- 1INSERM U1043, CNRS U5282, Center of Physiopathology from Toulouse Purpan, University Paul Sabatier, Toulouse, France
- 2European Group of Research (GDRE) Ca2+ Toolkit Coded Proteins as Drug Targets in Animal and Plant Cells
The role of voltage-dependent calcium (Cav1) channels is prominent in excitable cells while store-operated calcium channels (SOCC) were considered as characteristic of non-excitable cells. Cav1 channels are implicated in excitation transcription. Store-operated calcium channels (SOCC) activity is increased during cardiac stress and would contribute to Ca2+ influx and expression of genes responsible for cardiac hypertrophy and heart failure (Luo et al., 2012). Several lines of evidence now show the importance of Cav1 channels in non-excitable cells including lymphocytes (reviewed in Robert et al., 2011, 2013). Cav1 channels are defined by their voltage sensitivity and their sensitivity to drugs as dihydropyridines, phenylalkylamines, benzothiazepines, known to alter T-cell functions. However the drug concentrations needed were higher compared to excitable cells. The absence of cell membrane depolarization upon activation and possible non-specific effects of the drugs questioned the putative role of Cav1 channels in T-cells.
Cav1 channels are formed by the ion forming pore α1 subunit encoded by four genes conferring some tissue-specific expression pattern in excitable cells. Cav1.1 is characteristic of skeletal muscle cells. Cav1.2 is found in neurons, heart, and smooth muscle cells while Cav1.3 is detected in neuroendocrine cells. Cav1.2 and Cav1.3 can be found in the same tissues even if their role is not redundant as shown by the differential phenotypes of Cav1.2 and Cav1.3 null mice. Cav1.4 is the retinal form. Cav1 channel isoforms differ by their sensitivity to depolarization and to antagonizing drugs such as dihydropyridines (DHP) as well as by their inactivation properties (Lipscombe et al., 2004). For example, Cav1.4 channels activate at more negative potentials than Cav1.3 and Cav1.2, which highlights the potential involvement of Cav1.4 in non-excitable cells as mast cells (McRory et al., 2004) and more recently in mouse T-lymphocytes (Omilusik et al., 2011).
Calcium in T-Lymphocytes: Prominent Role of the Stim-Orai Pathway
In T-lymphocytes, Ca2+ ions are important for the activation of many enzymes including phospholipase C gamma (PLCγ), classical protein kinases C, for proper protein folding, for the accessibility of key enzymes in T-cell transduction, and as a second messenger (Vig and Kinet, 2009). Variations in the intracellular calcium concentration ([Ca]i) are responsible for modulating the transcription of more than 75% of genes induced or down-regulated by T-cell receptor engagement in T-lymphocytes (Feske et al., 2001). The intracellular [Ca]i that decides the cellular fate is tightly regulated in both resting and activated conditions. The calcium concentration in the external medium is about 1–2 mM, whereas the [Ca]i is about 50–100 nM and depends on the calcium channels expressed at both the cell and endoplasmic reticulum (ER) membranes, on exchangers, pumps, … Activation of potassium channels that extrude the potassium from the cell is needed for supporting the electrochemical driving force allowing the calcium influx. In T-lymphocytes, TCR engagement results in a cascade of tyrosine kinase activation, the constitution of a platform transducing the signal with the recruitment of adapters and enzymes such as PLCγ that generates inositol trisphosphate (IP3) and diacylglycerol. IP3 binds to its receptors on the ER membrane leading to the release of ER Ca2+ stores, which induces a conformational change of STIM1, an ER Ca2+ sensor. STIM1 then localizes near the cell membrane, and activates the SOCC ORAI1 at the cell membrane (Barr et al., 2009; Oh-hora, 2009; Vig and Kinet, 2009; Zhou et al., 2010). The sustained entry of Ca2+ into the cell through ORAI channels is responsible for the activation of calcineurin, resulting in the nuclear translocation of the transcription factor NFAT as well as the activation of calmodulin kinase-dependent pathways. The severe immunodeficiency observed in mice or Humans with defective STIM1 (Picard et al., 2009) and ORAI1 testifies the importance of these molecules in T-cell biology (Partiseti et al., 1994; Feske et al., 2006, 2012).
However, this scheme accounts neither for the heterogeneity of calcium responses induced by TCR stimulation depending upon the state of activation and differentiation of T-lymphocytes nor for the possible implication of other calcium channels at the T-cell membrane.
Cav1 Channels in T-Cells
An increasing line of evidence pleads for the involvement of Cav1 channels in T-lymphocyte biology (Kotturi et al., 2003, 2006; Stokes et al., 2004; Kotturi and Jefferies, 2005; Badou et al., 2006; Matza et al., 2008, 2009; Jha et al., 2009). Thus, the analysis of mice with ablation of the auxiliary subunits Cavβ3 (Jha et al., 2009) and Cavβ4 (Badou et al., 2006) and more recently of mice deleted for Cav1.4 (Omilusik et al., 2011) reveals the role of Cav1 channels in T-lymphocyte survival and activation. Cav1.4 was recently described as interacting with Vav and lck src kinase (Jha et al., 2009), which could result in Ca2+ entry required for maintaining [Ca]i and the ER Ca2+ stores (Figure 1A). As a consequence, Cav1.4 defective T-cells are more prone to apoptosis and have a reduced homeostatic proliferation capacity. Naïve Cav1.4 null T-cells also harbor defective calcium influx upon TCR stimulation suggesting the involvement of these channels in TCR-dependent Ca2+ signaling (Omilusik et al., 2011). Interestingly, the human Timothy syndrome which is associated to mutation in gene encoding for Cav1.2 resulting in excessive Ca2+ entry is associated in most patients with an immunosuppression suggesting a role for Cav1.2 channels in immune functions (Liao and Soong, 2010). It will be interesting to determine if and how the Cav1.2 mutation affects immune cell functions.
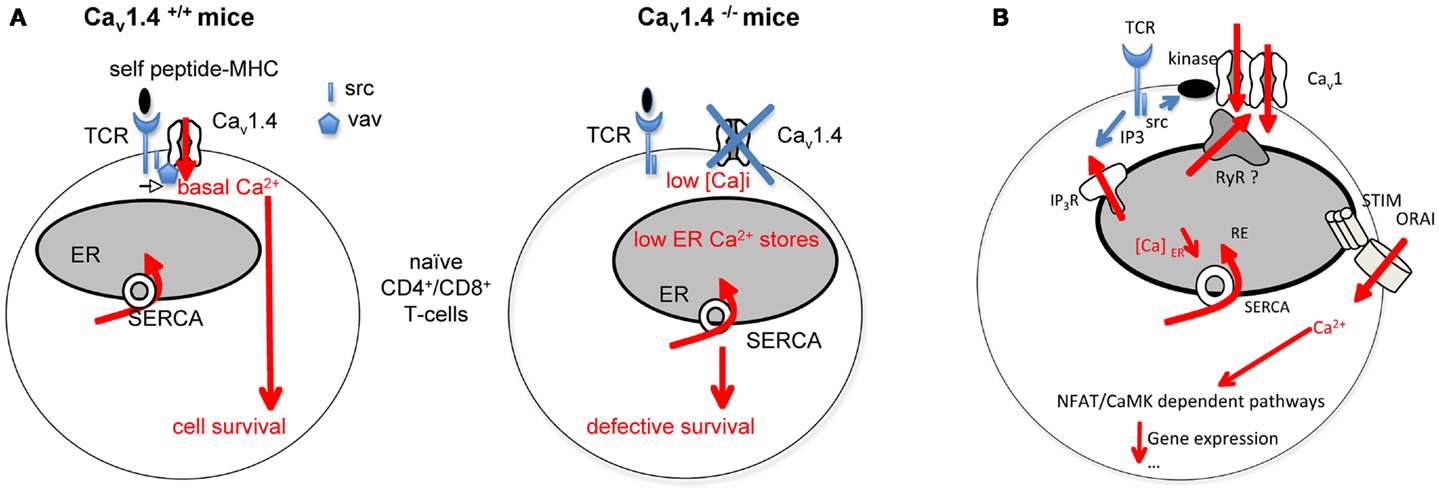
Figure 1. Role of Cav1 channels in T-cell Ca2+ responses and functions. (A) Cav1.4 is found localized in preformed complexes containing src kinase and Vav. Self peptide-MHC interactions with the TCR, independently of the antigen specificity would induce a survival signal in naïve T-cells. This signal requires some calcium entry depending upon the Cav1.4 containing complex. Cav1.4 would be also important for maintaining correct endoplasmic reticulum (ER) Ca2+ stores. Cav1.4 null T-cells exhibit defective calcium homeostasis associated with defective survival. (B) The scheme depicts how we assume Cav1.2 channel regulation in Th2-cells. TCR activation would lead to src and PKC enzyme activation. Possible PKC-Cav1.2 interactions would induce Cav1.2 channel opening. Cav1.2 channels can interact with Ryanodine receptors (RyR) at the membrane of the endoplasmic reticulum (ER). These channels release Ca2+ from the ER into the cytosol. The depletion of ER Ca2+ stores would allow conformational changes of the Ca2+ sensor STIM and the subsequent activation of ORAI channels. IP3R, IP3 receptors; MHC, major histocompatibility complex; SERCA, sarco/endoplasmic reticulum Ca2+ ATPase; TCR, T-cell receptor.
Cav1 Channels in Th2-Cells
Depending upon the strength of TCR stimulation, the chronicity of antigenic exposure, the route of antigen administration, and the cytokines present during T-cell differentiation, CD4+ T-cells can differentiate into Th1, Th2, and Th17-cells that produce distinct sets of cytokines and exert different functions. In addition, these subpopulations express lineage specific and common transcription factors. Th1-cells produce gamma interferon (IFN-γ) and are implicated in the eradication of intracellular pathogens, viruses; Th2-cells produce interleukin (IL)-4, IL-5, and IL-13, contribute to the elimination of parasites and Th17, producing IL-17 and IL-22, participate in the elimination of extracellular pathogens as fungi. These subsets may also be pathogenic. Th1 and Th17 can promote autoimmune diseases, whereas Th2-cells can cause allergic diseases. Especially, Th2-cells can induce all the cardinal features of allergic asthma through all the cytokines they produce.
The calcium signature differs between Th1, Th2, and Th17-cells suggesting that components regulating calcium entry may differ between each T-cell subsets. The resting [Ca]i is the lowest in Th1, the highest in Th2, and intermediate in Th17. Conversely the TCR-dependent increase in [Ca]i is the highest in Th1, intermediate in Th17, and less important and sustained in Th2-cells, which could be related to the differential dependence of calcium-regulated transcription factors as NFAT, NFkB, and CREB (Dolmetsch et al., 1997) in the different T-cell subsets. It was suggested that these differences could result from lower equipment in pumps or in potassium channels required for maintaining the electrochemical driving force that supports calcium entry in Th2-cells, compared with the other T-cell subsets (Fanger et al., 2000). Our group identified voltage-dependent calcium Cav1.2 and Cav1.3 channels as selectively overexpressed in Th2-cells (Badou et al., 1997; Savignac et al., 2001, 2004; Gomes et al., 2006; Djata Cabral et al., 2010). Knocking down Cav1.2 and/or Cav1.3 α1 subunits by transfection with specific antisense oligodeoxynucleotides (Cav1AS) did not affect the proliferative response of Th2-cells but strongly impaired the TCR-dependent increase in [Ca]i and Th2 cytokine production without any effect on Th1-cells. We have then injected OVA-specific DO11.10 transgenic Th2-cells transfected or not with Cav1.2 plus Cav1.3 AS into BALB/c mice that were given intranasal OVA. Th2 Cav1AS localized into the lungs and proliferated as well as control Th2-cells. However they were unable to support a sustained inflammation characteristic of asthma. On the contrary, Th1 Cav1AS were as effective as control Th1-cells in the induction of inflammation. Antisense oligodeoxynucleotides were shown to remain localized into the airways when given by inhalation (Tanaka and Nyce, 2001). A mixture of Cav1.2 and Cav1.3 AS given by this route protected mice against the development of asthma (Djata Cabral et al., 2010), suggesting that these channels may represent an interesting new approach in the treatment of allergic diseases. Interestingly TCR stimulation is associated with polarized signaling as shown by an enrichment of Ca2+ (Lioudyno et al., 2008) and other ionic channels near the immune synapse, an area where the T-cell membrane contacts the antigen-presenting cell (Cahalan and Chandy, 2009). It will be important to assess whether Cav1 channels traffic at the immune synapse upon TCR activation, in which areas and to identify the partners with which they associate.
Another important feature of the regulation of [Ca]i will be the understanding of ionic channels as potassium and non-specific cationic channels in Cav1 channel opening. Indeed K+ channels comprising voltage and Ca2+-activated channels maintain the electrochemical gradient of Ca2+ required for Ca2+ entry and tend to hyperpolarize the cell membrane favoring Cav1 channel inactivation. Conversely, TCR-dependent TRPM4 activation induces Na+ entry described as limiting the Ca2+ entry and permitting Ca2+ oscillations (Launay et al., 2004). It would be interesting to determine if TRPM4 can favor Cav1 channel opening.
Regulation of Cav1 Channels in Lymphocytes
All the authors showing the presence of Cav1 channels in T-lymphocytes agree that these channels are not voltage-operated in physiological conditions. Therefore, how they are regulated in T-lymphocytes must be explained. Differences in the sequence/structure of Cav1 channels in T-cells relative to the canonical forms in excitable cells have been reported and could provide an account for the absence of voltage sensitivity in T-cells (Stokes et al., 2004; Kotturi and Jefferies, 2005). However, the authors must demonstrate that the truncated Cav1 channels are true Ca2+ channels. The analysis of T-lymphocytes from Cav1.4 null and sufficient mice reveals the presence of voltage-gated currents in control cells, which were undetectable in null T-cells (Omilusik et al., 2011). Noticeably, the authors used peculiar conditions for their patch clamp experiments and mentioned that normal T-cells were pre-activated before recordings. This suggests that TCR activation could induce or enhance the number of Cav1 channels at the cell membrane. The TCR-induced opening of Cav1 channels may alternatively be explained by the existence of partners able to drive channel recruitment via two non-exclusive pathways: (i) post-translational modifications regulating Cav1.2 channel availability at the cell membrane without voltage change and/or (ii) modification of channel trafficking, targeting, recycling, or degradation induced by TCR stimulation. We demonstrate that the sequence of Cav1 channels in Th2-lymphocytes is similar to neuronal forms of the channel. However, Cav1 channels do not seem to be voltage-operated in Th2-lymphocytes. We have already demonstrated that TCR-induced L-type dependent calcium influx is at least sensitive to Src kinases and the PKC in an IL-4 producing T-cell hybridoma (Savignac et al., 2001). In fact, the application of PP2, an inhibitor of Src kinases or an inhibitor of PKCα on Th2-cells suppresses the Cav1 channel-dependent Ca2+ influx. In addition, we showed that PKC activator induced an entry of Ca2+, suppressed by an antagonist of Cav1 channels (Savignac et al., 2001). These data mean that kinase activation is implicated in Cav1 dependent currents (Figure 1B). PKCα is a good candidate since Cav1.2 channels can be constitutively activated at the resting potential of smooth arteriolar cells due to their interaction with PKCα (Navedo et al., 2005; Santana and Navedo, 2010). Ryanodine receptors (RyR) are channels releasing Ca2+ from the ER into the cytosol. They are activated directly or not by Cav1 channels. It is not known if Cav1 channels interact with RyR in T-lymphocytes, inducing ER Ca2+ depletion and the activation of the STIM-ORAI pathway (Figure 1B).
The pending questions deal with how Cav1 channels work in lymphocytes and their integration with other channels to generate a specific calcium signature. The relationships between STIM, ORAI, and Cav1 are puzzling. STIM was shown as a negative regulator of Cav1 signaling (Park et al., 2010). The possibility of a checkpoint controlling ORAI versus Cav1 channel-dependent calcium responses merits to be explored.
Acknowledgments
The INSERM, the ITMO IHP, and the association “111 des Arts” supported our work.
References
Badou, A., Jha, M. K., Matza, D., Mehal, W. Z., Freichel, M., Flockerzi, V., et al. (2006). Critical role for the beta regulatory subunits of Cav channels in T lymphocyte function. Proc. Natl. Acad. Sci. U.S.A. 103, 15529–15534. doi:10.1073/pnas.0607262103
Badou, A., Savignac, M., Moreau, M., Leclerc, C., Pasquier, R., Druet, P., et al. (1997). HgCl2-induced IL-4 gene expression in T cells involves protein kinase C-dependent calcium influx through L-type calcium channels. J. Biol. Chem. 272, 32411–32418. doi:10.1074/jbc.272.51.32411
Barr, V. A., Bernot, K. M., Shaffer, M. H., Burkhardt, J. K., and Samelson, L. E. (2009). Formation of STIM and Orai complexes: puncta and distal caps. Immunol. Rev. 231, 148–159. doi:10.1111/j.1600-065X.2009.00812.x
Cahalan, M. D., and Chandy, K. G. (2009). The functional network of ion channels in T lymphocytes. Immunol. Rev. 231, 59–87. doi:10.1111/j.1600-065X.2009.00816.x
Djata Cabral, M., Paulet, P. E., Robert, B., Gomes, M. L., Renoud, M., and Savignac, C. (2010). Knocking-down Cav1 calcium channels implicated in Th2-cell activation prevents experimental asthma. Am. J. Respir. Crit. Care Med. 181, 1310–1317. doi:10.1164/rccm.200907-1166OC
Dolmetsch, R. E., Lewis, R. S., Goodnow, C. C., and Healy, J. I. (1997). Differential activation of transcription factors induced by Ca2+ response amplitude and duration. Nature 386, 855–858. doi:10.1038/386855a0
Fanger, C. M., Neben, A. L., and Cahalan, M. D. (2000). Differential Ca2+ influx, KCa channel activity, and Ca2+ clearance distinguish Th1 and Th2 lymphocytes. J. Immunol. 164, 1153–1160.
Feske, S., Giltnane, J., Dolmetsch, R., Staudt, L. M., and Rao, A. (2001). Gene regulation mediated by calcium signals in T lymphocytes. Nat. Immunol. 2, 316–324. doi:10.1038/86318
Feske, S., Gwack, Y., Prakriya, M., Srikanth, S., Puppel, S. H., Tanasa, B., et al. (2006). A mutation in Orai1 causes immune deficiency by abrogating CRAC channel function. Nature 441, 179–185. doi:10.1038/nature04702
Feske, S., Skolnik, E. Y., and Prakriya, M. (2012). Ion channels and transporters in lymphocyte function and immunity. Nat. Rev. Immunol. 12, 532–547. doi:10.1038/nri3233
Gomes, B., Savignac, M., Cabral, M. D., Paulet, P., Moreau, M., Leclerc, C., et al. (2006). The cGMP/protein kinase G pathway contributes to dihydropyridine-sensitive calcium response and cytokine production in TH2 lymphocytes. J. Biol. Chem. 281, 12421–12427. doi:10.1074/jbc.M510653200
Jha, M. K., Badou, A., Meissner, M., McRory, J. E., Freichel, M., Flockerzi, V., et al. (2009). Defective survival of naive CD8+ T lymphocytes in the absence of the beta3 regulatory subunit of voltage-gated calcium channels. Nat. Immunol. 10, 1275–1282. doi:10.1038/ni.1793
Kotturi, M. F., Carlow, D. A., Lee, J. C., Ziltener, H. J., and Jefferies, W. A. (2003). Identification and functional characterization of voltage-dependent calcium channels in T lymphocytes. J. Biol. Chem. 278, 46949–46960. doi:10.1074/jbc.M309268200
Kotturi, M. F., Hunt, S. V., and Jefferies, W. A. (2006). Roles of CRAC and Ca(V)-like channels in T cells: more than one gatekeeper? Trends Pharmacol. Sci. 27, 360–367. doi:10.1016/j.tips.2006.05.007
Kotturi, M. F., and Jefferies, W. A. (2005). Molecular characterization of L-type calcium channel splice variants expressed in human T lymphocytes. Mol. Immunol. 42, 1461–1474. doi:10.1016/j.molimm.2005.01.014
Launay, P., Cheng, H., Srivatsan, S., Penner, R., Fleig, A., and Kinet, J. P. (2004). TRPM4 regulates calcium oscillations after T cell activation. Science 306, 1374–1377. doi:10.1126/science.1098845
Liao, P., and Soong, T. W. (2010). CaV1.2 channelopathies: from arrhythmias to autism, bipolar disorder, and immunodeficiency. Pflugers Arch. 460, 353–359. doi:10.1007/s00424-009-0753-0
Lioudyno, M. I., Kozak, J. A., Penna, A., Safrina, O., Zhang, S. L., Sen, D., et al. (2008). Orai1 and STIM1 move to the immunological synapse and are up-regulated during T cell activation. Proc. Natl. Acad. Sci. U.S.A. 105, 2011–2016. doi:10.1073/pnas.0706122105
Lipscombe, D., Helton, T. D., and Xu, W. (2004). L-type calcium channels: the low down. J. Neurophysiol. 92, 2633–2641. doi:10.1152/jn.00486.2004
Luo, X., Berdymammet, H., Jiang, N., Wang, Z. V., Tandan, S., Rakalin, A., et al. (2012). STIM1-dependent store-operated Ca2+ entry is required for pathological cardiac hypertrophy. J. Mol. Cell. Cardiol. 52, 136–147. doi:10.1016/j.yjmcc.2011.11.003
Matza, D., Badou, A., Jha, M. K., Willinger, T., Antov, A., Sanjabi, S., et al. (2009). Requirement for AHNAK1-mediated calcium signaling during T lymphocyte cytolysis. Proc. Natl. Acad. Sci. U.S.A. 106, 9785–9790. doi:10.1073/pnas.0902844106
Matza, D., Badou, A., Kobayashi, K. S., Goldsmith-Pestana, K., Masuda, Y., Komuro, A., et al. (2008). A scaffold protein, AHNAK1, is required for calcium signaling during T cell activation. Immunity 28, 64–74. doi:10.1016/j.immuni.2007.11.020
McRory, J. E., Hamid, J., Doering, C. J., Garcia, E., Parker, R., Hamming, K., et al. (2004). The CACNA1F gene encodes an L-type calcium channel with unique biophysical properties and tissue distribution. J. Neurosci. 24, 1707–1718. doi:10.1523/JNEUROSCI.4846-03.2004
Navedo, M. F., Amberg, G. C., Votaw, V. S., and Santana, L. F. (2005). Constitutively active L-type Ca2+ channels. Proc. Natl. Acad. Sci. U.S.A. 102, 11112–11117. doi:10.1073/pnas.0500360102
Oh-hora, M. (2009). Calcium signaling in the development and function of T-lineage cells. Immunol. Rev. 231, 210–224. doi:10.1111/j.1600-065X.2009.00819.x
Omilusik, K., Priatel, J. J., Chen, X., Wang, Y. T., Xu, H., Choi, K. B., et al. (2011). The Ca(v)1.4 calcium channel is a critical regulator of T cell receptor signaling and naive T cell homeostasis. Immunity 35, 349–360. doi:10.1016/j.immuni.2011.07.011
Park, C. Y., Shcheglovitov, A., and Dolmetsch, R. (2010). The CRAC channel activator STIM1 binds and inhibits L-type voltage-gated calcium channels. Science 330, 101–105. doi:10.1126/science.1191027
Partiseti, M., Le Deist, F., Hivroz, C., Fischer, A., Korn, H., and Choquet, D. (1994). The calcium current activated by T cell receptor and store depletion in human lymphocytes is absent in a primary immunodeficiency. J. Biol. Chem. 269, 32327–32335.
Picard, C., McCarl, C. A., Papolos, A., Khalil, S., Luthy, K., Hivroz, C., et al. (2009). STIM1 mutation associated with a syndrome of immunodeficiency and autoimmunity. N. Engl. J. Med. 360, 1971–1980. doi:10.1056/NEJMoa0900082
Robert, V., Triffaux, E., Savignac, M., and Pelletier, L. (2011). Calcium signalling in T-lymphocytes. Biochimie 93, 2087–2094. doi:10.1016/j.biochi.2011.06.016
Robert, V., Triffaux, E., Savignac, M., and Pelletier, L. (2013). Singularities of calcium signaling in effector T-lymphocytes. Biochim. Biophys. Acta. 1833, 1595–1602. doi:10.1016/j.bbamcr.2012.12.001
Santana, L. F., and Navedo, M. F. (2010). Natural inequalities: why some L-type Ca2+ channels work harder than others. J. Gen. Physiol. 136, 143–147. doi:10.1085/jgp.200910391
Savignac, M., Badou, A., Moreau, M., Leclerc, C., Guery, J. C., Paulet, P., et al. (2001). Protein kinase C-mediated calcium entry dependent upon dihydropyridine sensitive channels: a T cell receptor-coupled signaling pathway involved in IL-4 synthesis. FASEB J. 15, 1577–1579.
Savignac, M., Gomes, B., Gallard, A., Narbonnet, S., Moreau, M., Leclerc, C., et al. (2004). Dihydropyridine receptors are selective markers of Th2 cells and can be targeted to prevent Th2-dependent immunopathological disorders. J. Immunol. 172, 5206–5212.
Stokes, L., Gordon, J., and Grafton, G. (2004). Non-voltage-gated L-type Ca2+ channels in human T cells: pharmacology and molecular characterization of the major alpha pore-forming and auxiliary beta-subunits. J. Biol. Chem. 279, 19566–19573. doi:10.1074/jbc.M401481200
Tanaka, M., and Nyce, J. W. (2001). Respirable antisense oligonucleotides: a new drug class for respiratory disease. Respir. Res. 2, 5–9. doi:10.1186/rr153
Vig, M., and Kinet, J. P. (2009). Calcium signaling in immune cells. Nat. Immunol. 10, 21–27. doi:10.1038/ni.f.220
Citation: Pelletier L and Savignac M (2013) Ca2+ signaling in T-cell subsets with a focus on the role of Cav1 channels: possible implications in therapeutics. Front. Immunol. 4:150. doi: 10.3389/fimmu.2013.00150
Received: 28 May 2013; Accepted: 03 June 2013;
Published online: 20 June 2013.
Edited by:
Gergely Toldi, Semmelweis University, HungaryCopyright: © 2013 Pelletier and Savignac. This is an open-access article distributed under the terms of the Creative Commons Attribution License, which permits use, distribution and reproduction in other forums, provided the original authors and source are credited and subject to any copyright notices concerning any third-party graphics etc.
*Correspondence:bHVjZXR0ZS5wZWxsZXRpZXJAaW5zZXJtLmZy