doi: 10.1007/s00125-012-2774-z
39. Dal Canto E, Ceriello A, Rydén L, Ferrini M, Hansen TB, Schnell O, et al. Diabetes as a cardiovascular risk factor: An overview of global trends of macro and micro vascular complications. Eur J Prev Cardiol (2019) 26(2_suppl):25–32. doi: 10.1177/2047487319878371
40. Karstoft K, Winding K, Knudsen SH, Nielsen JS, Thomsen C, Pedersen BK, et al. The effects of free-living interval-walking training on glycemic control, body composition and physical fitness in type 2 diabetic patients: a randomized, controlled trial. Diabetes Care (2013) 36(2):228–36. doi: 10.2337/dc12-0658
41. Mitranun W, Deerochanawong C, Tanaka H, Suksom D. Continuous vs interval training on glycemic control and macro- and microvascular reactivity in type 2 diabetic patients. Scand J Med Sci Sports (2014) 24(2):e69–76. doi: 10.1111/sms.12112
42. Terada T, Friesen A, Chahal BS, Bell GJ, McCargar LJ, Boulé NG. Feasibility and preliminary efficacy of high intensity interval training in type 2 diabetes. Diabetes Res Clin Pract (2013) 99(2):120–9. doi: 10.1016/j.diabres.2012.10.019
43. Naci H, Salcher-Konrad M, Dias S, Blum MR, Sahoo SA, Nunan D, et al. How does exercise treatment compare with antihypertensive medications? a network meta-analysis of 391 randomised controlled trials assessing exercise and medication effects on systolic blood pressure. Br J Sports Med (2019) 53(14):859–69. doi: 10.1136/bjsports-2018-099921
44. Pedersen BK, Saltin B. Exercise as medicine - evidence for prescribing exercise as therapy in 26 different chronic diseases. Scand J Med Sci Sport. (2015) 25:1–72. doi: 10.1111/sms.12581
45. Pescatello LS, Franklin BA, Fagard R, Farquhar WB, Kelley GA, Ray CA, et al. American College of sports medicine position stand. exercise and hypertension. Med Sci Sports Exerc (2004) 36(3):533–53. doi: 10.1249/01.MSS.0000115224.88514.3A
46. Cornelissen VA, Smart NA. Exercise training for blood pressure: a systematic review and meta-analysis. J Am Hear. Assoc (2013) 2(1):e004473. doi: 10.1161/JAHA.112.0044732/1/e004473
47. Francois ME, Pistawka KJ, Halperin FA, Little JP. Cardiovascular benefits of combined interval training and post-exercise nutrition in type 2 diabetes. J Diabetes Complications (2018) 32(2):226–33. doi: 10.1016/j.jdiacomp.2017.10.002
48. Pattyn N, Coeckelberghs E, Buys R, Cornelissen VA, Vanhees L. Aerobic interval training vs. moderate continuous training in coronary artery disease patients: A systematic review and meta-analysis. Sport. Med (2014) 44(5):687–700. doi: 10.1007/s40279-014-0158-x
49. Ruffino JS, Songsorn P, Haggett M, Edmonds D, Robinson AM, Thompson D, et al. A comparison of the health benefits of reduced-exertion high-intensity interval training (REHIT) and moderate-intensity walking in type 2 diabetes patients. Appl Physiol Nutr Metab (2017) 42(2):202–8. doi: 10.1139/apnm-2016-0497
50. Storen Y, Helgerud J, Mona, Stoa EM, Bratland-Sanda S, Unhjem RJ, et al. The effect of age on the VO2max response to high-intensity interval training. Med Sci Sport. Exerc. (2017) 49(1):78–85. doi: 10.1249/MSS.0000000000001070
51. Stoa EM, Storen Ø. Response to comments on “High-intensity aerobic interval training improves aerobic fitness and HbA1c among persons diagnosed with type 2 diabetes.” Eur J Appl Physiol (2017) 117(7):1521–1. doi: 10.1007/s00421-017-3633-x
52. Kodama S, Saito K, Tanaka S, Maki M, Yachi Y, Asumi M, et al. Cardiorespiratory fitness as a quantitative predictor of all-cause mortality and cardiovascular events in healthy men and women: a meta-analysis. JAMA (2009) 301(19):2024–35. doi: 10.1001/jama.2009.681
53. Kokkinos P, Myers J. Exercise and physical activity: Clinical outcomes and applications. Circulation (2010) 122(16):1637–48. doi: 10.1161/CIRCULATIONAHA.110.948349
54. Ross R, Blair SN, Arena R, Church TS, Després JP, Franklin BA, et al. Importance of assessing cardiorespiratory fitness in clinical practice: A case for fitness as a clinical vital sign: A scientific statement from the American heart association. Circulation (2016) 134(24):e653–e699. doi: 10.1161/CIR.0000000000000461
55. Gollnick PD, Piehl K, Saltin B. Selective glycogen depletion pattern in human muscle fibres after exercise of varying intensity and at varying pedalling rates. J Physiol (1974) 241(1):45–57. doi: 10.1113/jphysiol.1974.sp010639
56. Altenburg TM, Degens H, Van Mechelen W, Sargeant AJ, De Haan A. Recruitment of single muscle fibers during submaximal cycling exercise. J Appl Physiol (2007) 103(5):1752–6. doi: 10.1152/japplphysiol.00496.2007
Keywords: diabetes mellitus, high-intensity interval training (HIIT), aerobic exercise (AE), glucose control, exercise is medicine
Citation: Gentil P, Silva LRBe, Antunes DE, Carneiro LB, de Lira CAB, Batista G, Oliveira JCMd, Cardoso JS, Souza DC and Rebelo ACS (2023) The effects of three different low-volume aerobic training protocols on cardiometabolic parameters of type 2 diabetes patients: A randomized clinical trial. Front. Endocrinol. 14:985404. doi: 10.3389/fendo.2023.985404
Received: 03 July 2022; Accepted: 03 January 2023;
Published: 23 January 2023.
Edited by:
João P. Magalhães, University of Lisbon, PortugalReviewed by:
Megan Hetherington-Rauth, Universidade de Lisboa, PortugalRomeu Mendes, University Porto, Portugal
Copyright © 2023 Gentil, Silva, Antunes, Carneiro, de Lira, Batista, Oliveira, Cardoso, Souza and Rebelo. This is an open-access article distributed under the terms of the Creative Commons Attribution License (CC BY). The use, distribution or reproduction in other forums is permitted, provided the original author(s) and the copyright owner(s) are credited and that the original publication in this journal is cited, in accordance with accepted academic practice. No use, distribution or reproduction is permitted which does not comply with these terms.
*Correspondence: Paulo Gentil, cGF1bG9nZW50aWxAaG90bWFpbC5jb20=