- 1Animal Microecology Institute, College of Veterinary Medicine, Sichuan Agricultural University, Chengdu, China
- 2Ya’an Agricultural Science and Technology Development Co., Ltd., Ya’an, China
Along with banning of antibiotics, necrotic enteritis (NE), especially subclinical NE (SNE) whereby no clinical signs are present in chicks, has become one of the most threatening problems in poultry industry. Therefore, increasing attention has been focused on research and application of effective probiotic strains, as an alternative to antibiotics, to prevent SNE in broilers. In the present study, we evaluated the effects of Lactobacillus johnsonii BS15 on the prevention of SNE in broilers. Specifically, assessment determined the growth performance and indexes related to intestinal mucosal immunity in the ileum and cecal tonsil of broilers. A total of 300 1-day-old Cobb 500 chicks were randomly distributed into the following 5 groups: control group (fed with basal diet + de Man, Rogosa, and Sharpe liquid medium [normal diet]), SNE group (normal diet), BS15 group (basal diet + 1 × 106 colony-forming units BS15/g as fed [BS15 diet]), treatment group (normal diet [days 1–28] + BS15 diet [days 29–42]), and prevention group (BS15 diet [days 1–28] + normal diet [days 29–42]) throughout a 42-day experimental period. SNE infection was treated for all chicks in the SNE, BS15, treatment, and prevention groups. The present results demonstrated that BS15 supplementation of feeds in BS15 and prevention groups exerted a positive effect on preventing negative influences on growth performance; these negative influences included low body weight gain and increased feed conversion ratio caused by SNE. Although no changes were detected in all determined indexes in cecal tonsils, BS15-treated broilers were free from SNE-caused damage in villi in the ileum. BS15 inhibited SNE-caused decrease in immunoglobulins in the ileum. In the lamina propria of ileum, T cell subsets of lymphocytes influenced by SNE were also controlled by BS15. BS15 affected antioxidant abilities of the ileum and controlled SNE-induced mitochondrion-mediated apoptosis by positively changing contents and/or mRNA expression levels of apoptosis-related proteins. These findings indicate that BS15 supplementation may prevent SNE-affected growth decline mainly through enhancing intestinal immunity in broilers.
Introduction
Necrotic enteritis (NE), a poultry disease that is mainly caused by Clostridium perfringens (CP), was first reported in 1961 and remains important in the poultry industry to date (1). Over the recent decades, NE has been prevented and treated by supplementation of antibiotics in feed (2). However, along with banning of antibiotics, NE has become one of the most threatening problems in the poultry industry. Subclinical NE (SNE) especially presents poor performance without mortality. As chicks show no clinical sign of the disease, most economic losses result from the presence of subclinical forms (3, 4). Aggravating the condition, meat containing affected nutrients or toxins may be purchased and consumed by customers (5). Therefore, an effective alternative to antibiotics must be developed for the prevention of SNE in broilers. Aside from the application of polysaccharides (6) and vaccines (7), probiotics is becoming a common method for prevention of SNE in postantibiotic era (8, 9). However, difficulty arises from determining the mechanism by which probiotics affect the gastrointestinal tract because probiotic strains exert their beneficial effects via different mechanisms in which other microbiota may be involved (10, 11). Kang et al. (12) reported that although live probiotics or their metabolites were found to interact with diverse immune cells and thus play a role as immune modulators, the effects of probiotics in prevention or modulation of diseases diversify even within the same species. In addition, Jacobsen et al. (13) previously demonstrated the difficulty of reliably extrapolating in vitro influences of probiotics to in vivo conditions. Therefore, although numerous studies have focused on the positive effects of probiotics in animals, the mechanisms by which probiotics can successfully exert beneficial effects on promoting growth performance and preventing diseases remain unclear (14).
Lactobacillus johnsonii BS15 (CCTCC M2013663), a probiotic strain which was proven to prevent non-alcoholic fatty liver disease in obese mice, was isolated from homemade yogurt collected from Hongyuan Prairie, Aba Autonomous Prefecture, China (15). In our recent previous studies, we applied BS15 to broilers and observed that BS15 supplementation can improve growth performance and significantly enhance the nutritional value of meat (16). Only live BS15 (not disrupted cells) may exert health benefits mainly by improving lipid metabolism, intestinal development, and gut microflora in the small intestine at the starter phase (17). In our preliminary study on the prevention of SNE, BS15 controlled growth performance, lipid deposits, and fatty acid composition of chicken meat during subclinical CP infection (5). Thus, on the basis of all our recent studies, we evaluated the effect of dietary supplementation of BS15 on prevention of SNE in broilers. Aiming to determine the relationship between preventive effects of BS15 and intestinal immunity, the present study assessed growth performance and indexes related to intestinal immunity in the ileum and cecal tonsil of broilers.
Materials and Methods
Feed Preparation
Viable counts of BS15 cell preparations were evaluated by heterotrophic plate counts after maintaining cultures in de Man, Rogosa, and Sharpe (MRS) broth at 37°C for 36 h under an anaerobic environment. Supplementation was performed before each feeding. The basic procedure was as follows: approximately 10 mL of BS15 solution/MRS liquid medium [diluted with the same amount of phosphate-buffered saline (PBS)] was thoroughly mixed with 1,000 g of diet (17).
Animals and Treatment
A total of 300 1-day-old male chicks (Cobb 500) with similar body weights were purchased from Chia Tai broiler hatchery (Chengdu, China). Chicks were weighed and divided into five treatment groups. Each group consisted of 6 replicates with 10 birds per replicate at the Key Laboratory of Animal Disease and Human Health of Sichuan Province, Sichuan Agricultural University. Birds were fed ad libitum and provided with free access to water throughout the entire experiment. Artificial light was provided all day using fluorescent light. Room temperature was maintained at 33°C for the first 3 days and then gradually reduced by 3°C weekly until a temperature of 24°C was reached; the final temperature was maintained until the end of experiment. Table 1 shows starter and finisher diet formulas. All diets were formulated to satisfy or exceed the National Research Council requirements for broilers (18). Five bird groups were fed with diets in mash form as follows: control group (basal diet + MRS liquid medium [normal diet]), SNE group (normal diet), BS15 group (basal diet + 1 × 106 colony-forming units [cfu]; BS15/g as fed [BS15 diet]), treatment group (normal diet [days 1–28] + BS15 diet [days 29–42]), and prevention group (BS15 diet [days 1–28] + normal diet [days 29–42]). Each feed amount was consumed by chicks within 3 h and resupplied every 3 h. All chicks in SNE, BS15, treatment, and prevention groups were gavaged orally with 20,000 Eimeria acervulina oocysts and 5,000 Eimeria maxima oocysts per bird at 15 days of age and then with 1 mL of CP (2.2 × 108 cfu/mL) from days 18–22 for SNE induction. The control group was gavaged with 1 mL of PBS at 15 days of age and days 18–22 (4, 19). Figure 1 summarizes treatments of each group. Chicks were weighed, and feed intake was recorded in the morning at days 7, 14, 21, 28, 35, and 42. Average daily gain (ADG), average daily feed intake (ADFI), and feed conversion ratio (FCR) were also calculated. All animal experiments were performed in accordance with the guidelines for the care and use of laboratory animals and approved by the Institutional Animal Care and Use Committee of Sichuan Agricultural University (approval number: SYXKchuan2014-187).
Sampling
On the morning of day 28, six birds (one bird per cage) from the control, SNE, and BS15 groups were randomly selected and sacrificed by exsanguination under anesthesia in accordance with institutional animal care guidelines. Subsequently, samples from the ileum and cecal tonsil were removed and washed with ice-cold sterilized saline. These samples were then frozen in liquid nitrogen and stored at −80°C for subsequent analysis of antioxidant abilities, enzyme activities, and/or gene expression. Total ileal RNA was extracted using RNAiso Plus (TaKaRa, Dalian, China) in accordance with manufacturer’s guidelines. Quality and quantity of isolated RNA were assessed from the ratio of absorbance values at 260 and 280 nm and by agarose gel electrophoresis. First-strand complementary DNA (cDNA) was synthesized from 1 µg of total RNA using PrimeScriptTM RT reagent kit with gDNA Eraser (TaKaRa, Dalian, China). All cDNA products were frozen at −20°C until further use.
Detection by Flow Cytometry Method (FCM)
Another six birds from the same three groups were selected and sacrificed. Ileum and cecal tonsil were collected from each bird. After isolating intraepithelial lymphocytes (IELs) and lamina propria lymphocytes (LPLs) from the ileum, IELs and LPLs from ileum and cecal tonsil were subjected to FCM to determine CD3+, CD3+CD4+, and CD3+CD8+ T cell percentages as described by Wu et al. (20). The methods described by Todd et al. (21) and Reséndiz-Albor et al. (22) were performed to isolate IELs and LPLs of ileums, respectively.
Histological Observation and Immunohistochemistry
Another six birds from the control, SNE, and BS15 groups were selected and sacrificed by the previously mentioned method. Samples of ileum sections (1 cm2) and cecal tonsils were collected and fixed in 10% neutral-buffered formalin, processed and trimmed, and embedded in paraffin. Sections with 5-µm thickness were stained with hematoxylin and eosin and observed by light microscopy. A light microscope (Olympus, Japan) was used to observe morphological changes. Immunoglobulin (Ig) A+ B cells were localized in the ileum, as observed by immunohistochemistry. Immunohistochemical staining and counting were performed as described by Liu et al. (23). Staining was performed in three independent replicates to confirm results. Tissue sections were dewaxed in xylene, rehydrated through a graded series of ethanol, washed in distilled water and PBS, and then blocked for endogenous peroxidase by incubation with 3% H2O2 in methanol for 15 min. Sections were subjected to antigen retrieval by microwaving in 0.01 M sodium citrate buffer (pH 6.0). Additional washing in PBS was performed before incubation in 10% normal goat serum at 37°C for 30 min. Tissue sections were incubated with diluted (1:100) primary antibodies at 4°C overnight. Antibodies used included polyclonal mouse anti-chicken IgA heavy chains (SouthernBiotech 8330-01, Birmingham, AL, USA). For negative controls, tissue sections received PBS in place of the primary antibody. After washing in PBS, sections were exposed to 1% biotinylated secondary antibody goat anti-mouse IgG (ZSGB-BIO SP Kit, ZSGB-BIO, Beijing, China) at 37°C for 1 h. Slices were then incubated with horseradish peroxidase-streptavidin (ZSGB-BIO SP Kit) for 30 min at 37°C. To visualize immunoreaction, tissue sections were immersed in diaminobenzidine hydrochloride. Reactions were monitored microscopically and stopped by immersion in distilled water as soon as a brown staining has been visualized. Slices were lightly counterstained with hematoxylin, dehydrated in ethanol, cleared in xylene, and mounted on glass slides. IgA+ B cells were counted using a computer-supported imaging system connected to a light microscope (Olympus AX70, Tokyo, Japan) and then quantified using Image-Pro Plus 5.1 (Silver Spring, MD, USA) image analysis software. Six sections were measured in each group, with each section measured five times and averaged.
Immunoglobulins and Antioxidant Indexes
Levels of Igs, including IgG, IgM, IgA, and secretory IgA (sIgA), in the ileum and cecal tonsil were quantified using chicken-specific enzyme-linked immunosorbent assay (ELISA) kits (RD Ltd., USA) following manufacturer’s instructions. Antioxidant indexes in ileum were measured using commercial kits by Nanjing Jiancheng Bioengineering Institute (Nanjing, Jiangsu, China); these indexes included total antioxidation capacity (T-AOC), activities of catalase (CAT), superoxide dismutase (SOD), glutathione peroxidase (GSH-Px), inhibition of hydroxy radical (IHR), and malondialdehyde (MDA) and GSH contents.
Apoptosis-Related Proteins, Cytokines, Matrix Metalloproteinase-2 (MMP-2), and Nrf-2
Levels of apoptosis-related proteins, including Bcl-2, Bax, and caspase-3, and cytokines, including interleukin (IL)-2, IL-6, IL-8, IL-10, IL-17, tumor necrosis factor-alpha (TNF-α), and interferon-gamma (IFN-γ), in the ileum were quantified using ELISA kits specific for chicken (RD Ltd., USA). mRNA expression levels were determined according to our recent study (17). By using the prepared cDNA products from the ileum and cecal tonsil, polymerase chain reaction (PCR) was performed using a CFX96 Real-Time PCR Detection System (Bio-Rad, Hercules, CA, USA) with SYBR Premix Ex Taq™ PCR kit (TaKaRa, Dalian, China). Thermocycling protocol was as follows: 5 min at 95°C, followed by 40 cycles of 15 s denaturation at 95°C, and 30 s annealing/extension at optimum temperature (Table 2). A final melting curve analysis was used to monitor purity of PCR products. Table 2 presents primer sequences for targeted genes. Standard curves were obtained from serial dilution of samples. ΔΔCt method was used to estimate mRNA abundance. Ct is calculated as (Cttarget − CtGAPDH)treatment − (Cttarget − CtGAPDH)control, where glyceraldehyde 3-phosphate dehydrogenase is the eukaryotic housekeeping gene used to normalize relative gene expression levels. All samples (n = 6) in each group were analyzed in triplicate. Mean values of measurements were used to calculate mRNA expression levels of Bcl-2, Bax, caspase-3, cytochrome c (Cytc c), apoptotic protease activating factor 1 (Apaf-1), caspase-9, IL-2, IL-6, IL-8, IL-10, IL-17, TNF-α, IFN-γ, MMP-2, and Nrf-2 in the ileum.
Data Analysis
All results were expressed as mean ± SD and analyzed by one-way ANOVA. Duncan’s multiple range test was used for multiple comparison when a significant interaction was detected. All statistical analyses were conducted using SigmaPlot for Social Sciences version 12. Differences at P < 0.05 were considered statistically significant. Data on growth performance were obtained on a cage basis (n = 6), whereas other information were based on individual broilers (six replicates of one chick per cage).
Results
Growth Performance
Table 3 and Figure 2 show growth performance results. At day 21, the SNE and treatment groups presented lower ADG and body weight than the other three groups (P < 0.05). Higher body weights were observed in the BS15 and prevention groups than in the SNE and treatment groups, respectively, at days 28, 35, and 42 (P < 0.05). High ADG and FCR were also observed in BS15 and prevention groups at days 22–42 (P < 0.05). As shown in Table 3, no significant differences were observed in days 1–21 and overall ADFI among all groups (P > 0.05), whereas a significantly lower ADFI was observed in the SNE group compared with the other four groups at days 22–42 (P < 0.05). At days 22–42, the control group showed significantly higher ADFI compared with the SNE and treatment groups (P < 0.05), whereas no significant differences were observed between BS15, treatment, and prevention groups (P > 0.05).
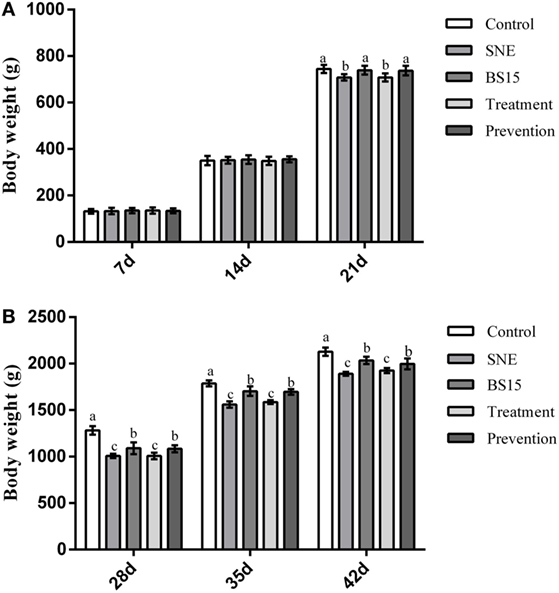
Figure 2. Body weight gain at 7, 14, 21, 28, 35, and 42 days. Bars with different letters significantly differ on the basis of Duncan’s multiple range tests (P < 0.05). Data are presented as means ± SD (n = 6). (A) At day 21, subclinical necrotic enteritis (SNE) and treatment groups showed lower body weight than the other three groups (P < 0.05). (B) Higher body weights were observed (P < 0.05) in the BS15 and prevention groups than in SNE and treatment groups, respectively, at 28, 35, and 42 days.
Histological Observation and Immunohistochemistry
As shown in Figure 3, histological examination showed intact villi structure in the control group, whereas those in the SNE group were destroyed and mainly presented the following features: shed epithelial cells, congested lamina propria, and increased number of lymphocytes. In the BS15 group, lamina propriae were lightly congested. No remarkable differences were observed in the cecal tonsil among the three groups. As shown in Figures 4 and 5, in the BS15 group, the number of IgA+ B cells in lamina propria of ileum was higher compared with that of the SNE group (P < 0.05) but lower than that of the control group (P < 0.05).
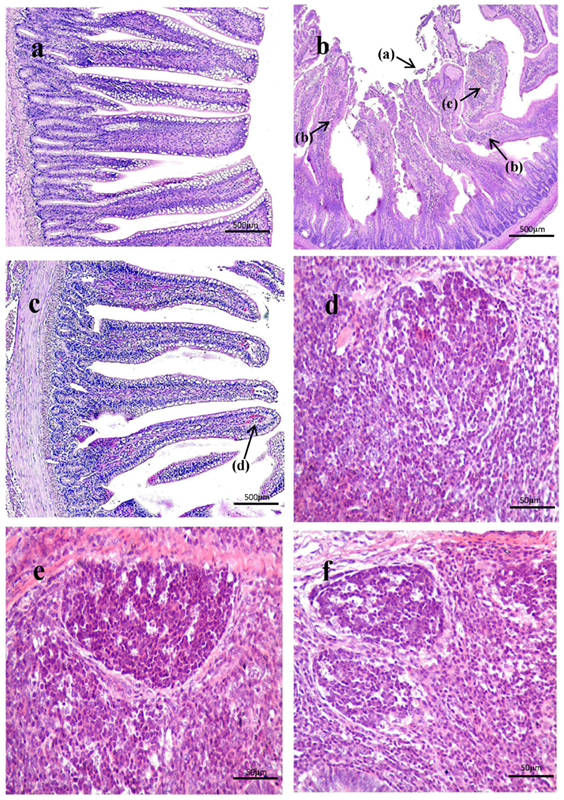
Figure 3. (A–C) Histological observation of ileum under original magnification (×40). (A) Ileum in the control group; (B) ileum in subclinical necrotic enteritis (SNE) group; (C) ileum in BS15 group; (D–F) histological observation of cecal tonsil under original magnification (×400); (D) cecal tonsil in the control group; (E) cecal tonsil in SNE group; (F) cecal tonsil in BS15 group. (1) Villi structure in the SNE group was destroyed and mainly presented the following features: shed epithelial cells (a), increased number of lymphocytes (b), and congested lamina propria (c). (2) In the BS15 group, lamina propriae were lightly congested (d). (3) No remarkable differences were observed in the cecal tonsil among the three groups.
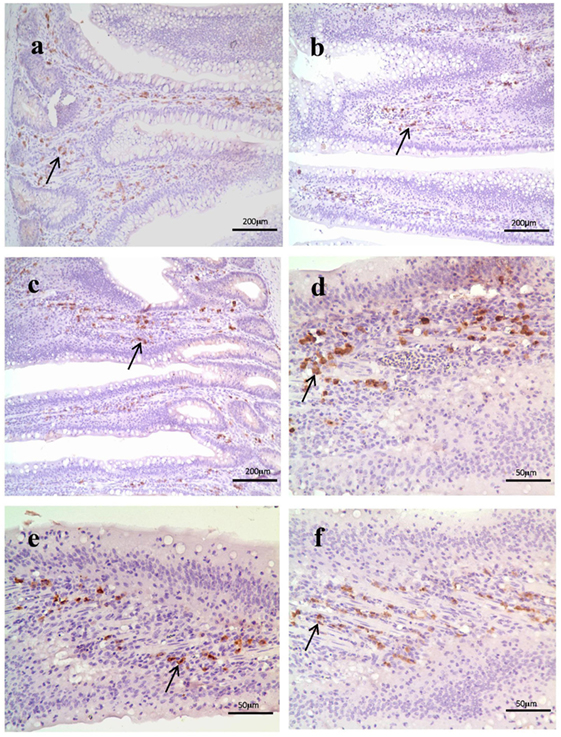
Figure 4. (A–C) IgA+ B cell numbers of the lamina propria in the ileum under original magnification (×100). (A) Ileum in the control group; (B) ileum in subclinical necrotic enteritis (SNE) group; (C) ileum in BS15 group; (D–F) IgA+ B cell numbers of the lamina propria in the ileum under original magnification (×400); (D) ileum in the control group; (E) ileum in SNE group; (F) ileum in BS15 group. In the BS15 group (C,F), the number of IgA+ B cells in lamina propria of ileum was higher compared with that of the SNE group (B,E) but lower than that of the control group (A,D).
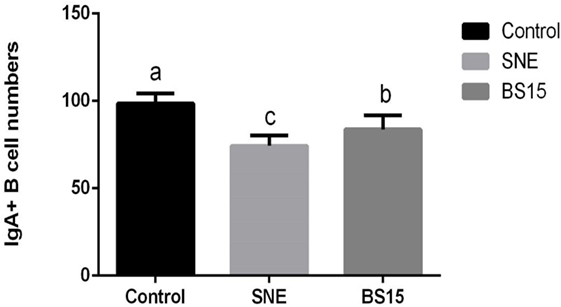
Figure 5. Changes in the numbers of IgA+ B cells in the lamina propria in the ileum. Bars with different letters significantly differ on the basis of Duncan’s multiple range tests (P < 0.05). Data are presented as mean ± SD (six replicates of one chick per cage). The number of IgA+ B cells in lamina propria of ileum in the BS15 group was higher compared with that of the subclinical necrotic enteritis (SNE) group (P < 0.05) but lower than that of the control group (P < 0.05).
Immunoglobulins
As shown in Figure 6, levels of IgG and IgA in ileum were significantly lower in SNE group than those in other groups (P < 0.05), but no significant difference was noted between BS15 and control groups (P > 0.05). In the BS15 group, sIgA level in the ileum was significantly lower than that of the control group (P < 0.05) and significantly higher than that in the SNE group (P < 0.05). No difference was detected in IgM levels in the ileum of the three experimental groups (P > 0.05), and no significant changes were observed in all determined Ig in the cecal tonsil (P > 0.05).
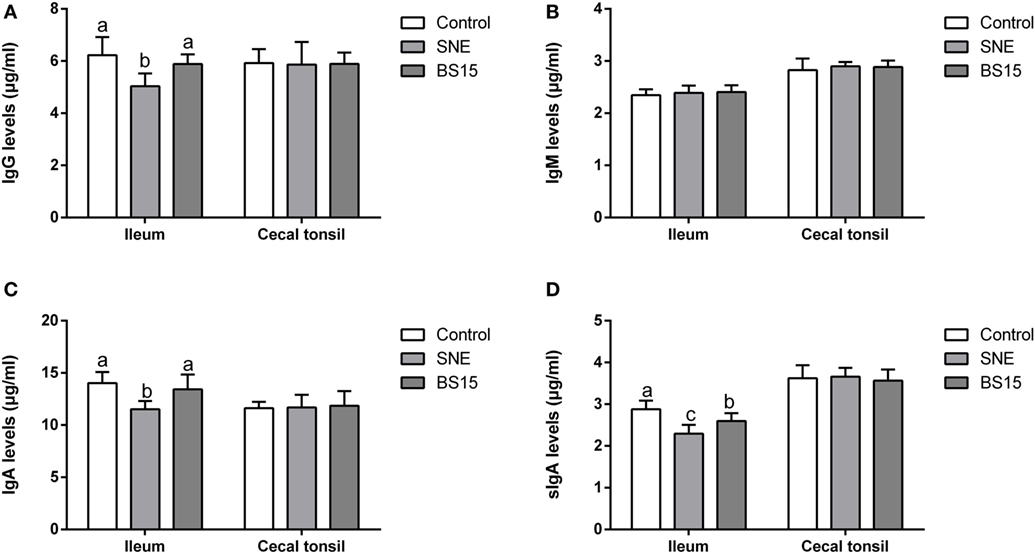
Figure 6. Changes in IgG, IgM, IgA, and sIgA levels in the ileum and cecal tonsil. Bars with different letters significantly differ on the basis of Duncan’s multiple range tests (P < 0.05). Data are presented as mean ± SD (six replicates of one chick per cage). (A,C) Levels of IgG and IgA in ileum were significantly lower in the subclinical necrotic enteritis (SNE) group than those in other groups (P < 0.05). (A–D) No significant difference was noted between BS15 and control groups (P > 0.05). (D) In the BS15 group, sIgA levels in the ileum were significantly lower than that of the control group (P < 0.05) and significantly higher than that of the SNE group (P < 0.05). (B) No difference was detected in IgM levels in the ileum among three experimental groups (P > 0.05). (A–D) No significant changes were observed on all determined Igs in the cecal tonsil (P > 0.05).
T Cell Subsets
Figure 7 displays results of T cell subsets as determined by FCM. No significant changes were observed in IELs of ileum and cecal tonsil among the three experimental groups (P > 0.05). In LPLs of ileum, percentages of CD3+, CD4+, and CD8+ T cells and CD4+/CD8+ ratio were significantly lower in BS15 group than those in the control group (P < 0.05), whereas percentages of CD4+ T cells and CD4+/CD8+ ratio in BS15 group were higher than those in the SNE group (P < 0.05). Except for CD4+ T cells, which were lower in number than those in the control group (P < 0.05), no significant difference was identified among the other three indexes (P > 0.05). All determined indexes related to T cell subsets showed no difference in cecal tonsil (P > 0.05).
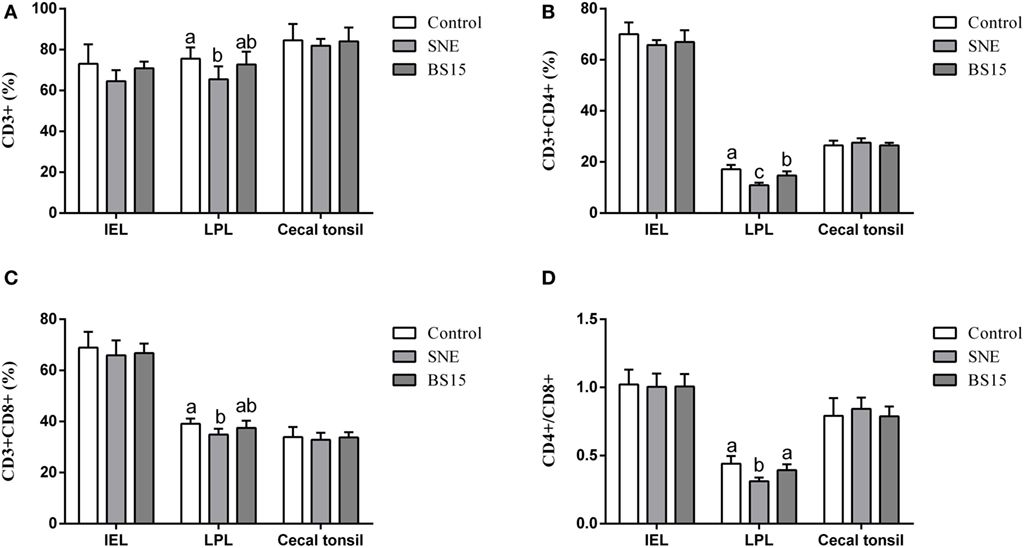
Figure 7. CD3+, CD4+, CD8+ T cells, and CD4+/CD8+ ratio in the ileum and cecal tonsil. Bars with different letters significantly differ on the basis of Duncan’s multiple range tests (P < 0.05). Data are presented as mean ± SD (six replicates of one chick per cage). (A–D) No significant changes were observed in intraepithelial lymphocytes (IELs) of ileum and cecal tonsil among the three experimental groups (P > 0.05). (A–D) In lamina propria lymphocytes (LPLs) of ileum, percentages of CD3+, CD4+, and CD8+ T cells and CD4+/CD8+ ratio were significantly lower in the BS15 group than those in the control group (P < 0.05). (B,D) Percentages of CD4+ T cells and CD4+/CD8+ ratio in BS15 group were higher than those in the SNE group (P < 0.05). (A–D) Except for CD4+ T cells, which were lower in number than those in the control group (P < 0.05), no significant difference was identified among the other three indexes (P > 0.05). (A–D) All determined indexes related to T cell subsets showed no difference in the cecal tonsil (P > 0.05).
Antioxidant Indexes
Figure 8 shows results on antioxidant indexes. As shown in Figures 8B,C, no changes were observed in all determined indexes in the cecal tonsil (P > 0.05). Results in Figure 8A show significantly lower T-AOC, CAT, SOD, and IHR activities in the ileum of the SNE group than those of the control group (P < 0.05). No significant differences (P > 0.05) in T-AOC and IHR in the ileum were observed between BS15 and control groups, but values for both were significantly higher than those in the SNE group (P < 0.05). No significant change was observed in GSH-Px (P > 0.05). As shown in Figure 8C, MDA levels in the ileum in BS15 group were lower than those in the SNE group (P < 0.05) and higher than those in the control group (P < 0.05). However, GSH level remained unchanged according to the present results (P > 0.05).
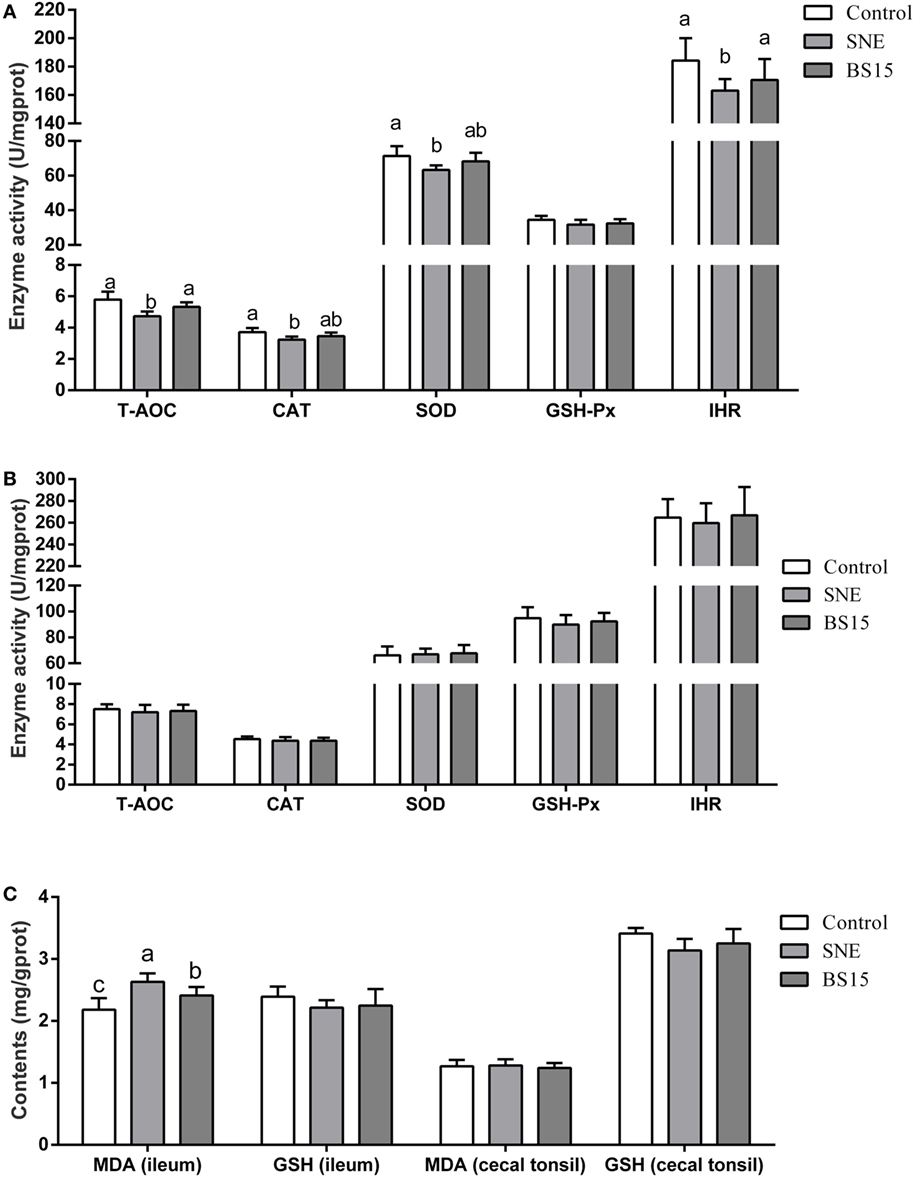
Figure 8. Antioxidant indexes in the ileum (A,C) and cecal tonsil (B,C). Bars with different letters significantly differ on the basis of Duncan’s multiple range tests (P < 0.05). Data are presented as mean ± SD (six replicates of one chick per cage). T-AOC, total antioxidation capacity; CAT, catalase; SOD, superoxide dismutase; GSH-Px, glutathione peroxidase; IHR, inhibiting hydroxy radical; MDA, malondialdehyde; GSH, glutathione; SNE, subclinical necrotic enteritis. (1) T-AOC, CAT, SOD, and IHR activities in the ileum were significantly lower in the SNE group than in the control group (P < 0.05). (2) No significant differences (P > 0.05) in T-AOC and IHR in the ileum were observed between BS15 and control groups, but values for both were significantly higher than those in the SNE group (P < 0.05). (3) No significant change was observed in GSH-Px (P > 0.05). (4) MDA levels in the ileum of the BS15 group were lower than those of the SNE group (P < 0.05) and higher than that of the control group (P < 0.05). (5) GSH level remained unchanged based on the present results (P > 0.05). (6) All determined indexes in cecal tonsil showed no change (P > 0.05).
Apoptosis-Related Proteins
Figure 9 presents results on apoptosis-related proteins in the ileum. Although no significant change in Bcl-2 protein content was detected (P > 0.05), mRNA expression levels of Bcl-2 in the SNE and BS15 groups were significantly lower than those in the control group (P < 0.05). Neither protein content nor mRNA expression level of Bax showed significant changes among the three groups (P > 0.05). Both mRNA expression level and protein content of Caspase-3 and Bax/Bcl-2 ratio in the BS15 group were lower than those in the SNE group (P < 0.05) and higher than those in the control group (P < 0.05). mRNA expression levels of Cytc c, Apaf-1, and Caspase-9 in SNE and BS15 groups were significantly higher than those in the control group (P < 0.05). mRNA expression levels of Cytc c, Apaf-1, and Caspase-9 in the SNE group were also significantly higher than those of the BS15 group but to a limited extent (P > 0.05).
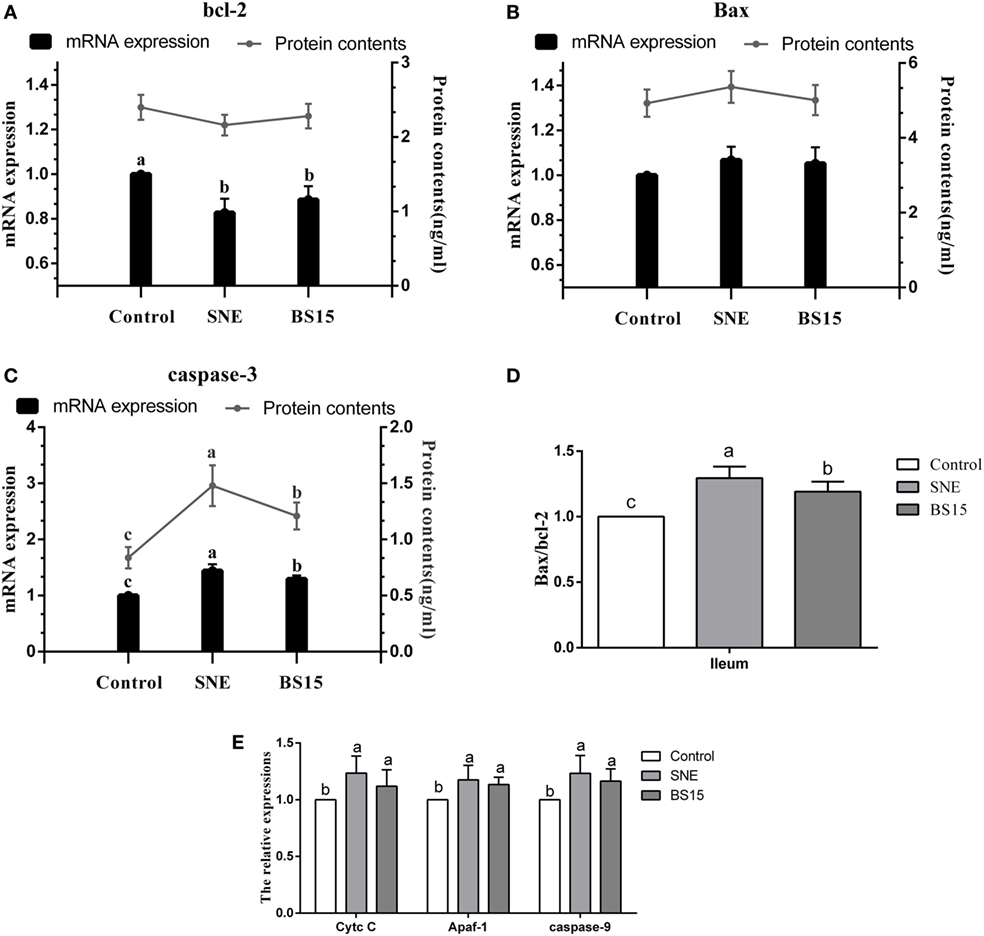
Figure 9. Levels of apoptosis-related proteins in the ileum. Bars with different letters significantly differ on the basis of Duncan’s multiple range tests (P < 0.05). Data are presented as mean ± SD (six replicates of one chick per cage). Cytc c, cytochrome c; Apaf-1, apoptotic protease activating factor 1. (A) Although no significant change in Bcl-2 protein content was detected (P > 0.05), mRNA expression levels of Bcl-2 in SNE and BS15 groups were significantly lower than those in the control group (P < 0.05). (B) Neither protein content nor mRNA expression level of Bax showed significant changes among the three groups (P > 0.05). (C,D) Both mRNA expression levels and protein contents of Caspase-3 and Bax/Bcl-2 ratio in the BS15 group were lower than those in the SNE group (P < 0.05) but higher than those in the control group (P < 0.05). (E) mRNA expression levels of Cytc c, Apaf-1, and Caspase-9 in SNE and BS15 groups were significantly higher than those in the control group (P < 0.05). (E) mRNA expression levels of Cytc c, Apaf-1, and Caspase-9 in the SNE group were also significantly higher than that of BS15 group but to a limited extent (P > 0.05).
Cytokines, MMP-2, and Nrf-2
Figure 10 displays results on cytokine levels in the ileum. mRNA expression levels of IL-2 were significantly lower in the SNE and BS15 groups than those in the control group (P < 0.05). However, protein contents of IL-2 showed no difference (P > 0.05). Both mRNA expression levels and protein contents of IL-8 in the BS15 group were higher than those in the SNE group (P < 0.05) but lower than those in the control group (P < 0.05). In the SNE group, both mRNA expression levels and protein contents of IFN-γ and IL-10 were higher than those in the control group (P < 0.05). Meanwhile, mRNA expression levels of IFN-γ and IL-10 in the BS15 group were lower than those in the SNE group (P < 0.05) but higher than those in the control group (P < 0.05). No significant changes were observed in the levels of other determined cytokines (P > 0.05). As shown in Figure 11, mRNA expression level of MMP-2 in the BS15 group was lower than that in the SNE group (P < 0.05) but significantly higher than that in the control group (P < 0.05). Nrf-2 mRNA expression level was higher in the BS15 group than that in the other two groups (P < 0.05).
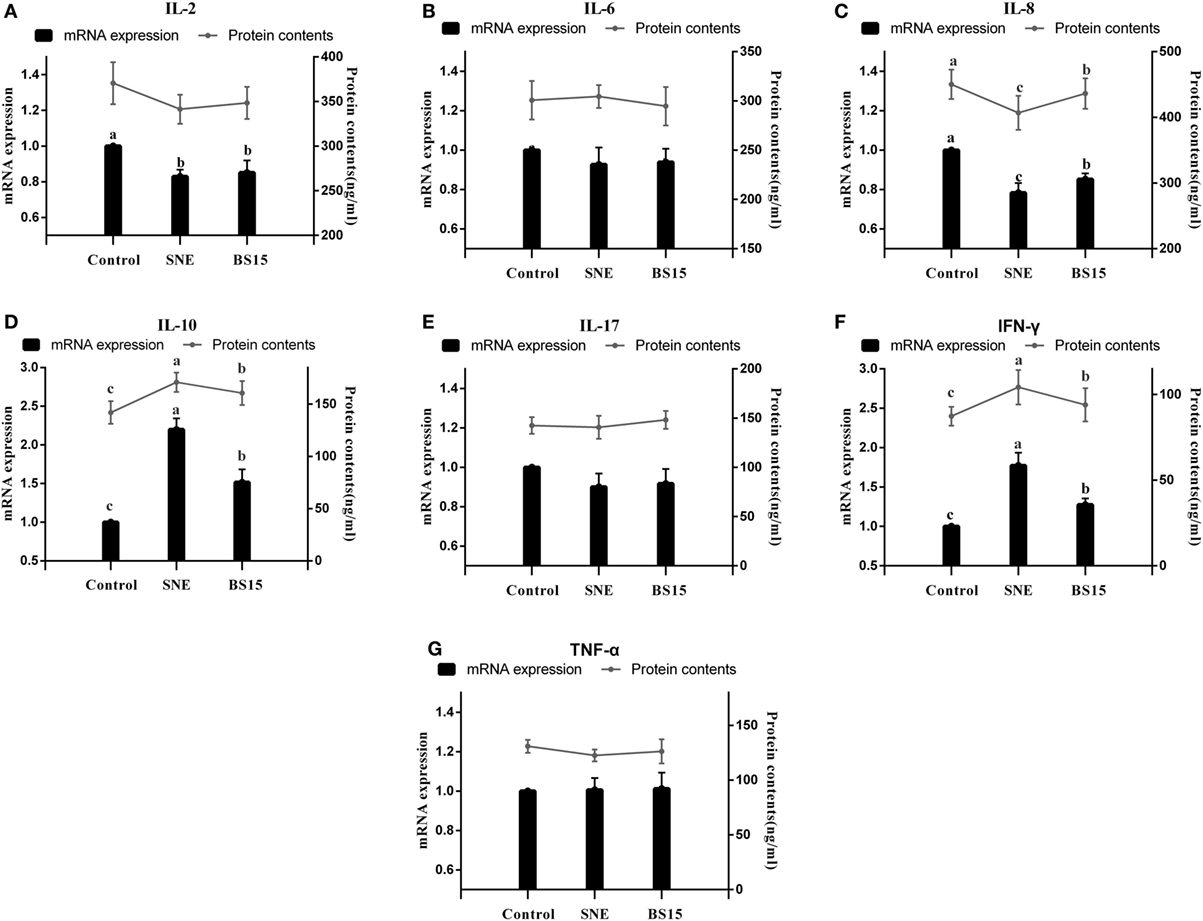
Figure 10. Cytokine levels in the ileum. Bars with different letters significantly differ on the basis of Duncan’s multiple range tests (P < 0.05). Data are presented as mean ± SD (six replicates of one chick per cage). TNF-α, tumor necrosis factor-alpha; IFN-γ, interferon-gamma. (A) mRNA expression levels of IL-2 were significantly lower in SNE and BS15 groups than in the control group (P < 0.05), but no difference in protein contents of IL-2 was observed (P > 0.05). (C) Both mRNA expression levels and protein contents of IL-8 in the BS15 group were higher than those in the SNE group (P < 0.05) but lower than those in the control group (P < 0.05). (D,F) In the SNE group, both mRNA expression levels and protein contents of IFN-γ and IL-10 were higher than those in the control group (P < 0.05). (D,F) mRNA expression levels of IFN-γ and IL-10 in the BS15 group were lower than those in the SNE group (P < 0.05) but higher than those in the control group (P < 0.05). (B,E,F) No significant change was detected among levels of other determined cytokines (P > 0.05).
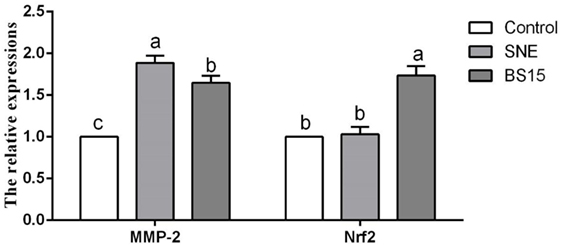
Figure 11. Levels of MMP-2 and Nrf-2 in the ileum. Bars with different letters significantly differ on the basis of Duncan’s multiple range tests (P < 0.05). Data are presented as mean ± SD (six replicates of one chick per cage). MMP-2, matrix metalloproteinase-2; SNE, subclinical necrotic enteritis. (1) mRNA expression level of MMP-2 in BS15 group was lower than that in SNE group (P < 0.05) but significantly higher than that in the control group (P < 0.05). (2) Nrf-2 mRNA expression level was higher in the BS15 group than that in the other two groups (P < 0.05).
Discussion
In animal industry, probiotics are proven to exert beneficial effects on health of animals and to prevent various gut diseases. For example, the dietary probiotic Bacillus coagulans can promote growth performance and improve meat quality of Guangxi Yellow chicken (24). Pan et al. (25) provided evidence that probiotic supplementation can protect weaned pigs against enterotoxigenic Escherichia coli K88 challenge by enhancing immune responses and attenuating intestinal damage. To further gain insights into the direct and indirect effects of probiotic strains on hosts and the mechanisms underlying such effects, numerous studies have been conducted from various aspects. Guo et al. (26) observed that supplementation of the probiotic Bacillus subtilis for rabbits can improve growth performance, intestinal homeostasis, immune organ index, innate immune response, and disease resistance. They also discovered that induction of β-defensin may be related to the beneficial effects of B. subtilis. In addition, Eeckhaut et al. (8) reported that probiotic Butyricicoccus pullicaecorum can reduce the abundance of some potentially important pathogens in the caeca and ileum and thus prevent NE in broilers. In our recent study on Lactobacillus plantarum, the giant panda-isolated probiotic can protect mice from enterotoxigenic E. coli infection by attenuating inflammation and maintaining integrity of the intestinal epithelial barrier (27). However, although the assumption that probiotics can protect the body from gut diseases by enhancing gut immunity (28), improving gut development (29), and altering gut microbiota (30) has been well accepted, the exact mechanisms on how beneficial microbes cooperate with immunity to provide resistance to pathogens and the role of probiotics in immunity remain unclear (31, 32).
Feng et al. (33) demonstrated in a previous study that NE changed composition of ileal bacterial microbiota of broiler chickens by significantly reducing the abundances of Lactobacilli and Lactobacillus aviaries; this result correlated negatively with the lesions of NE. As we have discovered in our recent study, L. johnsonii BS15 may enhance intestinal development and balance microbiota in jejunum and ileum and thus control growth performance in broilers with SNE (19). Hence, based on our previous results, the present study aimed to prove the effect of BS15 on gut immunity in preventing SNE in broilers. Aside from the ileum, the cecal tonsil—one of the intestinal sections affected by lesions—was also selected and partially examined. Cecal tonsil is the largest lymphoid organ of avian gut-associated lymphoid tissues, from which both T and B cells are present in the germinal centers (34). Cecal tonsil is also an important component of the mucosal immune system and performs unique immune functions (35). However, in our present study, no changes were determined in this organ.
Subclinical NE is characterized by poor growth performance without mortality. Previous statistical analyses showed strong correlations between SNE and increased FCR and retarded growth rate of broilers (36). Poor growth performance is considered to be primarily caused by coccidia-induced leakage of proteins, including plasma, into the lumen of small intestines; this condition provides nutrient substrates for rapid replication of CP and damages digestive ability of the small intestines (37). According to the present results on growth performance, SNE exerted a significant negative influence on FCR, agreeing with our recent results (5) and those of other former studies (38, 39), whereas BS15 can be effective in controlling growth performance under SNE. Jayaraman et al. (40) also reported that B. subtilis PB6 can control growth performance of broilers with SNE. Results also showed that BS15 mainly exerts its beneficial effects by influencing performance at the starter phase given the significantly improved growth performance (higher ADG and lower FCR) against SNE that was observed when adding BS15 before SNE infection, whereas limited effects were observed in the treatment group, in which BS15 was added after the development of SNE. This phenomenon revealed that BS15 may be ineffective when SNE has already developed. These results provide important information on the study and application of BS15. According to histological examinations, SNE significantly damaged villi structure and integrity of lamina propria, showing agreement with recent studies on SNE (41). Nevertheless, broilers in BS15 group were free from damaged villi structure. Considering the important role of the small intestine on absorptive, metabolic, and digestive functions (42), BS15 may have decreased FCR caused by SNE by maintaining integrity of the small intestine.
Avian IgA is present in the majority of intestinal cells, shows similarity to mammalian IgA, and releases sIgA into the gut lumen through transepithelial transport (43). IgA is widely considered critical for protecting mucosal surfaces against toxins, viruses, and bacteria by neutralizing or preventing these pathogens from binding to the mucosal surface. sIgA also plays a role in maintenance of mucosal homeostasis, which determines the composition of intestinal microbiota and influences the development of systemic immunity (44). According to results on Ig detection, the numbers of IgA+ B cells and contents of IgA and sIgA were significantly decreased by SNE infection but controlled by BS15. The same trend was also observed on the level of IgG, one of the three major Ig classes in intestinal mucosal immunity of chickens (45). Results indicated that BS15 can enhance Igs to prevent SNE in broilers.
Changes in T cell subsets are considered one of the most important indicators of the level of intestinal mucosal immunity. T cells can be divided into subsets based on the cell surface proteins that they express, specifically CD3, the molecular surface marker of mature T cells. CD4+ T cells are associated with major histocompatibility complex (MHC) class II molecules and act as helper or inflammatory T cells in response to exogenous antigens, whereas CD8+ T cells are associated with MHC class I molecules and play a crucial role as cytotoxic T cells in response to endogenous antigens (46). CD4+/CD8+ ratio is a direct index for evaluating the condition of body immunity (47). Recent studies demonstrated that probiotics can improve T cell subsets through regulation of gut microbiota (48). Huang et al. (49) observed that combined probiotics consisting of Streptococcus faecalis, Clostridium buthricum, and Bacillus mesentericus can induce T cell subsets in the intestine of broiler chicks. These probiotics mainly cause an influx of CD8+ T cells into the intestinal mucosa; this influx may enhance intestinal immunity. A previous study on human subjects also showed that dietary supplementation of probiotic Bacillus polyfermenticus, which can regulate gut microbiota, possesses a potentially positive effect on immune function by modulating the number of immune cell populations, such as CD4+ and CD8+ T cells (50). In the present study, significantly negative changes were observed in LPLs of the ileum of the SNE group. Olkowski et al. (3) provided evidence that the pathological process of NE in small intestines spreads first throughout the lamina propria, moves toward the center of villus, and then affects the epithelium, thus agreeing with our results. MMP-2, which plays a key role in mediation of collagen degradation in soft connective tissues, was induced by SNE and can therefore be considered an indicator of the disease (51). In the present study, in the ileum of the SNE group, we also detected significantly high mRNA expression levels of MMP-2, which was also controlled by BS15, suggesting that BS15 may downregulate MMP-2 and thus protect LPL in the ileum from SNE.
Antioxidant enzymes, including CAT, SOD, and GSH-Px, play important roles in degradation of superoxide anions and hydrogen peroxide and protect against oxidative stress (52). In broilers, antioxidant capacity of intestinal mucosa is also crucial for healthy intestinal function. Additionally, high levels of MDA contents imply enhancement of lipid peroxidation and accumulation of lipid peroxides in intestinal mucosa and significant oxidative damage to a wide range of biological molecules, including DNA, lipids, proteins, and carbohydrates (53). In the present study, BS15 exhibited a positive effect on antioxidant ability by significantly increasing T-AOC, SOD, and CAT activities and decreasing MDA level, suggesting the ability of BS15 to improve antioxidant capacity in the small intestine. Lactobacilli modulate epithelial cytoprotection through the Nrf-2 pathway, which is closely related to oxidative stress (54). In the present results, mRNA expression level of Nrf-2 in the BS15 group significantly increased, and this event may be correlated with improved antioxidant capacity. Oxidative damage can induce molecular lesions and trigger apoptosis (55). Proteins of Bcl-2 family located on the mitochondrial membrane can alter mitochondrial membrane permeability and trigger apoptosis (56). High Bax/Bcl-2 ratio is related to increased vulnerability to apoptotic activation (57). In the present study, the highest Bax/Bcl-2 ratio was observed in the SNE group, suggesting that mitochondria-mediated apoptosis was activated by SNE. BS15 also effectively controlled the side effects caused by SNE as indicated by the significantly lower Bax/Bcl-2 ratio in the BS15 group than in the SNE group. Once the balance between Bcl-2 and Bax is disrupted, caspase-dependent apoptotic pathway can be activated, resulting in increases in Cytc c, Apaf-1, Caspase-3, and Caspase-9. Briefly, Cytc c leaks out through the holes formed by Bax in the mitochondrial membrane, triggering the association of Apaf-1 to form an apoptosome; caspase-9 is activated through the apoptosome, which then triggers Caspase-3 activation and ultimately causes cell apoptosis (58). In the present study, all results of apoptosis-related proteins were in correspondence with the caspase-dependent apoptotic pathway, indicating that BS15 may control oxidative damage in the small intestine and regulate intestinal apoptosis while protecting broilers from SNE.
As determinants and modulators of immune pathology, cytokines are present in the normal intestinal mucosal membrane and function in mucosal immunity (59). Under various pathologic circumstances, the intestine has been proposed as a possible source of pro-inflammatory cytokines (60). In the present study, both contents and mRNA expression levels of IL-2, IL-8, IL-10, and IFN-γ were influenced by SNE infection and controlled by BS15. Although numerous cytokines in mammalian systems have been studied widely, little work has been performed on non-mammalian systems to date. Some cytokines exhibit different biological activities compared with those of mammals; gene sequences of proteins also differ substantially from those of other mammalian proteins (61). Similar to mammalian IL-2, chicken IL-2 exhibits potential as an immune activator, and treatment of recombinant chicken IL-2 protein induced peripheral blood lymphocytes to express cell surface IL-2 receptors within 48 h and resulted in increased proportions of peripheral blood CD4+ and CD8+ T cells (62). Chicken IL-2 was also reported to exhibit T cell proliferative activity (63). Therefore, the decreased levels of IL-2 mRNA expression in SNE group indicated inhibition of activation and proliferation of T cells; this result was in agreement with the negative influences of T cell subsets caused by SNE in the present study. Results of IL-8 agree with those of Xu et al. (64) in their study on control of NE by dietary selenium. Possibly, IL-8 is affected by the activation of Nrf-2/antioxidant response pathway, indicating a possible link between changes in IL-8 and antioxidant capacity in the ileum during SNE (31). Recent studies reported that altered production of either IFN-γ or IL-10 may or may not be considered a common marker that is associated with protective immunity against NE (65); increases in IFN-γ (66) and IL-10 (67) were also discussed in research on NE. In our present study, similar changes in both IFN-γ and IL-10 levels were observed in the ileum, suggesting the preventive ability of BS15 supplementation against SNE in broilers. As chicken cytokines remain poorly understood, especially in those the intestines, additional research are needed to demonstrate specific cytokine functions and the relationship between all cytokines and the avian immune system (68).
Conclusion
Feed supplementation with L. johnsonii BS15 may prevent SNE-caused decrease in growth performance of broilers. Changes influenced by BS15 may be related to the enhancement of intestinal immunity of the small intestine. Additional intensive research on improved levels of Igs, better T cell subsets, enhanced antioxidant and anti-apoptotic capacity, and improved cytokines are required before the mechanism of BS15 in prevention of SNE can be determined.
Ethics Statement
All animal experiments were performed in accordance with guidelines for the care and use of laboratory animals and approved by the Institutional Animal Care and Use Committee of Sichuan Agricultural University (approval number: SYXKchuan2014-187).
Author Contributions
HW, DZ, and XN designed the experiments. LL, KP, XQ, JL, and BJ performed the experiments. LL, GL, and AK analyzed the experimental data. HW and DZ wrote this paper. All authors read and approved the final manuscript.
Conflict of Interest Statement
The authors declare that the research was conducted in the absence of any commercial or financial relationships that could be construed as a potential conflict of interest.
Funding
This study was supported by the International Cooperative Project of Science and Technology Bureau of Sichuan Province (2013HH0055) and the Science and Technology Support Project of Science and Technology Bureau of Sichuan Province (2014FZ0076). Both funding bodies provided funding support for the animal purchase and index determination.
References
1. Smyth JA. Pathology and diagnosis of necrotic enteritis: is clear-cut? Avian Pathol (2016) 45:282–7. doi:10.1080/03079457.2016.1158780
2. Cooper KK, Songer JG. Virulence of Clostridium perfringens in an experimental model of poultry necrotic enteritis. Vet Microbiol (2010) 142:323–8. doi:10.1016/j.vetmic.2009.09.065
3. Olkowski AA, Wojnarowicz C, Chirino-Trejo M, Laarveld B, Sawicki G. Sub-clinical necrotic enteritis in broiler chickens: novel etiological consideration based on ultra-structural and molecular changes in the intestinal tissue. Res Vet Sci (2008) 85:543–53. doi:10.1016/j.rvsc.2008.02.007
4. Shojadoost B, Vince A, Prescott J. The successful experimental induction of necrotic enteritis in chickens by Clostridium perfringens: a critical review. Vet Res (2012) 43:74. doi:10.1186/1297-9716-43-74
5. Wang H, Ni X, Liu L, Zeng D, Lai J, Qing X, et al. Controlling of growth performance, lipid deposits and fatty acid composition of chicken meat through a probiotic, Lactobacillus johnsonii during subclinical Clostridium perfringens infection. Lipids Health Dis (2017) 16:38. doi:10.1186/s12944-017-0408-7
6. Tian X, Shao Y, Wang Z, Guo Y. Effects of dietary yeast β-glucans supplementation on growth performance, gut morphology, intestinal Clostridium perfringens population and immune response of broiler chickens challenged with necrotic enteritis. Anim Feed Sci Tech (2016) 215:144–55. doi:10.1016/j.anifeedsci.2016.03.009
7. Fernandes da Costa SP, Mot D, Bokori-Brown M, Savva CG, Basak AK, Van Immerseel F, et al. Protection against avian necrotic enteritis after immunisation with NetB genetic or formaldehyde toxoids. Vaccine (2013) 31:4003–8. doi:10.1016/j.vaccine.2013.05.063
8. Eeckhaut V, Wang J, Van Parys A, Haesebrouck F, Joossens M, Falony G, et al. The probiotic Butyricicoccus pullicaecorum reduces feed conversion and protects from potentially harmful intestinal microorganisms and necrotic enteritis in broilers. Front Microbiol (2016) 7:1416. doi:10.3389/fmicb.2016.01416
9. Zhou M, Zeng D, Ni X, Tu T, Yin Z, Pan K, et al. Effects of Bacillus licheniformis on the growth performance and expression of lipid metabolism-related genes in broiler chickens challenged with Clostridium perfringens-induced necrotic enteritis. Lipids Health Dis (2016) 15:48. doi:10.1186/s12944-016-0219-2
10. Serban DE. Gastrointestinal cancers: influence of gut microbiota, probiotics and prebiotics. Cancer Lett (2014) 345:258–70. doi:10.1016/j.canlet.2013.08.013
11. Patten DA, Laws AP. Lactobacillus-produced exopolysaccharides and their potential health benefits: a review. Benef Microbes (2015) 6:457–71. doi:10.3920/BM2014.0117
12. Kang HJ, Im SH. Probiotics as an immune modulator. J Nutr Sci Vitaminol (2015) 61:s103–5. doi:10.3177/jnsv.61.S103
13. Jacobsen CN, Rosenfeldt-Nielsen V, Hayford AE, Møller PL, Michaelsen KF, Paerregaard A, et al. Screening of probiotic activities of forty-seven strains of Lactobacillus spp. by in vitro techniques and evaluation of the colonization ability of five selected strains in humans. Appl Environ Microbiol (1999) 65:4949–56.
14. Srutkova D, Schwarzer M, Hudcovic T, Zakostelska Z, Drab V, Spanova A, et al. Bifidobacterium longum CCM 7952 promotes epithelial barrier function and prevents acute DSS-induced colitis in strictly strain-specific manner. PLoS One (2015) 10(7):e0134050. doi:10.1371/journal.pone.0134050
15. Xin J, Zeng D, Wang H, Ni X, Yi D, Pan K, et al. Preventing non-alcoholic fatty liver disease through Lactobacillus johnsonii BS15 by attenuating inflammation and mitochondrial injury and improving gut environment in obese mice. Appl Microbiol Biotechnol (2014) 98:6817–29. doi:10.1007/s00253-014-5752-1
16. Liu L, Ni X, Zeng D, Wang H, Jing B, Yin Z, et al. Effect of a dietary probiotic, Lactobacillus johnsonii BS15, on growth performance, quality traits, antioxidant ability, and nutritional and flavour substances of chicken meat. Anim Prod Sci (2016) 57:920–6. doi:10.1071/AN15344
17. Wang H, Ni X, Qing X, Zeng D, Luo M, Liu L, et al. Live probiotic Lactobacillus johnsonii BS15 promotes growth performance and lowers fat deposition by improving lipid metabolism, intestinal development, and gut microflora in broilers. Front Microbiol (2017) 8:1073. doi:10.3389/fmicb.2017.01073
18. National Research Council (NRC). Nutrient Requirements of Poultry. 9th ed. Washington, DC: National Academy Press (1994).
19. Qing X, Zeng D, Wang H, Ni X, Liu L, Lai J, et al. Preventing subclinical necrotic enteritis through Lactobacillus johnsonii BS15 by ameliorating lipid metabolism and intestinal microflora in broiler chickens. AMB Express (2017) 7:139. doi:10.1186/s13568-017-0439-5
20. Wu B, Guo H, Cui H, Peng X, Fang J, Zuo Z, et al. Pathway underlying small intestine apoptosis by dietary nickel chloride in broiler chickens. Chem Biol Interact (2015) 243:91–106. doi:10.1016/j.cbi.2015.11.010
21. Todd D, Singh AJ, Greiner DL, Mordes JP, Rossini AA, Bortell R. A new isolation method for rat intraepithelial lymphocytes. J Immunol Methods (1999) 224:111–27. doi:10.1016/S0022-1759(99)00015-0
22. Reséndiz-Albor A, Esquivel R, López-Revilla R, Verdín L, Moreno-Fierros L. Striking phenotypic and functional differences in lamina propria lymphocytes from the large and small intestine of mice. Life Sci (2005) 76:2783–803. doi:10.1016/j.lfs.2004.08.042
23. Liu J, Cui H, Peng X, Fang J, Zuo Z, Deng J, et al. Decreased IgA+ B cells population and IgA, IgG, IgM contents of the cecal tonsil induced by dietary high fluorine in broilers. Int J Environ Res Public Health (2013) 10:1775–85. doi:10.3390/ijerph10051775
24. Zhou X, Wang Y, Gu Q, Li W. Effect of dietary probiotic, Bacillus coagulans, on growth performance, chemical composition, and meat quality of Guangxi Yellow chicken. Poult Sci (2010) 89:588–93. doi:10.3382/ps.2009-00319
25. Pan L, Zhao PF, Ma XK, Shang QH, Xu YT, Long SF, et al. Probiotic supplementation protects weaned pigs against enterotoxigenic Escherichia coli K88 challenge and improves performance similar to antibiotics. J Anim Sci (2017) 95:2627–39. doi:10.2527/jas.2016.1243
26. Guo M, Wu F, Hao G, Qi Q, Li R, Li N, et al. Bacillus subtilis improves immunity and disease resistance in rabbits. Front Immunol (2017) 8:354. doi:10.3389/fimmu.2017.00354
27. Liu Q, Ni X, Wang Q, Peng Z, Niu L, Wang H, et al. Lactobacillus plantarum BSGP201683 isolated from giant panda feces attenuated inflammation and improved gut microflora in mice challenged with enterotoxigenic Escherichia coli. Front Microbiol (2017) 8:1885. doi:10.3389/fmicb.2017.01885
28. Vieira AT, Teixeira MM, Martins FS. The role of probiotics and prebiotics in inducing gut immunity. Front Immunol (2013) 4:445. doi:10.3389/fimmu.2013.00445
29. Chen GO, Sleman SMB, Mingan C, Paul AI. Novel probiotics: their effects on growth performance, gut development, microbial community and activity of broiler chickens. Anim Nutr (2015) 1:184–91. doi:10.1016/j.aninu.2015.07.003
30. Ubeda C, Pamer EG. Antibiotics, microbiota, and immune defense. Trends Immunol (2012) 33:459–66. doi:10.1016/j.it.2012.05.003
31. Zhang X, Chen X, Song H, Chen HZ, Rovin BH. Activation of the Nrf2/antioxidant response pathway increases IL-8 expression. Eur J Immunol (2005) 35:3258–67. doi:10.1002/eji.200526116
32. Sassonecorsi M, Raffatellu M. No vacancy: how beneficial microbes cooperate with immunity to provide colonization resistance to pathogens. J Immunol (2015) 194:4081–7. doi:10.4049/jimmunol.1403169
33. Feng Y, Gong J, Yu H, Jin Y, Zhu J, Han Y. Identification of changes in the composition of ileal bacterial microbiota of broiler chickens infected with Clostridium perfringens. Vet Microbiol (2010) 140:116–21. doi:10.1016/j.vetmic.2009.07.001
34. Lillehoj HS, Trout JM. Avian gut-associated lymphoid tissues and intestinal immune responses to Eimeria parasites. Clin Microbiol Rev (1996) 9:349–60.
35. Liu J, Cui H, Peng X, Fang J, Zuo Z, Wu B, et al. Changes induced by high dietary fluorine in the cecal tonsil cytokine content of broilers. Fluoride (2012) 45:94–9.
36. Kaldhusdal M, Hofshagen M. Barley inclusion and avoparcin supplementation in broiler diets. 2. Clinical, pathological, and bacteriological findings in a mild form of necrotic enteritis. Poult Sci (1992) 71:1145–53. doi:10.3382/ps.0711145
37. Van Immerseel F, Rood JI, Moore RJ, Titball RW. Rethinking our understanding of the pathogenesis of necrotic enteritis in chickens. Trends Microbiol (2008) 17:32–6. doi:10.1016/j.tim.2008.09.005
38. Dahiya JP, Wilkie DC, Van Kessel AG, Drew MD. Potential strategies for controlling necrotic enteritis in broiler chickens in post-antibiotic era. Anim Feed Sci Tech (2006) 129:60–88. doi:10.1016/j.anifeedsci.2005.12.003
39. Gholamiandehkordi AR, Timbermont L, Lanckriet A, Van DBW, Pedersen K, Dewulf J, et al. Quantification of gut lesions in a subclinical necrotic enteritis model. Avian Pathol (2007) 36:375–82. doi:10.1080/03079450701589118
40. Jayaraman S, Thangavel G, Kurian H, Mani R, Mukkalil R, Chirakkal H. Bacillus subtilis PB6 improves intestinal health of broiler chickens challenged with Clostridium perfringens-induced necrotic enteritis. Poult Sci (2013) 92:370–4. doi:10.3382/ps.2012-02528
41. Timbermont L, Haesebrouck F, Ducatelle R, Van IF. Necrotic enteritis in broilers: an updated review on the pathogenesis. Avian Pathol (2011) 40:341–7. doi:10.1080/03079457.2011.590967
42. Levin RJ. Review. The effect of hormones on the absorptive, metabolic and digestive functions of the small intestines. J Endocrinol (1969) 45:315–48. doi:10.1677/joe.0.0450315
43. Lindner C, Wahl B, Föhse L, Suerbaum S, Macpherson AJ, Prinz I, et al. Age, microbiota, and T cells shape diverse individual IgA repertoires in the intestine. J Exp Med (2012) 209:365–77. doi:10.1084/jem.20111980
44. Lammers A, Wieland WH, Kruijt L, Jansma A, Straetemans T, Schots A, et al. Successive immunoglobulin and cytokine expression in the small intestine of juvenile chicken. Dev Comp Immunol (2010) 34:1254–62. doi:10.1016/j.dci.2010.07.001
45. Mockett AP. Monoclonal antibodies used to isolate IgM from chicken bile and avian sera and to detect specific IgM in chicken sera. Avian Pathol (1986) 15:337–48. doi:10.1080/03079458608436297
46. Brisbin J, Zhou H, Gong J, Sabour P, Akbari M, Haghighi H, et al. Gene expression profiling of chicken lymphoid cells after treatment with Lactobacillus acidophilus cellular components. Dev Comp Immunol (2008) 32:563–74. doi:10.1016/j.dci.2007.09.003
47. Hernández J, Garfias Y, Nieto A, Mercado C, Montaño L, Zenteno E. Comparative evaluation of the CD4+CD8+ and CD4+CD8− lymphocytes in the immune response to porcine rubulavirus. Vet Immunol Immunopathol (2001) 79:249–59. doi:10.1016/S0165-2427(01)00259-8
48. Salzman NH. The role of the microbiome in immune cell development. Ann Allergy Asthma Immunol (2014) 113:593–8. doi:10.1016/j.anai.2014.08.020
49. Huang A, Shibata E, Nishimura E, Igarashi Y, Isobe N. Effects of probiotics on the localization of T cell subsets in the intestine of broiler chicks. J Poult Sci (2013) 50:275–81. doi:10.2141/jpsa.0120134
50. Kim HS, Park H, Cho IY, Paik HD, Park E. Dietary supplementation of probiotic Bacillus polyfermenticus, Bispan strain, modulates natural killer cell and T cell subset populations and immunoglobulin G levels in human subjects. J Med Food (2006) 9:321–7. doi:10.1089/jmf.2006.9.321
51. Wachtel M, Frei K, Ehler E, Fontana A, Winterhalter K, Gloor SM. Occludin proteolysis and increased permeability in endothelial cells through tyrosine phosphatase inhibition. J Cell Sci (1999) 112:4347–56.
52. Shen X, Yi D, Ni X, Zeng D, Jing B, Lei M, et al. Effects of Lactobacillus plantarum on production performance, immune characteristics, antioxidant status, and intestinal microflora of bursin-immunized broilers. Can J Microbiol (2014) 60:193–202. doi:10.1139/cjm-2013-0680
53. Marnett LJ. Lipid peroxidation-DNA damage by malondialdehyde. Mutat Res (1999) 424:83–95. doi:10.1016/S0027-5107(99)00010-X
54. Jones RM, Desai C, Darby TM, Luo L, Wolfarth AA, Scharer CD, et al. Lactobacilli modulate epithelial cytoprotection through the Nrf2 pathway. Cell Rep (2015) 12:1217–25. doi:10.1016/j.celrep.2015.07.042
55. Kasprzak KS. The role of oxidative damage in metal carcinogenicity. Chem Res Toxicol (1991) 4:604–15. doi:10.1021/tx00024a002
56. Jean-Claude M, Richard JY. Mitochondria in apoptosis: Bcl-2 family members and mitochondrial dynamics. Dev Cell (2011) 21:92–101. doi:10.1016/j.devcel.2011.06.017
57. Jarskog LF, Selinger ES, Lieberman JA, Gilmore JH. Apoptotic proteins in the temporal cortex in schizophrenia: high Bax/Bcl-2 ratio without caspase-3 activation. Am J Psychiatry (2004) 161:109–15. doi:10.1176/appi.ajp.161.1.109
58. Peng X, Chen K, Chen J, Fang J, Cui H, Zuo Z, et al. Aflatoxin B1 affects apoptosis and expression of Bax, Bcl-2, and Caspase-3 in thymus and bursa of fabricius in broiler chickens. Environ Toxicol (2015) 31:1113–20. doi:10.1002/tox.22120
59. Miguel N, Antonio F, Xavier F, Wlandimiro J, Anna F. Tumor necrosis factor and interleukin-6 in spontaneous bacterial peritonitis in cirrhosis: relationship with the development of renal impairment and mortality. Hepatology (2010) 27:1227–32. doi:10.1002/hep.510270507
60. Else KJ, Finkelman FD. Invited review intestinal nematode parasites, cytokines and effector mechanisms. Int J Parasitol (1998) 28:1145–58. doi:10.1016/S0020-7519(98)00087-3
61. Sundick RS, Gill-Dixon CA. Cloned chicken lymphokine homologous to both mammalian IL-2 and IL-15. J Immunol (1997) 159:720–5.
62. Hilton LS, Bean AG, Kimpton WG, Lowenthal JW. Interleukin-2 directly induces activation and proliferation of chicken T cells in vivo. J Interferon Cytokine Res (2002) 22:755–63. doi:10.1089/107999002320271341
63. Kaiser P, Mariani P. Promoter sequence, exon:intron structure, and synteny of genetic location show that a chicken cytokine with T-cell proliferative activity is IL2 and not IL15. Immunogenetics (1999) 49:26–35. doi:10.1007/s002510050460
64. Xu S, Lee SH, Lillehoj HS, Hong YH, Bravo D. Effects of dietary selenium on host response to necrotic enteritis in young broilers. Res Vet Sci (2015) 98:66–73. doi:10.1016/j.rvsc.2014.12.004
65. Lee KW, Lillehoj HS, Jeong W, Jeoung HY, An DJ. Avian necrotic enteritis: experimental models, host immunity, pathogenesis, risk factors, and vaccine development. Poult Sci (2011) 90:1381–90. doi:10.3382/ps.2010-01319
66. Lee KW, Kim DK, Lillehoj HS, Jang SI, Lee SH. Immune modulation by Bacillus subtilis-based direct-fed microbials in commercial broiler chickens. Anim Feed Sci Tech (2015) 200:76–85. doi:10.1016/j.anifeedsci.2014.12.006
67. Cao L, Yang XJ, Li ZJ, Sun FF, Wu XH, Yao JH. Reduced lesions in chickens with Clostridium perfringens-induced; necrotic enteritis by Lactobacillus fermentum 1.2029. Poult Sci (2013) 92:1143. doi:10.3382/ps.2013-92-4-1143
Keywords: subclinical necrotic enteritis, Lactobacillus johnsonii, intestinal immunity, probiotic, broiler
Citation: Wang H, Ni X, Qing X, Liu L, Lai J, Khalique A, Li G, Pan K, Jing B and Zeng D (2017) Probiotic Enhanced Intestinal Immunity in Broilers against Subclinical Necrotic Enteritis. Front. Immunol. 8:1592. doi: 10.3389/fimmu.2017.01592
Received: 05 September 2017; Accepted: 06 November 2017;
Published: 20 November 2017
Edited by:
Juarez Antonio Simões Quaresma, Universidade Federal do Pará, BrazilReviewed by:
Maryam Dadar, Razi Vaccine and Serum Research Institute, IranHerbert Leonel de Matos Guedes, Universidade Federal do Rio de Janeiro, Brazil
Copyright: © 2017 Wang, Ni, Qing, Liu, Lai, Khalique, Li, Pan, Jing and Zeng. This is an open-access article distributed under the terms of the Creative Commons Attribution License (CC BY). The use, distribution or reproduction in other forums is permitted, provided the original author(s) or licensor are credited and that the original publication in this journal is cited, in accordance with accepted academic practice. No use, distribution or reproduction is permitted which does not comply with these terms.
*Correspondence: Dong Zeng, zend@sicau.edu.cn
†These authors have contributed equally to this work.