- Department of Pediatrics, Leiden University Medical Center, Leiden, Netherlands
Two human natural killer (NK) cell subsets are usually distinguished, displaying the CD56dimCD16+ and the CD56brightCD16−/+ phenotype. This distinction is based on NK cells present in blood, where the CD56dim NK cells predominate. However, CD56bright NK cells outnumber CD56dim NK cells in the human body due to the fact that they are predominant in peripheral and lymphoid tissues. Interestingly, within the total CD56bright NK cell compartment, a major phenotypical and functional diversity is observed, as demonstrated by the discovery of tissue-resident CD56bright NK cells in the uterus, liver, and lymphoid tissues. Uterus-resident CD56bright NK cells express CD49a while the liver- and lymphoid tissue-resident CD56bright NK cells are characterized by co-expression of CD69 and CXCR6. Tissue-resident CD56bright NK cells have a low natural cytotoxicity and produce little interferon-γ upon monokine stimulation. Their distribution and specific phenotype suggest that the tissue-resident CD56bright NK cells exert tissue-specific functions. In this review, we examine the CD56bright NK cell diversity by discussing the distribution, phenotype, and function of circulating and tissue-resident CD56bright NK cells. In addition, we address the ongoing debate concerning the developmental relationship between circulating CD56bright and CD56dim NK cells and speculate on the position of tissue-resident CD56bright NK cells. We conclude that distinguishing tissue-resident CD56bright NK cells from circulating CD56bright NK cells is a prerequisite for the better understanding of the specific role of CD56bright NK cells in the complex process of human immune regulation.
Introduction
Since the discovery of natural killer (NK) cells in 1975 (1, 2), major advances were made in deciphering the role of NK cells in health and disease. It is currently accepted that NK cells are not just “killers” that lyse infected or transformed cells but can also play an important role in modulation of immune responses due to the secretion of immunoregulatory cytokines (e.g., IFN-γ and TNF-α) and chemokines (e.g., CCL3 and CCL4). Based on this cytokine secretion profile, NK cells are classified into group 1 of the large family of innate lymphoid cells (ILCs). Developmentally, NK cells are not related to the other (non-cytotoxic) ILCs, and can be distinguished from the remaining ILCs by the expression of the transcription factor Eomesodermin (EOMES) and the cytolytic protein perforin (3, 4).
In humans, two conventional NK cell subsets have been phenotypically defined based on CD56 and CD16 (FCRγIII) surface expression: CD56brightCD16−/+ and CD56dimCD16+. While the function of CD56 [neural cell adhesion molecule (NCAM)] on NK cells is not completely understood yet, CD16 can mediate antibody-dependent cellular cytotoxicity (5). Since most research in human NK cell biology is based on peripheral blood, the herein predominant CD56dim NK cell population is most extensively investigated. Based on circulating NK cells, CD56bright and CD56dim NK cells have usually been described as two functionally distinct subsets, cytokine producing and cytolytic, respectively. However, several observations challenge this strict difference, as that both subsets can be cytotoxic or produce cytokines, after appropriate in vitro stimulation. Upon target cell recognition, resting CD56dim NK cells are highly cytotoxic, but can produce cytokines as well (6–8). In contrast, CD56bright NK cells require monokine activation (combinations of IL2/IL12/IL15/IL18) to acquire cytolytic activity and produce cytokines (6, 9–11).
Although the CD56dim NK cells predominate in blood, the CD56bright NK cells are far more abundant in the human body due to their enrichment in lymphoid and non-lymphoid tissues (12–18). In addition, CD56bright NK cells comprise the major NK cell population in inflamed and cancer tissues (12, 14, 19). Recently, tissue-resident CD56bright NK cells were identified in liver, uterus, and lymphoid tissues, which points toward a tissue-specific function of CD56bright NK cells (13, 15–17, 20–22). In order to understand the NK cell diversity, it is essential to focus on how CD56bright NK cells develop, distribute, and acquire or alter their phenotype and function specifically in a particular organ. The first four developmental stages (i.e., from hematopoietic stem cell to CD56bright NK cell) were already reviewed extensively elsewhere and will not be discussed here (23, 24). This review attempts to improve the understanding of human circulating and tissue-resident CD56bright NK cells by reappraising their distribution and developmental, functional, and phenotypical characteristics. In addition, we will address to the developmental relationship between CD56bright (stage 4) and CD56dim NK cells (stage 5) and speculate on the position of tissue-resident CD56bright NK cells within the NK cell developmental pathway.
Distribution, Phenotype and Function
CD56bright NK cells are widely distributed throughout the human body. When compared with blood, CD56bright NK cells are enriched in most human tissues. They represent the majority of NK cells in lymph nodes, tonsil, stomach, gut, liver, uterus, adrenal gland, and visceral adipose tissue (12–18). Although CD56bright NK cells seem to be outnumbered by CD56dim NK cells in lung, kidney, mammillary tissue, bone marrow and spleen, this is probably a reflection of the high blood perfusion of these organs (12, 13, 18, 25). Most knowledge on the phenotype and function of CD56bright NK cells is derived from blood, but it is important to realize that unique subsets of tissue-resident CD56bright NK cells have been described in lymphoid tissues, liver and uterus (13, 15, 22, 26). Conceivably, more organs contain tissue-resident CD56bright NK cell populations. To the best of our knowledge, no tissue-resident CD56dim NK cells have been described to date. Although residency is often used as a term for organ-infiltrating NK cells, it is generally not discussed whether these NK cells are just trafficking through the organ, or truly tissue resident. In this review, we only apply the term “resident” if there is substantial evidence, which allows to distinguish the tissue-resident CD56bright NK cells from circulating CD56bright NK cells. The lack of CD56 expression on murine NK cells hampers the one to one comparison of CD56bright NK cells to their murine counterpart. Due to limitations in obtaining human tissue samples, important findings in mice will be included in this review to cover the lack of human data.
Hallmarks of Tissue-Resident CD56bright NK Cells
In order to be retained within the tissue, tissue-resident CD56bright NK cells should possess characteristics, which prevent egress from the tissue. One of the mechanisms involved in residency is attributed to CD69, which is absent from blood-derived NK cells (13). Originally, CD69 was identified as an early activation marker, but today CD69 is known to be associated with tissue residency by suppressing sphingosine-1-phospate receptor 1 (S1PR1) surface expression (27–29). Although initially identified in the context of T- and B-cell migration, S1PRs have also been proposed to mediate the egress of NK cells from tissues into blood and lymph in mice, driven by a S1P gradient (30–35). It has not been confirmed whether S1PRs are expressed as protein on the cell surface of human NK cell subsets in blood and tissues. At transcriptional level, however, both S1PR1 and S1PR5 are expressed in circulating human NK cells, with the latter being selective for CD56dim NK cells (33, 36, 37). In contrast to S1PR1, S1PR5 is not inhibited by CD69 (32). Another potential mechanism for tissue homing and/or residency is the engagement of chemokine receptors. For instance, CXCR6 and CCR5 are both highly expressed on tissue-resident CD56bright NK cells in lymphoid tissues and liver, but have a low expression on blood-derived CD56bright NK cells, which instead express CCR7 (13, 15, 38). A third mechanism of tissue retention is driven by the expression or absence of adhesion molecules. For instance, the integrin CD49a is highly expressed on uterine CD56bright NK cells, but absent from blood NK cells (20). Furthermore, tissue-resident CD56bright NK cells lack CD62L (L-selectin), which is like CCR7 involved in recruitment of circulating NK cells to lymphoid tissues via high endothelial venules (HEVs) (15, 17, 18, 38, 39). Altogether, based on the expression of CD69, chemokine receptors, and adhesion molecules, tissue-resident CD56bright NK cells can be distinguished from circulating CD56bright NK cells. In addition to the phenotypical differences, tissue-resident CD56bright NK cells are functionally different from their circulating counterparts as will be discussed in the next sections.
Lymphoid Tissues
Lymph Node
Lymph nodes contain 40% of the lymphocytes in the human body, of which 2–5% consist of NK cells (Figure 1A) (12, 13, 18, 40). More than 75% of the NK cells in lymph nodes have a CD56bright phenotype (Figure 1B) (13, 18, 25). Accumulating evidence suggests a model in which CD56bright NK cells circulate from the blood to tissues, enter the lymphatic system, and eventually migrate back to the periphery via the efferent lymph (41, 42). The mechanisms governing the migration to and infiltration of lymphoid tissues by CD56bright NK cells are mainly deduced from chemokine receptor expression on circulating CD56bright NK cells. As discussed earlier, circulating CD56bright NK cells express CCR7 and CD62L (38, 39). The chemokines engaging CCR7, CCL19, and CCL21 are both highly expressed in lymph nodes (12). HEVs might not be the only route for circulating CD56bright NK cells to enter the lymph node. NK cells in seroma fluid, which represents an accumulation of afferent lymph, resemble circulating CD56bright NK cells regarding low expression of CCR5, killer-cell immunoglobulin-like receptor (KIR), and CD16 and high expression of CCR7 and CD62L (12, 43). This suggests that circulating CD56bright NK cells enter the lymph node both via HEVs and afferent lymph vessels.
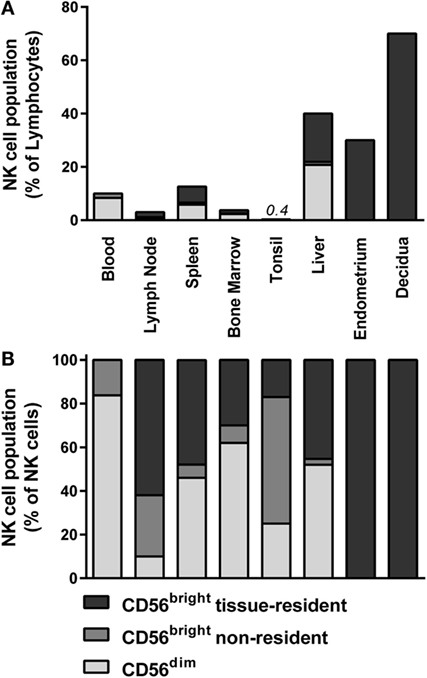
Figure 1. Distribution of NK cell populations in blood and tissues. The distribution of CD56dim, non-resident CD56bright, and tissue-resident CD56bright NK cells is depicted as percentage of (A) total lymphocytes and (B) total NK cells within blood (13), lymph node (13, 18, 25), spleen (13, 18, 25), bone marrow (13, 18, 25), tonsil (18, 44), liver (15, 45, 46), endometrium (16, 20), and decidua (20, 47). Tissue-resident CD56bright NK cells were defined as CD69+CXCR6+ (lymph node, spleen, and bone marrow), NKp44+CD103+ (tonsil), CD69+CXCR6+ (liver), and CD49a+ (endometrium and decidua). For phenotypical details, see Figure 2 and Table 1.
Recently, we identified a major lymphoid tissue-resident NK cell subset in lymph node, spleen, and bone marrow based on co-expression of CD69 and CXCR6 (Figure 2; Table 1) (13). In the lymph node, lymphoid tissue NK (ltNK) cells account for 60% of all NK cells and cover the majority of the CD56bright NK cell compartment (Figure 1B). LtNK cells display a slightly less intense CD56 and more intense NKp46 expression compared with circulating CD56bright NK cells. In addition, the majority of ltNK cells is CD16−, CD49a−, and CD27+ (13). Interestingly, most ltNK cells do not express DNAX accessory molecule 1 (DNAM1), an activating receptor which is uniformly expressed on circulating CD56bright NK cells (13). The remaining CD56brightCD69− NK cells closely resemble circulating CD56bright NK cells, suggesting that these cells are blood-derived CD56bright NK cells transiently circulating through the lymph node (13).
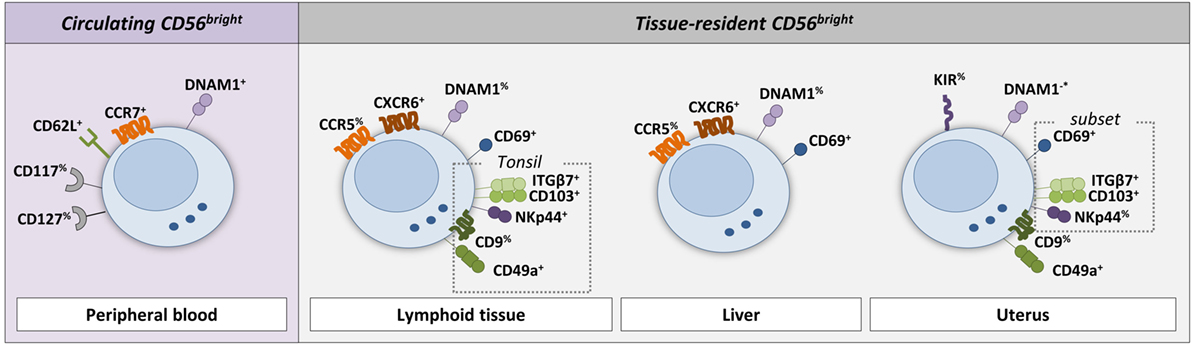
Figure 2. Phenotype of circulating and tissue-resident CD56bright NK cells. The cell surface markers on NK cells that are discriminative between circulating and tissue-resident NK cells in lymphoid tissue, liver, and uterus are shown (see references in text and Table 1). Circulating CD56bright NK cells typically express the lymphoid tissue homing makers CD62L (L-selectin) and CCR7. In addition, CD117 (c-kit) and CD127 (IL-7Rα) are expressed by a fraction of circulating CD56bright NK cells. Lymphoid tissue-resident NK cells express CD69 and CXCR6. Tonsil-resident NK cells (defined as NKp44+CD103+) express in addition ITGβ7, CD49a, and partly CD9. The majority of CD69+CXCR6+ liver-resident NK cells express CCR5. In contrast to circulating CD56bright NK cells, only a fraction of lymphoid tissue-, tonsil-, and liver-resident NK cells express DNAM1. A subset of CD49a+ uterus-resident NK cells (endometrium and decidua) expresses CD69, ITGβ7, CD103, and NKp44. The reported DNAM1 expression in the uterus is contradicting in the literature and therefore indicated with −*. % indicates that only a fraction of the NK cell population is positive for the marker.
Lymphoid tissue NK cells were tested in the functional assays classically used for NK cells. The ltNK cells were less potent IFN-γ producers compared with circulating CD56bright NK cells nor did they lyse K562 target cells as efficient as CD56dim NK cells (13). However, the expression of EOMES and perforin distinguishes ltNK cells from the helper-ILC1 group (13). These phenotypical and functional characteristics, combined with their specific location in lymphoid tissues where immune responses are initiated and shaped, point to a distinct yet undefined role of ltNK cells (50).
Notably, without the use of tissue-resident markers, CD69 and CXCR6, ltNK cells could previously not be distinguished from circulating CD56bright NK cells. Therefore, a re-examination of the function of CD56bright NK cells in lymphoid tissue is necessary, in particular in the lymph node where a large population of ltNK cells co-exists next to the non-resident or circulating CD56bright NK cells.
Spleen and Marrow
The spleen and bone marrow contain 14 and 10% of the total lymphocyte pool. NK cells constitute 5–20% and 4% of lymphocytes in spleen and marrow, respectively (Figure 1A) (13, 18, 40, 43). The CD56bright and CD56dim NK cells are equally distributed in the spleen, but 90% of CD56bright NK cells consist of ltNK cells (Figure 1B) (13, 25, 40). Similar to the spleen, the bone marrow is enriched for CD56bright NK cells, of which the majority consists of ltNK cells (13, 25). The phenotype of ltNK cells in spleen and marrow resembles the ltNK cell population in lymph node. As mentioned before, the non-resident CD56brightCD69− and CD56dim NK cells in marrow and spleen closely resemble the circulating CD56bright and CD56dim NK cells and are probably circulating NK cells contained in the tissue at time of isolation. Previously, the spleen has been reported to be enriched in CD27+ and NKp46bright NK cells, which could be a reflection of ltNK cells. These findings further illustrate the importance of using tissue-resident markers to distinguish circulating from tissue-resident CD56bright NK cells (51, 52). The manner in which NK cells enter the spleen differs from lymph node entrance, because the spleen does not contain afferent lymphatic vessels or HEVs (53). In mice, NK cells enter the spleen via arterioles in the marginal zone, rather than via arterioles directly connected to the red pulp, where most NK cells reside (54). Unfortunately, there is a lack of human studies focusing on how NK cells migrate to the spleen and bone marrow.
Tonsil
In tonsil, although the CD56bright subset is predominant, only 0.4% of the total lymphocytes consist of NK cells (Figure 1A) (18). Seventeen percent of the total NK cell population in the tonsil co-expresses CD69 and CXCR6 (Figure 1B) (44). In contrast to the ltNK cells in lymph node, marrow, and spleen, these tonsil-resident NK cells also express NKp44, CD103, CD49a, Integrinβ7, and partly CD9 (Figure 2; Table 1) (44). Of note, tonsil-resident NK cells should be distinguished from NKp44+ ILC3s, which are located in the mucosa surrounding the lymphoid follicles and secrete preferentially IL-22 (55, 56). Similar to circulating CD56bright NK cells, the total pool of CD56bright NK cells was shown to produce high levels of IFN-γ and to become cytolytic upon IL-2 and/or IL-12 stimulation (18). Tonsils do not have afferent lymph vessels but HEVs are present, which might support the trafficking of NK cells. Similarly, CCL19 and CCL21 are secreted to attract circulating CD56bright NK cells, which might explain the high content of CD56bright NK cells which lack a tissue-resident phenotype (57).
Liver
Hepatic NK cells comprise 40% of all hepatic lymphocytes (Figure 1A) (45). Recently, a major liver-resident EOMES+CD56bright NK cell population has been described, which comprises 45% of the hepatic NK cells and closely resembles ltNK cells phenotypically and functionally (Figure 1B) (15, 45, 46). Liver-resident CD56bright NK cells are characterized by a simultaneous expression of CD69 and CXCR6 (46). They have a high expression of CCR5 and NKp46, and low expression of DNAM1, as indirectly concluded from phenotypical analysis on total hepatic CD56bright NK cells (Figure 2; Table 1) (15, 45).
An independent report demonstrated the presence of a distinct minor liver-resident cell population characterized by CD49a expression (21). Those CD56brightCD49a+ cells make up 2% of the total NK cell compartment in the liver but are not present in every individual (41% of donors) (13, 15, 21). The expression of CXCR6 has not been described. However, these cells do not express EOMES, suggesting that they do not belong to the NK cell lineage (21). Due to the low prevalence of this CD49a+EOMES− cell population, we can indirectly conclude that the major CD69+CXCR6+ liver-resident NK cell population is negative for CD49a. The IFN-γ production of liver-resident CD56bright NK cells after 4-h stimulation with IL12 and IL18 was lower compared with the non-resident hepatic NK cells (46). Similar to ltNK cells, liver-resident CD56bright NK cells express perforin and granzyme B at a low level, further supporting a non-cytotoxic function (46).
Several studies in mice demonstrated the existence of hapten and virus-specific hepatic NK cell memory, mediated by cells expressing CD49a and CXCR6 (21, 58, 59). In contrast, splenic CXCR6+ NK cells, which potentially resemble the human ltNK cells, were not able to mediate a memory response (58). Thus, although CXCR6 expression is not restricted to the liver, only hepatic NK cells were found to mediate a memory response in mice. Nevertheless, it would be interesting to further study the memory capacities of the highly prevalent CXCR6+ liver- and lymphoid tissue-resident CD56bright NK cells in humans.
Uterus
The uterine mucosa is populated by EOMES+CD56bright NK cells (16, 17, 23). In contrast to blood, there are hardly any CD56dim NK cells detectable in endometrium (no pregnancy) and decidua (pregnancy) (16, 17, 20, 60). Independent of the stage of the menstrual cycle, NK cells make up 30% of the endometrial lymphocytes (Figure 1A), although the absolute number of lymphocytes and NK cells increases robustly in the secretory stage (16). During early pregnancy, however, more than 70% of the lymphocytes in the uterine decidua is represented by CD56bright NK cells (Figure 1A) (47). Phenotypically, endometrial and decidual CD56bright NK cells closely resemble each other, and will be further referred to as uterine CD56bright NK cells. The CD56 expression of the uterine NK cells is even more intense than their circulating CD56bright counterparts (Table 1) (17, 61). All uterine NK cells display CD49a but not CCR5, discriminating them from the circulating, lymphoid tissue, and liver-resident NK cell populations (Figure 2) (16, 20). DNAM1 has been reported to be absent on uterine NK cells, although a contradicting report on this observation exists (20, 48, 62). Recently, it was shown that a fraction of uterine NK cells expresses the heterodimer CD103/ITGβ7, NKp44, as well as CD69 (20, 62). Conversely, an earlier study reported that all decidual NK cells express CD69 (17). Despite this discrepancy concerning the CD69 expression, both the CD56brightCD103− and CD56brightCD103+ NK cells are likely to represent a tissue-resident CD56bright NK cell population, as demonstrated by the expression of KIRs, CD9, and poor IFN-γ production and cytotoxicity (16, 17, 20, 49, 63, 64). Moreover, transcriptome analysis of decidual NK cells and circulating NK cells highlighted the uniqueness of the uterine NK cells (17, 48). To the best of our knowledge, the presence of chemokine receptors, such as CXCR6 and CCR5, has not been reported. Compared with circulating CD56bright NK cells, decidual CD56bright NK cells highly express the activating receptors NKG2C and NKG2E at RNA level; however, <30% is NKG2C+ on protein level (17, 62, 65).
Initially, a suppressive function of decidual NK cells was thought to be essential to provide maternal–fetal tolerance (17, 64). However, accumulating evidence points toward a more active role of decidual CD56bright NK cells in regulating placentation, as reviewed elsewhere (66). Decidual NK cells are considered to stimulate trophoblast invasion and spinal artery remodeling via the production of various chemokines and angiogenic factors (including angiopoietins and GM-CSF) (67–69). Mice lacking decidual NK cells exhibit abnormalities in pregnancy, including abnormal vascular remodeling of decidual arteries (70). Although the process of placentation in humans is different, specific combinations of fetal HLA-C alleles, presented by trophoblasts, and maternal KIR expression were shown to be associated with successful placentation (69, 71). The similarities between endometrial and decidual CD56bright NK cells suggest that decidual CD56bright NK cells are a direct reflection of endometrial CD56bright NK cells in a pregnant tissue microenvironment. Taken together, the phenotypical and functional profile of the uterine CD56bright NK cell compartment supports their unique functional role during pregnancy.
Developmental Relationship Between Circulating CD56dim and CD56bright NK Cells
Thus far, we discussed the tissue-resident and circulating/non-resident CD56bright NK cells within the tissues. Still, the origin of the different CD56bright NK cell populations and their relation to the CD56dim NK cell subset remains unclear. The circulating CD56bright NK cells have been extensively investigated and are generally considered to be the precursors of the CD56dim NK cells. In the last section of this review, we will summarize the current evidence in favor and against the linear relationship between the circulating CD56bright and CD56dim NK cells, and speculate on the position of tissue-resident NK cells in this developmental pathway.
Several studies provided clues about the developmental relationship between CD56bright NK cells and CD56dim NK cells. First, it was shown that CD56bright, but not CD56dim NK cells, constitutively express the high-affinity IL-2Rα (CD25) and display a high proliferative response in the presence of picomolar concentrations of IL-2 (72, 73). Because CD56bright NK cells have significantly longer telomeres compared with CD56dim NK cells, they have been assumed to have a shorter proliferative history (74). A commonly used marker for immaturity, the tyrosine kinase c-kit (receptor for stem cell factor, CD117) is expressed on a fraction of CD56bright NK cells, but is absent on CD56dim NK cells (75, 76). In addition, the recovery of CD56bright NK cells in the first weeks after hematopoietic stem cell transplantation (HSCT) precedes the reconstitution of CD56dim NK, a sequential occurrence potentially pointing toward a developmental relationship (74, 77). Together, these findings resulted in the hypothesis that CD56dim NK cells are derived from CD56bright NK cells.
Differentiation from CD56bright to CD56dim NK Cells In Vitro
In efforts to provide evidence for this hypothesis, numerous studies aimed to recapitulate the differentiation from CD56bright to CD56dim NK cells in vitro. CD56bright NK cells were shown to acquire a CD56dim-like phenotype upon in vitro activation with IL-2, IL-15, and/or co-culture with T cells. This resulted in the upregulation of CD16 and KIRs and the downregulation of IL-7Rα (CD127), CD117, CXCR3, and CCR7 (10, 74). However, the intensity of CD56 expression was not reduced on monokine-activated CD56bright NK cells. The presence of fibroblast growth factor receptor 1 (FGFR1) was demonstrated to be critical for the in vitro differentiation of CD56bright NK cells to cytotoxic CD56dim NK cells in a contact-dependent manner (78). FGFR1 is a ligand for CD56 and is constitutively expressed on fibroblasts (79, 80). The high density of CD56 on CD56bright NK cells may thus be of importance in the interaction with fibroblasts and differentiation toward CD56dim NK cells.
Differentiation from CD56bright to CD56dim NK Cells In Vivo
The in vivo evaluation of the relationship between CD56bright and CD56dim NK cells is hampered by the lack of CD56 expression on murine NK cells. The vast majority of human CD56bright NK cells displayed a reduction of CD56 expression intensity after infusion into immune-deficient mice (78). Whether these in vivo differentiated CD56dim NK cells were phenotypically and functionally similar to human blood-derived CD56dim NK cells were not addressed in this study, leaving the possibility that the bright CD56 expression is not sustained in mice lacking human fibroblasts expressing FGFR1. An alternative for murine experiments can be provided by the study of rhesus macaques. Gene tracking data in rhesus macaques transplanted with lentiviral barcoded hematopoietic stem cells demonstrated that the lineage origin of the macaque NK cell homologs of CD56bright (CD56+CD16−) and CD56dim (CD56−CD16+) NK cells is different (81). While the CD56bright homolog was derived from the same progenitors as T-cell, B-cell, and myeloid cells, the CD56dim homolog displayed a unique clonal pattern, suggesting that these cells do not develop from the CD56bright population but may belong to an independent lineage (81).
In addition to mice and macaques studies, human NK cell deficiencies can provide clues about the developmental relationship between CD56bright and CD56dim NK cells. Mutations in the transcription factor gene GATA2 result in the absence of CD56bright NK cells while CD56dim NK cells are still present (82). This observation argues against the theory that CD56dim NK cells are derived from CD56bright NK cells. On the other hand, humans with a partial minichromosome maintenance complex 4 (MCM4) deficiency, a molecule involved in proliferation, have reduced numbers of circulating CD56dim NK cells but normal numbers of CD56bright NK cells (83). This could indicate that maintenance of the CD56dim NK cell subset requires proliferation, which might be dependent or independent of the CD56bright NK cells. To the best of our knowledge, there are no mutations in transcription factors described, which cause a lack of CD56dim NK cells while the CD56bright NK cells are spared. Recently, Zeb2 was identified as the essential regulator of terminal NK cell maturation in mice and shown to be higher expressed in circulating CD56dim compared with CD56bright NK cells (84). Together, these studies emphasize the need for additional experimental evidence on the transcriptional regulation of human NK cell development.
Intermediate Stages Connecting CD56bright to CD56dim NK Cells
If CD56bright and CD56dim NK cells are successive stages in the NK cell developmental pathway, developmental intermediates should exist. Independent studies reported the existence of phenotypic and functional intermediate stages in the progression from CD56bright to CD56dim NK cells in peripheral blood of healthy donors and patients after HSCT. These studies mainly focused on CD16, CD27, or CD117, for which CD56bright NK cells have a bimodal expression profile (10, 51, 85–88). Both CD16+ and CD27− CD56bright NK cells were independently suggested to represent intermediate populations based on phenotype and functional characteristics (10, 51, 88). A relative increase of CD16+, CD27−, and CD117− CD56bright NK cells was observed early after HSCT (85, 86). Notably, the expression of CD16, CD117, and CD27 on CD56bright NK cells can also be modulated by cytokine-activation (10, 51, 74). Because the post-HSCT setting presents a cytokine-rich environment, the “intermediate” CD16+, CD27−, and CD117− CD56bright NK cells may represent cytokine-activated CD56bright NK cells instead of developmental intermediates between CD56bright and CD56dim NK cells (85, 86).
In general, the potential differentiation of circulating CD56bright NK cells to CD56dim NK cells is characterized by loss of CD27, CD117, NKG2A, and CD62L expression and gain of CD16, KIRs, and CD57 expression. Both within the CD56bright compartment (CD117↓, CD27↓, and CD16↑) as well as within the CD56dim compartment (NKG2A↓, CD62L↓, KIRs↑, and CD57↑), the sequential loss and acquisition of these surface markers do not occur in a fixed order (10, 51, 87, 89, 90). Only the extremes of these markers, for instance CD117 and CD57, are mutually exclusively expressed. Together, this illustrates that uniform intermediate stages of differentiation between CD56bright and CD56dim NK cells cannot easily be identified.
Developmental Position of Tissue-Resident CD56bright NK Cells
Studies on the relationship between the NK cell populations have been based on blood-derived CD56bright and CD56dim NK cells. The starting point of most of these studies was a linear developmental relationship between CD56bright and CD56dim NK cells. However, the discovery of distinct tissue-resident CD56bright NK cell populations increases the number of possible relationships between the NK cell populations. Tissue-resident NK cells could be a precursor to circulating NK cells, but the absence of the immature markers CD117 and CD127 argues against this. It also seems unlikely that tissue-resident NK cells represent a transitory population between the circulating CD56bright and CD56dim NK cells. Detailed transcriptome analysis comparing uterine NK cells with both circulating CD56bright and CD56dim NK cells highlighted major differences in gene expression profile between the three NK cell populations (17). Moreover, data from transcription factor-deficient mice suggested that circulating and tissue-resident NK cells are derived from different cell lineages (91). In our opinion, the distinct phenotype and functional signature of the tissue-resident NK cell populations, together with their absence from blood, argues in favor of the hypothesis that tissue-resident CD56bright NK cells develop locally, independently of the circulating NK cells. It seems likely that the organ microenvironment is essential to induce the phenotype and retain tissue localization of tissue-resident cells. Nevertheless, additional studies are needed to shed new light on the developmental relationship between CD56bright, CD56dim and tissue-resident CD56bright NK cell populations.
Concluding Remarks
The recent identification of tissue-resident CD56bright NK cells in the lymphoid tissues, liver, and uterus led us to reappraise the characteristics of CD56bright NK cell populations in the circulation and tissues. The function of tissue-resident CD56bright NK cells in liver and lymphoid tissues has not been elucidated, although it is very likely that these cells, such as uterine NK cells, exert tissue-specific functions.
The existence of tissue-resident NK cells raises the question whether, and if so how, all the NK cell populations are developmentally related to each other. Based on the available evidence, we conclude that it is still possible that CD56dim NK cells develop independently from the CD56bright NK cells. Tissue-resident NK cells may develop from circulating CD56bright NK cells, or follow their own developmental pathway. Current in vitro models do not sufficiently mimic the in vivo situation, especially considering the potentially important role of the tissue microenvironment in shaping the features of tissue-resident CD56bright NK cells. As mouse models do not suffice in the evaluation of human NK cell subsets, other animal models might be exploited. Studying patients with aberrations in NK cell development due to genetic mutations could provide novel insights in the origin and development of tissue-resident NK cells. Furthermore, transcriptome analysis of non-resident and resident CD56bright NK cell populations will provide tools to further decipher the role of CD56bright NK cell populations in human immune responses. In conclusion, distinguishing tissue-resident CD56bright NK cells from circulating CD56bright NK cells is a prerequisite for the better understanding of the specific role of CD56bright NK cells in the complex process of human immune regulation.
Author Contributions
JM and GL wrote the manuscript. AL and MS critically revised the manuscript and approved it for publication.
Conflict of Interest Statement
The authors declare that the research was conducted in the absence of any commercial or financial relationships that could be construed as a potential conflict of interest.
Funding
This work was financially supported with a grant from the Dutch Cancer Society (#UL 2011-5133). GL was supported by a Leiden University Medical Center MD/PhD fellowship.
Abbreviations
DNAM1, DNAX accessory molecule 1; EOMES, eomesodermin; FGFR1, fibroblast growth factor receptor 1; HEVs, high endothelial venules; HSCT, hematopoietic stem cell transplantation; ILC, innate lymphoid cell; KIR, killer-cell immunoglobulin-like receptor; ltNK cells, lymphoid tissue natural killer cells; MCM4, minichromosome maintenance complex 4; S1PR1, sphingosine-1-phospate receptor 1.
References
1. Kiessling R, Klein E, Wigzell H. ‘Natural’ killer cells in the mouse. I. Cytotoxic cells with specificity for mouse Moloney leukemia cells. Specificity and distribution according to genotype. Eur J Immunol (1975) 5:112–7. doi:10.1002/eji.1830050208
2. Herberman RB, Nunn ME, Lavrin DH. Natural cytotoxic reactivity of mouse lymphoid cells against syngeneic acid allogeneic tumors. I. Distribution of reactivity and specificity. Int J Cancer (1975) 16:216–29. doi:10.1002/ijc.2910160205
3. Serafini N, Vosshenrich CA, Di Santo JP. Transcriptional regulation of innate lymphoid cell fate. Nat Rev Immunol (2015) 15:415–28. doi:10.1038/nri3855
4. Artis D, Spits H. The biology of innate lymphoid cells. Nature (2015) 517:293–301. doi:10.1038/nature14189
5. Lanier LL, Ruitenberg JJ, Phillips JH. Functional and biochemical analysis of CD16 antigen on natural killer cells and granulocytes. J Immunol (1988) 141:3478–85.
6. Fauriat C, Long EO, Ljunggren HG, Bryceson YT. Regulation of human NK-cell cytokine and chemokine production by target cell recognition. Blood (2010) 115:2167–76. doi:10.1182/blood-2009-08-238469
7. De Maria A, Bozzano F, Cantoni C, Moretta L. Revisiting human natural killer cell subset function revealed cytolytic CD56dimCD16+ NK cells as rapid producers of abundant IFN-γ on activation. Proc Natl Acad Sci U S A (2011) 108:728–32. doi:10.1073/pnas.1012356108
8. Anfossi N, André P, Guia S, Falk CS, Roetynck S, Stewart CA, et al. Human NK cell education by inhibitory receptors for MHC class I. Immunity (2006) 25:331–42. doi:10.1016/j.immuni.2006.06.013
9. Cooper M, Fehniger TA, Turner SC, Chen KS, Ghaheri BA, Ghayur T, et al. Human natural killer cells: a unique innate immunoregulatory role for the CD56bright subset. Blood (2001) 97:3146–51. doi:10.1182/blood.V97.10.3146
10. Béziat V, Duffy D, Quoc SN, Le Garff-Tavernier M, Decocq J, Combadière B, et al. CD56brightCD16+ NK cells: a functional intermediate stage of NK cell differentiation. J Immunol (2011) 186:6753–61. doi:10.4049/jimmunol.1100330
11. Takahashi E, Kuranaga N, Satoh K, Habu Y, Shinomiya N, Asano T, et al. Induction of CD16+ CD56bright NK cells with antitumour cytotoxicity not only from CD16- CD56bright NK cells but also from CD16- CD56dim NK cells. Scand J Immunol (2007) 65:126–38. doi:10.1111/j.1365-3083.2006.01883.x
12. Carrega P, Bonaccorsi I, Di Carlo E, Morandi B, Paul P, Rizzello V, et al. CD56brightPerforinlow noncytotoxic human NK cells are abundant in both healthy and neoplastic solid tissues and recirculate to secondary lymphoid organs via afferent lymph. J Immunol (2014) 192:3805–15. doi:10.4049/jimmunol.1301889
13. Lugthart G, Melsen JE, Vervat C, van Ostaijen-Ten Dam MM, Corver WE, Roelen DL, et al. Human lymphoid tissues harbor a distinct CD69+CXCR6+ NK cell population. J Immunol (2016) 197:78–84. doi:10.4049/jimmunol.1502603
14. Fehniger T, Cooper MA, Nuovo GJ, Cella M, Facchetti F, Colonna M, et al. CD56bright natural killer cells are present in human lymph nodes and are activated by T cell-derived IL-2: a potential new link between adaptive and innate immunity. Blood (2003) 101:3052–7. doi:10.1182/blood-2002-09-2876
15. Hudspeth K, Donadon M, Cimino M, Pontarini E, Tentorio P, Preti M, et al. Human liver-resident CD56bright/CD16neg NK cells are retained within hepatic sinusoids via the engagement of CCR5 and CXCR6 pathways. J Autoimmun (2016) 66:40–50. doi:10.1016/j.jaut.2015.08.011
16. Manaster I, Mizrahi S, Goldman-Wohl D, Sela HY, Stern-Ginossar N, Lankry D, et al. Endometrial NK cells are special immature cells that await pregnancy. J Immunol (2008) 181:1869–76. doi:10.4049/jimmunol.181.3.1869
17. Koopman LA, Kopcow HD, Rybalov B, Boyson JE, Orange JS, Schatz F, et al. Human decidual natural killer cells are a unique NK cell subset with immunomodulatory potential. J Exp Med (2003) 198:1201–12. doi:10.1084/jem.20030305
18. Ferlazzo G, Thomas D, Lin SL, Goodman K, Morandi B, Muller WA, et al. The abundant NK cells in human secondary lymphoid tissues require activation to express killer cell Ig-like receptors and become cytolytic. J Immunol (2004) 172:1455–62. doi:10.4049/jimmunol.172.3.1455
19. Dalbeth N, Gundle R, Davies RJ, Lee YC, McMichael AJ, Callan MF. CD56bright NK cells are enriched at inflammatory sites and can engage with monocytes in a reciprocal program of activation. J Immunol (2004) 173:6418–26. doi:10.4049/jimmunol.173.10.6418
20. Montaldo E, Vacca P, Chiossone L, Croxatto D, Loiacono F, Martini S, et al. Unique eomes+ NK cell subsets are present in uterus and decidua during early pregnancy. Front Immunol (2016) 6:646. doi:10.3389/fimmu.2015.00646
21. Marquardt N, Béziat V, Nyström S, Hengst J, Ivarsson MA, Kekäläinen E, et al. Cutting edge: identification and characterization of human intrahepatic CD49a+ NK cells. J Immunol (2015) 194:2467–71. doi:10.4049/jimmunol.1402756
22. Björkström NK, Ljunggren HG, Michaëlsson J. Emerging insights into natural killer cells in human peripheral tissues. Nat Rev Immunol (2016) 16:310–20. doi:10.1038/nri.2016.34
23. Montaldo E, Vacca P, Moretta L, Mingari MC. Development of human natural killer cells and other innate lymphoid cells. Semin Immunol (2014) 26:107–13. doi:10.1016/j.smim.2014.01.006
24. Yu J, Freud AG, Caligiuri MA. Location and cellular stages of natural killer cell development. Trends Immunol (2013) 34:573–82. doi:10.1016/j.it.2013.07.005
25. Eissens DN, Spanholtz J, van der Meer A, van Cranenbroek B, Dolstra H, Kwekkeboom J, et al. Defining early human NK cell developmental stages in primary and secondary lymphoid tissues. PLoS One (2012) 7:e30930. doi:10.1371/journal.pone.0030930
26. Manaster I, Mandelboim O. The unique properties of uterine NK cells. Am J Reprod Immunol (2010) 63:434–44. doi:10.1111/j.1600-0897.2009.00794.x
27. Bankovich AJ, Shiow LR, Cyster JG. CD69 suppresses sphingosine 1-phosophate receptor-1 (S1P1) function through interaction with membrane helix 4. J Biol Chem (2010) 285:22328–37. doi:10.1074/jbc.M110.123299
28. Shiow LR, Rosen DB, Brdicková N, Xu Y, An J, Lanier LL, et al. CD69 acts downstream of interferon-alpha/beta to inhibit S1P1 and lymphocyte egress from lymphoid organs. Nature (2006) 440:540–4. doi:10.1038/nature04606
29. Mackay LK, Braun A, Macleod BL, Collins N, Tebartz C, Bedoui S, et al. Cutting edge: CD69 interference with sphingosine-1-phosphate receptor function regulates peripheral T cell retention. J Immunol (2015) 194:2059–63. doi:10.4049/jimmunol.1402256
30. Rivera J, Proia RL, Olivera A. The alliance of sphingosine-1-phosphate and its receptors in immunity. Nat Rev Immunol (2008) 8:753–63. doi:10.1038/nri2400
31. Mayol K, Biajoux V, Marvel J, Balabanian K, Walzer T. Sequential desensitization of CXCR4 and S1P5 controls natural killer cell trafficking. Blood (2011) 118:4863–71. doi:10.1182/blood-2011-06-362574
32. Jenne CN, Enders A, Rivera R, Watson SR, Bankovich AJ, Pereira JP, et al. T-bet-dependent S1P5 expression in NK cells promotes egress from lymph nodes and bone marrow. J Exp Med (2009) 206:2469–81. doi:10.1084/jem.20090525
33. Walzer T, Chiossone L, Chaix J, Calver A, Carozzo C, Garrigue-Antar L, et al. Natural killer cell trafficking in vivo requires a dedicated sphingosine 1-phosphate receptor. Nat Immunol (2007) 8:1337–44. doi:10.1038/ni1523
34. Pappu R, Schwab SR, Cornelissen I, Pereira JP, Regard JB, Xu Y, et al. Promotion of lymphocyte egress into blood and lymph by distinct sources of sphingosine-1-phosphate. Science (2007) 316:295–8. doi:10.1126/science.1139221
35. Schwab SR, Pereira JP, Matloubian M, Xu Y, Huang Y, Cyster JG. Lymphocyte sequestration through S1P lyase inhibition and disruption of S1P gradients. Science (2005) 309:1735–9. doi:10.1126/science.1113640
36. Hanna J, Bechtel P, Zhai Y, Youssef F, McLachlan K, Mandelboim O, et al. Novel insights on human NK cells’ immunological modalities revealed by gene expression profiling. J Immunol (2004) 173:6547–63. doi:10.4049/jimmunol.173.11.6547
37. Wendt K, Wilk E, Buyny S, Buer J, Schmidt RE, Jacobs R. Gene and protein characteristics reflect functional diversity of CD56dim and CD56bright NK cells. J Leukoc Biol (2006) 80:1529–41. doi:10.1189/jlb.0306191
38. Campbell JJ, Qin S, Unutmaz D, Soler D, Murphy KE, Hodge MR, et al. Unique subpopulations of CD56+ NK and NK-T peripheral blood lymphocytes identified by chemokine receptor expression repertoire. J Immunol (2001) 166:6477–82. doi:10.4049/jimmunol.166.11.6477
39. Frey M, Packianathan NB, Fehniger TA, Ross ME, Wang WC, Stewart CC, et al. Differential expression and function of L-selectin on CD56bright and CD56dim natural killer cell subsets. J Immunol (1998) 161:400–8.
40. Westermann J, Pabst R. Distribution of lymphocyte subsets and natural killer cells in the human body. Clin Investig (1992) 70:539–44.
41. Caligiuri MA. Human natural killer cells. Blood (2008) 112:461–9. doi:10.1182/blood-2007-09-077438
42. Carrega P, Ferlazzo G. Natural killer cell distribution and trafficking in human tissues. Front Immunol (2012) 3:347. doi:10.3389/fimmu.2012.00347
43. Montalto E, Mangraviti S, Costa G, Carrega P, Morandi B, Pezzino G, et al. Seroma fluid subsequent to axillary lymph node dissection for breast cancer derives from an acculumulation of afferent lymph. Immunol Lett (2010) 131:67–72. doi:10.1016/j.imlet.2010.03.002
44. Fuchs A, Vermi W, Lee JS, Lonardi S, Gilfillan S, Newberry RD, et al. Intraepithelial type 1 innate lymphoid cells are a unique subset of IL-12- and IL-15-responsive IFN-γ-producing cells. Immunity (2013) 38:769–81. doi:10.1016/j.immuni.2013.02.010
45. Tanimine N, Tanaka Y, Abe T, Piao J, Chayama K, Ohdan H. Functional behavior of NKp46-positive intrahepatic natural killer cells against hepatitis C virus reinfection after liver transplantation. Transplantation (2016) 100:355–64. doi:10.1097/TP.0000000000001031
46. Stegmann KA, Robertson F, Hansi N, Gill U, Pallant C, Christophides T, et al. CXCR6 marks a novel subset of T-bet(lo)Eomes(hi) natural killer cells residing in human liver. Sci Rep (2016) 6:26157. doi:10.1038/srep26157
47. Bulmer JN, Morrison L, Longfellow M, Ritson A, Pace D. Granulated lymphocytes in human endometrium: histochemical and immunohistochemical studies. Hum Reprod (1991) 6:791–8.
48. Wang F, Zhou Y, Fu B, Wu Y, Zhang R, Sun R, et al. Molecular signatures and transcriptional regulatory networks of human immature decidual NK and mature peripheral NK cells. Eur J Immunol (2014) 44:2771–84. doi:10.1002/eji.201344183
49. Searle RF, Jones RK, Bulmer JN. Phenotypic analysis and proliferative responses of human endometrial granulated lymphocytes during the menstrual cycle. Biol Reprod (1999) 60:871–8. doi:10.1095/biolreprod60.4.871
50. Ferlazzo G, Pack M, Thomas D, Paludan C, Schmid D, Strowig T. Distinct roles of IL-12 and IL-15 in human natural killer cell activation by dendritic cells from secondary lymphoid organs. Proc Natl Acad Sci U S A (2004) 101:16606–11. doi:10.1073/pnas.0407522101
51. Vossen MT, Matmati M, Hertoghs KM, Baars PA, Gent MR, Leclercq G, et al. CD27 defines phenotypically and functionally different human NK cell subsets. J Immunol (2008) 180:3739–45. doi:10.4049/jimmunol.180.6.3739
52. Tomasello E, Yessaad N, Gregoire E, Hudspeth K, Luci C, Mavilio D, et al. Mapping of NKp46(+) cells in healthy human lymphoid and non-lymphoid tissues. Front Immunol (2012) 3:344. doi:10.3389/fimmu.2012.00344
53. Mebius RE, Kraal G. Structure and function of the spleen. Nat Rev Immunol (2005) 5:606–16. doi:10.1038/nri1669
54. Grégoire C, Cognet C, Chasson L, Coupet CA, Dalod M, Reboldi A, et al. Intrasplenic trafficking of natural killer cells is redirected by chemokines upon inflammation. Eur J Immunol (2008) 38:2076–84. doi:10.1002/eji.200838550
55. Cella M, Fuchs A, Vermi W, Facchetti F, Otero K, Lennerz JK, et al. A human natural killer cell subset provides an innate source of IL-22 for mucosal immunity. Nature (2008) 457:722–5. doi:10.1038/nature07537
56. Spits H, Artis D, Colonna M, Diefenbach A, Di Santo JP, Eberl G, et al. Innate lymphoid cells – a proposal for uniform nomenclature. Nat Rev Immunol (2013) 13:145–9. doi:10.1038/nri3365
57. Page G, Lebecque S, Miossec P. Anatomic localization of immature and mature dendritic cells in an ectopic lymphoid organ: correlation with selective chemokine expression in rheumatoid synovium. J Immunol (2002) 168:5333–41. doi:10.4049/jimmunol.168.10.5333
58. Paust S, Gill HS, Wang BZ, Flynn MP, Moseman EA, Senman B, et al. Critical role for the chemokine receptor CXCR6 in NK cell-mediated antigen-specific memory of haptens and viruses. Nat Immunol (2010) 11:1127–35. doi:10.1038/ni.1953
59. Peng H, Jiang X, Chen Y, Sojka DK, Wei H, Gao X, et al. Liver-resident NK cells confer adaptive immunity in skin-contact inflammation. J Clin Invest (2013) 123:1444–56. doi:10.1172/JCI66381
60. Kitaya K, Yasuda J, Nakayama T, Fushiki S, Honjo H. Effect of female sex steroids on human endometrial CD16neg CD56bright natural killer cells. Fertil Steril (2003) 79:730–4. doi:10.1016/S0015-0282(02)04818-5
61. Lukassen HG, Joosten I, van Cranenbroek B, van Lierop MJ, Bulten J, Braat DD, et al. Hormonal stimulation for IVF treatment positively affects the CD56bright/CD56dim NK cell ratio of the endometrium during the window of implantation. Mol Hum Reprod (2004) 10:513–20. doi:10.1093/molehr/gah067
62. Marlin R, Duriez M, Berkane N, de Truchis C, Madec Y, Rey-Cuille MA, et al. Dynamic shift from CD85j/ILT-2 to NKG2D NK receptor expression pattern on human decidual NK during the first trimester of pregnancy. PLoS One (2012) 7:e30017. doi:10.1371/journal.pone.0030017
63. Eriksson M, Meadows SK, Wira CR, Sentman CL. Unique phenotype of human uterine NK cells and their regulation by endogenous TGF-beta. J Leukoc Biol (2004) 76:667–75. doi:10.1189/jlb.0204090
64. Vacca P, Pietra G, Falco M, Romeo E, Bottino C, Bellora F, et al. Analysis of natural killer cells isolated from human decidua: evidence that 2B4 (CD244) functions as an inhibitory receptor and blocks NK-cell function. Blood (2006) 108:4078–85. doi:10.1182/blood-2006-04-017343
65. El Costa H, Casemayou A, Aguerre-Girr M, Rabot M, Berrebi A, Parant O, et al. Critical and differential roles of NKp46- and NKp30-activating receptors expressed by uterine NK cells in early pregnancy. J Immunol (2008) 181:3009–17. doi:10.4049/jimmunol.181.5.3009
66. Moffett A, Colucci F. Uterine NK cells: active regulators at the maternal-fetal interface. J Clin Invest (2014) 124:1872–9. doi:10.1172/JCI68107
67. Hanna J, Goldman-Wohl D, Hamani Y, Avraham I, Greenfield C, Natanson-Yaron S, et al. Decidual NK cells regulate key developmental processes at the human fetal-maternal interface. Nat Med (2006) 12:1065–74. doi:10.1038/nm1452
68. Lash GE, Schiessl B, Kirkley M, Innes BA, Cooper A, Searle RF, et al. Expression of angiogenic growth factors by uterine natural killer cells during early pregnancy. J Leukoc Biol (2006) 80:572–80. doi:10.1189/jlb.0406250
69. Xiong S, Sharkey AM, Kennedy PR, Gardner L, Farrell LE, Chazara O, et al. Maternal uterine NK cell-activating receptor KIR2DS1 enhances placentation. J Clin Invest (2013) 123:4264–72. doi:10.1172/JCI68991
70. Ashkar AA, Di Santo JP, Croy BA. Interferon gamma contributes to initiation of uterine vascular modification, decidual integrity, and uterine natural killer cell maturation during normal murine pregnancy. J Exp Med (2000) 192:259–70. doi:10.1084/jem.192.2.259
71. Hiby SE, Apps R, Sharkey AM, Farrell LE, Gardner L, Mulder A, et al. Maternal activating KIRs protect against human reproductive failure mediated by fetal HLA-C2. J Clin Invest (2010) 120:4102–10. doi:10.1172/JCI43998
72. Caligiuri MA, Murray C, Robertson MJ, Wang E, Cochran K, Cameron C, et al. Selective modulation of human natural killer cells in vivo after prolonged infusion of low dose recombinant interleukin 2. J Clin Invest (1993) 91:123–32. doi:10.1172/JCI116161
73. Baume DM, Robertson MJ, Levine H, Manley TJ, Schow PW, Ritz J. Differential responses to interleukin 2 define functionally distinct subsets of human natural killer cells. Eur J Immunol (1992) 22:1–6. doi:10.1002/eji.1830220102
74. Romagnani C, Juelke K, Falco M, Morandi B, D’Agostino A, Costa R, et al. CD56 bright CD16 − killer Ig-like receptor − NK cells display longer telomeres and acquire features of CD56 dim NK cells upon activation. J Immunol (2007) 178:4947–55. doi:10.4049/jimmunol.178.8.4947
75. Nagler A, Lanier LL, Phillips JH. Constitutive expression of high affinity interleukin 2 receptors on human CD16-natural killer cells in vivo. J Exp Med (1990) 171:1527–33. doi:10.1084/jem.171.5.1527
76. Matos ME, Schnier GS, Beecher MS, Ashman LK, William DE, Caligiuri MA. Expression of a functional c-kit receptor on a subset of natural killer cells. J Exp Med (1993) 178:1079–84. doi:10.1084/jem.178.3.1079
77. Nguyen S, Dhedin N, Vernant JP, Kuentz M, Al Jijakli A, Rouas-Freiss N, et al. NK-cell reconstitution after haploidentical hematopoietic stem-cell transplantations: immaturity of NK cells and inhibitory effect of NKG2A override GvL effect. Blood (2005) 105:4135–43. doi:10.1182/blood-2004-10-4113
78. Chan A, Hong DL, Atzberger A, Kollnberger S, Filer AD, Buckley CD, et al. CD56bright human NK cells differentiate into CD56dim cells: role of contact with peripheral fibroblasts. J Immunol (2007) 179:89–94. doi:10.4049/jimmunol.179.1.89
79. Root LL, Shipley GD. Normal human fibroblasts produce membrane-bound and soluble isoforms of FGFR-1. Mol Cell Biol Res Commun (2000) 3:87–97. doi:10.1006/mcbr.2000.0199
80. Williams EJ, Furness J, Walsh FS, Doherty P. Activation of the FGF receptor underlies neurite outgrowth stimulated by L1, N-CAM, and N-cadherin. Neuron (1994) 13:583–94. doi:10.1016/0896-6273(94)90027-2
81. Wu C, Li B, Lu R, Koelle SJ, Yang Y, Jares A, et al. Clonal tracking of rhesus macaque hematopoiesis highlights a distinct lineage origin for natural killer cells. Cell Stem Cell (2014) 14:486–99. doi:10.1016/j.stem.2014.01.020
82. Mace EM, Hsu AP, Monaco-Shawver L, Makedonas G, Rosen JB, Dropulic L, et al. Mutations in GATA2 cause human NK cell deficiency with specific loss of the CD56bright subset. Blood (2013) 121:2669–77. doi:10.1182/blood-2012-09-453969
83. Gineau L, Cognet C, Kara N, Lach FP, Dunne J, Veturi U, et al. Partial MCM4 deficiency in patients with growth retardation, adrenal insufficiency, and natural killer cell deficiency. J Clin Invest (2012) 122:821–32. doi:10.1172/JCI61014
84. van Helden MJ, Goossens S, Daussy C, Mathieu AL, Faure F, Marçais A, et al. Terminal NK cell maturation is controlled by concerted actions of T-bet and Zeb2 and is essential for melanoma rejection. J Exp Med (2015) 212:2015–25. doi:10.1084/jem.20150809
85. Vukicevic M, Chalandon Y, Helg C, Matthes T, Dantin C, Huard B, et al. CD56 bright NK cells after hematopoietic stem cell transplantation are activated mature NK cells that expand in patients with low numbers of T cells. Eur J Immunol (2010) 40:3246–54. doi:10.1002/eji.200940016
86. Dulphy N, Haas P, Busson M, Belhadj S, Peffault de Latour R, Robin M, et al. An unusual CD56brightCD16low NK cell subset dominates the early posttransplant period following HLA-matched hematopoietic stem cell transplantation. J Immunol (2008) 181:2227–37. doi:10.4049/jimmunol.181.3.2227
87. Yu J, Mao HC, Wei M, Hughes T, Zhang J, Park IK, et al. CD94 surface density identifies a functional intermediary between the CD56bright and CD56dim human NK-cell subsets. Blood (2010) 115:274–81. doi:10.1182/blood-2009-04-215491
88. Silva A, Andrews DM, Brooks AG, Smyth MJ, Hayakawa Y. Application of CD27 as a marker for distinguishing human NK cell subsets. Int Immunol (2008) 20:625–30. doi:10.1093/intimm/dxn022
89. Björkström NK, Riese P, Heuts F, Andersson S, Fauriat C, Ivarsson MA, et al. Expression patterns of NKG2A, KIR, and CD57 define a process of CD56 dim NK-cell differentiation uncoupled from NK-cell education. Blood (2010) 116:3853–64. doi:10.1182/blood-2010-04-281675
90. Juelke K, Killig M, Luetke-Eversloh M, Parente E, Gruen J, Morandi B, et al. CD62L expression identifies a unique subset of polyfunctional CD56dim NK cells. Blood (2010) 116:1299–307. doi:10.1182/blood-2009-11-253286
Keywords: CD56bright NK cell populations, tissue resident, lymphoid tissues, liver, uterus, NK cell development
Citation: Melsen JE, Lugthart G, Lankester AC and Schilham MW (2016) Human Circulating and Tissue-Resident CD56bright Natural Killer Cell Populations. Front. Immunol. 7:262. doi: 10.3389/fimmu.2016.00262
Received: 02 May 2016; Accepted: 21 June 2016;
Published: 30 June 2016
Edited by:
Eric Vivier, Centre d’Immunologie de Marseille-Luminy, FranceReviewed by:
Francisco Borrego, Cruces University Hospital, SpainFrancesco Colucci, University of Cambridge, UK
Copyright: © 2016 Melsen, Lugthart, Lankester and Schilham. This is an open-access article distributed under the terms of the Creative Commons Attribution License (CC BY). The use, distribution or reproduction in other forums is permitted, provided the original author(s) or licensor are credited and that the original publication in this journal is cited, in accordance with accepted academic practice. No use, distribution or reproduction is permitted which does not comply with these terms.
*Correspondence: Janine E. Melsen, j.e.melsen@lumc.nl