- 1College of Physical Education and Health, Guangxi Normal University, Guilin, China
- 2Department of Physical Education, Hebei University of Environmental Engineering, Qinhuangdao, China
Introduction: Exploring the energy expenditure and substrate metabolism data during exercise, 10-minute recovery, and 20-minute recovery phases in Tabata, HIIT(High-Intensity Interval Training), and MICT(Moderate-Intensity Continuous Training). This study explores the scientific aspects of weight reduction strategies, examining energy expenditure and substrate metabolism from various training perspectives. The aim is to establish a theoretical foundation for tailoring targeted exercise plans for individuals within the population with overweight/obesity.
Methods: This study used an experimental design with fifteen male university students with overweight/obesity. Participants underwent random testing with Tabata, HIIT, and MICT. Tabata involved eight sets of 20 seconds exercise and 10 seconds rest, totaling 4 minutes. HIIT included four sets of power cycling: 3 minutes at 80% VO2max intensity followed by 2 minutes at 20% VO2max. MICT comprised 30 minutes of exercise at 50% VO2max intensity. Gas metabolism indices were continuously measured. Subsequently, fat and glucose oxidation rates, along with energy expenditure, were calculated for each exercise type.
Results: During both the exercise and recovery phases, the Tabata group exhibited a significantly higher fat oxidation rate of (0.27 ± 0.03 g/min) compared to the HIIT group (0.20 ± 0.04 g/min, p<0.05) and the MICT group (0.20 ± 0.03g/min, p<0.001). No significant difference was observed between the HIIT and MICT groups (p=0.854). In terms of energy expenditure rate, the Tabata group maintained a substantially elevated level at 5.76 ± 0.74kcal/min compared to the HIIT group (4.81 ± 0.25kcal/min, p<0.01) and the MICT group (3.45 ± 0.25kcal/min, p<0.001). Additionally, the energy expenditure rate of the HIIT group surpassed that of the MICT group significantly (p<0.001).
Conclusion: The study finds that male college students with overweight/obesity in both exercise and recovery, Tabata group has lower fat and glucose oxidation rates, and energy expenditure compared to HIIT and MICT groups. However, over the entire process, Tabata still exhibits significantly higher rates in these aspects than HIIT and MICT. Despite a shorter exercise duration, Tabata shows a noticeable “time-efficiency” advantage. Tabata can be used as an efficient short-term weight loss exercise program for male college students with overweight/obesity.
1 Introduction
In recent years, the improvement of living standards has brought about various health issues, with obesity being a global public health concern. The sedentary lifestyle and changes in dietary habits associated with socio-economic development have contributed to a rapid increase in global obesity rates (1). According to the World Obesity Federation’s “World Obesity Atlas 2023” report, it is projected that over the next 12 years, more than 51% of the global population—exceeding 4 billion people—will be either obese or overweight. Research indicates that obesity is a significant risk factor for chronic diseases such as cardiovascular diseases, diabetes, and musculoskeletal disorders (2). The high prevalence of obesity significantly affects the health and societal development in China, making the scientific and efficient approach to weight loss a critical issue.
Traditional weight loss exercises often involve MICT(Moderate-Intensity Continuous Training), such as walking, brisk walking, running, and cycling, which has proven to be effective (3). However, the lengthy and monotonous nature of the exercise makes it challenging for many individuals with obesity to adhere to. In recent years, HIIT(High-Intensity Interval Training) has gained attention. Initially proposed by Reindell and Roskamm (4), HIIT has become popular among fitness enthusiasts. HIIT involves repeated cycles of high-intensity exercise interspersed with low-intensity exercise or rest periods with varying recovery times (5). Compared to traditional long-duration continuous exercise, HIIT has a relatively short exercise duration but higher intensity (6, 7). HIIT is considered an exercise method that increases the metabolic rate and promotes fat consumption (8). The primary energy systems during HIIT are the phosphagen and glycolytic systems, with glucose being the main metabolic substrate. Therefore, the efficacy of HIIT in reducing fat is not solely due to the energy expenditure during exercise but also the significant aerobic demand fluctuations that enhance post-exercise metabolic rate and fat utilization (9).
However, traditional HIIT training still requires more than 20 minutes. This is where Tabata comes into play. Originating in Japan, Tabata involves 20 seconds of exercise, 10 seconds of rest, repeated for eight sets, totaling a 4-minute interval training session (10, 11). Compared to HIIT and MICT, Tabata offers advantages such as a relatively short exercise duration, high enjoyment, unrestricted space requirements, and easy implementation, making it more accessible for individuals with overweight/obesity to adhere to.
Current research primarily focuses on the effects of different exercise modalities on body composition, body components, and weight loss. Many scholars have conducted comparative studies on the fitness effects of HIIT and MICT, finding that for the same energy expenditure, HIIT yields a greater increase in VO2max [23.8% vs 19.3% (12), 15.8% vs 8.0% (13), 14% vs 7% (14)]. Moreover, HIIT has been shown to reduce insulin and blood sugar levels, improve insulin sensitivity, and enhance the mechanisms of blood dynamics, metabolism, and endocrine factors involved in the pathogenesis of hypertension in healthy populations (13). The effects of HIIT are also superior to those of MICT for reducing total fat mass and abdominal fat mass, even with the same energy expenditure (15).
Physical exercise promotes browning of adipocytes, which is a transition from white adipose tissue to metabolically active brown adipose tissue. The characteristic of browning is an increase in the number of mitochondria and the dissipation of energy through heat generation, namely non shivering heat generation. Due to the large amount of energy consumed by brown adipose tissue in non shivering heat generation, this can lead to an overall increase in energy consumption. Physical exercise can promote more effective energy consumption in the body by activating these physiological processes. This is precisely why this study aims to provide new insights into the field of exercise and weight loss for obese individuals from the perspectives of energy expenditure and substrate metabolism through different exercise methods.
The debate over the superiority of weight loss effects between HIIT and MICT is a current hotspot. Although some studies suggest that HIIT is more effective for weight loss than MICT, due to differences in research methods and training programs, it is premature to conclude that HIIT is superior to MICT for weight loss (16, 17). With the emergence of Tabata, a form of exercise with shorter duration and higher intensity compared to HIIT and MICT, further research is needed to determine its potential superior effects on weight loss. Therefore, this study aims to conduct experimental research on randomly selected individuals with overweight/obesity undergoing Tabata, HIIT, and MICT. The analysis will focus on substrate metabolism and energy expenditure data during the exercise and recovery phases of 10 and 20 minutes, comparing the fat oxidation rate, glucose oxidation rate, and energy expenditure rate of the three exercise modalities. The findings aim to provide a theoretical basis for the tailored development of training plans for overweight/obese individuals.
2 Subjects and methods
This study employed an experimental research design involving a cohort of randomly selected fifteen male university students with overweight/obesity. Initial assessments included maximal oxygen consumption tests to determine the power corresponding to 20% VO2max, 50% VO2max, and 80% VO2max intensities on a stationary bicycle. Subsequently, participants underwent random Tabata, HIIT and MICT exercise tests, each separated by a 7-day interval. Continuous measurements of gas metabolism parameters were conducted during exercise and the 10-minute and 20-minute recovery periods. This data was then utilized to calculate the fat oxidation rate, glucose oxidation rate, and energy expenditure rate for each of the three exercise modalities.
2.1 Participants
Fifteen male university students with overweight/obesity were randomly selected for this study (mean ± standard deviation, age: 22.85 ± 2.40 years, height: 177 ± 5.64 cm, weight: 86.40 ± 4.80 kg, Body Fat Percentage(BFP): 24.82 ± 1.43%, BMI: 27.12 ± 1.48 kg/m2, Fat mass:21.33 ± 2.41 kg, lean mass:64.67 ± 3.07 kg). All participants had a BMI greater than 24 kg/m2, according to Chinese adult BMI standards (overweight BMI > 24 kg/m2, obesity BMI > 28 kg/m2), indicating good physical condition. They reported no regular exercise habits, were non-smokers, and had no history of diabetes or metabolic disorders. Exclusion criteria included smokers, individuals with a history of diabetes or metabolic diseases. In the week preceding the experiment, participants refrained from intense physical activity, smoking, alcohol consumption, and the intake of caffeinated beverages or medications for 48 hours prior to testing. Dietary habits were recorded for the week before the experiment, focusing on normal daily meals with avoidance of high-fat and high-sugar foods. During the experiment, participants were instructed to maintain the same dietary structure and quantity as the previous week to eliminate dietary interference. Adequate sleep was encouraged, with participants advised against staying up late or having irregular sleep schedules to minimize potential impacts on the experiment. Before testing, participants were fully informed about the purpose and procedures of the study and provided informed consent.
2.2 Experimental control
The indoor temperature was maintained within the range of 24–26°C, with a relative humidity level between 40% and 60%. All participants underwent exercise testing precisely 2 hours postprandial, commencing at 14:30 in the afternoon. The Tabata exercise test commenced 48 hours after the conclusion of the maximal oxygen consumption test. Subsequently, the HIIT exercise test and the MICT exercise test were conducted at 7 days intervals.
2.3 Preliminary test
Participants in this study underwent an initial maximal oxygen consumption test. The VO2max test protocol commenced with a load intensity of 20 watts, incrementing by 15 watts every two minutes. Participants were instructed to maintain a pedaling speed of 60 revolutions per minute on the stationary bicycle. The power output corresponding to 20% VO2max, 50% VO2max, and 80% VO2max intensities was determined by correlating VO2 levels with the corresponding power output (W) achieved during the test.
2.4 Experimental procedures
Participants were sequentially and randomly assigned to undergo the Tabata test, HIIT test, and MICT test, with a 7-day interval between each session. On the day of testing, participants arrived at the laboratory at 14:30, where they engaged in a 20-minute seated rest period followed by a warm-up exercise. Subsequently, they commenced the exercise test after donning the cardiopulmonary testing apparatus. Post-exercise, participants continued wearing the respiratory mask, with continuous monitoring of gas metabolism parameters during the 10-minute and 20-minute recovery periods.
The Tabata test comprised four exercises (squat, jumping jacks, high knees, burpees), each lasting 20 seconds, repeated for 2 sets. After each 20-second exercise, there was a 10-second rest, resulting in a total duration of 4 minutes.
The HIIT test involved cycling at 80% VO2max intensity for 3 minutes, followed by cycling at 20% VO2max intensity for 2 minutes, alternating for a total of 4 sets. Participants maintained a pedal speed of 60 revolutions per minute, and the entire session lasted 20 minutes.
For the MICT test, participants cycled at a load corresponding to 50% VO2max intensity, maintaining a pedal speed of 60 revolutions per minute, for a continuous duration of 30 minutes. Refer to (Figure 1) for the experimental protocol.
3 Measurements
3.1 Physiological indices measurement
BMI was determined as weight/height squared (kg/m2). The body fat rate was measured with a body composition testerVO2 (L/min) and VCO2 (L/min) were continuously measured with the exercise cardiopulmonary tester (cosmedk5,Italy) during exercise and recovery, and VO2max(L/min) was completed with the instrument.It is calculated that (18, 19): ① Oxidation rate of sugar (g/min) =4.585 × VCO2 (L/min) -3.2256 × VO2 (L/min) ② Oxidation amount of sugar (g) = Oxidation rate of sugar × Time (min) ③ Fat oxidation rate (g/min) =1.695 × VO2 (L/min) -1.701 × VCO2 (L/min) ④ Oxidation amount of fat (g) = Oxidation rate of fat × Time (min) ⑤ Energy consumption rate (kcal/min) =3.716 × VO2 (L/min) +1.332 × VCO2 (L/min) ⑥ Energy consumption (kcal) =[3.716 × VO2 (L/min) +1.332 × VCO2 (L/min)] × Time (min), HR(Garmin)was recorded every 1 min throughout the test period. Rating of perceived exertion (RPE) was recorded from the beginning to the end of the exercise using a Borg scale (20).
3.2 Statistical analysis
G*power3.1.9.7, with an effect size of 0.25, an alpha value of 0.05, and a power of 0.80, was used to estimate the sample size.Based on the calculation, the minimum sample size necessary to satisfy the test requirements was 8. All data were statistically analyzed using SPSS 26.0 software (Chicago, IL, USA) and expressed as mean ± standard deviation. The Shapiro-Wilk test showed that the data were normally distributed. One way repeated measures ANOVA was used to analyze the fat oxidation rate, sugar consumption rate, energy consumption rate, fat oxidation amount, sugar oxidation amount, and energy consumption in Tabata, HIIT, and MICT groups during exercise, 10, and 20 minutes of recovery. Corrected by the Greenhouse–Geisser method when the sphericity test was violated. If significant differences were found, Bonferroni post hoc test was used to find pairwise differences. Effect sizes were reported as partial eta squared (pη2) for ANOVA. (pη2the effect of small, medium and large were 0.04, 0.25 and 0.64.) (21) Significant differences were considered at p<0.05.
4 Results
Energy consumption and substrate metabolism are important indicators for evaluating exercise effectiveness. Glucose and fat are important indicators of substrate metabolism, and this study mainly monitors fat oxidation rate. Glucose oxidation rate, glucose oxidation. For energy consumption, the main monitoring is the energy expenditure rate. We monitor the energy consumption and substrate metabolism analysis data of three different exercise modes at three time points: exercise period, recovery period, exercise and the entire recovery process. The purpose is to provide theoretical basis for developing targeted training plans for overweight/obese populations from the perspectives of energy consumption and substrate metabolism.
4.1 During exercise
During the exercise phase, there was a statistically significant difference in the fat oxidation rate among the three exercise modes (F2,13 = 7.215, p<0.01, pη2 = 0.526). The fat oxidation rate in the Tabata group (0.43 ± 0.09g/min) did not significantly differ from the HIIT group (0.34 ± 0.10g/min) or the MICT group (0.46 ± 0.08g/min) (p>0.05). However, the fat oxidation rate in the MICT group (0.46 ± 0.08g/min) was significantly higher than that in the HIIT group (0.34 ± 0.10g/min) (p<0.05) (Figure 2A).
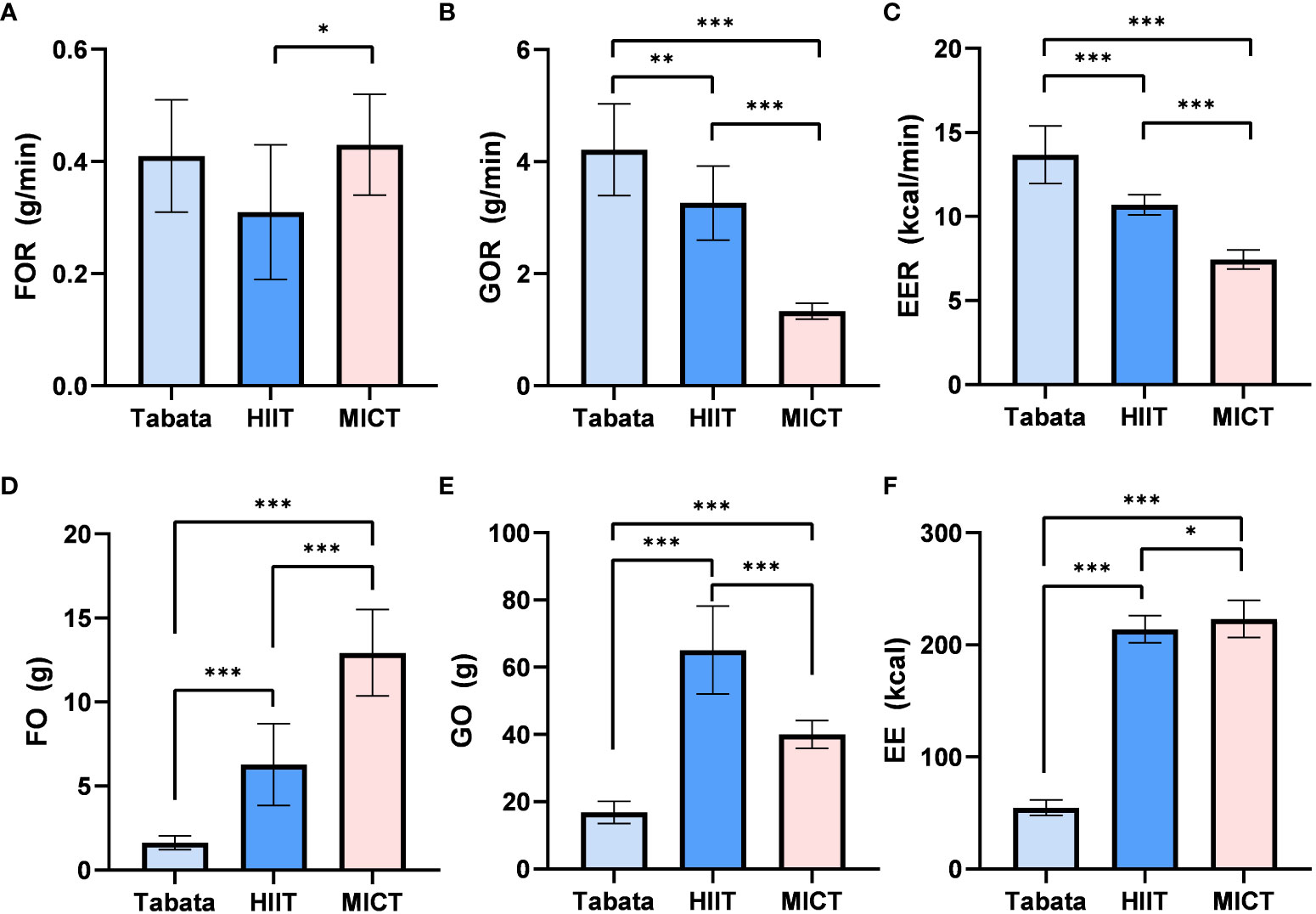
Figure 2 FO, Fat Oxidation; GO, Glucose Oxidation; EE, Energy Expenditure; Fat oxidation rate,Glucose Oxidation rate and Energy Expenditure rate of Tabata, HIIT and MICT during exercise [(A–C) n=15] Fat oxidation, Glucose Oxidation and Energy Expenditure of Tabata, HIIT and MICT during exerciese [(D–F) n=15] Data are expressed as mean ± SD. *p < 0.05, **p < 0.01, ***p<0.001.
There was a statistically significant difference in the glucose oxidation rate among the three exercise modes during the exercise phase (F2,28 = 169.501, p<0.001, pη2 = 0.924). The glucose oxidation rate in the Tabata group (4.31 ± 0.64g/min) was significantly higher than the HIIT group (3.28 ± 0.58g/min) (p<0.01) and the MICT group (1.30 ± 0.12g/min) (p<0.001). Additionally, the glucose oxidation rate in the HIIT group (3.28 ± 0.58g/min) was significantly higher than that in the MICT group (1.30 ± 0.12g/min) (p<0.001) (Figure 2B).
The energy expenditure rate during the exercise phase showed a statistically significant difference among the three exercise modes (F2,13 = 482.802, p<0.001, pη2 = 0.987). The energy expenditure rate in the Tabata group (13.67 ± 1.39kcal/min) was significantly higher than the HIIT group (10.64 ± 0.49kcal/min) and the MICT group (7.50 ± 0.46kcal/min) (p<0.001). Moreover, the energy expenditure rate in the HIIT group (10.64 ± 0.49kcal/min) was significantly higher than that in the MICT group (7.50 ± 0.46kcal/min) (p<0.001) (Figure 2C).
The fat oxidation amount during the exercise phase exhibited a statistically significant difference among the three exercise modes (F2,13 = 68.317, p<0.001, pη2 = 0.894). The fat oxidation amount in the Tabata group (1.65 ± 0.39g) was significantly lower than the HIIT group (6.30 ± 2.45g) (p<0.001) and the MICT group (12.87 ± 2.54g) (p<0.001). Additionally, the fat oxidation amount in the HIIT group was also significantly lower than that in the MICT group (p<0.001) (Figure 2D).
The glucose oxidation amount during the exercise phase demonstrated a statistically significant difference among the three exercise modes (F2,13 = 148.374, p<0.001, pη2 = 0.942). The glucose oxidation amount in the Tabata group (16.79 ± 3.31g) was significantly lower than the HIIT group (66.08 ± 13.58g) (p<0.001) and the MICT group (40.31 ± 3.79g) (p<0.001). Moreover, the glucose oxidation amount in the HIIT group was significantly higher than that in the MICT group (p<0.001) (Figure 2E).
The energy expenditure during the exercise phase displayed a statistically significant difference among the three exercise modes (F2,13 = 1572.458, p<0.001, pη2 = 0.989). The energy expenditure in the Tabata group (55.07 ± 6.94kcal) was significantly lower than the HIIT group (215.02 ± 11.89kcal) (p<0.001) and the MICT group (225.21 ± 17.28kcal) (p<0.001). Additionally, the energy expenditure in the HIIT group was significantly lower than that in the MICT group (p<0.05) (Figure 2F).
4.2 Recovery periods
4.2.1 10-minute recovery periods
During the 10-minute recovery period, there was a statistically significant difference in fat oxidation rates among the three exercise modes (F2,13 = 11.210, p<0.01, pη2 = 0.633). The fat oxidation rate in the Tabata group (0.09 ± 0.02g/min) did not significantly differ from the HIIT group (0.13 ± 0.05g/min) or the MICT group (0.07 ± 0.02g/min) (p>0.05). However, the fat oxidation rate in the HIIT group (0.13 ± 0.05g/min) was significantly higher than that in the MICT group (0.07 ± 0.02g/min) (p<0.05) (Figure 3A).
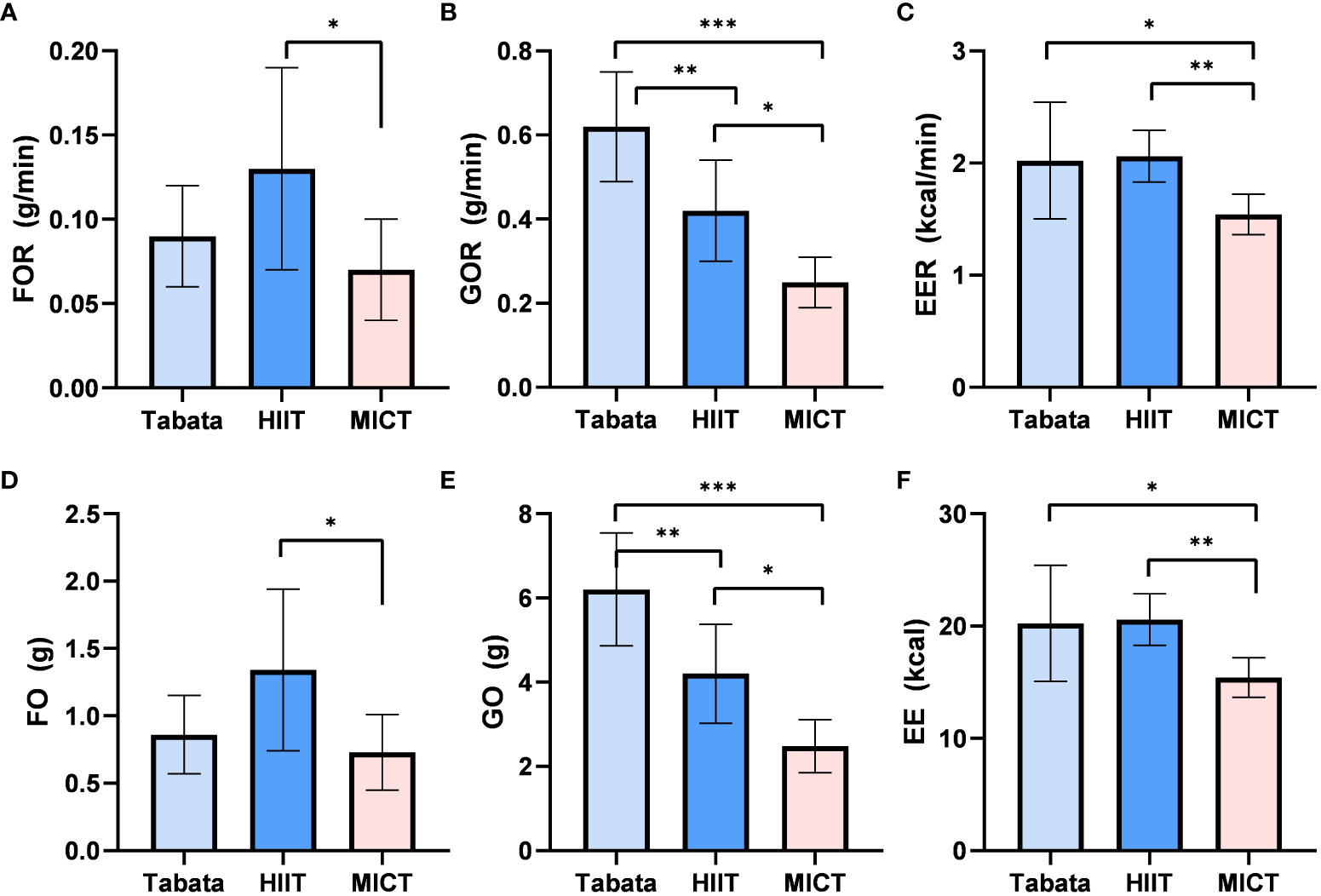
Figure 3 Fat oxidation rate, Glucose Oxidation rate and Energy Expenditure rate of Tabata, HIIT and MICT in 10 minutes of recovery period [(A–C) n=15] Fat oxidation,Glucose Oxidation and Energy Expenditure of Tabata, HIIT and MICT in 10minutes of recovery period [(D–F) n=15] Data are expressed as mean ± SD. *p < 0.05, **p < 0.01, ***p<0.001.
There was a statistically significant difference in glucose oxidation rates among the three exercise modes during the 10-minute recovery period (F2,28 = 74.885, p<0.001, pη2 = 0.842). The glucose oxidation rate in the Tabata group (0.63 ± 0.10g/min) was significantly higher than the HIIT group (0.42 ± 0.12g/min) (p<0.01) and the MICT group (0.25 ± 0.05g/min) (p<0.001). Additionally, the glucose oxidation rate in the HIIT group (0.42 ± 0.12g/min) was significantly higher than that in the MICT group (0.25 ± 0.05g/min) (p<0.05) (Figure 3B).
The energy expenditure rate during the 10-minute recovery period showed a statistically significant difference among the three exercise modes (F2,13 = 28.436, p<0.01, pη2 = 0.670). The energy expenditure rate in the Tabata group (2.03 ± 0.39kcal/min) was significantly higher than the MICT group (1.53 ± 0.14kcal/min) (p<0.05) and did not significantly differ from the HIIT group (2.10 ± 0.19kcal/min) (p>0.05). Moreover, the energy expenditure rate in the HIIT group (2.10 ± 0.19kcal/min) was significantly higher than that in the MICT group (1.53 ± 0.14kcal/min) (p<0.01) (Figure 3C).
The fat oxidation amount during the 10-minute recovery period exhibited a statistically significant difference among the three exercise modes (F2,13 = 11.210, p<0.01, pη2 = 0.633). The fat oxidation amount in the Tabata group (0.85 ± 0.30g) did not significantly differ from the HIIT group (1.32 ± 0.50g) or the MICT group (0.72 ± 0.30g), but the HIIT group was significantly higher than the MICT group (p<0.05) (Figure 3D).
There was a statistically significant difference in glucose oxidation amounts among the three exercise modes during the 10-minute recovery period (F2,13 = 74.885, p<0.001, pη2 = 0.842). The glucose oxidation amount in the Tabata group (6.19 ± 1.30g) was significantly higher than the HIIT group (4.21 ± 1.21g) (p<0.01) and the MICT group (2.51 ± 0.53g) (p<0.001). Additionally, the HIIT group was significantly higher than the MICT group (p<0.05) (Figure 3E).
The energy expenditure during the 10-minute recovery period showed a statistically significant difference among the three exercise modes (F2,13 = 28.436, p<0.01, pη2 = 0.670). The energy expenditure in the Tabata group (20.31 ± 5.03kcal) did not significantly differ from the HIIT group (20.48 ± 2.22kcal) (p=0.83), but the Tabata group was significantly higher than the MICT group (16.21 ± 1.35kcal) (p<0.05). Moreover, the HIIT group was significantly higher than the MICT group (p<0.01) (Figure 3F).
4.2.2 20-minute recovery periods
During the 20-minute recovery period, there was a statistically significant difference in fat oxidation rates among the three exercise modes (F2,13 = 99.948, p<0.001, pη2 = 0.877). The fat oxidation rate in the Tabata group (0.29 ± 0.04g/min) was significantly higher than the HIIT group (0.17 ± 0.05g/min) (p<0.01) and the MICT group (0.10 ± 0.02g/min) (p<0.001). Additionally, the fat oxidation rate in the HIIT group (0.17 ± 0.05g/min) was significantly higher than that in the MICT group (0.10 ± 0.02g/min) (p<0.05) (Figure 4A).
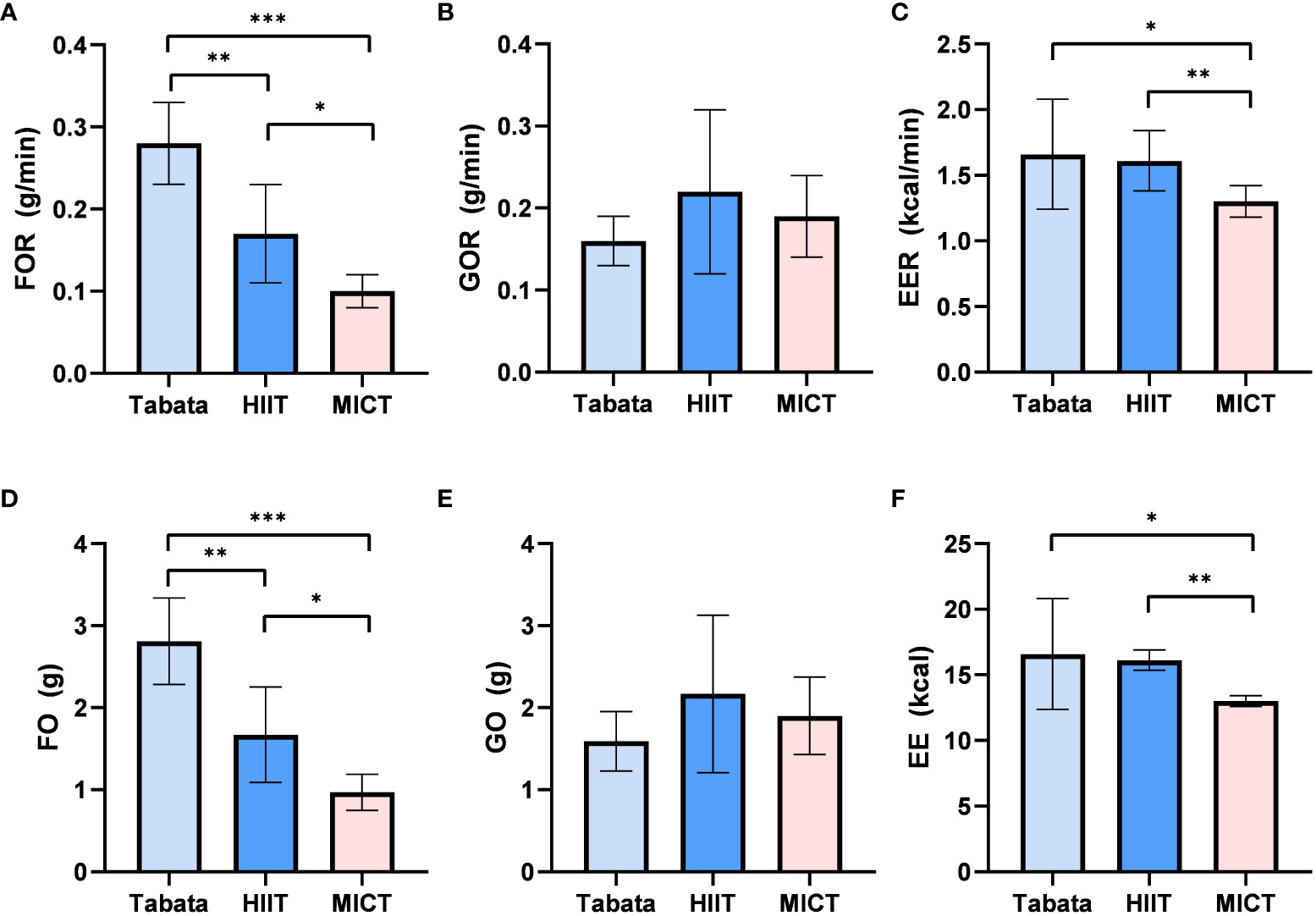
Figure 4 Fat oxidation rate, Glucose Oxidation rate and Energy Expenditure rate of Tabata, HIIT and MICT in 20 minutes of recovery period [(A–C) n=15] Fat oxidation,Glucose Oxidation and Energy Expenditure of Tabata, HIIT and MICT in 20 minutes of recovery period [(D–F n=15] Data are expressed as mean ± SD. *p < 0.05, **p < 0.01, ***p<0.001.
During the 20-minute recovery period, there was no significant difference in glucose oxidation rates among the three exercise modes (F2,28 = 3.624, p=0.083, pη2 = 0.652). The glucose oxidation rate in the Tabata group (0.17 ± 0.03g/min) did not significantly differ from the HIIT group (0.23 ± 0.09g/min) or the MICT group (0.19 ± 0.04g/min) (Figure 4B).
The energy expenditure rate during the 20-minute recovery period showed a statistically significant difference among the three exercise modes (F2,13 = 36.484, p<0.05, pη2 = 0.849). The energy expenditure rate in the Tabata group (1.67 ± 0.43kcal/min) was significantly higher than the MICT group (1.32 ± 0.10kcal/min) (p<0.05) and did not significantly differ from the HIIT group (1.60 ± 0.19kcal/min) (p>0.05). Moreover, the energy expenditure rate in the HIIT group (1.60 ± 0.19kcal/min) was significantly higher than that in the MICT group (1.32 ± 0.10kcal/min) (p<0.01) (Figure 4C).
The fat oxidation amount during the 20-minute recovery period exhibited a statistically significant difference among the three exercise modes (F2,13 = 99.948, p<0.001, pη2 = 0.877).The fat oxidation amount in the Tabata group (2.82 ± 0.48g) was significantly higher than the HIIT group (1.65 ± 0.54g) (p<0.01) and the MICT group (0.99 ± 0.22g) (p<0.001). Moreover, the HIIT group was significantly higher than the MICT group (p<0.05) (Figure 4D).
During the 20-minute recovery period, there was no significant difference in glucose oxidation amounts among the three exercise modes (F2,13 = 3.624, p=0.083, pη2 = 0.652). The glucose oxidation amount in the HIIT group (1.59 ± 0.35g) did not significantly differ from the Tabata group (2.16 ± 0.98g) or the MICT group (1.91 ± 0.47g) (Figure 4E).
The energy expenditure during the 20-minute recovery period showed a statistically significant difference among the three exercise modes (F2,13 = 36.484, p<0.05, pη2 = 0.849). The energy expenditure in the Tabata group (16.52 ± 4.20kcal) did not significantly differ from the HIIT group (16.09 ± 0.75kcal) (p>0.05) but was significantly higher than the MICT group (13.03 ± 0.41kcal) (p<0.05). Moreover, the HIIT group was significantly higher than the MICT group (p<0.01) (Figure 4F).
4.3 Throughout the exercise and recovery period
Throughout the exercise and recovery period, there was a statistically significant difference in fat oxidation rates (F2,28 = 23.416, p<0.001, pη2 = 0.626). The overall fat oxidation rate in the Tabata group (0.27 ± 0.03g/min) was significantly higher than the HIIT group (0.20 ± 0.04g/min) (p<0.05) and the MICT group (0.20 ± 0.03g/min) (p<0.001). There was no significant difference between the HIIT group and the MICT group (p=0.854) (Figure 5A).
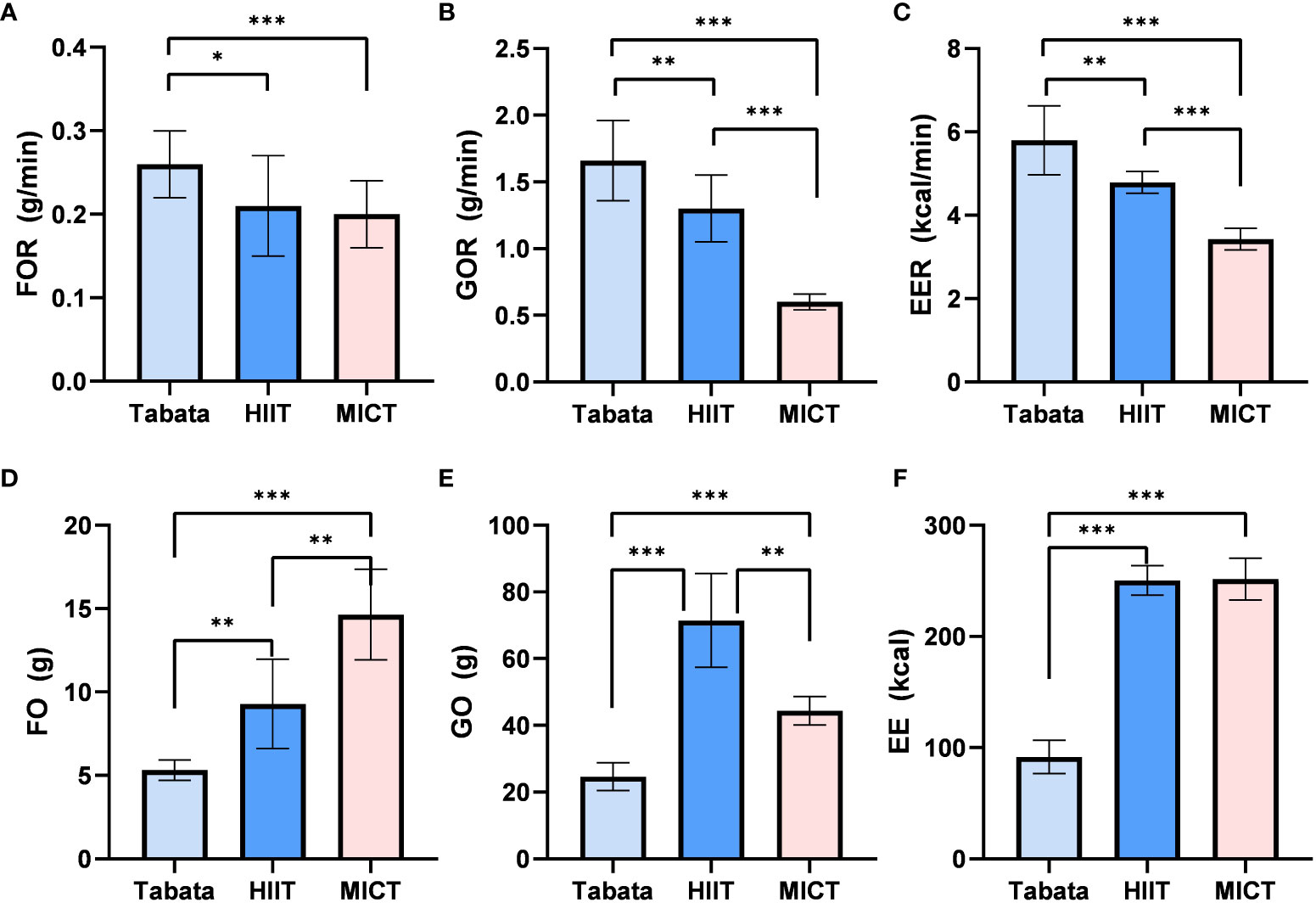
Figure 5 Fat oxidation rate, Glucose Oxidation rate and Energy Expenditure rate of Tabata, HIIT and MICT in the whole exercise and recovery period [(A–C) n=15] Fat oxidation,Glucose Oxidation and Energy Expenditure of Tabata, HIIT and MICT in the whole exercise and recovery period [(D–F) n=15] Data are expressed as mean ± SD. *p < 0.05, **p < 0.01, ***p<0.001.
The glucose oxidation rates throughout the exercise and recovery period showed a statistically significant difference (F2,13 = 48.023, p<0.001, pη2 = 0.901). The overall glucose oxidation rate in the Tabata group (1.67 ± 0.28g/min) was significantly higher than the HIIT group (1.31 ± 0.22g/min) (p<0.01) and the MICT group (0.61 ± 0.06g/min) (p<0.001). Additionally, the HIIT group had a significantly higher glucose oxidation rate than the MICT group (p<0.001) (Figure 5B).
Throughout the exercise and recovery period, there was a statistically significant difference in energy expenditure rates (F2,28 = 89.36, p<0.001, pη2 = 0.962). The overall energy expenditure rate in the Tabata group (5.76 ± 0.74kcal/min) was significantly higher than the HIIT group (4.81 ± 0.25kcal/min) (p<0.01) and the MICT group (3.45 ± 0.25kcal/min) (p<0.001). Furthermore, the HIIT group had a significantly higher energy expenditure rate than the MICT group (p<0.001) (Figure 5C).
Throughout the exercise and recovery period, there was a statistically significant difference in fat oxidation amounts (F2,13 = 34.782, p<0.001, pη2 = 0.762). The overall fat oxidation amount in the Tabata group (5.30 ± 0.61g) was significantly lower than the HIIT group (9.34 ± 2.66g) (p<0.01) and the MICT group (14.65 ± 2.70g) (p<0.001). Additionally, the HIIT group had a significantly lower fat oxidation amount than the MICT group (p<0.01) (Figure 5D).
Throughout the exercise and recovery period, there was a statistically significant difference in glucose oxidation amounts (F2,13 = 121.423, p<0.001, pη2 = 0.935). The overall glucose oxidation amount in the Tabata group (24.63 ± 4.20g) was significantly lower than the HIIT group (71.50 ± 14.17g) (p<0.001) and the MICT group (45.08 ± 4.32g) (p<0.001). However, the HIIT group had a significantly higher glucose oxidation amount than the MICT group (p<0.01) (Figure 5E).
Throughout the exercise and recovery period, there was a statistically significant difference in overall energy expenditure (F2,28 = 1306.546, p<0.001, pη2 = 0.987). The overall energy expenditure in the Tabata group (91.48 ± 14.91kcal) was significantly lower than the HIIT group (248.32 ± 13.32kcal) (p<0.001) and the MICT group (252.07 ± 19.02kcal) (p<0.001). However, there was no significant difference in energy expenditure between the HIIT group and the MICT group (Figure 5F).
4.4 Heart rate and rating of perceived exertion
During the exercise period, there was a statistically significant difference in heart rate among the three exercise modes (F2,28 = 322.533, p<0.001). In the 10-minute recovery period, there was a statistically significant difference in heart rate among the three exercise modes (F2,28 = 102.743, p<0.001). Similarly, during the 20-minute recovery period, there was a statistically significant difference in heart rate among the three exercise modes (F2,28 = 86.672, p<0.001) (Table 1).
Throughout the exercise period, there was a statistically significant difference RPE among the three exercise modes (F2,28 = 147.83, p<0.001). In the 10-minute recovery period, there was a statistically significant difference in RPE among the three exercise modes (F2,28 = 84.721, p<0.001). Additionally, during the 20-minute recovery period, there was a statistically significant difference in RPE among the three exercise modes (F2,28 = 93.472, p<0.001) (Table 2).
5 Discussion
5.1 Analysis of substrate metabolism and energy expenditure characteristics during Tabata, HIIT, and MICT
The findings of this study reveal that, during exercise, the MICT group exhibits a significantly higher fat oxidation quantity compared to the Tabata and HIIT groups. However, the fat oxidation rate of the MICT group only surpasses that of the HIIT group, and there is no significant difference in fat oxidation rates between the Tabata and MICT groups. The study suggests (22) that the duration of exercise significantly influences the body’s ratio of glucose to lipid energy provisioning. The possible explanation lies in the relatively stable state of the body during MICT exercise at 50% VO2max intensity. This steadiness allows the body’s oxygen intake to precisely match the required oxygen quantity, gradually promoting energy conservation primarily through the utilization of fat. It is speculated that this phenomenon signifies the initiation of the body’s energy-saving function. The reasons behind this may be linked to the gradual depletion of muscle glycogen, reduced glucose stores, leading to decreased levels of adrenaline and insulin, consequently enhancing the rate of fat consumption.
In terms of sugar metabolism, the HIIT group demonstrates a significantly higher glucose oxidation quantity than the Tabata and MICT groups. However, the sugar consumption rate in Tabata is notably higher than in the other two groups. Exercise intensity influences the efficiency of sugar oxidation during exercise, and as the intensity increases, so does the efficiency of sugar oxidation metabolism (23). High-intensity exercise results in rapid glycogen consumption. For instance, in 1 minute of exercise at 150% VO2max intensity, muscle glycogen levels can decrease by 20% (24). Conversely, a set of high-intensity intermittent exercises can reduce glycogen levels to 28%-37% of pre-exercise levels (24, 25). The energy consumption during exercise is significantly higher in the MICT group compared to the Tabata and HIIT groups. However, Tabata’s energy consumption rate is also significantly higher than the other two groups. Moderate-intensity continuous exercise, due to its lower intensity, is easier for subjects to sustain, resulting in higher energy consumption and fat oxidation rates. This proves beneficial in preventing cardiovascular diseases, obesity, diabetes, and reducing overall mortality rates (26–29).
At the same time, body composition has a significant impact on energy expenditure and substrate metabolism. Research has found that body weight has a significant impact on sugar and total energy expenditure, but has no significant effect on the proportion of fat and substrate metabolism energy supply. The reason may be that different body fat levels and body weight have a certain impact on exercise ability, with the greatest impact on defatted weight. Defatted weight is highly correlated with the aerobic and anaerobic capacity of the human body, and there is a significant difference in defatted weight between weights. Therefore, when sugar is needed for rapid oxidation energy supply, there is a significant difference in weight between weights. Gao Binghong’s research found that there is a high correlation between the defatted weight, muscle weight, and anaerobic capacity of judo athletes, with a correlation coefficient between 0.66 and 0.9. It can be considered that differences in body fat levels seriously affect substrate metabolism and energy consumption.
5.2 Substrate metabolism and energy expenditure analysis during the recovery period of Tabata, HIIT, and MICT
At the 10-minute recovery mark, the HIIT group exhibits a significantly higher fat oxidation rate and quantity than the MICT group, with no significant difference compared to the Tabata group. Regarding sugar metabolism, the Tabata group shows a significantly higher sugar oxidation rate and quantity than both the HIIT and MICT groups. The MICT group demonstrates significantly lower energy consumption and energy consumption rates compared to the other two groups. At the 20-minute recovery stage, the Tabata group’s fat oxidation rate and quantity are significantly higher than the other two groups. In terms of sugar metabolism, there are no significant differences among the three groups. Energy consumption induced by exercise includes not only during but also post-exercise excess post-exercise energy expenditure (EPEE). This study’s results indicate that while energy consumption during Tabata and HIIT exercises is significantly lower than MICT, the energy consumption during the recovery period is significantly higher than MICT. This aligns with previous research findings (30–32). With increasing exercise intensity, post-exercise sugar oxidation rates and quantities gradually decrease, while fat oxidation rates, quantities, and overall energy consumption increase. This is primarily due to the maintenance of high levels of endocrine hormones, body temperature, and pulmonary ventilation after high-intensity exercise. Oxygen reserves need rapid replenishment, the ATP-CP system requires quick supplementation, and lactate needs swift clearance. At this stage, the primary energy supply shifts from anaerobic to aerobic, with the main substance for aerobic energy supply transitioning from sugar to fat. post-exercise blood levels of free fatty acids significantly increase. Research demonstrates a correlation between the percentage of fat energy supply after exercise and exercise intensity and duration. Exercise intensity affects the amount of excess post-exercise oxygen consumption, while exercise duration extends the time of excess post-exercise oxygen consumption (33, 34). There is currently limited research on the recovery to resting state after high-intensity intermittent exercise, and changes in substrate levels can reflect changes in fat metabolism. Studies show that completing high-intensity intermittent exercise before eating results in a significantly greater decrease in total cholesterol and very low-density lipoprotein levels compared to the MICT group (35–37), demonstrating the superior effect of high-intensity intermittent exercise in enhancing post-exercise fat metabolism. This study confirms that Tabata significantly enhances post-exercise fat metabolism during the recovery period, surpassing both HIIT and MICT.
The fat mass of male college students with overweight/obesity is relatively higher than that of ordinary college students. Due to the higher oxidation and energy supply ratio of fat after high-intensity interval exercise, and the increase in exercise intensity, male college students with overweight/obesity can use high-intensity interval exercise as a supplement to play the role of excessive oxygen consumption after exercise.
5.3 Substrate metabolism and energy expenditure characteristics of Tabata, HIIT, and MICT during the entire exercise and recovery process
The results of this study indicate that throughout the exercise and recovery period, the fat oxidation rate of the Tabata group is significantly higher than that of the HIIT and MICT groups, with no significant difference between the HIIT and MICT groups. However, the fat oxidation quantity of the MICT group is significantly higher than the other two groups during the entire process. This is attributed to the short duration of Tabata exercises, lasting only 4 minutes. Considering that Tabata is 16 minutes shorter than HIIT and 26 minutes shorter than MICT, there is also a corresponding resting fat oxidation during this period, and the fat oxidation rate of Tabata is higher than the other two groups. Therefore, in reality, the difference in fat oxidation quantity of the Tabata group should be less. Combining the entire exercise and recovery period, it is found that, in terms of fat burning effectiveness, Tabata outperforms the MICT group, and the MICT group surpasses the HIIT group. In terms of overall sugar metabolism during the exercise and recovery period, both Tabata and HIIT show significantly higher sugar oxidation rates than MICT. Although the MICT exercise duration is 10 minutes longer than HIIT, the sugar oxidation quantity of HIIT is still significantly higher than MICT. The conversion of energy supply from anaerobic to aerobic in HIIT, characterized by the continuous repetition of cycles, may lead to a high dependence on sugar oxidation energy supply and a high proportion of aerobic oxidation energy of glycogen in HIIT. The changes in substrate metabolism during Tabata exercises and the recovery period may be related to changes in the quantity and activity of skeletal muscle fat metabolism-related enzymes induced by HIIT (38, 39), enhancing fat metabolism efficiency. Additionally, HIIT can rapidly deplete glycogen in a short period (40). The post-exercise glycogen compensation mechanism may induce a tendency for glycogen to be resynthesized after exercise and fat metabolism to be characterized by decomposition, oxidation, and energy supply. This study also found that, although the exercise duration of HIIT is shorter than MICT, the total energy consumption during the “exercise + recovery period” induced by exercise is not significantly different between HIIT and MICT. Although the energy consumption of the Tabata group is significantly lower than the other two groups, this is because of the shorter exercise duration compared to the other two groups. However, the energy consumption rate is still significantly higher than the HIIT and MICT groups. The direct reason for this phenomenon may be that Tabata and HIIT have a deeper impact on EPEE (energy consumption rates at 10 and 20 minutes during recovery are significantly higher than MICT). HIIT achieves the total energy consumption of a longer MICT exercise duration with less exercise time (41, 42), and Tabata has a shorter time compared to HIIT and MICT, with higher fat oxidation rates and energy consumption rates. It may be more suitable for individuals who don’t have time for long-duration low-intensity aerobic exercise.
6 Conclusion
This study found that male college students with overweight/obesity in the Tabata group had lower levels of fat oxidation, sugar oxidation, and energy expenditure throughout the exercise and recovery periods compared to the HIIT and MICT groups. However, during the entire process, the rates of fat oxidation, sugar oxidation, and energy consumption were significantly higher in the HIIT and MICT groups, and Tabata exercise time was significantly shorter than the other two groups, demonstrating a certain “time efficiency” advantage. Tabata can be used as an efficient short-term weight loss exercise program for male college students with overweight/obesity.
Data availability statement
The raw data supporting the conclusions of this article will be made available by the authors, without undue reservation.
Ethics statement
The studies involving humans were approved by ethical review of the Human Ethics Committee of Guangxi Normal University. Ethical review number: 20231129001. The studies were conducted in accordance with the local legislation and institutional requirements. The participants provided their written informed consent to participate in this study.
Author contributions
YBW: Writing – original draft. MCF: Writing – review & editing. LC: Writing – review & editing. YFW: Writing – review & editing. XDP: Writing – review & editing. CFL: Writing – review & editing. BYH: Writing – review & editing. LHW: Writing – review & editing.
Funding
The author(s) declare that no financial support was received for the research, authorship, and/or publication of this article.
Acknowledgments
The authors thank all the volunteers for their enthusiastic participation in this study.
Conflict of interest
The authors declare that the research was conducted in the absence of any commercial or financial relationships that could be construed as a potential conflict of interest.
Publisher’s note
All claims expressed in this article are solely those of the authors and do not necessarily represent those of their affiliated organizations, or those of the publisher, the editors and the reviewers. Any product that may be evaluated in this article, or claim that may be made by its manufacturer, is not guaranteed or endorsed by the publisher.
References
1. Hassan B, Farooqui SI, Khan MU, Farhad A, Adnan QUA. Effects of mode and duration of exercises on fat mass of obese population. J Coll Physicians Surg Pak. (2020) 30(4):412–6. doi: 10.29271/jcpsp.2020.04.412
2. Elagizi A, Kachur S, Carbone S, Lavie CJ, Blair SN. A review of obesity, physical activity, and cardiovascular disease. Curr Obes Rep. (2020) 9(4):571–81. doi: 10.1007/s13679-020-00403-z
3. Valkenborghs SR, van Vliet P, Nilsson M, Zalewska K, Visser MM, Erickson KI, et al. Aerobic exercise and consecutive task-specific training (AExaCTT) for upper limb recovery after stroke: A randomized controlled pilot study. Physiother Res Int. (2019) 24(3):1775. doi: 10.1002/pri.1775
4. Billat LV. Interval training for performance: A scientific and empirical practice. special recommendations for middle- and long-distance running. Part I: Aerobic Interval Training Sports Med. (2001) 31:13–31. doi: 10.2165/00007256-200131010-00002
5. Cadenas-Sanchez C, Fernández-Rodríguez R, Martínez-Vizcaíno V, de Los Reyes González N, Lavie CJ, Galán-Mercant A, et al. A systematic review and cluster analysis approach of 103 studies of high-intensity interval training on cardiorespiratory fitness. Eur J Prev Cardiol. (2023) 21:309. doi: 10.1093/eurjpc/zwad309
6. Yin M, Chen Z, Nassis GP, Liu H, Li H, Deng J, et al. Chronic high-intensity interval training and moderate-intensity continuous training are both effective in increasing maximum fat oxidation during exercise in overweight and obese adults: A meta-analysis. J Exerc Sci Fit. (2023) 21(4):354–65. doi: 10.1016/j.jesf.2023.08.001
7. Gilson ND, Andersson D, Papinczak ZE, Rutherford Z, John J, Coombes JS, et al. High intensity and sprint interval training, and work-related cognitive function in adults: A systematic review. Scand J Med Sci Sports. (2023) 33(6):814–33. doi: 10.1111/sms.14349
8. Martins C, Kazakova I, Ludviksen M, Mehus I, Wisloff U, Kulseng B, et al. High-intensity interval training and isocaloric moderate-intensity continuous training result in similar improvements in body domposition and fitness in obese individuals. Int J Sport Nutr Exerc Metab. (2016) 26(3):197–204. doi: 10.1123/ijsnem.2015-0078
9. Keating SE, Johnson NA, Mielke GI, Coombes JS. A systematic review and meta-analysis of interval training versus moderate-intensity continuous training on body adiposity. Obes Rev. (2017) 18(8):943–64. doi: 10.1111/obr.12536
10. Lee KJ, Noh B, An KO. Impact of synchronous online physical education classes using tabata training on adolescents during COVID-19: a randomized controlled study. Int J Environ Res Public Health. (2021) 18(19):10305. doi: 10.3390/ijerph181910305
11. Tabata I. Tabata training: one of the most energetically effective high-intensity intermittent training methods. J Physiol Sci. (2019) 69(4):559–72. doi: 10.1007/s12576-019-00676-7
12. Trapp EG, Chisholm DJ, Freund J, Boutcher SH. The effects of high-intensity intermittent exercise training on fat loss and fasting insulin levels of young women. Int J Obes (Lond). (2008) 32(4):684–91. doi: 10.1038/sj.ijo.0803781
13. Ciolac EG, Bocchi EA, Bortolotto LA, Carvalho VO, Greve JM, Guimarães GV. Effects of high-intensity aerobic interval training vs. moderate exercise on hemodynamic, metabolic and neuro-humoral abnormalities of young normotensive women at high familial risk for hypertension. Hypertens Res. (2010) 33(8):836–43. doi: 10.1038/hr.2010.72
14. Nybo L, Sundstrup E, Jakobsen MD, Mohr M, Hornstrup T, Simonsen L, et al. High-intensity training versus traditional exercise interventions for promoting health. Med Sci Sports Exerc. (2010) 42(10):1951–8. doi: 10.1249/MSS.0b013e3181d99203
15. Kolnes KJ, Petersen MH, Lien-Iversen T, Højlund K, Jensen J. Effect of exercise training on fat loss-energetic perspectives and the role of improved adipose tissue function and body fat distribution. Front Physiol. (2021) 24:737709(12). doi: 10.3389/fphys.2021.737709
16. Keating SE, Johnson NA, Mielke GI, Coombes JS. A systematic review and meta-analysis of interval training versus moderate-intensity continuous training on body adiposity. Obes Rev. (2017) 18(8):943–64. doi: 10.1111/obr.12536
17. Guo Z, Li M, Cai J, Gong W, Liu Y, Liu Z. Effect of high-intensity interval training vs. moderate-intensity continuous training on fat loss and cardiorespiratory fitness in the young and middle-aged a systematic review and meta-analysis. Int J Environ Res Public Health. (2023) 20(6):4741. doi: 10.3390/ijerph20064741
18. Trombold JR, Christmas KM, Machin DR, Kim IY, Coyle EF. Acute high-intensity endurance exercise is more effective than moderate-intensity exercise for attenuation of postprandial triglyceride elevation. J Appl Physiol. (1985) 114(6):792–800. doi: 10.1152/japplphysiol.01028.2012
19. Frayn KN. Calculation of substrate oxidation rates in vivo from gaseous exchange. J Appl Physiol. (1983) 55:628–34. doi: 10.1152/jappl.1983.55.2.628
20. Borg GA. Psychophysical bases of perceived exertion. Med Sci Sports Exerc. (1982) 14:377–81. doi: 10.1249/00005768-198205000-00012
21. Ferguson CJ. An effect size primer: A guide for clinicians and researchers. Prof Psychology: Res Pract. (2009) 40:532–8. doi: 10.1037/a0015808
22. Ronsen O, Haug E, Pedersen BK, Bahr R. Increased neuroendocrine response to a repeated bout of endurance exercise. Med Sci Sports Exerc. (2001) 33(4):568–75. doi: 10.1097/00005768-200104000-00010
23. Brun JF, Myzia J, Varlet-Marie E, Raynaud de Mauverger E, Mercier J. Beyond the calorie paradigm: taking into account in practice the balance of fat and carbohydrate oxidation during exercise? Nutrients. (2022) 14(8):1605. doi: 10.3390/nu14081605
24. Noakes TD, Prins PJ, Volek JS, D'Agostino DP, Koutnik AP. Low carbohydrate high fat ketogenic diets on the exercise crossover point and glucose homeostasis. Front Physiol. (2023) 14:1150265. doi: 10.3389/fphys.2023.1150265
25. Prins PJ, Noakes TD, Buga A, D'Agostino DP, Volek JS, Buxton JD, et al. Low and high carbohydrate isocaloric diets on performance, fat oxidation, glucose and cardiometabolic health in middle age males. Front Nutr. (2023) 10:1084021. doi: 10.3389/fnut.2023.1084021
26. Wewege MA, Thom JM, Rye KA, Parmenter BJ. Aerobic, resistance or combined training: a systematic review and meta-analysis of exercise to reduce cardiovascular risk in adults with metabolic syndrome. Atherosclerosis. (2018) 274:162–71. doi: 10.1016/j.atherosclerosis.2018.05.002
27. Chipkin SR, Klugh SA, Chasan-Taber L. Exercise and diabetes. Cardiol Clin. (2001) 19(3):489–505. doi: 10.1016/s0733-8651(05)70231-9
28. Zanuso S, Jimenez A, Pugliese G, Corigliano G, Balducci S. Exercise for the management of type 2 diabetes: a review of the evidence. Acta Diabetol. (2010) 47(1):15–22. doi: 10.1007/s00592-009-0126-3
29. Yang Z, CA S, Mao C, Tang J, Farmer AJ. Resistance exercise versus aerobic exercise for type 2 diabetes: a systematic review and meta-analysis. Sports Med. (2014) 44(4):487–99. doi: 10.1007/s40279-013-0128-8
30. Lu Y, Wiltshire HD, Baker JS, Wang Q, Ying S. The effect of tabata-style functional high-intensity interval training on cardiometabolic health and physical activity in female university students. Front Physiol. (2023) 14:1095315. doi: 10.3389/fphys.2023.1095315
31. Schaun GZ, Alberton CL, Ribeiro DO, Pinto SS. Acute effects of high-intensity interval training and moderate-intensity continuous training sessions on cardiorespiratory parameters in healthy young men. Eur J Appl Physiol. (2017) 117(7):1437–44. doi: 10.1007/s00421-017-3636-7
32. Matsuo T, Ohkawara K, Seino S, Shimojo N, Yamada S, Ohshima H, et al. An exercise protocol designed to control energy expenditure for long-term space missions. Aviat Space Environ Med. (2012) 83(8):783–9. doi: 10.3357/asem.3298.2012
33. Moon J, Oh M, Kim S, Lee K, Lee J, Song Y, et al. Intelligent estimation of exercise induced energy expenditure including excess post-exercise oxygen consumption (EPOC) with different exercise intensity. Sensors (Basel). (2023) 23(22):9235. doi: 10.3390/s23229235
34. McCarthy SF, Jarosz C, Ferguson EJ, Kenno KA, Hazell TJ. Intense interval exercise induces greater changes in post-exercise metabolism compared to submaximal exercise in middle-aged adults. Eur J Appl Physiol. (2023) 11:1–6. doi: 10.1007/s00421-023-05334-w
35. Maylor BD, Zakrzewski-Fruer JK, Orton CJ, Bailey DP. Beneficial postprandial lipaemic effects of interrupting sedentary time with high-intensity physical activity versus a continuous moderate-intensity physical activity bout: a randomised crossover trial. J Sci Med Sport. (2018) 21(12):1250–5. doi: 10.1016/j.jsams.2018.05.022
36. Gabriel B, Ratkevicius A, Gray P, Frenneaux MP, Gray SR. High-intensity exercise attenuates postprandial lipaemia and markers of oxidative stress. Clin Sci (Lond). (2012) 123(5):313–21. doi: 10.1042/CS20110600
37. Vitale K, Getzin A. Nutrition and supplement update for the endurance athlete: review and recommendations. Nutrients. (2019) 11(6):1289. doi: 10.3390/nu11061289
38. Bellou E, Magkos F, Kouka T, Bouchalaki E, Sklaveniti D, Maraki M, et al. Effect of high-intensity interval exercise on basal triglyceride metabolism in non-obese men. Appl Physiol Nutr Metab. (2013) 38(8):823–9. doi: 10.1139/apnm-2012-0468
39. Mengeste AM, Rustan AC, Lund J. Skeletal muscle energy metabolism in obesity. Obes (Silver Spring). (2021) 29(10):1582–95. doi: 10.1002/oby.23227
40. Frampton J, Murphy KG, Frost G, Chambers ES. Short-chain fatty acids as potential regulators of skeletal muscle metabolism and function. Nat Metab. (2020) 2(9):840–8. doi: 10.1038/s42255-020-0188-7
41. Panissa VLG, Fukuda DH, Staibano V, Marques M, Franchini E. Magnitude and duration of excess of post-exercise oxygen consumption between high-intensity interval and moderate-intensity continuous exercise: A systematic review. Obes Rev. (2021) 22(1):13099. doi: 10.1111/obr.13099
Keywords: exercise, obesity, fat oxidation, glucose oxidation, energy expenditure
Citation: Wang Y, Fan C, Cheng L, Wang Y, Peng D, Li F, Han Y and Wang H (2024) A comparative analysis of energy expenditure and substrate metabolism in male university students with overweight/obesity: Tabata vs HIIT and MICT. Front. Endocrinol. 15:1323093. doi: 10.3389/fendo.2024.1323093
Received: 17 October 2023; Accepted: 08 February 2024;
Published: 27 February 2024.
Edited by:
Xu Yan, Victoria University, AustraliaReviewed by:
Endre Károly Kristóf, University of Debrecen, HungaryRosaura Leis, University of Santiago de Compostela, Spain
Copyright © 2024 Wang, Fan, Cheng, Wang, Peng, Li, Han and Wang. This is an open-access article distributed under the terms of the Creative Commons Attribution License (CC BY). The use, distribution or reproduction in other forums is permitted, provided the original author(s) and the copyright owner(s) are credited and that the original publication in this journal is cited, in accordance with accepted academic practice. No use, distribution or reproduction is permitted which does not comply with these terms.
*Correspondence: Hongli Wang, d2hvbmdsaTIwMDRAZ3hudS5lZHUuY24=
†These authors have contributed equally to this work and share first authorship