- 1Department of Health, Research Institute for Tropical Medicine, Manila, Philippines
- 2Center for International Health Research, Rhode Island Hospital, Brown University Medical School, Providence, RI, USA
- 3Department of Pediatrics, Rhode Island Hospital, Brown University Medical School, Providence, RI, USA
- 4Department of Pathology and Laboratory Medicine, Rhode Island Hospital, Brown University Medical School, Providence, RI, USA
Schistosomiasis, caused by three principal species of diecious trematodes (flatworms), currently afflicts over 250 million individuals, results in an estimated 2–15% chronic disability, and contributes to poor health and economic stagnation in endemic areas. Although schistosomiasis is effectively treated with praziquantel, rapid reinfection with rebound morbidity precludes effective control based on chemotherapy alone and justifies current efforts to develop vaccines for these parasites. Paramyosin (Pmy), an invertebrate muscle-associated protein, has emerged as a promising vaccine candidate for both Schistosoma mansoni and Schistosoma japonicum. Herein, we discuss the discovery of Pmy, its development as a vaccine candidate in rodents and bovines, as well as studies of naturally occurring immune responses to Pmy in prospective, observational human studies. We conclude with a proposed developmental plan to move Pmy toward Phase I clinical trials.
Schistosomiasis, caused by three principle species of diecious trematodes (flatworms), currently afflicts over 250 million people worldwide (1, 2) and results in 1.53 million DALYs lost per annum (3), although this is likely a considerable underestimate. A recent reassessment of the global burden of schistosomiasis suggests that the actual health burden is 4–30 times greater than the previous WHO estimate (1, 4). Infection is characterized by the presence of adult worms within the portal and mesenteric veins for Schistosoma japonicum and Schistosoma mansoni or within the veins draining the urinary bladder for S. haematobium. Chronic infection results in reduced childhood growth and nutritional status, anemia, hepatosplenomegaly, hepatic or urinary bladder fibrosis, and bleeding esophageal varices. End organ and systemic pathology result from granulomatous inflammation and fibrosis induced by parasite eggs trapped in host tissues.
Efforts to mitigate the impact of schistosomiasis in endemic regions have focused on chemotherapy of active infections with praziquantel (PZQ), control of intermediate snail hosts, and improved sanitation.
In longitudinal cohort studies in the Philippines, we have demonstrated that improvements in nutritional status and hemoglobin levels associated with PZQ treatment are transitory; both nutritional status and hemoglobin returned to their pre-treatment morbid levels within 9–12 months (5). In addition, “rebound” morbidity, in which repeated cycles of annual treatment and reinfection result in more aggressive morbidity, has been described (6). Therefore, annual treatment programs with PZQ are insufficient to reverse these morbidities, while resource requirements to shorten the treatment interval are prohibitive. Because current control strategies employing chemotherapy with PZQ have not reduced transmission and morbidity to acceptable levels, there is an urgent need for complementary approaches, such as vaccines for schistosomiasis control.
Discovery of Paramyosin
In S. mansoni, Sher and colleagues demonstrated that mice vaccinated intradermally with schistosomula or adult worm extracts adjuvanted with BCG were protected from cercarial challenge (7). The sera from these mice were strikingly monospecific, recognizing a 97 kDa antigen in adult worm extracts and immunoprecipitating a 97 kDa antigen from detergent extracts of metabolically labeled worms (8). Size-fractionation analysis of adult worm extracts indicated that only fractions containing a protease sensitive 97 kDa antigen induced protection in this model (7). Monoclonal antibodies against Sm97 were developed from mice vaccinated intradermally with adult worm extract and BCG (8). Indirect immunofluorescence using monoclonal anti-Sm97 localized the antigen to regions just below the tegument and in the gut syncitia of adult worms. Sm97 was weakly recognized by chronically infected mouse sera and not recognized by sera from mice infected with irradiated cercariae (8). Immunoaffinity purified Sm97 was shown to elicit delayed-type hypersensitivity in intradermally vaccinated mice, suggesting that this molecule is also capable of evoking cell-mediated responses.
A rabbit anti-Sm97 serum immunoprecipitated a 97 kDa antigen from in vitro translation products of adult worm mRNA and identified a 1317 base pair clone, which encoded approximately 50% of the native protein (9). The clone had 36% homology with nematode myosin, but was designated paramyosin based on amino acid composition and cross reactivity of anti-Sm97 sera with other native paramyosins. Structural analysis of the deduced amino acid sequence and electron microscopy of purified schistosome paramyosin indicated that the protein adopts an alpha helical coiled-coil conformation with a seven residue repeat (10).
Paramyosin was localized to the contents of membrane bound elongate bodies within the tegument and subtegumental cell bodies using immunoelectron gold microscopy and polyclonal anti-paramyosin antisera (11). Paramyosin was in a non-filamentous form within these elongate bodies and staining was rarely observed in the thick filaments of cortical muscle.
A full length cDNA clone of S. mansoni paramyosin was sequenced and revealed significant homology to antigen B of Taenia solium and the IgG Fc γ-binding protein of Taenia crassiceps (12, 13). These homologous proteins bind the Fc region of IgG and collagen, and inhibit complement activation. Based on these homologies, schistosome paramyosin was shown to inhibit complement C1 and C9, and to bind polymeric collagen, and IgG (14–17). These immune-related properties may explain the tegumental localization of an otherwise muscle associated protein.
Murine Protection Studies in S. mansoni
Passive transfer of monoclonal or polyclonal anti-paramyosin antibodies did not protect mice from cercarial challenge (18); however, immunization with 4 μg of native or 40 μg of a partial recombinant paramyosin fragment with BCG induced 39 and 26% protection, respectively. These data suggested that protection induced by paramyosin was cell mediated and not antibody dependent. Schistosome myosin and heterologous nematode paramyosin did not induce protection, suggesting the requirement of schistosome paramyosin specific epitopes for protection. In other experiments, 5 μg of native paramyosin induced 24–53% protection in mice without adjuvant (19).
Native and partial recombinant paramyosin stimulated IFN-γ production and macrophage killing of schistosomula in vaccinated mice. In addition, splenocytes from mice vaccinated with paramyosin/BCG were stimulated to produce IFN-γ by 3-h-old and 7-day-old schistosomula in a dose dependent fashion (18).
Pmy Development in S. japonicum
In contradistinction to the S. mansoni model, a mouse monoclonal IgE antibody, subsequently demonstrated to recognize paramyosin (20), was shown to confer 19–58% protection against S. japonicum cercarial challenge following passive transfer (21, 22). In addition, the monoclonal stimulated eosinophill-mediated killing of schistosomula in vitro (22).
To determine if paramyosin would also induce protection against S. japonicum, we biochemically purified paramyosin from S. japonicum adult worms. SDS-PAGE demonstrated a single protein with a molecular weight of 97 kDa. In four separate experiments, vaccination of mice with S. japonicum paramyosin without adjuvant induced significant resistance (62–86%, p < 0.001) against cercarial challenge as compared to controls. These data suggest that S. japonicum paramyosin represents a promising candidate vaccine (23). Paramyosin has also been evaluated as a component of a multi-antigen DNA-based vaccine in mice; however, delivery in this format has not augmented the level of protection seen with recombinant or biochemically purified native paramyosin (24).
Based on these encouraging protection data, McManus and colleagues cloned the full length paramyosin gene from an S. japonicum cDNA library probed with a hyperimmune rabbit sera (25), while Nara et al. independently isolated the full-length cDNA of Sj97 by screening a distinct library using a mouse monoclonal IgE antibody, which recognizes a 97 kDa surface molecule on S. japonicum larvae (26). Alignment of the predicted amino acid sequences of S. mansoni and S. japonicum paramyosins revealed 95% identity.
Sj97 is the Target of Protective Th2 Biased Cytokine Responses in Humans
We conducted a longitudinal treatment–reinfection study design with 616 S. japonicum infected participants, 7–30 years of age. We evaluated the relationship between cytokine responses made by PMBCs in response to S. japonicum soluble adult worm extract (SWAP), Sj97, Sj67, and Sj22.6, measured 4 weeks after treatment with PZQ, and resistance to reinfection in a population from Leyte, The Philippines. Reinfection was measured every 3 months for a total of 18 months by duplicate Kato–Katz assessment on three stools per person per timepoint. We employed repeated measures models adjusted for both repeated measures within person, clustering by household and several potential confounders of reinfection intensity including: directly observed water contact, socio-economic status, age, sex, village, and baseline intensity of infection.
Schistosoma japonicum transmission was high: 54.8 and 91.1% were reinfected within 6 and 18 months, respectively. A Th2 bias in the following cytokine ratios, IL-5/IL-12, IL-13/IL-12, IL-4/IFN-g, IL-5/IFN-γ, and IL-13/IFN-γ, in response to Sj97, predicted a 30–41% lower intensity of reinfection (all p < 0.05) after adjustment for potential confounders. An example of one of these protective relationships (for IL-13/IFN-γ ratio) is presented in Figure 1A (27). Similar results were found for responses to SWAP.
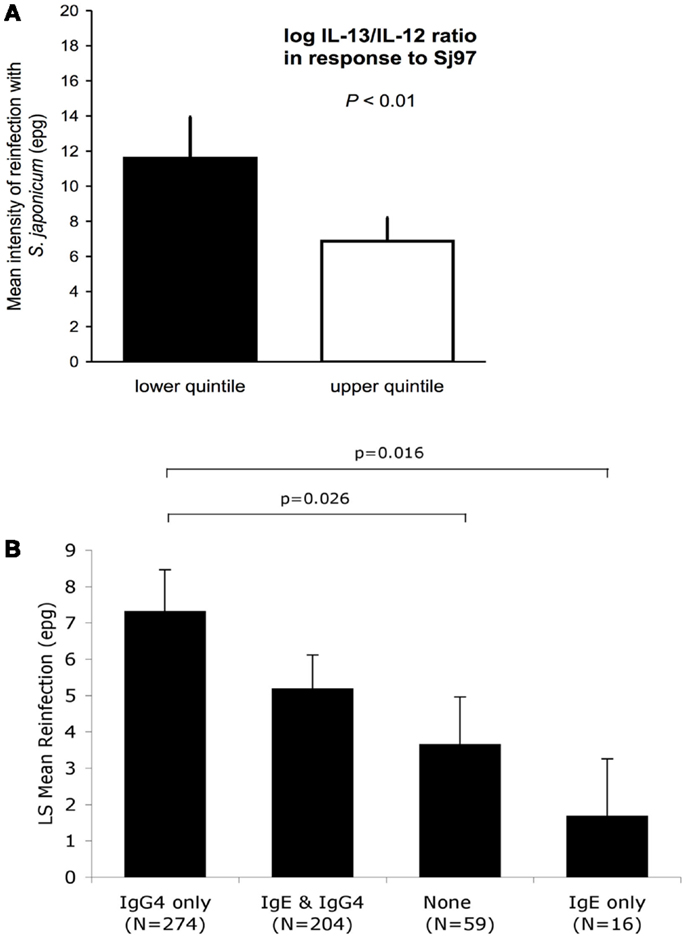
Figure 1. (A) Intensity of reinfection with S. japonicum 18 months after treatment with PZQ, as predicted by cytokine ratios in responses to SWAP (N = 493). White and black bars represent the back-transformed LS mean intensity of reinfection for the upper and lower quintile of IL13/IL12 log cytokine-ratio distribution. Error bars represent SEs. LS mean estimates, SEs, and p-values for difference in means between quintiles are adjusted for confounders and clustering. Adapted from Ref. (26). (B) IgE responses to rSj97 (paramyosin) predict resistance to S. japonicum reinfection at 12 months post-treatment, and are attenuated by lgG4. Least square (LS) means represent the mean reinfection egg burden after adjusting for potential confounders and clustering by household in a repeated measures model using the combined Sj97 IgE and lgG4 response variable (p = 0.023 for time by combined lgE–lgG4 variable interaction). Confounders in this model include age, gender, village of residence, exposure, and baseline intensity. p-values are for detailed comparisons between “lgG4 only” and the rest of the groups. Error bars represent SEs. Reprinted from Ref. (27).
Sj97 is the Target of Protective IgE Responses in Humans
Using the same study population and analytic approach described for the cytokine analyses, we have evaluated the relationship between antibodies to rSj97 and resistance to reinfection in humans. We obtained serum 4 weeks post-treatment and measured anti-rSj97 IgE, IgA, and IgG subclass specific antibody levels using a bead-based assay. Responders were defined based on the mean +2 SD of the fluorescence values obtained in a group of 10 unexposed North American controls. In repeated measures models, individuals with IgE but not IgG4 responses to rSj97 had a 77% lower intensity of reinfection at 12 months compared to individuals with IgG4 but not IgE responses, even after adjusting for potential confounders including directly observed water contact, village, age, sex, and baseline intensity of infection (p = 0.016) (28), see Figure 1B.
Bovine Vaccine Trials
Using partially purified rSj97, McManus et al. (29) demonstrated that Chinese buffalo immunized with rSj97 in Quill A developed specific anti-Sj97 antibodies and had 34% fewer worms after laboratory challenge compared to controls. Importantly, the authors also acknowledged that, “whereas the yields of rec-Sj97 are sufficient for small trials, the expression levels are very low and are inadequate for large-scale use.”
Pilot Scale Expression, Purification, and Lyophilization of rSj97
As indicated above, recombinant Sj97 has not been successfully produced at pilot scale despite considerable efforts (29, 30). After discovering that Th2 and IgE responses to Sj97 were associated with marked resistance to reinfection in humans, we have focused our efforts on overcoming this scale-up challenge (31). Our typical yield from a single 10 L fermentation is 600 lyophilized vials at >0.250 mg rSj97/vial.
Characterization of rSj97
We have conducted extensive functional, biochemical, biophysical, and immunologic analyses on rSj97 to demonstrate that our recombinant protein is free of concerning contaminants, is correctly folded, and has appropriate functional properties. Electrophoretic mobility and purity (Figure 2), sterility, endotoxin level (by FDA approved test), residual SDS concentration, identity (LC-MS), secondary and tertiary structural analysis (CD), collagen binding, IgG binding, and stability (>12 months) gave expected results (31).
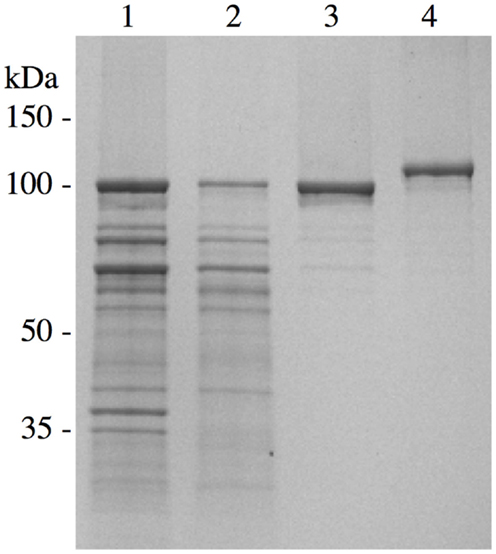
Figure 2. Chromatographic purification of S. japonicum paramyosin. A pET-30 plasmid containing paramyosin was expressed and purified similarly as described (28). Lane 1, inclusion body preparation; lane 2, anion exchange chromatography; lane 3, size exclusion chromatography; lane 4, purified S. japonicum paramyosin with a thioredoxin fusion tag. Reprinted from Ref. (30).
Future Product Development for rSj97
Together with colleagues, we are actively pursuing vaccination trials in both murine and bovine models with encouraging preliminary results. These experiments are designed to evaluate several doses of antigen as well as several adjuvants suitable for both veterinary and human use. Because Schistosomiasis japonica is a zoonosis, with bovines playing a key role in transmission to humans (32, 33), we are also evaluating the impact of bovine vaccination on human incidence of infection in endemic communities. Sj97 is a target of protective human IgE responses; therefore, we are also evaluating the potential for inducing hypersensitivity reactions by skin testing with rSj97 in infected bovines, again with encouraging preliminary results. If successful, these data, together with our GMP ready process for expression and purification, will form a compelling argument to move recombinant paramyosins toward Phase I trials in humans.
Conflict of Interest Statement
The authors declare that the research was conducted in the absence of any commercial or financial relationships that could be construed as a potential conflict of interest.
Acknowledgments
This work was supported by grants from the US National Institutes of Health (R01AI101274, R21AI097963, R56AI091753, R01AI48123, and TMRC P50 AI098481).
References
1. King CH, Dickman K, Tisch DJ. Reassessment of the cost of chronic helmintic infection: a meta-analysis of disability-related outcomes in endemic schistosomiasis. Lancet (2005) 365:1561–9. doi: 10.1016/S0140-6736(05)66457-4
2. Chitsulo L, Engels D, Montresor A, Savioli L. The global status of schistosomiasis and its control. Acta Trop (2000) 77:41–51. doi:10.1016/S0001-706X(00)00122-4
3. Murray CJL, Lopez AD. The Global Burden of Disease: A Comprehensive Assessment of Mortality and Disability from Diseases, Injuries, and Risk Factors in 1990 and Projected to 2020. Cambridge, MA: Harvard School of Public Health (1996).
4. Gryseels B, Polman K, Clerinx J, Kestens L. Human schistosomiasis. Lancet (2006) 368:1106–18. doi:10.1016/S0140-6736(06)69440-3
5. Coutinho HM, Acosta LP, McGarvey ST, Jarilla B, Jiz M, Pablo A, et al. Nutritional status improves after treatment of Schistosoma japonicum-infected children and adolescents. J Nutr (2006) 136:183–8.
6. Olveda RM, Daniel BL, Ramirez BD, Aligui GD, Acosta LP, Fevidal P, et al. Schistosomiasis japonica in the Philippines: the long-term impact of population-based chemotherapy on infection, transmission, and morbidity. J Infect Dis (1996) 174:163–72. doi:10.1093/infdis/174.1.163
7. Sher A, Pearce E, Hieny S, James S. Induction of protective immunity against Schistosoma mansoni by a nonliving vaccine. IV. Fractionation and antigenic properties of a soluble adult worm immunoprophylactic activity. J Immunol (1986) 136:3878–83.
8. Pearce EJ, James SL, Dalton J, Barrall A, Ramos C, Strand M, et al. Immunochemical characterization and purification of Sm-97, a Schistosoma mansoni antigen monospecifically recognized by antibodies from mice protectively immunized with a nonliving vaccine. J Immunol (1986) 137:3593–600.
9. Lanar DE, Pearce EJ, James SL, Sher A. Identification of paramyosin as schistosome antigen recognized by intradermally vaccinated mice. Science (1986) 234:593–6. doi:10.1126/science.3094144
10. Cohen C, Lanar DE, Parry DA. Amino acid sequence and structural repeats in schistosome paramyosin match those of myosin. Biosci Rep (1987) 7:11–6. doi:10.1007/BF01122722
11. Matsumoto Y, Perry G, Levine RJ, Blanton R, Mahmoud AA, Aikawa M. Paramyosin and actin in schistosomal teguments. Nature (1988) 333:76–8. doi:10.1038/333076a0
12. Kalinna B, McManus DP. An IgG (Fc gamma)-binding protein of Taenia crassiceps (Cestoda) exhibits sequence homology and antigenic similarity with schistosome paramyosin. Parasitology (1993) 106(Pt 3):289–96. doi:10.1017/S0031182000075119
13. Laclette JP, Landa A, Arcos L, Willms K, Davis AE, Shoemaker CB. Paramyosin is the Schistosoma mansoni (Trematoda) homologue of antigen B from Taenia solium (Cestoda). Mol Biochem Parasitol (1991) 44:287–95. doi:10.1016/0166-6851(91)90015-X
14. Laclette JP, Shoemaker CB, Richter D, Arcos L, Pante N, Cohen C, et al. Paramyosin inhibits complement C1. J Immunol (1992) 148:124–8.
15. Deng J, Gold D, LoVerde PT, Fishelson Z. Inhibition of the complement membrane attack complex by Schistosoma mansoni paramyosin. Infect Immun (2003) 71:6402–10. doi:10.1128/IAI.71.11.6402-6410.2003
16. Deng J, Gold D, LoVerde PT, Fishelson Z. Mapping of the complement C9 binding domain in paramyosin of the blood fluke Schistosoma mansoni. Int J Parasitol (2007) 37:67–75. doi:10.1016/j.ijpara.2006.09.011
17. Loukas A, Jones MK, King LT, Brindley PJ, McManus DP. Receptor for Fc on the surfaces of schistosomes. Infect Immun (2001) 69:3646–51. doi:10.1128/IAI.69.6.3646-3651.2001
18. Pearce EJ, James SL, Hieny S, Lanar DE, Sher A. Induction of protective immunity against Schistosoma mansoni by vaccination with schistosome paramyosin (Sm97), a nonsurface parasite antigen. Proc Natl Acad Sci U S A (1988) 85:5678–82. doi:10.1073/pnas.85.15.5678
19. Flanigan TP, King CH, Lett RR, Nanduri J, Mahmoud AA. Induction of resistance to Schistosoma mansoni infection in mice by purified parasite paramyosin. J Clin Invest (1989) 83:1010–4. doi:10.1172/JCI113942
20. Nara T, Tanabe K, Mahakunkijcharoen Y, Osada Y, Matsumoto N, Kita K, et al. The B cell epitope of paramyosin recognized by a protective monoclonal IgE antibody to Schistosoma japonicum. Vaccine (1997) 15:79–84. doi:10.1016/S0264-410X(96)00100-4
21. Kojima S, Janecharut T, Hata H, Niimura M. Role of a mouse monoclonal IgE antibody in passive transfer of immunity to Schistosoma japonicum infection. Mem Inst Oswaldo Cruz (1987) 82(Suppl 4):237–41. doi:10.1590/S0074-02761987000800045
22. Kojima S, Niimura M, Kanazawa T. Production and properties of a mouse monoclonal IgE antibody to Schistosoma japonicum. J Immunol (1987) 139:2044–9.
23. Ramirez BL, Kurtis JD, Wiest PM, Arias P, Aligui F, Acosta L, et al. Paramyosin: a candidate vaccine antigen against Schistosoma japonicum. Parasite Immunol (1996) 18:49–52. doi:10.1046/j.1365-3024.1996.d01-4.x
24. Tang CW, Liu SJ, Ma YB, Liang L, Guo P, Wang SY, et al. A multi-gene DNA vaccine encoding non-fusion membrane-anchoring antigen against Schistosoma japonicum. Zhongguo Ji Sheng Chong Xue Yu Ji Sheng Chong Bing Za Zhi (2008) 26:412–6.
25. Becker MM, Kalinna BH, Yang W, Harrop SA, Scott JC, Waine GJ, et al. Gene cloning and complete nucleotide sequence of Philippine Schistosoma japonicum paramyosin. Acta Trop (1995) 59:143–7. doi:10.1016/0001-706X(94)00090-N
26. Nara T, Matsumoto N, Janecharut T, Matsuda H, Yamamoto K, Irimura T, et al. Demonstration of the target molecule of a protective IgE antibody in secretory glands of Schistosoma japonicum larvae. Int Immunol (1994) 6:963–71. doi:10.1093/intimm/6.7.963
27. Leenstra T, Acosta LP, Wu HW, Langdon GC, Solomon JS, Manalo DL, et al. T-Helper-2 cytokine responses to Sj97 predict resistance to reinfection with Schistosoma japonicum. Infect Immun (2006) 74:370–81. doi:10.1128/IAI.74.1.370-381.2006
28. Jiz M, Friedman JF, Leenstra T, Jarilla B, Pablo A, Langdon G, et al. Immunoglobulin E (IgE) responses to paramyosin predict resistance to reinfection with Schistosoma japonicum and are attenuated by IgG4. Infect Immun (2009) 77:2051–8. doi:10.1128/IAI.00012-09
29. McManus DP, Wong JY, Zhou J, Cai C, Zeng Q, Smyth D, et al. Recombinant paramyosin (rec-Sj-97) tested for immunogenicity and vaccine efficacy against Schistosoma japonicum in mice and water buffaloes. Vaccine (2001) 20:870–8. doi:10.1016/S0264-410X(01)00405-4
30. Bergquist NR, Leonardo LR, Mitchell GF. Vaccine-linked chemotherapy: can schistosomiasis control benefit from an integrated approach? Trends Parasitol (2005) 21:112–7. doi:10.1016/j.pt.2005.01.001
31. Jiz M, Wu HW, Meng R, Pond-Tor S, Reynolds M, Friedman JF, et al. Pilot-scale production and characterization of paramyosin, a vaccine candidate for schistosomiasis japonica. Infect Immun (2008) 76:3164–9. doi:10.1128/IAI.00409-08
32. Wu H, Qin Y, Meng R, Chu K, Liu Y, McGarvey S, et al. High prevalence of S. japonicum infection in Philippine water buffalos assessed by qPCR. Am J Trop Med Hyg (2010) 82:645–52. doi:10.4269/ajtmh.2010.09-0638
Keywords: Schistosoma japonicum, vaccines, paramyosin, zoonoses, preclincal
Citation: Jiz MA, Wu H, Olveda R, Jarilla B and Kurtis JD (2015) Development of paramyosin as a vaccine candidate for schistosomiasis. Front. Immunol. 6:347. doi: 10.3389/fimmu.2015.00347
Received: 13 April 2015; Accepted: 23 June 2015;
Published: 21 July 2015
Edited by:
Ahmad Ali Othman, Tanta Faculty of Medicine, EgyptReviewed by:
Colin Matthew Fitzsimmons, University of Cambridge, UKKlaus D. Erttmann, Bernhard Nocht Institute for Tropical Medicine, Germany
Copyright: © 2015 Jiz, Wu, Olveda, Jarilla and Kurtis. This is an open-access article distributed under the terms of the Creative Commons Attribution License (CC BY). The use, distribution or reproduction in other forums is permitted, provided the original author(s) or licensor are credited and that the original publication in this journal is cited, in accordance with accepted academic practice. No use, distribution or reproduction is permitted which does not comply with these terms.
*Correspondence: Jonathan D. Kurtis, Center for International Health Research, Rhode Island Hospital, 55 Claverick Street, Room 325, Providence, RI 02906, USA,am9uYXRoYW5fa3VydGlzQGJyb3duLmVkdQ==