- 1National Pediatric Neuroinflammation Organization, Inc., Orlando, FL, United States
- 2College of Medicine, University of Central Florida, Orlando, FL, United States
The concept and recognized components of “neuroinflammation” are expanding at the intersection of neurobiology and immunobiology. Chemokines (CKs), no longer merely necessary for immune cell trafficking and positioning, have multiple physiologic, developmental, and modulatory functionalities in the central nervous system (CNS) through neuron–glia interactions and other mechanisms affecting neurotransmission. They issue the “help me” cry of neurons and astrocytes in response to CNS injury, engaging invading lymphoid cells (T cells and B cells) and myeloid cells (dendritic cells, monocytes, and neutrophils) (adaptive immunity), as well as microglia and macrophages (innate immunity), in a cascade of events, some beneficial (reparative), others destructive (excitotoxic). Human cerebrospinal fluid (CSF) studies have been instrumental in revealing soluble immunobiomarkers involved in immune dysregulation, their dichotomous effects, and the cells—often subtype specific—that produce them. CKs/cytokines continue to be attractive targets for the pharmaceutical industry with varying therapeutic success. This review summarizes the developing armamentarium, complexities of not compromising surveillance/physiologic functions, and insights on applicable strategies for neuroinflammatory disorders. The main approach has been using a designer monoclonal antibody to bind directly to the chemo/cytokine. Another approach is soluble receptors to bind the chemo/cytokine molecule (receptor ligand). Recombinant fusion proteins combine a key component of the receptor with IgG1. An additional approach is small molecule antagonists (protein therapeutics, binding proteins, and protein antagonists). CK neutralizing molecules (“neutraligands”) that are not receptor antagonists, high-affinity neuroligands (“decoy molecules”), as well as neutralizing “nanobodies” (single-domain camelid antibody fragment) are being developed. Simultaneous, more precise targeting of more than one cytokine is possible using bispecific agents (fusion antibodies). It is also possible to inhibit part of a signaling cascade to spare protective cytokine effects. “Fusokines” (fusion of two cytokines or a cytokine and CK) allow greater synergistic bioactivity than individual cytokines. Another promising approach is experimental targeting of the NLRP3 inflammasome, amply expressed in the CNS and a key contributor to neuroinflammation. Serendipitous discovery is not to be discounted. Filling in knowledge gaps between pediatric- and adult-onset neuroinflammation by systematic collection of CSF data on CKs/cytokines in temporal and clinical contexts and incorporating immunobiomarkers in clinical trials is a challenge hereby set forth for clinicians and researchers.
Introduction
The era of “biomarker-guided therapy” has introduced a paradigm shift in immunotherapeutics based on the biological make-up of the individual patient (1). Integral to the concept of personalized medicine or “precision medicine” is the use of molecular biomarkers to improve detection of neuroinflammation and predict drug benefit or adverse effects (2). Using the approach of a translation paradigm, it affords a rational alternative to traditional trial-and-error, broad-spectrum immunotherapy with its variable successes and failures. Patients with neuroinflammation are extremely heterogeneous, more than clinical phenotypes suggest, and non-targeted interventions using agents that “should work” for the disease, often miss their mark. Other clinical challenges include access and use of more effective methods to diagnose immune dysregulation and neuroinflammation (3), recognizing sustained subclinical disease activity that can foster disease progression (4), and understanding and predicting or preventing relapse. The biomarker-guided approach helps advance preclinical studies to clinical proof-of-concept studies in targeted drug development (2).
Because of cerebrospinal fluid (CSF) proximity to the inflammatory process in brain parenchyma, CSF biomarkers are highly sought in a panoply of disorders with primary or secondary neuroinflammation (3), and those, not abundantly researched blood markers, are not covered here to delimit the scope. Immune cell-specific markers measured through immunophenotyping have greatly aided detection of the cellular “players” in intrathecal inflammation (5), but inflammatory mediators are also a major part of the dynamics in directing the cell to action and orchestrating immunologic contexts (6). Chemokines (CKs) and other cytokines comprise the major inflammatory mediators, and many are detectable in CSF and qualify as biomarkers that delineate the inflammatory process (7). Breakthrough technological advances have led to the discovery of new molecular entities and made it easier for research labs to measure not just one, but panels of inflammatory mediators simultaneously in a single sample (3, 8, 9). Systematic measurement of CKs and non-CK cytokines would foster the goals of early and maintained targeting of inflammatory mediators and biomarker-guided initiation and monitoring of a drug.
This article is a conspectus on targeting CKs and other cytokines and their receptors or administering them therapeutically. The burgeoning field is so enormous, the review cannot be all inclusive, nor can it keep up with the daily publications and treatment updates. Instead, it purveys selected information considered necessary to consider targeted therapies in paradigmatic diseases and interpret neuroinflammation’s mosaic of clinical facades. Some biomarker-guided immunotherapies for unrelated human disorders are interweaved historically with those used for neuroinflammation or hold such potential for future applications to neuroinflammatory disorders, so they are covered here selectively. Certain other areas are only lightly touched upon or not covered. Extensive information on chemo/cytokines and signal transduction pathways already has been comprehensively reviewed elsewhere (6, 7, 10–14). Neurodegenerative disorders in adults, most of which do not overlap with children, were considered outside the scope. The field of psychoneuroimmunology is rapidly developing, but the article focuses primarily on neuroimmunologic conditions and neuroimmune pharmacology. A highlight of the review stems from the statistic that global central nervous system (CNS) biomarker markets were estimated to increase to $3 billion by 2015, yet most of the biomarker research has been on adult-onset neuroinflammatory disorders, not necessarily providing insight into the disorders that afflict children. Therefore, studies on both pediatric- and adult-onset neuroinflammatory disorders are included herein, with the hope of achieving a balanced view.
The layout of the review reflects its neuroimmune pharmacology orientation. “Cks/Cytokines and Their Receptors” provides a summary of the science behind inflammatory mediator targets; “Comparison of csf ck/Cytokine Immunomarker Profiles in Human Neuroinflammatory Disorders” presents CSF data on target measurements in human disease; “Targeting CKs or Other Cytokines” describes targeted clinical trial experience and ongoing trials; “Considerations in Designing Future Clinical Trials for Neuroimmunologic Application” discusses strategizing about next steps and future goals.
CKs/Cytokines and Their Receptors
Non-CK Cytokines
Cytokines comprise 300 soluble low-molecular-weight proteins or glycoproteins, such as interleukin (IL), interferon (IFN), tumor necrosis factor (TNF), colony-stimulating factors (G-CSF, GM-CSF), and other growth factors [tumor growth factor (TGF)] (6, 15). They perform various functions in the immune system in both health and disease. Non-CK cytokines are structurally and functionally diverse, with the largest group having a monomeric alpha structure (IL-2 and IL-4) and the smallest group, a beta structure (IL-1 and IL-18); others may be heterodimeric (IL-12, IL-23, and IL-23). They regulate lymphocytes (IL-2, IL-4, IL-10, and TGF-β), natural immunity (TNF-α, IL-1β, IFN-β, IL-5, IL-10, IL-12, and type 1 INF), and activate inflammatory cells [interferon-gamma (IFN-γ), TNF-α, TNF-β, IL-5, IL-10, and IL-12] (6).
Listing of “pro-inflammatory” vs “anti-inflammatory” is useful but not entirely straightforward, given that cytokine effects may differ depending on the microenvironment. Pro-inflammatory cytokines typically include TNF-α, IFN-γ, IL-1β, IL-2, IL-6, IL-8, IL-12, IL-17, IL-18, IL-23, and IL-36 (16–18). However, TNF-α can also function physiologically at brain synapses (7). Anti-inflammatory/immunoregulatory cytokines encompass IL-4, IL-5, IL-10, IL-13, IL-35, and IL-37 (19, 20).
The TNF cytokine superfamily is one of the largest family of cytokines, consisting of 19 cytokines and almost 30 receptors (21). The cytokines include TNF-α, TNF-β, OX40L, toll-like factor (TL1A), GITRL, TWEAK, RANK1, lymphotoxin (LT-α and LT-β), and many others. B cell-activating factor (BAFF), also called TNF ligand 13B, and a proliferation-induced ligand (APRIL) are members of the TNF family (22).
The IL-1 family includes inflammatory cytokines (IL-1α, IL-1β, IL-18, IL-33, and IL-36), an anti-inflammatory cytokine (IL-37), and receptor antagonists (IL-1Rα, IL-36Rα, and IL-38) (19). The IL-10 family also includes IL-22 (23). The IL-12 family comprises IL-12, IL-23, IL-27, and the anti-inflammatory cytokine IL-35 (20). IL-8 is also CXCL8 and belongs to a CK family (24). The IL-17 superfamily comprises IL-17A to IL-17F, and IL-17 plays a role in autoimmune diseases.
The cytokine network exhibits intriguing properties. It enables chemotaxis through formation of concentration gradients, has pleotropic effects (pleiotrophy) and has redundant effects (such as two different cytokines activating a particular cell type) (8). They may be synergistic or antagonistic. Some cytokines affect B cell differentiation and proliferation (IL-2, IL-4, IL-5, IL-6, IL-10 TGF-β, and IFN-γ). Others trigger activation of effector cells, such as CD8+ cytotoxic T cells (IL-2, IL-6, and IL-12) and natural killer (NK) cells (IL-2 and IL-12). Most cytokines act in the local milieu and are short lived. By contrast, M-CSF and TGF-β are able to act at a distance. IL-1α and IL-33 are preformed and released in the presence of tissue injury, therefore acting as alarmins.
There are three classes of IFNs: type 1 IFN (α and β), type 2 IFN-γ, and type 3 IFNs. Besides their role in antimicrobial defenses, IFNs activate macrophages and NK cells, an immune function.
The cellular source of non-CK cytokines provides a functional grouping. Cytokines of adaptive immunity are secreted predominantly by various T cells (Th1, Th2, and NKT cells), but also NK cells. Cytokines of innate immunity are produced primarily by macrophages, but also by dendritic cells (DCs), some T cells, and endothelial cells (25). Inflammasome activation is necessary for production of IL-1β and IL-18 (26). Because of the multiplicity of cellular sources of cytokines, the terms monokines (monocyte/macrophage-derived) and lymphokines (lymphocyte-derived) are less dichotomizing.
Cytokine Receptors (CRs)
Cytokine receptors, which are divided into several families, reside on target cells and mediate cytokine actions (26). Of these, the cytokine R type 1 and type 2 receptor classes bind the most non-CK cytokines (27). Type 1 CRs are typified by IL receptors; type 2, by IFN receptors. Type 1 and type 2 CRs are distinguished by unusual properties. One is the existence of soluble and decoy receptors, which bind cytokines without triggering signaling. Another is production of endogenous selective antagonist molecules, denoted as IL-1Ra, IL-31Ra, and IL-36Ra, which also bind to CRs and prevent signaling. There are also CR subfamilies: IL-12R is a subfamily of the IL-6R family (Table 1) (26).
Mature B cells express the CRs BAFF-R (highest affinity binding for BAFF), B cell maturation antigen (BCMA) or BCMA (highest affinity binding for APRIL, no BAFF binding), and transmembrane activator and calcium modulator and cyclophilin ligand interactor or TACI (BAFF and APRIL are both ligands) (28, 29).
Cytokine receptors may be soluble or cell membrane bound. Soluble CRs compete with cellular receptors for cytokine binding. sIL-27Rα, a naturally occurring soluble form of IL-27Rα, is an IL-27 antagonist produced by activated T cells, B cells, and myeloid cells (30). The soluble class II CR IL-22RA2 is a naturally occurring IL-22 antagonist (31). In the IL-1 cytokine family, there are naturally occurring inhibitors, such as IL-1 antagonists of IL-1R (19). There is also a soluble form of IL-6R resulting from cleavage by metalloprotein ADAM17 (32). There are also endogenous soluble receptors for TNF, IFN-γ, IL-2, and IL-4.
The TNF receptor family includes TNFR1 and TNFR2 receptors. TNFR1 is expressed on all nucleated cells, whereas TNFR2 is chiefly restricted to immune cells. Both membrane and soluble TNF-α activate TNFR1; TNFR2, mostly by mTNF-α (21). Genetic mutation of the TNF-R extracellular domain results fever and inflammation associated with TNF receptor-associated periodic syndrome.
The IFN receptor family includes receptors for type 1 (α, β, κ, and ω) and type 2 (γ) IFNs (26). The IFN-α receptor has 13 subunits: α1, α2, α4, α5, α6, α7, α8, α10, α13, α14, α16, α17, and α21 (26). IFN-R type 2, a decoy receptor, binds IL-1α, IL-β, and IL-1Ra (26).
Chemokines
Chemokines or “chemoattractive cytokines” are secreted proteins that govern leukocyte trafficking and positioning into targeted organs (33). They are well suited as biomarkers because they are most prevalent of human cytokines, have good performance characteristics, and can be correlated with clinical observations. Some are normal CSF constituents, while others are expressed in inflammation.
The newer structure-based nomenclature for CKs (34) has largely overtaken the older, descriptive nomenclature since introduced in 2000, but either are still used in the medical literature and can be compared (Table 2) (35). The naming comprises a prefix and number ID. CXCL10, for example, replaces “IFN-γ-inducible protein 10” (IFN-γ secretion is IL-12-family mediated) (36). C–X–C replaces the name α CKs; C–C, β CKs; C, γ CKs; and C–X–3C, δ CKs. CK ligands and their receptors comprise axes for chemotaxis of specific cell types.
C–C ligands (1–28), the largest group of CKs, correspond to C–C receptors (CCRs), just as C–X–C CKs (1–17), the second largest CK subgroup, do to C–X–C receptors (CXCRs), and there is functional relatedness between subgroup ligands (7). XCL (two types) and CX3C (one type) are smaller ligand subgroups. Different CKs are involved in the trafficking of different kinds of immune cells, such as neutrophils (CXCL1–8), monocytes (CCL1–5, 7, 8, 13–16, 18, 23), T cells (CXCL9–11), B cells (CXCL13, CXCL10), and monocytes/T cells [C–X–3C chemokine ligand motif (CX3CL1) or fractalkine] (22). CKs also have been stratified as to whether they are lymphoid CKs (CXCL12, CXCL13, CCL19, and CC21) or inflammatory CKs (CCL2, CXCL9, CXCL10, and CXCL11) (9).
“Homeostatic” CKs (CCL14, CCL19, CCL20, CCL21, CCL25, CCL27, CXCL12, and CXCL13) are also involved in the maintenance of homeostasis (12). During brain development, migration, proliferation, and differentiation of neural progenitor cell migration is guided by CXCL12 and CXCL14. Immunosurveillance involves immune cell trafficking in response to CCL2, CCL19, CCL20, CCL21, and CXCL12 (12). CX3CL1, which is expressed on neurons, is pivotal to maintenance of immune quiescence; the receptor is present in the CNS only on microglia (7). “Mucosal CKs” encompass CCL25, CCL28, CXCL14, and CXCL17 (37). “Angiogenic CKs” comprise many of the CXC CK family, such as CXCL1 through CXCL17 (38).
Chemokine Receptors (CKRs)
Classical or typical CKRs are seven membrane G protein-coupled-receptors (GPCRs), which have been the most successful target class for drug discovery (39). Their expression may be constitutive or inducible. They participate in ligand–receptor pairs or axes, with CKs functioning as receptor agonists (full, partial, and inverse) or antagonists as standardly defined (40). There are “major” and “minor” receptor “pockets” to which CKs bind, resulting in different functionalities. The domain of CKs comprises roughly 50 peptides and 20 receptors in humans (11), indicating that several ligand–receptor relationships are not exclusive (“promiscuous”). CKRs for inflammatory CKs are especially promiscuous, some lacking an endogenous CK (41). Those that are exclusive involve “cognate” receptors. Most CKs signal through GPCRs, targeted by about 30% of current pharmaceuticals, which have been called the most “druggable” receptor class (42). The repertoire of receptor expression depends on the type of leukocyte and its maturational stage (42). The receptors may bind CKs which exhibit opposite roles: CXCR7 binds CXCL12 and CXCL11 (39). While CKR groupings may be cell specific, CXCR4 is expressed by all immune cells. Collectively, these factors confer the differential responsiveness of target cells.
Recently, “atypical chemokine receptors” (ACKRs)—without GPCR signaling or chemotactic effects—that scavenge CKs at inflammatory sites have been described (43). ACKRs, formerly termed “decoy” or “scavenging” receptors based on their capacity for sequestering cognate CKR ligands, are important for CK regulation (44). They were also referred to as “silent” receptors, based on lack of classic CKR signaling and functional activities like directly inducing immune migration of immune cells (45). ACKRs are now conceptualized as “endogenous β-arrestin-biased signaling receptors” and bind multiple CKs (45). Most ACKRs are expressed on vascular endothelial cells; some have additional strategic locations: Purkinje cells (ACKR1), B1 B cells (ACKR2), and neurons (ACKR3) (44).
Most typical human CKs (C–C or C–X–C) are CKR agonists according to the IUPHAR classification (Table 3) (41, 42). A promiscuous CK may function exclusively as an agonist at more than one CKR, such as CCL2 (CCR2, CCR3, and CCR5); only as an antagonist at more than one receptor, such as CCL18 (CCR1 and CCR3); or as an agonist at one receptor and an antagonist at another, such as CCL4 (agonist at CCR5, antagonist at CCR1) (Figure 1). Certain CK ligands are agonists at both typical CKR and ACKR, such as CCL19 (CCR7, CXCR3, and ACKR4) or CXCL12 (CXCR3, CXCR4, and ACKR3). Receptor conformations on leukocytes are particularly important, potentially resulting in blocking signaling by one conformation but not the other. CCL17 and CCL22 both bind to CCR4, but to different conformations, although both can evoke CCR4 internalization (46). Many ACKRs are not yet specified as to agonist/antagonist status.
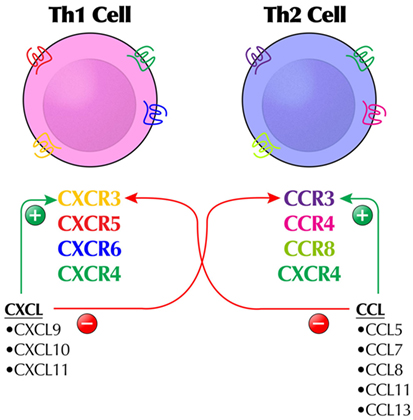
Figure 1. The complex regulation of the chemokine (CK) system by a combination of agonist and antagonist activity illustrates why some CKs or chemokine receptors are not easy targets for immunomodulation and how different immunologic outcomes may occur. For example, CXCL9–11 are agonists at CXCR3 (expressing Th1 cells), but antagonists at CCR3 expressed by Th2 cells. Likewise, CCL5, CCL7, CCL8, CCL11, and CCL13 are agonists at CCR3-expressing Th2 cells. The typical stylized receptor depiction is meant to show the extracellular and intracellular domains of the receptor, which is a seven-transmembrane receptor.
The concept that CKRs may serve as biomarkers in neuroinflammatory disorders has been proffered (47). CK/CR-expressing immune cells can be measured ex vivo through flow cytometry (5). T cells in the CSF express a delimited array of inflammatory CKRs: enrichment of CCR5, CCR6, CXCR3 (little CCR1, CCR2, and CCR3) independent of the presence of neuroinflammation (48). The cellular and spatial distribution of CKRs in the brain has been reviewed (49). CXCR2 (and ligand CXCL1) plays a developmental role in gliogenesis (7). Ties between the nervous and immune systems are well illustrated by the fact that gamma-aminobutyric acid (GABA)-A and GABA-B agonists/antagonists are allosteric modulators of CXCR4.
Cellular Sources of Chemo/Cytokines: Leukocyte Trafficking and Positioning
Because excessive recruitment of leukocytes to inflammatory sites is a hallmark feature of inflammatory disorders, specific inhibition of this recruitment would be an ideal anti-inflammatory strategy.
The initial approach to the study of leukocyte trafficking to the CNS is descriptive analysis of CKs in CSF (and peripheral blood), and CKRs expressed by cells circulating in these compartments (Table 4) (6, 7, 10–13, 25, 33, 34, 39, 47, 49–52). Hematogenous cells may enter the CNS through different barriers, such as blood–CSF and blood–brain (7). In neuroinflammatory disorders, pro-inflammatory cytokines are mainly secreted by invading leukocytes, whereas in neurodegenerative diseases they are produced principally by CNS-resident cells (innate immunity) (6). The process is dynamic: immune cells follow the gradient of CKs (also called “chemotactic factors”). CK concentrations in circulating blood are miniscule (10−12 M), but can increase 1,000-fold. Immune cells upregulate or down regulate their CKRs accordingly.
“Conversing” or signaling between T cells infiltrating the CNS and resident microglia, astrocytes, and other members of the innate immune system is important to the discussion of cellular sources of chemo/cytokines (25). CNS immunity has both afferent and efferent limbs. DCs, part of innate immunity, are components of the afferent limb, while central-memory T cells, entering the CSF from the circulation execute the efferent limb (53).
The response of the immune system to injury is sequential. Alarmin release and glial activation is followed (in minutes to hours) by inflammasome activation, cytokine production, and neutrophil recruitment, infiltration of monocytes (hours to days post injury), then recruitment of adaptive immune cells (days to weeks post injury) (54).
Infiltrating Immune Cells
T cell subsets produce “signature cytokines” in inflammatory disorders (55). CD4+ lymphocytes comprise effector and regulatory cells. While classic T helper (Th) cell Th1 and Th2 phenotypes have been long studied, effector CD4+ T cells can be further classified based on immunological function and other characteristics. Th1 and Th17 cells play a role in neuroinflammatory disorders; Th2 cells, in allergy (55). However, the plasticity of Th17 cells allows them to shift toward a Th1 or Th2 phenotype, displaying more aggressive or pathogenic actions than unshifted cells (56). Th1 cells (IFN-γ and TNF) are largely responsible for cellular immunity; Th2 cells (IL-5, IL-5, and IL-10), for humoral immunity. Th0 cells may also differentiate into Th17 cells (IL-6 and IL-17), Th22 cells (IL-22), Th9 cells, and follicular helper cells (Tfh) (21, 56). Atypical T cells include gamma/delta T cells and natural killer-like (NKT) cells. In the CNS, γδ T cells comprise two subsets with opposing roles. The Vγ4+ subset secretes IL-17 and other pro-inflammatory cytokines, whereas the Vγ1 subset produces CCR5 ligands (57).
B cell trafficking, differentiation, and survival have been better understood as a result of clinical application of anti-B cell monoclonal antibodies to human neuroinflammatory disorders, establishing a role for B cells in many, such as multiple sclerosis (MS) (40, 58). CXCL13, the preferential chemoattractant for B1 cells, plays a particular role in B cell trafficking and homing, and its CSF:serum ratio is a measure of chemotactic gradient (58). CXCR5, the cognate receptor for CXCL13, is expressed on almost all B cells, more so on B1 (T cell-independent) than B2 (T cell-dependent) cells. CXCR5 is also expressed by follicular helper CD4+ T cells (Tfh), which provide T cell help to B cells (59).
Dendritic cells, the most powerful professional antigen-presenting cells, play a pivotal role in initiating and perpetuating CNS-directed autoimmune responses involving B and T cells (60). The CNS lacks resident DCs, thereby conferring “immune-privilege” (7), so they operate at the interface of the adaptive and innate immune systems. CCR7 controls the cytoarchitecture, rate of endocytosis, survival, migratory speed, and maturation of DCs, modulating the immune response by regulating different functions of DCs. An important ligand for CCL7 is CCL21. DCs are a major source of CCL22 and CCL17. Also, activated T cells strongly upregulate CCR7 and migrate to CCL19.
Natural killer cells also use CSF as an intermediary compartment for trafficking into the CNS and for differentiation (61). CSF NKs exhibit reduced CX3CR1 expression compared with blood NKs. In blood, CD56dim CD16+ cells, which are cytotoxic and secrete more cytokines in response to IL-2, predominate. CKs play an important role in the phenotype and functionality of resting (CD56dim) vs activated (CD56bright) NK cells (62).
Monocytes invading the CNS transform into inflammatory macrophages or DCs (6). The chemoattraction of monocytes to inflammatory sites owes to their expression of CCR2 and corresponding ligand CCL2 (63). Monocytes, macrophages, and DCs together comprise the mononuclear phagocyte system (6).
Other cells include mast cells, first responders able to initiate and amplify CNS immune responses and secrete cytokines, and neutrophils (especially in bacterial meningitis), but will not further discussed.
Various infiltrating immune cells participate in lymphoneogenesis in the CNS. The CXCL13/CXCR5 ligand/receptor pair (like CCL21/CCR7 and CXCL12/CXCR4) has the capacity to induce the ectopic lymphoid-like tissue, common to chronic autoimmune diseases, thereby maintaining B and T cells in lymphocytic infiltrates. Inflamed brain can become a niche for B cell survival and proliferation through BAFF and B cell-activating factor receptor expressed on B cells. Chemo/cytokines linked to lymphoid neogenesis in the CNS include CXCL13, CXCL12, CXCL10, IL-6, and IL-10 (64).
Innate Immune Cells
The concept of bidirectional neuron–glia communication via CKs and other cytokines, one the one hand, and neurotransmitters and neuropeptides, on the other, is central to understanding physiological functionalities and pathological distortions. Certain inflammatory CKs cause synaptic hyperexcitability by altering glutamatergic and GABAergic neurotransmission and affect neuroplasticity (65).
Central nervous system-resident cells (neurons, astrocytes, microglia, oligodendrocytes, and endothelial cells) are sources of CKs (6), as demonstrated in postmortem studies of MS. Non-microglial myeloid cells include macrophages (choroid plexus, perivascular, and meningeal) and DCs (6). Two important categories of cell signaling (cross talk) are between neurons and microglia and between microglia/astrocytes and CNS-infiltrating cells (25).
Astrocytes, the most abundant non-neuronal cells in the brain, interact with neurons in brain development and function, providing anatomic support, such as end feet surrounding capillaries in the BBB, and physiologic support, including modulation of synaptic activity and blood flow, and regulating water, potassium, and glutamate in the CNS (25). As to the immunologic functions, CCL2/CCR2 is important for astroglial–neuronal signaling. CXCL12 and CX3CL1 (exclusively on microglia and astrocytes) are constitutively expressed. CCR3, CCR5, and CCR7, as well as CXCR2, CXCR3, and CXCR4 are constitutively expressed by astrocytes. In response to injury, astrocytes can secrete CXCL10 and BAFF, but also anti-inflammatory cytokines like IL-10. CX3CL1 and IL-34 are neuronally produced “help me” signals (42). In MS plaques, BAFF, CCL2, and CXCL10 are upregulated near hypertrophic astrocytes (50, 66). In surgical specimens of children with Rasmussen encephalitis (RE), neurons and astrocytes in damaged areas expressed CXCL10, and cytotoxic infiltrating T cells in the same area expressed CXCR3 (67). Astrocytes are the major source of IL-6 in the CNS and may help regulate synaptic function (68).
Microglia are the only lifelong resident immune cells of the CNS (25). Microglia precursors migrate early from the yolk sac to colonize developing brain (69). Other glial cells are neuroectoderm derived (51). High, not low, levels of CXCL12 activate CXCR4 on microglia, initiating TNF-α release, which causes astrocytes to release glutamate (excitotoxicity). CX3CL1/CX3CR1 is key to neuronal–microglial signaling after injury: neurons secrete CX3CL1, but CX3CR1 is expressed only on astrocytes and microglia. Microglia are activated by chronic neurodegeneration and systemic inflammation (25). Microglial–astrocyte interactions are vital to innate immunity in the CNS. IL-6R is present in microglia, not astrocytes (70).
Activated astrocytes and microglial cells release neuroactive “gliotransmitters” via exocytosis, akin to neurotransmitter release from nerve endings (71), and alter neuronal chloride (72). They modulate blood–brain barrier permeability and BBB-mediated neuroinflammation and affect cell-to-cell communication (73). Gliotransmitters include GABA and taurine (inhibitory gliotransmitters) (72), as well as glutamate, d-serine, ATP, and l-lactate (71).
Macrophages derived from invading monocytes are the predominant cell type presenting antigen to infiltrating T cells during neuroinflammation (6). Myeloid cells are the principal source of cytokines influencing T cell effector functions (74). The M1/M2 macrophage classification may not apply to CNS resident macrophages (6).
Endothelial Barriers
The homeostatic CKs expressed on the endothelial barriers include CXCL12, CCL19, CCL20, and CCL21 (12). DCs are proximal to the choroid plexus and meningeal blood vessels. The endothelium of the blood–brain barrier expresses CXCR4 and CXCR7, a scavenger receptor for CXCL12. CKs are presented on endothelial cells via binding to glycosaminoglycans (GAGs), which is required for leukocyte migration. CNS barriers have been reviewed extensively (10, 12, 25).
Signaling and Transduction Mechanisms of CK/Cytokine Actions
Intracellular chemo/cytokine signaling cascades are the focus of intensive research. As GPCRs, CKR couple to transducers, such as heterotrimeric G proteins and β-arrestins, which then engages intracellular signaling cascades (75). These result initially in desensitization and receptor internalization. The subsequent intracellular signaling cascade may involve activation of nuclear factor kappa-light-chain-enhancer of activated B cells (NF-κB), extracellular signal-regulating kinase (ERK), mitogen-activated protein kinase (MAPK), extracellular signal-regulated kinase (ERK), phosphatidylinositol-3 kinases, Janus kinase (JAK)/signal transducer and activator of trascription (STAT), or other proteins (76). Transcription of genes encoding IL-1 family cytokine precursor proteins (pro-IL-1β and pro-IL-18) occurs in response to tissue injury or infection, resulting in inflammatory cytokine production via the inflammasome (25). IL-1β is activated when enzymatically cleaved by activated capsase-1. Signaling and transduction involves JAK1, 3/STAT5 for IL-2; JAK2/STAT5 for IL-3, and glycoprotein 130 (gp130)/JAK/STAT for IL-6 (26). Not all chemo/cytokine signal transduction results in inflammation. A case in point is IL-37, a natural suppressor of inflammatory responses, which transduces anti-inflammatory signaling via suppression of NF-κB and MAPK (77). New therapeutic strategies may capitalize on the negative regulation of the NF-κB pathway (78). Biased signaling (or biased agonism) refers to the capacity of CKR to activate a particular signaling pathway from among others in a biased manner. Further commentary is deferred to other reviews (6, 7, 12–14).
Comparison of CSF CK/Cytokine Immunomarker Profiles in Human Neuroinflammatory Disorders
Strategizing how to target CKs and other cytokines relies on knowing which, if any, are part of the intrathecal immune response in a particular disease. The unique modality of profiling CSF CKs and other cytokines reveals the presence of immune dysregulation, or disturbed homeostasis (33). Proper evaluation of the CSF depends on reliable measurement of the biomarkers, normal ranges per patient age, and knowledge of the test limitations. First, it should be recognized that not all cytokines are equally detectable (Figures S1 and S2 in Supplementary Material and its legends). In CSF, CKs are found in greater concentrations than other cytokines. CXCL10 had the highest concentration, and CCL2, CXCL10, and IL-6 were detectable in all samples (35). Other CSF cytokines also are measurable, but their concentrations are extremely different. In children with non-inflammatory neurological disorders (35), the percent detectability was highest for IL-16, IL-1Ra, and IL-6, declining sharply for the others. Calculation of the CSF/serum or CSF/plasma chemo/cytokine gradient is not always reported, yet it is crucial for making inferences about intrathecal production and CNS compartmentalization of the immune response (79–81).
Disease Effects on Individual CSF Immunomarkers and Group Patterns in Non-Infectious Disorders
With the methodological advent of simultaneous measurement capability of multi-analyte CKs/cytokines in a small volume of CSF obtained by lumbar puncture (LP), there are now more studies of soluble CSF CKs/cytokines in various neuroinflammatory disorders (Table 5) (3, 9, 16, 17, 50, 52, 59, 79–121). Many are disorders studied in adults, but there has been an upgrowth of pediatric investigations, and with them the increasing availability of pediatric reference ranges (35, 52). Cameos of the pediatric-onset disorders can be found in the Table S1 in Supplementary Material.
Aggregate observations culled from the table provide evidence of dysregulated expression of CNS CKs and other cytokines and indicate several trends. First, CSF IL-6 was increased in all the presented disorders, and CXCL10 and CXCL13 were found to be elevated when they were measured. Second, both pro-inflammatory and anti-inflammatory cytokines (IL-10, IL-4, IL-5, and IL-13) were present in most disorders studied, but the IL-10 concentration was reduced in the degenerative disorders spinocerebellar atrophy (SCA) and MSA-C. Third, most intrathecal inflammatory mediators were found to be increased; however, concentrations of a few were decreased or unchanged. The concentration of CXCL12 was decreased in pOMS, N-methyl-d-aspartate receptor (NMDAR) encephalitis, pAnti-Hu/ANNA-1 paraneoplastic syndrome, and neuromyelitis optica (NMO), but increased in pMOG, adult AE, and neuropsychiatric systemic lupus erythematosus (NPSLE). The decrease may reflect CXCL12 relocation to the blood–brain barrier, decreased production, or increased consumption (81). CSF TNF-α was decreased in NPSLE, normal in pOMS, and increased in several other disorders. Fourth, while inflammatory markers raged in CSF, serum concentrations did not reflect it in NPSLE (106), pOMS (79–81), or stroke (112). Fifth, other than for MS, there have not been multiple studies of other disorders to compare with each other. One line of evidence that these immune mediators are operatives in MS is the marked decline in CSF concentrations of CKs (CXCL9, CXCL10, CXCL11, and CCL22) and other cytokines (IL-1β, IL-6, and IL-8) following natalizumab treatment (123).
Cerebrospinal fluid abnormalities listed in the “Other” column warrant mention, although data were fragmentary due to not being systematically measured, thereby limiting between-disease comparisons. Inflammatory cytokines prevailed: INF-γ [acute disseminated encephalomyelitis (ADEM), pADEM, AE, pAE], IL-12 [relapsing–remitting multiple sclerosis (RR-MS), chronic inflammatory demyelinating polyneuropathy (CIDP), pTBI, SCA, MSA-C, G-CSF (NMO, ADEM, and NPSLE), and GM-CSF (stroke, NPSLE, and SCA). The concentrations of BAFF and APRIL were mostly congruous, both usually being elevated (NMO and NPSLE), with a few notable exceptions (pOMS). The only two elevated CKs were CCL19 (RR-MS, pMOG, pADEM, and CIDP) and CCL2 (acute demyelinating polyradiculoneuropathy, NPSLE, CIDP, metachromatic leukodystrophy, and adrenoleukodystrophy).
There are also quantitative differences in the concentration of a particular CK/cytokine between neuroinflammatory disorders, best appreciated when more than one disorder is compared in the same study. Usefulness of CSF CK quantitation as a diagnostic aid in children with neuroinflammatory disorders has been advocated (124); however, no attempt was made here to denote quantitative differences between studies, because differences in biomarker sensitivities related to kits and laboratories would confound the comparison. The heterogeneity, variability and inconsistencies of chemo/cytokine concentrations in different studies in the same neuroinflammatory disorders may make it difficult to corroborate results and see unifying patterns due to variable severity of diseases, timing of CSF samples, prior treatment, and different assay methods. Some markers are investigated only sporadically.
Concentrations of immune markers may or may not correlate with those of diagnostic autoantibodies or CSF oligoclonal bands. CSF IL-6 correlated with anti-myelin oligodendrocyte glycoprotein (MOG) antibody titers (86), but only weakly with anti-aquaporin-4 antibodies (88). CSF IL-6 concentration, which is increased in monophasic acquired demyelinating syndromes more than in MS, correlated with plasma MOG antibodies (86). In anti-NMDAR encephalitis, elevated CSF CXCL13 correlated with intrathecal NMDAR-antibody synthesis (96).
Cerebrospinal fluid CKs/cytokines that correlate with disease severity or outcome are perhaps the most promising from the standpoint of clinical immunotherapeutics. In pOMS, CSF CXCL13 concentration correlated with motor severity and disease duration (81). In severe pediatric traumatic brain injury (TBI), CSF cytokines/CK concentrations did not correlate with the initial score on the Glasgow Coma Scale or outcome (114).
Various combinatorial panels of immunobiomarkers have been proposed as best able to reveal active intrathecal inflammation. One is the combination of CSF IL-8, IL-12p40, and CXCL13 (3). Another is TNF-α, IFN-γ, IL-6, IL-10, CXCL10, and CXCL13 (52). The combination of IL-2, IL-17, IFN-γ, IL-5, FGF-basic, and IL-15 was able to distinguish NPSLE from MS and NMO (107). Given the potential combinatorial diversity, other panels are being explored.
Other small studies also have been reported, but with pitfalls in interpretation (1). In a study of Aicardi–Goutières syndrome (AGS), multiple CSF cytokine and CK abnormalities were shown (125), but would not have been significant if correction for multiple comparisons had been made. In complex disorders, there may be a need for multiple active controls, such as seizures, movement disorders, fever, which may not be provided. Or the active controls may be provided, but without passive controls (102). Studies of one disease compared with another without passive controls (126) can only be interpreted in light of reference ranges that share similar methodology. Immunobiomarker studies in uncommon disorders are challenging but critical.
It is customary to differentiate “primary” vs “secondary” neuroinflammatory disorders, but the defining line is blurring. Comparison of the CSF profile of TBI or ischemic stroke, in which neuroinflammation is secondary, with RR-MS, in which neuroinflammation is presumed to be primary, is not revealing of the difference, based at least on the markers studied. More autoantibody-mediated neuroinflammatory disorders are being identified in the aftermath of CNS infections. Also, neuroinflammation may lead to neurodegeneration (66). Leukodystrophies are the most common cause of pediatric neurodegeneration (127), and in one of them (Krabbe disease), recent studies show that immune activation precedes clinical symptoms and pathology (127).
Cytokines and CKs also may aid in differential diagnosis of diagnostically undefined cases by revealing active intrathecal inflammation. One need involves being able to predict whether the clinically isolated syndrome (CIS) will progress to MS. CSF CXCL13 (128) and IL-12p40 (129) are characteristic of MS, not CIS. Subclinical central inflammation marked by CXCL8, but not IL-17, is also a risk for CIS conversion to MS (130).
Effects on CKR Expression
Cerebrospinal fluid CK/cytokine perturbations are best interpreted with knowledge of corresponding receptor expression on immune cells. This requires flow cytometry on freshly collected immune cells, a different technology than the immunomarker kits, so is less often performed. CKR profiling (Table 6) (48, 53, 80, 81, 131–133) has revealed several active CK ligand–receptor axes involving T cells and B cells in MS and pOMS. For example, the significance of an elevated concentration of CXCL13 is interpretable with the finding that memory B cells expressing the cognate receptor for CXCL13 (CXCR5) are expanded in frequency in the CSF.
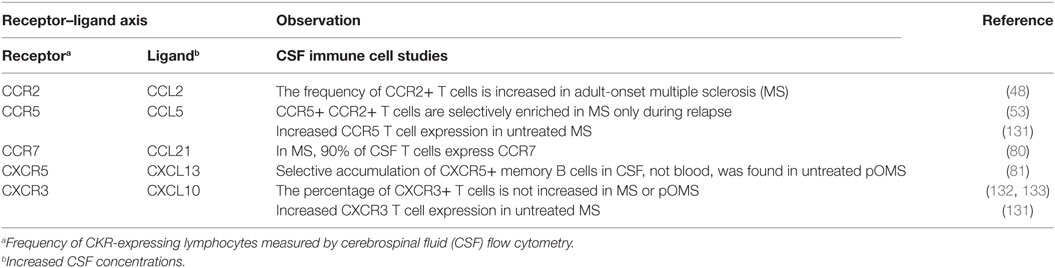
Table 6. Disease effect on chemokine receptor (CKR) expression in selected neuroinflammatory disorders.
CSF Immunomarkers in CNS Infections
From an immunological standpoint, non-infectious and infectious causes of neuroinflammation are separate, flip side considerations. However, a brief look at intrathecal inflammation in CNS infections affords the opportunity to compare the host inflammatory response to autoantigens vs exogenous microbial antigens (Table 7) (134–159) There were more similarities than differences, given increased CSF concentrations of IL-6, IL-8, and IL-10, but no clear split between bacterial vs viral diseases with the exception of subsclerosing panencephalitis, which stood apart (normal IL-10). The immunological mechanisms of bacterial meningitis (160) and viral meningoencephalitis (161) have been explicated.
Bacterial Infections
Cerebrospinal fluid cytokine profiling as a method of discriminating infectious agents and types of meningoencephalitis is gaining traction, especially in diagnostically uncertain cases. The very high concentrations of CSF CXCL13 have been suggested as a diagnostic marker of neuroborreliosis in adults (139) and children (142) and are associated with intrathecal synthesis of borrelial antibodies (162). They are also quite high in neurosyphilis (145), which is also spirochetal. In bacterial meningitis, CSF cytokine profiling may add discriminating value in the differential diagnosis of culture negative cases (134, 163, 164). CSF IFN-γ concentration alone, not 11 other cytokines, was higher in pneumococcal meningitis than meningococcal meningitis (165). In a comparative study of Streptococcus pneumonia, Neisseria meningitis, and Hemophilus influenza, pneumococcal meningitis was associated with higher CSF IFN-γ and CCL2 concentrations (166).
Viral Infections
As to viral encephalitides, increased CSF IL-6, IL-7, and IL-13 may differentiate viral encephalitis from autoimmune NMDAR encephalitis in children (150). CNS Dengue virus and Japanese encephalitis virus infection in children share similar cytokine profiles (167). CSF CXCL8, CXCL9, and CXCL10 are elevated in herpes simplex herpes simplex virus (HSV) 1 encephalitis and HSV2 meningitis, whereas CXCL11 and CCL8 are elevated in HSV2 meningitis alone (151). Among viral CNS meningoencephalitides, enterovirus and lentivirus have comparably higher CSF IL-12 and IFN-γ concentrations, whereas arbovirus is associated with lower CSF cytokine concentrations than other viral etiologies (168). In mumps meningitis in children, the concentrations of CSF CXCL8, IL-10, IL-12, IL-13, and IFN-γ were higher than in other viral meningitides (153). In H1N1 influenza-infected children, only CSF CCL5 was increased in those with encephalopathy compared with uncomplicated influenza (169).
Usually, treatment of the pathogen causes the neuroinflammation to regress, except when a secondary delayed autoimmune process is in play. There is also research on manipulating chemo/cytokines to hasten recovery and improve outcome. The importance of these chemo/cytokines to neurological outcome has been demonstrated experimentally in transgenic knockout animals (170). Animals lacking IL-1β, IL-6, or IL-12 may be resistant to fatal encephalitis or seizures caused by encephalitis, or have reduced neurological severity scores (170).
Human immunodeficiency virus infection (HIV1 and HIV2) is germane here because its predominant route of entry to monocytes and CD4+ cells is via the CCR5 co-receptor (171), which can be blocked by “entry inhibitors.” The CCR5-negative phenotype is resistant to HIV infection. HIV1 shifts its tropism from CCR5 to CXCR4 when the disease progresses to AIDS (172). Other HIV strains may use the CXCR4 receptor for entry (172).
The viral story comes back full circle to autoimmunity in that viral infection and viral-induced immunity may initiate autoimmune disease (170) through various mechanisms, such as direct bystander activation, epitope spreading, molecular mimicry, and release of cryptic epitopes (173). NMDAR encephalitis with positive CSF autoantibodies may follow HSV encephalitis several weeks later (174). Whether immunotherapy can prevent the development of autoimmunity following HSVE requires further study (174). The concept of the “infectome”—referring to “…the collection of an individual’s exposures to infectious agents…”—was introduced to trace infectious triggers of autoimmunity and better the understanding of microbial/host interactions in autoimmune diseases (175).
Applicability of Translating Findings in Adults to the Pediatric Setting
A reasoned discussion should note similarities and differences between patterns in pediatric- and adult-onset neuroinflammatory disorders, but data are limited. In the neuroinflammatory disorders, there were more similarities than differences in the CSF immunomarker pattern: ADEM (agreement on four markers), AE (five markers), NPSLE (six markers). However, the patterns for other markers were not identical. For comparison of CNS infections, the same markers not being measured in various studies. These data emphasize the need to obtain more direct CK/cytokine profiling data from the pediatric populations to allow more comprehensive evidence synthesis.
Pediatric neuroimmunology poses many challenges to that aim which must be surmounted. There are ethical constraints on CSF access—no healthy volunteers. Hence, there are fewer control data and reference ranges. There are technical constraints of smaller CSF volume, although this is not a problem for simultaneous measurement of multiple CSF immunomarkers on small aliquots from CSF samples of 1 ml. Performance of an LP requires sedation in toddlers and young children to prevent contamination of CSF by blood introduced traumatically into the CSF and for compassionate care. More studies of inflammatory mediators have been done in disorders for which LP is a required diagnostic tool (e.g., CNS infections) than in those where it is viewed as optional. The culture of monitoring CSF biomarkers is perhaps less established in pediatric neuroinflammatory disorders. There are pharmacodynamic differences in children based on immune cell development, such as the greater expression of monocytes compared with lymphocytes in infants, which could affect expression of CSF chemo/cytokines. These obstacles, however, can and have been overcome by some researchers in particular disorders. Methodologies and opportunities need to be embraced by the larger pediatric neurology community (176).
Targeting CKs or Other Cytokines
Targeting Approaches: Which to Target and How Best to Do It
The ultimate therapeutic goal is to gracefully overcome maladapted, disease-producing biologic processes, induce remission, and re-introduce immune tolerance without toxicity (1). Consistent with the definition of immunotherapy, biological response modifiers treat disease through induction, enhancement, or suppression of an immune response. Immunomodulation, defined as modification of an immune system function or induction of immune tolerance through treatment, encompassing immunosuppression or immune potentiation. Various immunomodulators may play different roles in the three-pronged targeting of inflammatory mediators: induction, consolidation, and maintenance/post-consolidation.
From standard-of-care practice, it is already well known that CKs and other cytokines can be immunomodulated by certain powerful “broad-spectrum” biologicals, such as high-dose corticosteroids or corticotropin, which reduce elevated CSF CKs CXCL10, CXCL13 and the cytokine BAFF in pediatric-onset opsoclonus–myoclonus syndrome (OMS) (79–81). “Treat-to-target” strategies hope to avoid the systemic side effects of steroids. It is also known that pathogens manipulate the human immune system, such as encoding for CKs, CKRs, soluble proteins, or extracellular matrix-mimicking components (177). All of these decoy proteins prevent CK binding to GAGs and extracellular CK gradients that attract circulating immune cells or CK binding to its cognate receptor (177). The provocative question was posed: if pathogens can co-opt the immune system for their purposes, why can’t we do so for therapeutic reasons? (178). Multiple targeting strategies have been devised, exhibiting a wide spectrum of activity spectrum, inclusive of agonists, partial agonists, and antagonists (Figure 2) (41, 177). Technical details of pharmacologic modulation of CKR function are available elsewhere (172).
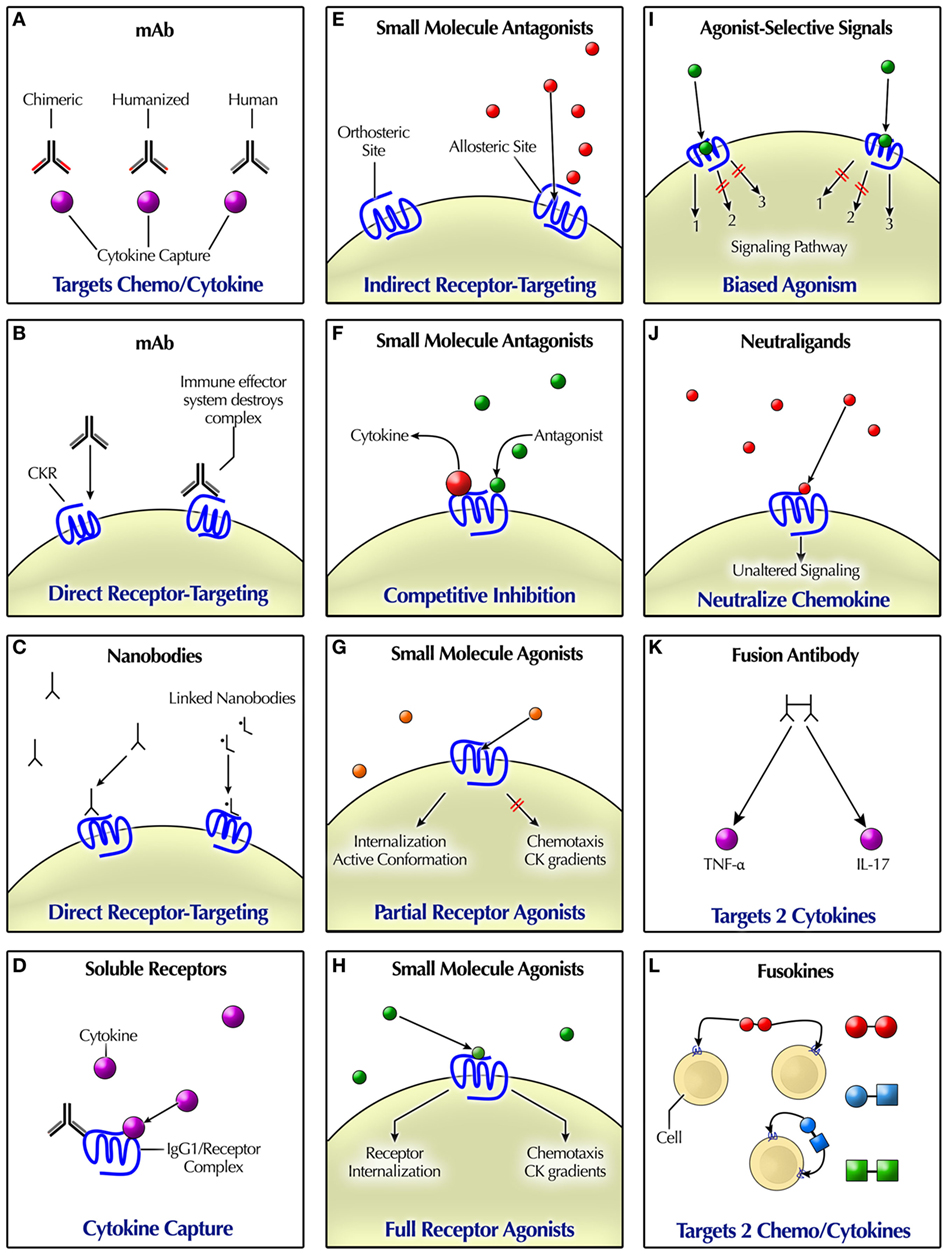
Figure 2. Modalities for pharmacologically targeting chemokine (CK)/cytokine ligands and receptors. (A) mAbs can bind to CKs or other cytokines. (B) Some mAbs mimic natural ligand binding, acting as agonists or antagonists. The effector immune system removes mAb-targeted cells. (C) Nanobodies are engineered “miniature” antibodies. (D) Soluble CKR may occur naturally, or they can be engineered as a recombinant fusion protein. Delivery of soluble receptors that bind ligands takes them out of play. (E) Indirect receptor targeting or functional antagonism refers to indirect antagonism, such as via binding to an allosteric site. By contrast, CKs usually bind at the primary (orthosteric) receptor site, indicative of a syntopic interaction. Some small molecule antagonists bind at an allosteric site. Allosteric agents may possess agonist, antagonist, or neutral effects. (F) Competitive inhibition or antagonism occurs when agonist and antagonist binding are mutually exclusive, such as if competing for the same binding site. Non-competitive antagonism refers to the occurrence of agonist and antagonist binding simultaneously. (G) Partial agonists have the capacity to produce some but not all of the effects of a full agonist. Their receptor binding may trigger receptor internalization, but perhaps not immune cell chemotaxis or a CK gradient. An inverse agonist reduces the number of receptors in active form. (H) A high-efficiency full agonist need only occupy a fraction of total receptors, leaving the rest “spare.” (I) Agonist-selective signaling may trigger different signaling based on biased agonism at the receptor. (J) Neutraligands bind to the receptor without altering cell signaling. Depicted are small molecules; however, neutral antibodies also exist. (K) Fusion antibodies are bispecific, designed to target two differernt cytokines. (L) Fusokines either target two cytokines, such as tumor necrosis factor (TNF)-α and IL-17, one cytokine and one CK, or two CKs, such as CXCL10 and XCL1.
Monoclonal Antibodies
The clinical mainstay of the chemo/cytokine-targeted approach has been using high-affinity designer monoclonal antibody (mAb or moAb) to bind directly to the chemo/cytokine, which resulted in the first mAb clinical usage in 1986 (179) and now more than 500 mAbs. In the trifunctional antibody, the two antigen-binding fragments confer antigen specificity; the single constant fragment, immune effector interaction (180). mAbs were developed in the mouse and then a chimeric mouse–human IgG1 (-xi-mab) was created (60–70% human), which combines human Ig constant regions with rodent variable regions. Humanized mAbs (>90% human) were followed later by human antibodies (100% human), by including the variable Ig regions. Humanized and human mAbs have largely replaced murine and chimeric mAbs for clinical use, and the murine hybridoma technology has been replaced by recombinant DNA technology, phage display, and transgenic mice (180). Therapeutic mAbs (~150 kDa) may directly target the chemo/cytokine or indirectly once its antigen is recognized by anti-dependent cell-mediated or complement-dependent toxicity (173). Most mAbs bind surface receptors without effects of cell signaling or functionality (180). Therapeutic mAbs may be antagonists or allosteric agonists, with binding distinct from the natural ligand (180).
mAbs have been classified by the WHO. The international non-proprietary nomenclature for mAbs can be confusing because the old convention (181) differs from the newer one (182), which is generally shorter. The mAb suffix is “-mab.” Each mAb usually has a unique prefix. Between the two lies a source code (source infix): “-o-mab” for mouse; “-xi-mab” for chimeric; “-lizu-” for humanized; and “-u-mab-” for human. There also may be a target code (target infix): “-limu-” denotes targeting the immune system; “-tu-” indicates tumors; and “-ki-” for IL. As examples, infliximab (infli-xi-mab) would be chimeric, bevacizumab (bevaci-zu-mab) would be humanized; adalimumab (ada-limu-mab) would be human; and canakinumab (cana-ki-umab) would be IL targeting and human, as would ixekizumab (ixe-ki-zumab). The new nomenclature will drop the source infix and devise more specific target inflixes (182).
Soluble Receptors
Another approach is to use soluble receptors to bind the chemo/cytokine molecule (receptor ligand). This cue is taken from human nature, in which soluble receptors are endogenous and functional. Recombinant fusion proteins combine a key component of the receptor with IgG1 (183). Examples include anakinra (IL-1-Ra) and etanercept (TNF-αR). The recombinant soluble receptor has a much longer half-life than the naturally occurring soluble receptor. Naturally occurring soluble receptors are cleaved from the surface of cells that express the receptor. A new generation of anti-IL-6R mAbs targets both soluble IL-6R (sIL-6R) and membrane-bound (mIL-6R) (184) with improved binding affinity and specificity and reduction in the occurrence of adverse events (185).
Small Molecule Antagonists
An additional tactic is use of small molecule antagonists (500–600 Da) protein therapeutics, binding proteins, protein antagonists (178). They stabilize the receptor in inactive conformation, usually binding residues in the transmembrane receptor helices, thereby preventing chemo/cytokine binding (42). Alternatively, some small molecule ligands appear not to bind directly to the chemo/cytokine receptor site (non-competitive) but rather to a topographically distinct allosteric (allotopic) site (172). Allosteric modulators may be positive (positive allosteric modulators), negative [negative allosteric modulators (NAMS)], or silent/neutral [silent allosteric modulators (SAMS)]. There are numerous patents on low-molecular-weight inhibitors of multiple types of CRs. Anakinra is a recombinant version of an IL-1R antagonist, which works by competitive inhibition of the binding of IL-1 to its receptor.
Small Molecule Agonists
Rather than conventional CKR blockade, small molecule agonists also are being developed (42). Although they activate the CKR, the functional effect may be partial (partially competitive), not the same as natural receptor ligands, due to different capacities for activating different signaling pathways (42). Allosteric agonists may exhibit functional selectivity, such as when the initial post-binding step of receptor internalization is comparable to that of a CK, but the agonist is unable to induce chemotaxis (172).
Neutraligands
Chemokine neutralizing molecules (“neutraligands” or “neutral ligands”) with anti-inflammatory activity that are not receptor antagonists also are being developed (177). Unlike receptor antagonists, they bind but do not alter CKR basal activity (constitutive activity) (177). Neutraligands mimic pathogen-encoded decoy proteins. There are high-affinity decoy traps for CCL17 and CCL22 (177).
Nanobodies (Nbs)/Antibody Fragments
Nanobodies were developed to exploit the advantages of mAb miniaturization, avoiding high cost and complexity. These are small “minimalist” antibodies (15 kDa) with a single-domain camelid antibody fragment (186). Nbs have been developed against CCL2, CCL5, CXCR7, CXCL11, and CXCL12 (187). They also can be linked together to form a “biparatopic nanobody.”
Bispecific Antibodies (BsAbs)
A developing strategy is more precise targeting of cytokines with BsAb (188). The TNF/IL-17 fusion antibody allows simultaneous targeting more than one cytokine (97). It is also possible to inhibit part of a signaling cascade to spare protective cytokine effects, as exemplified by targeting just one of the two TNF receptors (22) or only the trans-signaling of IL-6 triggered cascade (188). A pathogenic cell can be targeted, as in the case of using myeloid specific TNF inhibitor (188). BsAb can also target CKs: a fully humanized IgG-like BsAb for effective dual targeting of CXCR3 and CCR6 has been developed (189).
Scaffold Receptor Proteins
Engineered “protein scaffolds” are touted as next-generation antibody therapeutics (190). To eliminate the need for a chimeric intermediate, the complementarity-determining region (“donor”) is inserted into a human antibody scaffold (“acceptor”). The scaffolds are receptor proteins, not immunoglobulins, which are given binding function via combinational protein design (190). “Antibody mimetics” are another group of organic compounds (3–20 kDa) that specifically bind antigen but are not related to mAbs. They are based on a naturally occurring protein scaffold template that binds the desired ligand, which is then engineering enhanced (191).
Fusokines
Fusokines, proteins formed by physical coupling of two cytokines that are functionally different into a single bifunctional polypeptide (192), are in preclinical development. They are purposed for synergistic bioactivity, greater therapeutic potential, and bioavailability than cytokines administered singly or in combinations (193). Fusion of GM-CSF with an IL (IL-2, IL-15, and IL-21), coined “GIFTs,” co-opts the normal signaling machinery of IL receptors, favorably altering the response cell status (194), such as reprogramming B cells into regulatory cells (195). Other fusokines include CXCL10/XCL1 (193) and IL-15/TGF-β (FIST15”) (196). Cytokines also can be fused with CKs (“GMME”), such as GM-CSF with CCL2 or CCL7 (194).
Co-Receptor Targeting
Instead of targeting a CR directly, a new approach is targeting a co-receptor, such as receptor-associated gp130, a ubiquitously expressed signal-transducing subunit common to all members of the IL-6 family (185). It is part of the pathway of classic IL-6R signaling. However, naturally occurring sIL-6R may trigger of IL-6 “trans-signaling,” with deleterious effects” (32). Soluble gp130Fc is in phase II trials (32). A simplified schema for mIL-6R and sIL-6R targeting is illustrated (Figure 3) (32, 188, 197).
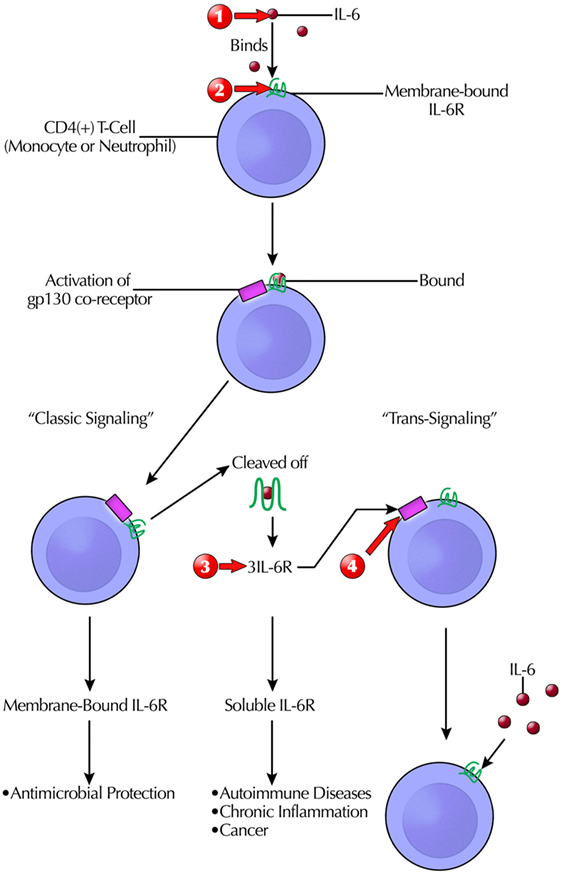
Figure 3. Simplified schema of the complicated targeting IL-6 actions at the receptor binding and signaling/transduction level that give rise to its pleotrophic effects. Only after formation of the three-way complex [IL-6, IL-6R, and glycoprotein 130 (gp130)] is IL-6 signaling initiated. Although gp130 is ubiquitously expressed, fewer cells express IL-6R. Clinically available therapeutic modalities target IL-6 or IL-6R. Another option in clinical trials is blocking sgp130Fc, which does not interfere with host antibacterial defenses. This prevents IL-6 trans-signaling, which is pro-inflammatory. Therapeutic intervention sites are marked with red arrows as follows: (1) anti-IL-6: siltuximab/elselimomab/clazakizumab; (2) anti-mIL-6Rα: tocilizumab/basiliximab/sarilumab; (3) anti-sIL-6R and sIL-6R: sarilumab/tocilizumab; and (4) anti-spg130: sgp130Fc. Abbreviations: STAT, signal transducer and activator of transcription; MAPK, mitogen-activated protein kinase; PI3K, phospitidylinositol-3 kinase; AKT, protein kinase B.
Inflammasome Targeting
Another promising approach is experimental anti-IL-1 targeting of NLRP3 inflammasome, a key contributor to the development of neuroinflammation that is amply expressed in the CNS (198). Inflammasomes are three-unit intracellular complexes, constitutive of the innate immune system. CSF NLRP3 is increased after severe TBI in infants and children (197).
Transduction Targeting
Agents indirectly affecting cytokines, such as JAK inhibitors, are also relevant. These include pan-JAK inhibitors (baricitinib, peficitinib, ruxolitinib, and tofacitinib) and selective JAK inhibitors (decernotinib, filgotinib, and upadacitinib) (199).
Other Agents
Serendipity (two-for-one benefit) is not to be discounted. Tacrolimus (FK506), a calcineurin and IL-2 inhibitor, has been found to selectively decrease CSF CXCL10 in an exploratory study of paraneoplastic cerebellar degeneration with anti-Yo and anti-Hu antibodies (99). As CSF CXCL10 is also increased in pOMS and not reduced by conventional agents or steroid sparers (80), the effect may be relevant for OMS and other neurological paraneoplastic syndromes, too.
Clinical Armamentarium of Immunoneutralizers
Various biopharmaceutical immunomodulators that target CKs or other cytokines have been tried, are in clinical trials, or have been U.S. Food and Drug Administration (FDA)-approved (Table 8) (26, 99, 179, 200–229). Reference to trials in non-neurologic disorders, such as asthma, cancer, Crohn’s disease, HIV, psoriasis, and rheumatoid arthritis (RA), initially may seem off topic, but the treatment of those disorders has fueled trials in neuroinflammatory disorders.
Systemic anti-cytokine therapy has been daubed the most dramatic therapeutic advance in the modern era of rheumatology (178), although the number of failures exceeded the successes (179). CK blockers have been called “therapeutics in the making” (230), yet the field awaits comparable breakthrough agents.
Although the highly anticipated rituximab therapeutic effect in systemic lupus erythematosus (SLE) was not demonstrated in clinical trials, targeting BAFF was successful (belimumab) (231). IL-1 blocking agents decreased pro-inflammatory cytokines and leukocyte subsets in CSF of pediatric patients with neonatal-onset multisystem inflammatory disease (113), although there were differences in efficacy in the intrathecal compartment (anakinra vs canakinumab). IL-6, a pivotal mediator, has been extensively targeted, both at the level of cytokine and its receptor, with FDA approval in each category. A surrogate marker of anti-IL-6 mAb therapeutic efficacy was inhibition of C-reactive protein (232).
Anti-IFN-γ antibodies have been tested in the treatment of various autoimmune diseases, including MS, schizophrenia, and various autoimmune skin conditions, some with purported clinical benefit (233). However, none have been FDA-approved. Contrariwise, exaggerated type 1 IFN production by DCs has been considered for targeting (60).
Clinical failures or disease exacerbation in some high phase clinical trials have quashed many an immunomarker-guided therapy. The high rate of attrition is multifactorial. Incorrectness of a priori hypothesis is one reason. The majority of human clinical trials of treatment for RA with mAbs or synthetic compounds intended to target CK signaling have failed to show clinical efficacy. Of those approved, the clinical response is inadequate in 40–50% (121). One failure was an anti-CCL2 antibody in RA, which paradoxically increased the circulating concentration of CCL2×2,000 (234).
Tumor necrosis factor inhibitors may be associated with CNS demyelinating disorders mimicking MS or myelopathy (235, 236). Serum sickness and encephalitis are other potential complications of anti-cytokine therapy (237). Studies of anti-IL-7; anti-IL-17 RA (receptor; also blocks IL-17 B–F); and anti-CD40-CD40L (toralizumab, H) were pulled due to untoward effects (238). Biologic-induced infections include reactivation of tuberculosis (anti-TNF therapy), viral infections, and reactivation of herpes zoster (26). IFN therapies are immunosuppressive, and neutropenia can lead to infections. In patients with SLE, there was an increased frequency of herpes zoster infections in sifalimumab- and anifrolumab-treated patients, and increased influenza rates in anifrolumab-treated patients (239). Tocilizumab can cause intestinal diverticulitis and perforation. Ustekinumab and briakinumab are associated with major cardiovascular events in some patients.
Two clinical trials of rituximab for patients with SLE failed. Although attributed to limitations of trial design and effects of other ongoing treatments, a post-rituximab surge in serum BAFF was found in relapsing patients. Based on the capacity of BAFF to allow autoreactive B cells to survive especially when B cell counts are low, a destructive feedback loop with autoantibody surges has been proposed (28). The authors argue that B cell depletion in SLE should be followed by BAFF inhibition.
Results in Neurologic Disorders
Although most anti-chemo/cytokine mAbs are not initially developed for CNS application, some are later tested, repurposed, and remarketed. Immunological interrelationships among diseases assist assessment of the probability of cross-utility of the agents.
Tumor necrosis factor neutralization in MS increased relapse frequency and intensity (240). The negative outcome was not predicted by preclinical studies. Although psoriasis and MS share certain immunological features, the IL-12/IL-23 neutralizing mAb ustekinumab was not found effective for MS (207). IL-23 promotes expansion of IL-17 producing Th17 cells. Because ustekinumab targets p40, the subunit common to IL-12 and IL-23, inhibition of IL-12 may also have occurred. IL-12 is an important cytokine for initiation of Th1-mediated inflammatory responses, which is produced by DCs, macrophages, and neutrophils responding to antigen stimulation. Because of the role of IL-17 in neuroinflammatory disorders (241), the anti-IL-17 mAb secukinumab was in a phase II study for MS, but the study was terminated by the sponsor because it had a better anti-17 mAb to test.
The results of targeting of BAFF/APRIL in MS with atacicept were unexpectedly disappointing: it worsened disease activity (NCT00642902, phase II study terminated). In RA, it exerted immunological but not clinical effects. Perhaps the problem is that atacicept impacts regulatory B cells without adequately depleting pathogenic B cells (58).
Adalimumab reduced seizure frequency 10-fold in an open pilot study of 11 patients with RE (242). The improvement was sustained in five patients, three of whom showed no further cognitive decline. RE is otherwise such a devastating, refractory hemiconvulsion/hemiplegia syndrome, it is usually treated by hemispherectomy, but there is limited experience with immunomodulatory treatments early (243).
A pediatric patient with NMO and Sjögren syndrome was successfully treated with tocilizumab (244). In a pilot study of tocilizumab in adults with NMO (245), relapse rate, neuropathic pain, and fatigue were reduced.
In an adult with limbic encephalitis associated with relapsing polychondritis, infliximab treatment was associated with sustained improvement (246). In refractory neurosarcoidosis treated with adjunctive infliximab, most improved clinically but still frequently relapsed (247).
Targeting CKR/CR
Even a non-exhaustive listing shows more immunomodulatory agents are available with which to target non-CK CRs than CKRs (Table 9) (15, 26, 113, 184, 248–266). Drug discovery initiatives have not delivered on effective agents to selectively manipulate CKs in neuroinflammatory disorders (267). The imputation is that CK binding/signaling is biologically complex and reliant on early synergism between CKs (267). However, CKRs are GPCRs, which have been the most successful target class for drug discovery (268). The concept of CKRs as biomarkers was studied in MS (47).
Chemokine receptor-targeted agents may function as agonists, partial agonists, inverse agonists, or antagonists at the receptor site (255). In clinical studies, daclizumab, a humanized mAb agonist at the IL-2 receptor (alpha chain) reversed intrathecal increased adaptive immune cells (CD4 and CD8 T cells; B cell) and increased innate lymphoid cells in MS (225). Anakinra, an IL-1 receptor antagonist, has recently been applied to the treatment of a few neurological disorders. In a case report of one critically ill child with refractory status epilepticus due to febrile infection-related epilepsy syndrome, anakinra treatment (100). This catastrophic epileptic syndrome is otherwise known for little response to conventional immunomodulatory therapy or antiepileptic drugs (269). Anakinra is also used to treat an autoimmune disorder caused by genetic deficiency of IL-1 receptor antagonist, leaving IL-1 signaling unopposed (270).
The profusion of preclinical studies is exciting, although much of the information is proprietary and unavailable. Anti-CCR1 lacked efficacy in MS (261). CCR2 receptor blockade prevented asthma in a primate model, and anti-CCR3 and anti-CCR4 were tested in asthma (271). Anti-CCR5 and anti-CCR7 have been proposed as future treatments for high-risk CLL (272). In studies of C–C motif CKs, inhibition of CCR6 reduced the severity of experimental autoimmune encephalitis (EAE), an animal model of MS, whether by neutralizing antibodies or a novel receptor antagonist (273). Anti-CCR9 mAbs have been used to demonstrate CCR9 mediation of antiapoptotic signals in cancer. A small molecular inhibitor of CCR9 lacked benefit for inflammatory bowel disease (IBD) (265).
As to C–X–C motif CKs, an mAb to CXCL13 (mAb 5261) was developed (274). Concomitant targeting of CXCL13 and the BAFF receptor mitigated murine Sjögren syndrome (27). Anti-CXCR3 neutralizing antibody may have a role in preventing or treating graft-vs-host disease (275). Targeting CXCR5 in a mouse model of non-Hodgkin lymphoma (276). Anti-CXCR4 (CX549) exhibited anti-inflammatory properties and reduced brain infarction in experimental stroke (277). An engineered, fully human, single-domain antibody-like scaffold or “i-body” has antagonist activity against CXCR4 in humans (278), and there is great interest in targeting CXCR4 (279).
The sIL-23R was delivered in EAE via a novel therapeutic strategy: use of adeno-associated virus vectors encoding sIL-23R (280). The anti-IL-23R mAb tildrakizumab also has been developed. The GM-CSF-MCP-1 fusokine selectively inhibited CCR2-expressing lymphomyeloid cells in experimental autoimmune encephalomyelitis (281).
Because TNF-α binds both to TNFR1 and TNFR2, targeting TNF-α itself would be expected to have different downstream effects than selectively targeting either receptor (Figure 4). Such factors contribute to the complexity of choosing the best target. In vitro, an agonist mAb enhanced TNFR2 signaling and rescued human neurons from oxidative stress-induced cell death (282). Conversely, TNFR2 antagonists inhibit regulatory T cell (Treg) proliferation, which has application in cancer therapy (283).
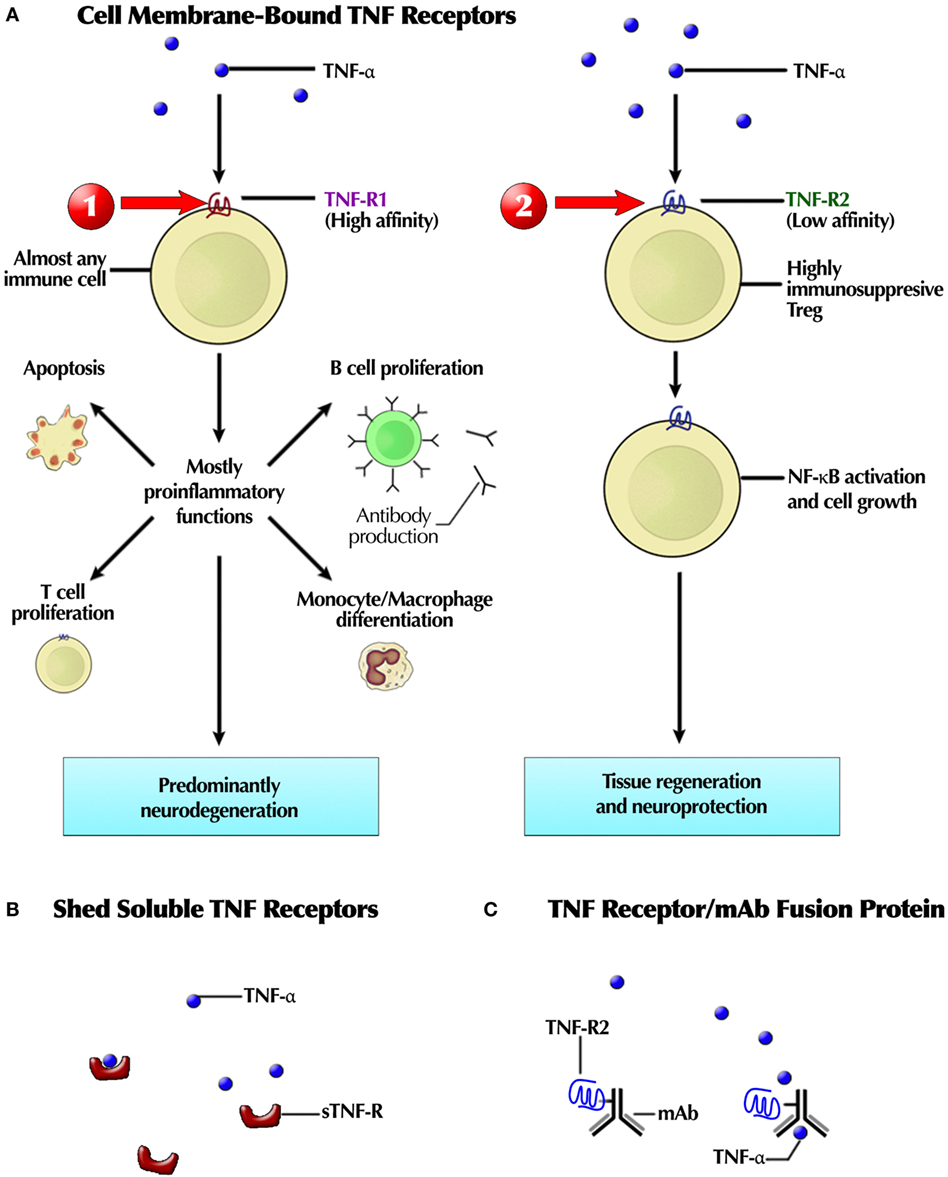
Figure 4. (A) Schematic depiction of the targetable differences between TNFR1 and TNFR2 receptor pathways after binding of tumor necrosis factor (TNF), a natural agonist at both receptors. TNF-α, produced by activated macrophages, is a natural ligand at both receptors. TNFR1 receptor stimulation may trigger eventual neurodegeneration, so a selective TNFR1 antagonist may block it. TNFR2 receptor stimulation is associated this tissue regeneration and neuroprotection, hence a selective TNFR2 agonist could also treat neurogenerative diseases. In support of that premise, a selective TNFR2 receptor antagonist has destructive effects: inhibits regulatory T cell (Treg) proliferation, TNFR2 secretion from cells, and promotes T effector cell expansion. (B) Endogenous TNF soluble or decoy receptors, shed from membrane-bound TNFR, sequester TNF-α, preventing its inflammatory effects. (C) A receptor-Fc construct, combining a TNFR2 fragment with an mAb. This recombinant fusion protein is ethanercept. Red arrows indicate therapeutic intervention sites: (1) TNFR1 antagonist and (2) selective TNFR2 agonist. Not shown is the therapeutic potential of a TNFR2 antagonist for the treatment of cancer, inhibiting Treg proliferation, soluble TNFR2 secretion from normal cells, but increased effector T cell expansion, as demonstrated in vitro. Thus, the target shifts with the therapeutic purpose and disease.
Cytokine Delivery as Immunotherapy
Although targeting chemo/cytokines has been the focus of the discussion thus far, direct delivery of cytokines is another therapeutic strategy. The most clinically and commercially successful venture has been with type 1 IFNs, a subclass of cytokines. IFN-α-1a and IFN-α-1b, as well as IFN-β-1a and IFN-α-2b are licensed and well-known therapies for MS (284). Abnormal upregulation of type 1 IFN signaling and gene expression stimulated by IFN have been tied to “interferonopathies,” a common moniker for a group of CNS diseases that includes AGS (284), a pediatric-onset epileptic encephalopathy characterized by increased CSF IFN-α, CCL2, and CXCL10 (104). Anti-type 1 IFN therapy has been attempted in SLE (238).
Interferon-gamma, the sole type 2 IFN, binds to the IFN-γ-R1 and IFN-γ-R2 receptors (also denoted IFNGR1 and R2). IFN-γ-1b is FDA-approved for chronic granulomatous diseases and osteopetrosis (285). A phase III study (NCT024155127) of IFN-γ-1b for the treatment of Friedreich ataxia has been completed recently, but without study results yet, based on positive results from as phase II study (143). Treatment with IFN-γ exacerbated MS (286).
There are fewer non-IFN cytokine therapies. Low-dose IL-2 was used as a treatment for refractory autoimmune encephalitis in 10 adults, based on the assumption that low-dose restores the balance of regulatory and effector T cells, with clinical improvement in 6 (287). In preclinical studies, administration of G-CSF in a murine model of Friedreich ataxia resulted in clinical improvement and reduction in inflammation and gliosis (288). IL-10 is such a powerful counteractant of pro-inflammatory cytokines, one would hope there is more progress in this area.
Although they would provoke, not treat, neuroinflammation, several cytokines are used to treat cancer. They include high-dose IL-2, IFN-γ, IL-15, and GM-CSF (289). By contrast, IL-1, TNF-α, IL-4, and IL-6 were ineffective.
Another concept is administering the cytokine in combination with an inhibitor of its breakdown. Combination of IFN-β with inhibitors of proteinases that degrade IFN-β has been conceived for MS (290). Such approaches may be applicable with other cytokines.
Considerations in Designing Future Clinical Trials for Neuroimmunologic Application
Avoiding Targeted Treatment Failure
Some of the more common reasons for failure of targeted treatment have been explicated (291). Several result from miscalculation of pharmacodynamic effects. Unsound target selection is common (291). Because biologic targets are dynamic, not static, therapeutic approaches must be highly anticipatory of downstream effects, particularly negative ones. Undesirable “off-target effects” can be stimulated (177) due to low specificity or rapid clearance rates of the ligand/drug (255). Insufficient dosing to achieve therapeutic receptor saturation can result in false negative results (291). Redundant or opposing chemo/cytokine functions may negate the therapeutic effect: receptor antagonists tend to have some degree of undesirable partial or inverse agonistic activities (177). Target validation in experimental animals may be problematic because pertinent human chemokine receptor–ligand pairs may have different roles (291).
Impractical clinical trial decisions are another reason for avoidable treatment failure. Changing successful clinical trial endpoints from a phase II trial to different ones in a phase III trial may compromise the latter (291). Sometimes a pharmacokinetic study would have been advisable. The clinical trial conditions may not be comparable or translatable to the “real world” clinical setting. Finding both active and passive controls can be challenging. Post-marketing adverse events are often unpredictable.
Strategic Insights
Clinical and preclinical studies of chemo/cytokine-targeted therapy have provided strategic insights for the next level of therapeutic intervention, which over time will become more nuanced (Table 10) (27, 58, 177–179, 187, 188, 206, 214, 237, 268, 285, 292). The fluctuating nature of responses to immunotherapy, the unintended consequences, and natural remission are cautionary sign posts along the road to innovation. There are also differences in biologicals and synthetics to consider.
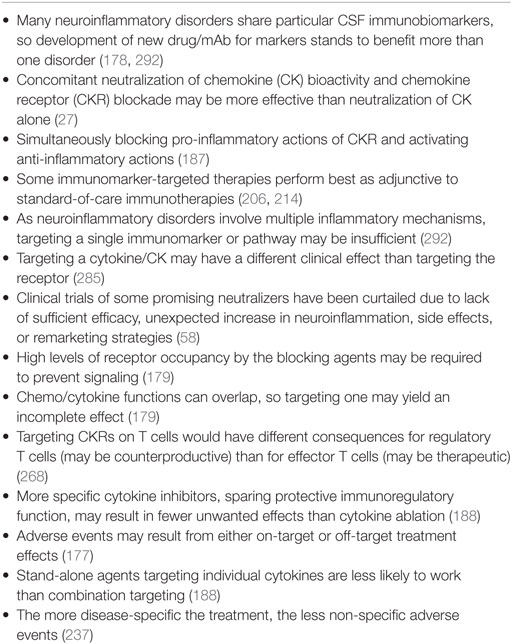
Table 10. Strategic insights from clinical trials and preclinical studies of chemo/cytokine-targeted therapy.
Under the “approach cautiously” category fall the pleotrophic cytokines (IL-2, IL-6, IL-10, IL-12, IL-13, IL-21, and IL-25). They are multipotent and multipurposed. There may be unwanted consequences of ablating or globally blockading these cytokines, prompting the development of more specific inhibitors to preserve protective immunoregulatory functions (188).
It would seem logical to apply agents to those disorders in which the specific CSF biomarker elevation has been demonstrated rather to ones in which the concentration is normal. Elevation of chemo/cytokine concentrations by themselves, of course, do not prove pathogenicity, and all biomarkers are not measured in all disorders, so data are sometimes scant or lacking. G-CSF was increased in NMO, ADEM, and NPSLE; GM-CSF in NPSLE, stroke, and SCA. APRIL was elevated in NPSLE, MOG(+) MS, and NMO (not pOMS). IL-13 was increased in MS, NMO, SCA, MSA-C, and AGS; IFN-γ in ADEM and AE.
The relation of BAFF and APRIL in neuroinflammatory disorders is curious: they often do not sort together. In the case of NPSLE, APRIL is elevated, BAFF less so, and BAFF is increased in plasma (29). In pOMS, CSF BAFF and CSF/serum BAFF ratio are elevated, but APRIL is not (79, 94). Responses to therapeutics differ as well: IVIg increased serum APRIL, not BAFF, in pOMS (79). These are important considerations in deciding in which disorders belimumab may be useful.
Ligand–receptor dynamics, although complex, can be further exploited. The systemic concentration of soluble IL-6 receptors (sIL-6R) normally exceeds that of IL-6 (188). Most harmful effects of IL-6 appear to be mediated by sIL-6R, whereas the host-protective functions of IL-6 are mediated by membrane-bound IL-6R (293). It would be informative and useful to start measuring concentrations of pertinent, naturally occurring, soluble chemo/cytokine receptor concentrations (30–32). As therapies targeting these soluble receptors advance, patient selection would benefit. The clinical availability of reference laboratories and costs would require evaluation.
Many existing drugs can be repurposed to treat neuroinflammation. The anti-HIV drug maraviroc, which prevents HIV entry into the cell through CCR5, has been proposed to blockade CCR5 in neuroinflammatory diseases (264). It is an available and well-tolerated approved drug. It may become feasible to screen for CCR5 receptor polymorphisms.
When CXCL13/CXCR5 targeting is available to neuroimmunology researchers, its use has been suggested for MS (83), NMDAR encephalitis (294), and OMS (81). Understanding the alternative roles of CXCR3 signaling will be required, however. In glial cells, CXCR3 improves EAE by reducing CNS-infiltrating Th17 cells through prevention of a pro-Th17 cytokine milieu (295). Also, CCL2 and CCR2 have been proposed as selective targets for the treatment of MS (296).
Leaving aside varied pharmacokinetic factors associated the wide spectrum of patient ages in neuroinflammatory disorders, there are several important pharmacodynamic considerations. What is the accessibility of the target? Activated intrathecal B and T cells become embedded in brain tissue in progressive MS, but not RR-MS, where they may be more difficult to rout. Early treatment prior to that stage may have better clinical impact. What is the tolerability of chronic pharmacologic blockade? Possible compensatory mechanisms should be anticipated. What are the specific windows of therapeutic opportunity, such: timing, context, developmental stage? What are the appropriate response-predictive biomarkers? There is a growing need for proof-of-concept studies, especially in orphan diseases for which there is no animal model.
Monitoring for anti-mAb and anti-drug antibodies, such as human antihuman or HAHA, spawned by their immunogenicity is an important concern for effective treatment, especially when there is a reduction in efficacy or loss of response (237). The percent of treated patients with antidrug antibodies is highly variable. In the case of IBD, infliximab ranked highest in antibody frequency (65%), but the antibodies were also detected after treatment with adalimumab, certolizumab pegol, golimumab, and ustekinumab (237).
Navigating Constraints in Pediatric Drug Trials
Clinical trials in pediatric-onset neuroinflammatory disorders require special handling. The pediatric population is designated as “vulnerable,” resulting in restrictions on clinical research. Ethical and federal regulatory issues surround vulnerable populations in pragmatic clinical trials (297). As to immunotherapy, there is a reliance on drugs tested in adults, especially FDA-approved drugs for other clinical indications, and perhaps fewer assessable immunopharmacological agents for clinical trials and use in pediatric neuroinflammatory disorders. Also, the propensity for pharmacokinetic differences between children and adults is well known. Pediatric medication safety research faces the difficulty of assessing child-reported adverse drug events (298).
In Vivo Imaging of Human Neuroinflammation
In the foreseeable future, molecular imaging with radiolabeled ligands could facilitate patient selection for targeted therapy. Radiolabeled CXCR4 ligands have been developed recently (279). In experimental animals, inflammasome activation has been revealed by in vivo imaging (299). 99mTNFR2-Fc-IL-1ra was developed for use with single-photon emission computed tomography imaging to image inflammation via TNF and IL-1 pathways in mice (300). Neuroimaging methods can target the activation of immune cells in the CNS with TSPO tracer [(11)C]PB28 (301). Iron oxide nanoparticles and MRI can be used to track CNS infiltration by monocytes and other circulating immune cells (301).
Future Perspectives
Targeting dysregulated CKs and other cytokines or their receptors is a provocative and growing therapeutic line of approach with complex implications, and an emerging era for pediatric neuroinflammatory disorders. The goal is to apply gathering evidence to treatment optimization with continual contemplation of potential consequences of targeted engagement. Central to this process is developing algorithms using biomarker criteria and a focused set of molecular measures. Several trends are driving the emergence of personalized medicine, for which biomarkers play a pivotal role. Greater understanding of disease mechanisms and pharmacodynamics is essential, and collecting data must be embraced by treating physicians now that standard tests for defining neuroinflammation are now obsolete. Tailored treatment approaches can be based on clinical and biological factors revealed by immunobiomarker interrogation of neuroinflammatory diseases.
Historically, targeted therapies being tested for neuroinflammatory disorders were developed for other disease applications and repositioned. Some have provided clinical benefit; others merely show proof-of-concept to date; some failed. Taken together, the present observations support the further application of agents developed for other disorders in clinical trials for neuroinflammation disorders given the redoubtable onslaught of disease. Because many inhibitor projects fail, it is crucial to obtain as much information about the target and its role in the disease immunopathophysiology before starting a drug-development program.
Cerebrospinal fluid biomarker studies thus far have revealed several lessons: patients with extreme levels of CSF biomarkers may show higher morbidity or mortality, CSF multi-analyte profiling may be more clinically helpful than single markers, and the use of several may have the best predictive value. More success has been obtained when non-CK cytokines and/or their receptors are targeted, and there have been no CK-targeting drugs licensed for treating neuroinflammation. FDA approvals often specify for individuals with previous inadequate response to two or more DMTs or failure of standard immunotherapy, so early intervention has not been adequately tested. More development of “risk-based” treatment approaches, used successfully in cancer therapeutics, is needed for neuroinflammatory disorders.
In most cases, CSF CK/cytokine data in pediatric-onset neuroinflammatory disorders are lacking, preventing systematic comparison with their adult-onset counterparts. Herein is a call-to-action to gather systematic data on CSF cytokine and CK concentrations particularly in pediatric-onset MS, neuropsychiatric lupus, and NMO. Literature on how to design phase II clinical trial designs for initial biomarker validation is already available (302). CSF CK/cytokine biomarkers should be added to clinical trials of new agents for neuroinflammation (9). Among more recent concepts, treating neuroinflammation alone may be insufficient, and neuroprotection of the innate immune system may be necessary (303). The goal of successfully treating serious diseases once considered as “undruggable” should propel research into the future of biomarker-targeted immunotherapeutics, optimistically renamed “difficult-to-drug” or “yet-to-be-drugged” (304).
Author Contributions
The author performed the literature searches and wrote the manuscript.
Conflict of Interest Statement
The author declares that the research was conducted in the absence of any commercial or financial relationships that could be construed as a potential conflict of interest.
The reviewer KK and handling editor declared their shared affiliation.
Acknowledgments
MP is a clinician-scientist; President and Founder of the National Pediatric Neuroinflammation Organization, Inc., which is a Florida charitable organization and registered 501(c)(3) non-profit organization; a Professor of Neurology at the University of Central Florida College of Medicine, Orlando, FL, USA; and an Adjoint Professor of Neurology at the University of Colorado School of Medicine, Denver, CO, USA; and certified by the American Board of Psychiatry and Neurology, with special competence in child neurology. The graphics were created from MP’s sketches by Ryan Dickerson, Medical Illustrator, Education Technology, University of Central Florida College of Medicine, Orlando, FL, USA.
Supplementary Material
The Supplementary Material for this article can be found online at https://www.frontiersin.org/articles/10.3389/fimmu.2018.00557/full#supplementary-material.
Figure S1. Cytokine concentrations in (A) cerebrospinal fluid (CSF) and (B) serum. Means with standard deviation (SD) in parentheses (larger figure) and medians with interquartile range in parentheses (insert) are ranked. Other medians could not be calculated due to the number of samples with undetectable levels.
Figure S2. Cytokine detection rates in CSF and serum in the entire dataset. Percent detectability was defined as the percentage of samples in which a given cytokine could be detected. The order of the cytokines displayed is from the highest to the lowest CSF detection rate.
Abbreviations
AAV, ANCA-associated vasculitis; ADEM, acute disseminated encephalomyelitis; AE, autoimmune encephalitis; AGS, Aicardi–Goutières syndrome; AIDP, acute demyelinating polyradiculoneuropathy; ALD, adrenoleukodystrophy; APRIL, a proliferation-induced ligand; ACKR, atypical chemokine receptor; AKT, protein kinase B; BAFF-R, B cell-activating factor receptor; BCMA, B cell maturation antigen; Breg, regulatory B cell; BsAb, bispecific antibody; C, chimeric; CAPS, cryopyrin-associated periodic syndromes; CCL, C–C cryopyrin-associated periodic syndromes; chemokine ligand motif, C-CSF, granulocyte colony-stimulating factor; CDR, complementarity-determining region; CIDP, chronic inflammatory demyelinating polyneuropathy; CIS, clinically isolated syndrome; CK, chemokine; CKR, chemokine receptor; CNS, central nervous system; CSF, cerebrospinal fluid; chemokine, chemoattractive cytokine; CXCL, C–X–C chemokine ligand motif; CX3CL1, C–X–3C chemokine ligand motif; DC, dendritic cell; EV-71, enterovirus-71; FDA, U.S. Food and Drug Administration; Fab, antigen-binding fragment; FC, single constant fragment; FIRES, febrile infection-related epilepsy syndrome; FP, fusion protein; GABA, gamma-aminobutyric acid; GAGs, glycosaminoglycans; GBS, Guillain–Barré syndrome; gp130, glycoprotein 130; GPCRS, G-protein-coupled receptors; GM-CSF, granulocyte–monocyte colony-stimulating factor; H, human/humanized; HIV, human immunodeficiency virus; IEM, inborn error of metabolism; IFN, interferon; IL, interleukin; IFN-γ, interferon-gamma; IL-Ra, IL-receptor antagonist; IS, infantile spasms; IV, intravenous; IVIg, intravenous immunoglobulin; JAK, Janus kinase; JRA, juvenile rheumatoid arthritis; LP, lumbar puncture; Lyme NB, neuroborreliosis; MAPK, mitogen-activated protein kinase; mAb, monoclonal antibody; MLD, metachromatic leukodystrophy; MOG, myelin oligodendrocyte glycoprotein; MS, multiple sclerosis; MSA-C, multiple system atrophy cerebellar type; NAMS, negative allosteric modulators; Nb, nanobody; NF-κB, nuclear factor kappa-light-chain-enhancer of activated B cells; NK, natural killer; NKT, natural killer-like T cell; NMDAR, N-methyl-d-aspartate receptor; N-Beçhet, neuro-Beçhet disease; NMO, neuromyelitis optica; NOMID, neonatal-onset multisystem inflammatory disease; NPSLE, neuropsychiatric systemic lupus erythematosus; OMS, opsoclonus–myoclonus syndrome; PAMS, positive allosteric modulators; PCD, paraneoplastic cerebellar degeneration; PI3K, phosphatidylinositol-3 kinase; pPPH, post-hemorrhagic hydrocephalus; RA, rheumatoid arthritis; RE, Rasmussen encephalitis; RFP, recombinant fusion protein; RH, recombinant humanized monoclonal antibody; RR-MS, relapsing–remitting multiple sclerosis; SAMS, silent allosteric modulators; SC, Sydenham chorea; SCA, spinocerebellar atrophy; Sjögren syndrome-associated meningoencephalitis; SLE, systemic lupus erythematosus; SP-MS, secondary-progressive MS; SSPE, subsclerosing panencephalitis; SQ, subcutaneous; STAT, signal transducer and activator of transcription; TACI, transmembrane activator and calcium modulator and cyclophilin ligand interactor; TBE, tick-borne encephalitis; TBI, traumatic brain injury; TGF, tumor growth factor; Th, T helper cell; Thf, T follicular helper cell; TNF, tumor necrosis factor; Treg, regulatory T cell; UC, ulcerative colitis; viral ME, meningoencephalitis.
References
1. Bielekova B, Pranzatelli MR. Promise, progress, and pitfalls in the search for CNS biomarkers in neuroimmunological diseases: a role for CSF immunophenotyping. Sem Pediatr Neurol (2017) 24(3):229–39. doi:10.1016/j.spen.2017.08.001
2. Arron JR, Townsend MJ, Keir ME, Yaspan BL, Chan AC. Stratified medicine in inflammatory disorders: from theory to practice. Clin Immunol (2015) 161(1):11–22. doi:10.1016/j.clim.2015.04.006
3. Bielekova B, Komori M, Xu Q, Reich DS, Wu T. Cerebrospinal fluid IL-12p40, CXCL13 and IL-8 as a combinatorial biomarker of active intrathecal inflammation. PLoS One (2012) 7(11):e48370. doi:10.1371/journal.pone.0048370
4. Rossi S, Motta C, Studer V, Macchiarulo G, Germani G, Finardi A, et al. Subclinical central inflammation is risk for RIS and CIS conversion to MS. Mult Scler (2015) 21(11):1443–52. doi:10.1177/1352458514564482
5. Anselmo A, Mazzon C, Borroni EM, Bonecchi R, Graham GJ, Locati M. Flow cytometry applications for the analysis of chemokine receptor expression and function. Cytometry (2014) 85:292–301. doi:10.1002/cyto.a.22439
6. Becher B, Spath S, Goverman J. Cytokine networks in neuroinflammation. Nat Rev Immunol (2017) 17:49–59. doi:10.1038/nri.2016.123
7. Ransohoff RM. Chemokines and chemokine receptors: standing at the crossroads of immunobiology and neurobiology. Immunity (2009) 31(5):711–21. doi:10.1016/j.immuni.2009.09.010
8. Pranzatelli MR, Tate ED, McGee NR, Colliver JA. Cytokines, cytokine antagonists, and soluble adhesion molecules in pediatric OMS and other neuroinflammatory disorders. J Neurol Sci (2013) 326(1–2):53–8. doi:10.1016/j.jns.2013.01.011
9. Edwards KR, Goyal J, Plavina T, Czerkowicz J, Goelz S, Ranger A, et al. Feasibility of the use of combinatorial chemokine arrays to study blood and CSF in multiple sclerosis. PLoS One (2013) 8(11):e81007. doi:10.1371/journal.pone.0081007
10. Holman DW, Klein RS, Ransohoff RM. The blood-brain barrier, chemokines and multiple sclerosis. Biochim Biophys Acta (2011) 1812(2):220–30. doi:10.1016/j.bbadis.2010.07.019
11. Griffith JW, Sokol CL, Luster AD. Chemokines and chemokine receptors: positioning cells for host defenses and immunity. Annu Rev Immunol (2014) 32:659–702. doi:10.1146/annurev-immunol-032713-120145
12. Williams JL, Holman DW, Klein RS. Chemokines in the balance: maintenance of homeostasis and protection at CNS barriers. Front Cell Neurosci (2014) 8:154. doi:10.3389/fncel.2014.00154
13. Striz I, Brabcova E, Kolesar L, Sekerkova A. Cytokine networking of innate immunity cells: a potential target of therapy. Clin Sci (Lond) (2014) 126(9):593–612. doi:10.1042/CS20130497
14. Kothur K, Weinholt L, Brilot F, Dale RC. CSF cytokines/chemokines as biomarkers in neuroinflammatory CNS disorders: a systematic review. Cytokine (2016) 77:227–37. doi:10.1016/j.cyto.2015.10.001
15. Hamilton JA. GM-CSF as a target in inflammatory/autoimmune disease: current evidence and future therapeutic potential. Expert Rev Clin Immunol (2015) 11(4):457–65. doi:10.1586/1744666X.2015.1024110
16. Franciotta D, Zardini E, Ravaglia S, Piccolo G, Andreoni L, Bergamaschi R, et al. Cytokines and chemokines in cerebrospinal fluid and serum of adult patients with acute disseminated encephalomyelitis. J Neurol Sci (2006) 247(2):202–7. doi:10.1016/j.jns.2006.05.049
17. Rentzos M, Angeli AV, Rombos A, Kyrozis A, Nikolaou C, Zouvelou V, et al. Proinflammatory cytokines in serum and cerebrospinal fluid of CIDP patients. Neurol Res (2012) 34(9):842–6. doi:10.1179/1743132812Y.0000000074
18. Furfaro F, Gilardi D, Allocca M, Cicerone C, Correale C, Fiorino G, et al. IL-23 blockade for Crohns disease: next generation of anti-cytokine therapy. Expert Rev Clin Immunol (2017) 13:1–11. doi:10.1080/1744666x.2017.1279055
19. Garlanda C, Dinarello CA, Mantovani A. The interleukin-1 family: back to the future. Immunity (2013) 39(6):1003–10018. doi:10.1016/j.immuni.2013.11.010
20. Sun L, He C, Nair L, Yeung J, Egwuagu CE. Interleukin 12 (IL-12) family cytokines: role in immune pathogenesis and treatment of CNS autoimmune diseases. Cytokine (2015) 75(2):249–55. doi:10.1016/j.cyto.2015.01.030
21. Meylan F, Siegel RM. TNF superfamily cytokines in the promotion of Th9 differentiation and immunopathology. Semin Immunopathol (2017) 39(1):21–8. doi:10.1007/s00281-016-0612-y
22. Kalliolias GD, Ivashkiv LB. TNF biology, pathogenic mechanism and emerging therapeutic strategies. Nat Rev Rheumatol (2016) 12:49–62. doi:10.1038/nrrheum.2015.169
23. Pan HF, Li XP, Zheng SG, Ye DQ. Emerging role of interleukin-22 in autoimmune diseases. Cytokine Growth Factor Rev (2013) 24(1):51–7. doi:10.1016/j.cytogfr.2012.07.002
24. Russo RC, Garcia CC, Teixeira MM, Amaral FA. The CXCL8/IL-8 chemokine family and its receptors in inflammatory diseases. Expert Rev Clin Immunol (2014) 10(5):593–619. doi:10.1586/1744666X.2014.894886
25. Ransohoff RM, Brown MA. Innate immunity in the central nervous system. J Clin Invest (2012) 122(4):1164–71. doi:10.1172/JCI58644
26. Furie R, Khamashta M, Merrill JT, Werth VP, Kalunian K, Brohawn P, et al. Anifrolumab, an anti-interferon-α receptor monoclonal antibody, in moderate-to-severe systemic lupus erythematosus. Arthritis Rheumatol (2017) 69(2):376–86. doi:10.1002/art.39962
27. Alexander SPH, Fabbro D, Kelly E, Marrion N, Peters JA, Benson HE, et al. The concise guide to PHARMACOLOGY 2015/2016: catalytic receptors. Br J Pharmacol (2015) 172(24):5979–6023. doi:10.1111/bph.13353
28. Sharma A, Kiriplosky J, Klimatcheva E, Howell A, Fereidouni F, Levenson R, et al. Early BAFF receptor blockade mitigates murine Sjögren’s syndrome: concomitant targeting of CXCL13 and the BAFF receptor prevents salivary hypofunction. Clin Immunol (2016) 164:85–94. doi:10.1016/j.clim.2016.01.015
29. Ehrenstein MR, Wing C. The BAFFling effects of rituximab in lupus: danger ahead? Nat Rev Rheumatol (2016) 12(6):367–72. doi:10.1038/nrrheum.2016.18
30. Dietrich C, Candon S, Ruemmele FM, Devergne O. A soluble form of IL-27Rα is a natural IL-27 antagonist. J Immunol (2014) 192(11):5382–9. doi:10.4049/jimmunol.1303435
31. Xu W, Presnell SR, Parrish-Novak J, Kindsvogel W, Jaspers S, Chen Z, et al. A soluble class II cytokine receptor, IL-22RA2, is a naturally occurring IL-22 antagonist. Proc Natl Acad Sci U S A (2001) 98(17):9511–6. doi:10.1073/pnas.171303198
32. Rose-John S. The soluble interleukin 6 receptor: advanced therapeutic options in inflammation. Clin Pharmacol Ther (2017) 102(4):591–8. doi:10.1002/cpt.782
33. Ransohoff RM, Schafer D, Vincent A, Blachère NE, Bar-Or A. Neuroinflammation: ways in which the immune system affects the brain. Neurotherapeutics (2015) 12:896–909. doi:10.1007/s13311-015-0385-3
34. Zlotnik A, Yoshie O. Chemokines: a new classification system and their role in immunity. Immunity (2000) 12:121–7. doi:10.1016/S1074-7613(00)80165-X
35. Pranzatelli MR, Tate ED, McGee NR, Colliver JA. Pediatric reference ranges for proinflammatory and anti-inflammatory cytokines in cerebrospinal fluid and serum by multiplexed immunoassay. J Interferon Cytokine Res (2013) 33(9):523–8. doi:10.1089/jir.2012.0132
36. Antonelli A, Ferrari SM, Giugglioli D, Ferrannini E, Ferri C, Fallahi P. Chemokine (C-X-C motif) ligand (CXCL)10 in autoimmune diseases. Autoimmun Rev (2014) 13(3):272–80. doi:10.1016/j.autrev.2013.10.010
37. Pease JE. Designing small molecule CXCR3 antagonists. Expert Opin Drug Discov (2017) 12(2):159–68. doi:10.1080/17460441.2017.1268597
38. Hernández-Ruiz M, Zlotnik A. Mucosal chemokines. J Interferon Cytokine Res (2017) 37(2):62–70. doi:10.1089/jir.2016.0076
39. Strieter RM, Polverini PJ, Arenberg DA, Kunkel SL. The role of CXC chemokines as regulators of angiogenesis. Shock (1995) 4(3):155–60. doi:10.1097/00024382-199509000-00001
40. Lazennec G, Richmond A. Chemokines and chemokine receptors: new insights into cancer-related inflammation. Trends Mol Med (2010) 16(3):133–44. doi:10.1016/j.molmed.2010.01.003
41. Neubig RR, Spedding M, Kenakin T, Christopoulos A; International Union of Pharmacology Committee on Receptor Nomenclature and Drug Classification. International union of pharmacology committee on receptor nomenclature and drug classification. XXXVIII. Update on terms and symbols in quantitative pharmacology. Pharmacol Rev (2003) 55:597–608. doi:10.1124/pr.55.4.4
42. Stone MJ, Hayward JA, Huang C, Huma ZE, Sanchez J. Mechanisms of regulation of the chemokine-receptor network. Int J Mol Sci (2017) 18:342. doi:10.3390/ijms18020342
43. Salvi V, Sozio F, Sozzani S, Del Prete A. Role of atypical chemokine receptors in microglial activation and polarization. Front Aging Neurosci (2017) 9:148. doi:10.3389/fnagi.2017.00148
44. Bacheleries F, Graham GJ, Locati M, Mantovani A, Murphy PM, Nibbs R, et al. An atypical addition to the chemokine receptor nomenclature: IUPHAR review 15. Br J Pharmacol (2015) 172(16):3945–9. doi:10.1111/bph.13182
45. Cancellieri C, Vacchini A, Locati M, Bonecchi R, Borroni EM. Atypical chemokine receptors: from silence to sound. Biochem Soc Trans (2013) 41(1):231–6. doi:10.1042/BST20120246
46. Ajram L, Begg M, Slack R, Cryan J, Hall D, Hodgson S, et al. Internalization of the chemokine receptor CCR4 can be evoked by orthosteric and allosteric antagonists. Eur J Pharmacol (2014) 729:75–85. doi:10.1016/j.ejphar.2014.02.007
47. Fox RJ, Kivisäkk P, Lee JC, Tucky B, Lucchinetti C, Rudick RA, et al. Chemokine receptors as biomarkers in multiple sclerosis. Dis Markers (2006) 22(4):227–33. doi:10.1155/2006/694283
48. Kivisäkk P, Trebst C, Liu Z, Tucky BH, Sørensen TL, Rudick RA, et al. T-cells in the cerebrospinal fluid express a similar repertoire of inflammatory chemokine receptors in the absence or presence of CNS inflammation: implications for CNS trafficking. Clin Exp Immunol (2002) 129:510–8. doi:10.1046/j.1365-2249.2002.01947.x
49. Cartier L, Hartley O, Dubois-Dauphin M, Krause KH. Chemokine receptors in the central nervous system: role in brain inflammation and neurodegenerative diseases. Brain Res Brain Res Rev (2005) 48(1):16–42. doi:10.1016/j.brainresrev.2004.07.021
50. Krumbholz M, Theil D, Derfuss T, Rosenwald A, Schrader F, Monoranu CM, et al. BAFF is produced by astrocytes and upregulated in multiple sclerosis lesions and primary central nervous system lymphoma. J Exp Med (2005) 210:195–200. doi:10.1084/jem.20041674
51. Suzumura A. Neuron-microglia interaction in neuroinflammation. Curr Protein Pept Sci (2013) 14(1):16–20. doi:10.2174/1389203711314010004
52. Kothur K, Weinholt L, Mohammad SS, Tantsis EM, Pillai S, Britton PN, et al. Utility of CSF cytokine/chemokines as markers of active intrathecal inflammation: comparison of demyelinating, anti-NMDAR and enteroviral encephalitis. PLoS One (2016) 11(8):e0161656. doi:10.1371/journal.pone.0161656
53. Kivisäkk P, Mahad DJ, Callahan MK, Sikora K, Trebst C, Tucky B, et al. Expression of CCR7 in multiple sclerosis: implications for CNS immunity. Ann Neurol (2004) 55(5):627–38. doi:10.1002/ana.20049
54. Gadini SP, Wlash JT, Lukens JR, Kipnis J. Dealing with danger in the CNS: the response of the immune system to injury. Neuron (2015) 87(1):47–62. doi:10.1016/j.neuron.2015.05.019
55. Raphael I, Nalawade SD, Eagar TN, Forsthuber TG. T cell subsets and their signature cytokines in autoimmune and inflammatory diseases. Cytokine (2015) 74(120):5–17. doi:10.1016/j.cyto.2014.09.011
56. Cosmi L, Maggi L, Santarlasci V, Liotta F, Annunziato F. T helper cells plasticity in inflammation. Cytometry A (2014) 85(1):36–42. doi:10.1002/cyto.a.22348
57. Blink SE, Caldis MW, Goings GE, Harp CT, Malissen B, Prinz I, et al. γδ T cell subsets play opposing roles in regulating experimental autoimmune encephalitis. Cell Immunol (2014) 290(1):39–51. doi:10.1016/j.cellimm.2014.04.013
58. Blüml S, McKeever K, Ettinger R, Smolen J, Herbst R. B-cell targeted therapeutics in clinical development. Arthritis Res Ther (2013) 15(Suppl 1):54.
59. Krumbholz M, Theil D, Cepok S, Hemmer B, Kivisäkk P, Ransohoff RM, et al. Chemokines in multiple sclerosis: CXCL12 and CXCL13 up-regulation is differentially linked to CNS immune cell recruitment. Brain (2006) 129(Pt 1):200211. doi:10.1093/brain/awh680
60. Sozzani S, Del Prete A, Bosisio D. Dendritic cell recruitment and activation in autoimmunity. J Autoimmun (2017). doi:10.1016/j.jaut.2017.07.012
61. Hamann I, Dörr J, Glumm R, Chanvillard C, Janssen A, Millward JM, et al. Characterization of natural killer cells in paired CSF and blood samples during neuroinflammation. J Neuroimmunol (2013) 254(1–2):165–9. doi:10.1016/j.jneuroim.2012.08.009
62. Roberston MJ. Role of chemokines in the biology of natural killer cells. J Leukoc Biol (2002) 71(2):173–83.
63. Huang DR, Wang J, Kivisakk P, Rollins BJ, Ransohoff RM. Absence of monocyte chemoattractive protein 1 in mice leads to decreased local macrophage recruitment and antigen-specific T helper cell type 1 immune response in experimental autoimmune encephalitis. J Exp Med (2001) 193:713–26. doi:10.1084/jem.193.6.713
64. Aloisi F, Columba-Cabezas S, Franciotta D, Rosicarelli B, Magliozzi R, Reynolds R, et al. Lymphoid chemokines in chronic neuroinflammation. J Neuroimmunol (2008) 198(1–2):106–12. doi:10.1016/j.jneuroim.2008.04.025
65. Pittaluga A. CCL5-glutamate cross-talk in astrocyte-neuron communication in multiple sclerosis. Front Immunol (2017) 8:1079. doi:10.3389/fimmu.2017.01079
66. Tanuma N, Sakuma H, Sasaki A, Matsumoto Y. Chemokine expression by astrocytes play a role in microglia/macrophage activation and subsequent neurodegeneration in secondary progressive multiple sclerosis. Acta Neuropathol (2006) 112(2):195–204. doi:10.1007/s00401-006-0083-7
67. Mirones I, de Prada I, Gómez AM, Luque A, Martín R, Pérez-Jiménez MÁ, et al. A role for the CXCR3/CXCL10 axis in Rasmussen encephalitis. Pediatr Neurol (2013) 49(6):451–7.e1. doi:10.1016/j.pediatrneurol.2013.07.019
68. Gruol DL. IL-6 regulation of synaptic function in the CNS. Neuropharmacology (2015) 96(Pt A):42–54. doi:10.1016/j.neuropharm.2014.10.023
69. Ginhoux F, Greter M, Leboeuf M, Nandi S, See P, Gokhan S, et al. Fate mapping analysis reveals that adult microglia derive from primitive macrophages. Science (2010) 330:841–5. doi:10.1126/science.1194637
70. Hsu MP, Frausto R, Rose-John S, Campbell IL. Analysis of IL-6/gp130 family receptor expression reveals that in contrast to astroglia, microglia lack the oncostatin M receptor and functional responses to oncostatin M. Glia (2015) 63(1):132–41. doi:10.1002/glia.22739
71. Gundersen V, Stoirm-Mathisen J, Bergersen LH. Neuroglial transmission. Physiol Rev (2015) 95(3):695–726. doi:10.1152/physrev.00024.2014
72. Wilson CS, Mongin AA. The signaling role for chloride in the bidirectional communication between neurons and astrocytes. Neurosci Lett (2018). doi:10.1016/j.neulet.2018.01.012
73. Osipova ED, Semyachkina-Glushkovskaya OV, Morgun AV, Pisareva NV, Malinovskaya NA, Boitsova EB, et al. Gliotransmitters and cytokines in the control of blood-brain barrier permeability. Rev Neurosci (2018). doi:10.1515/revneuro-20017-0092
74. Gutcher I, Becher B. APC-derived cytokines and T cell polarization in autoimmune inflammation. J Clin Invest (2007) 117:1119–27. doi:10.1172/JCI31720
75. Musnier A, Blanchot B, Reiter E, Crépieux P. GPCR signaling to the translation machinery. Cell Signal (2010) 22(5):707–16. doi:10.1016/j.cellsig.2009.10.012
76. Gadina M, Gazaninga N, Vian L, Furumoto Y. Small molecules to the rescue: inhibition of cytokine signaling in immune-mediated diseases. J Autoimmun (2017) 85:20–31. doi:10.1016/j.jaut.2017.06.006
77. Cavalli G, Dinarello CA. Suppression of inflammation and acquired immunity by IL-37. Immunol Rev (2018) 281(1):179–90. doi:10.1111/imr.12605
78. Afonina IS, Zhong Z, Karin M, Beyaert R. Limiting inflammation – the negative regulation of NF-κB and the NLRP3 inflammasome. Nat Immunol (2017) 18(8):861–9. doi:10.1038/ni.3772
79. Pranzatelli MR, Tate ED, McGee NR, Travelstead AL, Colliver JA, Ness JM, et al. BAFF/APRIL system in pediatric OMS: relation to severity, neuroinflammation, and immunotherapy. J Neuroinflammation (2013) 10:10. doi:10.1186/1742-2094-10-10
80. Pranzatelli MR, Tate ED, McGee NR, Travelstead AL, Verhulst SJ, Ransohoff RM. Expression of CXCR3 and its ligands CXCL9, -10, -11 in pediatric OMS. Clin Exp Immunol (2013) 172(2):427–36. doi:10.1111/cei.12065
81. Pranzatelli MR, Tate ED, McGee NR, Travelstead AL, Ransohoff RM, Ness JM, et al. Key role of CXCL13/CXCR5 axis for cerebrospinal fluid B cell recruitment in pediatric OMS. J Neuroimmunol (2012) 243(1–2):81–8. doi:10.1016/j.jneuroim.2011.12.014
82. Alvarez E, Piccio L, Mikesell RJ, Klawiter EC, Parks BJ, Naismith RT, et al. CXCL13 is a biomarker of inflammation in multiple sclerosis, neuromyelitis optica, and other neurological conditions. Mult Scler (2013) 19(9):1204–8. doi:10.1177/1352458512473362
83. Sellebjerg F, Börnsen L, Khademi M, Krakauer M, Olsson T, Frederiksen JL, et al. Increased cerebrospinal fluid concentrations of the chemokine CXCL13 in active MS. Neurology (2009) 73(23):2003–10. doi:10.1212/WNL.0b013e3181c5b457
84. Khaibullin T, Ivanova V, Martynova E, Cherepnev G, Khabirov F, Granatov E, et al. Elevated levels of proinflammatory cytokines in cerebrospinal fluid of multiple sclerosis patients. Front Immunol (2017) 8:531. doi:10.3389/fimmu.2017.00531
85. Schwarz A, Balint B, Korporal-Kuhnke M, Jarius S, von Engelhardt K, Füwentsches A, et al. B-cell populations discriminate between pediatric- and adult-onset multiple sclerosis. Neurol Neuroimmunol Neuroinflamm (2017) 4:e309. doi:10.1212/NXI.0000000000000309
86. Horellou P, Wang M, Keo V, Chrétien P, Serguera C, Waters P, et al. Increased interleukin-6 correlates with myelin oligodendrocyte glycoprotein antibodies in pediatric monophasic demyelinating diseases and multiple sclerosis. J Neuroimmunol (2015) 289:1–7. doi:10.1016/j.jneuroim.2015.10.002
87. Melamed E, Levy M, Waters PJ, Sato DK, Bennett JL, John GR, et al. Update on biomarkers in neuromyelitis optica. Neurol Neuroimmunol Neuroinflamm (2015) 2:e134. doi:10.1212/NXI.0000000000000134
88. Uzawa A, Mori M, Arai K, Sato Y, Hayakawa S, Masuda S, et al. Cytokine and chemokine profiles in neuromyelitis optica: significance of interleukin-6. Mult Scler (2010) 16(12):1443–52. doi:10.1177/1352458510379247
89. Wu A, Zhong X, Wang H, Xu W, Cheng C, Dai Y, et al. Cerebrospinal fluid IL-21 levels in neuromyelitis optica and multiple sclerosis. Can J Neurol Sci (2012) 39(6):813–20. doi:10.1017/S0317167100015663
90. Kang H, Cao S, Chen T, Jiang Z, Liu Z, Li Z, et al. The poor recovery of neuromyelitis optica spectrum disorder is associated with a lower level of CXCL12 in the human brain. J Neuroinflammation (2015) 289:56–61. doi:10.1016/j.jneuroim.2015.10.005
91. Ishizu T, Minohara M, Ichiyama T, Kira R, Tanaka M, Osoegawa M, et al. CSF cytokine and chemokine profiles in acute disseminated encephalomyelitis. J Neuroimmunol (2006) 175(1–2):52–8. doi:10.1016/j.jneuroim.2006.03.020
92. Li S, Yu M, Li H, Zhang H, Jiang Y. IL-17 and IL-22 in cerebrospinal fluid and plasma are elevated in Guillain-Barré syndrome. Mediators Inflamm (2012) 2012:260473. doi:10.1155/2012/260473
93. Sato S, Suzuki K, Nagoa R, Kashiwagi Y, Kawashima H, Tsuyuki K, et al. Detection of MCP-1 and IL-8 in the serum and cerebrospinal fluid of a child with Miller Fisher syndrome. J Clin Neurosci (2009) 16(12):1698–9. doi:10.1016/j.jocn.2009.03.044
94. Fühlhuber V, Bick S, Kirsten A, Hahn A, Gerriets T, Tschernatsch M, et al. Elevated B-cell activating factor BAFF, but not APRIL, correlated with CSF cerebellar antibodies in pediatric opsoclonus-myoclonus syndrome. J Neuroimmunol (2009) 210(1–2):87–91. doi:10.1016/j.jneuroim.2009.03.006
95. Pranzatelli MR, McGee NR. Neuroimmunology of OMS and ANNA-1/Anti-Hu paraneoplastic syndromes in a child with neuroblastoma. Neurol Neuroimmunol Neuroinflamm (2018) 5:e433. doi:10.1212/NXI.0000000000000433
96. Leypoldt F, Höftberger R, Titulaer MJ, Armangue T, Gresa-Arribas N, Jahn H, et al. Investigations on CXCL13 in anti-N-methyl-D-aspartate receptor encephalitis: a potential biomarker of treatment response. JAMA Neurol (2015) 72(2):180–6. doi:10.1001/jamaneurol.2014.2956
97. Liba Z, Kayserova J, Elisak M, Marusic P, Nohejlova H, Hanzalova J, et al. Anti-N-methyl-D-aspartate receptor encephalitis: the clinical course in light of the chemokine and cytokine levels in cerebrospinal fluid. J Neuroinflammation (2016) 13(1):55. doi:10.1186/s12974-016-0507-9
98. Church AJ, Dale RC, Cardoso F, Cardoso F, Candler PM, Chapman MD, et al. CSF and serum immune parameters in Sydenham’s chorea: evidence of an autoimmune disease. J Neuroimmunol (2003) 136:149–53. doi:10.1016/S0165-5728(03)00012-2
99. Roberts WK, Blachére NE, Frank MO, Dousmanis A, Ransohoff RM, Darnell RB. A destructive feedback loop mediated by CXCL10 in central nervous system inflammatory disease. Ann Neurol (2015) 78(4):619–29. doi:10.1002/ana.24494
100. Kenney-Jung DL, Vezzani A, Kahoud RJ, LaFrance-Corey RG, Ho ML, Muskardin TW, et al. Febrile infection-related epilepsy syndrome treated with anakinra. Ann Neurol (2016) 80:939–45. doi:10.1002/ana.24806
101. Takahashi Y, Mine J, Kubota Y, Yamazaki E, Fujiwara T. A substantial number of Rasmussen syndrome patients have increased IgG, CD4+ T cells, TNFα, and Granzyme B in CSF. Epilepsia (2009) 50(6):1419–1143. doi:10.1111/j.1528-1167.2008.01977.x
102. Tekgul H, Polat M, Tosun A, Serdaroglu G, Kutukculer N, Gokben S. Cerebrospinal fluid interleukin-6 levels in patients with West syndrome. Brain Dev (2006) 28:19–23. doi:10.1016/j.braindev.2005.03.007
103. Haginoya K, Noguchi R, Zhao Y, Munakata M, Yokoyama H, Tanaka S, et al. Reduced levels of interleukin-1 receptor antagonists in the cerebrospinal fluid in patients with West syndrome. Epilepsy Res (2009) 85(2–3):314–7. doi:10.1016/j.eplepsyres.2009.03.013
104. Van Heteren JT, Rozenberg F, Aronica E, Troost D, Lebon P, Kuijpers TW. Astrocytes produce interferon-alpha and CXCL10, but not IL-6 or CXCL8, in Aicardi-Goutières syndrome. Glia (2008) 56(5):568–78. doi:10.1002/glia.20639
105. Fragoso-Loyo H, Richaud-Patin Y, Orozco-Narváez A, Dávila-Maldonado L, Atisha-Fregoso Y, Llorente L, et al. Interleukin-6 and chemokines in the neuropsychiatric manifestations of systemic lupus erythematosus. Arthritis Rheum (2007) 56(4):1242–50. doi:10.1002/art.22451
106. Yoshio T, Okamoto H, Kurasawa K, Dei Y, Hirohata S, Minota S. IL-6, IL-8, IP-10, MCP-1 and G-CSF are significantly increased in cerebrospinal fluid but not in sera of patients with central neuropsychiatric lupus erythematosus. Lupus (2016) 25(9):997–1003. doi:10.1177/0961203316629556
107. Ichinose K, Arima K, Ushigusa T, Nishino A, Nakashima Y, Suzuki T, et al. Distinguishing the cerebrospinal fluid cytokine profile in neuropsychiatric systemic lupus erythematosus from other autoimmune neurological diseases. Clin Immunol (2015) 157(2):114–20. doi:10.1016/j.clim.2015.01.010
108. Pranzatelli MR, McGee NR, Wang ZY, Agrawal BK. Characteristics and pharmacodynamics of severe neuroinflammation in a child with neurolupus. Neurol Neuroimmunol Neuroinflamm (2016) 4(2):e316. doi:10.1212/NXI.0000000000000316
109. Akman-Demir G, Tüzün E, Içöz S, Yeşilot N, Yentür SP, Kürtüncü M, et al. Interleukin-6 in neuro-Behçet’s disease: association with disease subsets and long-term outcome. Cytokine (2008) 44(3):373–6. doi:10.1016/j.cyto.2008.10.007
110. Hatachi S, Nakazawa T, Morinobu A, Kasagi S, Kogata Y, Kageyama G, et al. A pediatric patient with neuro-Behçet’s disease. Mod Rheumatol (2006) 16(5):321–3. doi:10.3109/s10165-006-0507-1
111. Tarkowski E, Rosengren L, Blomstrand C, Wikkelsö C, Jensen C, Ekholm S, et al. Intrathecal release of pro- and anti-inflammatory cytokine during stroke. Clin Exp Immunol (1997) 110(3):492–9. doi:10.1046/j.1365-2249.1997.4621483.x
112. Zaremba J, Skrobański P, Losy J. The level of chemokine CXCL5 in the cerebrospinal fluid is increased during the first 24 hours of ischaemic stroke and correlates with the size of early brain damage. Folia Morphol (Warsz) (2016) 65(1):1–5.
113. Rodriguez-Smith J, Lin YC, Li Tsai W, Kim H, Montealegre-Sanchez G, Chapelle D, et al. CSF cytokines correlate with aseptic meningitis and blood brain barrier function in Neonatal-Onset Multisystem Inflammatory Disease (NOMID). Arthritis Rheumatol (2017). doi:10.1002/art.40055
114. Buttram SD, Wisniewski SR, Jackson EK, Adelson PD, Feldman K, Bayir H, et al. Multiplex assessment of cytokine and chemokine levels in cerebrospinal fluid following severe pediatric traumatic brain injury: effects of moderate hypothermia. J Neurotrauma (2007) 24(11):1707–17. doi:10.1089/neu.2007.0349
115. Amick JE, Yandora KA, Bell MJ, Wisniewski SR, Adelson PD, Carcillo JA, et al. The Th1 versus Th2 cytokine profile in cerebrospinal fluid after severe traumatic brain injury in infants and children. Pediatr Crit Care Med (2001) 2(3):260–4. doi:10.1097/00130478-200107000-00013
116. Habiyaremyew G, Morales DM, Morgan CD, McAllister JP, CreveCoeur TS, Han RH, et al. Chemokine and cytokine levels in the lumbar cerebrospinal fluid of preterm infants with post-hemorrhagic hydrocephalus. Fluids Barriers CNS (2017) 14(1):35. doi:10.1186/s12987-017-0083-0
117. Tanaka M, Matsushita T, Tateishi T, Ochi H, Kawano Y, Mei FJ, et al. Distinct CSF cytokine/chemokine profiles in atopic myelitis and other causes of myelitis. Neurology (2008) 71(13):974–81. doi:10.1212/01.wnl.0000326589.57128.c3
118. Hoshina T, Yamaguchi Y, Ohga S, Kira R, Ishmura M, Takada H, et al. Sjögren’s syndrome-associated meningoencephalomyelitis: cerebrospinal fluid cytokine levels and therapeutic utility of tacrolimus. J Neurol Sci (2008) 267(1–2):182–6. doi:10.1016/j.jns.2007.10.009
119. Dixit P, Garg RK, Malhotra HS, Jain A, Verma R, Sharma PK, et al. Cytokines and matrix metalloproteinases in the cerebrospinal fluid of patients with acute transverse myelitis: an outcome analysis. Inflamm Res (2016) 65(2):125–32. doi:10.1007/s00011-015-0896-8
120. Yamasaki R, Yamaguchi H, Matsushita T, Fujii T, Hiwatashi A, Kira JI. Early strong intrathecal inflammation in cerebellar type multiple system atrophy by cerebrospinal fluid cytokine/chemokine profiles: a case control study. J Neuroinflammation (2017) 14(1):89. doi:10.1186/s12974-017-0863
121. Thibert KA, Raymond GV, Tolar J, Miller WP, Orchard PJ, Lund TC. Cerebral spinal fluid levels of cytokines are elevated in patients with metachromatic leukodystrophy. Sci Rep (2016) 15(6):24579. doi:10.1038/srep24579
122. Lund TC, Stadem PS, Panoskaltsis-Mortari A, Raymond G, Miller WP, Tolar J, et al. Elevated cerebral spinal fluid cytokine levels in boys with cerebral adrenoleukodystrophy correlates with MRI severity. PLoS One (2012) 7(2):e32218. doi:10.1371/journal.pone.0032218
123. Mellergård J, Edström M, Vrethem M, Ernerudh J, Dahle C. Natalizumab treatment in multiple sclerosis: marked decline of chemokines and cytokines in cerebrospinal fluid. Mult Scler (2010) 16(2):208–17. doi:10.1177/1352458509355068
124. Maric LS, Lepej SZ, Gorenec L, Grgic I, Trkulja V, Rode IOD, et al. Chemokines CXCL10, CXCL11, and CXCL13 in acute disseminated encephalomyelitis, non-polio enterovirus aseptic meningitis, and neuroborreliosis: CXCL10 as initial discriminator in diagnostic algorithm? Neurol Sci (2017). doi:10.1007/s10072-017-3227-8
125. Takanohashi A, Prust M, Wang J, Gordish-Dressman H, Bloom M, Rice GI, et al. Elevation of proinflammatory cytokines in patients with Aicardi-Goutières syndrome. Neurology (2013) 80:997–1002. doi:10.1212/WNL.0b013e3182872694
126. Utz JR, Crutcher T, Schneider J, Sorgen P, Whitley CB. Biomarkers of central nervous system inflammation in infantile and juvenile gangliosidoses. Mol Genet Metab (2015) 1114(2):274–80. doi:10.1016/j.ymgme.2014.11.015
127. Potter GB, Petryniak MA. Neuroimmune mechanisms in Krabbe’s disease. J Neurosci Res (2016) 94(11):1341–8. doi:10.1002/jnr.23804
128. Brettschneider J, Czerwoniak A, Senel M, Fang L, Kasasubek J, Oibnkhardt E, et al. The chemokine CXCL13 is a prognostic marker in clinically isolated syndrome (CIS). PLoS One (2010) 5(8):e11986. doi:10.1371/journal.pone.0011986
129. Orbach R, Gurevich M, Achiron A. Interleukin-12p40 in the spinal fluid as a biomarker for clinically isolated syndrome. Mult Scler (2014) 20(1):35–42. doi:10.1177/1352458513491166
130. Lebrun C, Cohen M, Pignolet B, Seitz-Polski B, Bucciarelli F, Benzaken S, et al. Interleukin 17b alone is not a discriminant biomarker ion early demyelinating spectrum disorders. J Neurol Sci (2016) 368:334–6. doi:10.1016/j.jns.2016.07.052
131. Teleshova N, Pashenkov M, Huang YM, Söderström M, Kivisäkk P, Kostulas V, et al. Multiple sclerosis and optic neuritis: CCR5 and CXCR3 expressing T cells are augmented in blood and cerebrospinal fluid. J Neurol (2002) 249(6):723–9. doi:10.1007/s00415-002-0699-z
132. Nakajima H, Sugino M, Kimura F, Hanafusa T, Ikemoto T, Shimizu A. Increased intrathecal chemokine receptor CCR2 expression in multiple sclerosis. Biomark Insights (2007) 2:463–8. doi:10.1177/117727190700200028
133. Sato W, Tomita A, Ichikawa D, Lin Y, Kishida H, Miyake S, et al. CCR2(+) CCR5(+) T cells produce matrix metalloproteinase-9 and osteopontin in the pathogenesis of multiple sclerosis. J Immunol (2012) 189(10):5057–65. doi:10.4049/jimmunol.1202026
134. Ye Q, Shao W-X, Shang S-Q, Shen H-Q, Chen X-J, Tang Y-M, et al. Clinical value of assessing cytokine levels for the differential diagnosis of bacterial meningitis in a pediatric population. Medicine (Baltimore) (2016) 95(13):e3222. doi:10.1097/MD.0000000000003222
135. Asano T, Ichiki K, Koizumi S, Kaizu K, Hatori T, Fujino O, et al. IL-17 is elevated in cerebrospinal fluids in bacterial meningitis in children. Cytokine (2010) 51(1):101–6. doi:10.1016/j.cyto.2010.03.001
136. Haspolat S, Mihçi E, Coşkun M, Gümüslü S, Ozben T, Yeğin O. Interleukin-1beta, tumor necrosis factor-alpha, and nitrite levels in febrile seizures. J Child Neurol (2002) 17(10):749–51. doi:10.1177/08830738020170101501
137. Pashenkov M, Teleshova N, Kouwenhoven M, Smirnova T, Jin YP, Kostulas V, et al. Recruitment of dendritic cells to the cerebrospinal fluid in bacterial neuroinfections. J Neuroimmunol (2002) 122(1–2):106–16. doi:10.1016/S0165-5728(01)00451-9
138. Henningsson AJ, Tjernberg I, Malmvall B-E, Forsberg P, Ernerudh J. Indications of Th1 and Th17 responses in cerebrospinal fluid from patients with Lyme neuroborreliosis: a large retrospective study. J Neuroinflammation (2011) 8:36. doi:10.1186/1742-2094-8-36
139. Rupprecht TA, Pfister HW, Angele B, Kastenbauer S, Wilske B, Koedel U. The chemokine CXCL13 (BLC): a putative diagnostic marker for neuroborreliosis. Neurology (2005) 65:448–50. doi:10.1212/01.wnl.0000171349.06645.79
140. Grusell M, Widhe M, Ekerfelt C. Increased expression of the Th1-inducing cytokines interleukin-12 and interleukin-18 in cerebrospinal fluid but not sera from patients with Lyme neuroborreliosis. J Neuroimmunol (2002) 131:173–8. doi:10.1016/S0165-5728(02)00255-2
141. Van Burgel ND, Bakels F, Kroes AC, van Dam AP. Discriminating Lyme neuroborreliosis from other neuroinflammatory diseases by levels of CXCL13 in cerebrospinal fluid. J Clin Microbiol (2011) 49:2027–30. doi:10.1128/JCM.00084-11
142. Wutte N, Berghold A, Loffler S, Zenz W, Daghofer E, Krainberger I, et al. CXCL13 chemokine in pediatric and adult neuroborreliosis. Acta Neurol Scand (2011) 124:321–8. doi:10.1111/j.1600-0404.2010.01477.x
143. Liba Z, Kayserova J, Komarek V. Th1 and Th17 but no Th2-related cytokine spectrum in the cerebrospinal fluid of children with Borrelia-related facial nerve palsy. Fluids Barriers CNS (2013) 10:30. doi:10.1186/2045-8118-10-30
144. Dersch R, Hottenrott T, Senbel M, Lehmensiek V, Tumani H, Pauer S, et al. The chemokine CXCL13 is elevated in the cerebrospinal fluid of patients with neurosyphilis. Fluids Barriers CNS (2015) 12:12. doi:10.1186/s12987-015-0008-8
145. Mothapo KM, Verbeek MM, van der Velden LB, Ang CW, Koopmans PP, van der Ven A, et al. Has CXCL13 an added value in diagnosis of neurosyphilis. J Clin Microbiol (2015) 53(5):1693–6. doi:10.1128/JCM.02917-14
146. Narita M, Tanaka H, Togashi T, Abe S. Cytokines involved in CNS manifestations caused by Mycoplasma pneumoniae. Pediatr Neurol (2005) 33(2):105–9. doi:10.1016/j.pediatrneurol.2005.03.003
147. Grygorczuk S, Parczewski M, wierzbińska R, Czupryna P, Moniuszko A, Dunaj J, et al. The increased concentration of macrophage migration inhibitory factor in serum and cerebrospinal fluid of patients with tick-borne encephalitis. J Neuroinflammation (2017) 14(1):126. doi:10.1186/s12974-017-0898-2
148. Fowler A, Ygberg S, Bogdanovic G, Wickström R. Biomarkers in cerebrospinal fluid of children with tick-borne encephalitis: association with long-term outcome. Pediatr Infect Dis J (2016) 35(9):961–6. doi:10.1097/INF.0000000000001210
149. Kalita J, Srivastava R, Mishra MK, Basu A, Misra UK. Cytokines and chemokines in viral encephalitis: a clinicoradiological correlation. Neurosci Lett (2010) 473(1):48–51. doi:10.1016/j.neulet.2010.02.017
150. Ygberg S, Fowler A, Wickström R. Cytokine and chemokine expression in CSF may differentiate viral and autoimmune NMDAR encephalitis in children. J Child Neurol (2016) 31(13):1450–6. doi:10.1177/0883073816653780
151. Lind L, Studahl M, Berg LP, Eriksson K. CXCL11 production in cerebrospinal fluid distinguishes herpes simplex meningitis from herpes simplex encephalitis. J Neuroinflammation (2017) 14:134. doi:10.1186/s12974-017-0907-5
152. Li H, Li S, Zheng J, Cai C, Ye B, Yang J, et al. Cerebrospinal fluid Th1/Th2 cytokine profiles in children with enterovirus 71-associated meningoencephalitis. Microbiol Immunol (2015) 59(3):152–9. doi:10.1111/1348-0421.12227
153. Asano T, Ichiki K, Koizumi S, Kaizu K, Hatori T, Fujino O, et al. Enhanced expression of cytokines/chemokines in cerebrospinal fluids in mumps meningitis in children. Pediatr Int (2011) 53(2):143–6. doi:10.1111/j.1442-200X.2010.03215.x
154. De Almeida SM, Rotta I, Jiang Y, Raboni SM, Ribeiro CE, Smith D, et al. Biomarkers of chemotaxis and inflammation in cerebrospinal fluid and serum in individuals with HIV-1 subtype C versus B. J Neurovirol (2016) 22(6):715–24. doi:10.1007/s13365-016-0437-4
155. Dickson DW, Llena JF, Nelson SJ, Weidenheim KM. Central nervous system pathology in pediatric AIDS. Ann N Y Acad Sci (1993) 693:93–106. doi:10.1111/j.1749-6632.1993.tb26259.x
156. Saruhan-Direskeneli G, Gürses C, Demirbilek V, Yentür SP, Yilmaz G, Onal E, et al. Elevated interleukin-12 and CXCL10 in subacute sclerosing panencephalitis. Cytokine (2005) 32(2):104–10. doi:10.1016/j.cyto.2005.08.004
157. Aydin OF, Ichiyama T, Anlar B. Serum and cerebrospinal fluid cytokine concentrations in subacute sclerosing panencephalitis. Brain Dev (2010) 32(6):463–6. doi:10.1016/j.braindev.2009.04.018
158. Ichiyama T, Siba P, Suarkia D, Reeder J, Takasu T, Miki K, et al. Analysis of serum and cerebrospinal fluid cytokine levels in subacute sclerosing panencephalitis in Papua New Guinea. Cytokine (2006) 33(1):17–20. doi:10.1016/j.cyto.2005.11.009
159. Mehta PD, Kulczycki J, Mehta SP, Coyle PK, Wisniewski HM. Increased levels of interleukin-1beta and soluble intercellular adhesion molecule-1 in cerebrospinal fluid of patients with subacute sclerosing panencephalitis. J Infect Dis (1997) 175(3):689–92. doi:10.1093/infdis/175.3.689
160. Gerber J, Nau R. Mechanisms of injury in bacterial meningitis. Curr Opin Neurol (2010) 23(3):312–8. doi:10.1097/WCO.0b013e32833950dd
161. Swanson PA 2nd, McGavern DB. Viral diseases of the central nervous system. Curr Opin Virol (2015) 11:44–54. doi:10.1016/j.coviro.2014.12.009
162. Cerar T, Ogrinc K, Lotrič-Furlan S, Kobal J, Levičnik-Stezinar S, Strle F, et al. Diagnostic value of cytokines and chemokines in Lyme neuroborreliosis. Clin Vaccine Immunol (2013) 20(10):1578–84. doi:10.1128/CVI.00353-13
163. Srinivasan L, Kilpatrick L, Shah SS, Abbasi S, Harris MC. Cerebrospinal fluid cytokines in the diagnosis of bacterial meningitis in infants. Pediatr Res (2016) 80(4):566–72. doi:10.1038/pr.2016.117
164. Prasad R, Kapoor R, Srivastava R, Mishra OP, Singh TB. Cerebrospinal fluid TNF-α, IL-6, and IL-8 in children with bacterial meningitis. Pediatr Neurol (2014) 50(1):60–5. doi:10.1016/j.pediatrneurol.2013.08.016
165. Coutinho LG, Grandgirard D, Leib SL, Agnez-Lima LF. Cerebrospinal-fluid cytokine and chemokine profile in patients with pneumococcal and meningococcal meningitis. BMC Infect Dis (2013) 13:326. doi:10.1186/1471-2334-13-326
166. Grandgirard D, Gäumann R, Coulibaly B, Dangy JP, Sie A, Junghanss T, et al. The causative pathogen determines the inflammatory profile in cerebrospinal fluid and outcome in patients with bacterial meningitis. Mediators Inflamm (2013) 2013:312476. doi:10.1155/2013/312476
167. Li H, Li Y, Wen B, Zhang J, Wang C, Song Z, et al. Dengue virus and Japanese encephalitis virus infection of the central nervous system share similar profiles of cytokine accumulation in cerebrospinal fluid. Cent Eur J Immunol (2017) 42(2):218–22. doi:10.5114/ceji.2017.69366
168. Bastos MS, Coelho-dos-Reis JG, Zauli DAG, Naveca FG, Monte RL, Pimentel JP, et al. Divergent cerebrospinal fluid cytokine network induced by non-viral and different viral infections on the central nervous system. BMC Infect Dis (2015) 15:345. doi:10.1186/s12879-015-12035-4
169. Ito Y, Torii Y, Ohta R, Imai M, Hara S, Kawano Y, et al. Increased levels of cytokines and high-mobility group box 1 are associated with the development of severe pneumonia, but not acute encephalopathy, in 2009 H1N1 influenza-infected children. Cytokine (2011) 56:180–7. doi:10.1016/j.cyto.2011.07.016
170. Getts DR, Chastain EML, Terry RL, Miller SD. Virus infection, antiviral immunity, and autoimmunity. Immunol Rev (2013) 255(1):197–209. doi:10.1111/imr.12091
171. Lopalco L. CCR5: from natural resistance to a new anti-HIV strategy. Viruses (2010) 2:574–600. doi:10.3390/v2020574
172. Scholten DJ, Canals M, Maussang D, Roumen I, Smit MJ, Wijtmans M, et al. Pharmacological modulation of chemokine receptor function. Br J Pharmacol (2011) 165:1617–43. doi:10.1111/j.1476-5381.2011.01551.x
173. Olson JK, Croxford JL, Miller SD. Virus-induced autoimmunity: potential role of viruses in initiation, perpetuation, and progression of T-cell-mediated autoimmune disease. Viral Immunol (2001) 14(3):227–50. doi:10.1089/088282401753266756
174. Galli J, Clardy SL, Piquet AL. NMDAR encephalitis following herpes simplex virus encephalitis. Curr Infect Dis Rep (2017) 19(1):1. doi:10.10007/s11908-017-0556-y
175. Bogdanos DP, Smyk DS, Invernizzi P, Rigopoulou EI, Blank M, Pouria S, et al. Infectome: a platform to trace infectious triggers of autoimmunity. Autoimmun Rev (2013) 12(7):726–40. doi:10.1016/j.autrev.2012.12.005
176. Pranzatelli MR. Introduction [to special issue on immune mechanisms in neurological disorders]. Semin Pediatr Neurol (2017) 24(3):143–4. doi:10.1016/j.spen.2017.08.009
177. Abboud D, Daubeuf F, Do QT, Utard V, Villa P, Haiech J, et al. A strategy to discover decoy chemokine ligands with an anti-inflammatory activity. Sci Rep (2015) 5:14746. doi:10.1038/srep14746
178. Proudfoot AE, Bonvin P, Power CA. Targeting chemokines: pathogens do it, why can’t we? Cytokine (2015) 74(2):259–67. doi:10.1016/j.cyto.2015.02.011
179. Szekanecz Z, Koch AE. Successes and failures of chemokine-pathway targeting in rheumatoid arthritis. Nat Rev Rheumatol (2016) 12:5–13. doi:10.1038/nrrheum.2015.157
180. Horenstein AL, Chillemi A, Quarona V, Zito A, Mariani V, Faini AC, et al. Antibody mimicry, receptors and clinical applications. Hum Antibodies (2017) 25(3–4):75–85. doi:10.3233/HAB-160305
181. World Health Organization. General Policies for Monoclonal Antibodies. INN Working Document 09.251. (2009). Available from: http://www.who.int/medicines/services/inn/en/index.html (Accessed: March 17, 2018).
182. World Health Organization. Revised Monoclonal Antibody (mAb) Nomenclature Scheme. INN Working Doc. 17.416. Geneva (2017). Available from: www.who.int/entity/medicines/services/inn/Revised_mAb_nomenclature_scheme.pdf
183. Huang CJ, Lowe AJ, Batt CA. Recombinant immunotherapeutics: current state and perspectives regarding the feasibility and market. Appl Microbiol Biotechnol (2010) 87(2):401–10. doi:10.1007/s00253-010-2590-7
184. Scott LJ. Sarilumab: first global approval. Drugs (2017) 77(6):705–12. doi:10.1007/s40265-017-0724-2
185. Kampan NC, Xiang SD, McNally OM, Stephens AN, Quinn MA, Plebanski M. Immunotherapeutic interleukin-6 or interleukin-6 receptor blockade in cancer: challenges and opportunities. Curr Med Chem (2017). doi:10.2174/0929867324666170712160621
186. Bruce VJ, Ta AN, McNaughton BR. Minimalist antibodies and mimetics: an update and recent applications. Chembiochem (2016) 17(20):1892–9. doi:10.1002/cbic.201600303
187. Blanchelot C, Verzijl D, Mujić-Delić A, Bosch L, Rem L, Leurs R, et al. Neutralizing nanobodies targeting diverse chemokines effectively inhibit chemokine function. J Biol Chem (2013) 288(35):25173–82. doi:10.1074/jbc.M113.467969
188. Drutskaya MS, Efimov GA, Kruglov AA, Nedospasov SA. Can we design a better anti-cytokine therapy? J Leukoc Biol (2017) 102(3):783–90. doi:10.1189/jlb.3MA0117-025R
189. Robert R, Juglair L, Lim EX, Ang C, Wang CJH, Ebert G, et al. A fully humanized IgG-like bispecific antibody for effective dual targeting of CXCR3 and CCR6. PLoS One (2017) 12(9):e018278. doi:10.1371/journal.pone.0184278
190. Gebauer M, Skerra A. Engineered protein scaffolds as next-generation antibody therapeutics. Curr Opin Chem Biol (2009) 13(3):245–55. doi:10.1016/j.cbpa.2009.04.627
191. Yu X, Yang YP, Dikici E, Deo SK, Daunerts S. Beyond antibodies as binding partners: the role of antibody mimetics in bioanalysis. Annu Rev Anal Chem (Palo Alto Calif) (2017) 10(1):293–320. doi:10.1146/annurev-anchem-061516-045205
192. Ng S, Galipeau J. Concise review: engineering the fusion of cytokines for the modulation of immune cellular responses in cancer and autoimmune disorders. Stem Cells Transl Med (2015) 4:66–73. doi:10.5966/sctm.2014-0145
193. Sanchez-Lugo YE, Perez-Trujillo JJ, Gutierrez-Puente Y, Garcia-Garcia A, Rodriguez-Rocha H, Barboza-Quintana O, et al. CXCL10/XCL1 fusokine elicits in vitro and in vivo chemotaxis. Biotechnol Lett (2015) 37(4):779–85. doi:10.1007/s10529-014-1746-4
194. Williams P, Galipeau J. GM-CSF-based fusion cytokines as ligands for immune modulation. J Immunol (2011) 186:5527–32. doi:10.4049/jimmunol.1003699
195. Deng J, Galipeau J. Reprogramming of B cells into regulatory cells with engineered fusokines. Infect Disord Drug Targets (2012) 12(3):248–54. doi:10.2174/187152612800564392
196. Ng S, Deng J, Chinnadurai R, Yuan S, Pennati A, Galipeau J. Stimulation of natural killer cell-mediated tumor immunity by an IL-15/TGF-β neutralizing fusion protein. Cancer Res (2016) 76(19):5683–95. doi:10.1158/0008-5472.CAN-16-0386
197. Wallisch JS, Simon DW, Bayir H, Bell MJ, Kochanek PM, Clark RSB. Cerebrospinal fluid NLRP3 is increased after severe traumatic brain injury in infants and children. Neurocrit Care (2017) 27(1):44–50. doi:10.1007/s12028-017-0378-7
198. Song L, Pei L, Yao S, Wu Y, Shang Y. NLRP3 inflammation in neurological diseases, from functions to therapies. Front Cell Neurosci (2017) 11:6. doi:10.3389/fncel.2017.00063
199. Baker KF, Isaacs JD. Novel therapies for immune-mediated inflammatory diseases: what can we learn from their use in rheumatoid arthritis, spondyloarthritis, systemic lupus erythematous, psoriasis, Crohn’s disease and ulcerative colitis? Ann Rheum Dis (2017). doi:10.1136/annrheumdis-2017-211555
200. Kuemmerle-Deschner JB, Ramos E, Blank N, Roeslker J, Felix SD, Jung T, et al. Canakinumab (ACZ885, a fully human IgG1 anti-IL-1β mAb) induces sustained remission in pediatric patients with cryopyrin-associated periodic syndrome (CAPS). Arthritis Res Ther (2011) 13(1):R34. doi:10.1186/ar3266
201. Sibley CH, Chiota A, Felix S, Colin L, Chakraborty A, Plass N, et al. A 24-month open-label study of canakinumab in neonatal-onset multisystem inflammatory disease. Ann Rheum Dis (2015) 74(9):1714–9. doi:10.1136/annrheumdis-2013-204877
202. Pavord ID, Chanez P, Criner GJ, Kerstjens HAM, Korn S, Lugogo N, et al. Mepolizumab for eosinophilic chronic obstructive pulmonary disease. N Engl J Med (2017) 377(17):1613–29. doi:10.1056/NEJMoa1708208
203. Markowitz JE, Jobe L, Miller M, Frost C, Laney Z, Eke R. Safety and efficacy of reslizumab for children and adolescents with eosinophilic esophagitis treated over nine years. J Pediatr Gastroenterol Nutr (2017). doi:10.1097/MPG.0000000000001840
204. Casper C, Chaturvedi S, Munshi N, Wong R, Ming Q, Schaffer M, et al. Analysis of inflammatory and anemia-related biomarkers in a randomized, double-blind, placebo-controlled study of siltuximab (anti-IL6 monoclonal antibody) in patients with multicentric Castleman diseases. Clin Cancer Res (2015) 21(19):4294–304. doi:10.1158/1078-0432.CCR-15-0134
205. Chen R, Chen B. Siltuximab (CNTO 328): a promising option for human malignancies. Drug Des Devel Ther (2015) 9:3455–8. doi:10.2147/DDDT.S86438
206. Mease PJ, Gottlieb AB, Berman A, Drescher E, Xing J, Wong R, et al. The efficacy and safety of clazakizumab, an anti-interleukin-6 monoclonal antibody, in a phase IIb study of adults with active psoriatic arthritis. Arthritis Rheumatol (2016) 68(9):2163–73. doi:10.1002/art.39700
207. Segal BM, Constantinescu CS, Raychaudhuri A, Kim L, Fidelus-Gort R, Kasper LH. Repeated subcutaneous injections of IL12/23 p40 neutralizing antibody, ustekinumab, in patients with relapsing-remitting multiple sclerosis: a phase II, double-blind, placebo-controlled, randomized, dose-ranging study. Lancet Neurol (2008) 7(9):796–804. doi:10.1016/S1474-4422(08)70173-X
208. Gordon KB, Langley RG, Gottlieb AB, Papp KA, Krueger GC, Strober BE, et al. A phase III, randomized, controlled trial of the fully human IL-12/23 mAb briakinumab in moderate-to-severe psoriasis. J Invest Dermatol (2012) 132(2):304–14. doi:10.1038/jid.2011.304
209. Hanania NA, Korenblat P, Chapman KR, Bateman ED, Kopecky P, Paggiaroi P, et al. Efficacy and safety of lebrikizumab in patiuents with untrolled asthma (LAVOLTA I and LAVOLTA II): replicate, phase 3, randomized, double-blind, placebo-controlled trials. Lancet Resp Med (2016) 4(10):781–96. doi:10.1016/S2213-2600(16)30265-X
210. Miller JS, Morishima C, McNeel DG, Patel MR, Kohrt HE, Thompson JA, et al. A first-in-human phase 1 study of subcutaneous outpatient recombinant human (rhIL-15) in adults with advanced solid tumors. Clin Cancer Res (2017). doi:10.1158/1078-0432.CCR-17-2451
211. Jeon C, Sekhon S, YTan D, Afifi L, Nakamura M, Bhutani T. Monoclonal antibodies inhibiting IL-12, -23, and -17 for the treatment of psoriasis. Hum Vaccin Immunother (2017) 13(10):2247–59. doi:10.1080/21645515.2017.1356498
212. Van der Heijde D, Gladman DD, Kishimoto M, Okada M, Rathman SS, Moriarty SR, et al. Efficacy and safety of Ixekizumab in patients with active psoriatic arthritis: 52-week results from a phase III study (SPIRIT-PI). J Rheumatol (2017). doi:10.3899/jrheum.170429
213. Šenolt L, Leszczynski P, Dokoupilová E, Göthberg M, Valencia X, Hansen BB, et al. Efficacy and safety of anti-interleukin-20 monoclonal antibody in patients with rheumatoid arthritis: a randomized phase IIa trial. Arthritis Rheumatol (2015) 67(6):1438–48. doi:10.1002/art.39083
214. Guttman-Yassky E, Brunner PM, Neumann AU, Khattri S, Pavel AB, Malik K, et al. Efficacy and safety of fezakinumab (an anti-IL-22 monoclonal antibody) in adults with moderate-to-severe atopic dermatitis inadequately controlled by conventional treatments – a randomized, double-blind, phase 2a trial. J Am Acad Dermatol (2018). doi:10.1016/j.jaad.2018.01.016
215. Nemoto O, Hirose K, Shibata S, Li K, Kubo H. Safety and efficacy of guselkumab in Japanese patients with moderate-to-severe plaque psoriasis: a randomized, placebo-controlled, ascending-dose study. Br J Dermatol (2017). doi:10.1111/bjd.16236
216. De Gramont A, Faivre S, Raymond E. Novel TGF-β inhibitors ready for prime time in onco-immunology. Oncoimmunology (2016) 7(1):e1257453. doi:10.1080/2162402X.2016.1257453
217. Atsumi T, Tanaka Y, Yamamoto K, Takeuchi T, Yamanaka H, Ishiguro N, et al. Clinical benefit of 1-year certolizumab pegol (CZP) add-on therapy to methotrexate treatment in patients with early rheumatoid arthritis was observed following CZP discontinuation: 2-year results of the C-OPERA study, a phase III randomized trial. Ann Rheum Dis (2017) 76(8):1348–56. doi:10.1136/annrheumdis-2016-210246
218. Gelfand JM, Bradshaw MJ, Stern BJ, Clifford DB, Wang Y, Cho TA, et al. Infliximab for the treatment of CNS sarcoidosis: a multi-institutional series. Neurology (2017) 89(20):2092–100. doi:10.1212/WNL.0000000000004644
219. Silacci M, Lembke W, Woods R, Attinger-Toller I, Baenziger-Tobler N, Batey S, et al. Discovery and characterization of COVA322, a clinical-stage bispecific TNF/IL-17A inhibitor for the treatment of inflammatory diseases. MAbs (2016) 8:141–9. doi:10.1080/19420862.2015.1093266
220. Constantinesacu CS, Asher A, Fryze W, KLozubski W, Wagnber F, Aram J, et al. Randomized phase 1b trial of MOR103, a human antibody to GM-CSF, in multiple sclerosis. Neurol Neuroimmunol Neuroinflamm (2015) 2(4):e117. doi:10.1212/NXI.0000000000000117
221. Stohl W, Schwarting A, Okada M, Scheinberg M, Doria A, Hammer AE, et al. Efficacy and safety of subcutaneous belimumab in systemic lupus erythematosus: a fifty-two-week randomized, double-blind, placebo-controlled study. Arthritis Rheumatol (2017) 69(5):1016–27. doi:10.1002/art.40049
222. Lenert A, Lenert P. Current and emerging treatment options for ANCA-associated vasculitis: potential role of belimumab and other BAFF/APRIL targeting agents. Drug Des Devel Ther (2015) 9:333–47. doi:10.2147/DDDT.S67264
223. Furie RA, Leon G, Thomas M, Petri MA, Chu ASD, Hislop C, et al. A phase 2, randomized, placebo-controlled clinical trial of blisibimod, an inhibitor of B cell activating factor, in patients with moderate-to-severe systemic lupus erythematosus, the PEARL-SC study. Ann Rheum Dis (2015) 74(9):1667–75. doi:10.1136/annrheumdis-2013-205144
224. Merrill JT, Wallace DJ, Wax S, Kao A, Fraser PA, Chang P, et al. Efficacy and safety of atacicept in patients with systemic lupus erythematosus: results of a twenty-four-week, multicenter, randomized, double-blind, placebo-controlled, parallel-arm, phase IIb study. Arthritis Rheumatol (2018) 70(2):266–76. doi:10.1002/art.40360
225. Kalunian KC, Merrill JT, Maciuca R, McBride JM, Townsend MJ, Wei X, et al. A phasae II study of the efficacy and safety of rontalizumab (rhuMAb interferon-α) in patients with systemic lupus erythematosus (ROSE). Ann Rheum Dis (2016) 75(1):196–202. doi:10.1136/annrheumdis-2014-206090
226. Khamashta M, Merrill JT, Werth VP, Furie R, Kalunian K, Illei CG, et al. Sifalimumab, an anti-interferon-α monoclonal antibody, in moderate to sever systemic lupus erythematosus: a randomized, double-blind, placebo-controlled study. Ann Rheum Dis (2016) 75(11):1909–16. doi:10.1136/annrheumdis-2015-208562
227. Yellen M, Paliienko I, Balanescu A, Ter-Vartanian S, Tseluyko V, Xu LA, et al. A phase II, randomized, double-blind, placebo-controlled study evaluating the efficacy and safety of MDX-1100, a fully human anti-CXCL10 monoclonal antibody, in combination with methotrexate in patients with rheumatoid arthritis. Arthritis Rheum (2012) 64(6):1730–9. doi:10.1002/art.34330
228. Orange D, Frank M, Tian S, Dousmanis A, Marmur R, Buckley N, et al. Cellular immune suppression in paraneoplastic neurologic syndromes targeting intracellular antigens. Arch Neurol (2012) 69(9):1132–40. doi:10.1001/archneurol.2012.595
229. Mayer L, Sandborn WJ, Stepanov Y, Geboes K, Hardi R, Yellin M, et al. Anti-IP-10 antibody (BMS-936557) for ulcerative colitis: a phase II randomized study. Gut (2014) 63(3):442–50. doi:10.1136/gutjnl-2012-303424
230. Wells TN, Power CA, Shaw JP, Proudfoot AE. Chemokine blockers – therapeutics in the making. Trends Pharmacol Sci (2006) 27(1):41–7. doi:10.1016/j.tips.2005.11.001
231. Guerreiro Castro S, Isenberg DA. Belimumab in systemic lupus erythematosus (SLE): evidence-to-date and clinical usefulness. Ther Adv Musculoskelet Dis (2017) 9(3):75–85. doi:10.1177/1759720X17690474
232. Rossi JF, Lu ZY, Jourdan M, Klein B. Interleukin-6 as a therapeutic target. Clin Cancer Res (2015) 21(6):1248–57. doi:10.1158/1078-0432.CCR-14-2291
233. Skurkovich B, Skurkovich S. Anti-interferon-gamma antibodies in the treatment of autoimmune diseases. Curr Opin Mol Ther (2003) 5(1):52–7.
234. Haringman JJ, Gerlag DM, Smeets TJ, Baeten D, van den Bosch F, Bresnihan B, et al. A randomized controlled trial with an anti-CCL2 (anti-monocyte chemotactic protein 1) monoclonal antibody in patients with rheumatoid arthritis. Arthritis Rheum (2006) 54(8):2387–92. doi:10.1002/art.21975
235. Barreras P, Mealy MA, Pardo CA. TNF-alpha inhibitor associated myelopathies: a neurological complication in patients with rheumatological disorders. J Neurol Sci (2017) 373:303–6. doi:10.1016/j.jns.2017.01.023
236. Mohan N, Edwards ET, Cupps TR, Oliverio PJ, Sandberg G, Crayton H, et al. Demyelination occurring during anti-tumor necrosis factor α therapy for inflammatory arthritides. Arthritis Rheum (2001) 44:2862–9. doi:10.1002/1529-0131(200112)44:12<2862::AID-ART474>3.0.CO;2-W
237. Vermeire S, Van Assche G, Rutgeerts P. Serum sickness, encephalitis and other complications of anti-cytokine therapy. Best Pract Res Clin Gastroenterol (2009) 23(1):101–12. doi:10.1016/j.bpg.2008.12.005
238. Weidenbusch M, Kulkarni OP, Anders HJ. The innate immune system in human systemic lupus. Clin Sci (2017) 131(8):625–64. doi:10.1042/CS20160415
239. Kalunian KC. Interferon-targeted therapy in systemic lupus erythematosus: is this an alternative to targeting B and T cells? Lupus (2016) 25(10):1097–101. doi:10.1177/0961203316652495
240. The Lenercept Multiple Sclerosis Study Group and the University of British Columbia MS/MRI Analysis Group. TNF neutralization in MS: results of a randomized, placebo-controlled multicenter study. Neurology (1999) 53:457–65. doi:10.1212/WNL.53.3.457
241. Waisman A, Hauptmann J, Regen T. The role of IL-17 in CNS diseases. Acta Neuropathol (2015) 129(5):625–37. doi:10.1007/s00401-015-1402-7
242. Lagarde S, Villeneuve N, Trébuchon A, Kaphan E, Lepine A, McGonigal A, et al. Anti-tumor necrosis factor alpha therapy (adalimumab) in Rasmussen’s encephalitis: an open pilot study. Epilepsia (2016) 57:956–66. doi:10.1111/epi.13387
243. Granata T, Fusco L, Gobbi G, Freri E, Ragona F, Broggi G, et al. Experience with immunomodulatory treatments in Rasmussen’s encephalitis. Neurology (2003) 61(12):1807–10. doi:10.1212/01.WNL.0000099074.04539.E0
244. Marino A, Narula S, Lerman M. First pediatric patient with neuromyelitis optica and Sjogren’s syndrome successfully treated with tocilizumab. Pediatr Neurol (2017) 73:e5–6. doi:10.1016/j.pediatrneurol.2017.05.015
245. Araki M, Matsuoka T, Miyamoto K, Kusunoki S, Okamoto T, Murara M, et al. Efficacy of the anti-IL-6 receptor antibody tocilizumab in neuromyelitis optica: a pilot study. Neurology (2014) 82:1302–6. doi:10.1212/WNL.0000000000000317
246. Kondo T, Fukuta M, Takemoto A, Takami Y, Sato M, Takahashi N, et al. Limbic encephalitis associated with relapsing polychondritis responded to infliximab and maintained its condition without recurrence after discontinuation: a case report and review of the literature. Nagoya J Med Sci (2014) 76:361–8.
247. Cohen Aubart F, Bouvry D, Galanaud D, Dehais D, Mathey G, Psimaras D, et al. Long-term outcomes of refractory neurosarcoidosis treated with infliximab. J Neurol (2017) 264:891–7. doi:10.1007/s00415-017-8444-9
248. Smith CJ, Emsley HC, Udeh CT, Vail A, Hoadley ME, Rothwell NJ, et al. Interleukin-1 receptor antagonist reverses stroke-associated peripheral immune suppression. Cytokine (2012) 58:384–9. doi:10.1016/j.cyto.2012.02.016
249. Lin YC, Winokur P, Blake A, Wu T, Romm E, Bielekov B. Daclizumab reverses intrathecal immune cell abnormalities in multiple sclerosis. Ann Clin Transl Neurol (2015) 2(5):445–55. doi:10.1002/acn3.181
250. Gorman MP, Tillema JM, Ciliax AM, Guttmann CR, Chitnis T. Daclizumab use in patients with pediatric multiple sclerosis. Arch Neurol (2012) 69(1):78–81. doi:10.1001/archneurol.2011.581
251. Simpson EL, Bieber T, Guttman-Yassky E, Beck LA, Blauvelt A, Cork MJ, et al. Two phase 3 trials of dupilumab versus placebo in atopic dermatitis. N Engl J Med (2016) 375(24):2335–48. doi:10.1056/NEJMoa1610020
252. Nair P, WeEenzel S, Rabe KF, Bourdin A, Lugogo NL, Kuna P, et al. Oral glucocorticoid-sparing effect of benralizumab in severe asthma. N Engl J Med (2017) 376(25):2448–58. doi:10.1056/NEJMoa1703501
253. Sheppard M, Laskou F, Stapleton PP, Hadavi S, Dasgupta B. Tocilizumab (Actemra). Hum Vaccin Immunother (2017) 13(9):1972–88. doi:10.1080/21645515.2017.1316909
254. Lee WJ, Lee ST, Moon J, Sunwoo JS, Byun JI, Lim JA, et al. Tocilizumab in autoimmune encephalitis refractory to rituximab: an institutional cohort study. Neurotherapeutics (2016) 13:824–32. doi:10.1007/s13311-016-0442-6
255. Stone JH, Tuckwell K, Dimonaco S, Klearman M, Aringer M, Blockmans D, et al. Trial of tocilizumab in giant-cell arteritis. N Engl J Med (2017) 377(4):317–28. doi:10.1056/NEJMoa1613849
256. Ringelstein M, Ayzenberg I, Harmel J, Lauenstein AS, Lensch E, Stögbauer F, et al. Long-term therapy with interleukin 6 receptor blockade in highly active neuromyelitis optica spectrum disorder. JAMA Neurol (2015) 72(7):756–63. doi:10.1001/jamaneurol.2015.0533
257. Takeuchi T, Tanaka Y, Yamanaka H, Amano K, Nagamine R, Park W, et al. Efficacy and safety of olokizumab in Asian patients with moderate-to-severe rheumatoid arthritis, previously exposed to anti-TNF therapy: results from a randomized phase II trial. Mod Rheumatol (2016) 26(1):15–23. doi:10.3109/14397595.2015.1074648
258. Puig L. Brodalumab: the first anti-IL-17 receptor agent for psoriasis. Drugs Today (Barc) (2017) 53(5):283–97. doi:10.1358/dot.2017.53.5.2613690
259. Jinesh S. Pharmaceutical aspects of anti-inflammatory TNF-blocking drugs. Inflammopharmacology (2015) 23(2–3):71–7. doi:10.1007/s10787-015-0229-0
260. Burmester GR, McInnes IB, Kremer JM, Miranda P, Vencovský J, Godwood A, et al. Mavrilimumab, a fully human granulocyte-macrophage colony-stimulating factor receptors α monoclonal antibody: long-term safety and efficacy in patients with rheumatoid arthritis. Arthritis Rheumatol (2018). doi:10.1002/art.40420
261. Gladue RP, Brown MF, Zwillich SH. CCR1 antagonists: what have we learned from clinical trials. Curr Top Med Chem (2010) 10(13):1268–77. doi:10.2174/156802610791561237
262. Ni X, Langridge T, Duvic M. Depletion of regulatory T cells by targeting CC chemokines receptor type 4 with mogamulizumab. Oncoimmunology (2015) 4(7):e1011524. doi:10.1080/2162402X.2015.1011524
263. Jacobson JM, Lalezari JP, Thompson MA, Fichtenbaum CJ, Saag MS, Zingman BS, et al. Phase 2a study of the CCR5 monoclonal antibody PRO 140 administered intravenously to HIV-infected adults. Antimicrob Agents Chemother (2010) 54(10):4137–42. doi:10.1128/AAC.00086-10
264. Martin-Blondel G, Brassat D, Bauer J, Lassmann H, Liblau RS. CCR5 blockade for neuroinflammatory diseases – beyond control of HIV. Nat Rev Neurol (2016) 12(2):95–105. doi:10.1038/nrneurol.2015.248
265. Feagan BG, Sandborn WJ, D’Haens G, Lee SD, Allez M, Fedorak RN, et al. Randomized clinical trial: vercirnon, an oral CCR9 antagonist, vs. placebo as induction therapy in active Crohn’s disease. Aliment Pharmacol Ther (2015) 42(10):1170–81. doi:10.1111/apt.13398
266. Jantunen E, Varmavuo V, Valtola J. Plexifor injection: a hematopoietic stem cell mobilizer in non-Hodgkin lymphoma and multiple myeloma. Expert Rev Hematol (2016) 9(8):723–32. doi:10.1080/17474086.2016.1208082
267. Proudfoot AEI, Uguccioni M. Modulation of chemokine responses: synergy and cooperativity. Front Immunol (2016) 7:183. doi:10.3389/fimmu.2016.00183
268. Solari R, Pease JE. Targeting chemokine receptors in disease – a case study of CCR4. Eur J Pharmacol (2015) 763:169–77. doi:10.1016/j.ejphar.2015.05.018
269. Fox K, Wells ME, Tennison M, Vaugh B. Febrile infection-related epilepsy syndrome (FIRES): a literature review and case study. Neurodiagn J (2017) 57(3):224–33. doi:10.1080/21646821.2017.1355181
270. Aksentijevich I, Masters SL, Ferguson PJ, Dancey P, Frenkel J, van Royen-Kerkhoff A, et al. An autoinflammatory disease with deficiency of the interleukin-1-receptor antagonist. N Engl J Med (2009) 360:2426–37. doi:10.1056/NEJMoa0807865
271. Catlet MC, Coote J, Bari M, Tomlinson KL. Monoclonal antibodies for the treatment of asthma. Pharmacol Ther (2011) 231(3):333–51. doi:10.1016/j.pharmthera.2011.09.005
272. Cuesta-Mateos C, Loscertales J, Kreutzman A, Colom-Fernández B, Portero-Sáinz I, Pérez-Villar JJ, et al. Preclinical activity of anti-CCR7 immunotherapy in patients with high-risk chronic lymphocytic leukemia. Cancer Immunol Immunother (2015) 64(6):665–76. doi:10.1007/s00262-015-1670-z
273. Liston A, Kohler RE, Townley S, Haylock-Jacobs S, Comerford I, Caon AC, et al. Inhibition of CCR6 function reduces the severity of experimental autoimmune encephalomyelitis via effects on the priming phase of the immune response. J Immunol (2009) 182:3121–30. doi:10.4049/jimmunol.0713169
274. Klimatcheva E, Pandina T, Reilly C, Torno S, Bussler H, Scrivens M, et al. CXCL13 antibody for the treatment of autoimmune disorders. BMC Immunol (2015) 16:6. doi:10.1186/s12865-015-0068-1
275. He S, Cao Q, Qiu Y, Mi J, Zhang JZ, Jin M, et al. A new approach to the blocking of alloreactive T cell-mediated graft-vs-host disease by in vivo administration of anti-CXCR3 neutralizing antibody. J Immunol (2009) 181(11):7581–92. doi:10.4049/jimmunol.181.11.7581
276. Panjideh H, Müller G, Koch M, Wilde F, Scheu S, Moldenhauer G, et al. Immunotherapy of B-cell non-Hodgkin lymphoma by targeting the chemokine receptor CXCR5 in a preclinical mouse model. Int J Cancer (2014) 135(11):2623–32. doi:10.1002/ijc.28893
277. Wu KJ, Yu SJ, Shia KS, Wu CH, Song JS, Kuan HH, et al. A novel CXCR4 antagonist CX549 induces neuroprotection in stroke brain. Cell Transplant (2017) 26(4):571–83. doi:10.3727/096368916X693563
278. Griffiths K, Dolezal O, Cao B, Nilsson SK, See HB, Pfleger KD, et al. I-bodies, human single domain antibodies that antagonize chemokine receptor CXCR4. J Biol Chem (2016) 291(24):12641–57. doi:10.1074/jbc.M116.721050
279. Walenkamp AME, Lapa C, Hermann K, Wesyter HJ. CXCR4 ligands: the next big hit? J Nucl Med (2017) 58(Suppl 2):77S–82S. doi:10.2967/jnumed.116.186874
280. Miralles M, Eixarch H, Tejero M, Costa C, Hirota K, Castaño AR, et al. Clinical and histopathological encephalomyelitis by AAV vectors expressing a soluble interleukin-23 receptor. Neurotherapeutics (2017). doi:10.1007/s13311-017-0545-8
281. Rafei M, Campeau PM, Wu JH, Birman E, Forner K, Boivin MN, et al. Selective inhibition of CCR2 expressing lymphomyeloid cells in experimental autoimmune encephalomyelitis by a GMCSF-MCP1 fusokine. J Immunol (2009) 5:2620–7. doi:10.4049/jimmunol.0803495
282. Fischer R, Maier O, Siegmund M, Wajant H, Scheurich P, Pfizenmaier K. A TNF receptor 2 selective agonist rescues human neurons from oxidative stress-induced cell death. PLoS One (2011) 6(11):e27621. doi:10.1371/journal.pone.0027621
283. Torrey H, Butterworth J, Mera T, Okubo Y, Wang L, Baum D, et al. Targeting TNFR2 with antagonist antibodies inhibits proliferation of ovarian cancer cells and tumor-associated Tregs. Sci Signal (2017) 10(462):eaaf8608. doi:10.1126/scisignal.aaf8608
284. Blank T, Prinz M. Type I interferon pathway in CNS homeostasis and neurological disorders. Glia (2017) 65(9):1397–406. doi:10.1002/glia.23154
285. Wells M, Sever L, Schadt K, Lynch DR. IFN-γ for Friedreich ataxia: present evidence. Neurodegener Dis Manag (2015) 5(6):497–504. doi:10.2217/nmt.15.52
286. Panitch HS, Hirsch RL, Schindler J, Johnson KP. Treatment of multiple sclerosis with γ interferon: exacerbations associated with activation of the immune system. Neurology (1987) 37:1097–1092. doi:10.1212/WNL.37.7.1097
287. Lim JA, Lee ST, Moon J, Jun JS, Park BS, Byun JI, et al. New feasible treatment for refractory autoimmune encephalitis low-dose interleukin-2. J Neuroimmunol (2016) 299:107–11. doi:10.1016/j.jneuroim.2016.09.001
288. Kemp KC, Cerminara N, Hares K, Redondo J, Cook AJ, Haynes HR, et al. Cytokine therapy-mediated neuroprotection in a Friedreich’s ataxia mouse model. Ann Neurol (2017) 81:212–26. doi:10.1002/ana.24846
289. Galluzzi L, Vacchelli E, Bravo-San Pedro J-M, Buque A, Senovilla L, Baracco EE, et al. Classification of current anticancer immunotherapies. Oncotarget (2014) 5:12472–508. doi:10.18632/oncotarget.2998
290. Opdenakker G, van Damme J. Probing cytokines, chemokines and matrix metalloproteinases towards better immunotherapies of multiple sclerosis. Cytokine Growth Factor Rev (2011) 22:359–65. doi:10.1016/j.cytogfr.2011.11.005
291. Strazza M, Mor A. Consider the chemokines: a review of the interplay between chemokines and T cell subset function. Discov Med (2017) 24(130):31–9.
292. Bielekova B, Martin R. Development of biomarkers in multiple sclerosis. Brain (2004) 127(Pr 7):1463–78. doi:10.1093/brain/awh176
293. Garbers C, Aparicio-Siegmund S, Rose-John S. The IL-6/gp130/STAT3 signaling axis: recent advances towards specific inhibition. Curr Opin Immunol (2015) 34:75–82. doi:10.1016/j.coi.2015.02.008
294. Huber AK, Irani DN. Targeting CXCL13 during neuroinflammation. Adv Neuroimmune Biol (2015) 6(1):1–8. doi:10.3233/NIB-150101
295. Chung CY, Liao F. CXCR3 signaling in glial cells ameliorates experimental encephalomyelitis by restraining the generation of a pro-Th17 cytokine milieu and reducing CNS-infiltrating Th17 cells. J Neuroinflammation (2016) 13(1):76. doi:10.1186/s12974-016-0536-4
296. Mahad DJ, Ransohoff RM. The role of MCP-1 (CCL2) and CCR2 in multiple sclerosis and experimental encephalomyelitis (EAE). Semin Immunol (2003) 15(1):23–32. doi:10.1016/S1044-5323(02)00125-2
297. Welch MJ, Lally R, Miller JE, Pittman S, Brodsky L, Caplan AL, et al. The ethics and regulatory landscape of including vulnerable populations in pragmatic clinical trials. Clin Trials (2015) 12(5):503–10. doi:10.1177/1740774515597701
298. Carpenter D, Gonzalez D, Retsch-Bogart G, Sleath B, Wilfond B. Methodological and ethical issues in pediatric medication safety research. Pediatrics (2017) 149(3):e20170195. doi:10.1542/peds.2017-0195
299. Sagoo P, Garcia Z, Breart B, Lemaître F, Michonneau D, Albert ML, et al. In vivo imaging of inflammasome activation reveals a subcapsular macrophage burst response that mobilizes innate and adaptive immunity. Nat Med (2016) 22(1):64–71. doi:10.1038/nm.4016
300. Liu Z, Wyffels L, Barber C, Wan L, Xu H, Hui MM, et al. Characterization of 99mTc-labeled cytokine ligands for inflammation imaging via TNF and IL-1 pathways. Nucl Med Biol (2012) 39(7):9050915. doi:10.1016/j.nucmedbio.2012.05.003
301. Albrecht DS, Granziera C, Hooker JM, Loggia ML. In vivo imaging of human neuroinflammation. ACS Chem Neurosci (2016) 7(4):470–83. doi:10.1021/acschemneuro.6b00056
302. Mandrekar SJ, An MW, Sargent DJ. A review of phase II trial designs for initial marker validation. Contemp Clin Trials (2013) 36(2):597–604. doi:10.1016/j.cct.2013.05.001
303. Masgrau R, Guaza C, Ransohoff RM, Galea E. Should we stop saying “glia” and “neuroinflammation”? Trends Mol Med (2017). doi:10.1016/j.molmed.2017.04.005
Keywords: acute disseminated encephalomyelitis, multiple sclerosis, neuromyelitis optica, neuropsychiatric lupus, N-methyl-d-aspartate receptor encephalitis, opsoclonus–myoclonus syndrome, pediatric neuroinflammatory disorders, Rasmussen encephalitis
Citation: Pranzatelli MR (2018) Advances in Biomarker-Guided Therapy for Pediatric- and Adult-Onset Neuroinflammatory Disorders: Targeting Chemokines/Cytokines. Front. Immunol. 9:557. doi: 10.3389/fimmu.2018.00557
Received: 09 November 2017; Accepted: 05 March 2018;
Published: 04 April 2018
Edited by:
Fabienne Brilot, University of Sydney, AustraliaReviewed by:
Kavitha Kothur, University of Sydney, AustraliaRobert Adam Harris, Karolinska Institutet (KI), Sweden
Copyright: © 2018 Pranzatelli. This is an open-access article distributed under the terms of the Creative Commons Attribution License (CC BY). The use, distribution or reproduction in other forums is permitted, provided the original author(s) and the copyright owner are credited and that the original publication in this journal is cited, in accordance with accepted academic practice. No use, distribution or reproduction is permitted which does not comply with these terms.
*Correspondence: Michael R. Pranzatelli, bXByYW56YXRlbGxpQG9tc3VzYS5vcmc=