- 1Department of Clinical Laboratory Sciences, Faculty of Allied Medical Sciences, Tehran University of Medical Sciences, Tehran, Iran
- 2Department of Clinical Biochemistry, Faculty of Medicine, Tehran University of Medical Sciences, Tehran, Iran
- 3Faculty of Allied Medical Sciences, Tehran University of Medical Sciences, Tehran, Iran
Background: PCSK9 plays a key role in raising LDL-C levels, which contributes to heart attacks (MI). However, studies show that about half of MI patients have normal LDL-C levels. This study aims to explore the link between PCSK9, heart function, and oxidative stress markers in MI patients.
Methods: This investigation was carried out at Tehran Heart Centre Hospital on healthy individuals (n=63) and patients (n=63) with MI who had a coronary artery block above 50% (CAB > 50%). Oxidative stress (OS) parameters, such as total antioxidant capacity (TAC), malondialdehyde (MDA), myeloperoxidase (MPO), superoxide dismutase (SOD), catalase (CAT), and glutathione peroxidase (GPx) activity, PCSK9, oxidized Low-density lipoprotein (ox-LDL), high-sensitivity cardiac troponin I (hs-cTnI), and hs-CRP are assessed. Indeed, biochemical parameters and EF% were measured.
Results: Higher EF% (>37.5%), TAC (>1.05 mmol Fe²+;/L), GPx (>16.48 mU/mL), CAT (>11.32 nmol/min/mL), and SOD (>297.16 U/mL) were linked to a lower risk of CAB > 50%. In contrast, higher MDA (>32.07 nmol/mL), MPO (>17.77 U/L), hs-CRP (>5.5 mg/L), and ox-LDL (>64.87 μg/L) were associated with a higher risk. There was no significant difference in PCSK9 and LDL-C levels between groups. EF% was positively linked to SOD but negatively related to MDA, MPO, ox-LDL, hs-cTnI, and hs-CRP. Ox-LDL correlated positively with MPO but negatively with TAC, CAT, and GPx. PCSK9 showed a positive relationship with MDA. The best markers for CAB > 50% diagnosis were ox-LDL (AUC = 83.22, cut-off > 63.35 μg/L), EF% (AUC = 82.35, cut-off < 46.25%), and hs-cTnI (AUC = 81.3, cut-off > 0.265 ng/mL).
Conclusion: While PCSK9’s role in MI through LDL-C is well known, its impact on inflammation and oxidative stress may also be important, even when LDL-C and PCSK9 levels are normal. Additionally, ox-LDL and EF% are better indicators of CAB > 50% than hs-cTnI.
Introduction
Coronary artery disease (CAD) is one of the most prevalent cardiovascular diseases (CVD) and is mainly caused by atherosclerosis (1). It is the primary cause of mortality in the majority of countries across the globe (17.8 million deaths annually) (2) and Iran (46% of all deaths) (3). By 2030, CAD is expected to become the most critical and prevalent issue that poses a threat to human health (4). Atherosclerosis builds up inflammatory cells, lipids accumulation, oxidative stress (OS), and extracellular matrix (ECM) in the inner lining of artery walls and encourages the growth of intimal smooth muscle cells (SMC). This can make arteries stiff and restrict blood flow, which can eventually cause heart failure, myocardial infarction (MI), and death in those affected (5, 6). Among the CADs, people who have coronary artery block above 50% (CAB > 50%) have a higher risk of mortality (7). Traditional cardiovascular risk factors do have a role in the pathogenesis of CAD, but other unique risk factors may be also at play (2). Various risk factors, including chronic inflammation, age, gender, genetics, obesity, diabetes mellitus (DM), high blood pressure, elevated blood lipids and disturbances of these metabolisms, smoking, OS, a sedentary lifestyle, and trace elements, influence it (8–11). While the main result is not completely clear and needs further study.
Previous studies have shown that PCSK9 may decrease circulating levels of IL-6 and other cytokines. However, it has also been reported that IL-6 inhibition can decrease circulating PCSK9 protein levels, potentially indicating a bidirectional relationship. Furthermore, the hypothesis that PCSK9 ameliorates low-grade inflammation at the local level within the vasculature without affecting systemic inflammation has been included. Additionally, PCSK9 is able to counteract the deleterious effects of IL-6 on the activation of inflammatory pathways, the instigation of autophagy, and the induction of oxidative stress. A positive correlation between PCSK9 and IL-1β, IL-6, and LC3B2/1 has been demonstrated, suggesting that higher PCSK9 expression correlates with elevated levels of these inflammatory and autophagy markers (12).
OS happens when the production of reactive nitrogen species (RNS) and reactive oxygen species (ROS) is out of balance and the body’s antioxidant defense system isn’t strong enough (13). Elevated levels of inflammatory cells, cytokines, and high-sensitivity C-reactive protein (hs-CRP) have been associated with inflammation, a key pathophysiological mechanism in the development of atherosclerosis (14). Chronic inflammation with OS influences vascular homeostasis, which includes endothelial and SMC growth, proliferation, and apoptosis (15). Superoxide dismutases (SOD) are metalloenzymes that metabolize superoxide to hydrogen peroxide and oxygen; they operate as the primary step in protection against ROS (16). Catalase (CAT) and glutathione peroxidase (GPx) break down hydrogen peroxide into water and oxygen. Myeloperoxidase (MPO) creates ROS intermediates, such as hypochlorous acid (HOCl), which is released by neutrophils and monocytes at sites of inflammation (11). Excessive ROS causes lipid damage and produces oxidized low-density lipoprotein (ox-LDL) in the sub-intimal region. Foam cells arise when scavenger receptors internalize ox-LDL within macrophages in the arteries. Ox-LDL also makes SMCs multiply and has chemotactic and cytotoxic effects on endothelial and monocyte cells, which speeds up the development of atherosclerosis (11, 17). Free radicals attack the polyunsaturated fatty acid during lipid peroxidation, forming malondialdehyde (MDA) and 4-Hydroxynonenal as a marker of the OS effect in lipids (18). The total antioxidant capacity (TAC) of plasma and body fluids signifies the cumulative activity of all antioxidant groups (19).
Hepatocytes synthesize the proprotein convertase subtilisin/kexin type 9 (PCSK9), which regulates cholesterol levels (20). PCSK9 triggers an autocrine action by binding to the low-density lipoprotein receptor (LDLR), causing the complex to undergo endocytosis and degradation in lysosomes within hepatocytes. This process leads to a decrease in LDLR at the cell membrane and an increase in low-density lipoprotein cholesterol (LDL-C) in blood (21, 22). Recent research has shown that PCSK9 not only regulates LDL-C concentration but also facilitates platelet activation and coagulation, and increase Inflammation, OS, and endothelial dysfunction (20, 23), that increase risk of MI incidence. In a prospective investigation of people with CAD, researchers found a positive relationship between PCSK9 concentration and the development and severity of CAD (24). In 2023, Sotlar and collaborators showed that the main way that PCSK9 affects CAD is through a rising concentration of atherogenic lipids (22). Furthermore, research employing individuals with CAD revealed a direct relationship between PCSK9 levels and the onset and intensity of CAD (25).
However, research from recent years has demonstrated that half of patients whose LDL-c and high-density lipoprotein-cholesterol (HDL-C) levels are normal have hazardous plaque buildup in their arteries (1, 26), therefore alternative risk of MI incidence is evaluated. Researchers looked into the link between PCSK9 and CVD by looking at how PCSK9 affects the metabolism lipids and levels of cholesterol and LDL-C (27), but inflammation and OS were almost ignored. PCSK9 is a practical treatment for the prevention and control of CVD. Indeed, high-sensitivity cardiac troponin I (hs-cTnI) serves as a biomarker and the gold standard for detecting myocardial injury and MI in patients, but this has less sensitivity for detecting cardiomyopathies (28). Based on studies, patients with the highest levels of PCSK9 in their plasma were 2.62 times more likely to experience recurrent vascular events compared to those with lower levels of PCSK9 in their plasma (29). These findings were supported by experiments where recombinant human PCSK9, when added to healthy human platelet-rich plasma, was able to significantly increase platelet aggregation and reduce the aggregation lag time after stimulation with a subthreshold concentration of epinephrine (0.3 and 0.6 mM) (30). Similarly, platelets incubated with PCSK9, at the concentration found in the circulation of 1 patients with atrial fibrillation (AF), increased platelet aggregation and the release of thromboxane B2 (TxB2) (31), which is a marker of in vivo platelet activation (23, 32).
These are the first studies of their kind to look at how PCSK9 is related to heart parameters like hs-cTnI, EF%, ox-LDL, and hs-CRP, as well as OS parameters like TAC, SOD, CAT, GPx, MPO, and MDA in people who have CAB > 50%. Furthermore, we evaluated cardiac-related parameters for the best sensitivity and specificity for the diagnosis of CAB > 50%.
Materials and methods
Subjects and data collection
This case-control study was carried out at Tehran Heart Centre Hospital (October-August 2023, n = 126). A cardiologist confirmed that 63 of them had confirmed CAB > 50% through coronary angiography. An additional 63 control subjects had normal coronary arteries. The power analysis was conducted with the following parameters: Significance Level (α): 0.05, Statistical Power (1-β): 0.8, Effect Size: 0.5, Standard Deviation (σ): 1, Expected Difference in Means (Δ): 0.5 (n=((1.96 + 0.84)ˆ2·2)/0.5^2), n=((2.8)2·2/0.25), n=(7.84·2/0.25), n=(15.68/0.25), n=62.72).
Thus, the required sample size was determined to be 63 participants per group. We excluded individuals with underlying cardiovascular diseases (CVDs), cerebrovascular diseases, carotid artery stenosis, a history of myocardial infarction (MI), cancer, liver and kidney diseases, and autoimmune and inflammatory diseases. Participants who had taken antilipemic drugs or dietary supplements (e.g., vitamin E, vitamin D, and vitamin C) within one month before the study were also excluded. To ensure compliance with the exclusion criteria, detailed medical histories were obtained from all participants through structured interviews conducted by trained physicians. Additionally, medical records were reviewed to verify past diagnoses, medication use, and supplement intake. In cases of uncertainty, participants were asked to provide further clarification regarding their health status and medication history.
Ethics and consent
The researchers collected participant data through a questionnaire, while strictly adhering to the ethical guidelines outlined in the Declaration of Helsinki. The Tehran University of Medical Sciences Ethics Committee (IR.TUMS.MEDICINE.REC.1402.047) granted ethical approval, and all participants provided their informed consent.
Biochemical and demographic parameters
Various biochemical and anthropometric parameters have been collected from the participants in this study. The researchers calculated the body mass index (BMI) and EF% by employing the conventional formula: BMI = weight (kg)/height (m2) and EF% = stroke volume/end-diastolic volume *100. The physician used a standard sphygmomanometer to record participants’ systolic and diastolic blood pressure (SBP and DBP) measurements while they were seated. Following aseptic precautions 5 ml of fasting blood sample was drawn in red top tubes that were later centrifuged at 4000 rpm for 7 minutes to obtain the supernatant serum. The serum was separated and aliquoted and stored at -80 degree C until analysis. (Mindray BS200, China) and commercially available kits (Delta Darman Part, Iran) were used to assess biochemical parameters, including LDL-C, HDL-C, total cholesterol (TC), triglycerides (TG), very low-density lipoprotein (VLDL), fasting blood glucose (FBG), HbA1c (glycated hemoglobin), creatinine, urea, and albumin, following the manufacturer’s guidelines.
Oxidative stress parameters
OS parameters, such as TAC, MDA, MPO activity, SOD activity, CAT activity, and GPx activity, were measured using colorimetric method kits (NAVAND salamat/Iran).
ELISA and chemiluminescent assays
The enzyme-linked immunosorbent assay (ELISA) kit used to conduct the assay of PCSK9 and ox-LDL was obtained from Wuhan Feiyue, China (E23ONI609):. hs-cTnI and hs-CRP were measured using a chemiluminescent immunometric assay kit from Aldeal Lab-Tech, Cyprus (E23OAY398).
Statistical analysis
The qualitative parameters have been represented as numbers (%), whereas the quantitative parameters have been provided as means ± standard errors of the mean (mean ± SEM). We determined data normality using the Kolmogorov-Smirnov test. Group differences were determined using specific analyses, such as Chi-square or exact Fisher’s tests for qualitative parameters and one-sample t-tests or Mann-Whitney U tests for the quantitative parameters. Data correlations were explored using Spearman’s test. hs-CTnI frequency levels have been changed from asymmetrical distributions to ln to improve the linear model’s normality assumption. The logistic regression analysis examined the relationship between CAB > 50% and various parameters. The analysis included both crude and adjusted models, with adjustments made for age, BMI, and gender. Risk ratios (RR) of disease (CAB > 50%) are calculated by dividing the data into quartiles (Q1, Q2, Q3, and Q4), using the 1st quarter (Q1) as the reference for comparison. The Receiver Operating Characteristic (ROC) with an Area Under the Curve (AUC) at 95% Confidence Interval (CI) was used to figure out how useful the parameters were for diagnosing CAB > 50%. The Youden index was employed to calculate cut-off levels for each parameter based on their highest sensitivity and specificity. The analysis was conducted using SPSS software version 27, and graphs were drawn using GraphPad Prism software (version 9). Statistical significance was assessed using a significance value of p < 0.05.
Results
Demographic and biochemical parameters
Table 1 provides details of the demographic and biochemical parameters. Age (P = 0.002), SBP (P = 0.01), BMI (P = 0.01), FBG (P = 0.008), HbA1c (P = 0.02), and TC (P = 0.02) were higher in the CAB > 50% group compared to controls. There were no notable differences observed in gender, DBP, TG, LDL-C, HDL-C, VLDL, creatinine, urea, and albumin amongst the groups being studied.
Oxidative stress parameters
The healthy subjects exhibited a higher serum concentration of TAC (p < 0.001), GPx (P = 0.03), CAT (p < 0.001), and SOD (p < 0.001) activity compared to the CAB > 50% group. Alternatively, the findings indicated that those with a CAB > 50% exhibited elevated levels of MPO and MDA (p < 0.001).
MPO, MDA, TAC, GPx, CAT, and SOD activity
Figure 1 illustrates the comparison of MPO, MDA, and TAC levels, as well as the activity of GPx, CAT, and SOD enzymes, between the control and study groups. The results indicate that the study group exhibited significantly higher levels of MPO (Figure 1A, p < 0.001) and MDA (Figure 1B, p < 0.001) compared to the control group. Conversely, the total antioxidant capacity (TAC) was significantly lower in the study group (Figure 1C, p < 0.001).
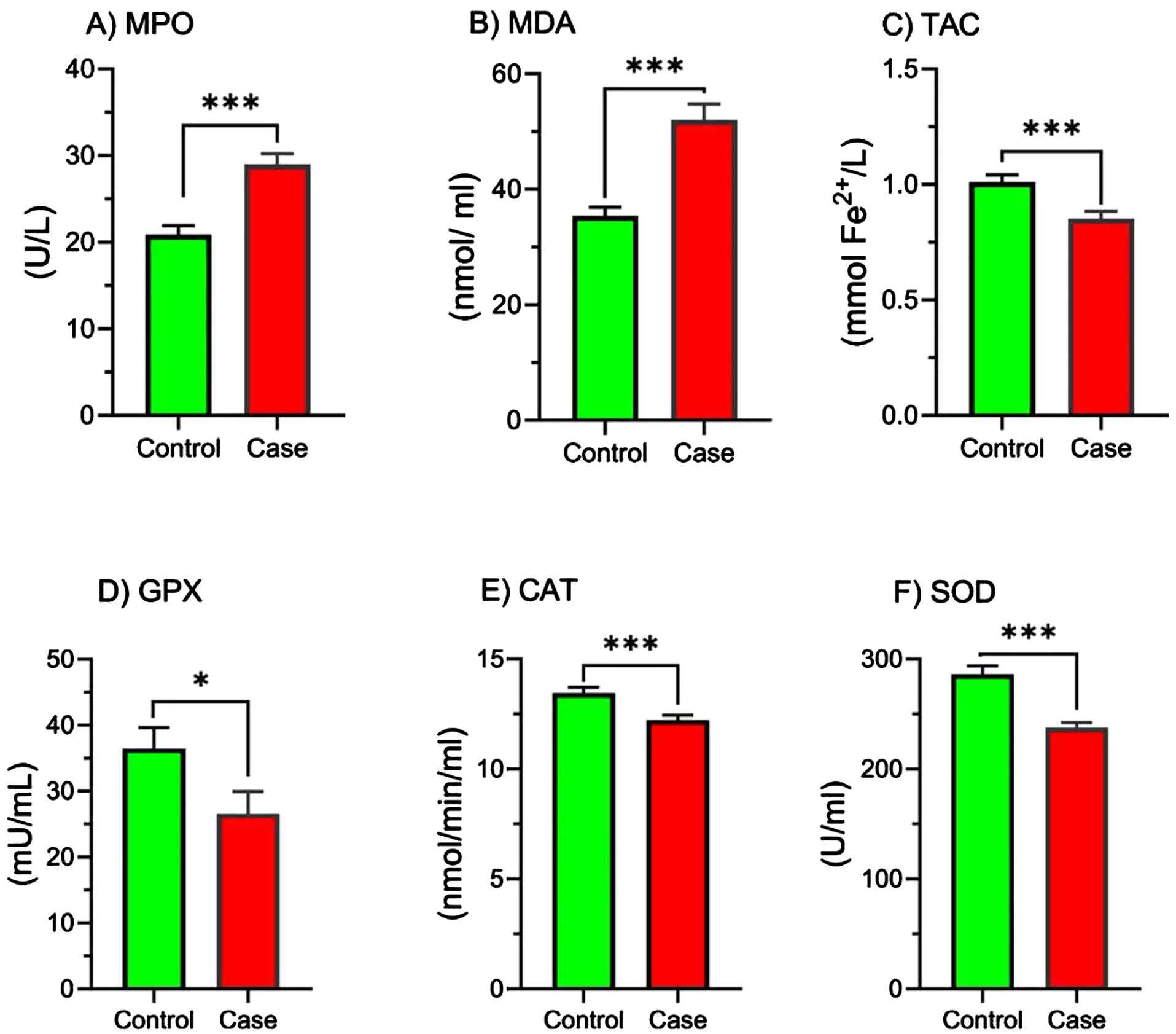
Figure 1. Comparison of MPO, MDA, TAC, GPx, CAT, and SOD activity in study groups. Comparison of oxidative stress markers and antioxidant enzyme activities between the control and case groups. Bar graphs illustrate the levels of (A) myeloperoxidase (MPO), (B) malondialdehyde (MDA), (C) total antioxidant capacity (TAC), (D) glutathione peroxidase (GPX), (E) catalase (CAT), and (F) superoxide dismutase (SOD) in the control (green bars) and case (red bars) groups. MPO, Myeloperoxidase; MDA, Malondialdehyde; TAC, Total antioxidant capacity; GPx, Glutathione Peroxidase; CAT, Catalase; SOD, Superoxide dismutase. Parameters are presented as mean ± SEM. One-sample t-tests or Mann-Whitney U tests were used to analyze data, and the significance level is as: *p < 0.05, ***p < 0.001.
Furthermore, the activity of the antioxidant enzymes GPx (Figure 1D, p < 0.05), CAT (Figure 1E, p < 0.001), and SOD (Figure 1F, p < 0.001) was significantly reduced in the study group.
EF%, hs-CRP, ox-LDL, hs-CTnI, and PCSK9 levels
Figure 2 elucidates the concentrations of hs-CRP, ox-LDL, hs-CTnI, PCSK9, and EF% levels in study groups. Based on these, hs-CRP, ox-LDL, and hs-CTnI have been greater in the CAB > 50% relative to the control (p < 0.001). The EF% was lower in the CAB > 50% group compared to the control (p < 0.001). Furthermore, no difference was shown in PCSK9 levels.
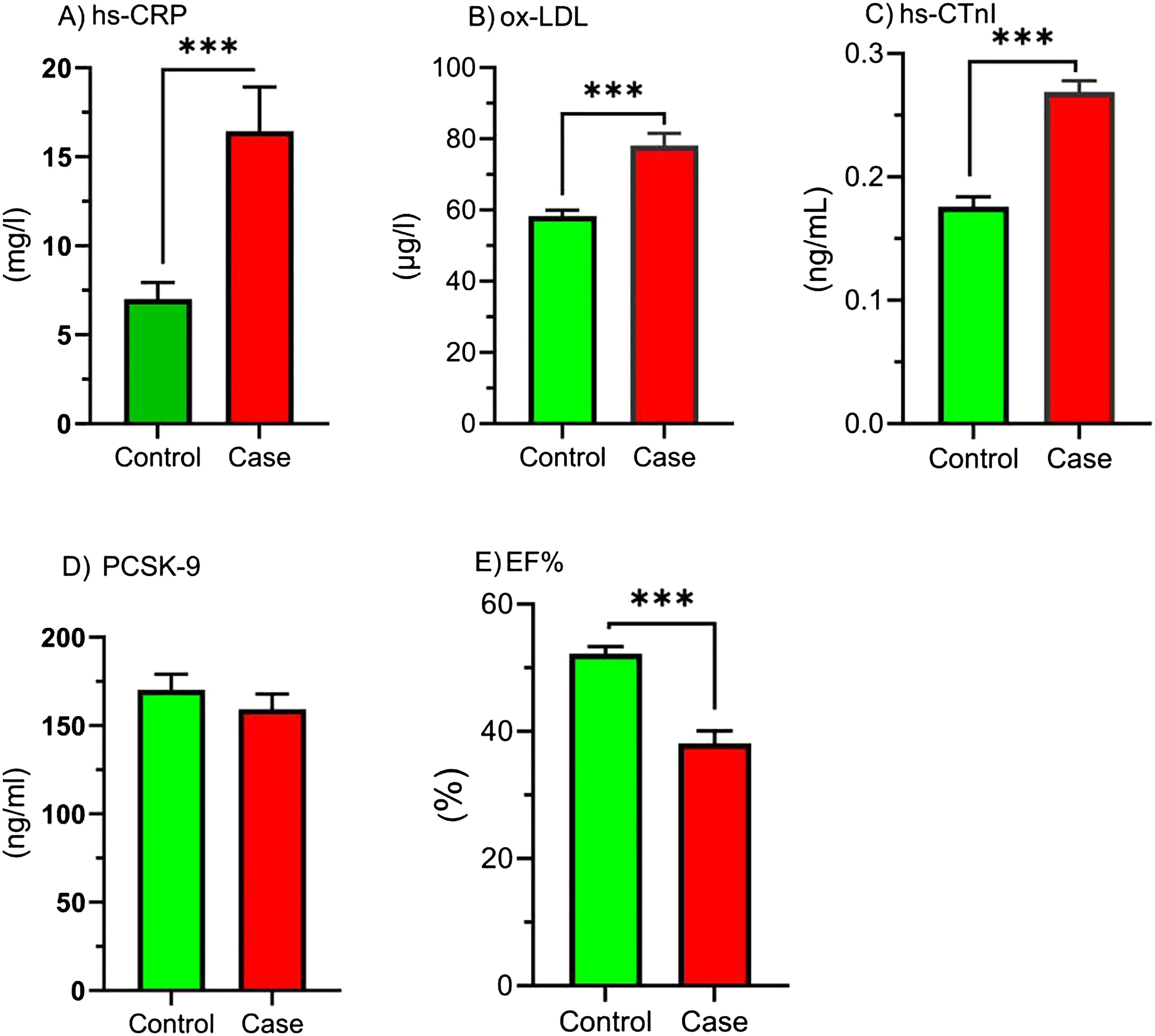
Figure 2. Comparison of hs-CRP, ox-LDL, hs-CTnI, PCSK9, and EF% in study groups. Comparison of inflammatory markers, oxidized LDL, cardiac marker, PCSK-9, and ejection fraction between control and case groups. Bar graphs show the levels of (A) high-sensitivity C-reactive protein (hs-CRP), (B) oxidized low-density lipoprotein (ox-LDL), (C) high-sensitivity cardiac troponin I (hs-cTnI), (D) proprotein convertase subtilisin/kexin type 9 (PCSK-9), and (E) ejection fraction (EF%) in the control (green bars) and case (red bars) groups. Parameters are presented as the mean ± SEM. One-sample t-tests or Mann-Whitney U tests were used to analyze the data, and the significance level is as follows: *** p < 0.001. hs-CRP, High-sensitivity C-reactive Protein; ox-LDL, Oxidized low-density lipoprotein; hs-CTnI, High-sensitivity cardiac troponin I; PCSK9, proprotein convertase subtilisin/kexin type 9; EF %, Ejection fraction percentage.
Correlation coefficient
Table 2 displays the correlation coefficients ranging from -0.39 to 0.55, obtained from Spearman’s correlation assessment aimed at ascertaining the link between variables. According to the table, EF% exhibits a positive link with SOD and a negative correlation with MDA, MPO, ox-LDL, hs-cTnI, and hs-CRP (p < 0.05). hs-cTnI has a positive correlation with MDA, MPO, and ox-LDL and a negative correlation with CAT, GPx, and SOD (p < 0.05). ox-LDL exhibits a positive correlation with MPO and an inverse correlation with TAC, CAT, and GPx (p < 0.05). MDA has a negative association with TAC and SOD (p < 0.05). SOD is directly associated with TAC and CAT (p < 0.05). hs-CRP has a negative correlation with TAC, and GPx has a positive correlation with TAC (p < 0.05). Indeed, MPO has a negative relationship with CAT, and PCSK9 positively related to MDA (p < 0.05).
Logistic regression
We conducted a logistic regression analysis based on quartiles, with Q1 as the reference for the other quartiles, to determine the impact of increasing or decreasing parameters on the risk of disease. This approach is particularly useful when parameters, such as oxidative stress markers (MPO and ox-LDL), lack established reference values or clinical thresholds. A logistic regression analysis was conducted to assess the severity of the disease (CAB > 50%). Data categorized into quartiles, specifically Q1, Q2, Q3, and Q4. The first quarter served as a reference for determining and establishing the risk ratio (RR) of the CAB > 50%. Table 3 displays the result. Adjusted TAC in 4th quartiles (TAC-Q4, RR = 0.13, P = 0.02), adjusted SOD in 4th quartiles (SOD-Q4, RR = 0.09, P = 0.003), adjusted CAT in 3rd and 4th quartiles (CAT-Q3, RR = 0.09, P = 0.005; CAT-Q4, RR = 0.05, P = 0.003), and adjusted GPx in the 2nd, 3rd, and 4th quartiles (GPx-Q2, RR = 0.06, P =0.003; GPx-Q3, RR = 0.08, P = 0.006; GPx-Q3, RR = 0.2, P = 0.04) reduce risk of disease. While adjusted EF% in all of the quartiles (EF%-Q2, RR = 0.07, p < 0.001; EF%-Q3, RR = 0.03, p < 0.001; EF%-Q4, RR = 0.01, p < 0.001) decrease the risk of diseases. Adjusted ln-hs-cTnI in 3rd and 4th quartiles (hs-cTnI-Q3, RR = 2.86, P = 0.04, hs-cTnI-Q4, RR = 6.29, p < 0.001), and adjusted ox-LDL in 3rd and 4th quartiles (ox-LDL-Q3, RR = 32.93, P = 0.008, ox-LDL-Q4, RR = 67.36, P = 0.004) elevate the risk of diseases. Furthermore, adjusted MDA in all quartiles (MDA-Q2, RR = 14.18, P = 0.03; MDA-Q3, RR = 30.31, P = 0.004; MDA-Q4, RR = 61.29, P = 0.001), adjusted MPO in all quartiles (MPO-Q2, RR = 7.38, P = 0.01; MPO-Q3, RR = 15.42, p < 0.001; MPO-Q4, RR= 0.01; hs-CRP-Q4, RR = 14.5, p < 0.001) increased risk of diseases. Also, adjusted PCSK9 in 2nd quartiles (PCSK9-Q2, RR = 7.89, p = 0.002) increases the risk of disease.
ROC curve
The AUC and ROC curve indicate the overall effectiveness of a test; the AUC for an optimal ROC curve is 100. ROC analysis determined the optimal cut-off with the highest specificity and sensitivity for MI (CAB > 50%) diagnostic parameters. Figure 3(A1, A2, ETC) and Table 4 illustrate the results of the study. Figure 3 displays the Receiver Operating Characteristic (ROC) curves and corresponding cut-off values for several diagnostic markers in relation to significant coronary artery blockage (CAB > 50%). The figure is organized into panels, each dedicated to a specific marker: ox-LDL (A1, A2), hs-CTnI (B1, B2), PCSK9 (C1, C2), hs-CRP (D1, D2), and EF% (E1, E2). For each marker, the left panel (A1, B1, C1, D1, E1) presents a scatter plot showing the distribution of marker levels in both control patients and those with CAB > 50%, with the optimal cut-off value indicated. The right panel (A2, B2, C2, D2, E2) displays the ROC curve itself, plotting sensitivity against 100% - specificity. The area under the curve (AUC) and its associated p-value are provided for each ROC curve, indicating the marker’s ability to discriminate between the two groups. The study determined the best AUC and the optimal cut-off value for variables in identifying the CAB > 50% categories as follows: ox-LDL (AUC = 83.22, cut-off > 63.35 μg/L, p < 0.001), EF% (AUC = 82.35, cut-off < 46.25%, p < 0.001), hs-cTnI (AUC = 81.3, cut-off > 0.265 ng/ml, p < 0.001), hs-CRP (AUC = 60.94, cut-off > 10.85 mg/ml, p = 0.04), and PCSK9 (AUC = 56.75, cut-off > 168.3 ng/ml, p = 0.24).
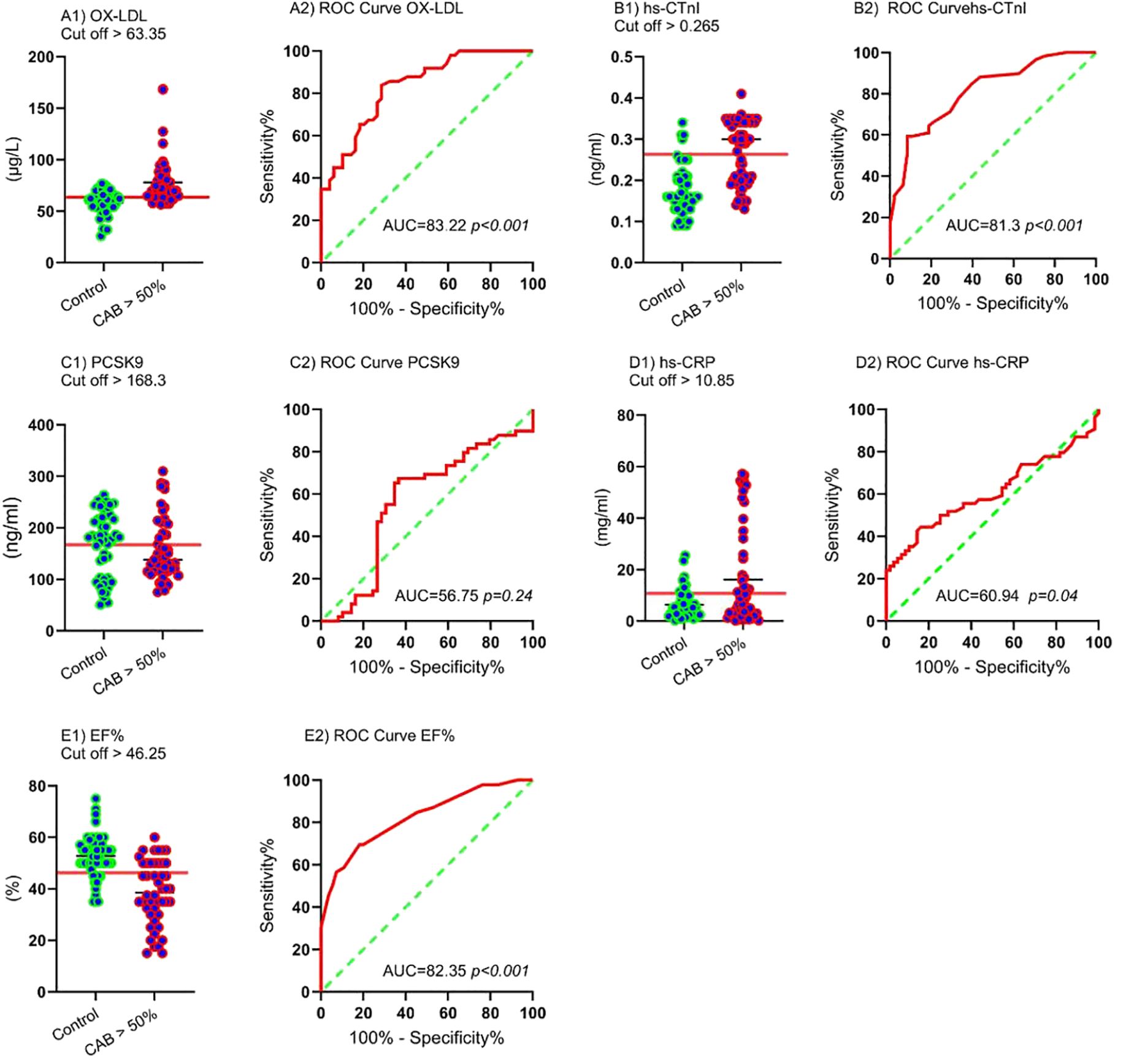
Figure 3. ROC curves and cut-off values for CAB > 50% ox-LDL: Cut-off (A1) and ROC curve (A2), hs-CTnI: Cut-off (B1) and ROC curve (B2), PCSK9: Cut-off (C1) and ROC curve (C2), hs-CRP: Cut-off (D1) and ROC curve (D2), EF%: Cut-off (E1) and ROC curve (E2).
Discussion
This study found that elevated MDA, MPO, hs-cTnI, hs-CRP, and ox-LDL increased the risk of CAB > 50% development, while higher TAC, SOD, GPx, CAT, and EF% lowered the risk of CAB > 50% development. However, there is no difference in PCSK9, LDL-C, and HDL-C levels, and they are associated with CAB > 50% development. EF% has a positive association with SOD and a negative association with MDA, MPO, ox-LDL, hs-cTnI, and hs-CRP. hs-cTnI has a direct link with MDA, MPO, and ox-LDL and an indirect link with CAT, GPx, and SOD. Indeed, ox-LDL has a direct correlation with MPO and an indirect correlation with TAC, CAT, and GPx. hs-CRP negatively associates with TAC, and PCSK9 has a positive correlation with OS. Furthermore, ox-LDL, EF%, and hs-cTnI have the highest AUC and sensitivity-specificity for detecting CAB > 50%.
PCSK9, alternatively referred to as neural apoptosis-regulated convertase-1 (NARC-1), was classified as the 9th member of the proteinase K subfamily when it was initially identified in 2003 (20, 33). The main function of PCSK9 in cholesterol regulation is to decrease LDLR on the surface of the hepatic membrane, increase LDL-C in circulation, and increase the risk of atherosclerosis and CVDs (22). PCSK9 has been implicated in activating platelets and causing thrombosis, altering lipid metabolism and increase LDL-C, increasing inflammation and activating inflammatory cells, promoting cell death through pyroptosis, autophagy, and apoptosis, raising levels of ROS and induce OS, and increase ox-LDL levels (20, 22, 23, 34). Based on these functions, PCSK9 inhibitors are used to reduce the risk of CVDs, atherosclerosis, and hypercholesterolemia in patients with recent MI and improve patient mortality. However power randomized controlled trial (RCT) studies are needed to prove this, and further surveys are needed (35). The results of these studies indicate that PCSK9 LDL-C, and HDL-C has no difference between the case and control, and there is positive correlation with MDA and OS. Almontashiri et al. reported elevated PCSK9 levels exclusively in acute myocardial infarction among CAD patients, while they remained unaltered in chronic myocardial infarction. Because CAD is a chronic condition, PCSK9 is not produced at elevated levels in all patients, but its production does increase during acute events. Additionally, some studies show that LDL-C and HDL-C levels are normal in patients and do not play a role in the incidence of atherosclerosis (1, 9, 36), indicating that other risk factors should be evaluated. Laugsand in the prospective population study showed PCSK9 levels increase the risk of MI in adjusted analysis with age and sex. Furthermore, they adjusted PCSK9 levels with LDL-C and other lipids observed to decrease the risk of MI (37). The findings of this study suggest that an increase in PCSK9 during acute conditions primarily influences the development of myocardial infarction (MI) and atherosclerosis through lipids and LDL-C. However, in chronic conditions where PCSK9 levels do not differ, other functions, such as oxidative stress (OS), are explored. According to Peng et al., PCSK9 levels in CAD plaques may act as a biomarker for detecting coronary artery disease (CAD) and can be linked to cardiac markers. However, the effectiveness of PCSK9 levels as a diagnostic marker for CAD remains uncertain (38). Also, ROC and AUC analyses indicated that PCSK9 is not worth investigating as a biomarker (AUC = 56.75, P = 0.24).
Inflammation and oxidative stress (OS) play a key role in the development of atherosclerosis and heart attacks (MI). This study found that MDA and oxidized LDL (ox-LDL) levels were higher in patients with coronary artery blockage (CAB) greater than 50%, while antioxidant systems like TAC, GPx, SOD, and CAT were less active. Research by Sagha et al. and Shiri et al. also shows increased OS in cases of CAB > 50%, along with higher nitric oxide and MDA levels, and lower TAC levels (1, 9). Furthermore, TAC (> 1.05 mmol Fe2+/L), SOD (> 297.16 U/ml), CAT (> 11.32 nmol/min/ml), and GPx (> 16.48 mU/mL) decrease the risk of disease, and MDA (> 32.07 nmol/ml) increases the risk of disease. During OS, ROS converts LDL-C to ox-LDL, is absorbed by macrophages, and then converted to foam cells (11). Mechanisms Involved in PCSK9’s Effects on OS: I) Impact on SIRT3: Research indicates that PCSK9i can counteract IL-6-induced inflammation, autophagy, and oxidative stress in endothelial cells. These protective effects are partially mediated by SIRT3; silencing SIRT3 reverses the benefits observed with PCSK9i treatment.
II) Effect on Autophagy: PCSK9i have been shown to reduce IL-6-induced autophagy in endothelial cells. This reduction is also mediated by SIRT3, as evidenced by the reversal of protective effects upon SIRT3 silencing. III) Effect on Oxidative Stress: PCSK9i can decrease mitochondrial ROS accumulation and improve mitochondrial membrane potential in endothelial cells. These effects are mediated by SIRT3, as silencing SIRT3 reverses the benefits of PCSK9i treatment. In summary, PCSK9i exhibit intrinsic anti-inflammatory, anti-autophagic, and antioxidant properties in endothelial cells, with these pleiotropic effects mediated, at least in part, by SIRT3. These findings provide new insights into the cardioprotective mechanisms of PCSK9i beyond LDL-cholesterol lowering (13). Also, The mechanism of PCSK9 in platelet activation is as follows: PCSK9 directly binds to the CD36 receptor on the platelet surface, enhancing platelet activation and downstream signaling, including Src and JNK kinases. Furthermore, PCSK9 increases ROS production through p38MAPK phosphorylation, leading to Nox2 activation, PLA2, arachidonic acid, and TxA2 signaling. Nox2-mediated ROS production increases ox-LDL formation, which amplifies platelet activation via both LOX1 and CD36 platelet receptors. All these events act as an amplifying signal for platelet activation, resulting in p-selectin expression, CD40L expression, and the release of granule contents (39).
PCSK9 enhances inflammation, oxidative stress (OS), and autophagy, though the exact mechanism is still unclear (12). Foam cells release inflammatory molecules and attractants like PDGF and TNF-α, which then activate vascular smooth muscle cells (VSMC), leading to the growth of the extracellular matrix (ECM) and the narrowing of arteries (6, 40). ROS-scavenging antioxidant systems correlate directly with EF% and with each other, and indirectly with ox-LDL, hs-CRP, and hs-cTnI. Increased myocardial infarction (MI) and myocardial damage lead to elevated hs-cTnI release. Studies by Muhammad et al. on aortic valve, mitral valve, and combined disease illustrated an increase in OS and related parameters, hs-cTnI and hs-CRP, an increase in the risk of disease, and a decrease in cardiac function (39). MPO and hs-CRP elevated in CAB > 50%, MPO (> 17.77 U/L), and hs-CRP (> 5.5 mg/l) increase the risk of disease. Indeed, they have positive correlations with hs-cTnI and negative correlations with TAC, SOD, and EF%. Neutrophils predominantly generate MPO, which is elevated in cases of MI and other inflammatory responses. Neutrophils secrete it into the extracellular fluid following OS and various inflammatory responses in MI (41). Mocatta et al. showed that MPO and OS are elevated in MI, and they increase the risk of MI and are negatively correlated with left ventricular ejection fraction (LVEF) (42). hs-CRP is a biomarker that is produced during the acute phase of inflammation. hs-CRP can influence the development of CADs via several mechanisms, including platelet and complement system activation, promotion of VSMC growth, macrophage activation, and lipid aggregation (43). In this study, hs-CRP boosted the risk of diseases and OS and reduced EF%. Also, ROC and AUC results reveal that hs-CRP (> 10.85 mg/ml) with an AUC of 60.94 is not good for CAB > %50 detection. But ox-LDL has the greatest AUC (AUC = 83.22, cutoff > 63.35 μg/L) for the diagnosis of CAB > %50.
This study indicated an EF% decrease in CAB > 50% and an EF% (> 37.5%) decrease in the risk of disease. An EF% > 50% is considered within the normal range. EF% < 40% suggests inadequate cardiac output and potential heart failure (44). Bauer et al. discovered a significant correlation between an EF% of 30 or lower with mortality in all patients throughout the subsequent five-year period (45). It was also shown that EF% was less than 47.25% with an AUC of 82.35 after ox-LDL, making it the best marker for detecting CAB > 50%. Furthermore, EF% has direct relationships with SOD and indirect relationships with MDA, MPO, ox-LDL, hs-cTnI, and MPO. hs-cTnI increases in CAB > 50% groups, and has positive associations with ox-LDL, MDA, and SOD and negative associations with CAT, GPx, and SOD. Cardiomyopathy and MI caused by OS are associated with signs such as chest pain, changes in the electrocardiogram, and a rise in cardiac biomarkers, including hs-cTnI (39). hs-cTnI serves as a biomarker and the gold standard for detecting MI in patients, but not specific for Cardiomyopathy (28). Based on this study, after ox-LDL and EF%, hs-cTnI (AUC = 81.3, cut-off > 0.265 ng/ml) was considered a biomarker for the detection of CAB > 50%. Indeed, FPG, HbA1c, BMI, and SBP elevated in CAB > 50% more than normal groups. Diabetic patients who do not have a prior history of CAD have an equal likelihood of developing CVDs. Nevertheless, diabetic people who have previously had an MI face a chance of more than 40% of encountering another MI (46). Psaty et al. found a direct association between SBP, DBP, pulse pressure, and the risk of developing MI and stroke. However, SBP demonstrated to be the most accurate indicator of cardiovascular events (47). Excess weight and elevated BMI can lead to lipid sequestration in the arteries. Arterial damage and obstruction, which impede the flow of blood to the heart, can result in a MI (48).
Furthermore, our study has both strengths and limitations. A key strength is its classification of myocardial infarction (MI) patients into those with significant coronary artery blockage (CAB > 50%) based on angiographic findings. We then measured PCSK9, other cardiac parameters, and oxidative stress markers in this CAB > 50% group, examining the interrelationships between these factors. Furthermore, we evaluated the diagnostic potential of ox-LDL, hs-CTnI, PCSK9, hs-CRP, and EF% as biomarkers for CAB > 50%. However, our study is limited by its relatively small sample size. We also lacked additional demographic and dietary information, as well as measurements of small dense LDL levels. Future research should investigate PCSK9 protein levels in both chronic and acute disease states, exploring their relationship with relevant signaling pathways and their influence on the frequency and severity of heart attacks and atherosclerosis. This will provide a more comprehensive understanding of PCSK9’s role in the pathophysiology of these conditions.
Conclusion
Our research indicated that even though PCSK9 plays a role in the incidence of MI by increasing LDL-C, other functions such as oxidative stress (OS) and inflammation must also be considered. Therefore, to properly evaluate PCSK9 function, LDL-C, inflammation, and OS parameters should be taken into account. Notably, while hs-cTnI is the gold standard for MI diagnosis, our findings suggest that ox-LDL (AUC = 83.22, > 63.35 μg/L) and EF% (AUC = 82.35, < 46.25%) outperform hs-cTnI (AUC = 81.3, > 0.265 ng/ml) in diagnosing CAB > 50%. This highlights the potential clinical utility of ox-LDL and EF% in early diagnosis and risk stratification, suggesting that they could serve as valuable complementary biomarkers for identifying high-risk patients.
Data availability statement
The original contributions presented in the study are included in the article/supplementary material. Further inquiries can be directed to the corresponding authors.
Ethics statement
The research protocol obtained endorsement from the ethical guidelines committee at TUMS (IR.TUMS.MEDICINE.REC.1402.047). The studies were conducted in accordance with the local legislation and institutional requirements. The participants provided their written informed consent to participate in this study. Written informed consent was obtained from the individual(s) for the publication of any potentially identifiable images or data included in this article.
Author contributions
AL: Writing – original draft. HS: Writing – review & editing. MI: Writing – review & editing. AK: Writing – review & editing. MS: Writing – review & editing. MR: Writing – review & editing. NE: Writing – original draft, Writing – review & editing. GP: Writing – original draft, Writing – review & editing.
Funding
The author(s) declare that financial support was received for the research and/or publication of this article. The research was funded partially by TUMS Grant No.1402-2-102-68020.
Conflict of interest
The authors declare that the research was conducted in the absence of any commercial or financial relationships that could be construed as a potential conflict of interest.
Publisher’s note
All claims expressed in this article are solely those of the authors and do not necessarily represent those of their affiliated organizations, or those of the publisher, the editors and the reviewers. Any product that may be evaluated in this article, or claim that may be made by its manufacturer, is not guaranteed or endorsed by the publisher.
Abbreviations
CAD, Coronary artery disease; OS, oxidative stress; LDL-C, Low-Density Lipoprotein-cholesterol; TAC, Total antioxidant capacity; CAT, Catalase; GPx, Glutathione Peroxidase; SOD, Superoxide dismutase; MDA, Malondialdehyde; MPO, Myeloperoxidase; ox-LDL, Oxidized low-density lipoprotein; PCSK9, Proprotein convertase subtilisin/kexin type 9; hs-CRP, High-sensitivity C-reactive Protein; EF%, Ejection fraction percentage; ROC, Receiver Operating Characteristic; AUC, Area Under the Curve.
References
1. Shiri H, Sagha A, Nasri H, Mehdeipour S, Fooladi S, Mehrabani M, et al. Lithium and zinc levels along with oxidative status in myocardial infarction: A case-control study. Heliyon. (2023) 9(11). doi: 10.1016/j.heliyon.2023.e21875
2. Brown JC, Gerhardt TE, Kwon E. Risk factors for coronary artery disease. In: StatPearls. StatPearls Publishing Copyright © 2023 StatPearls Publishing LLC, Treasure Island (FL (2023).
3. Sarrafzadegan N, Mohammmadifard N. Cardiovascular disease in Iran in the last 40 years: prevalence, mortality, morbidity, challenges and strategies for cardiovascular prevention. Arch Iran Med. (2019) 22:204–10.
4. Albeladi F, Salem IW, Zahrani M, Alarbedi L, Abukhudair A, Alnafei H, et al. Incidence of coronary artery disease in king abdulaziz university hospital, jeddah, Saudi Arabia, 2019–2020: A retrospective cohort study. Cureus. (2022) 14:e28770. doi: 10.7759/cureus.28770
5. Fooladi S, Faramarz S, Dabiri S, Kajbafzadeh A, Nematollahi MH, Mehrabani M. An efficient strategy to recellularization of a rat aorta scaffold: an optimized decellularization, detergent removal, and Apelin-13 immobilization. Biomater Res. (2022) 26:46. doi: 10.1186/s40824-022-00295-1
6. Soleimani AA, Ghasmpour G, Mohammadi A, Gholizadeh M, Abkenar BR, Najafi M. Focal adhesion kinase–related pathways may be suppressed by metformin in vascular smooth muscle cells in high glucose conditions. Endocrinology Diabetes Metab. (2022) 5:e351. doi: 10.1002/edm2.351
7. Charytan D, Kuntz RE, Mauri L, DeFilippi C. Distribution of coronary artery disease and relation to mortality in asymptomatic hemodialysis patients. Am J Kidney Dis. (2007) 49:409–16.
8. Liu J, Jing L, Tu X. Weighted gene co-expression network analysis identifies specific modules and hub genes related to coronary artery disease. BMC Cardiovasc Disord. (2016) 16:54.
9. Sagha A, Shiri H, Juybari KB, Mehrabani M, Nasri HR, Nematollahi MH, et al. The association between arsenic levels and oxidative stress in myocardial infarction: A case–control study. Cardiovasc Toxicol. (2023) 23:61–73. doi: 10.1007/s12012-022-09778-y
10. Hong CG, Florida E, Li H, Parel PM, Mehta NN, Sorokin AV. Oxidized low-density lipoprotein associates with cardiovascular disease by a vicious cycle of atherosclerosis and inflammation: A systematic review and meta-analysis. Front Cardiovasc Med. (2022) 9:1023651.
11. Dubois-Deruy E, Peugnet V, Turkieh A, Pinet F. Oxidative stress in cardiovascular diseases. Antioxidants. (2020) 9:864.
12. D’Onofrio N, Prattichizzo F, Marfella R, Sardu C, Martino E, Scisciola L, et al. SIRT3 mediates the effects of PCSK9 inhibitors on inflammation, autophagy, and oxidative stress in endothelial cells. Theranostics. (2023) 13:531–42.
13. Shiri H, Karimpour A, Sattari M, Hemmati S, Seyyedebrahimi S, Panahi G. Evaluation of antioxidant potential and free radical scavenging activity of methanol extract from Scrophularia striata. Acta Biochim Iranica. (2023) 1(2):71–7. doi: 10.18502/abi.v1i2.14103
14. Pahwa R, Goyal A, Jialal I. Chronic inflammation. Treasure Island (FL): StatPearls Publishing (2018).
15. Shafi O. Switching of vascular cells towards atherogenesis, and other factors contributing to atherosclerosis: a systematic review. Thromb J. (2020) 18:28. doi: 10.1186/s12959-020-00240-z
16. Sedaghat M-R, Shiri H, Tavakkol-Afshari J, Norouzmahani ME, Bahri F, Fooladi S, et al. Impact of a 50bp insertion/deletion polymorphism of the superoxide dismutase-1 on oxidative stress status and risk of keratoconus. Exp Eye Res. (2024) 238:109742. doi: 10.1016/j.exer.2023.109742
17. Parthasarathy S, Raghavamenon A, Garelnabi MO, Santanam N. Oxidized low-density lipoprotein. Methods Mol Biol. (2010) 610:403–17.
18. Abbasi-Jorjandi M, Asadikaram G, Abolhassani M, Fallah H, Abdollahdokht D, Salimi F, et al. Pesticide exposure and related health problems among family members of farmworkers in southeast Iran. A case-control study. Environ pollut. (2020) 267:115424.
19. Bastin A, Fooladi S, Doustimotlagh AH, Vakili S, Aminizadeh AH, Faramarz S, et al. A comparative study on the effect of blood collection tubes on stress oxidative markers. PloS One. (2022) 17:e0266567. doi: 10.1371/journal.pone.0266567
20. Puteri MU, Azmi NU, Kato M, Saputri FC. PCSK9 promotes cardiovascular diseases: recent evidence about its association with platelet activation-induced myocardial infarction. Life. (2022) 12:190. doi: 10.3390/life12020190
21. Seidah NG. The PCSK9 discovery, an inactive protease with varied functions in hypercholesterolemia, viral infections, and cancer. J Lipid Res. (2021) 62:100130.
23. Dounousi E, Tellis C, Pavlakou P, Duni A, Liakopoulos V, Mark P, et al. Association between PCSK9 levels and markers of inflammation, oxidative stress, and endothelial dysfunction in a population of nondialysis chronic kidney disease patients. Oxid Med Cell Longev. (2021) 2021:6677012. doi: 10.1155/2021/6677012
24. Li S, Zhang Y, Xu RX, Guo YL, Zhu CG, Wu NQ, et al. Proprotein convertase subtilisin-kexin type 9 as a biomarker for the severity of coronary artery disease. Ann Med. (2015) 47:386–93.
25. Alonso R, Mata P, Muñiz O, Fuentes-Jimenez F, Díaz JL, Zambón D, et al. PCSK9 and lipoprotein (a) levels are two predictors of coronary artery calcification in asymptomatic patients with familial hypercholesterolemia. Atherosclerosis. (2016) 254:249–53.
26. Jin Q, Lau ESH, Luk AO, Tam CHT, Ozaki R, Lim CKP, et al. High-density lipoprotein subclasses and cardiovascular disease and mortality in type 2 diabetes: analysis from the Hong Kong Diabetes Biobank. Cardiovasc Diabetol. (2022) 21:293. doi: 10.1186/s12933-022-01726-y
27. Seidah NG, Awan Z, Chrétien M, Mbikay M. PCSK9: a key modulator of cardiovascular health. Circ Res. (2014) 114:1022–36. doi: 10.1161/CIRCRESAHA.114.301621
28. Yan I, Börschel CS, Neumann JT, Sprünker NA, Makarova N, Kontto J, et al. High-sensitivity cardiac troponin I levels and prediction of heart failure: results from the biomarCaRE consortium. JACC: Heart Failure. (2020) 8:401–11. doi: 10.1016/j.jchf.2019.12.008
29. Navarese EP, Kolodziejczak M, Winter MP, Alimohammadi A, Lang IM, Buffon A, et al. Association of PCSK9 with platelet reactivity in patients with acute coronary syndrome treated with prasugrel or ticagrelor: The PCSK9-REACT study. Int J Cardiol. (2017) 227:644–9. doi: 10.1016/j.ijcard.2016.10.084
30. Camera M, Rossetti L, Barbieri SS, Zanotti I, Canciani B, Trabattoni D, et al. PCSK9 as a positive modulator of platelet activation. J Am Coll Cardiol. (2018) 71:952–4. doi: 10.1016/j.jacc.2017.11.069
31. Cammisotto V, Pastori D, Nocella C, Bartimoccia S, Castellani V, Marchese C, et al. PCSK9 regulates Nox2-mediated platelet activation via CD36 receptor in patients with atrial fibrillation. Antioxidants. (2020) 9:296. doi: 10.3390/antiox9040296
32. Cammisotto V, Baratta F, Simeone PG, Barale C, Lupia E, Galardo G, et al. Proprotein convertase subtilisin kexin type 9 (PCSK9) beyond lipids: The role in oxidative stress and thrombosis. Antioxidants. (2022) 11:569. doi: 10.3390/antiox11030569
33. Piper DE, Jackson S, Liu Q, Romanow WG, Shetterly S, Thibault ST, et al. The crystal structure of PCSK9: a regulator of plasma LDL-cholesterol. Structure. (2007) 15:545–52. doi: 10.1016/j.str.2007.04.004
34. Ma M, Hou C, Liu J. Effect of PCSK9 on atherosclerotic cardiovascular diseases and its mechanisms: Focus on immune regulation. Front Cardiovasc Med. (2023) 10:1148486.
35. Gencer B, Mach F. PCSK9 Inhibition could be Effective for Acute Myocardial Infarction. Curr medicinal Chem. (2022) 29:1016–26.
36. Fernández-Friera L, Fuster V, López-Melgar B, Oliva B, García-Ruiz JM, Mendiguren J, et al. Normal LDL-cholesterol levels are associated with subclinical atherosclerosis in the absence of risk factors. J Am Coll Cardiol. (2017) 70:2979–91. doi: 10.1016/j.jacc.2017.10.024
37. Laugsand LE, Åsvold BO, Vatten LJ, Janszky I, Platou CG, Michelsen AE, et al. Circulating PCSK9 and risk of myocardial infarction: the HUNT study in Norway. JACC: Basic to Trans Sci. (2016) 1:568–75. doi: 10.1016/j.jacbts.2016.06.007
38. Peng J, Liu MM, Jin JL, Cao YX, Guo YL, Wu NQ, et al. Association of circulating PCSK9 concentration with cardiovascular metabolic markers and outcomes in stable coronary artery disease patients with or without diabetes: a prospective, observational cohort study. Cardiovasc Diabetol. (2020) 19:167. doi: 10.1186/s12933-020-01142-0
39. Jan MI, Khan RA, Fozia, Ahmad I, Khan N, Urooj K. C-reactive protein and high-sensitive cardiac troponins correlate with oxidative stress in valvular heart disease patients. Oxid Med Cell Longev. (2022) 30:2022:5029853. doi: 10.1155/2022/5029853
40. Batty M, Bennett MR, Yu E. The role of oxidative stress in atherosclerosis. Cells. (2022) 11:3843.
41. Khan AA, Alsahli MA, Rahmani AH. Myeloperoxidase as an active disease biomarker: recent biochemical and pathological perspectives. Med Sci (Basel). (2018) 6(2). doi: 10.20944/preprints201802.0104.v1
42. Mocatta TJ, Pilbrow AP, Cameron VA, Senthilmohan R, Frampton CM, Richards AM, et al. Plasma concentrations of myeloperoxidase predict mortality after myocardial infarction. J Am Coll Cardiol. (2007) 49:1993–2000. doi: 10.1016/j.jacc.2007.02.040
43. Polyakova EA, Mikhaylov EN. The prognostic role of high-sensitivity C-reactive protein in patients with acute myocardial infarction. J Geriatr Cardiol. (2020) 17:379–83.
44. Wohlfahrt P, Jenča D, Melenovský V, Šramko M, Kotrč M, Želízko M, et al. Trajectories and determinants of left ventricular ejection fraction after the first myocardial infarction in the current era of primary coronary interventions. Front Cardiovasc Med. (2022) 9:1051995. doi: 10.3389/fcvm.2022.1051995
45. Bauer A, Barthel P, Schneider R, Ulm K, Müller A, Joeinig A, et al. Improved Stratification of Autonomic Regulation for risk prediction in post-infarction patients with preserved left ventricular function (ISAR-Risk). Eur Heart J. (2009) 30:576–83. doi: 10.1093/eurheartj/ehn540
46. Cui J, Liu Y, Li Y, Xu F, Liu Y. Type 2 diabetes and myocardial infarction: recent clinical evidence and perspective. Front Cardiovasc Med. (2021) 8:644189. doi: 10.3389/fcvm.2021.644189
47. Psaty BM, Furberg CD, Kuller LH, Cushman M, Savage PJ, Levine D, et al. Association between blood pressure level and the risk of myocardial infarction, stroke, and total mortality: the cardiovascular health study. Arch Internal Med. (2001) 161:1183–92. doi: 10.1001/archinte.161.9.1183
Keywords: PCSK9, oxidative stress, myocardial infarction, ox-LDL, EF%
Citation: Lafta AH, Shiri H, Iraji M, Karimpour A, Sattari M, Rahimkhani M, Einollahi N and Panahi G (2025) Investigation of the interplay of PCSK9, cardiac dynamics, oxidative stress in coronary artery disease: case-control study. Front. Endocrinol. 16:1494438. doi: 10.3389/fendo.2025.1494438
Received: 16 September 2024; Accepted: 04 March 2025;
Published: 28 April 2025.
Edited by:
Amir Mohammad Malvandi, Ospedale Galeazzi S.p.A, ItalyReviewed by:
Omer Iqbal, Loyola University Chicago, United StatesBelma Pojskic, Medical faculty University Zenica, Bosnia and Herzegovina
Copyright © 2025 Lafta, Shiri, Iraji, Karimpour, Sattari, Rahimkhani, Einollahi and Panahi. This is an open-access article distributed under the terms of the Creative Commons Attribution License (CC BY). The use, distribution or reproduction in other forums is permitted, provided the original author(s) and the copyright owner(s) are credited and that the original publication in this journal is cited, in accordance with accepted academic practice. No use, distribution or reproduction is permitted which does not comply with these terms.
*Correspondence: Nahid Einollahi, ZWlub2xhaG5Ac2luYS50dW1zLmFjLmly; Ghodratollah Panahi, cHNoYWhyaXlhckBnbWFpbC5jb20=; Z2hwYW5haGlAc2luYS50dW1zLmFjLmly