- 1Laboratory for Malaria Research, Instituto Oswaldo Cruz (IOC), Fundação Oswaldo Cruz (Fiocruz), Rio de Janeiro, Brazil
- 2Service of Primatology, Instituto de Ciência e Tecnologia em Biomodelos, Fiocruz, Rio de Janeiro, Brazil
- 3Laboratory of Immunoparasitology, Instituto Oswaldo Cruz (IOC), Fiocruz, Rio de Janeiro, Brazil
Non-human primates (NHP) are suitable models for studying different aspects of the human system, including pathogenesis and protective immunity to many diseases. However, the lack of specific immunological reagents for neo-tropical monkeys, such as Saimiri sciureus, is still a major factor limiting studies in these models. An alternative strategy to circumvent this obstacle has been the selection of immunological reagents directed to humans, which present cross-reactivity with NHP molecules. In this context and considering the key role of inhibitory immunoreceptors—such as the signal regulatory protein α (SIRPα)—in the regulation of immune responses, in the present study, we attempted to evaluate the ability of anti-human SIRPα monoclonal antibodies to recognize SIRPα in antigen-presenting S. sciureus peripheral blood mononuclear cells (PBMC). As shown by flow cytometry analysis, the profile of anti-SIRPα staining as well as the levels of SIRPα-positive cells in PBMC from S. sciureus were similar to those observed in human PBMC. Furthermore, using anti-SIRPα monoclonal antibody, it was possible to detect a decrease of the SIRPα levels on surface of S. sciureus cells after in vitro stimulation with lipopolysaccharides. Finally, using computed-based analysis, we observed a high degree of conservation of SIRPα across six species of primates and the presence of shared epitopes in the extracellular domain between humans and Saimiri genus that could be targeted by antibodies. In conclusion, we have identified a commercially available anti-human monoclonal antibody that is able to detect SIRPα of S. sciureus monkeys and that, therefore, can facilitate the study of the immunomodulatory role of SIRPα when S. sciureus is used as a model.
Introduction
Saimiri sciureus, also known as squirrel monkey, is a small species of non-human primate natively found in the tropical rainforests of South America (1, 2). As many other non-human primates (NHP), S. sciureus is used in diverse areas of biomedical research and, although its full genome has not yet been sequenced, the well-known close phylogenetic relationship of NHP to humans renders this model an accurate system to study biological, immunological, and pharmacologycal phenomena of medical importance (2). Indeed, S. sciureus has been shown to be susceptible to several human pathogens and, in this way, has been proposed as model for study the pathogenesis of malaria (3), measles (4), HTLV-associated diseases (5), BK virus infection (6), and vaginal trichomoniasis (7). Moreover, S. sciureus has been studied in the context of Parkinson’s disease therapy (8) and, as recommended by the World Health Organization (9), malaria vaccine candidates have been frequently tested in preclinical trials using S. sciureus in the last three decades (10, 11). However, the lack of specific immunological tools to assess immune response of S. sciureus represents a major factor limiting vaccinology and immunopathology studies using this model.
An alternative strategy to circumvent this limitation is the identification of immunological reagents directed to molecules of human immune system that also present reactivity with S. sciureus. In fact, a variety of anti-human monoclonal antibodies commercially available are able to satisfactorily detect surface molecules of immune cells as well as cytokines of S. sciureus (12–14) and other non-human primate models, such as common marmoset (Callithrix jacchus), rhesus macaque (Macaca mulatta), and chimpanzee (Pan troglodytes) (15–17). To the best of our knowledge, however, there is no evaluation concerning the signal regulatory protein α (SIRPα) in NHP.
Signal regulatory protein α is a transmembrane protein present in leukocytes of the myeloid lineage, including monocytes and dendritic cells (DC), which is implicated in inhibitory signaling of innate immune functions, such as phagocytosis, proinflammatory cytokine production, and DC maturation (18–20), as well as induction of programmed cell death (21). Comprehensively, SIRPα is believed to play a relevant role in the regulation of immune responses, impacting the pathogenesis of etiologically distinct diseases as well as vaccination (22–24). Nevertheless, SIRPα has not been investigated in non-human primate models. Thus, attempting to support further studies related to involvement of SIRPα in immune responses, in the present work, we evaluated by flow cytometry if monoclonal antibody directed to human SIRPα cross-reacts with peripheral blood mononuclear cells (PBMC) from S. sciureus.
Materials and Methods
Animals and Blood Samples
Seven clinically healthy S. sciureus monkeys from the breeding colony at the Department of Primatology of the Instituto de Ciência e Tecnologia em Biomodelos/Fiocruz, Rio de Janeiro, Brazil, were studied. Animals were male adults, aged 3–10 years, housed in accordance with the guidelines of the institutional ethical committee for animal use. For blood sample collection, animals were anesthetized with a combination of 0.1 mL midazolan and 0.4 mL ketamine and, then, 4 mL heparinized venous blood were drawn via femoral venipuncture. All animal experimentation was performed in compliance with the protocol reviewed and approved by the Fiocruz ethical committee (LW-9/14). Peripheral blood samples (4 mL) from five healthy human donors were also obtained by venipuncture in heparinized tubes, as approved by the Fiocruz Research Ethic Committee (46084015.1.0000.5248).
PBMC Isolation and Antigenic Stimulation
Peripheral blood mononuclear cells were isolated from S. sciureus whole blood through density gradient centrifugation using Histopaque-1077 (Sigma). Cells were washed twice in RPMI-1640 medium (Sigma) containing 2.05 mM l-glutamine, 25 mM Hepes, and 2.0 g/L sodium bicarbonate and, then, resuspended in RPMI medium supplemented with 200 U/mL penicillin (Gibco), 200 mg/mL streptomycin (Gibco), and 10% inactivated fetal calf serum (Gibco). Cells (2.5 × 105) were assayed ex vivo or after 24 h stimulation with Escherichia coli lipopolysaccharides (LPS, 5 µg/mL, Sigma) in 96-well culture plates (Falcon) at 37°C in 5% CO2.
Flow Cytometry Assay
Detection of SIRPα in S. sciureus PBMC was assayed by flow cytometry using allophycocyanin (APC)-conjugated anti-human SIRPα monoclonal antibody purchased from eBioscience (isotype: mouse/IgG2a, clone: 15-414). Cells (2.5 × 105) were washed in phosphate saline buffer (PBS) and, subsequently, incubated at 4°C for 30 min in PBS containing 10% fetal bovine serum (FBS) to reduce non-specific staining. After incubation, cells were stained with 2.0 µL anti-SIRPα monoclonal antibody or APC-conjugated isotype control (eBioscience) at 4°C for 40 min in 100 µL PBS containing 1% FBS. Cells were washed twice and, finally, analyzed by a FACSVerse flow cytometer (Becton Dickinson). In parallel, anti-SIRPα monoclonal antibody was tested ex vivo with PBMC obtained from blood human samples, as described in Section “PBMC Isolation and Antigenic Stimulation.”
Computer-Assisted Analysis of Sequence Alignment and Potential B-Cell Epitopes
To detect SIRPα protein homology among several primate species, protein BLAST were done and protein sequences of Homo sapiens (AAH26692.1), P. troglodytes (JAA44167.1), C. jacchus (JAB51896.1), Macaca fascicularis (XP_015313155.1), Gorilla gorilla (XP_004061735.2), and Saimiri boliviensis (XP_010350139.1) were analyzed. Multiple alignment CLUSTAL OMEGA, distance matrix, and the phylogenetic tree were done using the Megalign Pro 15 (Lasergene DNASTAR) program and the circular map of protein alignment was generated using the software GenVision 15 (Lasergene DNASTAR). The prediction of linear B-cell epitopes was carried out using the web server BepiPred. For each input FASTA sequence of extracellular domain of SIRPα, the server outputs a epitope prediction score for each amino acid. The recommended cutoff of 0.35 was used to determine potential B-cell linear epitopes, ensuring sensibility of 49% and specificity of 75%. Linear B-cell epitopes of SIRPα extracellular domain of H. sapiens and S. boliviensis were predicted to be located at the residues with the highest scores in at least nine consecutive amino acids.
Results and Discussion
Signal regulatory protein α has been studied by flow cytometric analysis in both human (25, 26) and animal models, i.e., mice, rats, and cattle (27–29), but the frequency and distribution of SIRPα-positive cells in peripheral blood has not been reported. Thus, to investigate the reactivity of anti-human SIRPα monoclonal antibody with S. sciureus PBMC by flow cytometry; we first evaluated anti-SIRPα staining profile in PBMC obtained from five normal healthy human donors.
Signal regulatory protein α is known as an immune inhibitory receptor present in leukocytes of the myeloid lineage and, therefore, it is expected that SIRPα in PBMC population is mainly detected on surface of cells showing monocyte morphology by size and granularity analysis in flow cytometry using forward scatter and sideward scatter parameters (30). Indeed, an elevated percentage (95.55 ± 1.16%) of SIRPα-positive cells was observed in the human monocyte population, while only 3.27 ± 3.38% cells presented SIRPα in the lymphocyte population (Figures 1A and 2). Moreover, SIRPα-positive cells corresponded to 18.98 ± 3.12% of total PBMC, agreeing with the frequency of total myeloid innate immune cells found in human PBMC samples, which manly comprises monocytes and DC (31, 32).
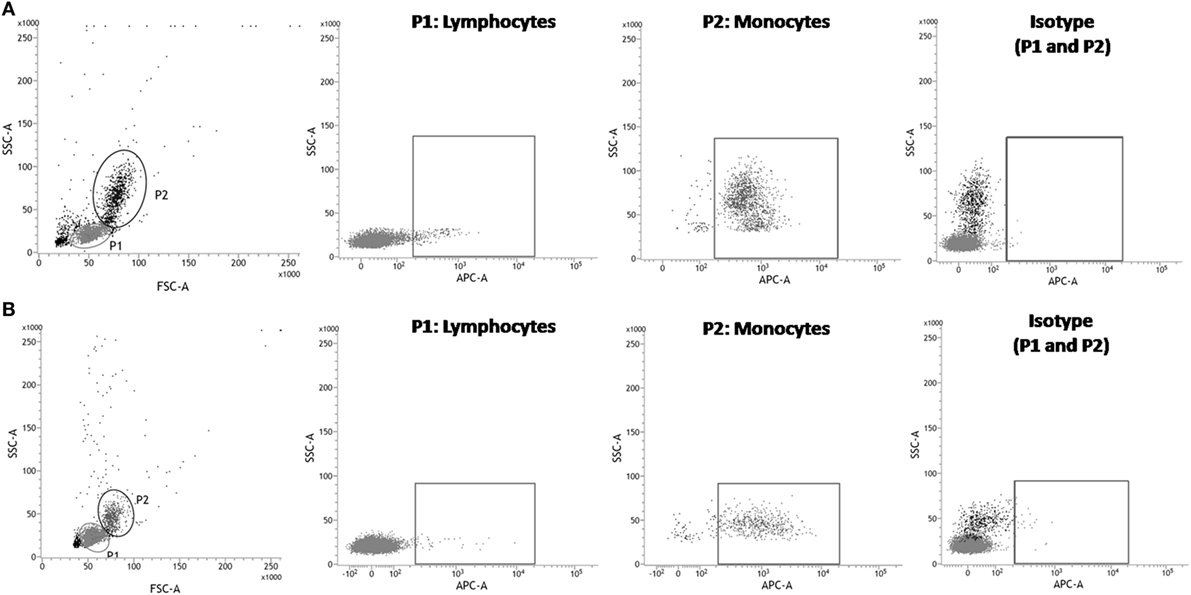
Figure 1. Flow cytometry analysis of anti-human signal regulatory protein α (SIRPα) monoclonal antibody cross-reactivity with Saimiri sciureus cells. Peripheral blood mononuclear cells (PBMC) were isolated from human or S. sciureus whole blood, stained with anti-SIRPα monoclonal antibody or isotype control and, then, analyzed by flow cytometry. Reactivity of anti-SIRPα antibodies [allophycocyanin (APC)] with human (A) and S. sciureus (B) PBMC was evaluated gating lymphocytes (P1) or monocytes populations, as defined by forward scatter (FSC) and sideward scatter (SSC) parameters.
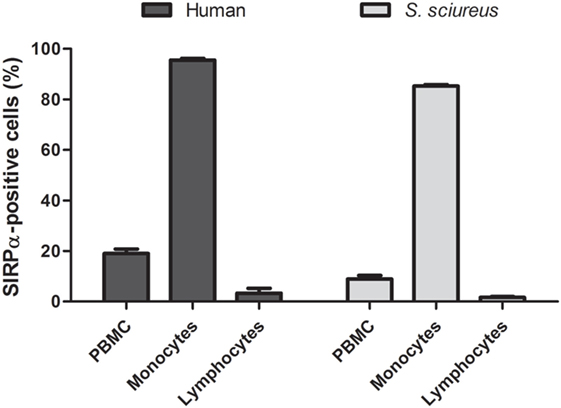
Figure 2. Frequency of signal regulatory protein α (SIRPα)-positive cells in peripheral blood mononuclear cells (PBMC) samples from humans and Saimiri sciureus monkeys. PBMC were isolated from whole blood, stained with anti-human SIRPα monoclonal antibody and, then, analyzed by flow cytometry. Cells presenting SIRPα were quantified considering three main cells populations by morphological criteria: total PBMC, monocytes, and lymphocytes, as shown in Figure 1. Data represent mean ± SEM for five humans and seven monkeys.
Subsequently, anti-human SIRPα monoclonal antibody was tested against S. sciureus cells. Previous reports demonstrated that different immune cell surface receptors as well as cytokines of S. sciureus can be detected by a range of antibodies directed to human (12–14) and, in the same way, we observed that anti-human SIRPα antibody cross-reacted with cell surface of S. sciureus PBMC. As shown in Figure 1B, the profile of anti-SIRPα staining in PBMC from S. sciureus was similar to that observed in human samples. SIRPα-positive S. sciureus cells corresponded to 8.92 ± 3.65% of total PBMC and 1.59 ± 1.03% of the lymphocyte population, while an increased frequency of SIRPα-presenting cells (85.27 ± 1.41%) was observed in monocytes population (Figure 2). These data suggest that anti-human SIRPα antibody recognizes a specific antigen present on surface of S. sciureus innate immune cells, possibly the cognate of human SIRPα in S. sciureus.
Although the cross-reactivity of antibodies cannot indicate per se the degree of homology between proteins across phyla, an increased similarity (>90%) has been shown through molecular approaches between human, S. sciureus, and other NHP concerning nucleotide sequence of genes coding for many cytokines (33, 34) as well as dopamine transport (35) and, therefore, it was already possible to quantify gene expression of 12 S. sciureus cytokines (IL-1A, IL-2, IL-4, IL-5, IL-6, IL-10, IL-12B, IL-17, IFN-β, IFN-γ, LTA, and TNF) by commercially available real-time PCR assays using predesigned human gene-specific primers and probes (14). Moreover, genomic studies demonstrate the presence of SIRPα gene in a vast group of animals, from cats to NHP, supporting that SIRPα is a ubiquitous molecule of innate immune system of mammalians (36, 37). In the case of S. sciureus SIRPα, however, there are no molecular data available, i.e., neither genome nor SIRPα gene was reported yet, limiting the analysis of S. sciureus SIRPα homology with their cognates in other primates.
In this scenario, to confirm that the cross-reactivity herein detected was a natural consequence of the similarity of SIRPα protein across primates, we aligned the amino acid sequences of SIRPα from six different primate species including Saimiri and Homo sapiens (Figure 3). As expected, a significant degree of identity was observed across the primates, which showed a complete matching in 72% of all sequences analyzed (Figure 3A). The homology rate ranged from 87% (M. Fascicularis vs. S. boliviensis) to 99% (P. troglodites vs. G. gorilla) and human SIRPα showed a high identity with its orthologs, ranging from 88% in S. boliviensis to 98% in G. gorilla, despite the deletion of 58 amino acid present in S. boliviensis sequence, which was determinant to reduce the homology rate (Figures 3A,B). Since the amino acid sequence to which the commercial anti-human SIRPα monoclonal binds is not available, we also checked if the deletion in S. boliviensis, which is taxonomically the closest to S. sciureus among the NHP species studied herein, could potentially influence the antibody recognition. In this way, we verified the potential epitopes shared between the H. sapiens and S. boliviensis through analysis of linear B-cell epitopes in SIRPα extracellular domain and we observed at least 10 B-cell epitopes that can be targeted by antibodies (Figure 4). Importantly, all of these regions were shared by both species, indicating that anti-human SIRPα antibodies can target SIRPα of Saimiri monkeys in a similar way to its orthologous in human.
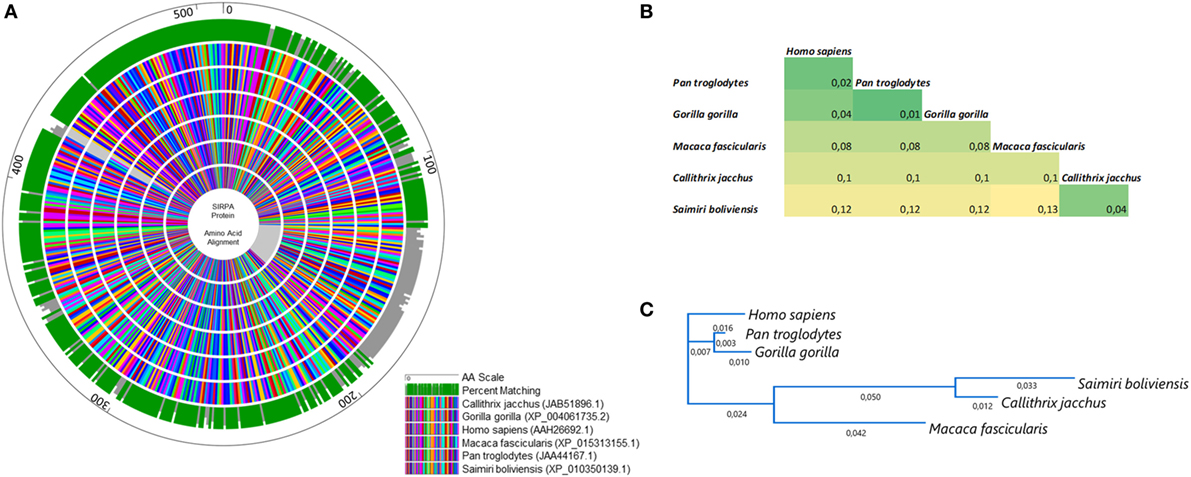
Figure 3. Homology analysis of signal regulatory protein α (SIRPα) across primates. (A) Circular alignment of amino acid sequences of SIRPα protein in human and five non-human primates (Pan troglodytes, Gorilla gorilla, Macaca fascicularis, Callithrix jacchus, Saimiri boliviensis). The outer circle shows the amino acid scale. Green and gray bars on the second circle show the percent matching among all sequences used in the analysis. Inner circles show the sequence alignment in which each amino acid was represented by a different color. (B) Pairwise distance among all primates studied and (C) phylogenetic tree based on SIRPα protein alignments.
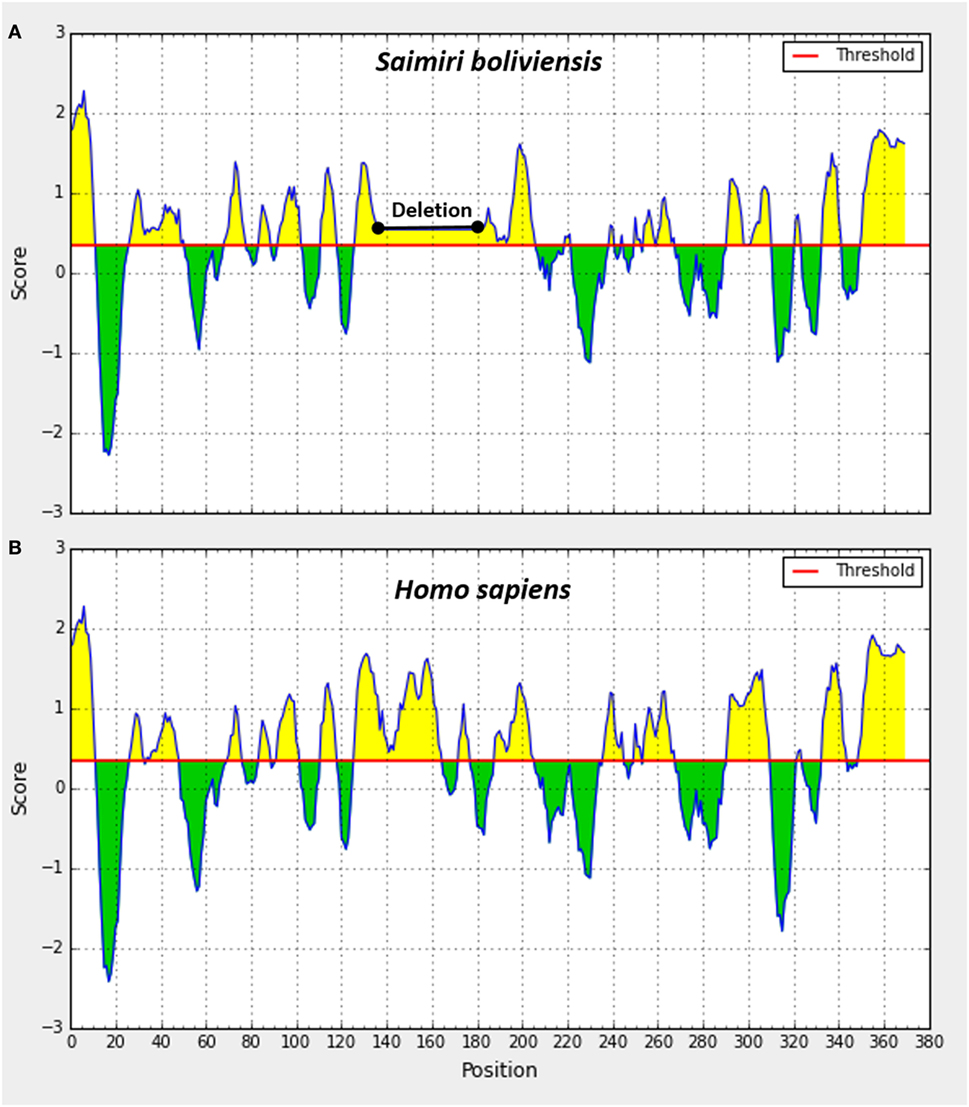
Figure 4. Prediction of linear B-cell epitopes in extracellular domain of signal regulatory protein α protein in Saimiri (A) and Homo sapiens (B). Linear B-cell epitopes were predicted to be located at the residues with the scores above 0.35 (yellow) and regions not predicted to be B-cell epitopes are under the threshold (green). The epitope score represents the average of the scores of least nine consecutive amino acids above the cut-off, and the sequences with higher mean values were detected as potential linear epitopes.
Thus, to better study the capacity of anti-human antibodies to detect S. sciureus SIRPα, we additionally evaluated the levels of this immune receptor on surface of PBMC after stimulation with LPS. It has been described that pathogen-associated molecular patterns present modulatory effects on SIRPα levels in macrophages and DC and, in this context, LPS was recognized as a negative modulator (24, 38, 39). Indeed, analyzing monocytes population by flow cytometry, which mainly includes innate immune cells present in PBMC, we found that the anti-human SIRPα monoclonal antibody was able to identify a significant reduction not only in the frequency of SIRPα-positive cells but also in the levels of SIRPα present on the surface of these cells after LPS stimulation (Figure 5). Despite LPS-mediated regulation of SIRPα expression has not been investigated in human or NHP PBMC, decreased levels of SIRPα on the surface of peripheral blood monocytes were found in LPS-treated pigs and it was already reported a downregulation of SIRPα gene expression in cultured primary mouse microglia following LPS-stimulation (40, 41), agreeing with our data on PBMC and, consequently, supporting that anti-human SIRPα antibodies can recognize SIRPα of S. sciureus, whose levels were downmodulated by LPS in monocyte population.
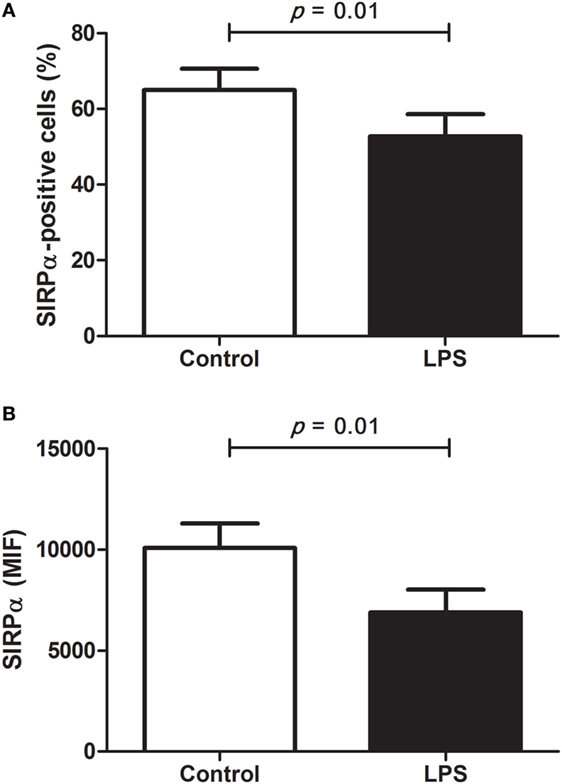
Figure 5. Modulation of signal regulatory protein α (SIRPα) levels by lipopolysaccharides (LPS) in Saimiri sciureus cells. Peripheral blood mononuclear cells (PBMC) of S. sciureus monkeys (n = 7) were incubated for 24 h in presence or absence (control) of LPS and, then, SIRPα was detected in monocytes population by flow cytometry using anti-human monoclonal antibody. (A) Frequency of SIRPα-positive cells in PBMC and (B) levels of SIRPα present on cell surface of SIRPα-positive cells (monocytes), as measured by mean fluorescence intensity. Data (mean ± SEM) are representative of two separate experiments. Statistical difference was tested by paired t-test in GraphPad Prism 5.0 software and p < 0.05 was considered significant.
Collectively, the flow cytometry assays showing that SIRPα-positive cells are similarly present and distributed in PBMC of human and S. sciureus, together with observation by computed-based analysis that SIRPα has a high degree of conservation across primates, with the presence of conserved B-cell epitopes in the extracellular domain between humans and the Saimiri genus, strongly indicate that anti-SIRPα antibodies directed to humans can detect SIRPα of S. sciureus. Take into account the role of SIRPα in the negative regulation of immune responses, we believe that further studies in S. sciureus or other non-human primate models, exploring SIRPα signaling with anti-human antibodies, may help the understanding of the immunopathogenesis of diseases, such as cancer, neurodegenerative disorders, and infectious diseases, and, consequently, contribute to the development of therapeutic and vaccinal strategies that mitigate their impact in public health.
Ethics Statement
This study was carried out in accordance with the recommendations and approved by the Fiocruz Ethics Committee on Animal Use (CEUA Licence LW-9/14).
Author Contributions
HS and EC-C performed the experiments and helped PT in drafting the manuscript. CB-J and MA carried out animal manipulation and helped in the experiments. LP-R performed the experiments and helped in the computed-based analysis. JL-J performed the computed-based analyses and reviewed the manuscript. CD-R reviewed the manuscript. PT performed data analysis and reviewed the manuscript.
Conflict of Interest Statement
The authors declare that the research was conducted in the absence of any commercial or financial relationships that could be construed as a potential conflict of interest.
Acknowledgments
This study was supported through funding from the Programa de Desenvolvimento Tecnológico em Insumos para Saúde (PDTIS/Fiocruz), the Programa de Apoio a Núcleos de Excelência (Pronex) [Departamento de Ciência e Tecnologia (DECIT) do Ministério da Saúde do Brasil, Conselho Nacional de Desenvolvimento Científico e Tecnológico (CNPq, Brazil) and Fundação de Amparo à Pesquisa do Estado do Rio de Janeiro (FAPERJ)], the Neglected Disease Program from FAPERJ, and the Instituto Oswaldo Cruz (Fiocruz, Brazil) POM grants. CTDR is recipient of a Research Productivity Fellowship from the CNPq and CTDR and JCLJ received grants from FAPERJ as “Cientista do Nosso Estado” and “Jovem Cientista do Nosso Estado”, respectively.
References
1. Lynch Alfaro JW, Boubli JP, Paim FP, Ribas CC, Silva MN, Messias MR, et al. Biogeography of squirrel monkeys (genus Saimiri): south-central Amazon origin and rapid pan-Amazonian diversification of a lowland primate. Mol Phylogenet Evol (2015) 82 Pt B:436–54. doi:10.1016/j.ympev.2014.09.004
2. Phillips KA, Bales KL, Capitanio JP, Conley A, Czoty PW, ‘t Hart BA, et al. Why primate models matter. Am J Primatol (2014) 76:801–27. doi:10.1002/ajp.22281
3. Contamin H, Behr C, Mercereau-Puijalon O, Michel J. Plasmodium falciparum in the squirrel monkey (Saimiri sciureus): infection of non-splenectomised animals as a model for exploring clinical manifestations of malaria. Microbes Infect (2000) 2:945–54. doi:10.1016/S1286-4579(00)00401-9
4. Delpeut S, Sawatsky B, Wong XX, Frenzke M, Cattaneo R, von Messling V. Nectin-4 interactions govern measles virus virulence in a new model of pathogenesis, the squirrel monkey (Saimiri sciureus). J Virol (2017) 91:e02490–16. doi:10.1128/JVI.02490-16
5. Kazanji M. HTLV type 1 infection in squirrel monkeys (Saimiri sciureus): a promising animal model for HTLV type 1 human infection. AIDS Res Hum Retroviruses (2000) 16:1741–6. doi:10.1089/08892220050193245
6. Zaragoza C, Li RM, Fahle GA, Fischer SH, Raffeld M, Lewis AM, et al. Squirrel monkeys support replication of BK virus more efficiently than simian virus 40: an animal model for human BK virus infection. J Virol (2005) 79:1320–6. doi:10.1128/JVI.79.2.1320-1326.2005
7. Gardner WA, Culberson DE, Scimeca JM, Brady AG, Pindak FF, Abee CR. Experimental genital trichomoniasis in the squirrel monkey (Saimiri sciureus). Genitourin Med (1987) 63:188–91.
8. Quik M, Mallela A, Ly J, Zhang D. Nicotine reduces established levodopa-induced dyskinesias in a monkey model of Parkinson’s disease. Mov Disord (2013) 28:1398–406. doi:10.1002/mds.25594
9. World Health Organization. Role of non-human primates in malaria vaccine development: memorandum from a WHO meeting. Bull World Health Organ (1988) 66:719–28.
10. James MA, Kakoma I, Ristic M, Cagnard M. Induction of protective immunity to Plasmodium falciparum in Saimiri sciureus monkeys with partially purified exoantigens. Infect Immun (1985) 49:476–80.
11. Carvalho LJ, Alves FA, Bianco C, Oliveira SG, Zanini GM, Soe S, et al. Immunization of Saimiri sciureus monkeys with a recombinant hybrid protein derived from the Plasmodium falciparum antigen glutamate-rich protein and merozoite surface protein 3 can induce partial protection with Freund and Montanide ISA720 adjuvants. Clin Diagn Lab Immunol (2005) 12:242–8. doi:10.1128/CDLI.12.2.242-248.2005
12. Contamin H, Loizon S, Bourreau E, Michel JC, Garraud O, Mercereau-Puijalon O, et al. Flow cytometry identification and characterization of mononuclear cells subsets in the neotropical primate Saimiri sciureus (squirrel monkey). J Immunol Methods (2005) 297:61–71. doi:10.1016/j.jim.2004.11.019
13. Carville A, Evans TI, Reeves RK. Characterization of circulating natural killer cells in neotropical primates. PLoS One (2013) 8:e78793. doi:10.1371/journal.pone.0078793
14. Riccio EK, Pratt-Riccio LR, Bianco-Júnior C, Sanchez V, Totino PR, Carvalho LJ, et al. Molecular and immunological tools for the evaluation of the cellular immune response in the neotropical monkey Saimiri sciureus, a non-human primate model for malaria research. Malar J (2015) 14:166. doi:10.1186/s12936-015-0688-1
15. Ozwara H, Niphuis H, Buijs L, Jonker M, Heeney JL, Bambra CS, et al. Flow cytometric analysis on reactivity of human T lymphocyte-specific and cytokine-receptor-specific antibodies with peripheral blood mononuclear cells of chimpanzee (Pan troglodytes), rhesus macaque (Macaca mulatta), and squirrel monkey (Saimiri sciureus). J Med Primatol (1997) 26:164–71. doi:10.1111/j.1600-0684.1997.tb00048.x
16. Kireta S, Zola H, Gilchrist RB, Coates PT. Cross-reactivity of anti-human chemokine receptor and anti-TNF family antibodies with common marmoset (Callithrix jacchus) leukocytes. Cell Immunol (2005) 236:115–22. doi:10.1016/j.cellimm.2005.08.017
17. Rodriguez AR, Hodara V, Murthy K, Morrow L, Sanchez M, Bienvenu AE, et al. T cell interleukin-15 surface expression in chimpanzees infected with human immunodeficiency virus. Cell Immunol (2014) 288:24–30. doi:10.1016/j.cellimm.2014.01.009
18. Latour S, Tanaka H, Demeure C, Mateo V, Rubio M, Brown EJ, et al. Bidirectional negative regulation of human T and dendritic cells by CD47 and its cognate receptor signal-regulator protein-alpha: down-regulation of IL-12 responsiveness and inhibition of dendritic cell activation. J Immunol (2001) 167:2547–54. doi:10.4049/jimmunol.167.5.2547
19. Smith RE, Patel V, Seatter SD, Deehan MR, Brown MH, Brooke GP, et al. A novel MyD-1 (SIRP-1alpha) signaling pathway that inhibits LPS-induced TNFalpha production by monocytes. Blood (2003) 102:2532–40. doi:10.1182/blood-2002-11-3596
20. Janssen WJ, McPhillips KA, Dickinson MG, Linderman DJ, Morimoto K, Xiao YQ, et al. Surfactant proteins A and D suppress alveolar macrophage phagocytosis via interaction with SIRP alpha. Am J Respir Crit Care Med (2008) 178:158–67. doi:10.1164/rccm.200711-1661OC
21. Irandoust M, Alvarez Zarate J, Hubeek I, van Beek EM, Schornagel K, Broekhuizen AJ, et al. Engagement of SIRPa inhibits growth and induces programmed cell death in acute myeloid leukemia cells. PLoS One (2013) 8:e52143. doi:10.1371/journal.pone.0052143
22. Barclay AN, Van den Berg TK. The interaction between signal regulatory protein alpha (SIRPα) and CD47: structure, function, and therapeutic target. Annu Rev Immunol (2014) 32:25–50. doi:10.1146/annurev-immunol-032713-120142
23. Yi T, Li J, Chen H, Wu J, An J, Xu Y, et al. Splenic dendritic cells survey red blood cells for missing self-CD47 to trigger adaptive immune responses. Immunity (2015) 43:764–75. doi:10.1016/j.immuni.2015.08.021
24. Liu Q, Wen W, Tang L, Qin CJ, Lin Y, Zhang HL, et al. Inhibition of SIRPα in dendritic cells potentiates potent antitumor immunity. Oncoimmunology (2016) 5:e1183850. doi:10.1080/2162402X.2016.1183850
25. Barros MM, Yamamoto M, Figueiredo MS, Cançado R, Kimura EY, Langhi DM Jr, et al. Expression levels of CD47, CD35, CD55, and CD59 on red blood cells and signal-regulatory protein-alpha,beta on monocytes from patients with warm autoimmune hemolytic anemia. Transfusion (2009) 49:154–60. doi:10.1111/j.1537-2995.2008.01936.x
26. Han Y, Wang H, Shao Z. Monocyte-derived macrophages are impaired in myelodysplastic syndrome. J Immunol Res (2016) 2016:5479013. doi:10.1155/2016/5479013
27. Stephens SA, Howard CJ. Infection and transformation of dendritic cells from bovine afferent lymph by Theileria annulata. Parasitology (2002) 124:485–93. doi:10.1017/S003118200200152X
28. Baba T, Nakamoto Y, Mukaida N. Crucial contribution of thymic Sirp alpha+ conventional dendritic cells to central tolerance against blood-borne antigens in a CCR2-dependent manner. J Immunol (2009) 183:3053–63. doi:10.4049/jimmunol.0900438
29. Signarovitz AL, Ray HJ, Yu JJ, Guentzel MN, Chambers JP, Klose KE, et al. Mucosal immunization with live attenuated Francisella novicida U112ΔiglB protects against pulmonary F. tularensis SCHU S4 in the Fischer 344 rat model. PLoS One (2012) 7:e47639. doi:10.1371/journal.pone.0047639
30. Autissier P, Soulas C, Burdo TH, Williams KC. Evaluation of a 12-color flow cytometry panel to study lymphocyte, monocyte, and dendritic cell subsets in humans. Cytometry A (2010) 77:410–9. doi:10.1002/cyto.a.20859
31. Hoffmann TK, Müller-Berghaus J, Ferris RL, Johnson JT, Storkus WJ, Whiteside TL. Alterations in the frequency of dendritic cell subsets in the peripheral circulation of patients with squamous cell carcinomas of the head and neck. Clin Cancer Res (2002) 8:1787–93.
32. Mortezagholi S, Babaloo Z, Rahimzadeh P, Ghaedi M, Namdari H, Assar S, et al. Evaluation of PBMC distribution and TLR9 expression in patients with systemic lupus erythematosus. Iran J Allergy Asthma Immunol (2016) 15:229–36.
33. Heraud JM, Lavergne A, Kazanji M. Molecular cloning, characterization, and quantification of squirrel monkey (Saimiri sciureus) Th1 and Th2 cytokines. Immunogenetics (2002) 54:20–9. doi:10.1007/s00251-002-0443-y
34. Alves FA1, Souza MT, Gonçalves EC, Schneider MP, Marinho AM, Muniz JA, et al. DNA sequencing of 13 cytokine gene fragments of Aotus infulatus and Saimiri sciureus, two non-human primate models for malaria. Cytokine (2010) 52:151–5. doi:10.1016/j.cyto.2010.09.004
35. Miller GM, Yatin SM, De La Garza R, Goulet M, Madras BK. Cloning of dopamine, norepinephrine and serotonin transporters from monkey brain: relevance to cocaine sensitivity. Mol Brain Res (2001) 87:124–43. doi:10.1016/S0169-328X(00)00288-6
36. van Beek EM, Cochrane F, Barclay AN, van den Berg TK. Signal regulatory proteins in the immune system. J Immunol (2005) 175:7781–7. doi:10.4049/jimmunol.175.12.7781
37. Flies AS, Blackburn NB, Lyons AB, Hayball JD, Woods GM. Comparative analysis of immune checkpoint molecules and their potential role in the transmissible tasmanian devil facial tumor disease. Front Immunol (2017) 8:513. doi:10.3389/fimmu.2017.00513
38. Kong XN, Yan HX, Chen L, Dong LW, Yang W, Liu Q, et al. LPS-induced down-regulation of signal regulatory protein α contributes to innate immune activation in macrophages. J Exp Med (2007) 204:2719–31. doi:10.1084/jem.20062611
39. Dong LW, Kong XN, Yan HX, Yu LX, Chen L, Yang W, et al. Signal regulatory protein α negatively regulates both TLR3 and cytoplasmic pathways in type I interferon induction. Mol Immunol (2008) 45:3025–35. doi:10.1016/j.molimm.2008.03.012
40. Ebdrup L, Krog J, Granfeldt A, Tønnesen E, Hokland M. Dynamic expression of the signal regulatory protein alpha and CD18 on porcine PBMC during acute endotoxaemia. Scand J Immunol (2008) 68:430–7. doi:10.1111/j.1365-3083.2008.02157.x
Keywords: non-human primates, Saimiri sciureus, immune response, signal regulatory protein α, flow cytometry
Citation: Souza HAS, Costa-Correa EH, Bianco-Junior C, Andrade MCR, Lima-Junior JC, Pratt-Riccio LR, Daniel-Ribeiro CT and Totino PRR (2017) Detection of Signal Regulatory Protein α in Saimiri sciureus (Squirrel Monkey) by Anti-Human Monoclonal Antibody. Front. Immunol. 8:1814. doi: 10.3389/fimmu.2017.01814
Received: 31 August 2017; Accepted: 01 December 2017;
Published: 14 December 2017
Edited by:
Greg Woods, University of Tasmania, AustraliaReviewed by:
Annalisa Pinsino, Istituto di biomedicina e di immunologia molecolare Alberto Monroy (CNR), ItalyHao-Ching Wang, Taipei Medical University, Taiwan
Copyright: © 2017 Souza, Costa-Correa, Bianco-Junior, Andrade, Lima-Junior, Pratt-Riccio, Daniel-Ribeiro and Totino. This is an open-access article distributed under the terms of the Creative Commons Attribution License (CC BY). The use, distribution or reproduction in other forums is permitted, provided the original author(s) or licensor are credited and that the original publication in this journal is cited, in accordance with accepted academic practice. No use, distribution or reproduction is permitted which does not comply with these terms.
*Correspondence: Paulo Renato Rivas Totino, cHJ0b3Rpbm9AaW9jLmZpb2NydXouYnI=
†These authors have contributed equally to this work.