- 1Institute of Immunity and Transplantation, University College London, London, United Kingdom
- 2Division of Cancer Studies, University College London, London, United Kingdom
Langerhans cells (LC) are a unique population of tissue-resident macrophages that form a network of cells across the epidermis of the skin, but which have the ability to migrate from the epidermis to draining lymph nodes (LN). Their location at the skin barrier suggests a key role as immune sentinels. However, despite decades of research, the role of LC in skin immunity is unclear; ablation of LC results in neither fatal susceptibility to skin infection nor overt autoimmunity due to lack of immune regulation. Our understanding of immune processes has traditionally been centered on secondary lymphoid organs as sites of lymphocyte priming and differentiation, which is exemplified by LC, initially defined as a paradigm for tissue dendritic cells that migrate to draining LN on maturation. But, more recently, an awareness of the importance of the tissue environment in shaping effector immunity has emerged. In this mini-review, we discuss whether our lack of understanding of LC function stems from our lymph node-centric view of these cells, and question whether a focus on LC as immune regulators in situ in the skin may reveal clearer answers about their function in cutaneous immunology.
Introduction
Langerhans cells (LC) are a unique population of mononuclear phagocytes that are seeded from common macrophage precursors in the skin epidermis before birth (1) (and reviewed in this topic). They are highly conserved across vertebrate species (2, 3) and this, with their location at the interface with the environment, suggests the strategic importance of LC as immune sentinels at the skin barrier surface.
Traditionally, immunologists have focused on secondary lymphoid organs as the center of T cell immunity, assuming that instructions given during the priming of naïve T cells by migratory and resident dendritic cells (DC) were sufficient for differentiation and effector function by T cells recruited to peripheral sites of tissue injury. However, the field is beginning to appreciate importance of the tissue environment in regulating effector and regulatory T cell function, and it is clear that antigen-presenting cell-T cell interactions play a key role in T cell survival and function outside lymphoid organs (4). Despite sharing an origin with other tissue-resident macrophages, differentiation of LC is associated with the acquisition of DC-like functions, namely the ability to migrate to skin-draining lymph nodes (LN) and interact with naïve T cells. Observation of this property in the 1980s has dominated the field, and as a result, studies to define LC function in the skin have focused largely on their role as DC-like cells in priming T cell immunity [reviewed by Romani et al. (5)]. However, to date, a consistent role for LC as primers of T cell immunity has not emerged. In particular, there are few scenarios, if any, in which removal of LC ablates immunity to infection, or results in the development of severe autoimmunity in mice; and, despite the common observation that LC are sufficient to prime T cell immunity after the experimental provision of antigen and adjuvants that may favor LC activation and migration [e.g., Ref. (6)], few papers have explicitly identified a crucial role for migratory LC, and not dermal DC, under physiological conditions.
We suggest that shifting our focus to potential roles for LC in situ in the skin, a function more in keeping with their development as macrophages, will provide clearer answers about the importance of these unique cells in skin immunity. In this mini-review, we will consider the evidence for LC functions within the skin (Figure 1), and discuss whether our historic focus on LC as exemplars of migrating DC has skewed our understanding of their role in skin immunity.
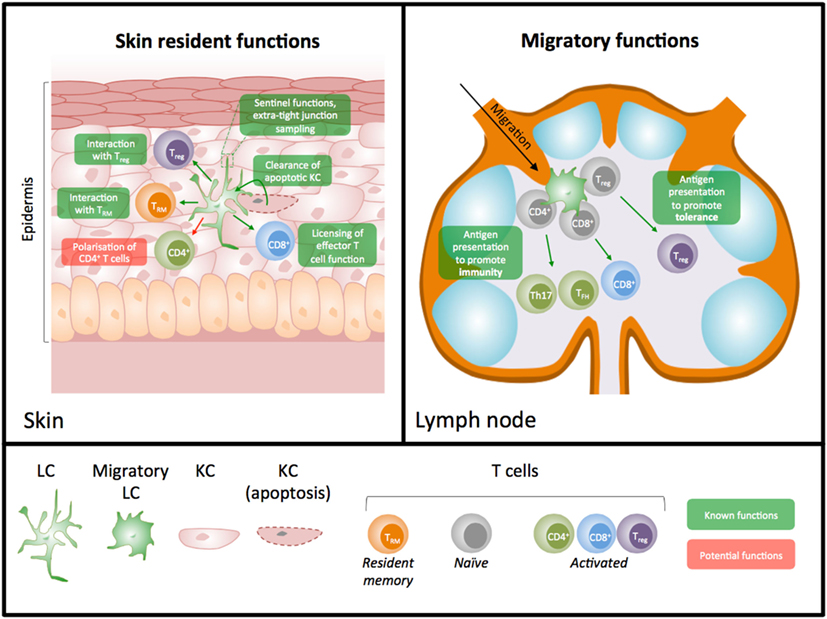
Figure 1. Diagram illustrating known and predicted skin resident versus migratory roles for Langerhans cells (LC). LC have important functions as resident cells in the skin and as migratory cells to the lymph nodes (LN). Studies have largely focused on their potential importance in priming T cell immunity in LN; however, it is now appreciated that LC have many functions in situ in the skin. Demonstrated functions (shown in green) in the skin include interaction with resident memory T cells, clearance of apoptotic keratinocytes, licensing of effector T cell function, sentinel functions, and interaction with regulatory T cells. Data further suggest the potential for other macrophage-like functions of LC such as a role in the polarization of CD4+ T cells in situ (shown in red).
Elucidating LC Function In Situ in the Skin
Barrier Site Surveillance
Sensing the Local Environment
Mononuclear phagocytes have important functions within tissues, and barrier site non-migratory macrophages are essential for surveillance of the local environment. CX3CR1+ intestinal macrophages form dendritic projections, termed transepithelial dendrites, which penetrate the intestinal epithelium to sense commensal microbes and sample luminal antigens. Barrier integrity is maintained by the formation of tight junctions between the macrophages and epithelial cells (7, 8). In the central nervous system, microglia are highly dynamic in their resting state and rapidly extend and retract their processes (9, 10). This motility, termed synaptic pruning, allows them to make frequent and transient contact with synapses, actively engulfing synaptic material (11, 12), and is essential in nervous system development and maintenance (9, 13, 14).
Langerhans cells also constantly extend and retract dendrites between keratinocytes, in a behavior termed dendritic surveillance extension and retraction cycling habitude (15). Barrier integrity is maintained by the de novo formation of tight junctions between keratinocytes and LC, enabling LC to sample the extra-tight junction environment without loss of integrity (16). Thus, in this respect, LC can be seen to closely mimic non-migratory cells, leading to the question of how LC behavior compares to other resident macrophage populations once foreign material has been detected.
Local Interaction with Viruses
Langerhans cells are ideally positioned to respond to viruses that enter the body via the skin, namely human immunodeficiency virus (HIV), herpes viruses such as herpes simplex virus (HSV) or varicella zoster virus, and poxviruses (human papilloma virus, HPV), and this interaction has been extensively reviewed elsewhere [e.g., Ref. (17–19)]. LC express a number of pattern recognition receptors but do not efficiently internalize bacteria in vitro, leading to the suggestion that they preferentially prime antiviral immunity (20, 21). However, infection of LC by HSV or HPV leads to their destruction; priming of T cell immunity in this situation probably depends on transfer of viral antigen to other DC. In the case of HSV, non-infected epidermal LC may acquire antigen from apoptotic LC in the skin and migrate to draining LN. Here, transfer of antigen to cross-presenting DC subsets is required for the initiation of CD8+ T cell immunity (22, 23). By comparison, while LC appear to be the major infected immune cell after HIV infection via the anogenital tract (24), there is evidence that they may restrict HIV replication or transmission (25, 26), and their protective role in HIV infection continues to be investigated (27). In this respect, LC resemble other tissue macrophages, which also restrict HIV and other viral infections (28, 29). Thus, LC may provide a repository of virally infected cells under some conditions, but the implications for this interaction for innate and adaptive immunity still remain unclear.
Innate Control of Skin Immune Homeostasis
A key role for macrophages as resident tissue cells is the maintenance of immune homeostasis. This occurs partly through their scavenger function, rapidly clearing debris from dying cells in the steady state and after infection. This uptake and recognition of apoptotic cells is an important mechanism for maintaining local immune tolerance to self-antigens (30, 31). TAM (TYRO3/AXL/MER) family receptor tyrosine kinases are expressed by macrophages, DC and natural killer cells in the immune system. Engagement of the receptors by their ligands, growth-arrest-specific (GAS)6, and protein S, enhances uptake of apoptotic cells and suppresses inflammation (32). In the gut, MER is upregulated by macrophages in response to induction of apoptosis in intraepithelial cells, probably to control local immune suppression to self-antigens (31). LC employ a similar innate mechanism to control local tolerance in the skin: apoptotic keratinocytes accumulate in the skin of mice depleted of LC (33); and transforming growth factor (TGF)-β induced expression of AXL by LC enhances uptake of GAS6-expressing apoptotic keratinocytes, inhibiting of production of inflammatory cytokines (34).
Regulation of T Cell Function in the Skin
In addition to innate control of the immune environment, tissue-resident cells may also influence adaptive immunity by recruited regulatory and conventional T cells. The following section considers the evidence for LC mediating this function directly in the skin.
Enhancing the Accumulation and Function of Regulatory T Cells In Situ
Tissue macrophages play a key role in the suppression of local adaptive immunity, via direct and indirect interaction with CD4+ regulatory T cells (Treg). CX3CR1-expressing macrophages are an abundant population in the lamina propria of the small intestine, where they regulate Treg differentiation in situ by production of IL-10, retinoid acid (RA) and TGF-β (35), and are critical for the local proliferation of Treg and induction of oral tolerance (36). Likewise, lung-resident tissue macrophages constitutively express TGF-β and RA to induce Treg within the lung tissue (37, 38), while IL-10 production by Kupffer cells in the liver, and microglia in the brain, has been implicated in the induction of Treg-induced tolerance (39–42). In addition to promoting cytokine-mediated differentiation and function, macrophages directly interact with Treg in situ. In a model of experimental autoimmune encephalomyelitis, direct contacts between sialoadhesin+ macrophages and Treg regulated local immune suppression to limit progression of disease (43), and in the liver, presentation of antigen and arrest of Treg by Kupffer cells led to local secretion of IL-10 (41).
Langerhans cells have been directly implicated in a number of Treg-dependent models of immune suppression in the skin but, consistent with the LN-centric view of LC research, it is often unclear whether cellular interactions between LC and Treg are occurring in the LN, the skin, or both. For example, while use of bone marrow chimeras in which radio-resistant LC are selectively deficient for the gene Cdkn1a suggests a clear role for LC in the priming of CD4+ Treg in LN after exposure to ionizing radiation (44), the precise location(s) at which LC are required has not been defined for the accumulation of Treg in the ear skin of Leishmania-infected mice (45), or expansion and function of activated ICOS+ Treg in a murine model of skin sensitization and tolerance (46). However, cognate interaction between LC and CD4+ T cells inhibits effector T cell responses after challenge with topical sensitizers (47), and resting LC from human skin selectively and specifically induce the activation and proliferation of skin resident Treg in vitro (48). Upregulation of receptor activator of nuclear factor κ B (RANK) ligand by apoptotic keratinocytes induces IL-10 production by LC (49), inferring the potential of LC to directly regulate effector T cell immunity in situ, after exposure to ultraviolet (UV)B radiation. This concept is supported by Loser et al. who demonstrated that UV-induced immunosuppression is mediated by RANK-RANKL signaling between LC and keratinocytes, resulting in an increased capacity of LC to induce IL-10-driven CD4+ Treg proliferation (50). CD300a is a glycoprotein expressed by myeloid cells, which binds phosphatidylserine when exposed on the plasma membrane of apoptotic cells. Interaction between CD300a+ LC and apoptotic epithelial cells in the skin restricts numbers of local Treg, mimicking interactions between epithelial cells and CD11b+CX3CR1+ cells in the lungs and gut. This interaction was required for control of S. typhimurium in the gut, but promoted deleterious inflammatory responses including atopic dermatitis in the skin (51). Together, these data strongly support a tissue role for LC in controlling immune suppression by Treg.
Elegant in vivo imaging studies by the Germain lab recently revealed close localization of migrating dermal DC with Treg clusters in mouse LN in the steady state (52), supporting a previous study that demonstrated a specific role for dermal RelB+ DC in the maintenance of skin tolerance (53). Given the sessile behavior of LC in the steady state (54), these data support a dominant role for dermal DC in maintaining day-to-day tolerance to skin antigens. Therefore, the challenge now is to understand whether there are contexts in which LC may take over this regulatory role in LN. A recent study attempted to address this question using a novel genetic model of inducible neo-antigen expression by LC, but not other Langerin+ DC, in the steady state (LCre-GFPOVA mice) (55). In this model, presentation of endogenous ovalbumin leads to priming of CD8+ T cells in LN and accumulation of cutaneous CD4+ Treg. It would be informative to test how the Treg are primed in this model and whether LC are required for their suppressive function in the skin.
Activation of Effector T Cell Immunity in the Skin
While it is accepted that the tissue-immune environment is important for the differentiation and function of Treg, textbook immunology tells us that priming of naïve conventional T cells in LN provides all the requisite signals for differentiation into functional effector/memory T cells. However, numerous studies have now demonstrated the importance of interactions between myeloid cells and conventional T cells for T cell function and survival within peripheral tissues [reviewed in Ref. (4), see also Ref. (56)]. We have demonstrated a unique and novel role for LC in licensing effector function of CD8+ T cells in the epidermis (57). In this section, we will consider the evidence to suggest that LC may also drive the function of other effector T cells in situ.
Licensing of CD8+ T Cell Function by LC
Langerhans cells are highly radio-resistant and, as such, persist following conditioning of patients prior to allogeneic stem cell transplantation for blood cancers and other hematopoietic diseases. Entry of activated donor T cells into inflamed organs, including the skin, frequently leads to graft-versus-host disease (GVHD) in these patients (reviewed in this topic by Santos e Sousa and Chakraverty). LC are sufficient to prime the donor T cell response leading to GVHD (58), but are not required for systemic GVHD in the presence of conventional DC populations (59). To understand whether LC are important in cutaneous GVHD, we combined the Langerin-diphtheria toxin receptor model [in which LC are inducibly depleted upon injection of diphtheria toxin (60)] with a murine allogeneic model of GVHD (61). We demonstrated that LC were not required for priming of donor T cells, in agreement with the published literature (59). However, when we focused on the cellular interactions occurring in the skin, we observed that LC licensed the upregulation of epidermal effector CD8+ T cell function, leading to GVHD at the site of inflammation (57). This research, therefore, revealed a novel role for LC outside LN.
LC-Dependent Control of CD4+ T Cell Function
Polarized CD4+ T cells that exit LN after priming maintain the plasticity to adapt to the tissue environment at their destination (62). This is particularly true for Th17 cells that may acquire a different functional fate depending on signals received from their surroundings. IL-23 production by monocytes and macrophages in inflamed tissues has been closely linked to the activation of IL-17-producing CD4+ T cells [e.g., Ref. (63–65)], and Foucher et al. demonstrated that interaction between human macrophages and memory CD4+ T cells via membrane-bound IL-1α is sufficient to polarize T cells toward Th1 or Th17-like phenotypes (66). Moreover, “inflammatory DC” from patient synovial fluid or tumor ascites stimulate IL-17 production from autologous CD4+ T cells, suggesting that myeloid cells may directly induce differentiation of Th17 cells within tissues (67).
Based on these macrophage data, it seems likely that LC perform a similar role in the skin, and it is clear that activated LC produce Th17-polarizing cytokines ex vivo (68–70). However, the ability of LC to induce differentiation and activation of epidermal Th17 cells in situ has not been directly investigated. LC are required for the priming of Th17 cells in response to topical Candida albicans infection (71, 72), and for the accumulation of both IL-17+CD4+ αβ and γδ T cells in the epidermis of mice with Staphylococcus aureus dysbiosis (73); however, neither study investigated whether LC are required in situ for the functional activity of cutaneous Th17 cells. Likewise, there are currently conflicting data on whether IL-23 production by LC is important for IL-17-dependent psoriasis-like disease (74, 75), and, to date, studies have focused on an Imiquimod-driven, αβ T cell-independent, γδ T cell-dependent model of disease. Thus, the potential importance of interactions between LC and epidermal CD4+ T cells in psoriasis has not been established.
LC and Cutaneous-Resident Memory T Cells
Human skin contains billions of T cells, and the majority express markers associated with retention as resident memory cells (Trm) (76). The concept of LC eliciting rapid localized immunity via activation of these cells is, therefore, very attractive. Precedent for interaction between tissue macrophages and Trm has been set in the vaginal mucosa, where macrophage-derived CCL5 is required for the recruitment and maintenance of clusters of CD4+ Trm that protect against viral challenge (77). However, despite evidence that LC interact directly with CD4+ memory T cells in human skin (48), and data demonstrating that LC interact with, and may control local migration of Trm within the epidermis (78), formation of CD8+ Trm is not impaired in the absence of LC (79).
IL-17 production by human skin Trm is associated with psoriasis (80). However, a subsequent study from the same lab demonstrated that incubation with IL-6, IL-1β, and IL-23 were not sufficient in vitro to induce IL-17 production by CD8+ Trm from healthy skin, suggesting a requirement for cognate T cell receptor-mediated interactions in situ (81). These data strongly infer a role for local interactions with LC, or other epidermal cells, in controlling Trm fate in the context of autoimmunity.
Concluding Remarks: Why Do LC Evolve the Capacity to Migrate?
Despite the development of LC from a common macrophage precursor (1), transcriptional profiling demonstrates a gene expression profile that is more similar to migrating DC than other macrophage populations (3, 82), and LC share an antigen processing and presentation machinery with DC (83). Resident macrophage precursors that are recruited into different sites respond to tissue-specific signals and differentiate into a functional cell type that is adapted to that niche (84). For example, lung macrophages develop the capacity to phagocytose excessive surfactant proteins, thereby preventing alveolar proteinosis (85). These observations lead us to anticipate the existence of environmental pressures in the epidermis leading to functional evolution of LC toward a DC-like cell. However, given the paucity of data showing a clear requirement for LC in priming immunity to infections, and the frequency of DC in the dermis, the unanswered question remains of why the acquisition of a DC-like function by LC is so important for protection of the organism?
Egress of LC from the keratinocyte network in the epidermis to LN is a carefully choreographed process. In the epidermis, inflammation and/or pathogen-derived signals block TGF-β-mediated retention by integrins (79, 86) and promote downregulation of the cell adhesion protein E-cadherin (87), together allowing release of LC from surrounding keratinocytes. Activated LC then secrete metalloproteinases (MMP) 2 and 9, required to break though the basement membrane and enter the dermis (88). Once in the dermis, LC upregulate the chemokine receptor CCR7 that conducts entry into the dermal lymphatics (89, 90), mirroring the migratory pathway used by tissue DC to enter LN.
The requirement to extricate themselves from the keratinocyte network in the epidermis means that LC are slower to arrive in draining LN than dermal DC after antigenic challenge, suggesting that dermal DC may dominate in priming T cell responses to antigens that enter the dermis (54, 91). However, there are scenarios in which LC appear to take charge. Epicutaneous infection models with the pathogens C. albicans or S. aureus have demonstrated clear roles for LC in priming protective CD4+ T cell responses (71, 72, 92), and ionizing radiation specifically activates LC to prime Treg in LN (44). Human, but not murine, LC express high levels of the invariant MHC-like molecule, CD1a. Recognition of CD1a on LC by recruited autoreactive T cells, or T cells specific for the poison ivy lipid urushiol, results in enhanced activation of IL-22- or IL-17-producing CD4+ T cells, respectively (93, 94). These data support murine studies suggesting that LC prime CD4+ T cells responses to some topically applied sensitizers that do not reach the dermis (95, 96), potentially demonstrating a requirement for migratory epidermal cells in the rapid initiation of T cell responses to topical sensitizers or irritants.
Langerhans cells have also emerged as key players in the activation of CD4+ T follicular helper cells (Tfh), which are required for germinal center formation and antibody affinity maturation in response to infection or vaccination (97). Here, ablation of LC results in clear defects in the formation of germinal centers and production of mature antibodies (92, 98). Importantly, this requirement for LC persists when antigen is delivered into the dermis and, therefore, should theoretically be preferentially acquired by dermal DC (97, 99). By contrast, an alternative study demonstrated that loss of LC led to increased production of autoantibodies in a murine model of lupus dermatitis (100), implying that, under steady state conditions, LC may also regulate activation of Tfh, either directly or indirectly via Treg. However, dermal DC also prime Tfh (101), indicating that the need to generate antibody responses to skin antigens is not a sufficient functional pressure to explain the acquisition of migratory function by LC.
In conclusion we argue that, while significant progress has been made in our understanding of the key role LC play in cutaneous immunity, we now need to shift our focus from LN to the skin. Precise definition of the sites of interaction between LC and T cells, or other cells, is needed to determine the selective pressures that drive the relative tissue-resident or migratory DC-like functions of these unique cells.
Author Contributions
HW and CB formulated opinions and concepts for the mini-review and wrote it together.
Conflict of Interest Statement
The authors declare that the research was conducted in the absence of any commercial or financial relationships that could be construed as a potential conflict of interest.
Acknowledgments
The authors thank Simon Yona and Amit Patel for helpful comments on the manuscript.
Funding
The Bennett lab is supported by funding from Bloodwise (12006), the BBSRC (BB/L001608/1), and the Royal Free charity. HW is funded by an MRC Ph.D. studentship.
References
1. Mass E, Ballesteros I, Farlik M, Halbritter F, Günther P, Crozet L, et al. Specification of tissue-resident macrophages during organogenesis. Science (2016) 353(6304):aaf4238. doi:10.1126/science.aaf4238
2. Lovy J, Savidant GP, Speare DJ, Wright GM. Langerin/CD207 positive dendritic-like cells in the haemopoietic tissues of salmonids. Fish Shellfish Immunol (2009) 27(2):365–8. doi:10.1016/j.fsi.2009.01.006
3. Carpentier S, Vu Manh TP, Chelbi R, Henri S, Malissen B, Haniffa M, et al. Comparative genomics analysis of mononuclear phagocyte subsets confirms homology between lymphoid tissue-resident and dermal XCR1(+) DCs in mouse and human and distinguishes them from Langerhans cells. J Immunol Methods (2016) 432:35–49. doi:10.1016/j.jim.2016.02.023
4. Bennett CL, Chakraverty R. Dendritic cells in tissues: in situ stimulation of immunity and immunopathology. Trends Immunol (2012) 33(1):8–13. doi:10.1016/j.it.2011.09.008
5. Romani N, Brunner PM, Stingl G. Changing views of the role of Langerhans cells. J Invest Dermatol (2012) 132(3 Pt 2):872–81. doi:10.1038/jid.2011.437
6. Idoyaga J, Lubkin A, Fiorese C, Lahoud MH, Caminschi I, Huang Y, et al. Comparable T helper 1 (Th1) and CD8 T-cell immunity by targeting HIV gag p24 to CD8 dendritic cells within antibodies to Langerin, DEC205, and Clec9A. Proc Natl Acad Sci U S A (2011) 108(6):2384–9. doi:10.1073/pnas.1019547108
7. Niess JH, Brand S, Gu X, Landsman L, Jung S, McCormick BA, et al. CX3CR1-mediated dendritic cell access to the intestinal lumen and bacterial clearance. Science (2005) 307(5707):254–8. doi:10.1126/science.1102901
8. Rescigno M, Urbano M, Valzasina B, Francolini M, Rotta G, Bonasio R, et al. Dendritic cells express tight junction proteins and penetrate gut epithelial monolayers to sample bacteria. Nat Immunol (2001) 2(4):361–7. doi:10.1038/86373
9. Davalos D, Grutzendler J, Yang G, Kim JV, Zuo Y, Jung S, et al. ATP mediates rapid microglial response to local brain injury in vivo. Nat Neurosci (2005) 8(6):752–8. doi:10.1038/nn1472
10. Nimmerjahn A, Kirchhoff F, Helmchen F. Resting microglial cells are highly dynamic surveillants of brain parenchyma in vivo. Science (2005) 308(5726):1314–8. doi:10.1126/science.1110647
11. Wake H, Moorhouse AJ, Jinno S, Kohsaka S, Nabekura J. Resting microglia directly monitor the functional state of synapses in vivo and determine the fate of ischemic terminals. J Neurosci (2009) 29(13):3974–80. doi:10.1523/JNEUROSCI.4363-08.2009
12. Paolicelli RC, Bolasco G, Pagani F, Maggi L, Scianni M, Panzanelli P, et al. Synaptic pruning by microglia is necessary for normal brain development. Science (2011) 333(6048):1456–8. doi:10.1126/science.1202529
13. Schafer DP, Lehrman EK, Kautzman AG, Koyama R, Mardinly AR, Yamasaki R, et al. Microglia sculpt postnatal neural circuits in an activity and complement-dependent manner. Neuron (2012) 74(4):691–705. doi:10.1016/j.neuron.2012.03.026
14. Tremblay ME, Lowery RL, Majewska AK. Microglial interactions with synapses are modulated by visual experience. PLoS Biol (2010) 8(11):e1000527. doi:10.1371/journal.pbio.1000527
15. Nishibu A, Ward BR, Jester JV, Ploegh HL, Boes M, Takashima A. Behavioral responses of epidermal Langerhans cells in situ to local pathological stimuli. J Invest Dermatol (2006) 126(4):787–96. doi:10.1038/sj.jid.5700107
16. Kubo A, Nagao K, Yokouchi M, Sasaki H, Amagai M. External antigen uptake by Langerhans cells with reorganization of epidermal tight junction barriers. J Exp Med (2009) 206(13):2937–46. doi:10.1084/jem.20091527
17. Cunningham AL, Abendroth A, Jones C, Nasr N, Turville S. Viruses and Langerhans cells. Immunol Cell Biol (2010) 88(4):416–23. doi:10.1038/icb.2010.42
18. Cunningham AL, Carbone F, Geijtenbeek TB. Langerhans cells and viral immunity. Eur J Immunol (2008) 38(9):2377–85. doi:10.1002/eji.200838521
19. Hertoghs N, Geijtenbeek TBH, Ribeiro CMS. Interplay between HIV-1 innate sensing and restriction in mucosal dendritic cells: balancing defense and viral transmission. Curr Opin Virol (2017) 22:112–9. doi:10.1016/j.coviro.2017.01.001
20. van der Aar AMG, Picavet DI, Muller FJ, de Boer L, van Capel TM, Zaat SA, et al. Langerhans cells favor skin flora tolerance through limited presentation of bacterial antigens and induction of regulatory T cells. J Invest Dermatol (2013) 133(5):1240–9. doi:10.1038/jid.2012.500
21. van der Aar AM, de Groot R, Sanchez-Hernandez M, Taanman EW, van Lier RA, Teunissen MB, et al. Cutting edge: virus selectively primes human Langerhans cells for CD70 expression promoting CD8+ T cell responses. J Immunol (2011) 187(7):3488–92. doi:10.4049/jimmunol.1101105
22. Allan RS, Smith CM, Belz GT, van Lint AL, Wakim LM, Heath WR, et al. Epidermal viral immunity induced by CD8alpha+ dendritic cells but not by Langerhans cells. Science (2003) 301(5641):1925–8. doi:10.1126/science.1087576
23. Bedoui S, Whitney PG, Waithman J, Eidsmo L, Wakim L, Caminschi I, et al. Cross-presentation of viral and self antigens by skin-derived CD103+ dendritic cells. Nat Immunol (2009) 10(5):488–95. doi:10.1038/ni.1724
24. Kawamura T, Koyanagi Y, Nakamura Y, Ogawa Y, Yamashita A, Iwamoto T, et al. Significant virus replication in Langerhans cells following application of HIV to abraded skin: relevance to occupational transmission of HIV. J Immunol (2008) 180(5):3297–304. doi:10.4049/jimmunol.180.5.3297
25. de Witte L, Nabatov A, Pion M, Fluitsma D, de Jong MA, de Gruijl T, et al. Langerin is a natural barrier to HIV-1 transmission by Langerhans cells. Nat Med (2007) 13(3):367–71. doi:10.1038/nm1541
26. Ribeiro CM, Sarrami-Forooshani R, Setiawan LC, Zijlstra-Willems EM, van Hamme JL, Tigchelaar W, et al. Receptor usage dictates HIV-1 restriction by human TRIM5alpha in dendritic cell subsets. Nature (2016) 540(7633):448–52. doi:10.1038/nature20567
27. Mayr L, Su B, Moog C. Langerhans cells: the ‘Yin and Yang’ of HIV restriction and transmission. Trends Microbiol (2017) 25(3):170–2. doi:10.1016/j.tim.2017.01.009
28. Antonucci JM, St Gelais C, Wu L. The dynamic interplay between HIV-1, SAMHD1, and the innate antiviral response. Front Immunol (2017) 8:1541. doi:10.3389/fimmu.2017.01541
29. Cline TD, Beck D, Bianchini E. Influenza virus replication in macrophages: balancing protection and pathogenesis. J Gen Virol (2017) 98(10):2401–12. doi:10.1099/jgv.0.000922
30. Ramirez-Ortiz ZG, Pendergraft WF III, Prasad A, Byrne MH, Iram T, Blanchette CJ, et al. The scavenger receptor SCARF1 mediates the clearance of apoptotic cells and prevents autoimmunity. Nat Immunol (2013) 14(9):917–26. doi:10.1038/ni.2670
31. Cummings RJ, Barbet G, Bongers G, Hartmann BM, Gettler K, Muniz L, et al. Different tissue phagocytes sample apoptotic cells to direct distinct homeostasis programs. Nature (2016) 539(7630):565–9. doi:10.1038/nature20138
32. Lemke G, Rothlin CV. Immunobiology of the TAM receptors. Nat Rev Immunol (2008) 8(5):327–36. doi:10.1038/nri2303
33. Hatakeyama M, Fukunaga A, Washio K, Taguchi K, Oda Y, Ogura K, et al. Anti-inflammatory role of Langerhans cells and apoptotic keratinocytes in ultraviolet-B-induced cutaneous inflammation. J Immunol (2017) 199(8):2937–47. doi:10.4049/jimmunol.1601681
34. Bauer T, Zagórska A, Jurkin J, Yasmin N, Köffel R, Richter S, et al. Identification of Axl as a downstream effector of TGF-beta1 during Langerhans cell differentiation and epidermal homeostasis. J Exp Med (2012) 209(11):2033–47. doi:10.1084/jem.20120493
35. Denning TL, Wang YC, Patel SR, Williams IR, Pulendran B. Lamina propria macrophages and dendritic cells differentially induce regulatory and interleukin 17-producing T cell responses. Nat Immunol (2007) 8(10):1086–94. doi:10.1038/ni1511
36. Hadis U, Wahl B, Schulz O, Hardtke-Wolenski M, Schippers A, Wagner N, et al. Intestinal tolerance requires gut homing and expansion of FoxP3+ regulatory T cells in the lamina propria. Immunity (2011) 34(2):237–46. doi:10.1016/j.immuni.2011.01.016
37. Soroosh P, Doherty TA, Duan W, Mehta AK, Choi H, Adams YF, et al. Lung-resident tissue macrophages generate Foxp3+ regulatory T cells and promote airway tolerance. J Exp Med (2013) 210(4):775–88. doi:10.1084/jem.20121849
38. Coleman MM, Ruane D, Moran B, Dunne PJ, Keane J, Mills KH. Alveolar macrophages contribute to respiratory tolerance by inducing FoxP3 expression in naive T cells. Am J Respir Cell Mol Biol (2013) 48(6):773–80. doi:10.1165/rcmb.2012-0263OC
39. Erhardt A, Biburger M, Papadopoulos T, Tiegs G. IL-10, regulatory T cells, and Kupffer cells mediate tolerance in concanavalin A-induced liver injury in mice. Hepatology (2007) 45(2):475–85. doi:10.1002/hep.21498
40. Breous E, Somanathan S, Vandenberghe LH, Wilson JM. Hepatic regulatory T cells and Kupffer cells are crucial mediators of systemic T cell tolerance to antigens targeting murine liver. Hepatology (2009) 50(2):612–21. doi:10.1002/hep.23043
41. Heymann F, Peusquens J, Ludwig-Portugall I, Kohlhepp M, Ergen C, Niemietz P, et al. Liver inflammation abrogates immunological tolerance induced by Kupffer cells. Hepatology (2015) 62(1):279–91. doi:10.1002/hep.27793
42. Ebner F, Brandt C, Thiele P, Richter D, Schliesser U, Siffrin V, et al. Microglial activation milieu controls regulatory T cell responses. J Immunol (2013) 191(11):5594–602. doi:10.4049/jimmunol.1203331
43. Wu C, Rauch U, Korpos E, Song J, Loser K, Crocker PR, et al. Sialoadhesin-positive macrophages bind regulatory T cells, negatively controlling their expansion and autoimmune disease progression. J Immunol (2009) 182(10):6508–16. doi:10.4049/jimmunol.0804247
44. Price JG, Idoyaga J, Salmon H, Hogstad B, Bigarella CL, Ghaffari S, et al. CDKN1A regulates Langerhans cell survival and promotes Treg cell generation upon exposure to ionizing irradiation. Nat Immunol (2015) 16(10):1060–8. doi:10.1038/ni.3270
45. Kautz-Neu K, Noordegraaf M, Dinges S, Bennett CL, John D, Clausen BE, et al. Langerhans cells are negative regulators of the anti-Leishmania response. J Exp Med (2011) 208(5):885–91. doi:10.1084/jem.20102318
46. Gomez de Agüero M, Vocanson M, Hacini-Rachinel F, Taillardet M, Sparwasser T, Kissenpfennig A, et al. Langerhans cells protect from allergic contact dermatitis in mice by tolerizing CD8(+) T cells and activating Foxp3(+) regulatory T cells. J Clin Invest (2012) 122(5):1700–11. doi:10.1172/JCI59725
47. Igyarto BZ, Jenison MC, Dudda JC, Roers A, Müller W, Koni PA, et al. Langerhans cells suppress contact hypersensitivity responses via cognate CD4 interaction and Langerhans cell-derived IL-10. J Immunol (2009) 183(8):5085–93. doi:10.4049/jimmunol.0901884
48. Seneschal J, Clark RA, Gehad A, Baecher-Allan CM, Kupper TS. Human epidermal Langerhans cells maintain immune homeostasis in skin by activating skin resident regulatory T cells. Immunity (2012) 36(5):873–84. doi:10.1016/j.immuni.2012.03.018
49. Yoshiki R, Kabashima K, Sakabe J, Sugita K, Bito T, Nakamura M, et al. The mandatory role of IL-10-producing and OX40 ligand-expressing mature Langerhans cells in local UVB-induced immunosuppression. J Immunol (2010) 184(10):5670–7. doi:10.4049/jimmunol.0903254
50. Loser K, Mehling A, Loeser S, Apelt J, Kuhn A, Grabbe S, et al. Epidermal RANKL controls regulatory T-cell numbers via activation of dendritic cells. Nat Med (2006) 12(12):1372–9. doi:10.1038/nm1518
51. Nakahashi-Oda C, Udayanga KG, Nakamura Y, Nakazawa Y, Totsuka N, Miki H, et al. Apoptotic epithelial cells control the abundance of Treg cells at barrier surfaces. Nat Immunol (2016) 17(4):441–50. doi:10.1038/ni.3345
52. Liu Z, Gerner MY, Van Panhuys N, Levine AG, Rudensky AY, Germain RN. Immune homeostasis enforced by co-localized effector and regulatory T cells. Nature (2015) 528(7581):225–30. doi:10.1038/nature16169
53. Azukizawa H, Döhler A, Kanazawa N, Nayak A, Lipp M, Malissen B, et al. Steady state migratory RelB+ langerin+ dermal dendritic cells mediate peripheral induction of antigen-specific CD4+ CD25+ Foxp3+ regulatory T cells. Eur J Immunol (2011) 41(5):1420–34. doi:10.1002/eji.201040930
54. Kissenpfennig A, Henri S, Dubois B, Laplace-Builhé C, Perrin P, Romani N, et al. Dynamics and function of Langerhans cells in vivo: dermal dendritic cells colonize lymph node areas distinct from slower migrating Langerhans cells. Immunity (2005) 22(5):643–54. doi:10.1016/j.immuni.2005.04.004
55. Strandt H, Pinheiro DF, Kaplan DH, Wirth D, Gratz IK, Hammerl P, et al. Neoantigen expression in steady-state Langerhans cells induces CTL tolerance. J Immunol (2017) 199(5):1626–34. doi:10.4049/jimmunol.1602098
56. Honda T, Egen JG, Lämmermann T, Kastenmüller W, Torabi-Parizi P, Germain RN. Tuning of antigen sensitivity by T cell receptor-dependent negative feedback controls T cell effector function in inflamed tissues. Immunity (2014) 40(2):235–47. doi:10.1016/j.immuni.2013.11.017
57. Bennett CL, Fallah-Arani F, Conlan T, Trouillet C, Goold H, Chorro L, et al. Langerhans cells regulate cutaneous injury by licensing CD8 effector cells recruited to the skin. Blood (2011) 117(26):7063–9. doi:10.1182/blood-2011-01-329185
58. Merad M, Hoffmann P, Ranheim E, Slaymaker S, Manz MG, Lira SA, et al. Depletion of host Langerhans cells before transplantation of donor alloreactive T cells prevents skin graft-versus-host disease. Nat Med (2004) 10(5):510–7. doi:10.1038/nm1038
59. Li H, Kaplan DH, Matte-Martone C, Tan HS, Venkatesan S, Johnson K, et al. Langerhans cells are not required for graft-versus-host disease. Blood (2011) 117(2):697–707. doi:10.1182/blood-2010-07-299073
60. Bennett CL, van Rijn E, Jung S, Inaba K, Steinman RM, Kapsenberg ML, et al. Inducible ablation of mouse Langerhans cells diminishes but fails to abrogate contact hypersensitivity. J Cell Biol (2005) 169(4):569–76. doi:10.1083/jcb.200501071
61. Chakraverty R, Côté D, Buchli J, Cotter P, Hsu R, Zhao G, et al. An inflammatory checkpoint regulates recruitment of graft-versus-host reactive T cells to peripheral tissues. J Exp Med (2006) 203(8):2021–31. doi:10.1084/jem.20060376
62. DuPage M, Bluestone JA. Harnessing the plasticity of CD4(+) T cells to treat immune-mediated disease. Nat Rev Immunol (2016) 16(3):149–63. doi:10.1038/nri.2015.18
63. Egan PJ, van Nieuwenhuijze A, Campbell IK, Wicks IP. Promotion of the local differentiation of murine Th17 cells by synovial macrophages during acute inflammatory arthritis. Arthritis Rheum (2008) 58(12):3720–9. doi:10.1002/art.24075
64. Allam JP, Duan Y, Heinemann F, Winter J, Götz W, Deschner J, et al. IL-23-producing CD68(+) macrophage-like cells predominate within an IL-17-polarized infiltrate in chronic periodontitis lesions. J Clin Periodontol (2011) 38(10):879–86. doi:10.1111/j.1600-051X.2011.01752.x
65. Arnold IC, Mathisen S, Schulthess J, Danne C, Hegazy AN, Powrie F. CD11c(+) monocyte/macrophages promote chronic Helicobacter hepaticus-induced intestinal inflammation through the production of IL-23. Mucosal Immunol (2016) 9(2):352–63. doi:10.1038/mi.2015.65
66. Foucher ED, Blanchard S, Preisser L, Descamps P, Ifrah N, Delneste Y, et al. IL-34- and M-CSF-induced macrophages switch memory T cells into Th17 cells via membrane IL-1alpha. Eur J Immunol (2015) 45(4):1092–102. doi:10.1002/eji.201444606
67. Segura E, Touzot M, Bohineust A, Cappuccio A, Chiocchia G, Hosmalin A, et al. Human inflammatory dendritic cells induce Th17 cell differentiation. Immunity (2013) 38(2):336–48. doi:10.1016/j.immuni.2012.10.018
68. Aliahmadi E, Gramlich R, Grützkau A, Hitzler M, Krüger M, Baumgrass R, et al. TLR2-activated human Langerhans cells promote Th17 polarization via IL-1beta, TGF-beta and IL-23. Eur J Immunol (2009) 39(5):1221–30. doi:10.1002/eji.200838742
69. Fujita H, Nograles KE, Kikuchi T, Gonzalez J, Carucci JA, Krueger JG. Human Langerhans cells induce distinct IL-22-producing CD4+ T cells lacking IL-17 production. Proc Natl Acad Sci U S A (2009) 106(51):21795–800. doi:10.1073/pnas.0911472106
70. Mathers AR, Janelsins BM, Rubin JP, Tkacheva OA, Shufesky WJ, Watkins SC, et al. Differential capability of human cutaneous dendritic cell subsets to initiate Th17 responses. J Immunol (2009) 182(2):921–33. doi:10.4049/jimmunol.182.2.921
71. Igyártó BZ, Haley K, Ortner D, Bobr A, Gerami-Nejad M, Edelson BT, et al. Skin-resident murine dendritic cell subsets promote distinct and opposing antigen-specific T helper cell responses. Immunity (2011) 35(2):260–72. doi:10.1016/j.immuni.2011.06.005
72. Kashem SW, Igyarto BZ, Gerami-Nejad M, Kumamoto Y, Mohammed JA, Jarrett E, et al. Candida albicans morphology and dendritic cell subsets determine T helper cell differentiation. Immunity (2015) 42(2):356–66. doi:10.1016/j.immuni.2015.01.008
73. Kobayashi T, Glatz M, Horiuchi K, Kawasaki H, Akiyama H, Kaplan DH, et al. Dysbiosis and Staphylococcus aureus colonization drives inflammation in atopic dermatitis. Immunity (2015) 42(4):756–66. doi:10.1016/j.immuni.2015.03.014
74. Wohn C, Ober-Blöbaum JL, Haak S, Pantelyushin S, Cheong C, Zahner SP, et al. Langerin(neg) conventional dendritic cells produce IL-23 to drive psoriatic plaque formation in mice. Proc Natl Acad Sci U S A (2013) 110(26):10723–8. doi:10.1073/pnas.1307569110
75. Yoshiki R, Kabashima K, Honda T, Nakamizo S, Sawada Y, Sugita K, et al. IL-23 from Langerhans cells is required for the development of imiquimod-induced psoriasis-like dermatitis by induction of IL-17A-producing gammadelta T cells. J Invest Dermatol (2014) 134(7):1912–21. doi:10.1038/jid.2014.98
76. Clark RA. Resident memory T cells in human health and disease. Sci Transl Med (2015) 7(269):269rv1. doi:10.1126/scitranslmed.3010641
77. Iijima N, Iwasaki A. T cell memory. A local macrophage chemokine network sustains protective tissue-resident memory CD4 T cells. Science (2014) 346(6205):93–8. doi:10.1126/science.1257530
78. Zaid A, Mackay LK, Rahimpour A, Braun A, Veldhoen M, Carbone FR, et al. Persistence of skin-resident memory T cells within an epidermal niche. Proc Natl Acad Sci U S A (2014) 111(14):5307–12. doi:10.1073/pnas.1322292111
79. Mohammed J, Beura LK, Bobr A, Astry B, Chicoine B, Kashem SW, et al. Stromal cells control the epithelial residence of DCs and memory T cells by regulated activation of TGF-beta. Nat Immunol (2016) 17(4):414–21. doi:10.1038/ni.3396
80. Cheuk S, Wikén M, Blomqvist L, Nylén S, Talme T, Ståhle M, et al. Epidermal Th22 and Tc17 cells form a localized disease memory in clinically healed psoriasis. J Immunol (2014) 192(7):3111–20. doi:10.4049/jimmunol.1302313
81. Cheuk S, Schlums H, Gallais Sérézal I, Martini E, Chiang SC, Marquardt N, et al. CD49a expression defines tissue-resident CD8+ T cells poised for cytotoxic function in human skin. Immunity (2017) 46(2):287–300. doi:10.1016/j.immuni.2017.01.009
82. Miller JC, Brown BD, Shay T, Gautier EL, Jojic V, Cohain A, et al. Deciphering the transcriptional network of the dendritic cell lineage. Nat Immunol (2012) 13(9):888–99. doi:10.1038/ni.2370
83. Boes M, Cerny J, Massol R, Op den Brouw M, Kirchhausen T, Chen J, et al. T-cell engagement of dendritic cells rapidly rearranges MHC class II transport. Nature (2002) 418(6901):983–8. doi:10.1038/nature01004
84. Okabe Y, Medzhitov R. Tissue-specific signals control reversible program of localization and functional polarization of macrophages. Cell (2014) 157(4):832–44. doi:10.1016/j.cell.2014.04.016
85. Epelman S, Lavine KJ, Randolph GJ. Origin and functions of tissue macrophages. Immunity (2014) 41(1):21–35. doi:10.1016/j.immuni.2014.06.013
86. Bobr A, Igyarto BZ, Haley KM, Li MO, Flavell RA, Kaplan DH. Autocrine/paracrine TGF-β1 inhibits Langerhans cell migration. Proc Natl Acad Sci U S A (2012) 109(26):10492–7. doi:10.1073/pnas.1119178109
87. Tang A, Amagai M, Granger LG, Stanley JR, Udey MC. Adhesion of epidermal Langerhans cells to keratinocytes mediated by E-cadherin. Nature (1993) 361(6407):82–5. doi:10.1038/361082a0
88. Ratzinger G, Stoitzner P, Ebner S, Lutz MB, Layton GT, Rainer C, et al. Matrix metalloproteinases 9 and 2 are necessary for the migration of Langerhans cells and dermal dendritic cells from human and murine skin. J Immunol (2002) 168(9):4361–71. doi:10.4049/jimmunol.168.9.4361
89. Ohl L, Mohaupt M, Czeloth N, Hintzen G, Kiafard Z, Zwirner J, et al. CCR7 governs skin dendritic cell migration under inflammatory and steady-state conditions. Immunity (2004) 21(2):279–88. doi:10.1016/j.immuni.2004.06.014
90. Tal O, Lim HY, Gurevich I, Milo I, Shipony Z, Ng LG, et al. DC mobilization from the skin requires docking to immobilized CCL21 on lymphatic endothelium and intralymphatic crawling. J Exp Med (2011) 208(10):2141–53. doi:10.1084/jem.20102392
91. Shklovskaya E, Roediger B, Fazekas de St Groth B. Epidermal and dermal dendritic cells display differential activation and migratory behavior while sharing the ability to stimulate CD4+ T cell proliferation in vivo. J Immunol (2008) 181(1):418–30. doi:10.4049/jimmunol.181.1.418
92. Ouchi T, Kubo A, Yokouchi M, Adachi T, Kobayashi T, Kitashima DY, et al. Langerhans cell antigen capture through tight junctions confers preemptive immunity in experimental staphylococcal scalded skin syndrome. J Exp Med (2011) 208(13):2607–13. doi:10.1084/jem.20111718
93. de Jong A, Peña-Cruz V, Cheng TY, Clark RA, Van Rhijn I, Moody DB. CD1a-autoreactive T cells are a normal component of the human alphabeta T cell repertoire. Nat Immunol (2010) 11(12):1102–9. doi:10.1038/ni.1956
94. Kim JH, Hu Y, Yongqing T, Kim J, Hughes VA, Le Nours J, et al. CD1a on Langerhans cells controls inflammatory skin disease. Nat Immunol (2016) 17(10):1159–66. doi:10.1038/ni.3523
95. Bennett CL, Noordegraaf M, Martina CA, Clausen BE. Langerhans cells are required for efficient presentation of topically applied hapten to T cells. J Immunol (2007) 179(10):6830–5. doi:10.4049/jimmunol.179.10.6830
96. Noordegraaf M, Flacher V, Stoitzner P, Clausen BE. Functional redundancy of Langerhans cells and Langerin+ dermal dendritic cells in contact hypersensitivity. J Invest Dermatol (2010) 130(12):2752–9. doi:10.1038/jid.2010.223
97. Crotty S. T follicular helper cell differentiation, function, and roles in disease. Immunity (2014) 41(4):529–42. doi:10.1016/j.immuni.2014.10.004
98. Zimara N, Florian C, Schmid M, Malissen B, Kissenpfennig A, Männel DN, et al. Langerhans cells promote early germinal center formation in response to Leishmania-derived cutaneous antigens. Eur J Immunol (2014) 44(10):2955–67. doi:10.1002/eji.201344263
99. Levin C, Bonduelle O, Nuttens C, Primard C, Verrier B, Boissonnas A, et al. Critical role for skin-derived migratory DCs and Langerhans cells in TFH and GC responses after intradermal immunization. J Invest Dermatol (2017) 137(9):1905–13. doi:10.1016/j.jid.2017.04.016
100. King JK, Philips RL, Eriksson AU, Kim PJ, Halder RC, Lee DJ, et al. Langerhans cells maintain local tissue tolerance in a model of systemic autoimmune disease. J Immunol (2015) 195(2):464–76. doi:10.4049/jimmunol.1402735
Keywords: Langerhans cells, skin, epidermis, macrophages, migration
Citation: West HC and Bennett CL (2018) Redefining the Role of Langerhans Cells As Immune Regulators within the Skin. Front. Immunol. 8:1941. doi: 10.3389/fimmu.2017.01941
Received: 17 October 2017; Accepted: 18 December 2017;
Published: 05 January 2018
Edited by:
Irina Caminschi, Monash University, AustraliaReviewed by:
Susan Kovats, Oklahoma Medical Research Foundation, United StatesMark Christopher Coles, University of Oxford, United Kingdom
Copyright: © 2018 West and Bennett. This is an open-access article distributed under the terms of the Creative Commons Attribution License (CC BY). The use, distribution or reproduction in other forums is permitted, provided the original author(s) or licensor are credited and that the original publication in this journal is cited, in accordance with accepted academic practice. No use, distribution or reproduction is permitted which does not comply with these terms.
*Correspondence: Heather C. West, aGVhdGhlci53ZXN0LjE0QHVjbC5hYy51aw==;
Clare L. Bennett, Yy5iZW5uZXR0QHVjbC5hYy51aw==