- Center for Biological Clocks Research, Department of Biology, Texas A&M University, College Station, TX, United States
Physical activity represents a potent, non-pharmacological intervention delaying the onset of over 40 chronic metabolic and cardiovascular diseases, including type 2 diabetes, coronary heart disease, and reducing all-cause mortality. Acute exercise improves glucose homeostasis, with regular participation in physical activity promoting long-term improvements in insulin sensitivity spanning healthy and disease population groups. At the skeletal muscle level, exercise promotes significant cellular reprogramming of metabolic pathways through the activation of mechano- and metabolic sensors, which coordinate downstream activation of transcription factors, augmenting target gene transcription associated with substrate metabolism and mitochondrial biogenesis. It is well established that frequency, intensity, duration, and modality of exercise play a critical role in the type and magnitude of adaptation; albeit, exercise is increasingly considered a vital lifestyle factor with a critical role in the entrainment of the biological clock. Recent research efforts revealed the time-of-day-dependent impact of exercise on metabolism, adaptation, performance, and subsequent health outcomes. The synchrony between external environmental and behavioural cues with internal molecular circadian clock activity is a crucial regulator of circadian homeostasis in physiology and metabolism, defining distinct metabolic and physiological responses to exercise unique to the time of day. Optimising exercise outcomes following when to exercise would be essential to establishing personalised exercise medicine depending on exercise objectives linked to disease states. We aim to provide an overview of the bimodal impact of exercise timing, i.e. the role of exercise as a time-giver (zeitgeber) to improve circadian clock alignment and the underpinning clock control of metabolism and the temporal impact of exercise timing on the metabolic and functional outcomes associated with exercise. We will propose research opportunities that may further our understanding of the metabolic rewiring induced by specific exercise timing.
1 Introduction
The circadian clock is an essential evolutionary mechanism permitting biological functions to be temporally controlled and pre-emptively coordinated in anticipation of food intake and physical activity. Located in the hypothalamic suprachiasmatic nucleus (SCN) of the brain, the central circadian clock or ‘master pacemaker’ is entrained by light and synchronises peripheral molecular clocks at the tissue level (1). The central and peripheral clocks ensure 24-hour rhythms of biological functions, including sleep-wake cycles, hormone secretion, cardiovascular function and whole-body metabolism (2). Although core clock machinery is ubiquitous across all tissues and cell types, clock transcriptional output is highly tissue-specific and is impacted by physiological cues such as eating, stress and physical activity (3); as such, the circadian clock induces 24-hour oscillations in substrate metabolism, glucose homeostasis (4), skeletal muscle insulin sensitivity (5) and oxidative capacity (6).
Circadian entrainment that aligns internal molecular clock rhythms with external time cues, such as waking and feeding times, is critical to maintaining circadian biological homeostasis. Thus, misalignment between the internal molecular clock and timed environmental cues is associated with several adverse health outcomes, as evidenced by large-scale epidemiological studies. For example, robust relationships between shift work, specifically night shifts, and metabolic disease risk with data from the Nurse Health Study revealing an increased risk of ischaemic stroke (7) and diabetes (8) in night-shift working participants compared to day working colleagues. In addition, Scandinavian population studies support the relationship between sleep disturbance and metabolic health, with Swedish and Danish night shift workers exhibiting greater obesity risk, lipid profile abnormalities, impaired glucose tolerance and diabetes compared to day-working colleagues (9, 10). Whilst correlation does not necessarily equal causality, it must be noted that simulated shift work experiments have revealed transcriptomic (11), endocrine (12, 13) and metabolic (14) circadian misalignment following relatively short intervention periods.
Shift working aside; the modern human lifestyle poses a severe threat to the entrainment and coordination of central and peripheral clocks with artificial 24-hour lighting, prolonged periods of inactivity and access to energy-dense foods, all disrupting the circadian rhythm (15–17). Notably, a recent cross-sectional investigation utilising primary skeletal muscle cells from type-2 diabetic and healthy control participants revealed divergent skeletal muscle mitochondrial metabolism (18) and altered clock-controlled gene expression, leading to impaired mitochondrial respiration in T2D skeletal muscle. Accordingly, exercise represents a significant, non-pharmacological intervention capable of preventing or delaying the onset of metabolic disease and reversing the adverse effects of the modern human lifestyle, including physical inactivity and poor diet (19, 20).
The timing of exercise has profound effects on physical capacity, homeostatic disturbance, and metabolic responses, potentially providing two-fold benefits. Firstly, exercise timing may act as a crucial time-giver (zeitgeber) for individuals and has been proposed as a strategy to synchronise and realign disrupted central and peripheral circadian clocks (21). Secondly, the circadian regulation of exercise, including the diurnal variation in exercise performance (22, 23) and alterations to substrate metabolism (17, 24, 25), may potentiate the beneficial effects of exercise and subsequent health outcomes.
On this basis, the present narrative review aims to provide an overview of the bimodal impact of exercise timing, i.e., the role of exercise as a zeitgeber to improve circadian clock alignment and the temporal impact of exercise timing on the metabolic and functional outcomes associated with exercise. We will highlight research opportunities that may identify exercise “prime times” for the biological outcomes and health benefits in response to exercise.
2 Exercise as a Zeitgeber
First proposed in 1951 by Jurgen Aschoff, the term zeitgeber (From the German for “time-giver”) (26) was described as an external stimulus that synchronises biological functions across a 24-hour cycle (27). Light is a critical zeitgeber that entrains the central circadian clock housed in hypothalamic suprachiasmatic nuclear (SCN) and synchronises peripheral tissue clocks (1) via neuroendocrine mechanisms (28, 29). The role of exercise as a potential zeitgeber has been discussed in the systematic review by Lewis and Colleagues (30), highlighting the impact of exercise on circadian phase shifts, the diurnal variation in exercise performance and the interaction between exercise and the circadian system to improve health.
Investigating the role of exercise as a zeitgeber is inherently difficult. It requires the precise control of both photic (light, either solar or ambient) and non-photic zeitgebers, including time of feeding and physical activity (31). Whilst the direct measurement of the molecular circadian clock activity is inherently problematic in humans, exercise has been repeatedly reported to induce significant phase shifts via several proxies of circadian rhythm, including hormone secretion (e.g., melatonin, cortisol and thyroid stimulating hormone [TSH]) and physiological parameters (e.g., body temperature and blood pressure) (32–38). Firstly, exercise can be introduced into ‘real-life’ situations of circadian misalignment to induce a circadian phase shift and realign circadian rhythms, albeit this approach is hampered by the difficulty in removing confounding variables. Attempting to alleviate the symptoms of jet lag and improve circadian alignment following trans-Atlantic travel, Milan-based endurance runners who completed daily evening exercise pre-travel observed improved sleep and circadian rest-activity cycles on arrival in New York (39). Despite reporting that bedtimes and wake times were controlled for each participant, the specific times were unclear in the study. As a result, altered light exposure may have contributed to improved adaptation masking the zeitgeber effect of exercise.
Alternatively, a “constant routine” approach may be implemented whereby sleep-wake cycles, mealtimes and physical activity are standardised before, during and after the introduction of the zeitgeber in question (i.e. exercise). By utilising this approach, any alteration in circadian rhythm may be attributed to introducing the ‘new’ zeitgeber, albeit with diminished ecological validity compared to the previous approach. Van Reeth et al. (36), were amongst the first to implement a constant routine approach to investigate the circadian phase-shifting effects of nocturnal exercise. To control for potential confounding zeitgebers, participants adhered to a constant routine for 7 days before each experimental trial, and food intake was prohibited. Instead, a constant intravenous infusion of glucose (5·g·kg·day-1) provided all caloric intake.
Additionally, exercise was standardised to the core body temperature nadir with the midpoint of exercise timed to 3 hours before, at and 2 hours after minimum core body temperature. Ultimately, night-time exercise was associated with melatonin and thyroid-stimulating hormone phase delays, with delays tending to be smaller when exercise was completed in the latter part of the night-time/early morning. Constant routine approaches have been implemented with varying levels of control, with some controlling for sleep-wake cycles, physical activity and nutritional intake with glucose infusion (33) or controlled mealtimes (32, 37) and have all shown robust melatonin phase delays when exercise is completed in the evening or overnight.
The mechanism by which exercise induces these phase shifts remains unclear. Nevertheless, the fact that repeated bouts of exercise stimulus were required to induce melatonin phase shifts suggests a zeitgeber effect of exercise was present (32). Specifically, morning exercise results in melatonin secretion phase delays whilst evening exercise-induced phase advances (33). The counter-intuitive phase shifting reported in response to exercise relative to light exposure adds to the notion that exercise per se induced the phase shift rather than light exposure.
Based on the current evidence available, it is appropriate to conclude that exercise has phase-shifting capabilities and based upon physiological changes in body temperature (38), melatonin (40) and thyroid stimulating hormone secretion (36) highlight exercise’s capacity to synchronise circadian rhythms. Furthermore, the entrainment of the biological clock may occur through exercise-induced changes in AMPK (41), transcriptional coactivator PGC-1α (42) and the transcription factor HIF-1α (43) activities, all of which have been associated with the molecular clock. Previous work from our group has highlighted the direct effect of exercise on the biological clock in mice (24) with supporting data in humans following both endurance (44) and resistance type exercise (45). Whilst the relationship between exercise and the biological clock is increasingly accepted, whether an optimal time exists to complete exercise remains to be resolved.
3 Exercise timing improves lifestyle factors and health
Whilst the benefits of exercise for health are beyond the scope of this review, the appreciation of physical activity as a key lifestyle factor for healthy living is well established (46–48). Nevertheless, the holistic benefits of exercise and the potential to correct misaligned circadian rhythms in at-risk populations present a unique opportunity to optimise exercise prescription (37). For instance, timed exercise may enhance the circadian rhythm entrainment in shift working individuals, potentially restoring temporal hormone secretion such as melatonin (33), improving sleep homeostasis and reducing the negative impact of health implications of the disturbance of sleep/wake cycles (11–13). When discussing the impact of timed exercise on circadian clock function and health, it is essential to consider the diurnal variation in exercise capacity (49). Improved exercise capacity coincides with several circadian-controlled biological functions collectively contributing to improved exercise capacity. Firstly, core body temperature is greatest during the late afternoon/early evening; unsurprisingly, skeletal muscle strength and oxidative capacity show comparable time-of-day variations and typically peak during the late afternoon and early evening, respectively (6, 50, 51). Furthermore, systemic hormone and metabolite concentrations show distinct responses to different times of exercise (24, 52, 53), which may contribute to time-specific exercise outcomes (54, 55).
The impact of exercise timing on diurnal exercise performance is a popular strategy for investigating the crosstalk between physical activity and circadian rhythms, with multiple studies utilising this approach (55–62). However, it is important to note that a ubiquitous limitation of this literature is the inability to isolate the impact of timed exercise relative to other zeitgebers, such as light exposure or mealtimes. Principally, morning exercise groups may wake and eat earlier, altering participants’ regular sleep-wake cycles and meal schedules, and may result in performance improvements due to phase advances. However, regarding performance differences, several studies have also reported changes in core temperature, with no difference in the circadian oscillation of core temperature between morning and evening exercise groups (60, 62). Additionally, no change in testosterone or cortisol was observed following 12 weeks of morning or evening exercise (55), raising the question of why changes in performance occur in the absence of changes in physiological markers of circadian rhythm.
Several studies highlight the interaction between exercise and the circadian system to improve health outcomes. As early as 1997, Van Someren and Colleagues (63) reported that 3 months of routine aerobic exercise, completed 3 times weekly, counteracted age-related fragmentation of the circadian clock, resulting in improved sleep quality in otherwise healthy older men (73 ± 2 years). Following the intervention, maximal aerobic fitness (VO2max) was significantly correlated with lower intraday variability in circadian rhythm, a critical finding given the association between aerobic capacity and all-cause mortality (46, 47). As well as improving sleep variables in older men, individualised exercise prescription improved sleep in patients with lung cancer compared to non-exercise controls (64), offsetting dysfunctional rest-activity rhythms pre-intervention (65). Notably, the benefit of prescribed exercise was greatest in individuals with poorer rest-activity rhythms, suggesting that the improvements observed may have had an underlying circadian component.
Human evidence highlighting the impact of exercise timing on health outcomes remains limited, with much of our current appreciation for the role of the circadian clock function and health inferred from animal models and epidemiological studies. However, recent evidence has provided crucial insight into the potential for timed exercise to improve metabolic health, with afternoon exercise revealing greater efficacy for improving glucose control in type 2 diabetes patients compared to morning-trained participants (66). Further multi-omics, multi-tissue (blood plasma, skeletal muscle, and adipose tissue) investigations from the same research group revealed significant temporal specificity following morning and evening exercise (67). Specifically, plasma carbohydrates were increased, with a decrease in skeletal muscle lipids following morning exercise, whereas afternoon HIT increased skeletal muscle lipids and mitochondrial protein content to a greater degree than morning training. Whilst the clinical significance of these findings warrants further investigation, the data presented by these authors support the notion that precisely timed exercise may lead to different exercise outcomes.
4 Omics approaches to capture metabolic signatures unique to exercise timing
The robust molecular interaction of the circadian clock to metabolic response to exercise indicates that exercise’s metabolic outputs might depend on its daily timing (17). Previous literature supports this notion that the timing of exercise, in addition to the quantity, duration, and type of exercise, could be one of the variables for metabolic and physiological responses to exercise (68, 69). However, understanding how the time of exercise rewires metabolic pathways in skeletal muscle and changes systemic metabolic adaptation remains an outstanding question. ‘Omics’ approaches, including metabolomics, transcriptomics, and proteomics, are considered effective in dissecting exercise’s time-of-day-dependent impact across the molecular time course of adaptation. Primarily global metabolite profiling provides a real-time fingerprint of metabolic activity and allows the identification of novel biochemicals unique to exercise timing. These may prove to be crucial diagnostic markers of metabolic disease patients and underpin differing metabolic responses to timed exercise.
Previously, our group performed high-throughput transcriptome and metabolome analyses in mouse skeletal muscle in response to a single-bout treadmill running exercise at different times of the day (24). Significantly, many transcripts and metabolites are intensively changed only upon exercise at the early active phase (biological morning) rather than the early rest phase (biological night), suggesting a substantial impact on skeletal muscle metabolic pathways. Performing integrative analysis of the transcriptome and metabolome dataset, we revealed that exercise in the morning selectively rewires skeletal muscle energy metabolic pathways, including glycolytic pathways, lipid oxidation, amino acid breakdown, and ketone metabolism. This skeletal muscle multi-omics study uncovers a functional commitment of exercise timing to determine exercise energy utilisation in skeletal muscle. An accompanying study from Asher’s group also performed mouse skeletal muscle metabolomic profiling upon exercise at the early versus the late active phase, underscoring the time-of-day-dependent impact of exercise on energy metabolism followed by endurance exercise performance (70). The molecular circadian clock modulates the exercise timing-dependent metabolic response and exercise capacity (53). In addition, proteomic and phosphoproteomic profiles upon acute exercise at different daily timing also point to differential biological responses in skeletal muscle unique to the exercise timing (71). Proteins involved in energy provision and catabolic pathways are preferentially enriched after morning and evening exercise. Lastly, skeletal muscle and multiple organ systems dependently and collectively respond to exercise unique to the time of day (52). Our follow-up study investigated global metabolite profiles on 8 different tissues (skeletal muscle, liver, heart, white adipose tissues, brown adipose tissue, hypothalamus, and blood) collected from mice subjected to an acute treadmill exercise at different times of the day (Figure 1A). This allowed the detection of hundreds of different signalling molecules, of both metabolic and endocrine origin, across multiple tissues and to investigate their change in response to exercise at differing times of the day. Comparative analyses of cross-tissue metabolite dynamics, as well as an arteriovenous sampling of hindlimb muscle and sampling across the liver, lead to a deeper understanding of time-of-day-specific tissue cross-talk following exercise (Figure 1B). Finally, the study identified new exercise-induced signalling molecules (exerkines) in multiple tissues, which require further investigation to understand how they can individually or collectively influence health. The most recent study unveiled a comprehensive response of multi-tissue and multi-omics to exercise training at different times of the day in men with type 2 diabetes, revealing coordinated systemic metabolic adaptation to timed exercise (67). Thus, omics resources direct further research that can help us better understand how exercise, if timed correctly, can improve human health.
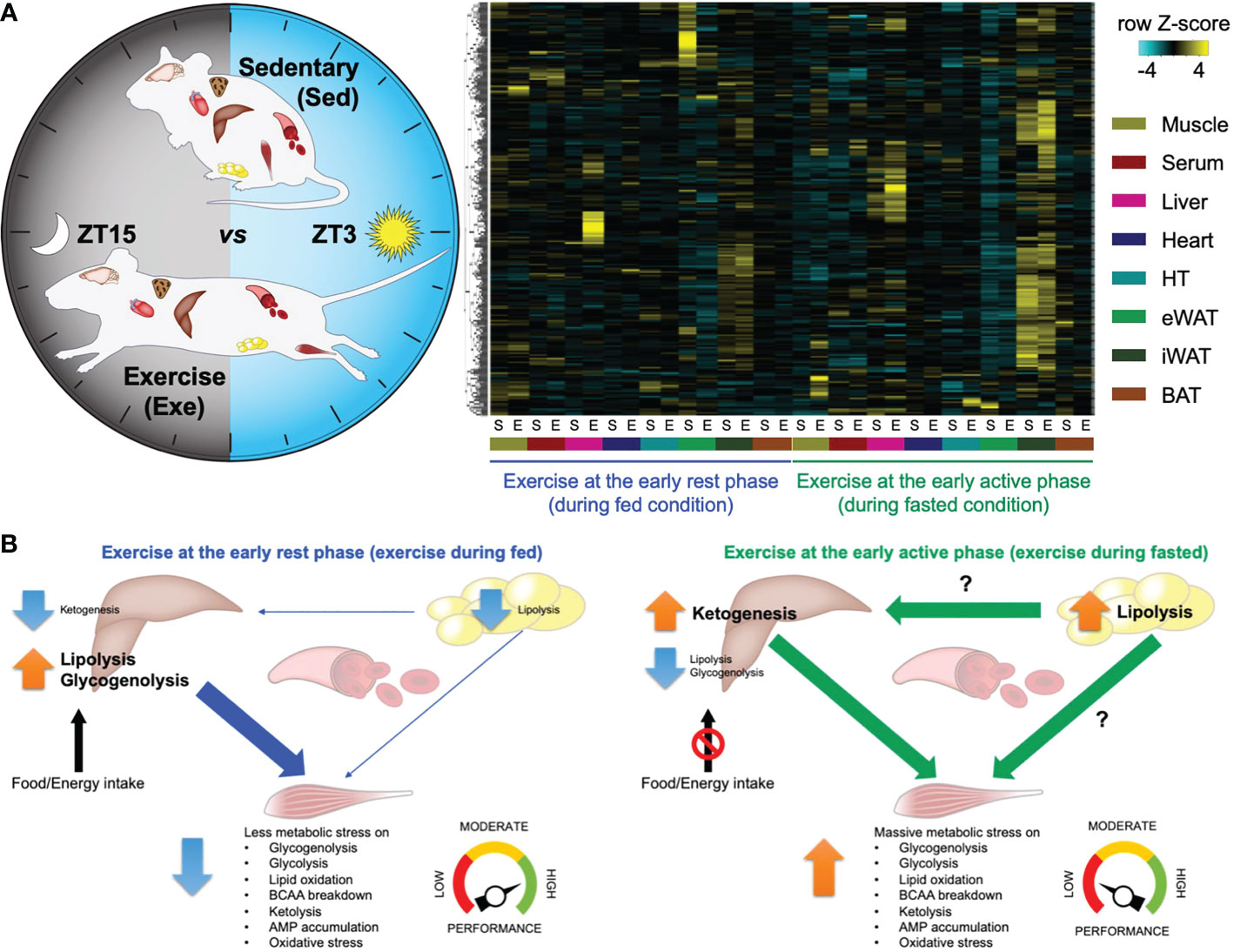
Figure 1 – Atlas of exercise metabolism unique to the time of day reveals tissue-dependent and collective metabolic responses to timed exercise (52). (A) Multi-tissue metabolomics upon exercise at the early rest (ZT3) versus the early active phase (ZT15). Heatmap displaying metabolites significantly changed only in a specific tissue and only after specific timing of exercise. (B) Schematic summary representing time-of-exercise-specificity of types of energy and the specific tissue of origin for energy during exercise. This figure represents a graphical representation of metabolomics data generated with the author’s permission and data from Sato et al., 2022 (52).
5 Research opportunities
Exercise-induced signalling metabolites (so-called exerkines) act as stimuli and substrates for cellular energy sensors and signalling molecules and induce adaptive rewiring of signalling pathways and transcriptional networks in response to exercise training (72). Novel investigations by our group have highlighted the impact of time-of-day of exercise on metabolic responses to acute exercise in skeletal muscle (24), with more recent work providing multi-tissue metabolomic analysis in response to time-of-day specific exercise (52). This work revealed exercise-stimulated signalling molecules capable of transducing metabolic information and mediating circadian reprogramming via energy sensors, AMP-activated protein kinase (AMPK), histone modifiers, e.g., histone deacetylases (HDACs) and transcription factors, including hypoxia-inducible factor 1α (HIF-1α). Alongside mechanisms for circadian reprogramming in response to exercise timing, work from our group highlights several metabolites of interest which have demonstrated capacity for clock modification through metabolic and epigenetic mechanisms. Whilst these metabolites have shown to be impactful in animal models, the translational applicability to humans remains to be seen. Future research should investigate whether these molecules’ administration may enhance the efficacy of timed exercise interventions or offer a potential pharmacological intervention for populations with misaligned circadian clocks to delay or prevent the onset of metabolic disease.
6 Conclusion
This review has discussed the bimodal impact of exercise timing on the circadian clock, including the realignment of misaligned clocks and the underpinning impact on metabolic regulation and health outcomes. First, it is essential to consider the population groups of interest within these studies. Crucially, individuals with metabolic disease risk are unlikely to be completing recommended daily/weekly physical activity; as such, the primary focus for this population should be promoting exercise participation, irrespective of timing to improve overall health. Secondly, exercise timing may only confer a trivial benefit relative to any exercise. Nevertheless, recent evidence has provided crucial insight into the metabolic impact of timed exercise in metabolically diseased humans, revealing robust differences between morning and evening exercise and providing early evidence to support the notion of “fine-tuning” exercise completion for the optimisation of health outcomes.
Whilst the timing of exercise may represent a novel strategy for optimising health outcomes and realignment of the circadian clock, it is essential to note that exercise at any time of day is of greater benefit than no exercise. In population groups who fail to meet daily recommended physical activity targets, the timing of exercise may offer trivial differences compared to exercise at any time.
Author contributions
SB and SS wrote the manuscript, prepared the figure, and approved the final version. All authors contributed to the article and approved the submitted version.
Funding
Start-up funds from Texas A&M University support SS.
Conflict of interest
The authors declare that the research was conducted in the absence of any commercial or financial relationships that could be construed as a potential conflict of interest.
Publisher’s note
All claims expressed in this article are solely those of the authors and do not necessarily represent those of their affiliated organizations, or those of the publisher, the editors and the reviewers. Any product that may be evaluated in this article, or claim that may be made by its manufacturer, is not guaranteed or endorsed by the publisher.
References
1. Hastings MH, Maywood ES, Brancaccio M. Generation of circadian rhythms in the suprachiasmatic nucleus. Nat Rev Neurosci (2018) 19(8):453–69. doi: 10.1038/s41583-018-0026-z
2. Rajaratnam SM, Arendt J. Health in a 24-h society. Lancet (2001) 358(9286):999–1005. doi: 10.1016/S0140-6736(01)06108-6
3. Takahashi JS. Transcriptional architecture of the mammalian circadian clock. Nat Rev Genet (2017) 18(3):164–79. doi: 10.1038/nrg.2016.150
4. Verrillo A, De Teresa A, Martino C, Di Chiara G, Pinto M, Verrillo L, et al. Differential roles of splanchnic and peripheral tissues in determining diurnal fluctuation of glucose tolerance. Am J Physiol (1989) 257(4 Pt 1):E459–65. doi: 10.1152/ajpendo.1989.257.4.E459
5. Van Cauter E, Blackman JD, Roland D, Spire JP, Refetoff S, Polonsky KS. Modulation of glucose regulation and insulin secretion by circadian rhythmicity and sleep. J Clin Invest (1991) 88(3):934–42. doi: 10.1172/JCI115396
6. van Moorsel D, Hansen J, Havekes B, Scheer FAJL, Jörgensen JA, Hoeks J, et al. Demonstration of a day-night rhythm in human skeletal muscle oxidative capacity. Mol Metab (2016) 5(8):635–45. doi: 10.1016/j.molmet.2016.06.012
7. Brown DL, Feskanich D, Sánchez BN, Rexrode KM, Schernhammer ES, Lisabeth LD. Rotating night shift work and the risk of ischemic stroke. Am J Epidemiol (2009) 169(11):1370–7. doi: 10.1093/aje/kwp056
8. Pan A, Schernhammer ES, Sun Q, Hu FB. Rotating night shift work and risk of type 2 diabetes: Two prospective cohort studies in women. PloS Med (2011) 8(12):e1001141. doi: 10.1371/journal.pmed.1001141
9. Karlsson B, Knutsson A, Lindahl B. Is there an association between shift work and having a metabolic syndrome? Results from a population based study of 27,485 people. Occup Environ Med (2001) 58(11):747–52. doi: 10.1136/oem.58.11.747
10. Hansen AB, Stayner L, Hansen J, Andersen ZJ. Night shift work and incidence of diabetes in the Danish nurse cohort. Occup Environ Med (2016) 73(4):262–8. doi: 10.1136/oemed-2015-103342
11. Kervezee L, Cuesta M, Cermakian N, Boivin DB. Simulated night shift work induces circadian misalignment of the human peripheral blood mononuclear cell transcriptome. Proc Natl Acad Sci USA (2018) 115(21):5540–5. doi: 10.1073/pnas.1720719115
12. Bescos R, Boden MJ, Jackson ML, Trewin AJ, Marin EC, Levinger I, et al. Four days of simulated shift work reduces insulin sensitivity in humans. Acta Physiol (Oxf) (2018) 223(2):e13039. doi: 10.1111/apha.13039
13. James FO, Cermakian N, Boivin DB. Circadian rhythms of melatonin, cortisol, and clock gene expression during simulated night shift work. Sleep (2007) 30(11):1427–36. doi: 10.1093/sleep/30.11.1427
14. Hampton SM, Morgan LM, Lawrence N, Anastasiadou T, Norris F, Deacon S, et al. Postprandial hormone and metabolic responses in simulated shift work. J Endocrinol (1996) 151(2):259–67. doi: 10.1677/joe.0.1510259
15. Asher G, Sassone-Corsi P. Time for food: The intimate interplay between nutrition, metabolism, and the circadian clock. Cell (2015) 161(1):84–92. doi: 10.1016/j.cell.2015.03.015
16. Panda S. Circadian physiology of metabolism. Science (2016) 354(6315):1008–15. doi: 10.1126/science.aah4967
17. Gabriel BM, Zierath JR. Circadian rhythms and exercise - re-setting the clock in metabolic disease. Nat Rev Endocrinol (2019) 15(4):197–206. doi: 10.1038/s41574-018-0150-x
18. Gabriel BM, Altıntaş A, Smith JAB, Sardon-Puig L, Zhang X, Basse AL, et al. Disrupted circadian oscillations in type 2 diabetes are linked to altered rhythmic mitochondrial metabolism in skeletal muscle. Sci Adv (2021) 7(43):eabi9654. doi: 10.1126/sciadv.abi9654
19. Booth FW, Gordon SE, Carlson CJ, Hamilton MT. Waging war on modern chronic diseases: primary prevention through exercise biology. J Appl Physiol (2000) 88(2):774–87. doi: 10.1152/jappl.2000.88.2.774
20. Ruegsegger GN, Booth FW. Health benefits of exercise. Cold Spring Harbor Perspect Med (2018) 8(7). doi: 10.1101/cshperspect.a029694
21. Aoyama S, Shibata S. Time-of-Day-Dependent physiological responses to meal and exercise. Front Nutr (2020) 7:18. doi: 10.3389/fnut.2020.00018
22. Chtourou H, Souissi N. The effect of training at a specific time of day: A review. J Strength Cond Res (2012) 26(7):1984–2005. doi: 10.1519/JSC.0b013e31825770a7
23. Facer-Childs E, Brandstaetter R. The impact of circadian phenotype and time since awakening on diurnal performance in athletes. Curr Biol (2015) 25(4):518–22. doi: 10.1016/j.cub.2014.12.036
24. Sato S, Basse AL, Schönke M, Chen S, Samad M, Altıntaş A, et al. Time of exercise specifies the impact on muscle metabolic pathways and systemic energy homeostasis. Cell Metab (2019) 30(1):92–110 e4. doi: 10.1016/j.cmet.2019.03.013
25. Vieira AF, Costa RR, Macedo RC, Coconcelli L, Kruel LF. Effects of aerobic exercise performed in fasted v. fed state on fat and carbohydrate metabolism in adults: A systematic review and meta-analysis. Br J Nutr (2016) 116(7):1153–64. doi: 10.1017/S0007114516003160
26. Aschoff J. Die 24-Stunden-Periodik der maus unter konstanten umgebungsbedingungen. Naturwissenschaften (1951) 38(21):506–7. doi: 10.1007/BF00628863
27. Aschoff J. Zeitgeber der tierischen tagesperiodik. Naturwissenschaften (1954) 41(3):49–56. doi: 10.1007/BF00634164
28. Erren TC, Reiter RJ. Melatonin: a universal time messenger. Neuroendocrinol Lett (2015) 36(3):187–92.
29. Kalsbeek A, Palm IF, La Fleur SE, Scheer FA, Perreau-Lenz S, Ruiter M, et al. SCN outputs and the hypothalamic balance of life. J Biol Rhythms (2006) 21(6):458–69. doi: 10.1177/0748730406293854
30. Lewis P, Korf HW, Kuffer L, Groß JV, Erren TC. Exercise time cues (zeitgebers) for human circadian systems can foster health and improve performance: A systematic review. BMJ Open Sport Exerc Med (2018) 4(1):e000443. doi: 10.1136/bmjsem-2018-000443
31. Atkinson G, Edwards B, Reilly T, Waterhouse J. Exercise as a synchroniser of human circadian rhythms: An update and discussion of the methodological problems. Eur J Appl Physiol (2007) 99(4):331–41. doi: 10.1007/s00421-006-0361-z
32. Barger LK, Wright KP Jr, Hughes RJ, Czeisler CA. Daily exercise facilitates phase delays of circadian melatonin rhythm in very dim light. Am J Physiol-Regul Integr Comp Physiol (2004) 286(6):R1077–84. doi: 10.1152/ajpregu.00397.2003
33. Buxton OM, Lee CW, L'Hermite-Baleriaux M, Turek FW, Van Cauter E. Exercise elicits phase shifts and acute alterations of melatonin that vary with circadian phase. Am J Physiol-Regul Integr Comp Physiol (2003) 284(3):R714–24. doi: 10.1152/ajpregu.00355.2002
34. Baehr EK, Eastman CI, Revelle W, Olson SH, Wolfe LF, Zee PC. Circadian phase-shifting effects of nocturnal exercise in older compared with young adults. Am J Physiol-Regul Integr Comp Physiol (2003) 284(6):R1542–50. doi: 10.1152/ajpregu.00761.2002
35. Youngstedt SD, Kline CE, Elliott JA, Zielinski MR, Devlin TM, Moore TA. Circadian phase-shifting effects of bright light vs. exercise and bright light and exercise combined. Med Sci Sports Exercise (2006) 38(5):S99–9. doi: 10.1249/00005768-200605001-01339
36. Vanreeth O, Sturis J, Byrne MM, Blackman JD, L'Hermite-Balériaux M, Leproult R, et al. Nocturnal exercise phase delays circadian-rhythms of melatonin and thyrotropin secretion in normal men. Am J Physiol (1994) 266(6):E964–74. doi: 10.1152/ajpendo.1994.266.6.E964
37. Miyazaki T, Hashimoto S, Masubuchi S, Honma S, Honma KI. Phase-advance shifts of human circadian pacemaker are accelerated by daytime physical exercise. Am J Physiol-Regul Integr Comp Physiol (2001) 281(1):R197–205. doi: 10.1152/ajpregu.2001.281.1.R197
38. Edwards B, Waterhouse J, Atkinson G, Reilly T. Exercise does not necessarily influence the phase of the circadian rhythm in temperature in healthy humans. J Sports Sci (2002) 20(9):725–32. doi: 10.1080/026404102320219437
39. Montaruli A, Roveda E, Calogiuri G, La Torre A, Carandente F. The sportsman readjustment after transcontinental flight: A study on marathon runners. J Sports Med Phys Fitness (2009) 49(4):372–81.
40. Youngstedt SD, Elliott JA, Kripke DF. Human circadian phase-response curves for exercise. J Physiol (2019) 597(8):2253–68. doi: 10.1113/JP276943
41. Lassiter DG, Sjögren RJO, Gabriel BM, Krook A, Zierath JR. AMPK activation negatively regulates GDAP1, which influences metabolic processes and circadian gene expression in skeletal muscle. Mol Metab (2018) 16:12–23. doi: 10.1016/j.molmet.2018.07.004
42. Liu C, Li S, Liu T, Borjigin J, Lin JD. Transcriptional coactivator PGC-1alpha integrates the mammalian clock and energy metabolism. Nature (2007) 447(7143):477–81. doi: 10.1038/nature05767
43. Peek CB, Levine DC, Cedernaes J, Taguchi A, Kobayashi Y, Tsai SJ, et al. Circadian clock interaction with HIF1alpha mediates oxygenic metabolism and anaerobic glycolysis in skeletal muscle. Cell Metab (2017) 25(1):86–92. doi: 10.1016/j.cmet.2016.09.010
44. Dickinson JM, D'Lugos AC, Naymik MA, Siniard AL, Wolfe AJ, Curtis D, et al. Transcriptome response of human skeletal muscle to divergent exercise stimuli. J Appl Physiol (1985) (2018) 124(6):1529–40. doi: 10.1152/japplphysiol.00014.2018
45. Zambon AC, McDearmon EL, Salomonis N, Vranizan KM, Johansen KL, Adey D, et al. Time- and exercise-dependent gene regulation in human skeletal muscle. Genome Biol (2003) 4(10):R61. doi: 10.1186/gb-2003-4-10-r61
46. Blair SN, Kohl HW 3rd, Barlow CE, Paffenbarger RS, Gibbons LW Jr, Macera CA. Changes in physical-fitness and all-cause mortality - a prospective-study of healthy and unhealthy men. Jama-Journal Am Med Assoc (1995) 273(14):1093–8. doi: 10.1001/jama.1995.03520380029031
47. Blair SN, Kohl HW 3rd, Paffenbarger RS, Clark DG Jr, Cooper KH, Gibbons LW. Physical-fitness and all-cause mortality - a prospective-study of healthy-men and women. Jama-Journal Am Med Assoc (1989) 262(17):2395–401. doi: 10.1001/jama.1989.03430170057028
48. Lee CD, Blair SN, Jackson AS. Cardiorespiratory fitness, body composition, and all-cause and cardiovascular disease mortality in men. Am J Clin Nutr (1999) 69(3):373–80. doi: 10.1093/ajcn/69.3.373
49. Lok R, Zerbini G, Gordijn MCM, Beersma DGM, Hut RA. Gold, silver or bronze: circadian variation strongly affects performance in Olympic athletes. Sci Rep (2020) 10(1):16088. doi: 10.1038/s41598-020-72573-8
50. Wens I, Hansen D. Muscle strength, but not muscle oxidative capacity, varies between the morning and the afternoon in patients with multiple sclerosis: A pilot study. Am J Phys Med Rehabil (2017) 96(11):828–30. doi: 10.1097/PHM.0000000000000703
51. de Goede P, Wefers J, Brombacher EC, Schrauwen P, Kalsbeek A. Circadian rhythms in mitochondrial respiration. J Mol Endocrinol (2018) 60(3):R115–30. doi: 10.1530/JME-17-0196
52. Sato S, Dyar KA, Treebak JT, Jepsen SL, Ehrlich AM, Ashcroft SP, et al. Atlas of exercise metabolism reveals time-dependent signatures of metabolic homeostasis. Cell Metab (2022) 34(2):329–45.e8. doi: 10.1016/j.cmet.2021.12.016
53. Adamovich Y, Dandavate V, Ezagouri S, Manella G, Zwighaft Z, Sobel J, et al. Clock proteins and training modify exercise capacity in a daytime-dependent manner. Proc Natl Acad Sci USA (2021) 118(35):e2101115118. doi: 10.1073/pnas.2101115118
54. Boukelia B, Gomes EC, Florida-James GD. Diurnal variation in physiological and immune responses to endurance sport in highly trained runners in a hot and humid environment. Oxid Med Cell Longev (2018) 2018:3402143. doi: 10.1155/2018/3402143
55. Kuusmaa M, Schumann M, Sedliak M, Kraemer WJ, Newton RU, Malinen JP, et al. Effects of morning versus evening combined strength and endurance training on physical performance, muscle hypertrophy, and serum hormone concentrations. Appl Physiol Nutr Metab (2016) 41(12):1285–94. doi: 10.1139/apnm-2016-0271
56. Edwards BJ, Edwards W, Waterhouse J, Atkinson G, Reilly T. Can cycling performance in an early morning, laboratory-based cycle time-trial be improved by morning exercise the day before? Int J Sports Med (2005) 26(8):651–6. doi: 10.1055/s-2004-830439
57. Blonc S, Perrot S, Racinais S, Aussepe S, Hue O. Effects of 5 weeks of training at the same time of day on the diurnal variations of maximal muscle power performance. J Strength Cond Res (2010) 24(1):23–9. doi: 10.1519/JSC.0b013e3181b295d6
58. Imafuku M. Body temperature rhythm and control of the time of the best physical condition by performing physical labor. Chronobiol Int (2016) 33(4):431–4. doi: 10.3109/07420528.2016.1144609
59. Souissi N, Gauthier A, Sesboüé B, Larue J, Davenne D. Effects of regular training at the same time of day on diurnal fluctuations in muscular performance. J Sports Sci (2002) 20(11):929–37. doi: 10.1080/026404102320761813
60. Chtourou H, Driss T, Souissi S, Gam A, Chaouachi A, Souissi N. The effect of strength training at the same time of the day on the diurnal fluctuations of muscular anaerobic performances. J Strength Cond Res (2012) 26(1):217–25. doi: 10.1519/JSC.0b013e31821d5e8d
61. Chtourou H, Chaouachi A, Driss T, Dogui M, Behm DG, Chamari K, et al. The effect of training at the same time of day and tapering period on the diurnal variation of short exercise performances. J Strength Cond Res (2012) 26(3):697–708. doi: 10.1519/JSC.0b013e3182281c87
62. Zbidi S, Zinoubi B, Vandewalle H, Driss T. Diurnal rhythm of muscular strength depends on temporal specificity of self-resistance training. J Strength Cond Res (2016) 30(3):717–24. doi: 10.1519/JSC.0000000000001144
63. VanSomeren EJW, Lijzenga C, Mirmiran M, Swaab DF. Long-term fitness training improves the circadian rest-activity rhythm in healthy elderly males. J Biol Rhythms (1997) 12(2):146–56. doi: 10.1177/074873049701200206
64. Chen HM, Tsai CM, Wu YC, Lin KC, Lin CC. Effect of walking on circadian rhythms and sleep quality of patients with lung cancer: A randomised controlled trial. Br J Cancer (2016) 115(11):1304–12. doi: 10.1038/bjc.2016.356
65. Chen HM, Wu YC, Tsai CM, Tzeng JI, Lin CC. Relationships of circadian rhythms and physical activity with objective sleep parameters in lung cancer patients. Cancer Nurs (2015) 38(3):215–23. doi: 10.1097/NCC.0000000000000163
66. Savikj M, Gabriel BM, Alm PS, Smith J, Caidahl K, Björnholm M, et al. Afternoon exercise is more efficacious than morning exercise at improving blood glucose levels in individuals with type 2 diabetes: A randomised crossover trial. Diabetologia (2019) 62(2):233–7. doi: 10.1007/s00125-018-4767-z
67. Savikj M, Stocks B, Sato S, Caidahl K, Krook A, Deshmukh AS, et al. Exercise timing influences multi-tissue metabolome and skeletal muscle proteome profiles in type 2 diabetic patients - a randomized crossover trial. Metabolism (2022) 135:155268. doi: 10.1016/j.metabol.2022.155268
68. Schroeder AM, Truong D, Loh DH, Jordan MC, Roos KP, Colwell CS. Voluntary scheduled exercise alters diurnal rhythms of behaviour, physiology and gene expression in wild-type and vasoactive intestinal peptide-deficient mice. J Physiol (2012) 590(23):6213–26. doi: 10.1113/jphysiol.2012.233676
69. Sasaki H, Ohtsu T, Ikeda Y, Tsubosaka M, Shibata S. Combination of meal and exercise timing with a high-fat diet influences energy expenditure and obesity in mice. Chronobiol Int (2014) 31(9):959–75. doi: 10.3109/07420528.2014.935785
70. Ezagouri S, Zwighaft Z, Sobel J, Baillieul S, Doutreleau S, Ladeuix B, et al. Physiological and molecular dissection of daily variance in exercise capacity. Cell Metab (2019) 30(1):78–91 e4. doi: 10.1016/j.cmet.2019.03.012
71. Maier G, Delezie J, Westermark PO, Santos G, Ritz D, Handschin C. Transcriptomic, proteomic and phosphoproteomic underpinnings of daily exercise performance and zeitgeber activity of training in mouse muscle. J Physiol (2022) 600(4):769–96. doi: 10.1113/JP281535
Keywords: exercise, cardiometabolic health, metabolic diseases, circadian biology, time-of-exercise, metabolism, physical activity, high-throughput omics data
Citation: Bennett S and Sato S (2023) Enhancing the metabolic benefits of exercise: Is timing the key? Front. Endocrinol. 14:987208. doi: 10.3389/fendo.2023.987208
Received: 05 July 2022; Accepted: 31 January 2023;
Published: 15 February 2023.
Edited by:
Karyn Esser, University of Florida, United StatesReviewed by:
Josiane Broussard, Colorado State University, United StatesBrendan Gabriel, University of Aberdeen, United Kingdom
Copyright © 2023 Bennett and Sato. This is an open-access article distributed under the terms of the Creative Commons Attribution License (CC BY). The use, distribution or reproduction in other forums is permitted, provided the original author(s) and the copyright owner(s) are credited and that the original publication in this journal is cited, in accordance with accepted academic practice. No use, distribution or reproduction is permitted which does not comply with these terms.
*Correspondence: Shogo Sato, c2hvZ28uc2F0b0BiaW8udGFtdS5lZHU=