- 1Department of Immunology, CHU Bicêtre, Le Kremlin-Bicêtre, France
- 2INSERM U-996, Clamart, France
- 3INSERM U-1012, Le Kremlin-Bicêtre, France
T cell immunity is characterized by striking tissue specialization. Tissue-specificity imprinting starts during priming by tissue-derived migratory dendritic cells in the non-random, specialized micro-anatomical area of the draining lymph node and is influenced by constitutive and induced cues from local environment. Besides tissue-specific effectors, memory cells also exhibit a tissue-specificity. Long-lived tissue-resident memory T cells likely play a considerable role in preventing pathogen invasion. Understanding of the mechanisms of tissue specialization of T cells is of major importance for the design of optimal vaccination strategies and therapeutic interventions in tissue/organ-specific inflammatory diseases. The present review summarizes our current knowledge and hypothesis about tissue-specificity imprinting and tissue residency of T cells.
Generation of long-lasting systemic and tissue-specific memory T cell responses is a fundamental characteristic of adaptive immunity. According to the current paradigm, naturally pre-programed and secondary lymphoid tissue (SLT)-oriented trafficking of recirculating naïve T cells switches dramatically to peripheral tissue-oriented after antigen (Ag) exposure and priming in the T cell zone environment of draining lymph node (DLN) (1). The role of peripheral tissue-derived migratory dendritic cells (DCs) in the imprinting of tissue-specific information is now well documented (2–5). Thus, DLNs can be considered as the body’s “central processing units” incorporating tissue-specific information into generated adaptive T cell responses. This response is generated from two recirculating precursors characterized by constitutive LN-tropism: naive and central memory (TCM) T cells. Indeed, both populations share high expression of the LN-homing molecules (CCR7 and CD62L) and recirculation marker S1P1 and generate tissue-specific short-lived effector cells (SLECs), the major pool of terminally differentiated T cells highly active in pathogen clearance. Although the role of CD8α+ resident DCs in generation of tissue-specific SLECs in some types of infections has been documented (6), peripheral tissue-derived migratory DCs (CD207+CD103+ and CD11b+CD207−CD103−dermal DCs and CD207+CD103− Langerhans cells, LCs) likely represent major players involved in processing and transfer of skin-specific information during priming and cross-priming processes (6, 7).
Recent multiparameter 3D imaging approach revealed striking differences in migratory DCs-subset distribution within discrete micro-anatomical compartments of skin DLN with preferential localization of CD11b+ dermal DCs within interfollicular and outer paracortex area close to B cell zone and preferential positioning of dermal CD207+CD103+ DCs and LCs within deeper T cell zone (8). This non-random spatial segregation and positioning of tissue-derived DCs subpopulations in DLN mirrors controlled trafficking and positioning of naive and TCM cell subsets following priming and during recall T cell response, respectively. In contrast to steady state where naive CD8+ T cells localize mostly in the deep T cell area, recent observations point to the crucial role of subfollicular region previously known as cortical ridge and interfollicular area (IFA) as preferential sites of both, positioning following CD8+ T cell priming and constitutive prepositioning of CD8+ TCM cells (9, 10). Notably, in both cases, tissue-specific SLECs are generated, which suggest a striking spatial overlap between local DLN functional microdomain involved in generation of SLECs progeny regardless of the type of adaptive immune response (primary or secondary). CXCL9-CXCR3 receptor chemotactic axis has been identified to be a key mechanism regulating repositioning of TCM to cortical ridge zone and IFA and CXCR3 has been proposed to be a factor involved in early programing of CD8+ T cells differentiation into SLECs since CXCR3−/− CD8+ T cells preferentially differentiate into memory precursor cells (MPECs) (9, 11, 12). Importantly, CXCR3 is a functional marker shared by recently activated naïve T cells, SLECs, and different subsets of memory T cells including the recently identified memory stem cell subset (TSCM) (13). Whether re-stimulation of TSCM cells also takes place in the cortical ridge zone and IFA remains to be established.
Tissue-specific imprinting during T cell priming and subsequent T cell differentiation into tissue-specific SLECs is thought to involve induction of a set of surface homing molecules which combinatorial usage generates lymphocyte’s tissue-specific zip code. Only a few molecular mechanisms involved in the peripheral tissue tropism of SLECs have so far been identified. Small-intestine tropism of SLECs is imprinted by gut-associated DCs in an all-trans retinoic acid (RA)-dependent manner. This involves induction of a small intestine-specific zip code consisting of strong integrin α4β7 and chemokine receptor CCR9 expression (4, 14). Likewise, skin-derived DCs induce chemokine receptor CCR10 expression on T cells in a 1,25(OH)2D3-dependent manner and thereby program generated SLECs to home to the skin (15). The skin-specific zip code is characterized by concomitant and sequential usage of E-/P-selectin ligands (CLA), CCR4, CCR10, CCR8, CCR6, αMβ2 (CD11b/CD18), and α4β1 (CD49d/CD29), among other homing molecules (16). De novo expression of E-selectin ligands on skin-tropic T cells requires activation-induced expression of the α(1,3)fucosyltransferases FucT-IV and FucT-VII glycosylation enzymes (17, 18). Of note, expression of skin-tropic molecules on lymphocytes is inhibited by RA, while 1,25(OH)2D3 negatively regulates RA-controlled expression of α4β7 and CCR9 (14). The molecular mechanisms that control homing of effector T cells to other tissues remain largely unknown except of the recently proposed role of CLEVER-1/stabilin-1 and VAP-1 in liver-specific recruitment of Foxp3+ Tregs (19).
The lymphocyte tissue-specific zip codes in turn recognize yet poorly defined vascular zip codes, a combinatorial set of endothelial counter receptors for lymphocyte’s zip codes expressed by post-capillary venules. Importantly, both types of zip code may modify through induction of new surface markers and down regulation of others, thus adapting cell trafficking to the type of immune response and to dynamically changing inflammatory conditions. The last notion is indeed supported by substantial heterogeneity and dynamics of microvascular endothelial phenotype described within the same tissue compartment in response to inflammatory stimuli (20). Phenotypic and functional modifications of local post-capillary endothelial cells (ECs) induced by pro-inflammatory cytokines and inflammation-related factors, likely includes: (i) rearranged expression of adhesion molecules, glycosaminoglycan (GAGs) structures, and specific glycosylation patterns of the endothelial cell surface-associated molecules, (ii) active transport, transcytosis, and presentation of inflammatory chemokines induced at sites of inflammation, at the abluminal surface of endothelial cells, (iii) synthesis of lymphocyte-activating cytokines including chemokines (21–23). Thus, although organ/tissue-specificity seems to be pre-determined in the context of DLNs, precise tissue-specific positioning of SLECs seems to be characterized by sequential modifications of the expressed zip codes which are dynamically adjusted according to the given step of cell trafficking process and local inflammatory and metabolic cues.
Despite clear evidence for the imprinting of a specific homing pattern during T cell priming, this elegant model likely has certain limitations, as several experimental studies of infectious diseases have shown wider recirculation pattern and substantial promiscuity in the trafficking programing, as well as a surprisingly flexible tissue distribution of in vivo-induced SLECs (24–26). However, large or biased homing patterns observed in some models could be due to several immune and pathogen-related factors: (i) in vivo model used, (ii) artifacts related to high number transfer of Ag-specific transgenic T cells (iii) low level of induction of broad-spectrum homing molecules such as mucosal T cells-associated marker α4β7 integrin or inflammation-related molecules (iv) immunization route (v) priming such as Ag load, priming threshold, adjuvants and costimulation, polarization, or regulatory signals, and (vi) target tissue-analyzed (more promiscuous, mucosa-associated, and more restricted skin-specific homing pattern), among others (24–29).
While the mechanisms controlling homing pattern of SLECs has been extensively investigated, less attention has been paid to the mechanisms that control the trafficking and tissue positioning of long-lived memory T cell subsets, namely peripheral tissue-resident memory (TRM) T cells. The simultaneous presence of memory T cells with different anatomical distributions (systemic and tissue/organ-specific) is likely to reflect the role of compartmentalization in the global strategy used by the immune system to ensure optimal surveillance and protection (Figure 1). In this scenario, similarly to SLECs, TEM cells do not express the LN-homing molecules CD62L and CCR7, and thus traffic preferentially to inflammatory sites of Ag encounter. However, a subset of TEM cells likely can egress from peripheral inflammatory sites to local DLN via afferent lymph, following transient expression of CCR7, and then to the bloodstream and spleen (30–32). The proportion of cells expressing CD62L within the circulating memory T cell population increases with increasing time after immunization. This could be related to the higher survival and homeostatic proliferation of TCM relative to TEM (29). TCM cells continually re-circulate between peripheral blood and SLT and account for the bulk of long-lasting systemic immune memory and are the most important precursors of tissue-specific TEM pool during recall response. However, they are only moderately capable of homing to sites of inflammation, and their ability to control localized infections within peripheral tissues is thus very limited (33–36).
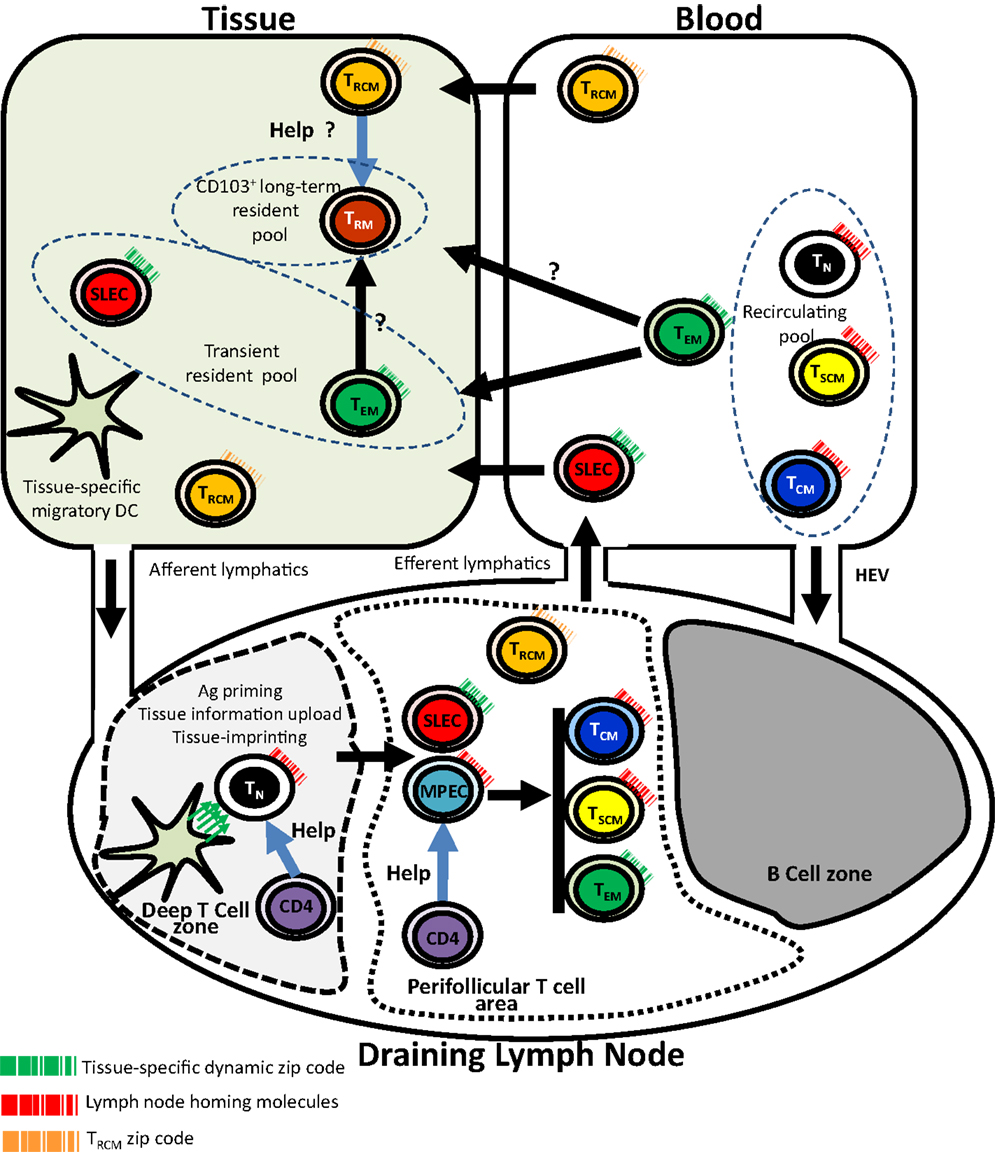
Figure 1. Tissue-specific imprinting, trafficking, and tissue residency of effector and memory CD8 T cells. In this model, tissue-derived migratory DC imprints tissue-specific homing pattern (symbolized by the green arrows) in naive T cell during priming in the context of the deep T cell zone of the draining lymph node. Primed T cells including SLECs and MPECs relocalize into perifollicular T cell area. While SLECs migrate into tissues through the blood, MPECs complete their differentiation into memory subsets. Recirculating (TN, TCM, TSCM), transient (SLECs, TEM), and long-term (TRM) non-recirculating CD8 T cell pools are shown. Unusual trafficking pattern of CD4+ TRCM is also shown. CD4 helping signals for CD8 memory generation and possibly for TRM maintenance are also shown. TN, naive CD8 T cell; SLEC, short-lived effector T Cell; MPEC, memory precursor CD8 T cell; TSCM, stem cell memory CD8 T cell; TCM, central memory CD8 T cell; TEM, effector memory CD8 T cell; TRM, resident memory CD8 T cell; TRCM, recirculating memory CD4 T cell; HEV, high endothelial venules.
Tissue-resident memory cells in contrast are non-recirculating long-lived pathogen-specific resident memory T cells permanently associated with body surfaces (skin, intestine, lung, vaginal mucosa) and with organs such as the brain, kidney, and pancreas, thus providing global organ/area-targeted protection (34, 37, 38). Skin TRM cells have potent effector capacities and appear be superior to recirculating TCM cells at providing rapid long-term protection against cutaneous re-infection (34). Several data indicate that local environmental cues are required for TRM cell maintenance within epithelia, as described for LC (38–41). According to this scenario, locally produced TGFβ could instruct specific precursors within the TEM pool to follow a TRM fate. Some TRM populations have been described to constitutively express CD8αα homodimer (42). A possible hypothesis is that TRM precursors require CD8αα and the interaction with its high affinity ligand(s) such as thymic leukemia antigen (TL) for their differentiation and survival, as described for intestinal intraepithelial lymphocytes (IELs) (43).
Currently, there are no data supporting the hypothesis that the TRM pool results from seeding by blood-borne TCM-derived progeny. In contrast, it has been shown that TEM generated following skin infection accumulate as TRM in a organ-specific manner both, at site of infection and at distant sites (34). This suggests that the TEM stage is the link between migratory DCS that harbor tissue-specific information and TRM cells. This implies that the tissue-specific information is conserved and transmitted along this TEM–TRM differentiation pathway. However, TRM cells differ markedly from blood TEM pool and the exact relations between TEM and TRM is yet to be determined, especially whether TRM may be viewed only as a resident form of blood-derived TEM (38, 44–46). Commonly observed signature of the barrier tissue-associated TRM cell population include strong expression of CD103, E-/P-selectin ligands, CD44, CD69 (a negative regulator of recirculation marker S1P1) (47), and CD49a/VLA-1. This phenotype is partially shared with other E-cadherin-dependent epithelia-resident cells such as dendritic epidermal γδ T cells (DETC) and IELs (35, 48). High level of CD103 expression likely represents a key marker of permanent barrier tissue residency of different types of TRM subsets (42, 49). The phenotype of TRM cells is probably much more heterogeneous, and future studies will likely identify new molecules involved in TRM cell positioning and maintenance within barrier as well as non-barrier peripheral tissues. Several observations suggest that TRM cells can persist long-term without any external input (33, 44, 45, 49), therefore providing prolonged protection against pathogens. However, the observations of numerous cases of progressive multifocal leukoencephalopathy (a devastating demyelinating disease due to JC virus reactivation in the brain) in multiple sclerosis patients treated with natalizumab (50), a therapeutic monoclonal antibody directed against the cell adhesion molecule α4-integrin that blocks T cell trafficking through the blood brain barrier, raises question about the long-term maintenance of efficient brain-resident T cell memory without cell input from peripheral blood.
A point of interest is the recent demonstration in the skin of recirculating memory (TRCM) CD4 T cells which phenotypically differ from TEM, as they are CCR7pos, CD62Lint, CD103± in addition to the expression of the peripheral homing receptor E-selectin ligand (51). This unusual migration pattern is partially shared by Foxp3+ Tregs that migrate from skin to draining LNs via afferent lymphatics (52). Following activation, TRCM expressed CD40L and produced IL-2 (51). Numerous reports have shown the requirement of helping signals from CD4 T cell subsets for the generation, maintenance, and reactivation of efficient systemic CD8 memory (53–57). Whether help from CD4 TRCM is required for the maintenance of CD8 TRM is a point that deserves further investigations. Such a requirement of help from recirculating CD4 T cells may provide an explanation for the deleterious role of natalizumab or efalizumab (another therapeutic monoclonal antibody that targets the member of the integrin family CD11a) (50) on brain-resident CD8 memory, despite the intrinsic long-term survival of CD8 TRM cells. Another question of interest is whether there is a CD8 counterpart of CD4 TRCM cells. Figure 1 summarizes key points of our current understanding of trafficking and tissue residency of effector and memory T cell subsets.
We are still at the early beginning of the story and many questions remain unanswered about the mechanisms of tissue-specific imprinting of effector and memory T cells and the maintenance of resident T cell memory as well as the lineage relationships between TRM and blood memory T cells. Unraveling those mechanisms is of major importance for the design of new vaccination strategies capable of inducing strong cell-mediated organ/tissue-specific immunity and protection. Molecules involved in tissue/organ-specific positioning of memory T cells might also represent interesting therapeutic targets for a variety of tissue/organ-specific inflammatory diseases.
Conflict of Interest Statement
The authors declare that the research was conducted in the absence of any commercial or financial relationships that could be construed as a potential conflict of interest.
References
1. Masopust D, Vezys V, Marzo AL, Lefrancois L. Preferential localization of effector memory cells in nonlymphoid tissue. Science (2001) 291(5512):2413–7. doi:10.1126/science.1058867
2. Dudda JC, Lembo A, Bachtanian E, Huehn J, Siewert C, Hamann A, et al. Dendritic cells govern induction and reprogramming of polarized tissue-selective homing receptor patterns of T cells: important roles for soluble factors and tissue microenvironments. Eur J Immunol (2005) 35(4):1056–65. doi:10.1002/eji.200425817
3. Johansson-Lindbom B, Svensson M, Pabst O, Palmqvist C, Marquez G, Forster R, et al. Functional specialization of gut CD103+ dendritic cells in the regulation of tissue-selective T cell homing. J Exp Med (2005) 202(8):1063–73. doi:10.1084/jem.20051100
4. Mora JR, Bono MR, Manjunath N, Weninger W, Cavanagh LL, Rosemblatt M, et al. Selective imprinting of gut-homing T cells by Peyer’s patch dendritic cells. Nature (2003) 424(6944):88–93. doi:10.1038/nature01726
5. Mora JR, Cheng G, Picarella D, Briskin M, Buchanan N, von Andrian UH. Reciprocal and dynamic control of CD8 T cell homing by dendritic cells from skin- and gut-associated lymphoid tissues. J Exp Med (2005) 201(2):303–16. doi:10.1084/jem.20041645
6. Allan RS, Waithman J, Bedoui S, Jones CM, Villadangos JA, Zhan Y, et al. Migratory dendritic cells transfer antigen to a lymph node-resident dendritic cell population for efficient CTL priming. Immunity (2006) 25(1):153–62. doi:10.1016/j.immuni.2006.04.017
7. Henri S, Poulin LF, Tamoutounour S, Ardouin L, Guilliams M, de Bovis B, et al. CD207+ CD103+ dermal dendritic cells cross-present keratinocyte-derived antigens irrespective of the presence of Langerhans cells. J Exp Med (2009) 207(1):189–206. doi:10.1084/jem.20091964
8. Gerner MY, Kastenmuller W, Ifrim I, Kabat J, Germain RN. Histo-cytometry: a method for highly multiplex quantitative tissue imaging analysis applied to dendritic cell subset microanatomy in lymph nodes. Immunity (2012) 37(2):364–76. doi:10.1016/j.immuni.2012.07.011
9. Kastenmuller W, Brandes M, Wang Z, Herz J, Egen JG, Germain RN. Peripheral prepositioning and local CXCL9 chemokine-mediated guidance orchestrate rapid memory CD8+ T cell responses in the lymph node. Immunity (2013) 38(3):502–13. doi:10.1016/j.immuni.2012.11.012
10. Sung JH, Zhang H, Moseman EA, Alvarez D, Iannacone M, Henrickson SE, et al. Chemokine guidance of central memory T cells is critical for antiviral recall responses in lymph nodes. Cell (2012) 150(6):1249–63. doi:10.1016/j.cell.2012.08.015
11. Hu JK, Kagari T, Clingan JM, Matloubian M. Expression of chemokine receptor CXCR3 on T cells affects the balance between effector and memory CD8 T-cell generation. Proc Natl Acad Sci U S A (2011) 108(21):E118–27. doi:10.1073/pnas.1101881108
12. Kurachi M, Kurachi J, Suenaga F, Tsukui T, Abe J, Ueha S, et al. Chemokine receptor CXCR3 facilitates CD8(+) T cell differentiation into short-lived effector cells leading to memory degeneration. J Exp Med (2011) 208(8):1605–20. doi:10.1084/jem.20102101
13. Gattinoni L, Lugli E, Ji Y, Pos Z, Paulos CM, Quigley MF, et al. A human memory T cell subset with stem cell-like properties. Nat Med (2011) 17(10):1290–7. doi:10.1038/nm.2446
14. Iwata M, Hirakiyama A, Eshima Y, Kagechika H, Kato C, Song SY. Retinoic acid imprints gut-homing specificity on T cells. Immunity (2004) 21(4):527–38. doi:10.1016/j.immuni.2004.08.011
15. Sigmundsdottir H, Pan J, Debes GF, Alt C, Habtezion A, Soler D, et al. DCs metabolize sunlight-induced vitamin D3 to “program” T cell attraction to the epidermal chemokine CCL27. Nat Immunol (2007) 8(3):285–93. doi:10.1038/ni1433
16. Reiss Y, Proudfoot AE, Power CA, Campbell JJ, Butcher EC. CC chemokine receptor (CCR)4 and the CCR10 ligand cutaneous T cell-attracting chemokine (CTACK) in lymphocyte trafficking to inflamed skin. J Exp Med (2001) 194(10):1541–7. doi:10.1084/jem.194.10.1541
17. Fuhlbrigge RC, Kieffer JD, Armerding D, Kupper TS. Cutaneous lymphocyte antigen is a specialized form of PSGL-1 expressed on skin-homing T cells. Nature (1997) 389(6654):978–81. doi:10.1038/40166
18. Weninger W, Ulfman LH, Cheng G, Souchkova N, Quackenbush EJ, Lowe JB, et al. Specialized contributions by alpha(1,3)-fucosyltransferase-IV and FucT-VII during leukocyte rolling in dermal microvessels. Immunity (2000) 12(6):665–76. doi:10.1016/S1074-7613(00)80217-4
19. Shetty S, Weston CJ, Oo YH, Westerlund N, Stamataki Z, Youster J, et al. Common lymphatic endothelial and vascular endothelial receptor-1 mediates the transmigration of regulatory T cells across human hepatic sinusoidal endothelium. J Immunol (2011) 186(7):4147–55. doi:10.4049/jimmunol.1002961
20. Petzelbauer P, Bender JR, Wilson J, Pober JS. Heterogeneity of dermal microvascular endothelial cell antigen expression and cytokine responsiveness in situ and in cell culture. J Immunol (1993) 151(9):5062–72.
21. Carter NM, Ali S, Kirby JA. Endothelial inflammation: the role of differential expression of N-deacetylase/N-sulphotransferase enzymes in alteration of the immunological properties of heparan sulphate. J Cell Sci (2003) 116(Pt 17):3591–600. doi:10.1242/jcs.00662
22. Palframan RT, Jung S, Cheng G, Weninger W, Luo Y, Dorf M, et al. Inflammatory chemokine transport and presentation in HEV: a remote control mechanism for monocyte recruitment to lymph nodes in inflamed tissues. J Exp Med (2001) 194(9):1361–73. doi:10.1084/jem.194.9.1361
23. Taylor KR, Gallo RL. Glycosaminoglycans and their proteoglycans: host-associated molecular patterns for initiation and modulation of inflammation. FASEB J (2006) 20(1):9–22. doi:10.1096/fj.05-4682rev
24. Liu L, Fuhlbrigge RC, Karibian K, Tian T, Kupper TS. Dynamic programming of CD8+ T cell trafficking after live viral immunization. Immunity (2006) 25(3):511–20. doi:10.1016/j.immuni.2006.06.019
25. Masopust D, Vezys V, Usherwood EJ, Cauley LS, Olson S, Marzo AL, et al. Activated primary and memory CD8 T cells migrate to nonlymphoid tissues regardless of site of activation or tissue of origin. J Immunol (2004) 172(8):4875–82.
26. Stevceva L, Alvarez X, Lackner AA, Tryniszewska E, Kelsall B, Nacsa J, et al. Both mucosal and systemic routes of immunization with the live, attenuated NYVAC/simian immunodeficiency virus SIV(gpe) recombinant vaccine result in gag-specific CD8(+) T-cell responses in mucosal tissues of macaques. J Virol (2002) 76(22):11659–76. doi:10.1128/JVI.76.22.11659-11676.2002
27. Badovinac VP, Haring JS, Harty JT. Initial T cell receptor transgenic cell precursor frequency dictates critical aspects of the CD8(+) T cell response to infection. Immunity (2007) 26(6):827–41. doi:10.1016/j.immuni.2007.04.013
28. Marzo AL, Klonowski KD, Le Bon A, Borrow P, Tough DF, Lefrancois L. Initial T cell frequency dictates memory CD8+ T cell lineage commitment. Nat Immunol (2005) 6(8):793–9. doi:10.1038/ni1227
29. Obar JJ, Khanna KM, Lefrancois L. Endogenous naive CD8+ T cell precursor frequency regulates primary and memory responses to infection. Immunity (2008) 28(6):859–69. doi:10.1016/j.immuni.2008.04.010
30. Brown MN, Fintushel SR, Lee MH, Jennrich S, Geherin SA, Hay JB, et al. Chemoattractant receptors and lymphocyte egress from extralymphoid tissue: changing requirements during the course of inflammation. J Immunol (2010) 185(8):4873–82. doi:10.4049/jimmunol.1000676
31. Debes GF, Arnold CN, Young AJ, Krautwald S, Lipp M, Hay JB, et al. Chemokine receptor CCR7 required for T lymphocyte exit from peripheral tissues. Nat Immunol (2005) 6(9):889–94. doi:10.1038/ni1238
32. Mackay CR, Marston WL, Dudler L, Spertini O, Tedder TF, Hein WR. Tissue-specific migration pathways by phenotypically distinct subpopulations of memory T cells. Eur J Immunol (1992) 22(4):887–95. doi:10.1002/eji.1830220402
33. Gebhardt T, Whitney PG, Zaid A, Mackay LK, Brooks AG, Heath WR, et al. Different patterns of peripheral migration by memory CD4+ and CD8+ T cells. Nature (2011) 477(7363):216–9. doi:10.1038/nature10339
34. Jiang X, Clark RA, Liu L, Wagers AJ, Fuhlbrigge RC, Kupper TS. Skin infection generates non-migratory memory CD8+ T(RM) cells providing global skin immunity. Nature (2012) 483(7388):227–31. doi:10.1038/nature10851
35. Mackay LK, Stock AT, Ma JZ, Jones CM, Kent SJ, Mueller SN, et al. Long-lived epithelial immunity by tissue-resident memory T (TRM) cells in the absence of persisting local antigen presentation. Proc Natl Acad Sci U S A (2012) 109(18):7037–42. doi:10.1073/pnas.1202288109
36. Weninger W, Crowley MA, Manjunath N, von Andrian UH. Migratory properties of naive, effector, and memory CD8(+) T cells. J Exp Med (2001) 194(7):953–66. doi:10.1084/jem.194.7.953
37. Sheridan BS, Lefrancois L. Regional and mucosal memory T cells. Nat Immunol (2011) 12(6):485–91. doi:10.1038/ni.2029
38. Wakim LM, Woodward-Davis A, Bevan MJ. Memory T cells persisting within the brain after local infection show functional adaptations to their tissue of residence. Proc Natl Acad Sci U S A (2010) 107(42):17872–9. doi:10.1073/pnas.1010201107
39. El-Asady R, Yuan R, Liu K, Wang D, Gress RE, Lucas PJ, et al. TGF-{beta}-dependent CD103 expression by CD8(+) T cells promotes selective destruction of the host intestinal epithelium during graft-versus-host disease. J Exp Med (2005) 201(10):1647–57. doi:10.1084/jem.20041044
40. McCully ML, Ladell K, Hakobyan S, Mansel RE, Price DA, Moser B. Epidermis instructs skin homing receptor expression in human T cells. Blood (2012) 120(23):4591–8. doi:10.1182/blood-2012-05-433037
41. Kel JM, Girard-Madoux MJ, Reizis B, Clausen BE. TGF-beta is required to maintain the pool of immature Langerhans cells in the epidermis. J Immunol (2010) 185(6):3248–55. doi:10.4049/jimmunol.1000981
42. Zhu J, Peng T, Johnston C, Phasouk K, Kask AS, Klock A, et al. Immune surveillance by CD8alphaalpha+ skin-resident T cells in human herpes virus infection. Nature (2013) 497(7450):494–7. doi:10.1038/nature12110
43. Masopust D, Vezys V, Wherry EJ, Barber DL, Ahmed R. Cutting edge: gut microenvironment promotes differentiation of a unique memory CD8 T cell population. J Immunol (2006) 176(4):2079–83.
44. Carbone FR, Mackay LK, Heath WR, Gebhardt T. Distinct resident and recirculating memory T cell subsets in non-lymphoid tissues. Curr Opin Immunol (2013) 25(3):329–33. doi:10.1016/j.coi.2013.05.007
45. Masopust D, Choo D, Vezys V, Wherry EJ, Duraiswamy J, Akondy R, et al. Dynamic T cell migration program provides resident memory within intestinal epithelium. J Exp Med (2010) 207(3):553–64. doi:10.1084/jem.20090858
46. Masopust D, Ha SJ, Vezys V, Ahmed R. Stimulation history dictates memory CD8 T cell phenotype: implications for prime-boost vaccination. J Immunol (2006) 177(2):831–9.
47. Shiow LR, Rosen DB, Brdickova N, Xu Y, An J, Lanier LL, et al. CD69 acts downstream of interferon-alpha/beta to inhibit S1P1 and lymphocyte egress from lymphoid organs. Nature (2006) 440(7083):540–4. doi:10.1038/nature04606
48. Casey KA, Fraser KA, Schenkel JM, Moran A, Abt MC, Beura LK, et al. Antigen-independent differentiation and maintenance of effector-like resident memory T cells in tissues. J Immunol (2012) 188(10):4866–75. doi:10.4049/jimmunol.1200402
49. Gebhardt T, Wakim LM, Eidsmo L, Reading PC, Heath WR, Carbone FR. Memory T cells in nonlymphoid tissue that provide enhanced local immunity during infection with herpes simplex virus. Nat Immunol (2009) 10(5):524–30. doi:10.1038/ni.1718
50. Major EO. Progressive multifocal leukoencephalopathy in patients on immunomodulatory therapies. Annu Rev Med (2010) 61:35–47. doi:10.1146/annurev.med.080708.082655
51. Bromley SK, Yan S, Tomura M, Kanagawa O, Luster AD. Recirculating memory T cells are a unique subset of CD4+ T cells with a distinct phenotype and migratory pattern. J Immunol (2013) 190(3):970–6. doi:10.4049/jimmunol.1202805
52. Tomura M, Honda T, Tanizaki H, Otsuka A, Egawa G, Tokura Y, et al. Activated regulatory T cells are the major T cell type emigrating from the skin during a cutaneous immune response in mice. J Clin Invest (2010) 120(3):883–93. doi:10.1172/JCI40926
53. de Goër de Herve MG, Dembele B, Vallée M, Herr F, Cariou A, Taoufik Y. Direct CD4 help provision following interaction of memory CD4 and CD8 T cells with distinct antigen-presenting dendritic cells. J Immunol (2010) 185(2):1028–36. doi:10.4049/jimmunol.0904209
54. de Goër de Herve MG, Jaafoura S, Vallée M, Taoufik Y. FoxP3(+) regulatory CD4 T cells control the generation of functional CD8 memory. Nat Commun (2012) 3:986. doi:10.1038/ncomms1992
55. Janssen EM, Lemmens EE, Wolfe T, Christen U, von Herrath MG, Schoenberger SP. CD4+ T cells are required for secondary expansion and memory in CD8+ T lymphocytes. Nature (2003) 421(6925):852–6. doi:10.1038/nature01441
56. Shedlock DJ, Shen H. Requirement for CD4 T cell help in generating functional CD8 T cell memory. Science (2003) 300(5617):337–9. doi:10.1126/science.1082305
Keywords: effector T cells, memory T cells, tissue-specific imprinting, tissue residency
Citation: Krzysiek R, de Goër de Herve M-G, Yang H and Taoufik Y (2013) Tissue competence imprinting and tissue residency of CD8 T cells. Front. Immunol. 4:283. doi: 10.3389/fimmu.2013.00283
Received: 07 May 2013; Accepted: 31 August 2013;
Published online: 17 September 2013.
Edited by:
Susan Swain, University of Massachusetts Medical School, USAReviewed by:
Joshua J. Obar, Montana State University, USAKim Klonowski, University of Georgia, USA
Copyright: © 2013 Krzysiek, de Goër de Herve, Yang and Taoufik. This is an open-access article distributed under the terms of the Creative Commons Attribution License (CC BY). The use, distribution or reproduction in other forums is permitted, provided the original author(s) or licensor are credited and that the original publication in this journal is cited, in accordance with accepted academic practice. No use, distribution or reproduction is permitted which does not comply with these terms.
*Correspondence: Roman Krzysiek, Department of Immunology, CHU Bicêtre, 78 Avenue du Général Leclerc, 94276 Le Kremlin-Bicêtre, France e-mail:cm9tYW4ua3J6eXNpZWtAYmN0LmFwaHAuZnI=;
Yassine Taoufik, 78 Avenue du Général Leclerc, 94276 Le Kremlin-Bicêtre, France e-mail:eWFzc2luZS50YW91ZmlrQGJjdC5hcGhwLmZy