- 1Shanghai Key Laboratory for Prevention and Treatment of Bone and Joint Diseases with Integrated Chinese-Western Medicine, Shanghai Institute of Traumatology and Orthopedics, Ruijin Hospital, Shanghai Jiaotong University School of Medicine, Shanghai, China
- 2Department of Orthopedics, Shanghai Tenth People’s Hospital, Tongji University School of Medicine, Shanghai, China
- 3Guanghua Integrative Medicine Hospital and Institute of Arthritis Research, Shanghai Academy of Chinese Medical Sciences, Shanghai, China
- 4Experimental and Molecular Immunology and Neurogenetics (INEM), UMR 7355 CNRS and University of Orleans, Orleans, France
Functional cross-talk between osteoblasts and osteoclasts is a key process for bone homeostasis. Although osteoblast hypoxia-inducible factor-1α (HIF-1α) pathway activation results in impaired osteoclastogenesis via the direct regulation of osteoprotegerin (OPG), it is unclear whether there are other efficient mediators are involved in osteoblast HIF-1α pathway activation-restrained osteoclast formation. In addition to upregulated OPG, we observed that osteoblast HIF-1α activation led to increased interleukin-33 (IL-33) expression, which was found to inhibit osteoclastogenesis. Mechanistically, HIF-1α facilitates IL-33 expression by binding to −1,504/−1,500 bp on the Il-33 promoter. IL-33, thereby, acts on bone marrow-derived monocytes (BMMs) to reduce their osteoclastic differentiation. Moreover, microRNA-34a-5p (miR-34a-5p)-inhibited Notch1 activation was observed to play a central role in this process. Thereby, the identification of IL-33-miR-34a-5p-Notch1 pathway in the inhibitory effect of osteoblast HIF-1α pathway on osteoclastogenesis uncovers a new mechanism for understanding the effects of HIF-1α on bone remodeling.
Introduction
As a dynamic tissue, bone undergoes life-long remodeling: osteoblasts regulated bone formation and osteoclasts mediated bone destruction or resorption (1). The functional cross-talk between osteoblasts and osteoclasts is a key process for bone homeostasis. Both defects in the cells and obstruction of intercellular communication can lead to debilitating bone disorders such as osteopetrosis, osteoporosis, rheumatoid arthritis, and even tumor metastasizing to the bone (2, 3).
It has been well established that osteoblasts play a crucial role in the process of differentiation and maturation of osteoclasts by producing several factors that bind directly to osteoclastic progenitors (2). Besides expressing macrophage colony-stimulating factor (M-CSF) and receptor activator of nuclear factor kappa-B ligand (RANKL) to promote the survival, differentiation, and activation of osteoclast progenitors, osteoblasts also secrete osteoprotegerin (OPG), which acts as a decoy receptor, highly specific to RANKL, that inhibits the differentiation of osteoclasts (4). When under the effects of certain physical and chemical factors, osteoblasts can also express some cytokines to regulate osteoclast formation (5, 6). Studies have also revealed that differentiated osteoblasts can express interleukin-33 (IL-33) to block osteoclast formation from bone marrow-derived macrophages (7, 8), and that rates of osteoclastogenesis are higher in IL-33 receptor-ST2 deficient mice (8). However, whether IL-33 is involved in the cross-talk between osteoblasts hypoxia-inducible factor (HIF)-1α pathway activation and osteoclasts formation is unknown.
During bone development, osteoblasts are exposed to mild hypoxic conditions. Studies have shown that the oxygen-sensing pathway related proteins, such as von Hippel–Lindau tumor suppressor protein (VHL), prolyl hydroxylase-domain proteins (PHDs), and HIFs, are all expressed in osteoblasts (9). Our results reveal that HIF-1α in osteoblasts accelerates angiogenesis as well as osteogenesis via increasing vascular endothelial growth factor (VEGF) expression during bone development (10, 11), and upregulated VEGF expression in Vhl-deficient osteoblasts facilitates bone marrow stromal cells (BMSCs) proliferation and osteogenic differentiation (11). In addition to coordinating osteoblastic–angiogenic coupling, both VHL/HIF and PHD/HIF signaling pathways in osteoblasts have been proved to facilitate bone homeostasis by regulating osteoclastogenesis through the direct regulation of OPG (12, 13). Thus, besides increasing angiogenesis or osteoblastic activity, hypoxia/HIF-1α pathway activation pormotes bone development may also via disturbing osteoblast and osteoclast coupling and thereby dampens osteoclast activation. However, whether there are other efficient mediators besides OPG are involved in osteoblast HIF-1α pathway activation-restrained osteoclastogenesis still need for further exploration.
In the present study, we proved that, in addition to OPG, IL-33 is essential for osteoblast HIF-1α pathway-inhibited osteoclastogenesis. Furthermore, we provided evidence that IL-33 reduces osteoclastogenesis via the miR-34a-5p-Notch1 pathway.
Materials and Methods
Reagents
Alpha modification of Eagle medium (α-MEM), penicillin/streptomycin and fetal bovine serum were purchased from Gibco-BRL (Sydney, NSW, Australia). Recombinant soluble mouse M-CSF (Catalog#315-02) and mouse RANKL (Catalog#315-11) were purchased from Peprotech (USA). Primary antibodies of anti-HIF-1a (NB100-105), anti-Notch1 (NB100-78486), anti-Hes-1 (NBP1-30912), and anti-β-actin were purchased from Novus Biologicals (USA). IL-33 (3626-ML), anti-IL-33 (MAB3626), IL-33-neutralizing antibody (AF3626), OPG-neutralizing antibody (AF459), recombinant jagged-1 protein was provided by R&D Systems (USA). Desferrioxamine (DFO), the tartrate-resistant acid phosphatase (TRAP) staining kit was bought from Sigma Aldrich (St Louis, MO, USA).
Generation of Conditional Knockout Mice
Generation and genotyping analysis of the Vhlflox/flox and OC-Cre transgenic mice have previously been described (10). Briefly, Vhlflox/flox transgenic mice and mice containing osteocalcin (OC) promoter-driven Cre recombinase were intercrossed to generate osteoblast Vhl conditional knockout (Vhl-CKO) mice. Therefore, for all experiments with these mice, age- and sex-matched Cre-recombinase positive mice were used as VHL-CKO group while the Cre-recombinase negative mice were used as VHL-CON group. All procedures involving mice were performed in accordance with the Shanghai Jiaotong University Animal Study Committee.
Primary Osteoblasts Isolation and Conditioned Medium (CM) Preparation
The preparation of CM was generated as previously described (11). The calvariae of newborn Vhlflox/flox transgenic mice was used for primary osteoblasts isolation by serial round of digestion with 1.8 mg/ml type I collagenase (Sigma). To disrupt Vhl in vitro, the osteoblasts after three passages were infected with control adenovirus (Ad-GFP) or adenovirus expressing Cre recombinase (Ad-CRE, Vector Biolabs) at an MOI of 100. After incubation for 48 h, real-time RT-hypoxia response element (PCR) and western blot were conducted to detect the knock down efficiency of Vhl in osteoblasts.
Before the collection of culture medium of osteoblasts infected with Ad-GFP (CM-GFP) or culture medium of osteoblasts infected with Ad-CRE (CM-CRE), the cells were changed to incubate with α-MEM without serum or penicillin/streptomycin. In this study, the CM-GFP and CM-CRE were harvested after 24, 72, and 120 h and centrifuged at 3,000 rpm for 15 min, respectively, and then stored at −80°C. Additionally, 50% CM-GFP or CM-CRE was used in the following experiment and was not given special labeling in the figures.
In Vitro Osteoclastogenesis and Osteoclast Activity Assay
Tibiae and femurs of 8-week-old mice were used for primary bone marrow-derived monocytes (BMMs) isolation by flushing the bone marrow with α-MEM. BMMs were cultured in α-MEM for 16 h, then non-adherent cells were harvested and cultured in CM-GFP or CM-CRE complete medium supplemented with 50 ng/ml M-CSF for 3 days. Adherent cells were harvested as osteoclast progenitors and were further cultured with CM-GFP or CM-CRE containing M-CSF (30 ng/ml) and RANKL (50 ng/ml) for another 3–5 days. To investigate the other mediators besides of OPG in the inhibitory effect of CM-CRE on osteoclastogenesis, cells were differentiated withthe OPG-neutralizing antibody (200 ng/ml) as previously described (14). For the research of IL-33 on osteoclastogenesis, various concentrations of IL-33 or IL-33-neutralizing antibody were used to treated the BMMs. TRAP staining was performed according to the manufacturer’s instructions (Sigma-Aldrich). TRAP-positive cells with three or more nuclei were counted under a microscope (15). For the in vitro TRAP activity assay, cells were lysed by passive lysis buffer (Promega), 40 µl of supernatant was transferred to a 96 well plate and the test of TRAP activity was made with use of a Tartrate Resistant Acid Phosphatase Assay Kit (Beyotime Biotechnology, China). Absorbance at 405 nm was measured in a Tecan plate reader after 5~10 min of incubation. BCA Protein Assay Kit (Beyotime Biotechnology, China) was used to measure the protein concentration in each well. TRAP activity in each well was normalized to the corresponding protein concentration.
Bone Resorption Assay and F-Actin Ring Formation Assay
The bone resorption assay was carried out as previously report (16). Briefly, osteoclast progenitors were plated on bovine bone slices with a density of 8 × 104 cells/mL, cultured in CM-GFP or CM-CRE supplemented with 30 ng/mL M-CSF and 50 ng/mL RANKL. Osteoclasts adherented on the slices were removed out by sonication after 7 days of culture; the resorption pits stained with toluidine blue were photographed under a high-quality microscope. Resorption analysis was quantitated with the Image J software (Bethesda, MD, USA), three fields of view were randomly selected on each section.
To perform F-actin ring formation assay, osteoclasts treated with CM-GFP or CM-CRE were stained with rhodamine-conjugated phalloidin (Life Technologies) for 30 min at room temperature and then stained with 4,6-diamidino-2-phenylindole (Sigma) for nuclei detection. The F-actin ring formation was photograhped with a fluorescence microscope (Carl Zeiss, Jena, Germany), and the average number of normal F-actin rings was calculated as described (17).
Quantitative Real-time RT-PCR
Trizol reagent (Invitrogen, Carlsbad, CA, USA) was used for total RNA extraction. For reverse transcription of mRNA and miR-34a-5p, 1 µg of total RNA was used for reverse transcription with Prime-Script RT reagent kits: Cat#RR036A and Cat#RR037A (TaKaRa Biotechnology, Japan), respectively. Quantitative real-time PCR was performed to amplify the cDNA by the SYBR Premix Ex Tag kit (TaKaRa Biotechnology, Japan) and ABI 7500 Sequencing Detection System (Applied Biosystems, Foster City, CA, USA). β-actin and U6 were used as endogenous control for quantitation of mRNAs and miR-34a-5p, respectively. The specific primer sequences for real-time RT-PCR were described in Table S1 in Supplementary Material.
Bone Histomorphometry and Immunohistochemistry (IHC)
Bone tissues were embedded with paraffin after decalcification. 5 μm-thick sections were stained with hematoxylin and eosin (H&E) or TRAP according to standard methods, respectively. The ratio of osteoclast numbers to the trabecular bone surface (N.Oc/BS) was quantified with Osteomeasure Analysis System (Osteometrics, Atlanta, GA, USA) on TRAP-stained sections at 200× magnification.
For IHC analysis, deparaffinized sections were incubated with 3% H2O2 for 15 min, and then treated with 5% BSA for 10 min. Next, the sections were incubated with HIF-1α (1:50) and IL-33 (1:100) primary antibodies overnight at 4°C, respectively. Followed by incubated with biotin conjugated secondary antibodies, and visualized with the streptavidin-biotin staining technique. Nucleus was stained with hematoxylin and the slides were photographed by a microscope (ZEISS, AXIO).
MicroRNA (miRNA) Transfection
Cells were transfected with synthetic miRNA for anti-miR-34a (miR-34a antagomir) and its negative control (NC) using Lipofectamine™ RNAiMAX (Life Technologies). The miR-34a antagomir and NC were designed and synthesized by GenePharma (Shanghai, China). The sequences of miR-34a antagomir and NC used were as follows: miR-34a antagomir: 5′-CCAGCTAAGACACTGCCA-3′, NC: 5′-CAGUACUUUUGUGUAGU-3′. After incubation for 48 h, the cellular lysates were harvested to analysis the expression of genes of interest.
Western Blot Analysis
Cells were lysed with RIPA buffer supplemented with protease inhibitor cocktail (Sigma, St Louis, MO, USA). BCA protein assay kit (Beyotime Biotechnology, China) was used to determine the protein concentration. About 20 µg proteins were loaded, separated in 10% SDS-PAGE gels, and then transferred to PVDF membranes (Millipore, Bedford, MA, USA). Subsequently blocked with 5% skimmed milk solution and labeled with primary antibodies at 4°C overnight. Followed by immunoblot with peroxidase-conjugated secondary antibodies, the bands were visualized by the enhanced chemiluminescence detection system. β-actin was used as the endogenous control. Signal intensities were quantified using Image J software.
Enzyme-Linked Immunosorbent Assay (ELISA)
The concentration of IL-33 in osteoblasts culture supernatant were detected using Mouse IL-33 Quantikine ELISA Kit (M3300, R&D Systems) according to the manufacturer’s instructions.
Flow Cytometry Analysis for Osteoclast Progenitors
After incubation for the indicated times, osteoclast progenitors were harvested and made to a single-cell suspension and followed by incubated with FITC anti-CD11c (eBioscience), APC anti-CD206 (eBioscience) antibodies. Flow cytometric analysis was performed using FACS LSR II (BD Biosciences, San Jose, CA, USA).
Luciferase Reporter Assay
The −2,172~−134 bp of IL-33 encoding region was selected as the promoter. The promoter region was analyzed in the JASPAR core database, which revealed the presence of putative hypoxia response element (HRE) at −1,504/−1,500 bp relative to the transcription start site on IL-33 promoter, whose specific sequences is ACGTG. Then, IL-33 promoter reporter (−2,172~−134 bp, including the WT and mutants with putative binding site detection) was directly cloned into a pGL3-Basic luciferase vector. Primers used for amplifying mouse IL-33 WT and mutated promoters by PCR were as follows: WT, forward: 5-CTACTCACTAGCGCATGATTCAG-3, reverse: 5-CGATGATTCTGCCGTGATTTCTCC-3; mutant with putative binding site deletion, bp −1,504 to bp −1,500, forward: 5-TAACTTACTCTTCCTACGGGAGGGTAGTCACTCT-3, reverse: 5-AGAGTGACTACCCTCCCGTAGGAAGAGTAAGTTA-3. After 24 h of Ad-CRE incubation, mouse primary osteoblasts were seeded into 24-well plates that were co-transfected with different plasmids (firefly reporter constructs containing the WT or mutant IL-33 promoter and a Renilla-expressing plasmid). Firefly and Renilla luciferase activities were measured 24-h post-transfection by a Dual Luciferase Assay System (Promega).
Statistical Analysis
Data were collected from three or more independent experiments and expressed as mean ± SEM. A two-sided Student’s t-test was used to analyze the difference between groups. One-way analysis of variance was performed to show the difference between groups. P < 0.05 was considered significantly different.
Results
Osteoblast HIF-1α Pathway Mediates Osteoclastogenesis Partly through IL-33
To detect functional cross-talk between osteoblasts HIF-1α pathway activation and osteoclastogenesis, we activated the HIF-1α pathway via the Cre-Loxp system to knockdown Vhl in osteoblasts. Compared with the control adenovirus (Ad-GFP) treated group, transfected with the Ad-CRE in osteoblasts significantly increased HIF-1α expression (Figure 1A). Next, we examined the effect of osteoblast HIF-1α activation on osteoclast formation. TRAP staining and TRAP activity assays results revealed that compared with group stimulated with the conditioned culture medium of osteoblasts infected with Ad-GFP (CM-GFP), the conditioned culture medium of osteoblasts infected with Ad-CRE (CM-CRE) treatment strongly reduced osteoclastogenesis (Figures 1B–D). Furthermore, the F-actin ring (Figures 1E,F) and resorption pit formation (Figures 1G,H) assay demonstrated that HIF-1α activation in osteoblasts significantly inhibited mature osteoclast formation and osteoclastic bone resorption.
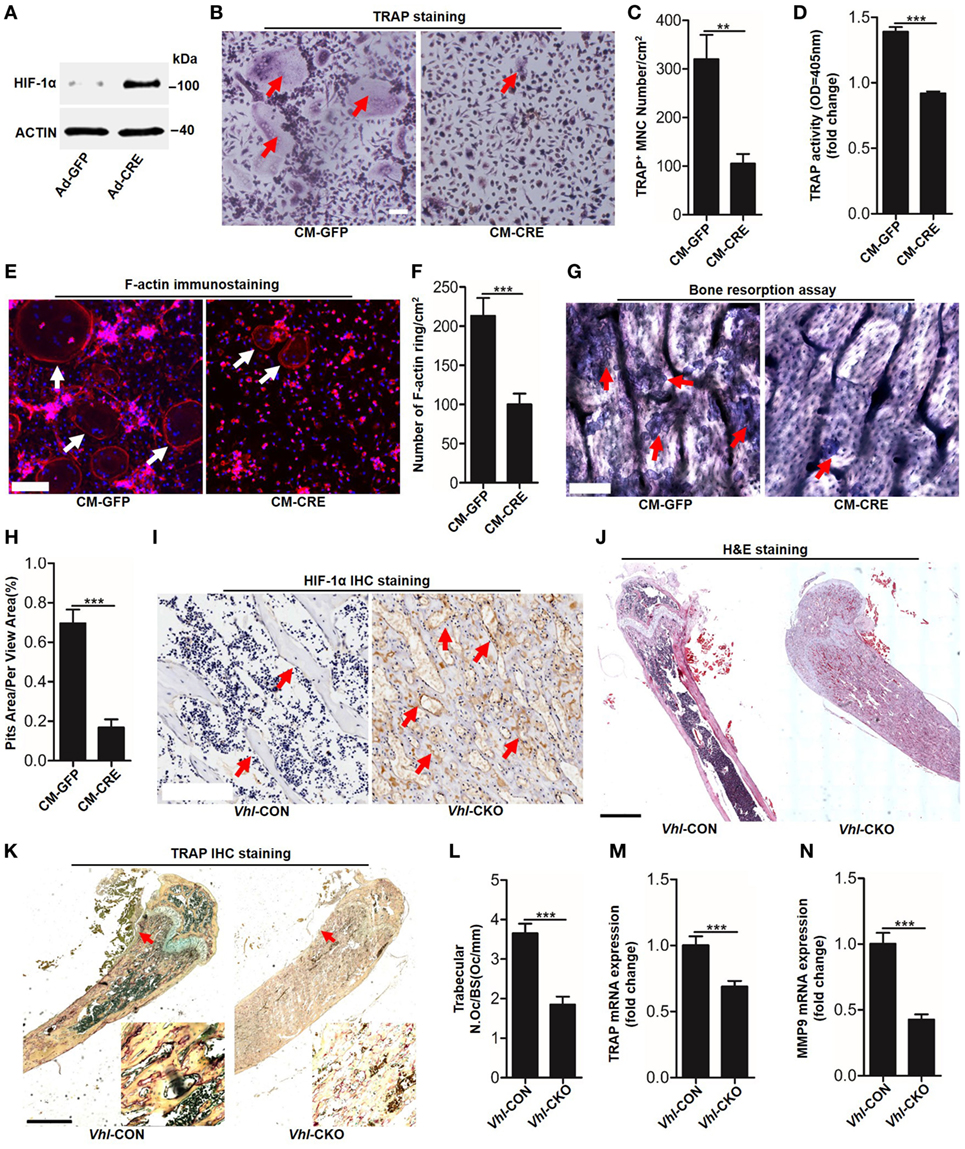
Figure 1. Hypoxia-inducible factor (HIF)-1α activation in osteoblasts restrains osteoclastogenesis. (A) Western blot analysis of HIF-1α in osteoblasts after transfected with Ad-GFP or Ad-CRE. (B–D) Bone marrow-derived monocytes (BMMs) osteoclastic formation and tartrate-resistant acid phosphatase (TRAP) activity assay after treating with the CM-CRE or CM-GFP. Red arrows indicated multinucleated osteoclasts. (E–H) F-actin staining and resorption pit formation in BMMs after treating with the CM-CRE or CM-GFP. White arrows indicated F-actin rings, red arrows indicated resorption pits. (I) Immunohistochemical staining of HIF-1α in trabecular bone sections from Vhl-CON and Vhl conditional knockout (Vhl-CKO) mice. (J) H&E staining of trabecular bone sections from Vhl-CON and Vhl-CKO mice. (K,L) TRAP staining of trabecular bone sections from Vhl-CON and Vhl-CKO mice. Arrows designate the region of 200× magnification shown in the lower right corner. (M,N) Quantification of TRAP and MMP9 mRNA levels in trabecular bone tissues from Vhl-CON and Vhl-CKO mice. Scale bars represent 100 µm. Ad-CRE represents adenovirus expressing Cre recombinase, while Ad-GFP represents the control adenovirus. CM-CRE represents the culture medium of osteoblasts transfected with Ad-CRE, while CM-GFP represents the culture medium of osteoblasts transfected with Ad-GFP. Vhl-CKO means Cre-recombinase positive mice while Vhl-CON means Cre-recombinase negative mice. **P < 0.01, ***P < 0.001. P values were analyzed by t-test.
Consistent with the in vitro results, the inhibitory effect of HIF-1α activation on osteoclast formation was further demonstrated in vivo. Immunohistochemical staining showed that there were higher numbers of HIF-1α positive cells surrounding the trabecular bone of Vhl-conditional knockout mice (Cre-recombinase positive mice, Vhl-CKO) than the control mice (Cre-recombinase negative mice, Vhl-CON) (Figure 1I). Hematoxylin and eosin (H&E) staining revealed more trabecular bone formation in the Vhl-CKO mice than in the Vhl-CON mice (Figure 1J). In line with these results, TRAP staining analysis, quantification of TRAP, and MMP9 mRNA levels in trabecular bone tissues revealed that osteoclastogenesis was reduced in the Vhl-CKO mice compared to the Vhl-CON mice (Figures 1K–N).
Osteoblasts HIF-1α signaling pathway activation by Vhl or PHD silencing plays a critical role in bone homeostasis by regulating osteoclastogenesis through OPG (12, 13). However, it is unclear whether osteoblast HIF-1α activation can mediate osteoclastogenesis through other “paracrine” approaches. To test it, we blocked the function of OPG by applying an OPG-specific neutralizing antibody. The results of TRAP staining (Figures 2A,B), TRAP activity assays (Figure 2C) and osteoclastogenesis related genes expression, such as Trap (Figure 2D), cathepsin-K (Figure 2E), dendritic cell specific transmembrane protein (Dc-stamp) (Figure 2F), and nuclear factor of activated T cells c1 (NFATc1) (Figure 2G) showed that OPG neutralizing antibody significantly dampened the inhibitory effect of CM-CRE on osteoclastogenesis. Simultaneously, the results also revealed that the OPG neutralizing antibody could not completely restore the osteoclast formation to the same level as the control group, which suggested that osteoblast HIF-1α pathway activation-restrained osteoclastogenesis might through other mediators.
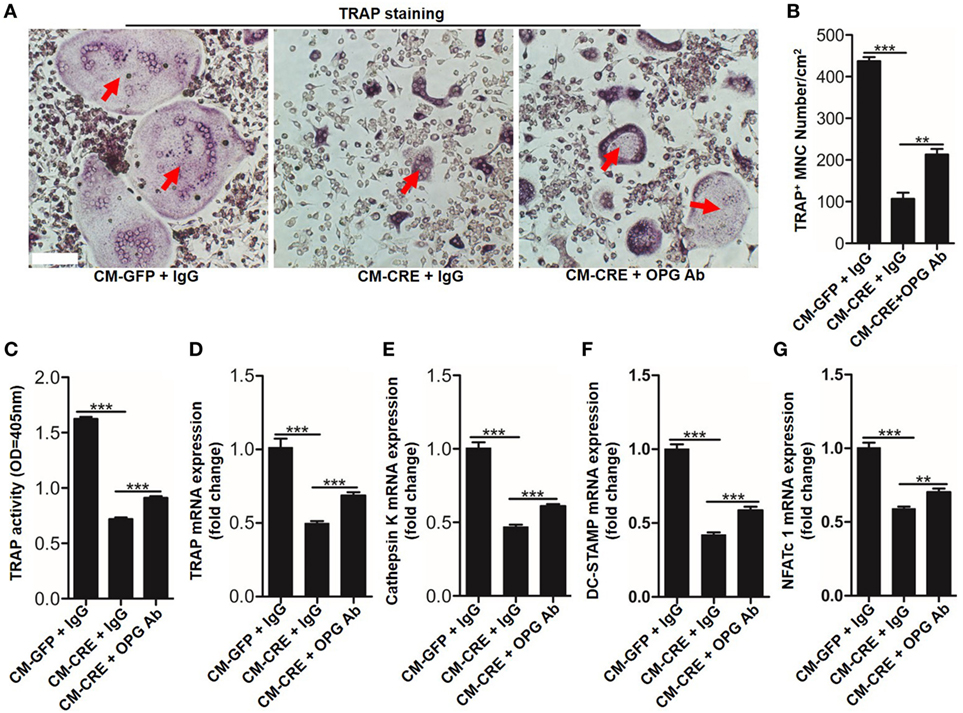
Figure 2. Hypoxia-inducible factor (HIF)-1α activation in osteoblasts restrains osteoclastogenesis in part through osteoprotegerin (OPG). (A–C) BMMs osteoclastic formation and tartrate-resistant acid phosphatase (TRAP) activity assay after treated with CM-GFP and CM-CRE in the presence of OPG neutralizing antibody. Red arrows indicated multinucleated osteoclasts. (D–G) Quantification of Trap, Cathepsin K, Dc-stamp, and Nfatc1 expression in BMMs treated with CM-GFP and CM-CRE in the presence of OPG neutralizing antibody. Scale bars represent 100 µm. CM-CRE represents the culture medium of osteoblasts transfected with Ad-CRE, while CM-GFP represents the culture medium of osteoblasts transfected with Ad-GFP. ***P < 0.001. P values were analyzed by one-way ANOVA.
We next sought to identify which mediators took part in osteoblast HIF-1α pathway-inhibited osteoclastogenesis. PCR screening assay results showed that besides OPG, Vhl silence in osteoblasts increased Il-33 expression (Figure 3A). Increased IL-33 expression in Vhl-silenced osteoblasts was also demonstrated by western blot analysis (Figure 3B). As IL-33 has been proven to be an effective inhibitor of osteoclast formation by bone marrow precursors (7, 8), we predicted that IL-33 might play a role in osteoblast HIF-1α pathway-mediated osteoclastogenesis. To test our hypothesis, we first detected IL-33 secretion after Vhl knockdown. ELISA results showed that after 5 days of Ad-CRE transfection, there was approximately 600 pg/ml IL-33 in the culture medium of the osteoblasts, and the concentration of IL-33 in the control group was approximately 200 pg/ml (Figure 3C). Real-time PCR and immunohistochemical staining revealed that IL-33 levels were strongly increased in the trabecular bone of Vhl-CKO mice compared to those in the Vhl-CON mice (Figures 3D,E). Our results in Figure 1 have demonstrated that osteoclastogenesis was reduced in Vhl-CKO mice, and osteoclasts are formed from monocytic progenitors of the hematopoietic lineage. We then measured IL-33 concentration in the bone marrow cavity by ELISA and found that concentration of IL-33 was much higher in the Vhl-CKO mice than in the Vhl-CON mice (Figure 3F). Taken together, these results demonstrate that HIF-1α pathway activation increased IL-33 expression in osteoblasts.
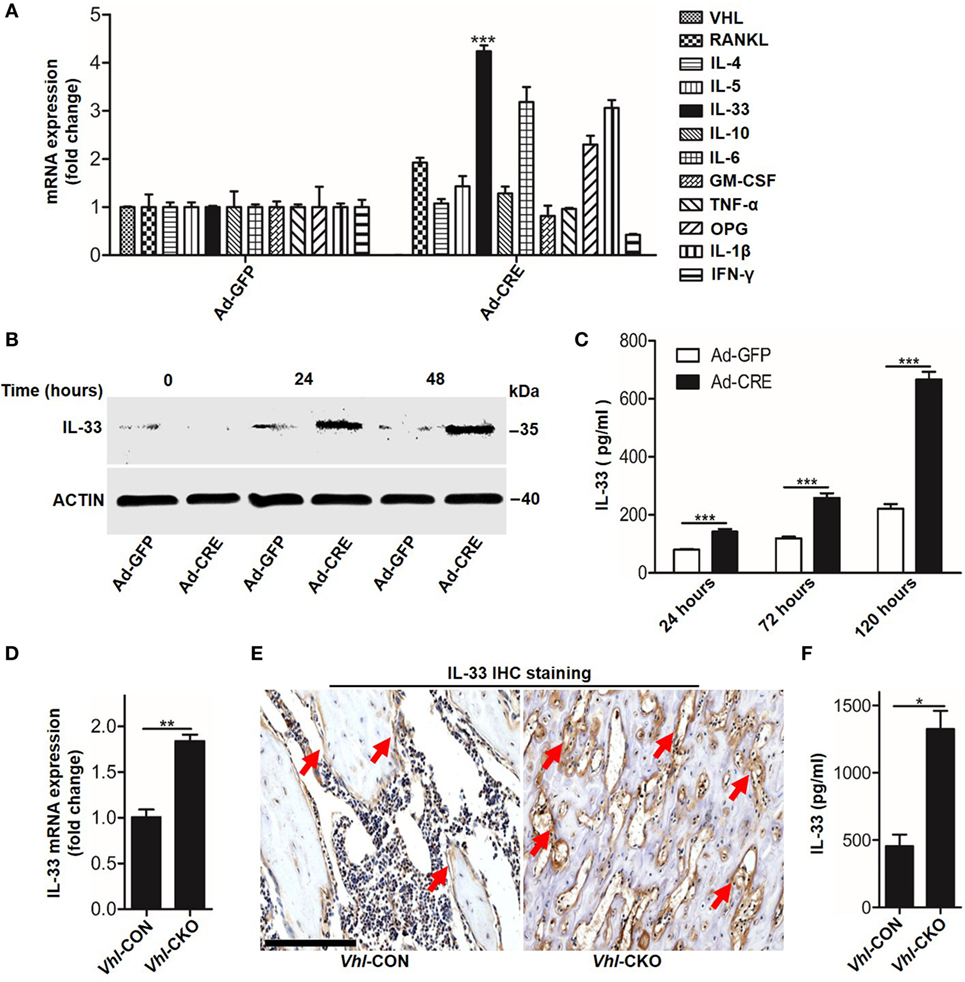
Figure 3. Hypoxia-inducible factor (HIF)-1α activation in osteoblasts increases interleukin-33 (IL-33) expression. (A) Quantification of expression of different genes in osteoblasts transfected with Ad-GFP or Ad-CRE. (B) Western blot analysis of IL-33 in osteoblasts at different times following Ad-GFP and Ad-CRE transfection. (C) Enzyme-linked immunosorbent assay (ELISA) quantification of IL-33 in the supernatant of osteoblast culture medium at different times following Ad-GFP and Ad-CRE transfection. (D) Quantification of IL-33 mRNA levels in trabecular bone tissues from Vhl-CON and Vhl conditional knockout (Vhl-CKO) mice. (E) Immunohistochemical staining of IL-33 in trabecular bone sections from Vhl-CON and Vhl-CKO mice. Red arrows indicated IL-33-positive cells. (F) ELISA quantification of IL-33 in the trabecular bone marrow cavities of Vhl-CON and Vhl-CKO mice. Scale bars represent 100 µm. Ad-CRE represents adenovirus expressing Cre recombinase, while Ad-GFP represents the control adenovirus. CM-CRE represents the culture medium of osteoblasts transfected with Ad-CRE, while CM-GFP represents the culture medium of osteoblasts transfected with Ad-GFP. Vhl-CKO means Cre-recombinase positive mice, while Vhl-CON means Cre-recombinase negative mice. **P < 0.01, ***P < 0.001. P values were analyzed by t-test.
Having observed that HIF-1α pathway activation increased IL-33 expression, we next sought to explore whether IL-33 was involved in osteoblast HIF-1α pathway-regulated osteoclastogenesis. We first blocked IL-33 activation in the culture medium by applying an IL-33 neutralizing antibody. TRAP staining, TRAP activity analysis, and osteoclast formation related genes expression all demonstrated that the inhibitory effect of osteoblast HIF-1α activation on osteoclastogenesis was significantly reduced when IL-33 was blocked (Figures 4A–H). Consistent with previous reports (8), we found that IL-33 inhibited osteoclast formation from BMMs in a dose-dependent manner. Concentrations as low as 5 ng/ml of IL-33 significantly reduced osteoclast formation and expression of osteoclast formation-related genes (Figure S1 in Supplementary Material). Moreover, we found that IL-33 inhibited the process of osteoclastogenesis at an early stage, as adding IL-33 in the early stage (0–3 and 0–6 days) had a stronger inhibitory effect on osteoclast formation, TRAP activity and expression of osteoclast formation-related genes than in the late stage (3–6 days) (Figures 4I–O). In addition, the increased percentage of M2 macrophages (CD206+) and the decreased proportion of M1 macrophages (CD11C+) revealed that IL-33 and CM-CRE facilitates M2 macrophages differentiation of BMMs (Figure S2 in Supplementary Material), which is also consistent with previous report (8). Taken together, these data demonstrate that IL-33 is involved in osteoblast HIF-1α pathway activation-inhibited osteoclastogenesis.
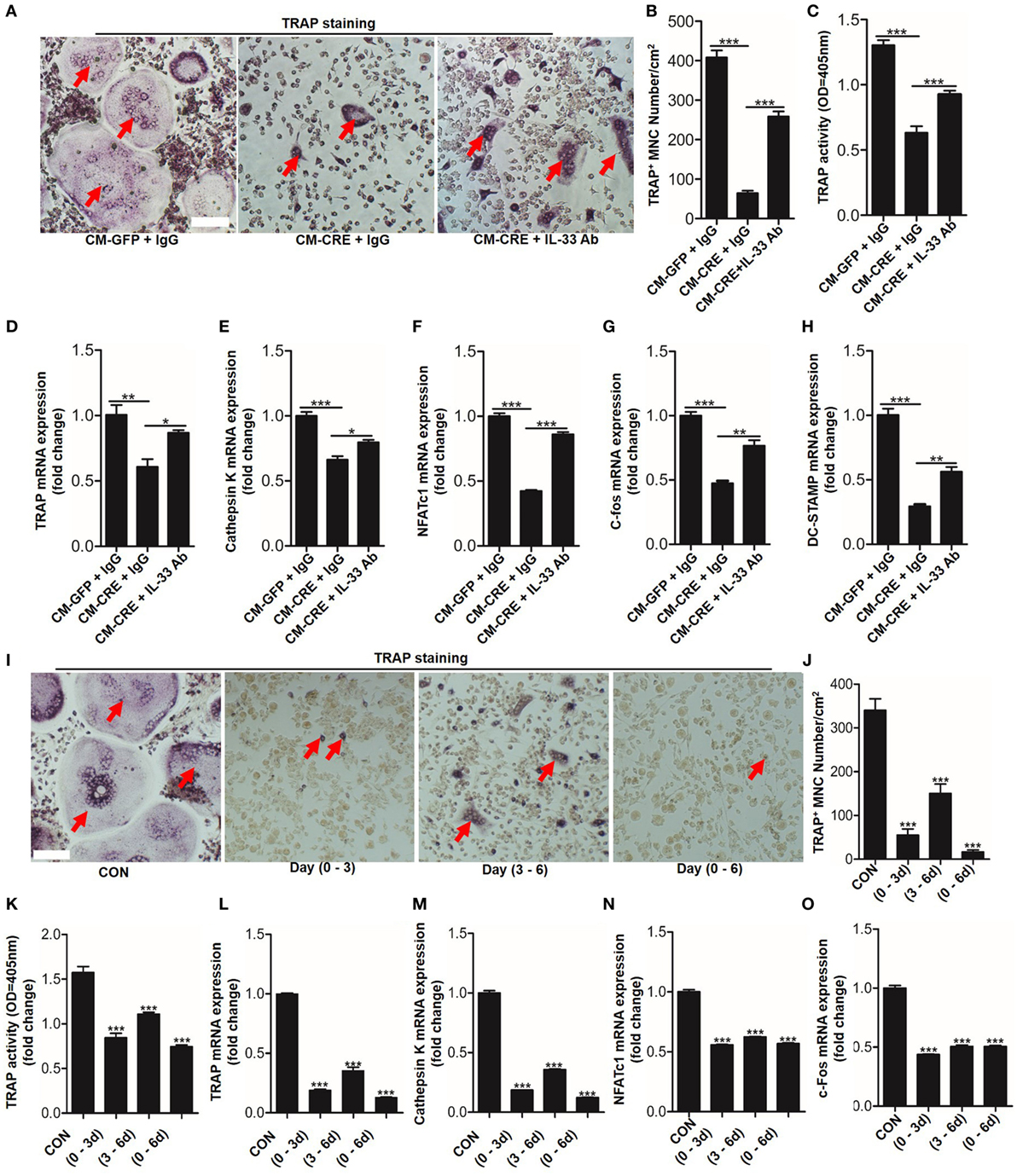
Figure 4. Interleukin-33 (IL-33) is involved in osteoblast hypoxia-inducible factor (HIF)-1α pathway-mediated bone marrow-derived monocytes (BMMs) osteoclastogenesis. (A–C) BMMs osteoclastic formation and tartrate-resistant acid phosphatase (TRAP) activity assay after treatment with the CM-GFP and CM-CRE in the presence of IL-33 neutralizing antibody. Red arrows indicated multinucleated osteoclasts. (D–H) Quantification of Trap, Cathepsin K, Nfatc1, C-fos, and Dc-stamp mRNA levels in BMMs treated with the CM-GFP and CM-CRE in the presence of IL-33 neutralizing antibody. (I–K) IL-33 (20 ng/ml) inhibits BMMs osteoclastic formation and TRAP activity at an early stage. Red arrows indicated multinucleated osteoclasts. (L–O) IL-33 inhibits Trap, Cathepsin K, Nfatc1, and C-fos mRNA expression in BMMs at an early stage. Scale bars represent 100 µm. CM-CRE represents the culture medium of osteoblasts transfected with Ad-CRE, while CM-GFP represents the culture medium of osteoblasts transfected with Ad-GFP. (1–3) means treated BMMs from day 1 to day 3, (1–6) means treated BMMs from day 1 to day 6, (3–6) means treated BMMs from day 3 to day 6. *P < 0.05, **P < 0.01, ***P < 0.001. P values were analyzed by one-way ANOVA.
HIF-1α Mediates IL-33 Expression by Regulating Il-33 Promoter Activity
Having observed that IL-33 was essential for osteoblast HIF-1α pathway-inhibited osteoclastogenesis, we next sought to explore the underlying mechanisms by which the HIF-1α pathway regulates IL-33 expression. As Vhl silencing lead to HIF-1α accumulation in the nucleus, where it forms a dimer with the HIF-1β subunit through its bHLH-PAS domain and binds to the promoter region of target genes to facilitate their expression, we hypothesized that HIF-1α might bind to the promoter of Il-33 to promote its expression. To test this hypothesis, we used the dual-luciferase reporter gene assay system to detect promoter activity of IL-33 in the presence of HIF-1α activation. The results showed that in parallel with HIF-1α (Figures 5A,B), IL-33 (Figures 5A–D), and VEGFA (Figure S3 in Supplementary Material) upregulation, Il-33 promoter activity was also significantly increased under hypoxia (Figure 5E) or Ad-CRE transfection (Figure 5F). We next sought to identify the (HRE) in the Il-33 promoter. Analysis of mouse Il-33 promoter sequence using the JASPAR core database (18) revealed the presence of one putative HIF-1α binding site at −1,504/−1,500 bp on the mouse Il-33 promoter, whose specific sequence is ACGTG. To further examine if the predicted binding site is necessary for IL-33 promoter regulation by HIF-1α, we deleted the putative binding site. Cells were transfected with the WT and deleted constructs and luciferase activity was measured following Vhl-CRE adenovirus transfection in osteoblasts. The results showed that −1,504/−1,500 bp deletion caused a decrease in Il-33 promoter activity compared to the WT promoter (Figure 5G). Since DFO has been proven to be a potent HIF-1α activator, acting by inhibiting prolyl hydroxylases in osteoblasts (19), we used DFO as an inducer of HIF-1α in osteoblasts. The results showed that HIF-1α and IL-33, as well as Il-33 promoter activity, were abundantly increased in the osteoblasts in response to DFO stimulation (Figures 5H–K). However, the promoter activity induced by DFO was significantly reduced after the −1,504/−1,500 bp deletion (Figure 5L). Together, these results predict that HIF-1α regulates Il-33 promoter activity by binding to −1,504/−1,500 bp on the Il-33 promoter.
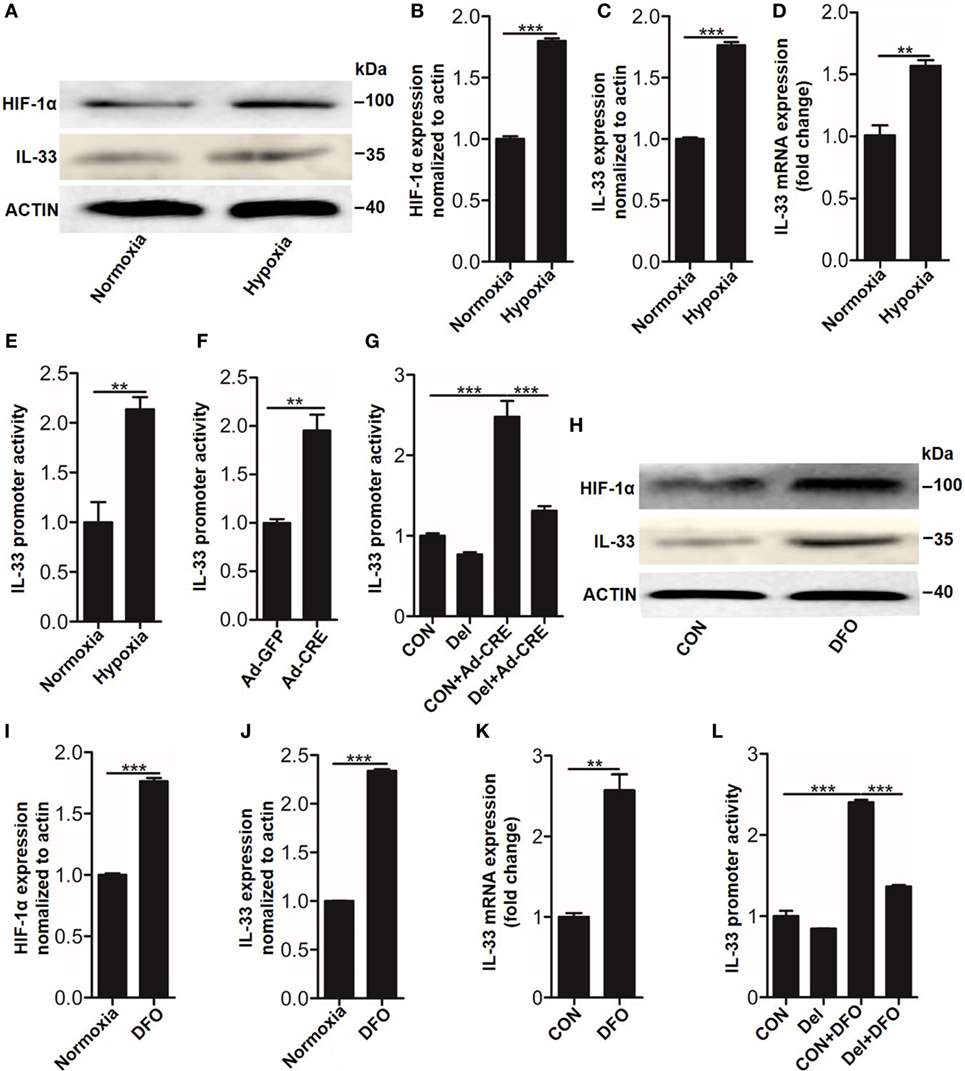
Figure 5. Hypoxia-inducible factor (HIF)-1α regulates interleukin-33 (IL-33) expression by binding to the promoter region of Il-33. (A–D) HIF-1α and IL-33 expression in osteoblasts under hypoxia. (E,F) Il-33 promoter activity detected by the dual-luciferase reporter gene assay system in osteoblasts under hypoxia and after Vhl knockdown. (G) Il-33 promoter activity induced by Ad-CRE and Ad-GFP in osteoblasts with or without putative HIF-1α binding site deletion in the Il-33 promoter. (H–K) HIF-1α and IL-33 expression in osteoblasts induced by desferrioxamine (DFO). (L) Il-33 promoter activity induced by 12 µM DFO in osteoblasts with or without putative HIF-1α binding site deletion in the Il-33 promoter. Ad-CRE represents adenovirus expressing Cre recombinase, while Ad-GFP represents the control adenovirus. Del represents the constructs of IL-33 promoter with putative binding site detection. **P < 0.01, ***P < 0.001. P values were analyzed by t-test in (B–D) and one-way ANOVA in (E,G,H).
IL-33 Mediates BMMs Osteoclastic Differentiation via the MicroRNA-34a-Notch1 Pathway
Recently, studies have shown that non-coding miRNAs play an important role in osteogenesis (20–22). However, whether these molecules are implicated in the process of IL-33-repressed osteoclastic differentiation is still unknown. To explore this issue, the expression of 27 miRNAs, which are involved in BMMs differentiation, were quantitated in response to 20 ng/ml IL-33 stimulation in osteoclast progenitors. Among these miRNAs, 8 were upregulated, 18 were downregulated, while miRNA-224-5p was detected with no significant change (Figure S4 in Supplementary Material). Interestingly, we found the increase of miR-34a-5p was most pronounced among these upregulated miRNAs (Figure S4A in Supplementary Material). Given that miR-34a-5p has been proved to work as a suppressor in osteoblast differentiation (20), we hypothesized that miR-34a-5p might be involved in IL-33-restrained osteoclast formation. Quantification mRNA expression results showed that IL-33 induced miR-34a-5p expression in a dose-dependent manner in osteoclast progenitors (Figure 6A). TRAP staining and TRAP activity assay results revealed that the inhibitory effect of IL-33 on osteoclast formation was strongly reduced in the presence of miR-34a-5p antagomir (Figures 6B–D). Lastly, expression of osteoclast formation related genes, such as Trap, capthekin-K, Nfatc1, and C-fos, further demonstrated that IL-33 inhibited the process of osteoclastogenesis via miR-34a-5p, as these genes that decrease expression in the IL-33 group were significantly restored in the presence of miR-34a-5p antagomir (Figures 6E–H).
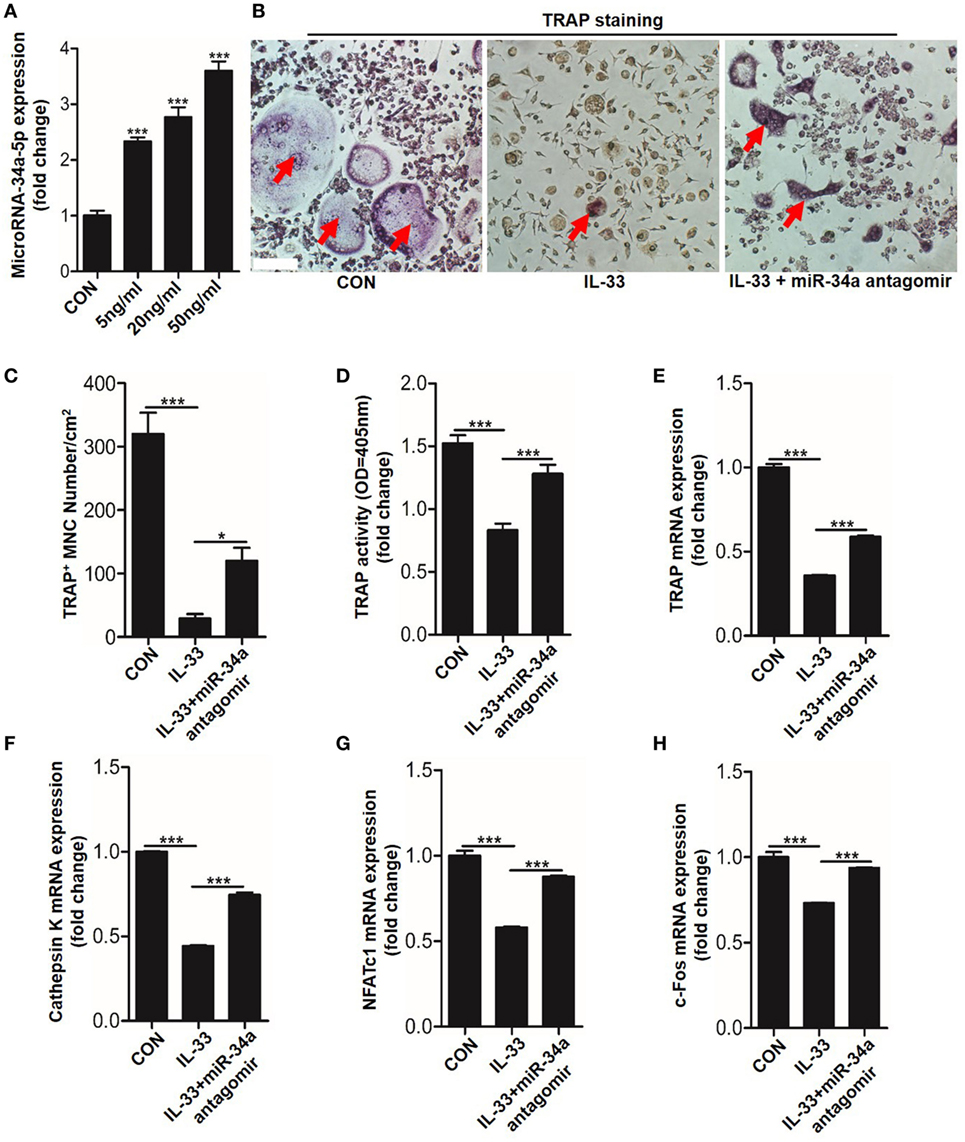
Figure 6. Interleukin-33 (IL-33) restrains bone marrow-derived monocytes (BMMs) osteoclastogenesis by increasing miR-34a-5p expression. (A) Quantification of miR-34a-5p expression induced by different doses of IL-33 in osteoclast progenitors. (B–D) BMMs osteoclastic formation and tartrate-resistant acid phosphatase (TRAP) activity assay after treatment with 20 ng/ml IL-33 in the presence of miR-34a-5p antagomir. Red arrows indicated multinucleated osteoclasts. Scale bars represent 100 µm. (E–H) Quantification of Trap, Cathepsin K, Nfatc1, and C-fos expression in osteoclast progenitors treated with 20 ng/ml IL-33 in the presence of miR-34a-5p antagomir. *P < 0.05, ***P < 0.001. P values were analyzed by one-way ANOVA.
We next sought to test whether miR-34a-5p was also involved in CM-CRE-restrained osteoclast formation. Real-time RT-PCR result revealed that miR-34a-5p was significantly upregulated upon CM-CRE incubation (Figure 7A). Furthermore, TRAP staining, TRAP activity assay and the quantitation of Trap, capthekin-K, Nfatc1, and C-fos expression results demonstrated that miR-34a-5p was also crucial for CM-CRE-inhibited osteoclastogenesis (Figures 7B–H).
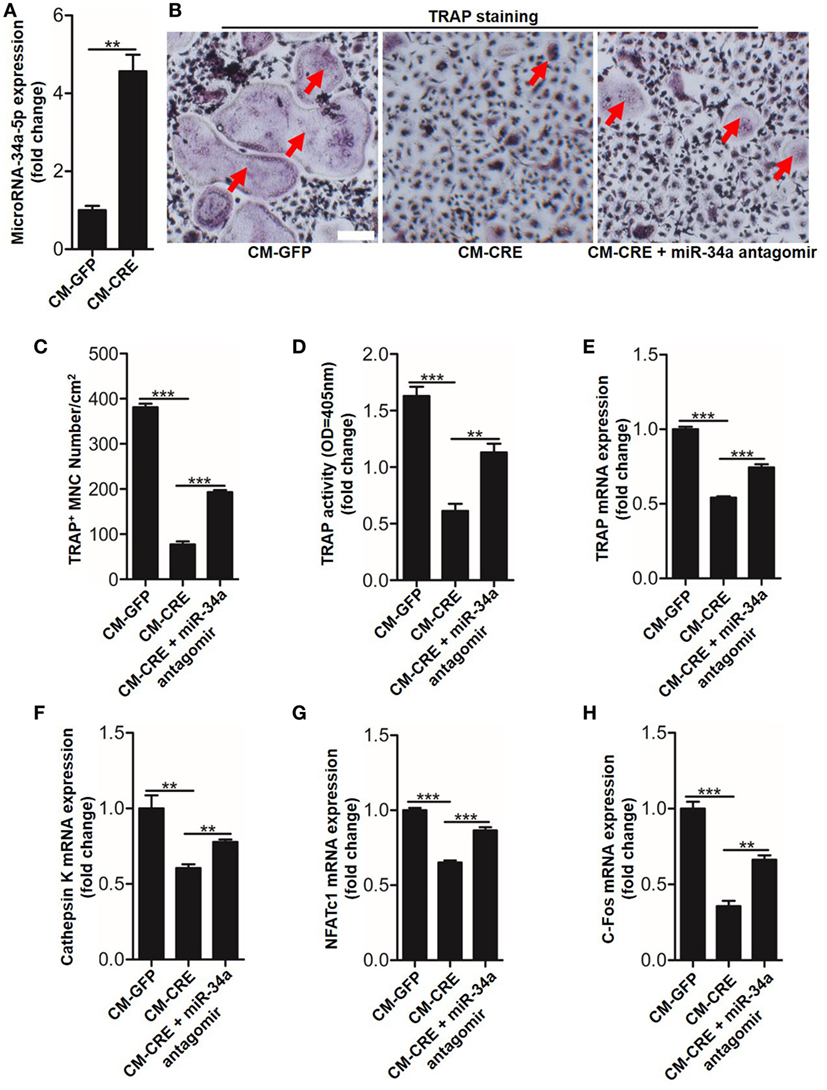
Figure 7. CM-CRE restrains bone marrow-derived monocytes (BMMs) osteoclastogenesis by increasing miR-34a-5p expression. (A) Quantification of miR-34a-5p expression induced by CM-CRE in osteoclast progenitors. (B–D) BMMs osteoclastic formation and tartrate-resistant acid phosphatase (TRAP) activity assay incubated with CM-CRE in the presence of miR-34a-5p antagomir. Red arrows indicated multinucleated osteoclasts. Scale bars represent 100 µm. (E–H) Quantification of TRAP, Cathepsin K, Nfatc1, and C-fos mRNA levels in osteoclast progenitors incubated with CM-CRE in the presence of miR-34a-5p antagomir. Scale bars represent 100 µm. **P < 0.01, ***P < 0.001. P values were analyzed by t-test in (A), one-way ANOVA.
Having observed the critical role of miR-34a-5p in CM-CRE and IL-33-inhibited osteoclastogenesis, we next sought to detect the underlying mechanism by which miR-34a-5p mediates osteoclast formation. Since several studies have described the role of Notch1 in osteoclast formation (23) and our previous work has demonstrated that miR-34a-5p mediates bone marrow stromal cell osteogenic differentiation via Notch1 (21), we hypothesized that miR-34a-5p regulates osteoclast formation through Notch1. Our results showed that the expression of Notch1, the Notch1 ligand Jagged1 (Jag1), and its target gene hairy and enhancer of split-1 (Hes1) in BMMs were strongly reduced by CM-CRE and IL-33. However, this effect was significantly weakened by application of miR-34a-5p antagomir (Figures 8A–H; Figure S5 in Supplementary Material). Together, these results demonstrate that miR-34a-5p is the mediator of CM-CRE and IL-33-decreased Notch1 pathway activity.
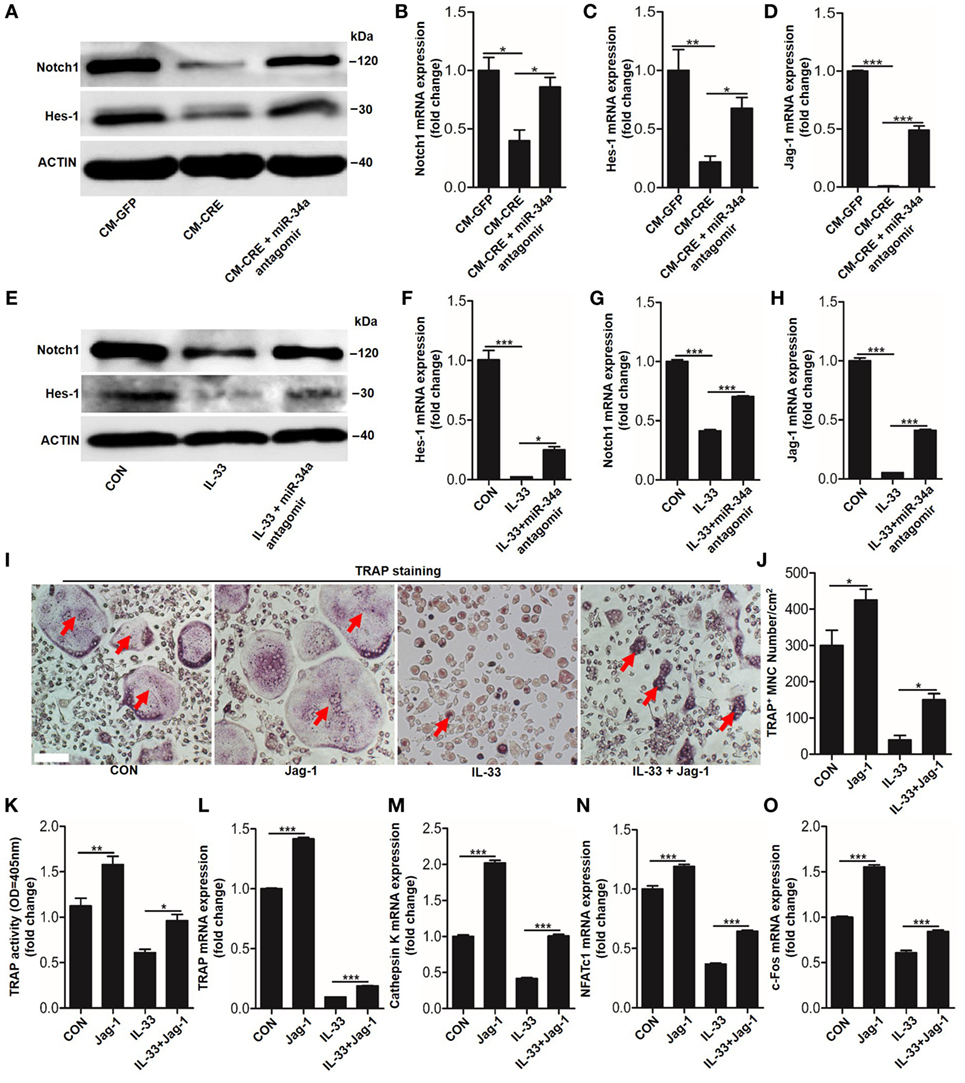
Figure 8. Interleukin-33 (IL-33) decreases bone marrow-derived monocytes (BMMs) osteoclastogenesis by inhibiting Notch1 activity. (A) Western blot analysis of Notch1 and Hes-1 expression in osteoclast progenitors incubated with CM-CRE in the presence of miR-34a-5p antagomir. (B–D) Quantification of Notch1, Jag1, and Hes-1 expression in osteoclast progenitors incubated with CM-CRE in the presence of miR-34a-5p antagomir. (E) Western blot analysis of Notch1 and Hes-1 expression in osteoclast progenitors induced by IL-33 in the presence of miR-34a-5p antagomir. (F–H) Quantification of Notch1, Jag1, and Hes-1 expression in osteoclast progenitors treated with IL-33 in the presence of miR-34a-5p antagomir. (I–K) BMMs osteoclastic formation and tartrate-resistant acid phosphatase (TRAP) activity assay after treatment with 20 ng/ml IL-33 in the presence of Jag-1. Red arrows indicated multinucleated osteoclasts. Scale bars represent 100 µm. (L–O) Quantification of Trap, Cathepsin K, Nfatc1, and C-fos expression in osteoclast progenitors treated with 20 ng/ml IL-33 in the presence of Jag-1. *P < 0.05, **P < 0.01, ***P < 0.001. P values were analyzed by one-way ANOVA.
Next, we explored whether reduced Notch1 activity was essential for IL-33 inhibited-osteoclast formation. TRAP staining and TRAP activity assay results showed that activation of Notch1 by JAG1 restored IL-33-inhibited osteoclast formation (Figures 8I–K). In addition, the quantification of mRNA levels of TRAP, cathepsin-K, Nfatc1, and C-fos further demonstrated that IL-33-inhibited osteoclastogenesis by decreasing Notch1 activity (Figures 8L–O).
Discussion
Osteoblasts have been reported to play a pivotal role in osteoclasts differentiation and function by several different mechanisms (3). Although some studies have revealed that osteoblasts HIF-1α pathway activation results in impaired osteoclastogenesis via the direct regulation of OPG (12, 13), whether there are other mediators are involved in osteoblast HIF-1α pathway activation-restrained osteoclasts formation still need for further investigation. Here, we proved that in addition to upregulation of OPG, HIF-1α activation in osteoblasts by Vhl silencing leads to increased IL-33 expression, and IL-33 plays a role in inhibiting osteoclastogenesis. Mechanistically, HIF-1α facilitates IL-33 expression by binding at −1,504/−1,500 bp on the Il-33 promoter. IL-33 then acts through the miR-34a-5p-Notch1 pathway on BMMs to reduce their differentiation in osteoclasts. The identification of IL-33 as an intermediator of the inhibitory effect of the osteoblast HIF-1α pathway on osteoclastogenesis and the discovery of its induction and action pathways, provides a previous unknown mechanism for understanding HIF-1α pathway in bone remodeling.
As oxygen-sensitive cells, osteoblasts are exposed to mild hypoxic conditions during bone development. Therefore, it is meaningful to investigate how osteoblasts regulate the maturation of osteoclasts under hypoxia. HIFs are the main regulators of adaptive response to changes in oxygen tension (pO2) (24). Besides promoting osteogenesis by upregulation of VEGF, transforming growth factor β1 (TGF-β1) and insulin-like growth factor II, osteoblasts are also reported to enhance bone resorption by increasing osteoclast activity in response to hypoxia (25). Our previous study showed that activation of HIF-1α in mature osteoblasts through Vhl-CKO profoundly increases angiogenesis and osteogenesis, whereas mice with Hif-1α CKO in osteoblast had decreased bone volume and vascularity (10). Except of directly promoting bone development by increasing angiogenesis or osteoblastic activity (11), our results also suggested that activating the hypoxia/HIF-1α pathway might disturb osteoblast-osteoclast coupling to dampen osteoclast differentiation by OPG (12). Whether HIF-1α pathway activation triggers the production of other factors that are involved in vascular regeneration as well as osteoclast differentiation is still unknown. Here, we found that HIF-1 pathway activation in osteoblasts could also mediate osteoclastogenesis via IL-33.
Interleukin-33, also named IL-1F11, is reported to be expressed by endothelial and epithelial cells after stress and is released from cells to initiate inflammatory responses (26). IL-33 induces signal transduction by binding to a heterodimeric receptor complex consisting of interleukin-1 receptor-like 1 (ST2) and IL-1 receptor accessory protein. ST2 is mainly expressed on mast cells, Th2 cells, keratinocytes, and macrophages (27–30). In addition to functioning as a proinflammatory cytokine in the pathogenesis of asthma, atopic dermatitis, and allergic shock, the diseases characterized by Th2 inflammatory responses (26, 27, 31, 32), IL-33 was also found to be essential for the initiation of Rheumatoid arthritis, which is an autoimmune disease characterized by Th1/Th17 responses (33, 34). Recently, it was reported that IL-33 was upregulated during osteoblast differentiation and worked as a suppressor of osteoclast formation (7, 8). However, whether IL-33 is involved in osteoblast HIF-1α pathway mediated osteoclast differentiation is less known. Here, we found IL-33 is increased in Vhl-silenced osteoblasts in vivo and in vitro, and osteoblast HIF-1α pathway activation inhibited osteoclastogenesis partly through IL-33, as an IL-33 blocking antibody strongly reduced the inhibitory effect of CM-CRE on osteoclastogenesis in BMMs, including osteoclast formation, TRAP activity, and bone resorption activity. Mechanistically, Vhl deficiency leads to HIF-1α accumulation and upregulated IL-33 expression by binding to the −1,504/−1,500 bp site on the promoter of Il-33.
As a emerging group of small (~20 nucleotides), non-coding, single-stranded RNA molecules, miRNAs are reported to negatively regulate their target genes by inducing mRNA degradation or through inhibition of translation (35). Recently, miRNAs have emerged as important regulatory elements in bone metabolism. Besides our previous report that miR-34a-5p was involved in dexamethasone-damped BMSCs proliferation and osteogenic differentiation (21), miR-148a was reported to regulate osteoclast formation by targeting V-maf musculoaponeurotic fibrosarcoma oncogene homolog B (36). miR-503 regulates osteoclastogenesis by targeting RANK (22). However, whether miRNAs take part in IL-33-mediated osteoclastogenesis was unknown. Here, we found that IL-33 upregulated miR-34a-5p expression in a dose-dependent manner, and this increase in miR-34a-5p was essential for IL-33 and CM-CRE inhibited osteoclastogenesis as miR-34a-5p antagomir drastically reduced the inhibitory effect of IL-33 and CM-CRE on osteoclast differentiation, TRAP activity and osteoclastic bone resorption activity. Simultaneously, our data also revealed that miR-34a-5p antagomir did not completely reverse the inhibitory effects of IL-33 on osteoclast formation. Besides miR-34a-5p, we found miR-125a-5p and miR-23a-3p were also significantly upregulated in response to IL-33 stimulation, thereby, whether miR-125a-5p, miR-23a-3p and/or other miRNAs were involved in IL-33 restrained-osteoclastogenesis still need for further investigation.
It has also been reported that RANKL stimulation triggers the activation of Notch1, which is essential for bone marrow precursors to differentiate into osteoclasts (23). In the present study, we found that Notch1 accumulation induced by RANKL was significantly reduced by IL-33, and activation of Notch1 by JAG1 significantly inhibited IL-33-increased miR-34a-5p expression, as well as abundantly restored the osteoclast formation inhibited by IL-33. Therefore, our study identified a previously unknown mechanism: IL-33-miR-34a-5p pathway inhibited osteoclastogenesis via Notch1. However, as the results in Figure 8 revealed that Jag-1 stimulation did not completely rescue the dampened osteoclast formation induced by IL-33, which predicts that IL-33-miR-34a-5p inhibition of osteoclastogenesis could also act through an alternative pathway.
However, we would like to point out some potential limitations of our study. First, although our results revealed that IL-33 mediated osteoclastogenesis via the miR-34a-5p-Notch1 pathway, this conclusion is based on observations of mouse BMMs. Whether the same mechanisms are shared in human BMMs is unknown. Furthermore, the exact functional mechanisms of IL-33 on osteoclastogenesis in vivo require further investigation. Finally, the hypothesis that osteoblast HIF-1α pathway regulates osteoclastogenesis via IL-33-miR-34a-5p-Notch1 still needs to be confirmed in animal models of osteoporosis, and the clinical relevance of this pathway in osteoporosis or osteopetrosis remains to be elucidated.
In conclusion, our study uncovers that IL-33 is an intermediate factor in the cross-talk between osteoblasts HIF-1α pathway activation and osteoclasts formation. The identification of the IL-33-miR-34a-5p-Notch1 pathway provides new molecular mechanisms, which contribute to anti-osteoclastogenesis and may ultimately lead to the development of novel treatments for bone metabolism diseases.
Ethics Statement
All animal experiments were performed in accordance with the protocol approved by the Shanghai Jiao Tong University (SJTU) Animal Care and Use Committee and in accordance with the Ministry of Science and Technology of the People’s Republic of China Animal Care guidelines.
Author Contributions
Conceived and designed the experiments: CL, LD, and HK; performed the experiments: HK, KY, JQ, LG, YY, CG, and FW. Analyzed the data: CL and HK; contributed reagents/materials/analysis tools: LD, CL, LX, and BR. Wrote the paper: CL.
Conflict of Interest Statement
The authors declare that the research was conducted in the absence of any commercial or financial relationships that could be construed as a potential conflict of interest.
Acknowledgments
We are grateful to Prof. Thomas L. Clemens from Johns Hopkins University School of Medicine for kindly provided Vhlflox/flox and OC-Cre transgenic mice. This work was supported by Shanghai Sailing program (No. 16YF1410100), National Natural Science Foundation of China grants (No. 81371958, 81572099) and Research project of Shanghai municipal health and Family Planning Commission (No. 20164Y0164).
Funding
This work was supported by Shanghai Sailing program (No. 16YF1410100), National Natural Science Foundation of China grants (No. 81371958, 81572099), and Research project of Shanghai municipal health and Family Planning Commission (No. 20164Y0164).
Supplementary Material
The Supplementary Material for this article can be found online at http://www.frontiersin.org/article/10.3389/fimmu.2017.01312/full#Supplementary-Material.
Abbreviations
HIF-1α, hypoxia inducible factor-1α; IL-33, interleukin-33; OPG, osteoprotegerin; BMMs, bone marrow-derived monocytes; RANKL, receptor activator of nuclear factor kappa-B ligand; VHL, von Hippel–Lindau tumor suppressor protein; PHDs, prolyl hydroxylase-domain proteins; VEGF, vascular endothelial growth factor; Ad-CRE, adenovirus expressing Cre recombinase; Ad-GFP, adenovirus expressing green fluoresence protein; TRAP, tartrate-resistant acid phosphatase; CM-GFP, culture medium of osteoblasts infected with Ad-GFP; CM-CRE, culture medium of osteoblasts infected with Ad-CRE; Vhl-CKO, Vhl-conditional knockout mice, Cre-recombinase positive mice; Vhl-CON, control mice, Cre-recombinase negative mice; DFO, Desferrioxamine; Jag1, Jagged1; Hes1, hairy and enhancer of split-1; N.Oc/BS, osteoclast numbers per millimeter of trabecular bone surface.
References
1. Li D, Liu J, Guo B, Liang C, Dang L, Lu C, et al. Osteoclast-derived exosomal miR-214-3p inhibits osteoblastic bone formation. Nat Commun (2016) 7:10872. doi:10.1038/ncomms10872
2. Phan TC, Xu J, Zheng MH. Interaction between osteoblast and osteoclast: impact in bone disease. Histol Histopathol (2004) 19(4):1325–44. doi:10.14670/HH-19.1325
3. Kalbasi Anaraki P, Patecki M, Tkachuk S, Kiyan Y, Haller H, Dumler I. Urokinase receptor mediates osteoclastogenesis via M-CSF release from osteoblasts and the c-Fms/PI3K/Akt/NF-kappaB pathway in osteoclasts. J Bone Miner Res (2015) 30(2):379–88. doi:10.1002/jbmr.2350
4. Kassem A, Lindholm C, Lerner UH. Toll-like receptor 2 stimulation of osteoblasts mediates Staphylococcus aureus induced bone resorption and osteoclastogenesis through enhanced RANKL. PLoS One (2016) 11(6):e0156708. doi:10.1371/journal.pone.0156708
5. Schett G, Gravallese E. Bone erosion in rheumatoid arthritis: mechanisms, diagnosis and treatment. Nat Rev Rheumatol (2012) 8(11):656–64. doi:10.1038/nrrheum.2012.153
6. Souza PP, Lerner UH. The role of cytokines in inflammatory bone loss. Immunol Invest (2013) 42(7):555–622. doi:10.3109/08820139.2013.822766
7. Saleh H, Eeles D, Hodge JM, Nicholson GC, Gu R, Pompolo S, et al. Interleukin-33, a target of parathyroid hormone and oncostatin M, increases osteoblastic matrix mineral deposition and inhibits osteoclast formation in vitro. Endocrinology (2011) 152(5):1911–22. doi:10.1210/en.2010-1268
8. Schulze J, Bickert T, Beil FT, Zaiss MM, Albers J, Wintges K, et al. Interleukin-33 is expressed in differentiated osteoblasts and blocks osteoclast formation from bone marrow precursor cells. J Bone Miner Res (2011) 26(4):704–17. doi:10.1002/jbmr.269
9. Towler DA. Vascular biology and bone formation: hints from HIF. J Clin Invest (2007) 117(6):1477–80. doi:10.1172/JCI32518
10. Wang Y, Wan C, Deng L, Liu X, Cao X, Gilbert SR, et al. The hypoxia-inducible factor alpha pathway couples angiogenesis to osteogenesis during skeletal development. J Clin Invest (2007) 117(6):1616–26. doi:10.1172/JCI31581
11. Zhang LF, Qi J, Zuo G, Jia P, Shen X, Shao J, et al. Osteoblast-secreted factors promote proliferation and osteogenic differentiation of bone marrow stromal cells via VEGF/heme-oxygenase-1 pathway. PLoS One (2014) 9(6):e99946. doi:10.1371/journal.pone.0099946
12. Shao J, Zhang Y, Yang T, Qi J, Zhang L, Deng L. HIF-1alpha disturbs osteoblasts and osteoclasts coupling in bone remodeling by up-regulating OPG expression. In Vitro Cell Dev Biol Anim (2015) 51(8):808–14. doi:10.1007/s11626-015-9895-x
13. Wu C, Rankin EB, Castellini L, Alcudia JF, LaGory EL, Andersen R, et al. Oxygen-sensing PHDs regulate bone homeostasis through the modulation of osteoprotegerin. Genes Dev (2015) 29(8):817–31. doi:10.1101/gad.255000.114
14. Albers J, Keller J, Baranowsky A, Beil FT, Catala-Lehnen P, Schulze J, et al. Canonical Wnt signaling inhibits osteoclastogenesis independent of osteoprotegerin. J Cell Biol (2013) 200(4):537–49. doi:10.1083/jcb.201207142
15. Kim JH, Youn BU, Kim K, Moon JB, Lee J, Nam KI, et al. Lhx2 regulates bone remodeling in mice by modulating RANKL signaling in osteoclasts. Cell Death Differ (2014) 21(10):1613–21. doi:10.1038/cdd.2014.71
16. Yuan X, Cao J, Liu T, Li YP, Scannapieco F, He X, et al. Regulators of G protein signaling 12 promotes osteoclastogenesis in bone remodeling and pathological bone loss. Cell Death Differ (2015) 22(12):2046–57. doi:10.1038/cdd.2015.45
17. Wilson SR, Peters C, Saftig P, Bromme D. Cathepsin K activity-dependent regulation of osteoclast actin ring formation and bone resorption. J Biol Chem (2009) 284(4):2584–92. doi:10.1074/jbc.M805280200
18. Tran CM, Fujita N, Huang BL, Ong JR, Lyons KM, Shapiro IM, et al. Hypoxia-inducible factor (HIF)-1alpha and CCN2 form a regulatory circuit in hypoxic nucleus pulposus cells: CCN2 suppresses HIF-1alpha level and transcriptional activity. J Biol Chem (2013) 288(18):12654–66. doi:10.1074/jbc.M112.448860
19. Wan C, Gilbert SR, Wang Y, Cao X, Shen X, Ramaswamy G, et al. Activation of the hypoxia-inducible factor-1alpha pathway accelerates bone regeneration. Proc Natl Acad Sci U S A (2008) 105(2):686–91. doi:10.1073/pnas.0708474105
20. Chen L, Holmstrom K, Qiu W, Ditzel N, Shi K, Hokland L, et al. MicroRNA-34a inhibits osteoblast differentiation and in vivo bone formation of human stromal stem cells. Stem Cells (2014) 32(4):902–12. doi:10.1002/stem.1615
21. Kang H, Chen H, Huang P, Qi J, Qian N, Deng L, et al. Glucocorticoids impair bone formation of bone marrow stromal stem cells by reciprocally regulating microRNA-34a-5p. Osteoporos Int (2016) 27(4):1493–505. doi:10.1007/s00198-015-3381-x
22. Chen C, Cheng P, Xie H, Zhou HD, Wu XP, Liao EY, et al. miR-503 regulates osteoclastogenesis via targeting RANK. J Bone Miner Res (2014) 29(2):338–47. doi:10.1002/jbmr.2032
23. Choi YH, Ann EJ, Yoon JH, Mo JS, Kim MY, Park HS. Calcium/calmodulin-dependent protein kinase IV (CaMKIV) enhances osteoclast differentiation via the up-regulation of Notch1 protein stability. Biochim Biophys Acta (2013) 1833(1):69–79. doi:10.1016/j.bbamcr.2012.10.018
24. Lee SB, Frattini V, Bansal M, Castano AM, Sherman D, Hutchinson K, et al. An ID2-dependent mechanism for VHL inactivation in cancer. Nature (2016) 529(7585):172–7. doi:10.1038/nature16475
25. Arnett TR. Acidosis, hypoxia and bone. Arch Biochem Biophys (2010) 503(1):103–9. doi:10.1016/j.abb.2010.07.021
26. Liew FY, Pitman NI, McInnes IB. Disease-associated functions of IL-33: the new kid in the IL-1 family. Nat Rev Immunol (2010) 10(2):103–10. doi:10.1038/nri2692
27. Allakhverdi Z, Smith DE, Comeau MR, Delespesse G. Cutting edge: the ST2 ligand IL-33 potently activates and drives maturation of human mast cells. J Immunol (2007) 179(4):2051–4. doi:10.4049/jimmunol.179.4.2051
28. Kalambokis G, Stefanou D, Arkoumani E, Kitsanou M, Bourantas K, Tsianos EV. Fulminant bronchiolitis obliterans organizing pneumonia following 2 d of treatment with hydroxyurea, interferon-alpha and oral cytarabine ocfosfate for chronic myelogenous leukemia. Eur J Haematol (2004) 73(1):67–70. doi:10.1111/j.1600-0609.2004.00252.x
29. Joshi AD, Oak SR, Hartigan AJ, Finn WG, Kunkel SL, Duffy KE, et al. Interleukin-33 contributes to both M1 and M2 chemokine marker expression in human macrophages. BMC Immunol (2010) 11:52. doi:10.1186/1471-2172-11-52
30. Li C, Li H, Jiang Z, Zhang T, Wang Y, Li Z, et al. Interleukin-33 increases antibacterial defense by activation of inducible nitric oxide synthase in skin. PLoS Pathog (2014) 10(2):e1003918. doi:10.1371/journal.ppat.1003918
31. Ali S, Huber M, Kollewe C, Bischoff SC, Falk W, Martin MU. IL-1 receptor accessory protein is essential for IL-33-induced activation of T lymphocytes and mast cells. Proc Natl Acad Sci U S A (2007) 104(47):18660–5. doi:10.1073/pnas.0705939104
32. Li C, Maillet I, Mackowiak C, Viala C, Di Padova F, Li M, et al. Experimental atopic dermatitis depends on IL-33R signaling via MyD88 in dendritic cells. Cell Death Dis (2017) 8(4):e2735. doi:10.1038/cddis.2017.90
33. Carriere V, Roussel L, Ortega N, Lacorre DA, Americh L, Aguilar L, et al. IL-33, the IL-1-like cytokine ligand for ST2 receptor, is a chromatin-associated nuclear factor in vivo. Proc Natl Acad Sci U S A (2007) 104(1):282–7. doi:10.1073/pnas.0606854104
34. Palmer G, Talabot-Ayer D, Lamacchia C, Toy D, Seemayer CA, Viatte S, et al. Inhibition of interleukin-33 signaling attenuates the severity of experimental arthritis. Arthritis Rheum (2009) 60(3):738–49. doi:10.1002/art.24305
35. Ambros V. The functions of animal microRNAs. Nature (2004) 431(7006):350–5. doi:10.1038/nature02871
Keywords: hypoxia-inducible factor-1α, interleukin-33, osteoblast, osteoclastogenesis, microRNA-34a-5p, Notch1
Citation: Kang H, Yang K, Xiao L, Guo L, Guo C, Yan Y, Qi J, Wang F, Ryffel B, Li C and Deng L (2017) Osteoblast Hypoxia-Inducible Factor-1α Pathway Activation Restrains Osteoclastogenesis via the Interleukin-33-MicroRNA-34a-Notch1 Pathway. Front. Immunol. 8:1312. doi: 10.3389/fimmu.2017.01312
Received: 18 June 2017; Accepted: 28 September 2017;
Published: 16 October 2017
Edited by:
Ji Ming Wang, National Cancer Institute at Frederick, United StatesReviewed by:
Toshikazu Kondo, Wakayama Medical University, JapanMassimiliano Mazzone, Flanders Institute for Biotechnology, Belgium
Copyright: © 2017 Kang, Yang, Xiao, Guo, Guo, Yan, Qi, Wang, Ryffel, Li and Deng. This is an open-access article distributed under the terms of the Creative Commons Attribution License (CC BY). The use, distribution or reproduction in other forums is permitted, provided the original author(s) or licensor are credited and that the original publication in this journal is cited, in accordance with accepted academic practice. No use, distribution or reproduction is permitted which does not comply with these terms.
*Correspondence: Changwei Li, Y2hhbmd3ZWkzOTMzMzFAMTYzLmNvbQ==;
Lianfu Deng, bGZfZGVuZ0AxMjYuY29t
†These authors have contributed equally to this work.