- 1Human Cellular Therapy Laboratory, Division of Transfusion Medicine, Department of Laboratory Medicine and Pathology, Mayo Clinic, Rochester, MN, USA
- 2Division of Hematology, Department of Medicine, Mayo Clinic, Rochester, MN, USA
Dendritic cells are an important target in cancer immunotherapy based on their critical role in antigen presentation and response to tumor development. The capacity of dendritic cells to stimulate anti-tumor immunity has led investigators to use these cells to mediate anti-tumor responses in a number of clinical trials. However, these trials have had mixed results. The typical method for generation of ex vivo dendritic cells starts with the purification of CD14+ cells. Our studies identified a deficiency in the ability to generate mature dendritic cell using CD14+ cells from cancer patients that corresponded with an increased population of monocytes with altered surface marker expression (CD14+HLA-DRlo/neg). Further studies identified systemic immune suppression and increased concentrations of CD14+HLA-DRlo/neg monocytes capable of inhibiting T-cell proliferation and DC maturation. Together, these findings strongly suggest that protocols aimed at immune stimulation via monocytes/dendritic cells, if optimized on normal monocytes or in systems without these suppressive monocytes, are unlikely to engender effective DC maturation in vitro or efficiently trigger DC maturation in vivo. This highlights the importance of developing optimal protocols for stimulating DCs in the context of significantly altered monocyte phenotypes often seen in cancer patients.
Dendritic Cells as Cancer Vaccines
Dendritic cells are potent signal transducers in the immune system. These cells present antigen, are the essential bridge between the innate and adaptive arms of the immune system, and serve as regulators to modulate immune response to pathogenic invasion, tissue injury, and tumor development. As such, dendritic cells have received significant focus as a promising vehicle for the development of vaccines for cancer immunotherapy. We now have a more complete understanding of DC ontology with the realization that DCs exist in diverse subsets, all capable of activating T cells but possessing unique functions. DCs are classified into two broad categories. The first are monocyte-derived DCs resulting from stimulation due to inflammation or infection. The second category are steady-state DCs which include resident CD8+ DCs located in the thymus, resident CD8− DCs in the spleen, plasmacytoid DCs (pDCs), migratory DCs, and Langerhans cells [reviewed in Ref. (1, 2)]. Each of these classes of DCs has been demonstrated to play a key role in immune surveillance and response but for the purpose of DC-based vaccines for immunotherapy in cancer, the focus has been on CD14+ monocyte-derived DCs.
The in vivo pathways associated with the development of dendritic cells from monocyte precursors and the mechanisms and consequences of pathogenic activation have been described (3, 4). Briefly, DCs arise from monocyte progenitors into an immature state (iDC) responsible for immune surveillance via pathogen detection. Once activated, iDC further differentiate into mature dendritic cells (mDC) and travel to lymph nodes to activate the adaptive (typically T- and B-cell responses) and innate immune response (5). DCs also play a role in limiting the immune response against self antigen (self-tolerance) as well as limiting response to tissue damage in the absence of pathogenic signals (6). iDC can suppress immunity and have been shown to be capable of eliminating antigen-specific T cells (7). Restriction of the capacity of iDC to differentiate into mDC has been a mechanism used by viruses, parasites, and bacteria to maintain a state of self-tolerance and to enable microbial pathology (8–10). Thus, manipulation and maintenance of a state of iDC with a block on the ability to differentiate into mDC is a key mechanism of immune suppression.
To generate mDC in vitro for clinical use, the CD14+ monocytes are the preferred precursor due to their abundance and ease of collection. CD14+ monocytes are purified from mononuclear cells via adherence to plastic, antibody selection, or size centrifugation and used as source material to differentiate DC. To drive the immune response, the DCs are pulsed with tumor antigens in the form of peptides, RNA, or lysates derived from whole tumors or cell lines (11, 12). Additionally, viruses can be a potent mechanism to deliver tumor antigens (13–15). Manufacturing methods reported among clinical trials vary greatly. As a variety of methods with subtle optimizations of DC cultures have been published, there are few constants (DC activation state, tumor source, patient status, underlying disease etc.) that allow useful comparisons between the growing numbers of trials and the underlying methods and characteristics used to generate and describe the drug (in this case DC). Often, this key aspect of drug development (optimizing and describing the purity and potency of the drug) is overlooked. However, one constant regarding the majority of the trials remains; that is the use of CD14+ cells as a starting material.
Monocyte Precursors of DC are Often Altered in Cancer Patients and are Immune Suppressive
In our efforts to establish a DC vaccine protocol, we worked to optimize the maturation of DCs in cancer patients. During those studies, we discovered that in many patients, CD14+-isolated monocytes were incapable of differentiation into mDC using standard DC generation protocols (16–18). This result that monocytes from cancer patients were potentially altered in their capacity to differentiate into DC, led us to search for correlative markers. We identified an increased population of monocytes with an altered surface marker expression (CD14+HLA-DRlo/neg) in a number of malignancies (16–20) (Figure 1A). This phenotype has also been reported by others in melanoma (21–23), bladder cancer (24), non-small cell lung cancer (25), and hepatocellular cancer (26, 27). Our studies in glioblastoma identified evidence of systemic immune suppression and increased concentrations of CD14+HLA-DRlo/neg monocytes capable of inhibiting T-cell proliferation and DC maturation that could also be re-capitulated in vitro co-culture systems using tumor cell lines (18). These same immunosuppressive monocytes have been characterized with increased populations in bladder carcinoma that significantly correlate with decreased T-cell proliferation and IFN-γ production (24). These cells suppress immune function in multiple ways (Table 1), and therefore must be considered for any approach to DC vaccine strategies.
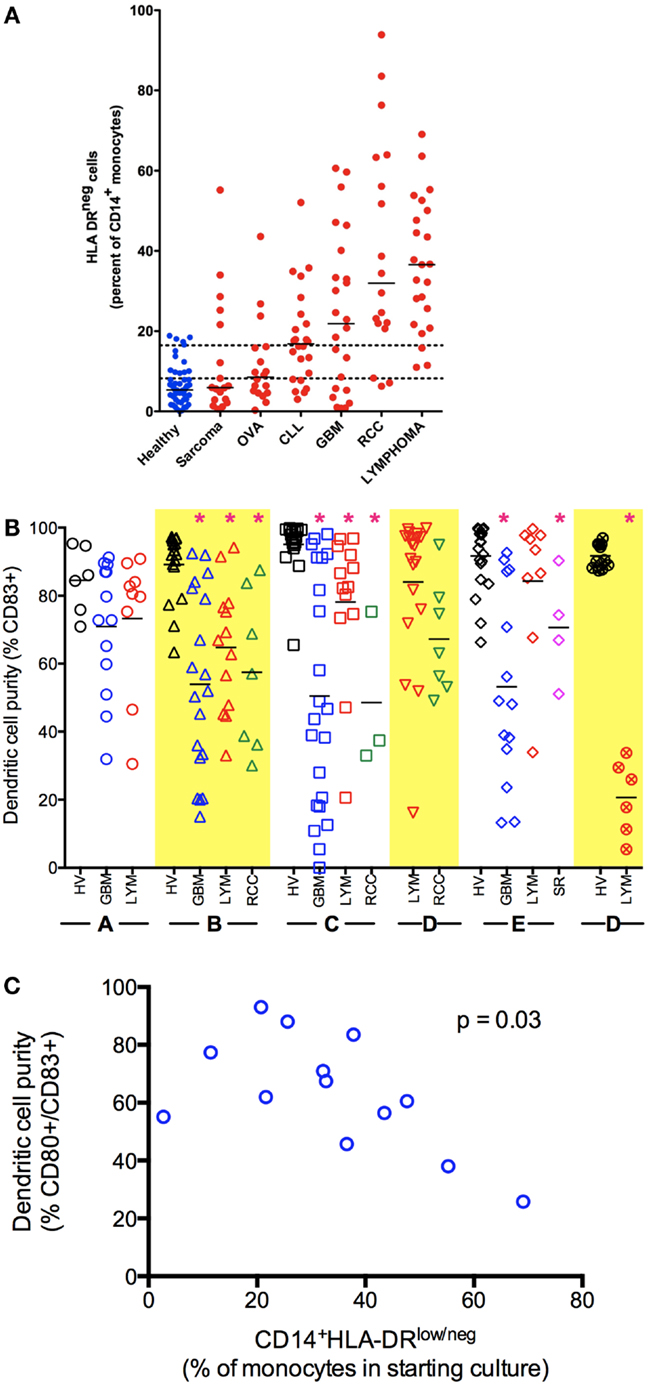
Figure 1. Monocyte and dendritic cell defects in cancer. (A) Cancer patients have an increased percentage of CD14+HLA-DRlo/neg monocytes in circulation. Peripheral blood of healthy volunteers and cancer patients was analyzed by flow cytometry for immune phenotype. (B) Monocytes from cancer patients have decreased capacity to differentiate to mDC (CD83+) under a variety of stimulation conditions. Monocytes were selected from blood of healthy volunteers (HV) or cancer patients (GBM, glioblastoma multiforme LYM, B-cell lymphoma; RCC, renal cell carcinoma; SR, sarcoma) by CD14+ immunomagnetic beads and cultured under different methods as labeled in X axis. Method A, fast-DC (28); B, ex vivo media with 5 days culture as described (29, 30); C, 5 days culture in StemLine media and GM-CSF, maturation factors TNFα and PGE2 added in the last 2 days of culture; D, method C with IL-4 added for 5 days of culture; E, method D with poly I:C added to maturation factors; F, method D with CpG used as maturation factor in place of TNF-α (*p < 0.05). (C) Decreased generation of mDC correlates with increased percentage of CD14+HLA-DRlo/neg in the monocytes selected for culture (Method B).
There is also mounting evidence that correlates increased concentrations of CD14+HLA-DRlo/neg monocytes in patients with poor clinical outcome. Populations of CD14+HLA-DRlo/neg monocytes and TGF-β levels were significantly expanded in metastatic melanoma patients as compared to healthy donors and correlated to a lack of response to administered granulocyte–macrophage colony-stimulation factor (GM-CSF) vaccine (22). Increased CD14+HLA-DRlo/neg monocytes correlated to both extrathoracic metastasis and poor response to chemotherapy in non-small lung cancer patients (25). Increased CD14+HLA-DRlo/neg monocytes are associated with more aggressive disease and poorer prognosis in lymphoma (16) and hepatocellular carcinoma (40). Increased CD14+HLA-DRlo/neg monocytes were associated with decreased time to progression in patients with chronic lymphocytic leukemia (CLL) (19). Increased CD14+HLA-DRlo/neg monocytes and decreased CD4+ T cells can predict poor overall survival across a number of malignancies (20).
The finding that CD14+HLA-DRlo/neg monocytes are detectable systemically in patients with a variety of malignancies and that they are functionally immune suppressive raises important questions regarding their influence in ex vivo DC vaccine preparations. To address this, we have studied the effects of these altered monocytes on mDC generation across several cancer types using an ex vivo culture system. Briefly, CD14+ mononuclear cells were isolated from buffy coats or apheresis leukoreduction system chambers of normal donors using immunomagnetic selection (41). Control DCs were cultured with 1% human AB serum, stimulated with GM-CSF and IL-4 (base media) for 3 days when one-third volume of fresh base media was added. Non-adherent cells were collected on day 6, re-suspended in base media with the addition of tumor necrosis factor alpha (TNF-α) and prostaglandin E2 (PGE2) to mature the DCs. This recipe is based on a classic method of generation of mDCs (28, 29, 41). Alternatively, the system was modified using a serum-free media and changes in cytokines to generate mDCs (Figure 1B).
Cancer patients showed significant deficits in the ability to generate mDCs independent of the underlying tumor. There is also substantially more variability in the efficiency of DC generation using monocytes from cancer patients (Figure 1B). While we primarily used CD83 up-regulation as indicative of DC maturation, we also noted a lack of CD80 expression and specific functional deficient of these cells. Increased efficiency of DC maturation can be correlated with decreased presence of CD14+HLA-DRlo/neg monocytes in the starting culture (Figure 1C). However, we could consistently improve the ability to generate mDCs using serum-free methods with the addition of IL-4. Even so, it was difficult to recapitulate the efficient generation of mDCs we observed using monocytes from healthy volunteers compared to cancer patients. Knowing that CD14+HLA-DRlo/neg monocytes have significant capacity to influence ex vivo DC cultures implies that these cells and the pathways to both generate and eliminate them are high-value targets to improve cancer therapies. It is striking to note that these effects occurred in the complete absence of tumors and in the continual presence (for days) with the cells in excess cytokines. This deficit likely represents a significant block in the differentiation pathway. This strongly suggests that immune stimulation in vivo, even with precise targeting of the pathways known to convert mature DC, is unlikely to efficiently trigger mDC maturation in patients. Further understanding of the biology of these CD14+HLA-DRlo/neg monocytes is needed; strategies to overcome the effects of these cells can lead to better DC generation and immune reconstitution.
Implications of CD14+HLA-DRlo/neg on DC-Based Cancer Vaccines
Most currently active DC-based cancer immunotherapy protocols differ in either the cell source or some of the methods associated with the generation of DC. The most common approach has been the ex vivo generation of mature DCs from patient myeloid-derived monocyte precursors by co-culturing with GM-CSF and various cocktails of cytokines and TLR agonists to produce mature DCs. Optimizing DC culture conditions using normal healthy donors will likely not directly translate into the protocols needed for cancer patients. It will be important for those protocols that use CD14+ cells to generate their DC product from primary patient samples to confirm and optimize the manufacturing method and assure that potent DCs are being generated. In our hands, a serum-free method that includes IL-4 is a good starting point. Adequate sampling size of the patient population is needed to determine the range of differentiation efficiency in each specific cancer patient population to inform the design of release criteria for vaccine manufacturing. Our data also have clear implications for other approaches attempting to mediate anti-tumor immune stimulation. Adjuvants known to work in healthy people may not work in cancer patients if their approach is to target the DC or DC differentiation pathways.
As we improve our understanding of the importance of CD14+HLA-DRlo/neg monocytes in promoting immunosuppression, it is imperative that we adjust our clinical practices to ensure effective outcomes for patients using DC-based immunotherapy. This will require continued efforts to develop optimal protocols for generating ex vivo DC vaccine preparations and testing these protocols in individual patients. The complexity of the human immune system and individual tumor micro environments will likely require an element of individualized protocol development to achieve optimal clinical benefit.
Conflict of Interest Statement
The authors declare that the research was conducted in the absence of any commercial or financial relationships that could be construed as a potential conflict of interest.
References
1. Moore AJ, Anderson MK. Dendritic cell development: a choose-your-own-adventure story. Adv Hematol (2013) 2013:949513. doi: 10.1155/2013/949513
2. Merad M, Sathe P, Helft J, Miller J, Mortha A. The dendritic cell lineage: ontogeny and function of dendritic cells and their subsets in the steady state and the inflamed setting. Annu Rev Immunol (2013) 31:563–604. doi:10.1146/annurev-immunol-020711-074950
3. Chatterjee B, Smed-Sörensen A, Cohn L, Chalouni C, Vandlen R, Lee BC, et al. Internalization and endosomal degradation of receptor-bound antigens regulate the efficiency of cross presentation by human dendritic cells. Blood (2012) 120(10):2011–20. doi:10.1182/blood-2012-01-402370
4. Dudek AM, Martin S, Garg AD, Agostinis P. Immature, semi-mature, and fully mature dendritic cells: toward a DC-cancer cells interface that augments anticancer immunity. Front Immunol (2013) 4:438. doi:10.3389/fimmu.2013.00438
5. Cella M, Sallusto F, Lanzavecchia A. Origin, maturation and antigen presenting function of dendritic cells. Curr Opin Immunol (1997) 9(1):10–6. doi:10.1016/S0952-7915(97)80153-7
6. Steinman RM, Hawiger D, Liu K, Bonifaz L, Bonnyay D, Mahnke K, et al. Dendritic cell function in vivo during the steady state: a role in peripheral tolerance. Ann N Y Acad Sci (2003) 987:15–25. doi:10.1111/j.1749-6632.2003.tb06029.x
7. Dhodapkar MV, Steinman RM, Krasovsky J, Munz C, Bhardwaj N. Antigen-specific inhibition of effector T cell function in humans after injection of immature dendritic cells. J Exp Med (2001) 193(2):233–8. doi:10.1084/jem.193.2.233
8. De Santo C, Salio M, Masri SH, Lee LY, Dong T, Speak AO, et al. Invariant NKT cells reduce the immunosuppressive activity of influenza A virus-induced myeloid-derived suppressor cells in mice and humans. J Clin Invest (2008) 118(12):4036–48. doi:10.1172/JCI36264
9. Van Ginderachter JA, Beschin A, De Baetselier P, Raes G. Myeloid-derived suppressor cells in parasitic infections. Eur J Immunol (2010) 40(11):2976–85. doi:10.1002/eji.201040911
10. Martino A, Badell E, Abadie V, Balloy V, Chignard M, Mistou MY, et al. Mycobacterium bovis bacillus Calmette-Guerin vaccination mobilizes innate myeloid-derived suppressor cells restraining in vivo T cell priming via IL-1R-dependent nitric oxide production. J Immunol (2010) 184(4):2038–47. doi:10.4049/jimmunol.0903348
11. Palucka K, Banchereau J. Cancer immunotherapy via dendritic cells. Nat Rev Cancer (2012) 12(4):265–77. doi:10.1038/nrc3258
12. Palucka K, Banchereau J. Dendritic-cell-based therapeutic cancer vaccines. Immunity (2013) 39(1):38–48. doi:10.1016/j.immuni.2013.07.004
13. Kantoff PW, Schuetz TJ, Blumenstein BA, Glode LM, Bilhartz DL, Wyand M, et al. Overall survival analysis of a phase II randomized controlled trial of a poxviral-based PSA-targeted immunotherapy in metastatic castration-resistant prostate cancer. J Clin Oncol (2010) 28(7):1099–105. doi:10.1200/JCO.2009.25.0597
14. Marshall JL, Gulley JL, Arlen PM, Beetham PK, Tsang KY, Slack R, et al. Phase I study of sequential vaccinations with fowlpox-CEA(6D)-TRICOM alone and sequentially with vaccinia-CEA(6D)-TRICOM, with and without granulocyte-macrophage colony-stimulating factor, in patients with carcinoembryonic antigen-expressing carcinomas. J Clin Oncol (2005) 23(4):720–31. doi:10.1200/JCO.2005.10.206
15. Blalock LT, Landsberg J, Messmer M, Shi J, Pardee AD, Haskell R, et al. Human dendritic cells adenovirally-engineered to express three defined tumor antigens promote broad adaptive and innate immunity. Oncoimmunology (2012) 1(3):287–357. doi:10.4161/onci.18628
16. Lin Y, Gustafson MP, Bulur PA, Gastineau DA, Witzig TE, Dietz AB. Immunosuppressive CD14+HLA-DR(low)/- monocytes in B-cell non-Hodgkin lymphoma. Blood (2011) 117(3):872–81. doi:10.1182/blood-2010-05-283820
17. Vuk-Pavlovic S, Bulur PA, Lin Y, Qin R, Szumlanski CL, Zhao X, et al. Immunosuppressive CD14+HLA-DRlow/- monocytes in prostate cancer. Prostate (2010) 70(4):443–55. doi:10.1002/pros.21078
18. Gustafson MP, Lin Y, New KC, Bulur PA, O’Neill BP, Gastineau DA, et al. Systemic immune suppression in glioblastoma: the interplay between CD14+HLA-DRlo/neg monocytes, tumor factors, and dexamethasone. Neuro Oncol (2010) 12(7):631–44. doi:10.1093/neuonc/noq001
19. Gustafson MP, Abraham RS, Lin Y, Wu W, Gastineau DA, Zent CS, et al. Association of an increased frequency of CD14+ HLA-DR lo/neg monocytes with decreased time to progression in chronic lymphocytic leukaemia (CLL). Br J Haematol (2012) 156(5):674–6. doi:10.1111/j.1365-2141.2011.08902.x
20. Gustafson MP, Lin Y, LaPlant B, Liwski CJ, Maas ML, League SC, et al. Immune monitoring using the predictive power of immune profiles. J Immunother Cancer (2013) 1(7): doi:10.1186/2051-1426-1-7
21. Meyer C, Cagnon L, Costa-Nunes CM, Baumgaertner P, Montandon N, Leyvraz L, et al. Frequencies of circulating MDSC correlate with clinical outcome of melanoma patients treated with ipilimumab. Cancer Immunol Immunother (2014) 63(3):247–57. doi:10.1007/s00262-013-1508-5
22. Filipazzi P, Valenti R, Huber V, Pilla L, Canese P, Iero M, et al. Identification of a new subset of myeloid suppressor cells in peripheral blood of melanoma patients with modulation by a granulocyte-macrophage colony-stimulation factor-based antitumor vaccine. J Clin Oncol (2007) 25(18):2546–53. doi:10.1200/JCO.2006.08.5829
23. Poschke I, Mougiakakos D, Hansson J, Masucci GV, Kiessling R. Immature immunosuppressive CD14+HLA-DR-/low cells in melanoma patients are Stat3hi and overexpress CD80, CD83, and DC-sign. Cancer Res (2010) 70(11):4335–45. doi:10.1158/0008-5472.CAN-09-3767
24. Yuan XK, Zhao XK, Xia YC, Zhu X, Xiao P. Increased circulating immunosuppressive CD14(+)HLA-DR(-/low) cells correlate with clinical cancer stage and pathological grade in patients with bladder carcinoma. J Int Med Res (2011) 39(4):1381–91. doi:10.1177/147323001103900424
25. Huang A, Zhang B, Wang B, Zhang F, Fan KX, Guo YJ. Increased CD14(+)HLA-DR (-/low) myeloid-derived suppressor cells correlate with extrathoracic metastasis and poor response to chemotherapy in non-small cell lung cancer patients. Cancer Immunol Immunother (2013) 62(9):1439–51. doi:10.1007/s00262-013-1450-6
26. Arihara F, Mizukoshi E, Kitahara M, Takata Y, Arai K, Yamashita T, et al. Increase in CD14+HLA-DR -/low myeloid-derived suppressor cells in hepatocellular carcinoma patients and its impact on prognosis. Cancer Immunol Immunother (2013) 62(8):1421–30. doi:10.1007/s00262-013-1447-1
27. Hoechst B, Ormandy LA, Ballmaier M, Lehner F, Krüger C, Manns MP, et al. A new population of myeloid-derived suppressor cells in hepatocellular carcinoma patients induces CD4(+)CD25(+)Foxp3(+) T cells. Gastroenterology (2008) 135(1):234–43. doi:10.1053/j.gastro.2008.03.020
28. Alldawi L, Takahashi M, Narita M, Ayres F, Tsukada N, Osman Y, et al. Effect of prostaglandin E2, lipopolysaccharide, IFN-gamma and cytokines on the generation and function of fast-DC. Cytotherapy (2005) 7(2):195–202. doi:10.1080/14653240510018127
29. Jonuleit H, Kühn U, Müller G, Steinbrink K, Paragnik L, Schmitt E, et al. Pro-inflammatory cytokines and prostaglandins induce maturation of potent immunostimulatory dendritic cells under fetal calf serum-free conditions. Eur J Immunol (1997) 27(12):3135–42. doi:10.1002/eji.1830271209
30. Sallusto F, Lanzavecchia A. Efficient presentation of soluble antigen by cultured human dendritic cells is maintained by granulocyte/macrophage colony-stimulating factor plus interleukin 4 and downregulated by tumor necrosis factor alpha. J Exp Med (1994) 179(4):1109–18. doi:10.1084/jem.179.4.1109
31. Yu J, Du W, Yan F, Wang Y, Li H, Cao S, et al. Myeloid-derived suppressor cells suppress antitumor immune responses through IDO expression and correlate with lymph node metastasis in patients with breast cancer. J Immunol (2013) 190(7):3783–97. doi:10.4049/jimmunol.1201449
32. Mougiakakos D, Jitschin R, von Bahr L, Poschke I, Gary R, Sundberg B, et al. Immunosuppressive CD14+HLA-DRlow/neg IDO+ myeloid cells in patients following allogeneic hematopoietic stem cell transplantation. Leukemia (2013) 27(2):377–88. doi:10.1038/leu.2012.215
33. Maeda A, Kawamura T, Ueno T, Usui N, Miyagawa S. Monocytic suppressor cells derived from human peripheral blood suppress xenogenic immune reactions. Xenotransplantation (2014) 21(1):46–56. doi:10.1111/xen.12067
34. Zea AH, Rodriguez PC, Atkins MB, Hernandez C, Signoretti S, Zabaleta J, et al. Arginase-producing myeloid suppressor cells in renal cell carcinoma patients: a mechanism of tumor evasion. Cancer Res (2005) 65(8):3044–8. doi:10.1158/0008-5472.CAN-045-4505
35. Poschke I, Mao Y, Adamson L, Salazar-Onfray F, Masucci G, Kiessling R. Myeloid-derived suppressor cells impair the quality of dendritic cell vaccines. Cancer Immunol Immunother (2012) 61(6):827–38. doi:10.1007/s00262-011-1143-y
36. Kusmartsev S, Nefedova Y, Yoder D, Gabrilovich DI. Antigen-specific inhibition of CD8+ T cell response by immature myeloid cells in cancer is mediated by reactive oxygen species. J Immunol (2004) 172(2):989–99.
37. Kusmartsev S, Eruslanov E, Kübler H, Tseng T, Sakai Y, Su Z, et al. Oxidative stress regulates expression of VEGFR1 in myeloid cells: link to tumor- induced immune suppression in renal cell carcinoma. J Immunol (2008) 181(1):346–53.
38. Srivastava MK, Sinha P, Clements VK, Rodriguez P, Ostrand-Rosenberg S. Myeloid-derived suppressor cells inhibit T-cell activation by depleting cystine and cysteine. Cancer Res (2010) 70(1):68–77. doi:10.1158/0008-5472.CAN-09-2587
39. Ostrand-Rosenberg S. Myeloid-derived suppressor cells: more mechanisms for inhibiting antitumor immunity. Cancer Immunol Immunother (2010) 59(10):1593–600. doi:10.1007/s00262-010-0855-8
40. Shen P, Wang A, He M, Wang Q, Zheng S. Increased circulating Lin CD33 HLA-DR myeloid-derived suppressor cells in hepatocellular carcinoma patients. Hepatol Res (2013). doi:10.1111/hepr.12167
Keywords: CD14+HLA-DRlo/neg, MDSC, dendritic cells, immunotherapy, monocytes
Citation: Laborde RR, Lin Y, Gustafson MP, Bulur PA and Dietz AB (2014) Cancer vaccines in the world of immune suppressive monocytes (CD14+HLA-DRlo/neg cells): the gateway to improved responses. Front. Immunol. 5:147. doi: 10.3389/fimmu.2014.00147
Received: 10 January 2014; Accepted: 21 March 2014;
Published online: 04 April 2014.
Edited by:
Lisa Helene Butterfield, University of Pittsburgh, USAReviewed by:
Geanncarlo Lugo-Villarino, Centre National de la Recherche Scientifique, FranceMatthew Collin, Newcastle University, UK
Copyright: © 2014 Laborde, Lin, Gustafson, Bulur and Dietz. This is an open-access article distributed under the terms of the Creative Commons Attribution License (CC BY). The use, distribution or reproduction in other forums is permitted, provided the original author(s) or licensor are credited and that the original publication in this journal is cited, in accordance with accepted academic practice. No use, distribution or reproduction is permitted which does not comply with these terms.
*Correspondence: Allan B. Dietz, Human Cellular Therapy Laboratory, Department of Laboratory Medicine and Pathology, Division of Transfusion Medicine, Mayo Clinic, 200 First Street Southwest, Rochester, MN 55905, USA e-mail:ZGlldHouYWxsYW5AbWF5by5lZHU=