- 1Sanquin Research, Department of Immunopathology, Amsterdam, Netherlands
- 2Landsteiner Laboratory, Academic Medical Centre, University of Amsterdam, Amsterdam, Netherlands
- 3Academic Medical Centre, Department of Medical Microbiology, University of Amsterdam, Amsterdam, Netherlands
- 4Department of Microbiology and Immunology, Weill Medical College of Cornell University, New York, NY, United States
Immunoglobulin G (IgG) can contain N-linked glycans in the variable domains, the so-called Fab glycans, in addition to the Fc glycans in the CH2 domains. These Fab glycans are acquired following introduction of N-glycosylation sites during somatic hypermutation and contribute to antibody diversification. We investigated whether Fab glycans may—in addition to affecting antigen binding—contribute to antibody stability. By analyzing thermal unfolding profiles of antibodies with or without Fab glycans, we demonstrate that introduction of Fab glycans can improve antibody stability. Strikingly, removal of Fab glycans naturally acquired during antigen-specific immune responses can deteriorate antibody stability, suggesting in vivo selection of stable, glycosylated antibodies. Collectively, our data show that variable domain N-linked glycans acquired during somatic hypermutation can contribute to IgG antibody stability. These findings indicate that introducing Fab glycans may represent a mechanism to improve therapeutic/diagnostic antibody stability.
Introduction
Immunoglobulin G (IgG) is the most abundant class of immunoglobulins in human serum. IgGs can contain N-linked glycans in the variable domains, the so-called Fab glycans (Figure 1), in addition to the Fc glycans in the CH2 domains. Fab glycans are present in about 15% of circulating IgGs (1). These Fab glycans are acquired following introduction of N-glycosylation sites during somatic hypermutation and thereby constitute part of the physiological repertoire of antibodies (2). We previously showed that these N-glycosylation sites are acquired predominantly at positions in the variable domains where a single nucleotide mutation turns a “latent” or “progenitor” glycosylation motif into an actual N-glycosylation motif. We furthermore showed that Fab glycans are usually localized close to antigen-binding sites and can influence antigen binding and contribute to affinity maturation (3). However, we also observed only minimal or no effects in quite a few cases, suggesting other functions of Fab glycans may exist.
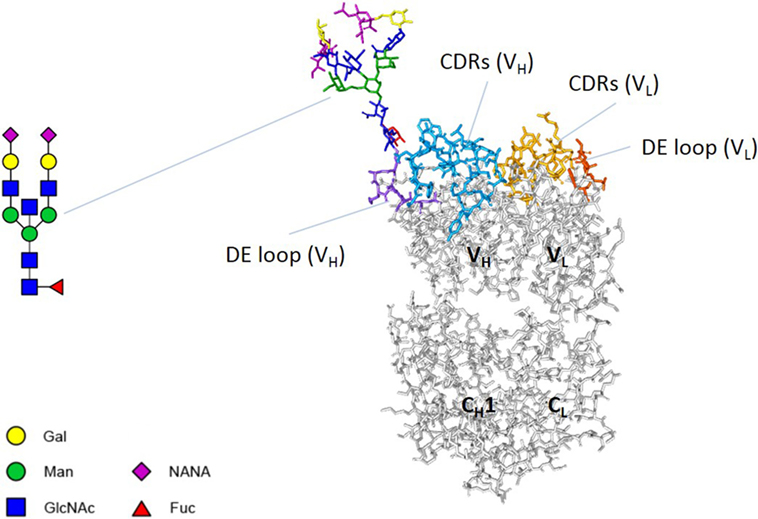
Figure 1. Fab glycosylation. An antibody Fab arm consists of two variable domains (VH and VL) and two constant domains (CH1 and CL). Both variable domains contain three complementarity determining regions (CDRs) and a DE loop. The Fab arm of adalimumab NH82 contains a glycan at position 82 of the heavy chain. A biantennary glycan was modeled using the online tool Sweet (http://www.glycosciences.de/modeling/sweet2/doc/index.php) (4). Subsequently, using Discovery Studio Visualizer v16.1 and UCSF Chimera v1.11.2, the glycan was introduced into the crystal structure of adalimumab Fab (PDB 3WD5), attached to N82 of the heavy chain. The side chain of N82 was reoriented to allow a structurally/chemically plausible linkage with the glycan. The resulting structure was not further optimized. Abbreviations: Gal, galactose; Man, mannose; GlcNAc, N-acetylglucosamine; NANA, N-acetylneuraminic acid; Fuc, fucose.
It has been suggested that glycans in the variable domains enhance antibody aggregation (5–7). On the other hand, recent literature indicates that Fab glycans may contribute to antibody solubility and stability and may prevent aggregation (8–10). For example, Courtois and colleagues showed that introduction of glycans specifically introduced to mask aggregation-prone regions improves antibody stability to a similar extent as removing those regions by replacement of hydrophobic amino acid residues with hydrophilic ones.
We wondered whether this contribution of Fab glycans to antibody stability might also apply to naturally acquired Fab glycans in circulating IgGs. Specifically, mutations acquired during somatic hypermutation that improve antigen binding can have destabilizing effects, and additional mutations are introduced to compensate for that (11). Perhaps, acquiring Fab glycans represents a possible mechanism to compensate for those destabilizing mutations. Therefore, we hypothesized that, in addition to their effects on antigen binding, another role of Fab glycans is to improve antibody stability, possibly by shielding hydrophobic residues in the antigen-binding site.
In this study, we demonstrate by analyzing thermal unfolding profiles that introduction of Fab glycans can improve antibody stability. Strikingly, removal of naturally acquired Fab glycans can deteriorate antibody stability, suggesting in vivo selection of stable, glycosylated antibodies. Collectively, our data show that Fab glycans can contribute to IgG antibody stability.
Materials and Methods
Therapeutic Antibodies
Recombinant therapeutic antibodies used in this study are adalimumab (Humira, Abbvie), cetuximab (Erbitux, Merck), and omalizumab (Xolair, Novartis). Adalimumab is a human monoclonal anti-TNFα IgG1κ antibody, cetuximab is a chimeric monoclonal anti-EGFR IgG1κ antibody, and omalizumab is a humanized monoclonal anti-IgE IgG1κ antibody. Intravenous immunoglobulin (IVIg) was obtained from Sanquin (Nanogam, Amsterdam, The Netherlands) and contains IgGs from thousands of donors.
Fab Glycovariants
The chimeric monoclonal anti-TNP IgG1κ antibody was produced as described previously (12). The nucleotide sequence of this clone was analyzed for positions where a single nucleotide mutation would suffice to introduce a glycosylation site (hereafter referred to as “progenitor glycosylation site”). A Fab glycovariant with such a glycosylation site effectuated (in the variable domain of the heavy chain) was designed, and a construct coding for the respective variable domain was ordered (Integrated DNA Technologies). The asparagine residue to which a glycan can be attached is located in CDR1 (NH29). The human monoclonal anti-TNFα IgG1κ antibody (adalimumab) and adalimumab Fab glycovariants were designed and produced as described previously (3). Briefly, the nucleotide sequence of adalimumab was analyzed for progenitor glycosylation sites. Fab glycovariants with such glycosylation sites effectuated were designed, and constructs coding for the respective variable domains were ordered (Integrated DNA Technologies), 4 with a glycosylation site in the variable domain of the heavy chain and 3 with a glycosylation site in the variable domain of the light chain. The asparagine residues to which glycans can be attached are located in CDR1 (NL37), CDR2 (NH59), and FR3 (NH77, NH82, NH84, NL79, and NL86). The human monoclonal patient-derived anti-adalimumab and anti-infliximab antibodies and mutants were obtained, designed, and produced as described previously (3, 13, 14). Briefly, single antigen-specific memory B cells were isolated from patients producing antibodies against adalimumab or infliximab and cultured and screened for specificity. Variable domains were sequenced and analyzed for the presence of glycosylation sites. The asparagine residues to which glycans can be attached are located in CDR1 (anti-infliximab 1.3, NH29), FR3 (anti-adalimumab 2.2, NL79; anti-adalimumab 2.6, NH77; anti-infliximab 2.1, NH66), and CDR3 (anti-infliximab 1.4, NL110). Constructs coding for anti-adalimumab and anti-infliximab antibodies with naturally acquired glycosylation sites removed (mutated back to germline) were ordered (Integrated DNA Technologies).
Ordered VH and VL sequences in pANY vectors were transformed into DH5α competent cells to amplify DNA. DNA was extracted according to manufacturer’s instructions (NucleoSpin Plasmid EasyPure) and digested out of the pANY vector and ligated/cloned into the pcDNA3.1 expression vector together with the constant domains of the human IgG1 (CH) or kappa (CL) genes. Next, these sequences were transformed into DH5α competent cells to amplify DNA, and DNA was extracted according to manufacturer’s instructions (NucleoSpin Plasmid EasyPure). Antibodies were expressed by transient cotransfection of heavy and light chain containing vectors into HEK293F cells with either fectin or PEI and Opti-MEM, using the FreeStyle HEK293F expression system according to the instructions supplied by the manufacturer. This expression system reproducibly yields (Fc) glycosylation patterns that closely resemble those found on human serum IgG (15). Cells were incubated for 5 days at 37°C in humidified 8% CO2 on a shaker at 125 rpm. Antibodies were purified from culture supernatants at day 5 after transfection using either protein A or protein G Sepharose. Culture supernatants were centrifuged, filtered (0.2 µm), and purified using a HiTrap protein A/G column (GE Healthcare) equilibrated with PBS. IgG was eluted with 0.1 M glycine pH 2.5–3. The fractions were immediately neutralized using 2 M Tris pH 9 and then dialyzed against PBS overnight at 4°C and stored at 4°C.
Gel Electrophoresis
Samples were analyzed by SDS-PAGE by loading 5 µg IgG on precast 4–12% Bis–Tris gels (NuPAGE), visualized with Coomassie Blue. To examine heavy and light chains separately, samples were reduced with DTT. Figure S1 in Supplementary Material shows original images of gels.
Lectin ELISAs
Lectin ELISAs were performed as described previously (3). In brief, samples were coated on plates and detected with biotinylated lectin [wheat germ agglutinin (WGA, Vector Laboratories), Ricinus communis agglutinin (RCA, Vector Laboratories), or Sambucus nigra agglutinin (SNA, Vector Laboratories)], streptavidin labeled with horseradish peroxidase, and tetramethylbenzidine. The reaction was stopped with 2 M H2SO4, and the absorbance was measured at 450 and 540 nm using a BioTek microtiter plate reader. We previously confirmed that SNA does not bind Fc glycans (3).
Neuraminidase Treatment
Neuraminidase treatment was performed as described previously (16, 17). Adalimumab NH82 and anti-infliximab 1.4 NL110 were dialyzed against a 50 mM sodium citrate buffer (pH 6) and incubated for 48 h at 37°C with about 20 U/mL of neuraminidase (20 U/mg IgG) (New England BioLabs). After neuraminidase treatment, samples were dialyzed against PBS and stored at 4°C.
SNA Lectin Affinity Chromatography
Three different batches of IVIg were fractionated using SNA lectin affinity chromatography as described previously (3) into fractions highly enriched for Fab glycosylation (SA+) or depleted for Fab glycosylation (SA−). As demonstrated by many studies, SNA binds virtually exclusively to sialic acid residues in Fab glycans due to the relative inaccessibility of Fc glycans in natively folded IgG (18–20). More than 90% of Fab glycans carry terminal sialic acid residues (21).
Measurement of Antibody Stability
Thermofluor assay analysis was performed using 8-anilino-1-naphthalenesulfonate (ANS) ammonium salt (Fluka), a fluorescent probe that binds to hydrophobic pockets during antibody unfolding (22, 23). This assay was performed analogously to previously described methods (24, 25). Samples of antibodies in PBS containing 60 µM ANS were heated from 45 to 95°C at 1°C/min using a StepOnePlus thermocycler (Thermo Fisher), and fluorescence was measured (excitation by blue diode, emission in channel 1, corresponding to ca. 505–535 nm). Initial runs with adalimumab, cetuximab, and omalizumab at concentrations between 0.1 and 10 mg/mL were carried out, and 0.5 mg/mL was chosen as optimal concentration showing clear transitions with fluorescence signals going through a maximum at the expected transition temperatures (Tm); except for anti-adalimumab 2.2, anti-adalimumab 2.6, and anti-infliximab 1.3, where a concentration of 0.5–0.72, 2, and 1 mg/mL was used, respectively. Raw thermograms were smoothed by converting to a 5-point running average, and buffer control runs only containing ANS were subtracted from the data (Figure S2 in Supplementary Material). Reported Tms are (local) maxima in the fluorescence vs temperature plots, as indicated in Figures S2C, 3B,D, and 4C Supplementary Material.
Differential Scanning Calorimetry (DSC)
Thermal denaturation was studied using a Nano DSC Calorimeter (TA Instruments) as described previously (26). Samples of the following four antibodies in PBS were diluted to 0.33 mg/mL: adalimumab WT, adalimumab NH82, anti-infliximab 1.4 WT, and anti-infliximab 1.4 N110S. After loading the sample into the cell, thermal denaturation was probed from 25 to 90°C at a scan rate of 1°C/min. Buffer correction, normalization, and baseline subtraction procedures were applied, and the data were analyzed using NanoAnalyze Software v.3.7.5 (TA Instruments). The data were fitted using a non-two-state model with two (adalimumab WT, adalimumab NH82, anti-infliximab 1.4 WT, and anti-infliximab 1.4 N110S) or three (anti-infliximab 1.4 N110S) peaks and obtained values of Tm were reported. Reported Tms are maxima of the 2/3 peaks in the kJ/mol K vs temperature plots. Indicated in Figures 3F and 4H are maxima of the main peak. All samples were measured in two independent DSC runs.
Statistical Analysis
In all box plots, medians of 3–10 replicates with IQR are shown. To compare two samples, unpaired t-tests were done, and to compare multiple samples, one-way ANOVAs compared with WT/total were performed (**P < 0.01, ***P < 0.001, and ****P < 0.0001).
Results
Thermostability of an Anti-TNP Clone Carrying Fab Glycans
To evaluate the potential stabilizing effects of variable domain glycans, we first analyzed a chimeric anti-TNP antibody produced in our lab (human IgG1κ; mouse variable domains), and a variant carrying Fab glycans due to introduction of an N-linked glycosylation motif. The glycan was introduced at a position designated to be a “progenitor” glycosylation site, meaning that in the context of an antibody immune response, a single nucleotide mutation during somatic hypermutation would suffice to introduce an N-linked glycan at this position (3). We confirmed that the anti-TNP variant indeed carried Fab glycans (Figures 2A,B). We noticed that the parent antibody, but not the variant with Fab glycans, has a slight tendency to precipitate. To investigate the thermostability of these clones, we determined thermal unfolding profiles using thermofluor assay analysis with ANS, a fluorescent probe that binds to hydrophobic pockets during protein unfolding (see Materials and Methods). We confirmed that the transition temperatures (Tm) of three therapeutic antibodies (adalimumab, cetuximab, and omalizumab) obtained by this analysis with ANS are in the range of previously published Tms [Figure S3 and Table S1 in Supplementary Material (25, 27, 28)]. Interestingly, we observed that the anti-TNP variant with Fab glycans had a higher Tm compared with the antibody without Fab glycans (Figures 2C,D; Table S2 in Supplementary Material), indicating that the variable domain glycans of this clone indeed had a stabilizing effect.
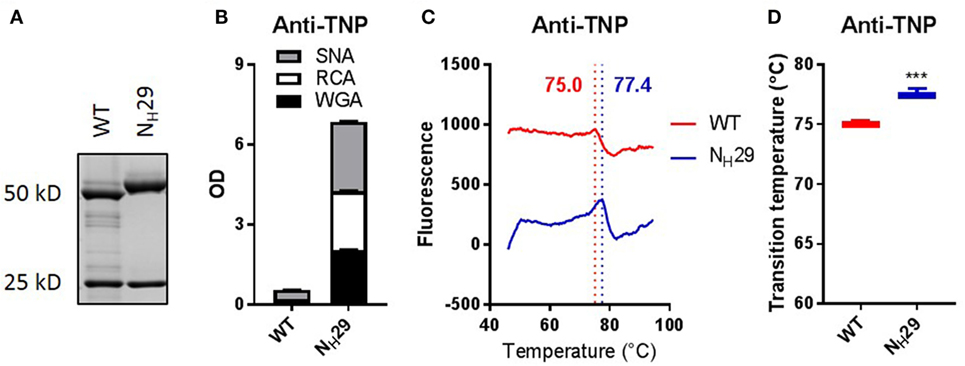
Figure 2. Introducing Fab glycans improves anti-TNP stability. (A) Gel electrophoresis of anti-TNP wild-type (WT) and mutant (NH29). (B) Lectin ELISA data for anti-TNP WT and NH29. WGA recognizes N-acetylglucosamine, RCA recognizes galactose, and SNA recognizes sialic acid. (C) Thermal unfolding profiles of anti-TNP WT (red) and NH29 (blue) were determined as described in Figure S3 in Supplementary Material. Thermal unfolding profiles were shifted up or down for clarity. Values in graphs represent obtained Tm (local maxima). Shown are representative data of three replicates. (D) Tm of anti-TNP variants, determined as described in Figure S3 in Supplementary Material. Shown are medians of three replicates with IQR. Unpaired t-test compared with WT, ***P < 0.001.
Introduction of Fab Glycans at Predisposed Locations Can Improve Antibody Stability
To investigate the effects of Fab glycans on antibody stability in more detail, we next compared the thermostability of adalimumab, a human anti-TNF antibody, with a panel of variants in which we systematically introduced Fab glycosylation sites at several positions across the variable domains corresponding to “progenitor” glycosylation sites as explained earlier. We generated four variants with glycans in the variable domain of the heavy chain (NH59, NH77, NH82, and NH84) and three variants with glycans in the variable domain of the light chain (NL37, NL79, and NL86). We previously confirmed that the adalimumab variants indeed carried Fab glycans (3). Figure 1 shows the predicted structure of adalimumab NH82. Some introduced glycans were localized close to more hydrophilic residues (NH59, NH82, NH84, and NL86), while some glycans were localized close to more hydrophobic residues (NH77, NL37, and NL79) (Figure 3A). We found that several Fab glycovariants had a higher Tm compared with adalimumab without Fab glycans (NH59, NH82, and NH84, Figures 3B,C; Table S2 in Supplementary Material), which indicates that the Fab glycans introduced in these variants improved antibody stability. For adalimumab variant NH82, the stabilizing effects of the Fab glycans were confirmed using DSC (Figure 3F; Table S4 in Supplementary Material). For the other variants, similar thermostabilities were observed with and without Fab glycans. Furthermore, the thermal unfolding profiles of multiple variants, including adalimumab NL37, where similar Tms were observed, did show a broader transition in comparison with adalimumab (Figures 3D,E; Table S2 in Supplementary Material). Interestingly, we did not see this broader peak for all clones with Fab glycans (Table S2 in Supplementary Material). There was no clear correlation between the position of the glycan and the impact on thermostability in terms of proximity to hydrophobic pockets (Figure 3). Our data indicate that sialic acid is not required for the improved stability, since removal of sialic acid by treatment with neuraminidase (Figure S4A in Supplementary Material) did not affect the Tm for adalimumab NH82 (Figures 3B,C; Table S2 in Supplementary Material).
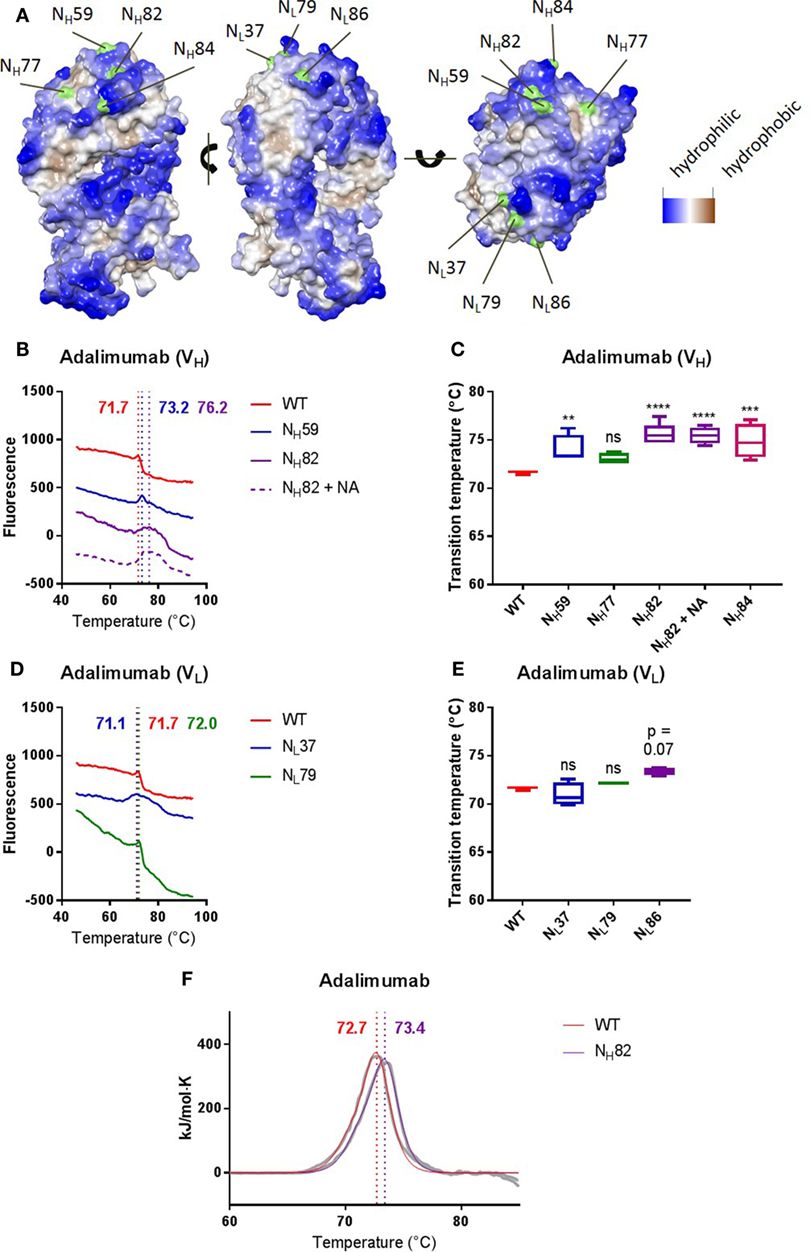
Figure 3. Introducing Fab glycans can improve adalimumab stability. (A) The Fab arm of adalimumab with introduced glycosylation sites indicated in green. Sites were introduced in the heavy (left) or light (middle) chain. The more blue, the more hydrophilic, and the more brown, the more hydrophobic. (B) Thermal unfolding profiles of adalimumab wild-type (WT, red), VH mutants (NH59, blue; NH82, purple), and NH82 treated with neuraminidase (NH82 + NA, dotted purple) were determined as described in Figure S3 in Supplementary Material. Thermal unfolding profiles were shifted up or down for clarity. Values in graphs represent obtained Tm (local maxima). Shown are representative data of at least four replicates. (C) Tm of adalimumab VH variants, determined as described in Figure S3 in Supplementary Material. Shown are medians of at least four replicates with IQR. One-way ANOVA compared with WT, **P < 0.01, ***P < 0.001, and ****P < 0.0001. (D) Thermal unfolding profiles of adalimumab WT (red) and VL mutants (NL37, blue; NL79, green) were determined as described in Figure S3 in Supplementary Material. Thermal unfolding profiles were shifted up or down for clarity. Values in graphs represent obtained Tm (local maxima). Shown are representative data of at least four replicates. (E) Tm of adalimumab VL variants, determined as described in Figure S3 in Supplementary Material. Shown are medians of at least four replicates with IQR. One-way ANOVA compared with WT. (F) Thermal unfolding profiles of adalimumab WT (red) and NH82 (purple) were determined using differential scanning calorimetry. The data are shown in gray and the fits in red and purple. The data were fitted using a non-two-state model with two peaks, and values in graphs represent obtained Tm (maxima) of the main peak. Shown are representative data of two replicates.
Removal of Naturally Acquired Fab Glycans Can Deteriorate Antibody Stability
To investigate whether Fab glycans naturally acquired during an immune response would also be able to positively contribute to antibody stability, we next compared the thermostability of IVIg enriched for Fab glycans (SA+, ca. 10%) with that of IVIg depleted for Fab glycans (SA−, ca. 90%) by SNA lectin affinity chromatography. We confirmed the enrichment and depletion of IVIg for Fab glycans by gel electrophoresis and lectin ELISAs (Figures 4A,B). Interestingly, the (average) Tm of SA+ IVIg (75.6 ± 0.570) was higher than that of SA− IVIg (71.0 ± 0.778), which was similar to that of total IVIg (71.1 ± 0.399) (Figures 4C,D). Relatively broad transitions and high SEMs were observed, which could be explained by the fact that IVIg is a polyclonal antibody preparation. We therefore also investigated the effects of naturally acquired Fab glycans in a number of monoclonal antibodies, with Fab glycans that were acquired via natural selection during somatic hypermutation. To this end, we analyzed antigen-specific clones isolated from patients that are treated with adalimumab or infliximab and make antibodies to these biologicals, of which a high proportion carries Fab glycans (3). We compared the thermostability of anti-adalimumab and anti-infliximab antibodies with naturally acquired Fab glycans at different positions [Figure 4E (13, 14)] with mutants in which we removed their Fab glycosylation sites. We previously confirmed that the anti-adalimumab and anti-infliximab mutants indeed did not carry Fab glycans (3). Three out of five tested clones showed a lower Tm for the mutants without Fab glycans compared with the antibodies with Fab glycans (Figures 4F,G; Table S3 in Supplementary Material), indicating that the naturally acquired Fab glycans in these clones improved antibody stability. For anti-infliximab 1.4, the stabilizing effects of the Fab glycans were confirmed using DSC (Figure 4H; Table S4 in Supplementary Material). Again, sialic acid is not required for this improved stability of anti-infliximab 1.4 (Figures S4B,G and Table S3 in Supplementary Material).
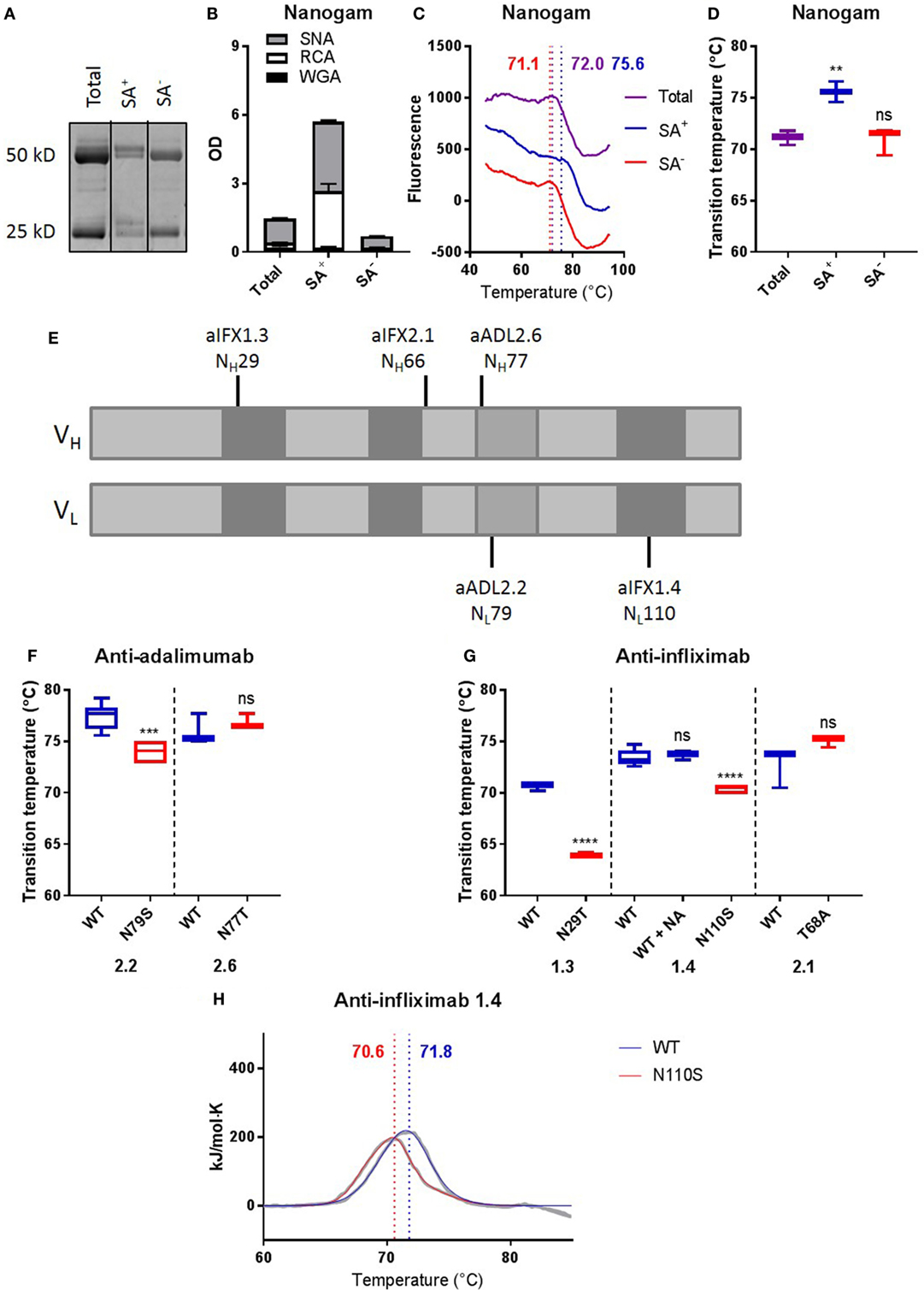
Figure 4. Removing Fab glycans can deteriorate antibody stability. (A) Gel electrophoresis of nanogam total, sialic acid enriched (SA+), and sialic acid depleted (SA−). (B) Lectin ELISA data for Nanogam total, SA+, and SA−. WGA recognizes N-acetylglucosamine, RCA recognizes galactose, and SNA recognizes sialic acid. (C) Thermal unfolding profiles of Nanogam total (purple), SA+ (blue), and SA− (red) were determined as described in Figure S3 in Supplementary Material. Thermal unfolding profiles were shifted up or down for clarity. Values in graphs represent obtained Tm (local maxima). Shown are representative data of 10 replicates. (D) Tm of Nanogam, determined as described in Figure S3 in Supplementary Material. Shown are medians of three batches with three to four replicates for each batch with IQR. One-way ANOVA compared with total, ****P < 0.0001. (E) Positions of glycosylation sites in sequences of two anti-adalimumab and three anti-infliximab clones. Light gray represents framework regions, dark gray represents complementarity determining regions, and middle gray represents DE loop. (F) Tm of anti-adalimumab variants, determined as described in Figure S3 in Supplementary Material. Shown are medians of at least three replicates with IQR. Unpaired t-test compared with wild-type (WT), ***P < 0.001. (G) Tms of anti-infliximab variants were determined as described in Figure S3 in Supplementary Material. Shown are medians of at least three replicates with IQR. One-way ANOVA or unpaired t-test compared with WT, ****P < 0.0001. (H) Thermal unfolding profiles of anti-infliximab 1.4 WT (blue) and mutant (N110S, red) were determined using differential scanning calorimetry. The data are shown in gray and the fits in blue and red. The data were fitted using a non-two-state model with two (WT) or three (N110S) peaks, and values in graphs represent obtained Tm (maxima) of the main peak. Shown are representative data of two replicates.
Discussion
This study is the first to systematically demonstrate that Fab glycans acquired during antigen-specific immune responses may contribute to antibody stability. Our data suggest that improved B-cell receptor (BCR) stability through Fab glycans may contribute to selection of antibodies with Fab glycans in vivo. Whether and how stabilizing Fab glycans are indeed selected in vivo requires more thorough investigation. It might be possible that Fab glycans affect intracellular stability of BCRs and if a B cell carries a BCR with stabilizing Fab glycans, this B cell might be positively selected. In line with this, affinity-enhancing mutations can sometimes destabilize, which may be counteracted by stability-enhancing mutations (11). The fact that Fab glycans are not present already in naive immunoglobulins but can be introduced at various positions during somatic hypermutation makes this flexible mechanism, subject to the selection mechanisms that ultimately result in the best antigen binders. The alternative, Fab glycans at fixed permanent positions, would not allow a co-evolutionary development of clones that are both optimized for antigen binding and at the same time optimized in terms of stability.
How Fab glycans stabilize antibodies remains to be investigated. It was shown that introduction of glycans specifically introduced to mask aggregation-prone regions improves antibody stability to a similar extent as removing those regions by replacement of hydrophobic amino acid residues with hydrophilic ones (8), suggesting that Fab glycans localized close to hydrophobic residues would shield those. However, for the investigated adalimumab variants, we did not find a correlation between the position of the glycan and the impact on thermostability in terms of proximity to hydrophobic pockets. However, it is difficult to estimate whether attached glycans would be able to shield those hydrophobic residues, since glycans can be very flexible. Negatively charged sialic acid residues might change the overall charge of IgG molecules and thereby stabilize antibodies. However, we show that sialic acid is not required for the improved stability caused by Fab glycans.
Our finding that the introduction of Fab glycans can improve antibody stability is promising for therapeutic as well as diagnostic purposes (facilitation of manufacturing and storage, increase of serum half-life/efficacy, and range of practical applications). However, introduction of Fab glycans to improve antibody stability may affect antigen binding. Nevertheless, we previously showed that for more than one of the generated adalimumab clones with Fab glycans, there were no or relatively small effects of these introduced glycans on antigen binding (3). In particular, the Fab glycans of adalimumab NH84 had no effects on antigen binding, whereas they positively contributed to antibody stability, indicating that this could be an interesting drug candidate. For most of the clones where we removed the naturally acquired Fab glycans, we found either no effects or a modestly (twofold) better antigen binding in the presence of Fab glycans.
We determined thermal unfolding profiles using thermofluor assay analysis with ANS, a fluorescent probe that binds to hydrophobic pockets during protein unfolding, and we observed that fluorescence was different for clones with Fab glycans. One could argue that Fab glycans do not necessarily influence antibody stability, but affect ANS binding, or that ANS influences protein unfolding. However, for cetuximab, a therapeutic antibody carrying Fab glycans (27), DSC, a technique without use of a fluorescent probe, provided a Tm in a similar range as obtained by this analysis with ANS. In addition, for two clones we confirmed the stabilizing effects of the Fab glycans using DSC. For some clones, the position of the peaks was not altered, only their widths. A broader peak and higher SEM might indicate more heterogeneity, and more structural variability through Fab glycans could explain this. Whether such differences in widths of peaks translate in differences in in vivo stability is unknown. Also, using DSC, we did not observe such broader peaks.
In conclusion, we demonstrate in this study that variable domain N-linked glycans acquired during somatic hypermutation can contribute to IgG antibody stability. Our findings indicate that introducing Fab glycans may represent a mechanism to improve therapeutic/diagnostic antibody stability.
Author Contributions
TR designed the study; FB, ND, MB, ST, PO-d-H, and RS acquired, analyzed, and interpreted the data; TR supervised the project; FB and TR wrote the manuscript. All coauthors critically reviewed and approved the final manuscript.
Conflict of Interest Statement
The authors declare that the research was conducted in the absence of any commercial or financial relationships that could be construed as a potential conflict of interest.
Supplementary Material
The Supplementary Material for this article can be found online at https://www.frontiersin.org/articles/10.3389/fimmu.2018.00740/full#supplementary-material.
References
1. van de Bovenkamp FS, Hafkenscheid L, Rispens T, Rombouts Y. The emerging importance of IgG Fab glycosylation in immunity. J Immunol (2016) 196(4):1435–41. doi:10.4049/jimmunol.1502136
2. Dunn-Walters D, Boursier L, Spencer J. Effect of somatic hypermutation on potential N-glycosylation sites in human immunoglobulin heavy chain variable regions. Mol Immunol (2000) 37(3–4):107–13. doi:10.1016/S0161-5890(00)00038-9
3. van de Bovenkamp FS, Derksen NIL, Ooijevaar-de Heer P, van Schie KA, Kruithof S, Berkowska MA, et al. Adaptive antibody diversification through N-linked glycosylation of the immunoglobulin variable region. Proc Natl Acad Sci U S A (2018) 115(8):1901–6. doi:10.1073/pnas.1711720115
4. Bohne A, Lang E, von der Lieth CW. SWEET – WWW-based rapid 3D construction of oligo- and polysaccharides. Bioinformatics (1999) 15(9):767–8. doi:10.1093/bioinformatics/15.9.767
5. Holm E, Sletten K, Husby G. Structural studies of a carbohydrate-containing immunoglobulin-lambda-light-chain amyloid-fibril protein (AL) of variable subgroup III. Biochem J (1986) 239(3):545–51. doi:10.1042/bj2390545
6. Middaugh CR, Litman GW. Atypical glycosylation of an IgG monoclonal cryoimmunoglobulin. J Biol Chem (1987) 262(8):3671–3.
7. Karimi M, Sletten K, Westermark P. Biclonal systemic AL-amyloidosis with one glycosylated and one nonglycosylated AL-protein. Scand J Immunol (2003) 57(4):319–23. doi:10.1046/j.1365-3083.2003.01230.x
8. Courtois F, Agrawal NJ, Lauer TM, Trout BL. Rational design of therapeutic mAbs against aggregation through protein engineering and incorporation of glycosylation motifs applied to bevacizumab. MAbs (2016) 8(1):99–112. doi:10.1080/19420862.2015.1112477
9. Wu SJ, Luo J, O’Neil KT, Kang J, Lacy ER, Canziani G, et al. Structure-based engineering of a monoclonal antibody for improved solubility. Protein Eng Des Sel (2010) 23(8):643–51. doi:10.1093/protein/gzq037
10. Pepinsky RB, Silvian L, Berkowitz SA, Farrington G, Lugovskoy A, Walus L, et al. Improving the solubility of anti-LINGO-1 monoclonal antibody Li33 by isotype switching and targeted mutagenesis. Protein Sci (2010) 19(5):954–66. doi:10.1002/pro.372
11. Julian MC, Li L, Garde S, Wilen R, Tessier PM. Efficient affinity maturation of antibody variable domains requires co-selection of compensatory mutations to maintain thermodynamic stability. Sci Rep (2017) 7:1–13. doi:10.1038/srep45259
12. Kruijsen D, Einarsdottir HK, Schijf MA, Coenjaerts FE, van der Schoot EC, Vidarsson G, et al. Intranasal administration of antibody-bound respiratory syncytial virus particles efficiently primes virus-specific immune responses in mice. J Virol (2013) 87(13):7550–7. doi:10.1128/JVI.00493-13
13. van Schouwenburg PA, Kruithof S, Votsmeier C, van Schie K, Hart MH, de Jong RN, et al. Functional analysis of the anti-adalimumab response using patient-derived monoclonal antibodies. J Biol Chem (2014) 289(50):34482–8. doi:10.1074/jbc.M114.615500
14. van Schie KA, Ooijevaar-De Heer P, Kruithof S, Plasencia C, Jurado T, Pascual Salcedo D, et al. Infusion reactions during infliximab treatment are not associated with IgE anti-infliximab antibodies. Ann Rheum Dis (2017) 76(7):1285–8. doi:10.1136/annrheumdis-2016-211035
15. Dekkers G, Plomp R, Koeleman CA, Visser R, von Horsten HH, Sandig V, et al. Multi-level glyco-engineering techniques to generate IgG with defined Fc-glycans. Sci Rep (2016) 6. doi:10.1038/srep36964
16. Nagelkerke SQ, Dekkers G, Kustiawan I, van de Bovenkamp FS, Geissler J, Plomp R, et al. Inhibition of Fc R-mediated phagocytosis by IVIg is independent of IgG-Fc sialylation and Fc RIIb in human macrophages. Blood (2014) 124(25):3709–18. doi:10.1182/blood-2014-05-576835
17. Kaneko Y, Nimmerjahn F, Ravetch JV. Anti-inflammatory activity of immunoglobulin G resulting from Fc sialylation. Science (2006) 313(5787):670–3. doi:10.1126/science.1129594
18. Käsermann F, Boerema DJ, Rüegsegger M, Hofmann A, Wymann S, Zuercher AW, et al. Analysis and functional consequences of increased Fab-sialylation of intravenous immunoglobulin (IVIG) after lectin fractionation. PLoS One (2012) 7(6):e37243. doi:10.1371/journal.pone.0037243
19. Guhr T, Bloem J, Derksen NI, Wuhrer M, Koenderman AH, Aalberse RC, et al. Enrichment of sialylated IgG by lectin fractionation does not enhance the efficacy of immunoglobulin G in a murine model of immune thrombocytopenia. PLoS One (2011) 6(6):e21246. doi:10.1371/journal.pone.0021246
20. Stadlmann J, Weber A, Pabst M, Anderle H, Kunert R, Ehrlich HJ, et al. A close look at human IgG sialylation and subclass distribution after lectin fractionation. Proteomics (2009) 9(17):4143–53. doi:10.1002/pmic.200800931
21. Bondt A, Rombouts Y, Selman MH, Hensbergen PJ, Reiding KR, Hazes JM, et al. Immunoglobulin G (IgG) Fab glycosylation analysis using a new mass spectrometric high-throughput profiling method reveals pregnancy-associated changes. Mol Cell Proteomics (2014) 13(11):3029–39. doi:10.1074/mcp.M114.039537
22. Easterbrook-Smith SB, Dwek RA. The use of ANS fluorescence as a probe for immunoglobulin flexibility. FEBS Lett (1980) 121(2):253–6. doi:10.1016/0014-5793(80)80355-3
23. Rispens T, Lakemond CMM, Derksen NIL, Aalberse RC. Detection of conformational changes in immunoglobulin G using isothermal titration calorimetry with low-molecular-weight probes. Anal Biochem (2008) 380(2):303–9. doi:10.1016/j.ab.2008.06.001
24. Ericsson UB, Hallberg BM, Detitta GT, Dekker N, Nordlund P. Thermofluor-based high-throughput stability optimization of proteins for structural studies. Anal Biochem (2006) 357(2):289–98. doi:10.1016/j.ab.2006.07.027
25. McConnell AD, Zhang X, Macomber JL, Chau B, Sheffer JC, Rahmanian S, et al. A general approach to antibody thermostabilization. MAbs (2014) 6(5):1274–82. doi:10.4161/mabs.29680
26. de Taeye SW, Ozorowski G, Torrents de la Peña A, Guttman M, Julien JP, van den Kerkhof TL, et al. Immunogenicity of stabilized HIV-1 envelope trimers with reduced exposure of non-neutralizing epitopes. Cell (2015) 163(7):1702–15. doi:10.1016/j.cell.2015.11.056
27. Hintersteiner B, Lingg N, Janzek E, Mutschlechner O, Loibner H, Jungbauer A. Microheterogeneity of therapeutic monoclonal antibodies is governed by changes in the surface charge of the protein. Biotechnol J (2016) 11(12):1617–27. doi:10.1002/biot.201600504
Keywords: antibody stability, variable domain glycosylation, Fab glycosylation, adalimumab, IVIg, thermal unfolding
Citation: van de Bovenkamp FS, Derksen NIL, van Breemen MJ, de Taeye SW, Ooijevaar-de Heer P, Sanders RW and Rispens T (2018) Variable Domain N-Linked Glycans Acquired During Antigen-Specific Immune Responses Can Contribute to Immunoglobulin G Antibody Stability. Front. Immunol. 9:740. doi: 10.3389/fimmu.2018.00740
Received: 22 January 2018; Accepted: 26 March 2018;
Published: 12 April 2018
Edited by:
Deborah K. Dunn-Walters, University of Surrey, United KingdomReviewed by:
Christian Margreitter, King’s College London, United KingdomToma Keser, University of Zagreb, Croatia
Copyright: © 2018 van de Bovenkamp, Derksen, van Breemen, de Taeye, Ooijevaar-de Heer, Sanders and Rispens. This is an open-access article distributed under the terms of the Creative Commons Attribution License (CC BY). The use, distribution or reproduction in other forums is permitted, provided the original author(s) and the copyright owner are credited and that the original publication in this journal is cited, in accordance with accepted academic practice. No use, distribution or reproduction is permitted which does not comply with these terms.
*Correspondence: Theo Rispens, dC5yaXNwZW5zQHNhbnF1aW4ubmw=