- 1Department of Experimental Medicine, Istituto Pasteur-Fondazione Cenci-Bolognetti, Fondazione Eleonora Lorillard Spencer Cenci, Sapienza University, Rome, Italy
- 2Department of Molecular Medicine, Istituto Pasteur-Fondazione Cenci-Bolognetti, Fondazione Eleonora Lorillard Spencer Cenci, Sapienza University, Rome, Italy
Target cell recognition by cytotoxic lymphocytes implies the simultaneous engagement and clustering of adhesion and activating receptors followed by the activation of an array of signal transduction pathways. The cytotoxic immune synapse represents the highly specialized dynamic interface formed between the cytolytic effector and its target that allows temporal and spatial integration of signals responsible for a defined sequence of processes culminating with the polarized secretion of lytic granules. Over the last decades, much attention has been given to the molecular signals coupling receptor ligation to the activation of cytolytic machinery. Moreover, in the last 10 years the discovery of genetic defects affecting cytotoxic responses greatly boosted our knowledge on the molecular effectors involved in the regulation of discrete phases of cytotoxic process at post-receptor levels. More recently, the use of super resolution and total internal reflection fluorescence imaging technologies added new insights on the dynamic reorganization of receptor and signaling molecules at lytic synapse as well as on the relationship between granule dynamics and cytoskeleton remodeling. To date we have a solid knowledge of the molecular mechanisms governing granule movement and secretion, being not yet fully unraveled the machinery that couples early receptor signaling to the late stage of synapse remodeling and granule dynamics. Here we highlight recent advances in our understanding of the molecular mechanisms acting in the activation of cytolytic machinery, also discussing similarities and differences between Natural killer cells and cytotoxic CD8+ T cells.
Introduction
Natural killer (NK) cells and cytotoxic T lymphocytes (CTLs) are major actors in immune protection against viral infections and cell transformation, and also mediate, in certain conditions, the killing of autologous or allogeneic un-diseased cells (1, 2). Target cell killing can occur upon the polarized secretion of cytotoxic mediators, such as perforin and granzymes, stored in specialized secretory lysosomes termed lytic granules (3).
While CTLs are activated by specific antigen recognition, the activation of NK cells is regulated by a balance of activating and inhibitory signals through a multitude of germ-line encoded receptors following the recognition of ligands expressed on the surface of target cells (4).
Based on recent acquisitions, this review attempts to draw a comprehensive picture on the coupling of receptor proximal signals to the late stages of synapse remodeling and granule dynamics; rather than covering how signals from discrete activation receptors cooperate to control NK-cell activation, a topic which has been extensively addressed in recent excellent reviews (5), we would try to recapitulate for every individual phase of the cytolytic process how the molecular signals arising upon receptor ligation are coupled to the distal molecular effectors responsible for the activation of cytolytic machinery, also highlighting the differences between CTLs and NK cells.
Cytolytic Synapse Formation
The cytotoxic event is a well defined multistep process starting with the formation of a cell–cell contact specialized area called cytolytic synapse (3, 6) devoted to the polarized secretion of cytotoxic molecules.
Upon target recognition, receptors and signaling molecules rapidly segregate in the cytolytic synapse forming a supramolecular activation cluster (SMAC) that can be divided into concentrical zones: the central (cSMAC) and the peripheral (pSMAC) SMAC that is thought to be the focal point for the exocytosis of secretory lysosomes.
The formation of a mature synapse is not always essential for cell lysis by CTLs (7, 8), but it is believed to increase the efficiency of lytic granule polarization and target cell killing (9). Indeed, intra-vital imaging of the behavior of individual CTL or NK-cell infiltrating solid tumors in a mouse model has revealed that while CTLs tend to form more stable contacts with tumor cells, NK cells establish dynamic contacts (10).
An early stage in the commitment to cytolytic synapse formation is actin reorganization. As shown by 3-D confocal microscopy studies, actin rapidly polymerizes at the synapse periphery of both CTLs and NK cells to arrange a dense ring of cortical F-actin surrounding a central area through which lytic granules are secreted (6, 11).
Recently, the model of NK cells secreting lytic granules through a central region devoid of F-actin has been exceeded. A couple of companion papers (12, 13), both using very high-resolution imaging techniques, reveal that F-actin forms a pervasive network at the synapse, and that following activating receptor engagement, lytic granules are secreted through the filamentous network by accessing minimally sufficient sized clearances instead of a large-scale clearing of actin filaments. Such remodeling of cortical actin occurs within the central region of the synapse establishing secretory domain where lytic granules dock.
Strictly dependent on actin dynamics, activating signals are initiated by the formation of receptor micro-clusters at the periphery of the synapse in CTLs (14) and NK cells (15) undergoing a centripetal migration toward the synapse center. This movement is directed by actin depolymerization flow from an actin-rich periphery into an actin-poor area as shown by total internal reflection fluorescence microscopy (TIRF)-based studies in live T cells on lipid bilayer (16, 17).
Although, LFA1 ligation by ICAM-1 can signal on its own in NK cells (18), the formation of a stable and symmetric F-actin ring at cytolytic synapse requires integrin and NKG2D activating receptor co-ligation (12, 19). Similarly, in T cells, T cell receptor (TCR) and LFA1 co-aggregation is needed for the efficient synapse formation (20) (Figure 1A).
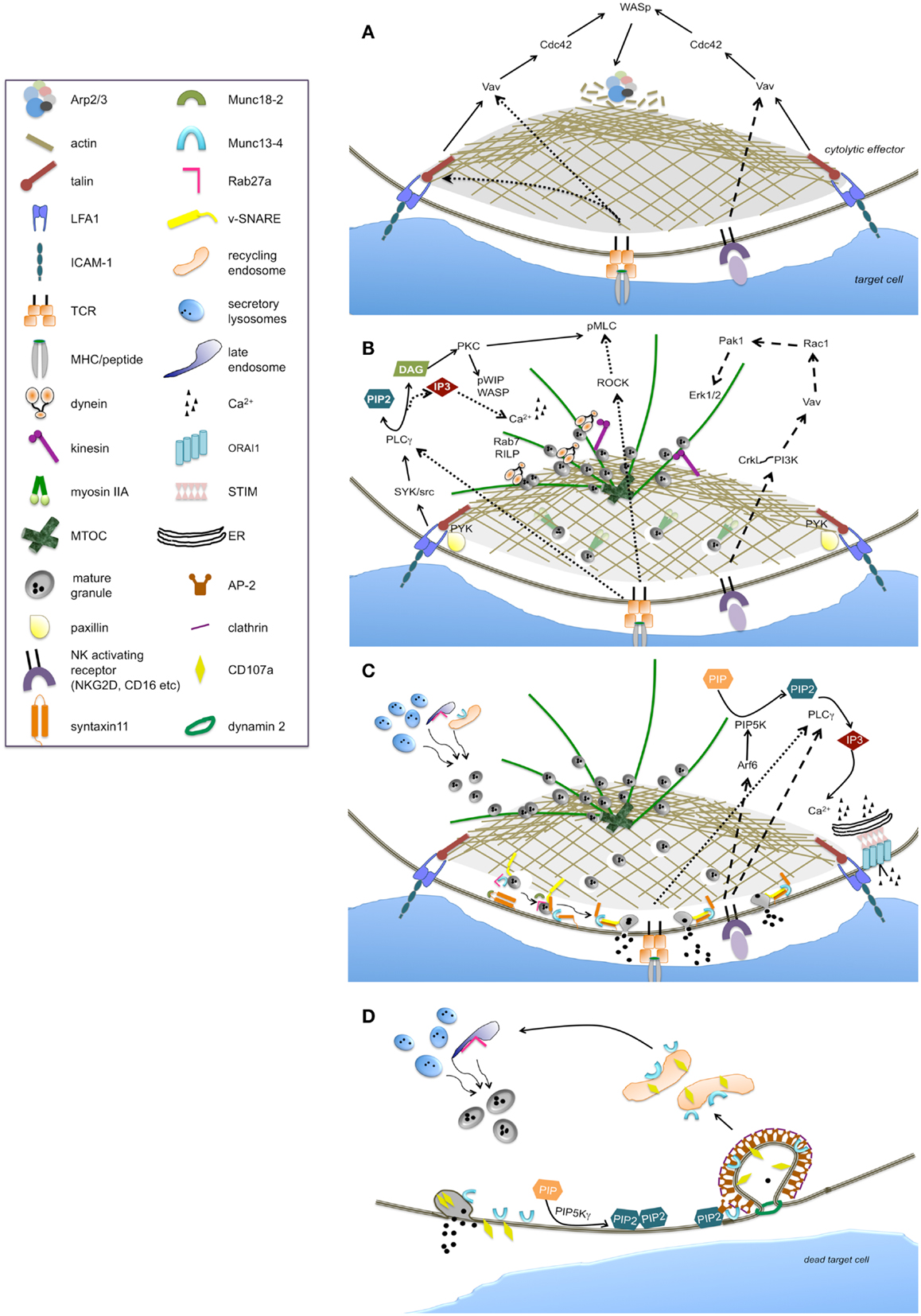
Figure 1. Main signaling pathways and molecular effectors implicated in the regulation of individual phases of the cytolytic process. The sequence of steps leading to the activation of cytolytic machinery are represented as: lytic synapse formation (A), secretory apparatus polarization (B), lytic granule secretion (C), and lytic granule trafficking and retrieval (D). The continuous line arrows indicate the signaling pathway likely common to NK cells and CTLs. Large-dashed arrows indicate the exclusive pathways of NK cells, while fine-dashed arrows indicate the exclusive pathways of CTLs.
Downstream to LFA1, Cdc42 becomes active (21) and exhibits an oscillatory activation behavior at NK synapse (22); its molecular effector, Wiskott–Aldrich syndrome protein (WASp) is directly responsible for actin polymerization through the activation of the actin nucleator Arp2/3 complex. Accordingly, in the absence of WASp, as it occurs in the immune disorder WAS, or in the presence of actin inhibitors, F-actin accumulation at the synapse and the ability to kill is reduced in both NK cells (23–25) and CTLs (26) (Figure 1A). WASp activation strictly depends on phosphoinositide phosphatidylinositol 4,5-bisphosphate (PIP2) that is rapidly consumed at the cytolytic synapse (27). In this context the role of the actin binding protein talin has been clarified: its binding to the cytoplasmic tail of beta2 integrin mediates the recruitment of Arp2/3 which initiates actin polymerization upon LFA1 ligation (28).
Secretory Apparatus Polarization
The activation of cytolytic machinery is achieved through a strong cell polarization driven by the reorganization of microtubule and actin cytoskeleton allowing the polarized secretion of lytic granules (Figure 1B).
Lytic granule journey starts with a retrograde minus-end transport on microtubules toward the centrosome or microtubule-organizing center (MTOC), followed by movement of the MTOC with clustered granules toward the edge of cSMAC both in CTLs and NK cells (29, 30). Microtubule-based molecular motor dynein has been implicated in the retrograde transport of granules to the MTOC and the subsequent movement of the MTOC toward the immune synapse in an actin-independent manner (31, 32). While in T cells the MTOC was believed to associate closely with the synapse to directly deliver lytic granule without the need of additional plus-ended granule motors (29), a recent report demonstrates that the microtubule motor protein kinesin-1 complexed to the small G protein Rab27a and synaptotagmin-like protein (slp)-3, acts in the terminal anterograde transport of cytotoxic granules close to the plasma membrane in CTLs (33). Whether kinesin-1 is required for the final microtubule transport to position lytic granule in NK cells needs further studies.
Recently, describing a spatio-temporal dissociation of the MTOC with lytic granules, the question of the requirement of MTOC polarization for efficient lethal hit delivery in CTLs has been raised (34). Beside microtubules, the movement of the MTOC/lytic granule complex toward the synapse also involves actin dynamics (6, 32). Indeed, in a pre-final step, the motor protein non-muscle myosin IIA mediates F-actin association with lytic granules (35, 36) and drives the final transit through the minimal clearance in the F-actin network across the cytolytic synapse, thus allowing granule approximation to synaptic membrane in NK cells. Furthermore, also in CTLs the two motor complexes dynein and myosin II has been described to work in a collaborative manner (37). The mutation of the gene encoding myosin IIA leads to May–Hegglin anomaly implying a reduced NK cytotoxicity (38).
In human NK cells signals for granule polarization can be uncoupled from degranulation: LFA1 engagement by ICAM-1 is sufficient to induce granule redistribution (18) thus featuring the minimal requirement for secretory lysosome polarization.
PLC gamma is regarded as a major factor in driving microtubule polarity and granule redistribution. Recently, LFA1-dependent Syk phosphorylation has been linked to the activation of PLC gamma-protein kinase C (PKC)-dependent pathway required for granule polarization (39) in NK cells. Additionally, Src kinases downstream to activating receptors, have been also implicated in the repositioning of MTOC and lytic granule both in CTL and NK cells (40, 41). Moreover, beta2 integrin-dependent phosphorylation of the molecular scaffold paxillin that associates with the tyrosine kinase Pyk2 has been shown to participate in directing MTOC polarization at NK synapse (42, 43). In CTLs the combined signal LFA1 and TCR is required for paxillin recruitment (44) to the site of integrin engagement.
PLC gamma defines a critical polarization pathway mediating the localized accumulation of second messenger diacylglycerol (DAG), which in turn promotes the recruitment of dynein at the MTOC/granule clusters (45). Notably, in agreement with the failure of integrin receptors to be coupled to Ca2+-dependent pathways, polarization resulted unaffected in the absence of calcium signaling in NK cells (39, 41). Differently in CTLs, variation in the Ca2+ concentration is thought to determine the kinetics of granule recruitment to the MTOC (9, 46).
Downstream to DAG, MTOC dynamics and polarization is driven by PKC isozymes recruited to the synaptic membrane. In CTLs, PKCη, PKCε, and PKCθ function redundantly to regulate the two motor complexes dynein and myosin II in driving MTOC polarization. Recent findings clarified how TCR signaling is coupled to the force-generating machinery demonstrating that PKCs activity controls myosin II localization directly by phosphorylating inhibitory sites within the myosin regulatory light chain; concurrently, Rho kinase (ROCK), mediating the phosphorylation of distinct sites within myosin regulatory light chain, induces myosin II clustering behind the MTOC (37). Additionally, PKC delta has been shown to co-localize with polarized granules in T cells (47).
In NK cells PKCθ is required for the WASp interacting protein WIP activation and association with secretory lysosomes at cytolytic synapse; the subsequent interaction with F-actin and myosin IIA (48, 49) links lytic granules to the actomyosin-dependent movement.
Another protein that plays a role in lytic granule movement in CTLs, is the small GTPase Rab7 acting by recruiting dynein-dynactin motor complex to secretory lysosomes through its molecular effector Rab interacting lysosomal protein, RILP, (50). Rab7 is recruited to the WASp-WIP-F-actin complex at the NK lytic synapse (48).
Cdc42-dependent signals have also been implicated in MTOC polarization through CDC42-interacting protein (CIP4), which contributes to anchor MTOC to the synapse by interacting with WASp and tubulin (51).
Proximal signals required for MTOC polarization in NK cells also include the extracellular regulated kinases, Erk1/2. The well-characterized phosphatidylinositol 3-kinase (PI3K)-dependent Rac1 → p21-activated kinase 1 (Pak1) → MEK-ERK1/2 pathway has been long referred as critical for lytic granule polarization in NK cells (43, 52, 53). On the opposite, in CTLs Erk activity is dispensable for MTOC reorientation (44). Erk activation has been uncoupled from integrin receptors while it is turned on downstream to activating receptors. Following NKG2D ligation for instance, PI3K-dependent pathway involving the adaptor CrkL and the small GTPase Rap1, has been shown to be required for MTOC polarization (54).
Despite multiple evidences, the involvement of Erk in microtubule remodeling is not fully understood. Erk2 was found to co-localize with microtubules (55), whereas paxillin phosphorylation has been linked to the PI3K-Erk pathway (56).
The final part of granule journey involves actin remodeling which occurs independently from LFA1 co-ligation (57), and defines a further “checkpoint” in the cytotoxic process: recent evidences demonstrate that NK-cell activation through several activating receptors including CD16 and NKG2D, leads to the remodeling of the cortical actin mesh at the synapse center to produce discrete nanometer-scale domains, as above mentioned (12). Contextually, micro-clusters of Grb2 and Vav1 signaling molecules rapidly reorganize to form a ring-shaped structure at the synapse center. Indeed, the pathway Vav1 → Rac1 → Pak1 is activated following CD16 and NKG2D ligation (58, 59) and regulates actin and microtubule dynamics (53) (Figure 1B). Whether remodeling of cortical actin within the central region of the NK-cell synapse is also controlled by this pathway, requires further investigation.
Lytic Granule Secretion
Once lytic granules have reached the plasma membrane they are highly dynamic and mobile (60) and, contextually, docking and priming occur. Rab27a GTPase plays a critical role in granule docking; ultrastructural analysis evidenced that polarized granules fail to dock at the plasma membrane in response to TCR stimulation (61). The gene encoding Rab27a is mutated in the immunodeficiency Griscelli syndrome 2 (GS2) and in Ashen mice, resulting in severely reduced cytotoxic activity in CTLs (62, 63). In CTLs Rab27a binds to the synaptotagmin-like proteins Slp1 and Slp2 facilitating granule tethering (64, 65). In NK cells a Rab27a-independent pathway for CD16-mediated killing has also been reported (66). The finding of a residual degranulation in NK cells from GS2 patients is thought to indicate a relative redundancy of Rab protein activity (67).
Upon granule docking, the Rab27a binding partner, Munc13-4, mediates the priming of lytic granule in CTLs and NK cells (68, 69). Munc13-4 is mutated in familial hemophagocytic lymphohistiocytosis type 3 (FHL3); in the absence of Munc13-4, cytotoxic granules dock at the site of secretion but cannot fuse with the plasma membrane, thus leading to a severe reduction of cytotoxic activity. Munc13-4 has been postulated to open the conformation of the target (t-)SNARE syntaxin11 by removal of Munc18-2 which is required for syntaxin11 stabilization in NK cells (70). Indeed, Munc18-2 mutation causes FHL5 resulting in reduced granule exocytosis in NK cells (71). Moreover, mutations of syntaxin11 in FHL4 (72, 73) implicate a partial impairment of granule exocytosis both in NK cells and CTLs; the observation that the defect can be partially restored by IL-2 stimulation suggests the redundancy of syntaxin isoforms in the secretory mechanisms. Functional studies have shown that deficiency in vesicle (v-)SNAREs, VAMP7, VAMP8, and VAMP4 results in defective granule exocytosis (74–76) (Figure 1C).
An additional role of Munc13-4 in enabling the maturation of perforin-containing granules has been highlighted in CTLs: a seminal study demonstrated that close to the cytolytic synapse, Munc13-4 promotes the coalescence of a pool of endosomal/recycling vesicles carrying effectors of cytolytic machinery such as Rab27a and Munc13-4, with perforin-containing granules, leading to the formation of a unique mature “exocytic vesicle” (77). The observation that in NK cells neither Rab27 or Munc13-4 are present on the lytic granule surface but associate with them upon receptor engagement, suggests a similar role; moreover, separate signaling routes are used in NK cells to direct Munc13-4 and Rab27a to lytic granule (67).
While the nature of the signals that couples activating receptors to secretory machine remains partially unknown, the strict calcium-dependence remains the hallmark for lytic granule secretion (78). The essential role of PLCgamma2 in granule exocytosis has been demonstrated in knock out mice (79). Downstream to TCR or NK activating receptors, the activation of PLC gamma results in the hydrolysis of PIP2 to generate the second messenger inositol-1,4,5-trisphosphate (IP3) which triggers the mobilization of intracellular Ca2+ from endoplasmic reticulum; the resulting depletion of Ca2+ stores and aggregation of endoplasmic reticulum Ca2+ sensor, STIM, trans-activate the plasma membrane Ca2+ release-activated Ca2+ channel ORAI1, leading to the store operated Ca2+ entry (SOCE). Patients with mutation in either STIM1 or ORAI1 exhibit a defect in secretion, whereas lytic granule polarization results unaffected (80). Phosphatidylinositol-4-phosphate 5-kinase (PIP5K)alpha and gamma isoforms are the enzymes mainly responsible for PIP2 synthesis in NK cells; they synergistically act in the control IP3/Ca2+ levels required for lytic granule exocytosis (27); in contrast, PIP5Ks isoenzymes behave redundantly in the control of granule polarization which is in line with the lack of Ca2+-dependence, as above mentioned. Downstream to CD16, PIP5Kalpha activation is regulated by small G protein Arf6; accordingly, interfering with Arf6 function leads to reduced lytic granule exocytosis (81).
The Ca2+-dependent factors required for lytic granule exocytosis are largely unknown. The high-affinity Ca2+ binding protein synaptotagmin is a possible candidate; recently, synaptotagmin VII has been implicated in exocytosis of lytic granules (82) (Figure 1C).
Because Munc13-4 contains two C2, Ca2+ binding domains, it also might represent a Ca2+ sensor for exocytosis. Actually, the translocation of Munc13-4 to membrane rafts, indicating granule fusion with plasma membrane, occurs in Ca2+-independent manner (83).
Lytic Granule Trafficking and Retrieval
The demonstration that Rab11+ recycling endosome polarizes at cytolytic synapse along with secretory apparatus in order to allow Munc13-4-dependent granule maturation into a fusion-competent lytic organelle (77), have raised the disrupting concept that the cytolytic synapse behaves as a focal point for both exocytosis and endocytosis. Indeed, a bidirectional trafficking of lytic granule proteins exposed at the plasma membrane on degranulation has been demonstrated; both lysosome-associated membrane protein-1 (LAMP-1, also known as CD107a) (84) and Munc13-4 (83) undergo a rapid endocytosis, leading to the hypothesis that granule exocytosis is coordinated with the retrieval of cytolytic machinery components. Additionally, cytolytic mediators are also recaptured into early endosomes of NK cells via a clathrin-dependent route after target cell stimulation thus contributing to the cytolytic potential (85).
In this framework, the ability of cytolytic effectors to execute multiple killing cycles in a short time period (86–88) is thought to depend both on the release of a fraction of lytic granules (77) and on a continuum refilling of the granule store through newly synthesized cytotoxic mediators (89). Whether secretory lysosome retrieval could facilitate recycling and reusing of cytotoxic machinery components thus contributing to the serial killing potential, is not fully understood.
The molecular signals controlling the endocytic traffic at cytolytic synapse have begun to be clarified. Recent findings reported that a constitutive PIP5Kgamma-dependent PIP2 pool is involved in the control of Munc13-4 re-internalization through a clathrin/AP2 dependent endocytic route, which is functional to ensure the full serial killing potential in NK cells (83). Such findings strengthen the analogy between neuronal and cytolytic synapse where PIP5K gamma also triggers the clathrin-mediated retrieval of synaptic vesicles (90) (Figure 1D).
An additional effector molecule involved in granule recapture during exocytosis can be the fission factor dynamin 2 which have been shown to be required for cytotoxicity in NK cells (91).
Perspectives
One of the aspects that remains enigmatic in the biology of lymphocyte cytotoxicity is the serial killing potential. The ability of NK cells and CTLs to mediate the sequential attack of successive targets by a single effector was suggested in early observations; only recently, however, intra-vital and real-time imaging of a single cell behavior have shed light on a marked heterogeneity in the cytotoxic potential and on relevant differences in the contact dynamic with target cells (87, 88): CTLs forming stable independent synapse are able to simultaneously bind and attack multiple targets, while NK cells forming short-lived synapse, allows the detachment and the subsequent engagement of other targets. Intriguingly, NK cells are proposed to integrate signals arising form previous and current targets resulting in a continuous signaling that persist until formation of a new synapse (87). A considerable challenge for the future will be the understanding of the spatial and temporal coordination of molecular signals which may account for different qualities of cytolytic responses. In such framework the knowledge on how NK-cell contact with target is terminated and the molecular basis of retrieval processes also represent future challenges.
Also we need to learn more on NK-cell education: in particular, while the molecular basis of MHC-I-dependent licensing have began to be clarified (92, 93), completely unknown remains the molecular basis of NK hypo-responsiveness that follows the sustained stimulation of certain activating receptors.
The unraveling of NK functional plasticity would have a major impact in NK-cell-based immunotherapeutic approaches and could drive a renewed interest in signal transduction processes.
Conflict of Interest Statement
The authors declare that the research was conducted in the absence of any commercial or financial relationships that could be construed as a potential conflict of interest.
Acknowledgments
Supported by grants from Italian Association for Cancer Research (AIRC), the Italian Ministry for University and Research (MIUR), and the Center of Excellence (BEMM).
References
1. Zhang N, Bevan MJ. CD8(+) T cells: foot soldiers of the immune system. Immunity (2011) 35:161–8. doi: 10.1016/j.immuni.2011.07.010
2. Vivier E, Raulet DH, Moretta A, Caligiuri MA, Zitvogel L, Lanier LL, et al. Innate or adaptive immunity? The example of natural killer cells. Science (2011) 331:44–9. doi:10.1126/science.1198687
3. Stinchcombe JC, Griffiths GM. Secretory mechanisms in cell-mediated cytotoxicity. Annu Rev Cell Dev Biol (2007) 23:495–517. doi:10.1146/annurev.cellbio.23.090506.123521
4. Lanier LL. Up on the tightrope: natural killer cell activation and inhibition. Nat Immunol (2008) 9:495–502. doi:10.1038/ni1581
5. Long EO, Sik Kim H, Liu D, Peterson ME, Rajagopalan S. Controlling natural killer cell responses: integration of signals for activation and inhibition. Annu Rev Immunol (2013) 31:227–58. doi:10.1146/annurev-immunol-020711-075005
6. Orange JS, Harris KE, Andzelm MM, Valter MM, Geha RS, Strominger JL. The mature activating natural killer cell immunologic synapse is formed in distinct stages. Proc Natl Acad Sci U S A (2003) 100:14151–6. doi:10.1073/pnas.1835830100
7. Purbhoo MA, Irvine DJ, Huppa JB, Davis MM. T cell killing does not require the formation of a stable mature immunological synapse. Nat Immunol (2004) 5:524–30. doi:10.1038/ni0604-658a
8. Wiedemann A, Depoil D, Faroudi M, Valitutti S. Cytotoxic T lymphocytes kill multiple targets simultaneously via spatiotemporal uncoupling of lytic and stimulatory synapses. Proc Natl Acad Sci U S A (2006) 103:10985–90. doi:10.1073/pnas.0600651103
9. Beal AM, Anikeeva N, Varma R, Cameron TO, Vasiliver-Shamis G, Norris PJ, et al. Kinetics of early T cell receptor signaling regulate the pathway of lytic granule delivery to the secretory domain. Immunity (2009) 31:632–42. doi:10.1016/j.immuni.2009.09.004
10. Deguine J, Breart B, Lemaître F, Di Santo JP, Bousso P. Intravital imaging reveals distinct dynamics for natural killer and CD8(+) T cells during tumor regression. Immunity (2010) 33:632–44. doi:10.1016/j.immuni.2010.09.016
11. Stinchcombe JC, Bossi G, Booth S, Griffiths GM. The immunological synapse of CTL contains a secretory domain and membrane bridges. Immunity (2001) 5:751–61. doi:10.1016/S1074-7613(01)00234-5
12. Brown AC, Oddos S, Dobbie IM, Alakoskela JM, Parton RM, Eissmann P, et al. Remodelling of cortical actin where lytic granules dock at natural killer cell immune synapses revealed by super-resolution microscopy. PLoS Biol (2011) 9:e1001152. doi:10.1371/journal.pbio.1001152
13. Rak GD, Mace EM, Banerjee PP, Svitkina T, Orange JS. Natural killer cell lytic granule secretion occurs through a pervasive actin network at the immune synapse. PLoS Biol (2011) 9:e1001151. doi:10.1371/journal.pbio.1001151
14. Varma R, Campi G, Yokosuka T, Saito T, Dustin ML. T cell receptor-proximal signals are sustained in peripheral microclusters and terminated in the central supramolecular activation cluster. Immunity (2006) 25:117–27. doi:10.1016/j.immuni.2006.04.010
15. Abeyweera TP, Merino E, Huse M. Inhibitory signaling blocks activating receptor clustering and induces cytoskeletal retraction in natural killer cells. J Cell Biol (2011) 192:675–90. doi:10.1083/jcb.201009135
16. Beemiller P, Jacobelli J, Krummel MF. Integration of the movement of signaling microclusters with cellular motility in immunological synapses. Nat Immunol (2012) 13:787–95. doi:10.1038/ni.2364
17. Babich A, Li S, O’Connor RS, Milone MC, Freedman BD, Burkhardt JK. F-actin polymerization and retrograde flow drive sustained PLCγ1 signaling during T cell activation. J Cell Biol (2012) 197:775–87. doi:10.1083/jcb.201201018
18. Bryceson YT, March ME, Barber DF, Ljunggren HG, Long EO. Cytolytic granule polarization and degranulation controlled by different receptors in resting NK cells. J Exp Med (2005) 202:1001–12. doi:10.1084/jem.20051143
19. Culley FJ, Johnson M, Evans JH, Kumar S, Crilly R, Casasbuenas J, et al. Natural killer cell signal integration balances synapse symmetry and migration. PLoS Biol (2009) 7:e1000159. doi:10.1371/journal.pbio.1000159
20. Anikeeva N, Somersalo K, Sims TN, Thomas VK, Dustin ML, Sykulev Y. Distinct role of lymphocyte function-associated antigen-1 in mediating effective cytolytic activity by cytotoxic T lymphocytes. Proc Natl Acad Sci U S A (2005) 102:6437–42. doi:10.1073/pnas.0502467102
21. Stabile H, Carlino C, Mazza C, Giliani S, Morrone S, Notarangelo LD, et al. Impaired NK-cell migration in WAS/XLT patients: role of Cdc42/WASp pathway in the control of chemokine-induced beta2 integrin high-affinity state. Blood (2010) 115:2818–26. doi:10.1182/blood-2009-07-235804
22. Carlin LM, Evans R, Milewicz H, Fernandes L, Matthews DR, Perani M. A targeted siRNA screen identifies regulators of Cdc42 activity at the natural killer cell immunological synapse. Sci Signal (2011) 4:ra81. doi:10.1126/scisignal.2001729
23. Orange JS, Ramesh N, Remold-O’Donnell E, Sasahara Y, Koopman L, Byrne M, et al. Wiskott-Aldrich syndrome protein is required for NK cell cytotoxicity and colocalizes with actin to NK cell-activating immunologic synapses. Proc Natl Acad Sci U S A (2002) 99:11351–6. doi:10.1073/pnas.162376099
24. Gismondi A, Cifaldi L, Mazza C, Giliani S, Parolini S, Morrone S, et al. Impaired natural and CD16-mediated NK cell cytotoxicity in patients with WAS and XLT: ability of IL-2 to correct NK cell functional defect. Blood (2004) 104:436–43. doi:10.1182/blood-2003-07-2621
25. Butler B, Cooper JA. Distinct roles for the actin nucleators Arp2/3 and hDia1 during NK-mediated cytotoxicity. Curr Biol (2009) 19:1886–96. doi:10.1016/j.cub.2009.10.029
26. De Meester J, Calvez R, Valitutti S, Dupré L. The Wiskott-Aldrich syndrome protein regulates CTL cytotoxicity and is required for efficient killing of B cell lymphoma targets. J Leukoc Biol (2010) 88:1031–40. doi:10.1189/jlb.0410197
27. Micucci F, Capuano C, Marchetti E, Piccoli M, Frati L, Santoni A, et al. PI5KI-dependent signals are critical regulators of the cytolytic secretory pathway. Blood (2008) 111:4165–72. doi:10.1182/blood-2007-08-108886
28. Mace EM, Monkley SJ, Critchley DR, Takei F. A dual role for talin in NK cell cytotoxicity: activation of LFA-1-mediated cell adhesion and polarization of NK cells. J Immunol (2009) 182:948–56.
29. Stinchcombe JC, Majorovits E, Bossi G, Fuller S, Griffiths GM. Centrosome polarization delivers secretory granules to the immunological synapse. Nature (2006) 443:462–5. doi:10.1038/nature05071
30. Stinchcombe JC, Salio M, Cerundolo V, Pende D, Arico M, Griffiths GM. Centriole polarisation to the immunological synapse directs secretion from cytolytic cells of both the innate and adaptive immune systems. BMC Biol (2011) 9:45. doi:10.1186/1741-7007-9-45
31. Daniele T, Hackmann Y, Ritter AT, Wenham M, Booth S, Bossi G, et al. A role for Rab7 in the movement of secretory granules in cytotoxic T lymphocytes. Traffic (2011) 12:902–11. doi:10.1111/j.1600-0854.2011.01194.x
32. Mentlik AN, Sanborn KB, Holzbaur EL, Orange JS. Rapid lytic granule convergence to the MTOC in natural killer cells is dependent on dynein but not cytolytic commitment. Mol Biol Cell (2010) 21:2241–56. doi:10.1091/mbc.E09-11-0930
33. Kurowska M, Goudin N, Nehme NT, Court M, Garin J, Fischer A, et al. Terminal transport of lytic granules to the immune synapse is mediated by the kinesin-1/Slp3/Rab27a complex. Blood (2012) 119:3879–89. doi:10.1182/blood-2011-09-382556
34. Bertrand F, Müller S, Roh KH, Laurent C, Dupré L, Valitutti S. An initial and rapid step of lytic granule secretion precedes microtubule organizing center polarization at the cytotoxic T lymphocyte/target cell synapse. Proc Natl Acad Sci U S A (2013) 110:6073–8. doi:10.1073/pnas.1218640110
35. Andzelm MM, Chen X, Krzewski K, Orange JS, Strominger JL. Myosin IIA is required for cytolytic granule exocytosis in human NK cells. J Exp Med (2007) 204:2285–91. doi:10.1084/jem.20071143
36. Sanborn KB, Mace EM, Rak GD, Difeo A, Martignetti JA, Pecci A, et al. Phosphorylation of the myosin IIA tailpiece regulates single myosin IIA molecule association with lytic granules to promote NK-cell cytotoxicity. Blood (2011) 118:5862–71. doi:10.1182/blood-2011-03-344846
37. Liu X, Kapoor TM, Chen JK, Huse M. Diacylglycerol promotes centrosome polarization in T cells via reciprocal localization of dynein and myosin II. Proc Natl Acad Sci U S A (2013) 110:11976–81. doi:10.1073/pnas.1306180110
38. Sanborn KB, Rak GD, Maru SY, Demers K, Difeo A, Martignetti JA, et al. Myosin IIA associates with NK cell lytic granules to enable their interaction with F-actin and function at the immunological synapse. J Immunol (2009) 182:6969–84. doi:10.4049/jimmunol.0804337
39. March ME, Long EO. β2 integrin induces TCRζ-Syk-phospholipase C-γ phosphorylation and paxillin-dependent granule polarization in human NK cells. J Immunol (2011) 186:2998–3005. doi:10.4049/jimmunol.1002438
40. Tsun A, Qureshi I, Stinchcombe JC, Jenkins MR, de la Roche M, Kleczkowska J, et al. Centrosome docking at the immunological synapse is controlled by Lck signaling. J Cell Biol (2011) 192:663–74. doi:10.1083/jcb.201008140
41. James AM, Hsu HT, Dongre P, Uzel G, Mace EM, Banerjee PP, et al. Rapid activation receptor- or IL-2-induced lytic granule convergence in human natural killer cells requires Src, but not downstream signaling. Blood (2013) 121:2627–37. doi:10.1182/blood-2012-06-437012
42. Gismondi A, Bisogno L, Mainiero F, Palmieri G, Piccoli M, Frati L, et al. Proline-rich tyrosine kinase-2 activation by beta 1 integrin fibronectin receptor cross-linking and association with paxillin in human natural killer cells. J Immunol (1997) 159:4729–36.
43. Sancho D, Nieto M, Llano M, Rodríguez-Fernández JL, Tejedor R, Avraham S, et al. The tyrosine kinase PYK-2/RAFTK regulates natural killer (NK) cell cytotoxic response, and is translocated and activated upon specific target cell recognition and killing. J Cell Biol (2000) 149:1249–62. doi:10.1083/jcb.149.6.1249
44. Robertson LK, Ostergaard HL. Paxillin associates with the microtubule cytoskeleton and the immunological synapse of CTL through its leucine-aspartic acid domains and contributes to microtubule organizing center reorientation. J Immunol (2011) 187:5824–33. doi:10.4049/jimmunol.1003690
45. Quann EJ, Merino E, Furuta T, Huse M. Localized diacylglycerol drives the polarization of the microtubule-organizing center in T cells. Nat Immunol (2009) 10:627–35. doi:10.1038/ni.1734
46. Kuhné MR, Lin J, Yablonski D, Mollenauer MN, Ehrlich LI, Huppa J, et al. Linker for activation of T cells, zeta-associated protein-70, and Src homology 2 domain-containing leukocyte protein-76 are required for TCR-induced microtubule-organizing center polarization. J Immunol (2003) 171:860–6.
47. Ma JS, Haydar TF, Radoja S. Protein kinase C delta localizes to secretory lysosomes in CD8+ CTL and directly mediates TCR signals leading to granule exocytosis-mediated cytotoxicity. J Immunol (2008) 181: 4716–22.
48. Krzewski K, Chen X, Orange JS, Strominger JL. Formation of a WIP-, WASp-, actin-, and myosin IIA-containing multiprotein complex in activated NK cells and its alteration by KIR inhibitory signaling. J Cell Biol (2006) 173:121–32. doi:10.1083/jcb.200509076
49. Krzewski K, Chen X, Strominger JL. WIP is essential for lytic granule polarization and NK cell cytotoxicity. Proc Natl Acad Sci U S A (2008) 105:2568–73. doi:10.1073/pnas.0711593105
50. Jordens I, Fernandez-Borja M, Marsman M, Dusseljee S, Janssen L, Calafat J, et al. The Rab7 effector protein RILP controls lysosomal transport by inducing the recruitment of dynein-dynactin motors. Curr Biol (2001) 1:1680–5. doi:10.1016/S0960-9822(01)00531-0
51. Banerjee PP, Pandey R, Zheng R, Suhoski MM, Monaco-Shawver L, Orange JS. Cdc42-interacting protein-4 functionally links actin and microtubule networks at the cytolytic NK cell immunological synapse. J Exp Med (2007) 204:2305–20. doi:10.1084/jem.20061893
52. Jiang K, Zhong B, Gilvary DL, Corliss BC, Hong-Geller E, Wei S, et al. Pivotal role of phosphoinositide-3 kinase in regulation of cytotoxicity in natural killer cells. Nat Immunol (2000) 1:419–25. doi:10.1038/80859
53. Graham DB, Cella M, Giurisato E, Fujikawa K, Miletic AV, Kloeppel T, et al. Vav1 controls DAP10-mediated natural cytotoxicity by regulating actin and microtubule dynamics. J Immunol (2006) 177:2349–55.
54. Segovis CM, Schoon RA, Dick CJ, Nacusi LP, Leibson PJ, Billadeau DD. PI3K links NKG2D signaling to a CrkL pathway involved in natural killer cell adhesion, polarity, and granule secretion. J Immunol (2009) 182:6933–42. doi:10.4049/jimmunol.0803840
55. Chen X, Allan DS, Krzewski K, Ge B, Kopcow H, Strominger JL. CD28-stimulated ERK2 phosphorylation is required for polarization of the microtubule organizing center and granules in YTS NK cells. Proc Natl Acad Sci U S A (2006) 103:10346–51. doi:10.1073/pnas.0604236103
56. Robertson LK, Mireau LR, Ostergaard HL. A role for phosphatidylinositol 3-kinase in TCR-stimulated ERK activation leading to paxillin phosphorylation and CTL degranulation. J Immunol (2005) 175:8138–45.
57. Brown AC, Dobbie IM, Alakoskela JM, Davis I, Davis DM. Super-resolution imaging of remodeled synaptic actin reveals different synergies between NK cell receptors and integrins. Blood (2012) 120:3729–40. doi:10.1182/blood-2012-05-429977
58. Billadeau DD, Brumbaugh KM, Dick CJ, Schoon RA, Bustelo XR, Leibson PJ. The Vav-Rac1 pathway in cytotoxic lymphocytes regulates the generation of cell-mediated killing. J Exp Med (1998) 188:549–59. doi:10.1084/jem.188.3.549
59. Galandrini R, Palmieri G, Piccoli M, Frati L, Santoni A. Role for the Rac1 exchange factor Vav in the signaling pathways leading to NK cell cytotoxicity. J Immunol (1999) 162:3148–52.
60. Mace EM, Wu WW, Ho T, Mann SS, Hsu HT, Orange JS. NK cell lytic granules are highly motile at the immunological synapse and require F-actin for post-degranulation persistence. J Immunol (2012) 189:4870–80. doi:10.4049/jimmunol.1201296
61. Stinchcombe JC, Barral DC, Mules EH, Booth S, Hume AN, MacHesky LM, et al. Rab27a is required for regulated secretion in cytotoxic T lymphocytes. J Cell Biol (2001) 152:825–34. doi:10.1083/jcb.152.4.825
62. Wilson SM, Yip R, Swing DA, O’Sullivan TN, Zhang Y, Novak EK, et al. A mutation in Rab27a causes the vesicle transport defects observed in ashen mice. Proc Natl Acad Sci U S A (2000) 97:7933–8. doi:10.1073/pnas.140212797
63. Ménasché G, Pastural E, Feldmann J, Certain S, Ersoy F, Dupuis S, et al. Mutations in RAB27A cause Griscelli syndrome associated with haemophagocytic syndrome. Nat Genet (2000) 25:173–6. doi:10.1038/76024
64. Ménasché G, Ménager MM, Lefebvre JM, Deutsch E, Athman R, Lambert N, et al. A newly identified isoform of Slp2a associates with Rab27a in cytotoxic T cells and participates to cytotoxic granule secretion. Blood (2008) 112:5052–62. doi:10.1182/blood-2008-02-141069
65. Holt O, Kanno E, Bossi G, Booth S, Daniele T, Santoro A, et al. Slp1 and Slp2-a localize to the plasma membrane of CTL and contribute to secretion from the immunological synapse. Traffic (2008) 9:446–57. doi:10.1111/j.1600-0854.2008.00714.x
66. Gazit R, Aker M, Elboim M, Achdout H, Katz G, Wolf DG, et al. NK cytotoxicity mediated by CD16 but not by NKp30 is functional in Griscelli syndrome. Blood (2007) 109:4306–12. doi:10.1182/blood-2006-09-047159
67. Wood SM, Meeths M, Chiang SC, Bechensteen AG, Boelens JJ, Heilmann C, et al. Different NK cell-activating receptors preferentially recruit Rab27a or Munc13-4 to perforin-containing granules for cytotoxicity. Blood (2009) 114:4117–27. doi:10.1182/blood-2009-06-225359
68. Feldmann J, Callebaut I, Raposo G, Certain S, Bacq D, Dumont C, et al. Munc13-4 is essential for cytolytic granules fusion and is mutated in a form of familial hemophagocytic lymphohistiocytosis (FHL3). Cell (2003) 115:461–73. doi:10.1016/S0092-8674(03)00855-9
69. Marcenaro S, Gallo F, Martini S, Santoro A, Griffiths GM, Aricó M, et al. Analysis of natural killer-cell function in familial hemophagocytic lymphohistiocytosis (FHL): defective CD107a surface expression heralds Munc13-4 defect and discriminates between genetic subtypes of the disease. Blood (2006) 108:2316–23. doi:10.1182/blood-2006-04-015693
70. Elstak ED, Neeft M, Nehme NT, Voortman J, Cheung M, Goodarzifard M, et al. The munc13-4-rab27 complex is specifically required for tethering secretory lysosomes at the plasma membrane. Blood (2011) 118:1570–8. doi:10.1182/blood-2011-02-339523
71. Côte M, Ménager MM, Burgess A, Mahlaoui N, Picard C, Schaffner C, et al. Munc18-2 deficiency causes familial hemophagocytic lymphohistiocytosis type 5 and impairs cytotoxic granule exocytosis in patient NK cells. J Clin Invest (2009) 119:3765–73. doi:10.1172/JCI40732
72. Zur Stadt U, Beutel K, Kolberg S, Schneppenheim R, Kabisch H, Janka G, et al. Mutation spectrum in children with primary hemophagocytic lymphohistiocytosis: molecular and functional analyses of PRF1, UNC13D, STX11, and RAB27A. Hum Mutat (2006) 27:62–8. doi:10.1002/humu.20274
73. Bryceson YT, Rudd E, Zheng C, Edner J, Ma D, Wood SM, et al. Defective cytotoxic lymphocyte degranulation in syntaxin-11 deficient familial hemophagocytic lymphohistiocytosis 4 (FHL4) patients. Blood (2007) 110:1906–15. doi:10.1182/blood-2007-02-074468
74. Marcet-Palacios M, Odemuyiwa SO, Coughlin JJ, Garofoli D, Ewen C, Davidson CE, et al. Vesicle-associated membrane protein 7 (VAMP-7) is essential for target cell killing in a natural killer cell line. Biochem Biophys Res Commun (2008) 366:617–23. doi:10.1016/j.bbrc.2007.11.079
75. Loo LS, Hwang LA, Ong YM, Tay HS, Wang CC, Hong W. A role for endobrevin/VAMP8 in CTL lytic granule exocytosis. Eur J Immunol (2009) 39:3520–8. doi:10.1002/eji.200939378
76. Krzewski K, Gil-Krzewska A, Watts J, Stern JN, Strominger JL. VAMP4- and VAMP7-expressing vesicles are both required for cytotoxic granule exocytosis in NK cells. Eur J Immunol (2011) 41:3323–9. doi:10.1002/eji.201141582
77. Ménager MM, Ménasché G, Romao M, Knapnougel P, Ho CH, Garfa M, et al. Secretory cytotoxic granule maturation and exocytosis require the effector protein hMunc13-4. Nat Immunol (2007) 8:257–67. doi:10.1038/ni1431
78. Pores-Fernando AT, Zweifach A. Calcium influx and signaling in cytotoxic T-lymphocyte lytic granule exocytosis. Immunol Rev (2009) 231:160–73. doi:10.1111/j.1600-065X.2009.00809.x
79. Tassi I, Colonna M. The cytotoxicity receptor CRACC (CS-1) recruits EAT-2 and activates the PI3K and phospholipase Cgamma signaling pathways in human NK cells. J Immunol (2005) 175:7996–8002.
80. Maul-Pavicic A, Chiang SC, Rensing-Ehl A, Jessen B, Fauriat C, Wood SM, et al. ORAI1-mediated calcium influx is required for human cytotoxic lymphocyte degranulation and target cell lysis. Proc Natl Acad Sci U S A (2011) 108:3324–9. doi:10.1073/pnas.1013285108
81. Galandrini R, Micucci F, Tassi I, Cifone MG, Cinque B, Piccoli M, et al. Arf6: a new player in FcgammaRIIIA lymphocyte-mediated cytotoxicity. Blood (2005) 106:577–83. doi:10.1182/blood-2004-10-4100
82. Fowler KT, Andrews NW, Huleatt JW. Expression and function of synaptotagmin VII in CTLs. J Immunol (2007) 178:1498–504.
83. Capuano C, Paolini R, Molfetta R, Frati L, Santoni A, Galandrini R. PIP2-dependent regulation of Munc13-4 endocytic recycling: impact on the cytolytic secretory pathway. Blood (2012) 119:2252–62. doi:10.1182/blood-2010-12-324160
84. Liu D, Bryceson YT, Meckel T, Vasiliver-Shamis G, Dustin ML, Long EO. Integrin-dependent organization and bidirectional vesicular traffic at cytotoxic immune synapses. Immunity (2009) 31:99–109. doi:10.1016/j.immuni.2009.05.009
85. Li P, Zheng G, Yang Y, Zhang C, Xiong P, Xu Y, et al. Granzyme B is recovered by natural killer cells via clathrin-dependent endocytosis. Cell Mol Life Sci (2010) 67:3197–208. doi:10.1007/s00018-010-0377-8
86. Bhat R, Watzl C. Serial killing of tumor cells by human natural killer cells – enhancement by therapeutic antibodies. PLoS One (2007) 2:e326. doi:10.1371/journal.pone.0000326
87. Choi PJ, Mitchison TJ. Imaging burst kinetics and spatial coordination during serial killing by single natural killer cells. Proc Natl Acad Sci U S A (2013) 110:6488–93. doi:10.1073/pnas.1221312110
88. Vanherberghen B, Olofsson PE, Forslund E, Sternberg-Simon M, Khorshidi MA, Pacouret S, et al. Classification of human natural killer cells based on migration behavior and cytotoxic response. Blood (2013) 121:1326–34. doi:10.1182/blood-2012-06-439851
89. Isaaz S, Baetz K, Olsen K, Podack E, Griffiths GM. Serial killing by cytotoxic T lymphocytes: T cell receptor triggers degranulation, re-filling of the lytic granules and secretion of lytic proteins via a non-granule pathway. Eur J Immunol (1995) 25:1071–9. doi:10.1002/eji.1830250432
90. Di Paolo G, Moskowitz HS, Gipson K, Wenk MR, Voronov S, Obayashi M, et al. Impaired PtdIns(4,5)P2 synthesis in nerve terminals produces defects in synaptic vesicle trafficking. Nature (2004) 431:415–22. doi:10.1038/nature02896
91. Arneson LN, Segovis CM, Gomez TS, Schoon RA, Dick CJ, Lou Z, et al. Dynamin 2 regulates granule exocytosis during NK cell-mediated cytotoxicity. J Immunol (2008) 181:6995–7001.
92. Guia S, Jaeger BN, Piatek S, Mailfert S, Trombik T, Fenis A, et al. Confinement of activating receptors at the plasma membrane controls natural killer cell tolerance. Sci Signal (2011) 4:ra21. doi:10.1126/scisignal.2001608
Keywords: NK cell, CTL, cytotoxicity, cytolytic synapse, signal transduction
Citation: Galandrini R, Capuano C and Santoni A (2013) Activation of lymphocyte cytolytic machinery: where are we? Front. Immunol. 4:390. doi: 10.3389/fimmu.2013.00390
Received: 01 October 2013; Paper pending published: 15 October 2013;
Accepted: 06 November 2013; Published online: 19 November 2013.
Edited by:
Konrad Krzewski, National Institutes of Health, USAReviewed by:
Jordan Orange, Baylor College of Medicine, USAEric O. Long, National Institute of Allergy and Infectious Diseases, USA
Copyright: © 2013 Galandrini, Capuano and Santoni. This is an open-access article distributed under the terms of the Creative Commons Attribution License (CC BY). The use, distribution or reproduction in other forums is permitted, provided the original author(s) or licensor are credited and that the original publication in this journal is cited, in accordance with accepted academic practice. No use, distribution or reproduction is permitted which does not comply with these terms.
*Correspondence: Ricciarda Galandrini, Department of Experimental Medicine, Sapienza University, viale Regina Elena, 324, 00161 Rome, Italy e-mail:cmljY2lhcmRhLmdhbGFuZHJpbmlAdW5pcm9tYTEuaXQ=