- 1Department of Biology, The Faculty of Natural Sciences, University of Haifa, Haifa, Israel
- 2Faculty of Dental Medicine, Institute for Dental Sciences, Hadassah Medical School, Hebrew University of Jerusalem, Jerusalem, Israel
The complete resolution of inflammation requires the uptake of apoptotic polymorphonuclear cells (PMN) by local macrophages (efferocytosis) and the consequent reprogramming of the engulfing phagocytes to reparative and pro-resolving phenotypes. The tyrosine kinase receptors TYRO3, AXL, and MERTK (collectively named TAM) are fundamental mediators in regulating inflammatory responses and efferocytosis. Protein S (PROS1) is a ligand for all TAM receptors that mediates various aspects of their activity. However, the involvement of PROS1 in the resolution of inflammation is incompletely understood. Here, we report the upregulation of Pros1 in macrophages during the resolution of inflammation. Selective knockout of Pros1 in the myeloid lineage significantly downregulated macrophage pro-resolving properties. Hence, Pros1-deficient macrophages engulfed fewer apoptotic PMN remnants in vivo, and exogenous PROS1 rescued impaired efferocytosis ex vivo. Moreover, Pros1-deficient peritoneal macrophages secreted higher levels of the pro-inflammatory mediators TNFα and CCL3, while they secreted lower levels of the reparative/anti-inflammatory IL-10 following exposure to lipopolysaccharide in comparison to their WT counterparts. Moreover, Pros1-deficient macrophages expressed less of the anti-inflammatory/pro-resolving enzymes arginase-1 and 12/15-lipoxygenase and produced less of the specialized pro-resolving mediator resolvin D1. Altogether, our results suggest that macrophage-derived PROS1 is an important effector molecule in regulating the efferocytosis, maturation, and reprogramming of resolution phase macrophages, and imply that PROS1 could provide a new therapeutic target for inflammatory and fibrotic disorders.
Introduction
Inflammation normally resolves in an active process that eliminates the inflammatory effector components that harm the host (1–4). This is hallmarked by leukocyte apoptosis and clearance by macrophages (5–8). Apoptotic cell (AC) engulfment by phagocytes is mediated by signals that are expressed on the surface of ACs and their corresponding receptors [reviewed in Refs. (7, 9)]. Efferocytosis leads to macrophage reprogramming/immune-silencing (5, 10–13) in response to bacterial moieties through specific kinases, such as p38 MAPK, JNK, and cAMP production (14, 15). Macrophage reprogramming is defined by a reduction in the release of pro-inflammatory cytokines and chemokines, concomitant with the production of TGFβ and IL-10 (16–18), cytokines that can promote resolution and wound repair. In addition, the uptake of ACs promotes the expression of 15-lipoxygenase (LO)-1, which is involved in the generation of pro-resolving lipid mediators by macrophages (19–21). A new resolution phase macrophage phenotype—distinguishable from either M1 or M2—and characterized by low expression of CD11b, is generated from the CD11bhigh phenotype upon the engulfment of threshold numbers of apoptotic polymorphonuclear cells (PMN) (21). This phenotypic conversion of macrophages results in significant immune-silencing in addition to the reduction in the expression of arginase-1, surface CD11b, and F4/80 (21). Specifically, CD11blow macrophages stop producing TNFα and IL-1β, but increase the production of TGFβ and the expression of 12/15-LO, and emigrate to the lymphatics (21).
Protein S (PROS1) is a secreted multifunctional glycoprotein encoded by the Pros1 gene and best known for its potent anticoagulant activity as a cofactor for activated protein C (22). Outside the coagulation system, PROS1 functions as an agonist for the TAM family of receptor tyrosine kinases, comprising TYRO3, AXL, and MERTK (23, 24), which are negative regulators of immunity (25–28). TAM receptors and their cognate ligands PROS1 and growth-arrest-specific-6 (GAS6) are expressed by immune cells, including macrophages and dendritic cells (DCs) (29, 30). This signaling axis dampens immune reactivity and contributes toward resolving inflammation through at least two distinct mechanisms: the molecular inhibition of the production and the secretion of pro-inflammatory cytokines and by the phagocytic clearance of ACs.
In macrophages, MERTK inhibits the production and secretion of the pro-inflammatory cytokine TNFα following lipopolysaccharide (LPS) exposure (31), while unchallenged macrophages isolated from TAM triple-mutant mice express aberrantly high levels of MHC class II molecules and IL-12 (32). The role of PROS1 as a TAM agonist in vivo was demonstrated in the phagocytic clearance of photoreceptor outer segments by retinal-pigment epithelial cells (33) and in T-cell–DC immune interaction (29). However, its role in macrophage-mediated resolution of inflammation has not been investigated.
To test the physiological role of PROS1 in resolution phase macrophages, we generated mice genetically ablated for Pros1 expression in the myeloid lineage and assessed macrophage efferocytosis and reprogramming in a model of zymosan A-induced peritonitis. Here, we report that Pros1 is produced by resolution phase macrophages and promotes key features of these macrophages. We show that in vivo efferocytosis is compromised in PROS1-deficient macrophages. Exogenous PROS1 was able to rescue the engulfment of ACs ex vivo. We also show that PROS1 ablation attenuates macrophage conversion toward anti-inflammatory/reparative phenotypes, as determined by their cytokine secretion balance. Moreover, PROS1 deficiency resulted in the hampered expression of the pro-resolving enzymes arginase-1 and 12/15-LO, as well as the latter’s product, resolvin D1 (RvD1). Hence, PROS1 is a key mediator in successful resolution of inflammation.
Materials and Methods
Reagents
ELISA kits for mouse TNFα, IL-10, and IL-6 were obtained from Biolegend; a mouse CCL3 detection kit was obtained from R&D Systems. LPS (from Escherichia coli, clone 055:B5) and zymosan A (from Saccharomyces cerevisiae) were purchased from Sigma-Aldrich (St. Louis, MI, USA). Docosahexaenoic acid (DHA) was purchased from Cayman Chemicals.
Murine Peritonitis
Male C57BL/6 LysMCre/+; Pros1fl/fl and Pros1fl/fl mice (8–10 week old), were injected intra-peritoneally (i.p.) with freshly prepared zymosan A (1 mg/25 g body weight; Sigma-Aldrich) from S. cerevisiae in sterile PBS or left unchallenged. After 24, 48, and 66 hrs, mice were euthanized and peritoneal exudates were collected by lavage with 5 ml of sterile PBS. All animal experiments were approved by the Hebrew University—Hadassah ethics committee.
Macrophages Isolation
Peritoneal exudates were recovered with 5 ml PBS and centrifuged (1300 rpm for 5 min). Then, cells and cell-free peritoneal fluids were separated. Peritoneal macrophages were isolated from exudate cells by EasySep™ mouse PE-positive selection magnetic beads kit (StemCell Technologies) using PE anti-mouse F4/80 antibody (#123110, Biolegend). Isolated macrophages were used for RT-qPCR, microscopic analysis, and ex vivo stimulation experiments.
Quantitative Real-time PCR (qPCR)
Isolated macrophages were harvested, washed once with PBS, and their RNA content was isolated with TRIZOL (Sigma-Aldrich). cDNA was synthesized with qScript cDNA synthesis kit (Quanta Biosciences). Real-time PCR reactions were performed in triplicates using KAPA SYBR FAST qPCR Kit (KAPA-Biosystems) following the manufacturer’s instructions on a CFX96 Real-Time PCR Cycler (Bio-Rad). The reactions were normalized to mGapdh using the ΔΔ threshold cycle (Ct) method. Specificity of the primers was confirmed by dissociation curves. Mouse primer sequences were as follows: mPros1 Forward 5'-TTC CGT GTT GGC TCA TTC C-3'; mPros1 Reverse 5'-TTG GTC TGA GAT GGC TTT GAC A-3', mGapdh-Forward 5'-AGT TGG GAT AGG GCC TCT CTT-3', and mGapdh-Reverse 5'-TCC CAC TCT TCC ACC TTC GA-3'.
In Vivo Engulfment Assay
Peritoneal macrophages were isolated at the indicated time points and plated (2 × 105 cells/well) onto eight-well chamber glass slides for 2 h at 37°C in RPMI 1640 (Gibco) to allow adhesion. Then, the cells were rinsed briefly with PBS, fixed for 15 min with 4% paraformaldehyde (PFA) containing 5% sucrose in PBS, and washed twice in PBS. Fixed cells were incubated overnight at 4°C with phalloidin CF488A conjugate (5 units/ml, for F-actin, Biotium). Then, the cells were washed twice with PBS, stained with Hoechst (20 µg/ml for nuclear DNA, Invitrogen H3570) for 5 min, and washed thoroughly, but gently. Mounted slides were kept in the dark at 4°C until analyzed. Macrophages and engulfed apoptotic remnants were enumerated under a confocal fluorescent microscope (Zeiss) as previously described (21). Briefly, macrophages from five to eight fields per chamber (approx. 200 cells) were analyzed per mouse, and the average number of neutrophils engulfed per macrophage, as well as the number of macrophages that have actively engulfed apoptotic moieties, was calculated. Engulfed nuclear material was identified by a spherical bright nuclear Hoechst staining of 1–10 µm in diameter. Then, phagocytic efficiency was calculated as in Ref. (34). Briefly, the phagocytic efficiency index was calculated based on a weighted average of ingested apoptotic DNA-containing particles per macrophage and the number of macrophages containing a certain number of such particles. Phagocytic efficiency (%) = [(1 × X(1) + 2 × X(2) + 3 × X(3) … + n × X(n))/total number of macrophages] × 100. X(n) represents the number of macrophages containing n apoptotic particles (n = 1, 2, 3, …, up to a maximum of six points for more than five apoptotic particles ingested per macrophage).
In Vitro Engulfment of ACs
Jurkat cells (10 × 106) were induced for apoptosis with staurosporine (Sigma-Aldrich, 1 µM/106 cells in 1 ml RPMI medium containing pen/strep/glutamine and 10% FBS) for 5 h. Cells were washed three times in PBS, labeled with CypHer5E (GE Healthcare; 1 µl CypHer5E/1 ml serum-free medium) for 30 min, and washed twice with PBS. Peritoneal macrophages were isolated as described, and 150 × 103 cells were plated on an eight-well glass chamber slide (Nunc) and fed with 750 × 103 pre-labeled apoptotic Jurkat cells for 4 h in a total of 150-µl medium with or without soluble PROS1 (25 nM; from Enzyme Research Laboratories). Next, the medium was aspirated, and bound cells were washed gently with PBS. Adherent cells were subsequently fixed in 200 µl of 4% PFA; 5% sucrose for 15 min. Cells were washed in PBS and incubated with 200 µl phalloidin (5 U/ml, CF488A conjugate, Biotium) at 4°C overnight. Then, cells were washed three times with PBS (10 min each) and stained in 200 µl of Hoechst 3570 (20 µg/ml, Invitrogen) for 5 min, and rinsed with PBS. The chambers were removed, mounted with Fluoromount G, and visualized under a Nikon A1 confocal microscope. The number of CypHer5E+ engulfed ACs per macrophage was scored.
Cytokine and Chemokine Secretion Ex Vivo
Peritoneal macrophages were isolated using PE selection magnetic beads (StemCell Technologies) and incubated (5 × 106 cells in 5 ml of culture media) with LPS (1 µg/ml) or vehicle in RPMI 1640 under a humidified 5% CO2 atmosphere at 37°C. After 24 h, the supernatants were collected, and their TNFα, IL-6, CCL3, and IL-10 contents were determined by standard ELISA (Biolegend kits for TNFα, IL-6, and IL-10 and R&D Systems kit for CCL3).
Western Blot Analysis
Protein extracts from equal volumes of peritoneal fluids or equal total protein content of isolated macrophages were subjected to SDS-PAGE using 10% polyacrylamide gels, transferred (1 h, 15 V) to PVDF membranes (Bio-Rad), and blocked for 1 h with 5% BSA in TBST (0.1% Tween 20 in Tris-buffered saline). Then, membranes were immuno-blotted overnight at 4°C with either goat anti-mouse CD11b (M-19, 1:200, SantaCruz Biotechnology), goat anti-mouse arginase-1 (ab60176, 1:20,000, Abcam), rabbit anti-mouse 12/15-LO (160704, 1:1,000, Cayman Chemical), rabbit anti-mouse PROS1 (AB15928, 1:1,000, Merck Millipore), and goat anti-mouse actin (I-19, 1:500, SantaCruz Biotechnology). Then, the membranes were washed three times with TBST and incubated with the appropriate HRP-conjugated secondary antibodies (1:10,000, 1 h, room temperature, Jackson ImmunoResearch). Blots were washed and developed using the EZ-ECL (Biological Industries) chemiluminescence kit and analyzed using the LAS-4000 luminescent image analyzer (Fujifilm) and the TotalLab TL-100 software (Nonlinear Dynamics). Band densitometric intensities among different samples were normalized to actin.
RvD1 Quantification
Peritoneal macrophages were isolated 66 h post peritonitis initiation from the indicated mice; 106 cells were resuspended in 1-ml medium and immediately supplemented with DHA (20 µM, Cayman Chemicals) for 4 h. Then, the incubation was stopped with cold MeOH, the supernatants were collected in a glass tube, and the MeOH was allowed to evaporate completely; 5 ml of ddH2O and 200 µl of MeOH (pH 3.5) were added to the tubes and the samples were loaded through activated Sep-pak Vac 6cc (500 mg) C18 Cartridges (Waters, WAT043395) allowing for MeOH-activated lipid binding. Next, the bound lipids were released using methyl formate, which was then evaporated completely while adding small amounts of MeOH under nitrogen flow. Finally, RvD1 content in lipid-extracted samples was determined using a commercial ELISA kit (Cayman Chemicals), according to the manufacturer’s instructions.
Statistical Analysis
Experiments were repeated at least three times with at least three replicates per experiment. Results were analyzed by two-way analysis of variance (for multiple groups) or Student’s t-test (for comparison between two groups), unless otherwise mentioned. P-values (P), *P ≤ 0.05, **P ≤ 0.01, and ***P ≤ 0.001, were considered statistically significant. Results are expressed as means ± SEM.
Results
PROS1 Expression Is Upregulated in Resolution Phase Macrophages
The phagocytosis of apoptotic neutrophils is a key step during the resolution of inflammation. To investigate the role of PROS1 during the resolution of inflammation, we utilized the zymosan A-induced peritonitis as a prototypic model (35). Peritoneal macrophages were harvested from unchallenged mice or during the inflammatory (24 h) and resolving (48–66 h) phases of peritonitis. Pros1 mRNA levels in isolated macrophages were quantified by RT-qPCR and normalized to Gapdh. Our results indicate that resident macrophages (present at 0 h), inflammatory, and early resolution phase macrophages (24 h) expressed very little Pros1, whereas a sharp upregulation of Pros1 mRNA in resolving macrophages (66 h) was observed (Figure 1A). To verify Pros1 upregulation in macrophages, we measured Pros1 transcripts in peritoneal macrophages of LysMCre/+; Pros1fl/fl mice, in which Pros1 expression is ablated following Cre-mediated excision in myeloid cells. We did not observe basal expression nor zymosan-induced upregulation of Pros1 in peritoneal macrophages isolated from LysMCre/+; Pros1fl/fl mice (36, 37) (Figure 1B). During the resolution phase, PROS1 protein levels present in the peritoneal fluids of LysMCre/+; Pros1fl/fl mice, were also significantly reduced compared to Pros1fl/fl controls (Figure 1C). Thus, macrophages seem to express higher levels of PROS1 during the resolution of inflammation and contribute to its peritoneal levels.
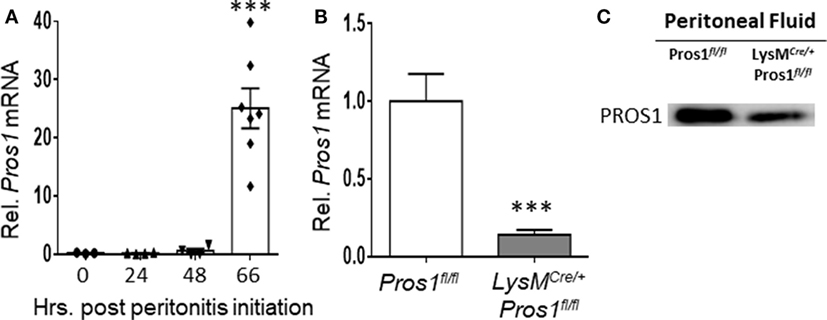
Figure 1. Protein S (PROS1) mRNA expression in resolution phase macrophages. (A) Peritoneal cells were harvested from unchallenged Pros1fl/fl mice or mice undergoing zymosan A-initiated peritonitis for 24, 48, or 66 hrs. RNA was extracted from isolated macrophages, and Pros1 transcript levels were quantified by RT-qPCR and normalized to GAPDH (n = 3–7 mice per time point). Results represent the distribution of individual mice and the mean ± SEM. Statistical significance by one-way ANOVA (***P-value < 0.0001) is indicated. (B) PROS1 expression in Pros1fl/fl and LysMCre/+; Pros1fl/fl resolution phase macrophages (66 h post peritonitis initiation) was quantified by RT-qPCR. Results are presented as the mean ± SEM from six mice/genotype. Student’s t-test, ***P ≤ 0.0001. (C) Peritoneal fluids from individual mice were collected 66 h after zymosan A injection and analyzed for PROS1 protein levels by Western blot. A representative blot of three experiments (three to five mice per experiment) is shown.
PROS1 Deficiency in Resolution Phase Macrophages Impairs Their Ability to Engulf Apoptotic Remnants
The clearance of apoptotic neutrophils from resolving inflammation sites is essential for resolution and return to homeostasis (38). Considering the role of PROS1 in the phagocytosis of ACs (39), and its expression by resolution phase macrophages (Figure 1), we examined whether Pros1-deficient macrophages exert modified uptake of apoptotic neutrophils. To this end, we determined the phagocytic capacity in vivo of peritoneal resolution phase macrophages between LysMCre/+; Pros1fl/fl (cKO) and their control Pros1fl/fl littermates. Our results indicate that macrophages deficient in PROS1 display a reduced ability to phagocytose apoptotic particles in vivo (Figures 2A–C). Quantification of this phenomenon showed that PROS1-deficient macrophages had impaired phagocytic capacity, reflected by a lower phagocytic efficiency index compared to control cells (174 ± 16 and 271 ± 20%, respectively, Figure 2B). The majority of PROS1-deficient macrophages did not engulf any apoptotic particles (55 ± 4% compared to 37 ± 3% in controls) (Figure 2C). While a similar percentage of cKO and control cells had engulfed one or two apoptotic particles (25.9 ± 2 and 26.6 ± 2%, respectively), control cells were twice as active in engulfing three to seven apoptotic moieties compared to cKO cells (31.4 ± 2.5 and 16.2 ± 2.6%, respectively). Finally, the uptake efficiency declined for both cell types scored with 8 or more Hoechst-positive foci, with a nonsignificant trend pointing to decreased efferocytosis in cKO cells, with 5.4 ± 1% of control cells, but only 2.8 ± 1% of cKO cells (Figure 2C).
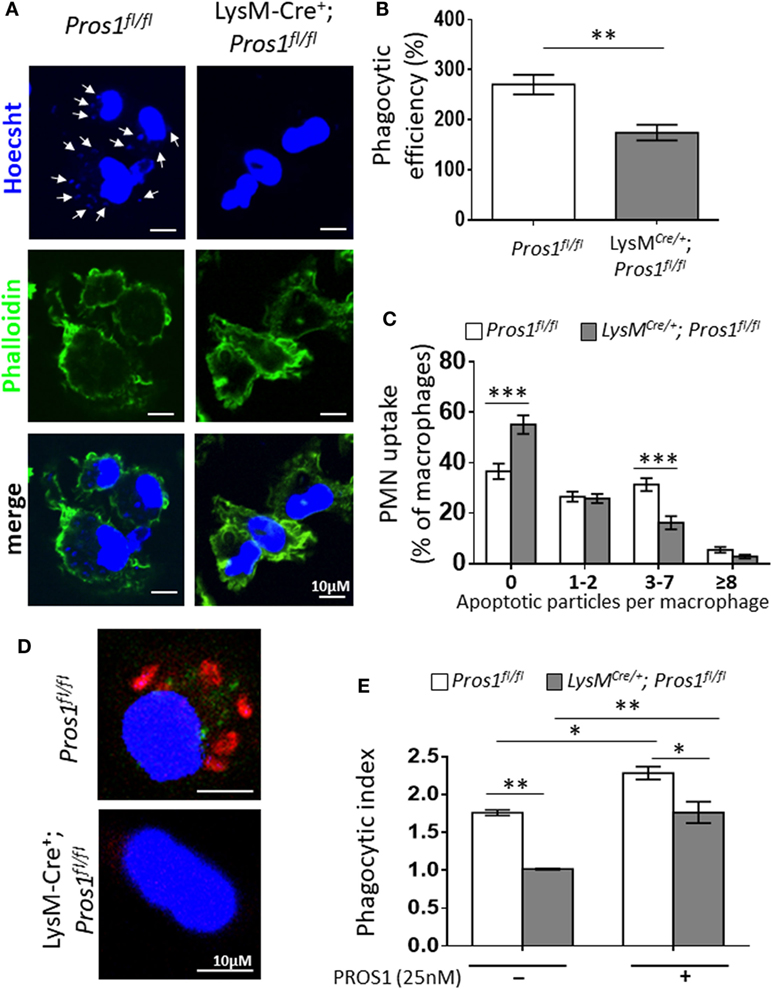
Figure 2. Protein S (PROS1)-deficient macrophages demonstrate a decreased engulfment of apoptotic neutrophils in vivo. (A) Resolution phase peritoneal macrophages from Pros1fl/fl (left) and LysMCre/+; Pros1fl/fl (right) mice were isolated and stained with Hoechst (blue) and FITC-phaloidin (green). Z-stack 3D confocal images were taken with a Nikon A1-R microscope. (B) The number of PMN particles in each macrophage was enumerated using the Nikon NIS-Elements microscope imaging software, and the percentage of phagocytic macrophages and the number of apoptotic particles (arrows in A) per macrophage were calculated into the overall phagocytic efficiency index. **P = 0.004 (t-test). (C) Engulfment according to thresholds of intracellular apoptotic particles was calculated. Two-way analysis of variance (ANOVA), ***P ≤ 0.0001. (D) Confocal images of in vitro phagocytosis using pre-labeled apoptotic Jurkat cells (red). (E) The phagocytic index was calculated in the absence or presence of purified PROS1. Two-way ANOVA, *P ≤ 0.02, **P = 0.005. Results are representative images (A,D) or means ± SEM (B,C,E) from at least three independent experiments (n = 8–11 mice; over 1,500 macrophages scored).
We next tested whether the addition of purified PROS1 would rescue the impaired phagocytosis exhibited by PROS1-deficient macrophages. For this, we performed an ex vivo phagocytosis assay, assessing the efferocytosis of resolution phase peritoneal macrophages that were fed with pre-labeled apoptotic Jurkat cells (Figure 2D). Akin to their in vivo performance, a decreased phagocytic index was recorded for PROS1-cKO macrophages (1 ± 0.008 compared to 1.7 ± 0.03 in controls) (Figure 2E). The supplementation of exogenous PROS1 rescued the phagocytic performance of cKO cells, bringing it to normal levels of untreated control macrophages, and augmented the phagocytic capacity of Pros1fl/fl control cells (phagocytic indices of 1.7 ± 0.14 and 2.2 ± 0.08, respectively) (Figure 2E).
Taken together, our results identify endogenously expressed PROS1 as an important mediator of efferocytosis in resolution phase macrophages. Given the importance of phagocytic macrophages in clearing apoptotic neutrophils during the resolution of inflammation, we conclude that Pros1-deficient macrophages exhibit a hampered pro-resolving phagocytic phenotype.
PROS1-Deficient Macrophages Display Reduced Reprogramming
Apoptotic neutrophil engulfment by resolution phase macrophages results in their conversion from pro-inflammatory cells to anti-inflammatory/reparative ones (40). These M2-like macrophages prevent unwanted excessive inflammatory responses during the resolution phase of inflammation and promote tissue repair. To determine whether PROS1 expressed by resolution phase macrophages plays a role in this in vivo transition, termed reprogramming, we isolated resolution phase peritoneal macrophages 66 h post zymosan A treatment from Pros1-proficient or deficient mice. Isolated macrophages were then stimulated with LPS, and the secretion of pro-inflammatory cytokines and chemokines (TNFα, IL-6, and CCL3) or the anti-inflammatory cytokine IL-10 was determined (Figures 3A–D). Our results indicate that following LPS stimulation, PROS1-deficient macrophages secreted significantly elevated levels of TNFα in comparison to their Pros1fl/fl counterparts (820 ± 114.6 and 320.3 ± 61.3 pg/ml, respectively). The secretion of CCL3 and IL-6 was also elevated in cKO macrophages following stimulation with LPS in comparison to Pros1fl/fl ones, although the latter was not statistically significant (25.6 ± 3.3 versus 10.7 ± 1.8 pg/ml for CCL3 by cKO and controls, and 12,771 ± 4,432 versus 5,106 ± 2,684 pg/ml for IL-6 by cKO and control cells, respectively) (Figures 3B,C). Concomitantly, the secretion of IL-10 from LysMCre/+; Pros1fl/fl macrophages was significantly lower than its secretion by Pros1fl/fl macrophages under baseline conditions and upon stimulation with LPS (861 ± 136 and 1,990 ± 258 pg/ml for unstimulated cKOs and controls, and 1,165.5 ± 431 and 2,783.2 ± 368 pg/ml for stimulated cKO and controls, respectively) (Figure 3D). Thus, Pros1-deficient macrophages present a shift toward exacerbated pro-inflammatory cytokine secretion, suggesting hampered reprogramming compared to their control counterparts. Since IL-10 is a key cytokine in macrophage reprogramming (5), we next determined the effect of exogenous PROS1 on AC-induced IL-10 secretion ex vivo. Our results indicate that ACs promoted the secretion of IL-10 in both control and Pros1-cKO mice when unstimulated, but upon LPS stimulation, this effect was absent in PROS1-deficient macrophages (Figure 3E). Unexpectedly, the addition of PROS1 was unable to enhance the effect of ACs on IL-10 secretion in PROS1-deficient mice and in fact reduced IL-10 secretion from untreated and LPS-stimulated macrophages (both WT and PROS1-deficient). This may be due to the biochemical nature of PROS1. PROS1 acts as a bridging molecule that binds phosphatidylserine (PstSer) exposed on the outer leaflets of ACs via its amino terminus and to the extracellular domain of TAM receptors on phagocytes and macrophages via its carboxy terminus (24). Thus, the addition of excess PROS1 to the combined culture of macrophages and ACs may saturate the binding sites on ACs and macrophages without physically bridging between them. It is conceivable that the controlled and sequential addition of PROS1 to macrophages and ACs would favor the bridging and subsequent reduction of IL-10 production. Nevertheless, our results indicate that compared to controls, PROS1-deficient macrophages present a hampered response to AC uptake that results in a pro-inflammatory imbalance with elevated TNFα and CCL3 as well as lower IL-10 production and could only partially be rescued by exogenous PROS1.
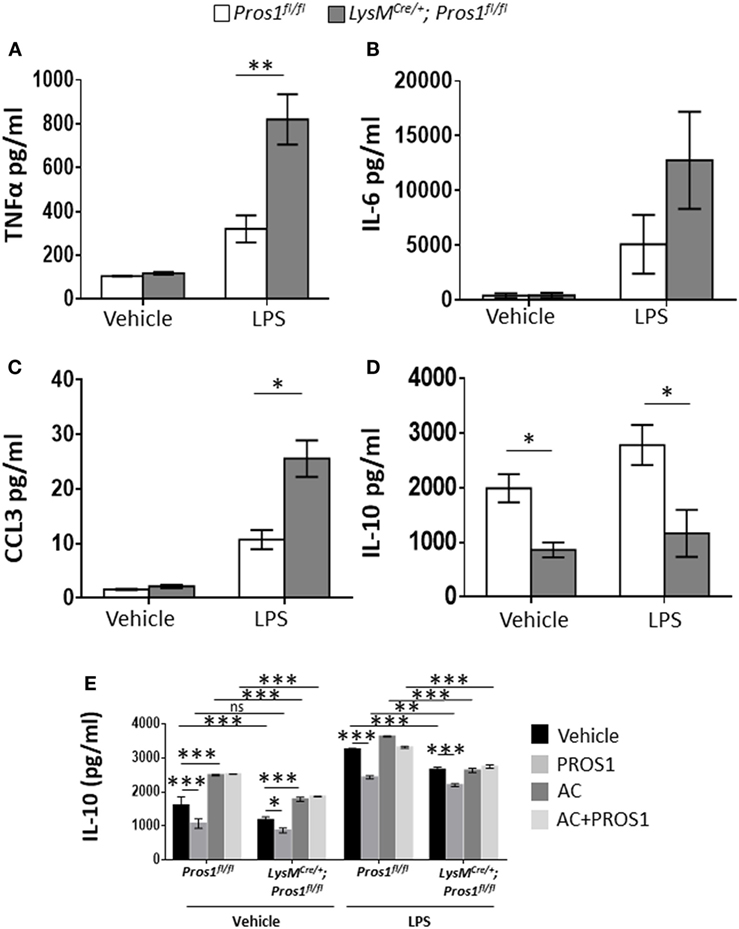
Figure 3. Protein S (PROS1)-deficient macrophages display reduced reprogramming. Macrophages from Pros1fl/fl or LysMCre/+; Pros1fl/fl mice were isolated from peritoneal exudates 66 h after zymosan A injection and incubated for 24 h with vehicle or lipopolysaccharide (LPS, 1 µg/ml). Then, supernatants were collected and analyzed for their content of TNFα [(A), **P = 0.003], IL-6 (B), CCL3 [(C), *P = 0.02], and IL-10 [(D); *P ≤ 0.03] by standard ELISA. (E) IL-10 secretion by untreated or LPS-stimulated control and Pros1-cKO peritoneal macrophages, or by macrophages supplemented with either PROS1 (25 nM), apoptotic cells (AC) or both (AC + PROS1). Results represent the means ± SEM of at least three independent experiments. Two-way ANOVA, *P = 0.02, ***P ≤ 0.0001.
Pros1-Deficient Macrophages Express Reduced Levels of Pro-Resolving Enzymes
During the resolution of inflammation, the engulfment of apoptotic neutrophils leads to macrophage metamorphosis from an M1-like phenotype to an M2-like phenotype and then to a pro-resolving phenotype (Mres) highlighted by the expression of the functionally important enzyme 12/15-LO (40). These changes are associated with reprogramming of the engulfing macrophages (41). Since our results so far indicate that Pros1 expression by resolution phase macrophages is required for their uptake of apoptotic neutrophils and reprogramming, we sought to analyze the expression of proteins that are instrumental to inflammation and its resolution (19, 42–44). 12/15-LO is a key enzyme involved in the synthesis of lipoxins, protectins, resolvins and other specialized lipid mediators that promote the resolution of inflammation by macrophages (45–47). Arginase-1 enzymatically inhibits nitric oxide (NO) production by inducible NO synthase (iNOS), thereby supporting an anti-inflammatory/reparative milieu (48). Since iNOS characterizes M1-like macrophages and is highly expressed by pro-inflammatory macrophages, the levels of arginase-1 expression reflect the maturation level of macrophages and their progression to the reparative phenotype during resolution (40). To evaluate macrophage maturation and differentiation, peritoneal macrophages from LysMCre/+; Pros1fl/fl; and Pros1fl/fl mice were isolated 66 h post peritonitis initiation, and their protein content was immuno-blotted for the macrophage M2/maturation markers arginase-1 and CD11b as well as for the pro-resolving enzyme 12/15-LO (Figure 4). Our results indicate that LysMCre/+; Pros1fl/fl macrophages express significantly lower levels of all three proteins (86, 83, and 81% of controls, for 12/15-LO, arginase-1, and CD11b, respectively). Thus, LysMCre/+; Pros1fl/fl macrophages display a reduced capacity to mature and convert to the M2-like and Mres phenotypes during the resolution of inflammation. 12/15-LO catalyzes the derivation of DHA into resolvin (Rv) D1. Given the importance of RvD1 as a key mediator involved in the resolution of inflammation, we tested whether the decreased levels of 12/15-LO in PROS1-deficient macrophages (Figure 4) would also affect the potential production of RvD1 by these cells. We found a 25% decrease in the production of RvD1 by PROS1-defiecient cells compared to that of controls (1,899 ± 33.2 and 2,527 ± 57.8 pg/ml for cKO and controls, respectively) (Figure S1 in Supplementary Material). Thus, the reduced expression of 12/15-LO in PROS1-deficient resolution phase macrophages results in a reduced capacity to produce specialized pro-resolving lipid mediators. Taken together, our results indicate that macrophage-derived PROS1 is a molecular effector in the uptake of apoptotic neutrophils and participates in the consequent reprogramming and maturation of macrophages in resolving inflammation.
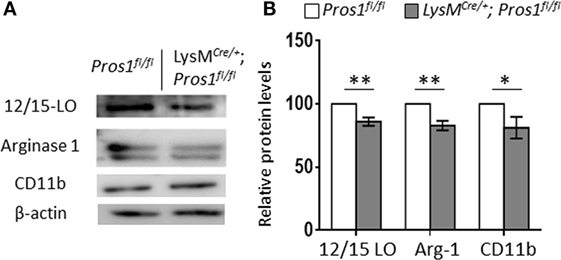
Figure 4. Protein S (PROS1)-deficient macrophages express reduced levels of resolution phase enzymes. Peritoneal macrophages from Pros1fl/fl or LysMCre/+; Pros1fl/fl mice (n = 5/group) were isolated 66 h after zymosan A injection and lysed, and their protein content was analyzed by Western blot for the resolution phase markers 12/15-LO, arginase-1, and CD11b. A representative blot is shown (A). Band intensities were quantified, and graphs represent the normalized means ± SEM from three independent experiments (B). Student’s t-test, *P = 0.03, **P ≤ 0.02.
Discussion
Protein S is a pleiotropic mediator involved in various processes, such as vasculogenesis, blood clotting, and immune regulation (29, 33, 37, 49). While hepatocytes are considered to be the major source of plasma PROS1, significant contributions to the volume and function of PROS1 were attributed to other sources, such as endothelial and T cells (29, 33, 37). Our findings now indicate that PROS1 is produced by macrophages during the resolution of murine peritonitis, and consequently its production and release by peritoneal macrophages as well as other cells contribute to peritoneal levels of PROS1 (Figure 1). While peritoneal PROS1 levels probably increase during the inflammatory phase of peritonitis, due to exudation of plasma proteins, it is unlikely that peritoneal PROS1 will remain at high levels in the absence of additional sources. Along these lines, PROS1 from macrophages seems to be an important component in its peritoneal levels during resolution. Notably, PROS1 produced by resolution phase macrophages is functionally important. This could be due to a critical concentration of the peritoneal protein or due to a local regulation of PROS1 function as seen in the lymph nodes (29) and the retina (33). Similarly, our results indicate that the discrete secretion of macrophage-generated PROS1 is essential for key-resolving features of efferocytosis and molecular reprogramming. Such localized secretion could be envisioned in the contact area between apoptotic PMN and the macrophages that engulf them (50).
Protein S was previously found to bind the tyrosine kinase receptors from the TAM family and mediate their interactions with ACs through the binding of PstSer (39, 51–53). As expected in this setting, the deficiency in PROS1 production by efferocytosis-competent macrophages resulted in a significant reduction in the uptake of apoptotic PMNs in LysMCre/+; Pros1fl/fl macrophages, which was rescued following the addition of purified PROS1 (Figure 2). The engulfment of apoptotic PMNs is essential for the resolution of inflammation [reviewed in Refs. (5, 40)]. This is in part due to the phenotypic changes that take place in the engulfing monocytes/macrophages (5, 10, 54). These changes, also termed macrophage reprogramming/immune-silencing, are characterized by a reduction in the production of pro-inflammatory cytokines and chemokines concomitant with an increase in the production of anti-inflammatory cytokines, such as IL-10, upon exposure to bacterial moieties (21, 41, 55). Notably, the TAM receptors were previously shown to block TNFα- and TLR-mediated inflammatory signals (25, 31, 56). In addition, the PROS1-mediated phagocytosis of ACs by peripheral blood monocytes contributes to the elimination of dying and dead cells in the circulation (39), thus avoiding the induction of harmful inflammatory responses. Our current results indicate that a myeloid-specific deficiency in Pros1 culminates in increased amounts of the pro-inflammatory mediators TNFα and CCL3 and reduced amounts of the anti-inflammatory/pro-resolving cytokine IL-10, upon stimulation with LPS (Figure 3), a profile resembling pro-inflammatory rather than resolution phase macrophages.
During murine peritonitis, CD11bmed monocytes differentiate to CD11bhigh macrophages that engulf apoptotic PMNs in a self-limiting fashion and convert to CD11blow-satiated macrophages (21, 35). These phenotypic changes are associated with a temporal increase in the expression of arginase-1, a hallmark of M2 macrophages that is induced by AC uptake (19, 57). 12/15-LO, an enzyme that is involved in the production of pro-resolving lipid mediators including RvD1 (1, 58) and the uptake of ACs (59), is progressively upregulated by macrophages during the resolution of inflammation. Moreover, it is a hallmark of macrophage conversion from the CD11bhigh to the CD11blow phenotype (21). Although CD11b protein levels were reduced in Pros1-cKO-resolving macrophages, the expression of arginase-1 and 12/15-LO—two bona fide markers of resolution phase macrophages—was lower in efferocytosing macrophages lacking PROS1 (Figure 4). In line with the reduced levels of 12/15-LO, less RvD1 is produced by macrophages devoid of PROS1 (Figure S1 in Supplementary Material). Together, with their impaired efferocytosis and a pro-inflammatory cytokine profile, these results suggest that the conversion to resolution phase macrophages in the absence of PROS1 is either incomplete or hampered. Hence, PROS1 production by local macrophages seems to be important in their reprogramming during the resolution phase of inflammation.
In sum, our results indicate that Pros1 production and action in pro-resolving macrophages are key events in the termination of inflammation. PROS1 acts in various modes as it regulates both the uptake of apoptotic PMNs and the consequent reprogramming of macrophages. These findings suggest that PROS1 might be harnessed as a new strategy for the treatment of inflammatory and autoimmune disorders.
Ethics Statement
All animal experiments were approved by the Hebrew University – Hadassah ethics committee.
Author Contributions
DL, TB-C, and AA designed the research. DL, SS, AM, and SS-Z performed experiments, analyzed, and visualized data. TB-C and AA supervised the project. DL, TB-C, and AA wrote the manuscript.
Conflict of Interest Statement
The authors declare that the research was conducted in the absence of any commercial or financial relationships that could be construed as a potential conflict of interest.
Funding
This work was supported by grants from the Israel Science Foundation (grant number 678/13 to AA and grant number 984/2012 to TB-C), the Rosetrees Trust, and the Wolfson Family Charitable Trust to AA and the Israel Cancer Research Foundation to TB-C. DL was awarded with the Azrieli postdoctoral fellowship and supported by the Ministry of Aliya and Integration (MOIA) of the State of Israel.
Supplementary Material
The Supplementary Material for this article can be found online at http://www.frontiersin.org/articles/10.3389/fimmu.2018.00358/full#supplementary-material.
References
1. Levy BD, Clish CB, Schmidt B, Gronert K, Serhan CN. Lipid mediator class switching during acute inflammation: signals in resolution. Nat Immunol (2001) 2(7):612–9. doi:10.1038/89759
2. Majno G, Joris I. Cells, Tissues, and Disease—Principles of General Pathology. New York: Oxford University Press (2004).
3. Serhan CN, Brain SD, Buckley CD, Gilroy DW, Haslett C, O’Neill LAJ, et al. Resolution of inflammation: state of the art, definitions and terms. FASEB J (2007) 21(2):325–32. doi:10.1096/fj.06-7227rev
4. Ortega-Gómez A, Perretti M, Soehnlein O. Resolution of inflammation: an integrated view. EMBO Mol Med (2013) 5(5):661–74. doi:10.1002/emmm.201202382
5. Fadok VA, Bratton DL, Konowal A, Freed PW, Westcott JY, Henson PM. Macrophages that have ingested apoptotic cells in vitro inhibit proinflammatory cytokine production through autocrine/paracrine mechanisms involving TGF-beta, PGE2, and PAF. J Clin Invest (1998) 101(4):890–8. doi:10.1172/jci1112
6. Rossi AG, Haslett C. Inflammation, cell injury, and apoptosis. In: Said SI, editor. Pro-Inflammatory and Anti-Inflammatory Peptides. New York, NY: Marcel Dekker Inc. (1998). p. 9–24.
7. Savill J, Dransfield I, Gregory C, Haslett C. A blast from the past: clearance of apoptotic cells regulates immune responses. Nat Rev Immunol (2002) 2(12):965–75. doi:10.1038/nri957
8. Greenlee-Wacker MC. Clearance of apoptotic neutrophils and resolution of inflammation. Immunol Rev (2016) 273(1):357–70. doi:10.1111/imr.12453
9. Ravichandran KS, Lorenz U. Engulfment of apoptotic cells: signals for a good meal. Nat Rev Immunol (2007) 7(12):964–74. doi:10.1038/nri2214
10. Voll RE, Herrmann M, Roth EA, Stach C, Kalden JR, Girkontaite I. Immunosuppressive effects of apoptotic cells. Nature (1997) 390(6658):350–1. doi:10.1038/37022
11. Hanayama R, Tanaka M, Miwa K, Shinohara A, Iwamatsu A, Nagata S. Identification of a factor that links apoptotic cells to phagocytes. Nature (2002) 417(6885):182–7. doi:10.1038/417182a
12. Kim S, Elkon KB, Ma X. Transcriptional suppression of interleukin-12 gene expression following phagocytosis of apoptotic cells. Immunity (2004) 21(5):643–53. doi:10.1016/j.immuni.2004.09.009
13. Stables MJ, Shah S, Camon EB, Lovering RC, Newson J, Bystrom J, et al. Transcriptomic analyses of murine resolution-phase macrophages. Blood (2011) 118(26):e192–208. doi:10.1182/blood-2011-04-345330
14. Patel VA, Longacre A, Hsiao K, Fan H, Meng F, Mitchell JE, et al. Apoptotic cells, at all stages of the death process, trigger characteristic signaling events that are divergent from and dominant over those triggered by necrotic cells: implications for the delayed clearance model of autoimmunity. J Biol Chem (2006) 281(8):4663–70. doi:10.1074/jbc.M508342200
15. Bystrom J, Evans I, Newson J, Stables M, Toor I, van Rooijen N, et al. Resolution-phase macrophages possess a unique inflammatory phenotype that is controlled by cAMP. Blood (2008) 112(10):4117–27. doi:10.1182/blood-2007-12-129767
16. Byrne A, Reen DJ. Lipopolysaccharide induces rapid production of IL-10 by monocytes in the presence of apoptotic neutrophils. J Immunol (2002) 168(4):1968–77. doi:10.4049/jimmunol.168.4.1968
17. Huynh M-LN, Fadok VA, Henson PM. Phosphatidylserine-dependent ingestion of apoptotic cells promotes TGF-β1 secretion and the resolution of inflammation. J Clin Invest (2002) 109(1):41–50. doi:10.1172/jci11638
18. Mitchell S, Thomas G, Harvey K, Cottell D, Reville K, Berlasconi G, et al. Lipoxins, aspirin-triggered Epi-lipoxins, lipoxin stable analogues, and the resolution of inflammation: stimulation of macrophage phagocytosis of apoptotic neutrophils in vivo. J Am Soc Nephrol (2002) 13(10):2497–507. doi:10.1097/01.asn.0000032417.73640.72
19. Freire-de-Lima CG, Xiao YQ, Gardai SJ, Bratton DL, Schiemann WP, Henson PM. Apoptotic cells, through transforming growth factor-β, coordinately induce anti-inflammatory and suppress pro-inflammatory eicosanoid and NO synthesis in murine macrophages. J Biol Chem (2006) 281(50):38376–84. doi:10.1074/jbc.M605146200
20. Schwab JM, Chiang N, Arita M, Serhan CN. Resolvin E1 and protectin D1 activate inflammation-resolution programmes. Nature (2007) 447(7146):869–74. doi:10.1038/nature05877
21. Schif-Zuck S, Gross N, Assi S, Rostoker R, Serhan CN, Ariel A. Saturated-efferocytosis generates pro-resolving CD11 blow macrophages: modulation by resolvins and glucocorticoids. Eur J Immunol (2011) 41(2):366–79. doi:10.1002/eji.201040801
22. Ten Kate MK, van der Meer J. Protein S deficiency: a clinical perspective. Haemophilia (2008) 14(6):1222–8. doi:10.1111/j.1365-2516.2008.01775.x
23. Stitt TN, Conn G, Gore M, Lai C, Bruno J, Radziejewski C, et al. The anticoagulation factor protein S and its relative, GAS6, are ligands for the Tyro 3/Axl family of receptor tyrosine kinases. Cell (1995) 80(4):661–70. doi:10.1016/0092-8674(95)90520-0
24. Lemke G. Biology of the TAM receptors. Cold Spring Harb Perspect Biol (2013) 5(11):a009076. doi:10.1101/cshperspect.a009076
25. Rothlin CV, Ghosh S, Zuniga EI, Oldstone MB, Lemke G. TAM receptors are pleiotropic inhibitors of the innate immune response. Cell (2007) 131(6):1124–36. doi:10.1016/j.cell.2007.10.034
26. Lemke G, Rothlin CV. Immunobiology of the TAM receptors. Nat Rev Immunol (2008) 8(5):327–36. doi:10.1038/nri2303
27. Lemke G, Burstyn-Cohen T. TAM receptors and the clearance of apoptotic cells. Ann N Y Acad Sci (2010) 1209(1):23–9. doi:10.1111/j.1749-6632.2010.05744.x
28. Rothlin CV, Carrera-Silva EA, Bosurgi L, Ghosh S. TAM receptor signaling in immune homeostasis. Annu Rev Immunol (2015) 33:355–91. doi:10.1146/annurev-immunol-032414-112103
29. Carrera Silva EA, Chan PY, Joannas L, Errasti AE, Gagliani N, Bosurgi L, et al. T cell-derived protein S engages TAM receptor signaling in dendritic cells to control the magnitude of the immune response. Immunity (2013) 39(1):160–70. doi:10.1016/j.immuni.2013.06.010
30. Zagorska A, Traves PG, Lew ED, Dransfield I, Lemke G. Diversification of TAM receptor tyrosine kinase function. Nat Immunol (2014) 15(10):920–8. doi:10.1038/ni.2986
31. Camenisch TD, Koller BH, Earp HS, Matsushima GK. A novel receptor tyrosine kinase, Mer, inhibits TNF-alpha production and lipopolysaccharide-induced endotoxic shock. J Immunol (1999) 162(6):3498–503.
32. Lu Q, Lemke G. Homeostatic regulation of the immune system by receptor tyrosine kinases of the Tyro 3 family. Science (2001) 293(5528):306–11. doi:10.1126/science.1061663
33. Burstyn-Cohen T, Lew ED, Traves PG, Burrola PG, Hash JC, Lemke G. Genetic dissection of TAM receptor-ligand interaction in retinal pigment epithelial cell phagocytosis. Neuron (2012) 76(6):1123–32. doi:10.1016/j.neuron.2012.10.015
34. Koenigsknecht J, Landreth G. Microglial phagocytosis of fibrillar beta-amyloid through a beta1 integrin-dependent mechanism. J Neurosci (2004) 24(44):9838–46. doi:10.1523/JNEUROSCI.2557-04.2004
35. Bannenberg GL, Chiang N, Ariel A, Arita M, Tjonahen E, Gotlinger KH, et al. Molecular circuits of resolution: formation and actions of resolvins and protectins. J Immunol (2005) 174(7):4345–55. doi:10.4049/jimmunol.174.7.4345
36. Clausen BE, Burkhardt C, Reith W, Renkawitz R, Forster I. Conditional gene targeting in macrophages and granulocytes using LysMcre mice. Transgenic Res (1999) 8(4):265–77. doi:10.1023/A:1008942828960
37. Burstyn-Cohen T, Heeb MJ, Lemke G. Lack of protein S in mice causes embryonic lethal coagulopathy and vascular dysgenesis. J Clin Invest (2009) 119(10):2942–53. doi:10.1172/JCI39325
38. Bratton DL, Henson PM. Neutrophil clearance: when the party is over, clean-up begins. Trends Immunol (2011) 32(8):350–7. doi:10.1016/j.it.2011.04.009
39. Anderson HA, Maylock CA, Williams JA, Paweletz CP, Shu H, Shacter E. Serum-derived protein S binds to phosphatidylserine and stimulates the phagocytosis of apoptotic cells. Nat Immunol (2003) 4(1):87–91. doi:10.1038/ni871
40. Ariel A, Serhan CN. New lives given by cell death: macrophage differentiation following their encounter with apoptotic leukocytes during the resolution of inflammation. Front Immunol (2012) 3:4. doi:10.3389/fimmu.2012.00004
41. Pashover-Schallinger E, Aswad M, Schif-Zuck S, Shapiro H, Singer P, Ariel A. The atypical chemokine receptor D6 controls macrophage efferocytosis and cytokine secretion during the resolution of inflammation. FASEB J (2012) 26(9):3891–900. doi:10.1096/fj.11-194894
42. Sica A, Mantovani A. Macrophage plasticity and polarization: in vivo veritas. J Clin Invest (2012) 122(3):787–95. doi:10.1172/jci59643
43. Chávez-Galán L, Olleros ML, Vesin D, Garcia I. Much more than M1 and M2 macrophages, there are also CD169+ and TCR+ macrophages. Front Immunol (2015) 6:263. doi:10.3389/fimmu.2015.00263
44. Roszer T. Understanding the mysterious M2 macrophage through activation markers and effector mechanisms. Mediators Inflamm (2015) 2015:16. doi:10.1155/2015/816460
45. Merched AJ, Ko K, Gotlinger KH, Serhan CN, Chan L. Atherosclerosis: evidence for impairment of resolution of vascular inflammation governed by specific lipid mediators. FASEB J (2008) 22(10):3595–606. doi:10.1096/fj.08-112201
46. Serhan CN, Chiang N, Van Dyke TE. Resolving inflammation: dual anti-inflammatory and pro-resolution lipid mediators. Nat Rev Immunol (2008) 8(5):349–61. doi:10.1038/nri2294
47. Krönke G, Katzenbeisser J, Uderhardt S, Zaiss MM, Scholtysek C, Schabbauer G, et al. 12/15-lipoxygenase counteracts inflammation and tissue damage in arthritis. J Immunol (2009) 183(5):3383–9. doi:10.4049/jimmunol.0900327
48. Rőszer T. Phagosomal and lysosomal NO synthesis. The Biology of Subcellular Nitric Oxide. Dordrecht: Springer (2012). p. 145–55.
49. Suleiman L, Negrier C, Boukerche H. Protein S: a multifunctional anticoagulant vitamin K-dependent protein at the crossroads of coagulation, inflammation, angiogenesis, and cancer. Crit Rev Oncol Hematol (2013) 88(3):637–54. doi:10.1016/j.critrevonc.2013.07.004
50. Poon IKH, Lucas CD, Rossi AG, Ravichandran KS. Apoptotic cell clearance: basic biology and therapeutic potential. Nat Rev Immunol (2014) 14(3):166–80. doi:10.1038/nri3607
51. Davra V, Kimani SG, Calianese D, Birge RB. Ligand activation of TAM family receptors-implications for tumor biology and therapeutic response. Cancers (Basel) (2016) 8(12):E107. doi:10.3390/cancers8120107
52. Kasikara C, Kumar S, Kimani S, Tsou W-I, Geng K, Davra V, et al. Phosphatidylserine sensing by TAM receptors regulates AKT-dependent chemoresistance and PD-L1 expression. Mol Cancer Res (2017) 15(6):753–64. doi:10.1158/1541-7786.mcr-16-0350
53. Lemke G. Phosphatidylserine is the signal for TAM receptors and their ligands. Trends Biochem Sci (2017) 42(9):738–48. doi:10.1016/j.tibs.2017.06.004
54. Golpon HA, Fadok VA, Taraseviciene-Stewart L, Scerbavicius R, Sauer C, Welte T, et al. Life after corpse engulfment: phagocytosis of apoptotic cells leads to VEGF secretion and cell growth. FASEB J (2004) 18(14):1716–8. doi:10.1096/fj.04-1853fje
55. Millet A, Martin KR, Bonnefoy F, Saas P, Mocek J, Alkan M, et al. Proteinase 3 on apoptotic cells disrupts immune silencing in autoimmune vasculitis. J Clin Invest (2015) 125(11):4107–21. doi:10.1172/jci78182
56. Sharif MN, Šošić D, Rothlin CV, Kelly E, Lemke G, Olson EN, et al. Twist mediates suppression of inflammation by type I IFNs and Axl. J Exp Med (2006) 203(8):1891–901. doi:10.1084/jem.20051725
57. Titos E, Rius B, González-Périz A, López-Vicario C, Morán-Salvador E, Martínez-Clemente M, et al. Resolvin D1 and its precursor docosahexaenoic acid promote resolution of adipose tissue inflammation by eliciting macrophage polarization toward an M2-like phenotype. J Immunol (2011) 187(10):5408–18. doi:10.4049/jimmunol.1100225
58. Ariel A, Li P-L, Wang W, Tang W-X, Fredman G, Hong S, et al. The docosatriene protectin D1 is produced by TH2 skewing and promotes human T cell apoptosis via lipid raft clustering. J Biol Chem (2005) 280(52):43079–86. doi:10.1074/jbc.M509796200
Keywords: inflammation, macrophages, protein S, apoptosis, efferocytosis
Citation: Lumbroso D, Soboh S, Maimon A, Schif-Zuck S, Ariel A and Burstyn-Cohen T (2018) Macrophage-Derived Protein S Facilitates Apoptotic Polymorphonuclear Cell Clearance by Resolution Phase Macrophages and Supports Their Reprogramming. Front. Immunol. 9:358. doi: 10.3389/fimmu.2018.00358
Received: 02 July 2017; Accepted: 08 February 2018;
Published: 01 March 2018
Edited by:
Janos G. Filep, Université de Montréal, CanadaReviewed by:
Ian Dransfield, University of Edinburgh, United KingdomJoan Clària, Hospital Clínic de Barcelona, Spain
Copyright: © 2018 Lumbroso, Soboh, Maimon, Schif-Zuck, Ariel and Burstyn-Cohen. This is an open-access article distributed under the terms of the Creative Commons Attribution License (CC BY). The use, distribution or reproduction in other forums is permitted, provided the original author(s) and the copyright owner are credited and that the original publication in this journal is cited, in accordance with accepted academic practice. No use, distribution or reproduction is permitted which does not comply with these terms.
*Correspondence: Amiram Ariel, YW1pcmFtQHJlc2VhcmNoLmhhaWZhLmFjLmls;
Tal Burstyn-Cohen, dGFsYnVAbWFpbC5odWppLmFjLmls
†These authors have contributed equally to this work.