- Laboratory for Immunotherapy, RIKEN Center for Integrative Medical Sciences (IMS), Yokohama, Japan
Vaccines against a variety of infectious diseases have been developed and tested. Although there have been some notable successes, most are less than optimal or have failed outright. There has been discussion about whether either B cells or dendritic cells (DCs) could be useful for the development of antimicrobial vaccines with the production of high titers of antibodies. Invariant (i)NKT cells have direct antimicrobial effects as well as adjuvant activity, and iNKT-stimulated antigen-presenting cells (APCs) can determine the form of the ensuing humoral and cellular immune responses. In fact, upon activation by ligand, iNKT cells can stimulate both B cells and DCs as via either cognate or non-cognate help. iNKT-licensed DCs generate antigen-specific follicular helper CD4+ T cells, which in turn stimulate B cells, thus leading to long-term antigen-specific antibody production. Follicular helper iNKT cell-licensed B cells generally produce rapid, but short-term antibody. However, under some conditions in the presence of Th cells, the antibody production can be prolonged. With regards to humoral immunity, the quality and quantity of Ab produced depends on the APC type and the form of the vaccine. In terms of cellular immunity and, in particular, the induction of cytotoxic CD8+ T cells, iNKT-licensed DCs show prominent activity. In this review, we discuss differences in iNKT-stimulated APC types and the quality of the ensuing immune response, and also discuss their application in vaccine models to develop successful preventive immunotherapy against infectious diseases.
Introduction
The success of vaccination strategies against viral infection or cancer depends on the efficient generation of appropriate antigen-specific T and B cell responses. Adequate antibody (Ab) responses of appropriate specificity elicited by vaccination are required to control and protect against many viral pathogens, such as influenza, human immunodeficiency virus, and human papilloma virus (1). Not only the suitable form of antigen, e.g., the commonly used inactivated virus, live attenuated virus, and recombinant viral protein, but also the optimal adjuvant are required for a successful vaccine. The development of ideal vaccine systems has been intensively explored to enhance the efficacy of weak antigens and broaden the immune response profile, leading to generation of high titer broadly neutralizing anti-viral antibodies.
Vaccines targeting B cells are essentially, of two types, T-dependent and T-independent, based on the requirement for T-cell help for Ab production (2). T-independent B cell responses are usually elicited by non-protein antigens that are unable to stimulate Th cells. Multimeric haptens or polysaccharides are typical T-independent B cell antigens that are recognized via the B cell antigen receptor (BCR). T-independent antigens generally induce robust and rapid B cell antibody responses, but with a low level of somatic hypermutation and thus affinity maturation, and limited isotype switching. T-dependent responses are typically induced by protein antigens and, as the term implies, there is cognate T-cell help for the antigen-specific B cells (3), which is provided by a specialized subset of CD4+ T cells called T follicular helper (Tfh) cells. When antigens contact B cells in the follicles of secondary lymphoid organs, the antigen is internalized by the B cells upon binding to antigen-specific BCRs. The antigen is then processed and antigen-derived peptides are presented in the context of MHC class II (MHC II) molecules. Subsequently, the activated B cells are recruited to the border of the T cell and B cell zones, in which Tfh cells are generated following interacting with dendritic cells (DCs) presenting the same antigen. For the generation of Tfh cells, upregulation of the transcriptional repressor Bcl-6, costimulation by CD28, and stimulation with IL-21 have been reported as important factors (3). Also, by upregulating CXCR5, Tfh cells in turn localize to the boundary of the T and B cell zone (3), which is critical location for B cells to encounter Tfh cells.
Besides these classical T-dependent and T-independent vaccines, NKT cell-mediated vaccines have also been tested as a third vaccine candidate. NKT cells constitute approximately 0.05–0.2% of lymphocytes among human peripheral blood mononuclear cells and are classified into two groups: type I NKT cells express the invariant Vα14-Jα18 TCRα chain paired with either Vβ2, Vβ7, or Vβ8 in mice and Vα24-Jα18/Vβ11 in humans (4). The type I, invariant NKT cells (hereafter iNKT) recognize glycolipids, such as α-GalCer. By contrast, type II NKT cells display more diverse αβ-TCR pairings and respond to sulfatide, but do not to α-GalCer (5). Several reports have shown that iNKT cells can deliver helper signals to B cells directly or indirectly. In infectious diseases, neutralizing Ab production induced by vaccines represents a major protection mechanism against pathogens. Here, we review the features of iNKT cell-mediated Ab production, particularly by interacting directly or indirectly with B cells. We also discuss how these two pathways, i.e., vaccines utilizing iNKT cell help for B cells or iNKT cell help for DCs, augment efficient antigen-specific Ab production in the development of vaccination strategies against infectious diseases.
The Role of iNKT Cells in Infectious Diseases
Realization of the importance of iNKT cells in protection from infectious diseases has largely been based studies of the responses of Jα18- or CD1d-deficient mice, both of which lack iNKT cells, to viruses, bacteria, and parasites (6, 7). The outcome of most of these infectious models is worse in the iNKT-deficient animals. In studies of viral infections, iNKT cells play a protective role against influenza virus and cytomegalovirus (8, 9), herpes simplex virus type 1, and hepatitis B virus (10). In bacterial infection models, iNKT cells have been shown to be important against Pseudomonas aeruginosa, Streptococcus pneumoniae, Mycobacterium tuberculosis (11), Chlamydia pneumoniae, Sphingomonas paucimobilis, and Staphylococcus aureus (12). The protective responses of iNKT cells during infections are mediated by two mechanisms. First is the direct activation by stimulation of the NKT TCR by iNKT cell ligands expressed on various pathogens. Second is indirect activation of iNKT cells is through other immune cells and is due to the cytokine milieu and toll-like receptors (TLRs) agonists. In the first type of response, iNKT cells directly recognize glycolipids and lipoproteins, highly abundant in cell walls of many pathogens. These include glycosphingolipid in Gram-negative bacteria Sphingomonas, diacylglycerol in Borrelia burgdorferi, phosphatidylinositol mannoside in Mycobacterium tuberculosis, and glycosphingophospholipid in the protozoa Leishmania donovani (13). In the second type of response, iNKT cells are activated through macrophages during microbial infections. When infected, antigen-presenting cells (APCs) recognize bacterial signals via innate receptors, such as TLRs of macrophages, e.g., TLR4, TLR7, and TLR9. These allow macrophages to produce inflammatory cytokines, e.g., IL-12, which activate iNKT cells (14).
Two Types of iNKT Based Vaccines by Focusing on iNKT-Licensed B Cells and iNKT-Licensed DCs to Induce Humoral Immunity
B cell response is generally defined as being cognate helper T (Th), namely Tfh-dependent (TD) or Tfh-independent (TI) (3). In terms of iNKT-mediated vaccines directed toward B cell responses, there are two strategies using “iNKT mediated-DC therapy” or “iNKT mediated-B cell therapy” (Figure 1). For the induction of humoral responses, iNKT cell-licensed DCs (non-cognate help) can induce Tfh cells, resulting in long-lasting antibody responses. On the other hand, iNKT cell-licensed B cells (cognate help) generally result in rapid and robust, but short-term responses. However, under some conditions, iNKT cell and B cell interactions seem to be more flexible and may be dependent on the experimental models. These are further discussed below.
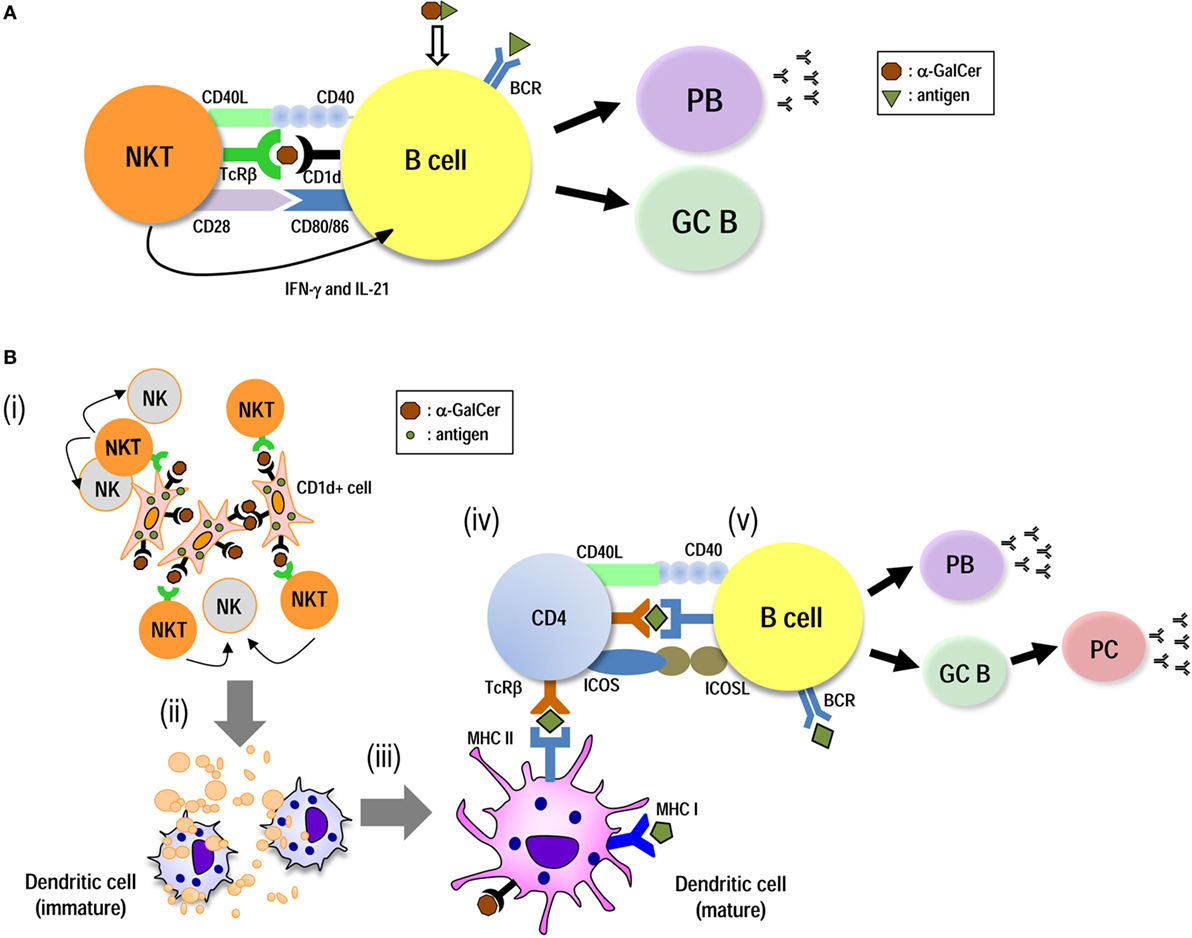
Figure 1. Induction of humoral immunity by utilizing iNKT-licensed B cells and iNKT-licensed dendritic cells (DCs). iNKT cells can provide both direct (A) and DC-mediated (B) help for antigen-specific B cell responses. (A) Innate iNKTfh cells recognize glycolipid antigen-CD1d on B cells and directly provide helper signals. These initiate plasmablast (PB) expansion and germinal center (GC) formation, leading to the primary class-switched antibody (Ab) production. (B) Antigen-expressing artificial adjuvant vector cells [e.g., artificial adjuvant vector cells (aAVC)-hemagglutinin (HA)] are comprised of the CD1d–α-GalCer complex on the surface of the aAVC and HA are expressed in the cytosol. (i) Administration of aAVC-HA initially stimulates iNKT cells. (ii) The aAVC-HA is killed by iNKT cells and NK cells and then HA tumor antigen released from them can be captured by endogenous CD11c+ DCs. (iii) The CD11c+ DCs then undergo iNKT cell-induced maturation. (iv) The activated DCs can then induce an HA-specific CD4+ T cell response. Thus, the CD11c+ DCs in situ are able to present HA antigen derived from phagocytosed aAVC-HA to CD4+ T cells. (v) On the other hand, B cells also capture HA antigen. B cells can then be stimulated by antigen-specific CD4+ T cells, resulting in PB expansion, GC formation, and long-term Ab production.
Vaccines Using iNKT-Licensed B Cell Responses to Induce iNKTfh-Mediated Humoral Immunity
A lipid antigen component, e.g., a hapten or a lipid antigen conjugated to CD1d-binding glycolipids, can induce T-independent Ab production (15, 16). In addition to lipid antigens, a coadministration of T-dependent protein antigen plus α-GalCer, certain protein antigen conjugates, including protein–α-GalCer conjugates or protein incorporated into α-GalCer-liposomes, have been introduced as vaccine formulations for cognate iNKT help (15).
When such immunogens are taken up via antigen-specific BCRs, they promote extensive BCR cross-linking and enhance BCR internalization. Simultaneously, the iNKT cell ligand from the immunogen is loaded on CD1d inside of B cells and then expressed on the cell surface, resulting in a strong cognate iNKT–B cell interaction (16–19) (Figure 1A). Without incorporating the iNKT cell ligand, a T-independent response usually elicits only short-lived IgM antibody without germinal center (GC) formation, affinity maturation, and class switch recombination (18). However, with an iNKT cell ligand, even as a T-independent response, iNKT cells are converted to iNKTfh cells in a BCL6-dependent manner. iNKT cells help B cells to proliferate and differentiate into extrafollicular plasma cells. They also robustly and rapidly induce GC formation, low-affinity antibody maturation, secretion of high titers of specific IgM, and early class-switched antibodies. On the other hand, this humoral immunity is short-lived and does not form a memory response (20–22).
In studies of the mechanism of iNKT-licensed B cell-dependent Ab production by lipid- and/or protein-conjugated complexes, several factors have turned out to be essential. Since iNKT cells in steady state are usually located in the marginal zone in spleen or interfollicular or medulla areas in LNs (23, 24), iNKT cells can more easily be activated by contacting marginal zone B cells than by follicular B cells after administration of α-GalCer (16, 21). The cognate help of iNKT cells for B cells presenting CD1d-lipid is dependent on IFN-γ, IL-21, CD40/CD40L, and B7-1/2 (16, 20, 21). SAP expression in iNKT cells plays a key role in the cognate help for antigen-specific B cells, although this is not the case for non-cognate helper functions (25).
In terms of vaccine strategy, several types of vaccines have been described including T-dependent protein antigens combined with α-GalCer and these in nanoparticle liposome formulations (20–22). These showed the direct interaction between B cells and iNKT cells in response to T-dependent antigen, however, these strategies did not bring about high or long duration Ab production (20–22). On the other hand, other investigators have used different conditions and could demonstrate long-term responses. Lang et al. showed that immunization with T-dependent protein antigen together with α-GalCer in the presence of Th cells resulted in long-lasting Ab titers (26–28). A model using coadministration of NP-KLH and α-GalCer elicits Ig class-switched Ab production and requires Th cells and CD1d on B cells, but not on DCs. Here, BAFF- and APRIL-secreting NKT cells play a role in plasma cell longevity (27, 28). Also, liposomal nanoparticles displaying both lipid and polysaccharide antigens induce interactions with DCs for iNKT cell activation and then can elicit long-term B cell memory (29). Thus, the successful induction of iNKT cell-licensed B cells in situ demands three signals (Figure 1), BCR cross-linking (signal 1), costimulatory molecules, such as CD28-CD80/CD86, SLAM-family receptor signaling, and CD40-CD40L (signal 2), and inflammatory cytokines (IFN-γ and IL-21) (signal 3).
Vaccines Using iNKT-Licensed DC Responses to Induce Tfh-Mediated Humoral Immunity
We previously showed that coadministration of OVA protein antigen plus α-GalCer elicited both CD4+ and CD8+ T cell responses via DC maturation (30). Similar responses have been demonstrated in some cancer and infectious disease models (31–33). We elucidated the mechanism of iNKT-licensed DCs for T cell response; activated iNKT cells promote DC maturation via CD40/40L signaling and cytokines (IFN-γ and TNF-α). DCs in situ capture the protein antigen and α-GalCer-simultaneously, then present α-GalCer on CD1d to iNKT cells and the peptide on MHC class II to CD4+ T cells or class I to CD8+ T cells (34, 35). The antigen-specific CD4+ Tfh cells that are derived from the helper CD4+ T cells stimulate antigen-specific B cells that had already taken up the protein via the BCR and presented peptide in MHC class II (18, 36) (Figure 1B). Therefore, expression of both CD1d and MHC class II on DCs and MHC, II but not CD1d on B cells is essential for Ab production (37). Such a vaccine elicits a strong Ab response, i.e., characterized by GC formation, high affinity maturation, primary class-switched Ab, plasma cells, and memory B cells (37, 38).
In the steady state, DCs express CD80/86 to some extent. After activation by iNKT cells, expression of costimulatory molecules, i.e., CD80/86 and CCR7, on DCs is promptly upregulated. The mature DCs are key players for priming CD4+ Tfh cells (3). When focused on DC subsets, we and others showed that the XCR1− DC (CD8− DC) subset is superior to the CD8+ DC subset for CD4+ Tfh cell priming (39). Shin et al. demonstrated this by using mAb to identify specific DC subsets, i.e., anti-DEC205 for the CD8+ DC subset or anti-DCIR2 antibodies for the CD8− DC subset, and also showed that the CD8− DCs subset is superior to the CD8+ DC subset because of its dominant ICOSL and OX40L expression (40).
The location of DC and iNKT cells before and after iNKT cell activation has recently been clarified. As discussed previously, in the steady state, iNKT cells are localized in the marginal zone (MZ) of the spleen or medulla in LN (41), whereas XCR1− DCs (CD8− DC or 33D1+ DCs) are localized in the bridging channel (BC), a unique region of the spleen that spans the interface between the red pulp (RP) and white pulp (WP) and XCR1+ DCs reside in the MZ. After i.v. immunization with antigens and/or adjuvants, the majority of both DC subsets migrate into the WP, but XCR1+ DCs preferentially go to the CD8+ T cell area and XCR1− DCs prefer to go to the CD4+ T cell area (42, 43). After activation by α-GalCer or ligand-containing cells, activated iNKT cells accumulate in the MZ or BC and can be in close contact with DCs that have already taken up antigen and α-GalCer (44, 45). iNKT-licensed DCs then relocate to each T cell area.
Germinal center formation in secondary lymphoid organs is considered key for inducing better Ig class switching, somatic mutation, affinity maturation, and long-lasting Ab responses (46). In addition to Bcl6fl/flCD4-cre mice, LTα−/− mice and Lyn−/− mice are deficient in GC formation. When LTα−/− mice and Lyn−/− mice are immunized with antigen plus adjuvant, they respond with long-lasting Ab production, probably due to the generation of long-lived plasmablasts (19, 47–51). However, judging from the data using Bcl6fl/flCD4-cre mice, the formation of GC is essential for iNKT cell-mediated Ab production. When NKTfh and Tfh cells are compared, iNKTfh cells induce earlier GC formation, but it is more short-lived compared to GCs induced by Tfh cells (4). On the other hand, Tfh cells help in the induction of mature GCs better than iNKTfh cells (4). The expression of Bcl6 by Th cells is apparently crucial for efficient Ab production. Thus, iNKT cell-licensed DCs induce antigen-specific CD4+ Tfh cells and drive them into the B cell zone (Figure 1B). Then, Tfh cells are engaged in cognate interactions with B cells, resulting in the formation of early GCs and also leading to the long-lasting production of antigen-specific Abs.
The development of effective vaccines is a critical need. As discussed above, immunization by coadministration of protein antigen together with an iNKT cell ligand clearly generates potent Ab production. Traditional immunization protocols usually require a high dose of protein, e.g., 100–500 µg OVA protein per mouse is typically injected (15) yet, even so, Ab production is not impressively high (52). We have reported the artificial adjuvant vector cell (aAVC) system as an efficient vaccine strategy that can potently induce innate and adaptive immunity and this will be discussed in detail in the next section. But, to summarize this section on the induction of antigen-specific Tfh cells and Ab, effective iNKT cell-licensed DCs in situ are required (30, 34, 35, 53). The DCs require three factors: (i) expression of the appropriate antigen peptide–MHC complex, (ii) upregulation of costimulatory and chemokine molecules, including CD80/CD86, ICOSL, and OX40L, and (iii) production of inflammatory cytokines and chemokines, such as IL-6, IL-12, and CCL17.
An Efficient Strategy Using iNKT-Licensed DCs to Induce Humoral Immunity
We have established the aAVC system, comprised of a CD1d+ cellular vaccine incorporating foreign protein antigen plus an iNKT cell glycolipid antigen. We chose “adjuvant vector cell” as the name for this cellular vaccine to describe the fact that the “vector like cells” deliver the antigen as well as an iNKT cell adjuvant to host DCs. We used NIH3T3 cells for mouse and HEK293 cells for humans as vector cells (54, 55). These cells are co-transfected with CD1d and antigen mRNA, and then loaded with α-GalCer for use (54–56). The aAVC, therefore, express the α-GalCer–CD1d complex on their surface and antigen protein intracellularly. The aAVCs directly activate iNKT cells via the α-GalCer ligand, and iNKT cells producing IFN-γ can then simultaneously activate NK cells. The combination of innate killer iNKT/NK cells capable of producing IFN-γ then eliminates the adjuvant vector cells, which is not syngeneic with the recipient. Subsequently, the killed aAVC are taken up by DCs (CD8+ or XCR1+ DCs) in situ, thereby several immunogenic features of DCs are engaged. The aAVC captured by DCs in lung, liver, and spleen undergo maturation due to interaction with CD40L on iNKT cells and then produce inflammatory cytokines. The aAVC vaccine can efficiently generate antigen-specific CD8+ T cells and memory T cells (36). Interestingly, in aAVC-vaccinated mice, antigen-specific CD4+ Tfh primed by XCR1− DCs and GC were both generated, resulting in induction of long-term Ab production (39). Killed aAVCs are phagocytosed, mainly by XCR1+ DC (CD8+ DC) cells and, presumably, the separated protein antigen and α-GalCer are endocytosed simultaneously by the XCR1− DC subset. MHC II−/− mice do not have CD4+ T cells but do have iNKT cells. Immunization with aAVC-OVA elicited no detectable specific Ab response in these mice (39), indicating that iNKT cell-licensed DC strategies require MHC II for CD4+ T cell-mediated humoral immunity. Although both Tfh and iNKTfh co-exist in the aAVC-mouse models, Tfh cells are superior to iNKTfh cells for inducing Ab production (39).
Application of an iNKT Cell-Triggered DC-Designed Vaccine “Artificial Adjuvant Vector Cell” for Influenza Infection
Influenza virus is a member of the orthomyxoviridae family that contains a segmented negative-sense single-stranded RNA genome (57). Influenza infection is a major global health problem, which is initially caused by viral infection of the respiratory tract. Upon viral infection, iNKT cells in the follicular areas of LNs facilitate IL-4 signaling to B cells which triggers the seeding of GC cells and B cell immunity (23). However, to provide sufficient protection against influenza virus in humans, both adaptive T cell and Ab-producing B cells need to be established and maintained as immunological memory.
Immunity against influenza virus is largely mediated by neutralizing antibodies that target the major surface glycoprotein hemagglutinin (HA) (58), in particular, the immunodominant head region of HA, or the viral neuraminidase (NA) (59). Preexisting neutralizing Ab, rather than the recall of virus-specific CTL, is thought to account for memory anti-viral protection. Unfortunately, however, the antiviral antibodies generated by immunization or natural infection are only effective against a limited number of viral strains.
Several studies have addressed the possibility of using a combined vaccine approach, i.e., coadministration of inactivated influenza virus (IIV) together with α-GalCer, to enhance protective efficacy via subcutaneous or intranasal administration (60–62). We established CD1d+ HA mRNA-transfected cells loaded with α-GalCer (aAVC-HA) and demonstrated that this is a more efficient strategy for generating antigen-specific Ab production than coadministration of antigen plus α-GalCer (Figure 2) (39). The efficacy of aAVC appears to depend on the GC and Tfh. We could easily modify the HA protein, depending on the circulating virus strain, and the manufacturing process for this vaccine has a much shorter timeframe than others, e.g., egg-derived vaccines, which would be especially valuable during a flu pandemic. The aAVC vaccine thus holds great promise as a potential broad spectrum prophylactic or therapeutic agent and for the development of a universal influenza or other viral vaccine (39). As a future study, if the optimal stem region antigen were incorporated into an iNKT cell-mediated vaccine, a universal HA stem antigen-expressing aAVC could be developed.
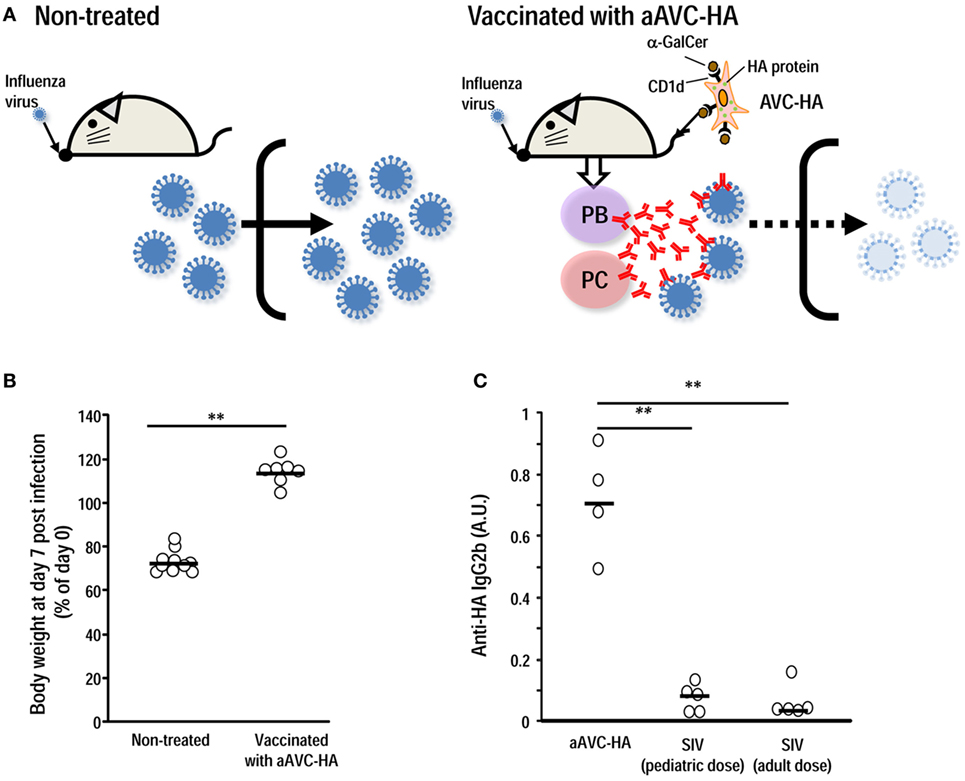
Figure 2. Vaccination with aAVC-HA protects against influenza virus infection. (A) Mice were initially vaccinated with 5 × 105 aAVC-HA. Two weeks later, non-treated and the aAVC-HA vaccinated mice were challenged with a lethal dose of PR8 influenza virus to assess production of virus-neutralizing antibody. (B) Non-treated and vaccinated mice were evaluated for weight loss a week after an infection. (C) HA-specific antibody in the serum was assessed by ELISA 2 weeks after administration of three types of vaccines to C57BL/6 mice, two standard doses of the standard influenza vaccine (0.75 µg/kg, the human pediatric dose, and 0.3 µg/kg, the human adult dose) or the aAVC-HA (mean ± SEM, n = 4–7) **P < 0.01.
Conclusion
iNKT cells play an immunomodulatory role during immune responses. To utilize this capacity as an adjuvant in terms of novel vaccine strategies, it is essential to understand the interaction of iNKT cells with APCs in the host. We here summarized details of the relationship of iNKT cell-triggered B cells and DCs with Ab production and compared them in terms of vaccine development. Now we need to consider the future directions and challenges in translating these findings from experimental data obtained from mice to use in the clinic.
Author Contributions
SF and KS conceptualized, wrote, and edited the manuscript. SY and YS wrote and edited the manuscript.
Conflict of Interest Statement
The authors declare that the research was conducted in the absence of any commercial or financial relationships that could be construed as a potential conflict of interest.
Acknowledgments
The authors are grateful to Dr. Peter Burrows for peer-reviewing and helpful comments in the preparation of the manuscript. This work is supported by grants from the Ministry of Education, Culture, Sports, Science and Technology of Japan (to KS and SF, grant number 15K09590) and the Japan Agency for Medical Research and Development (translational research network program) (to SF, grant number 15lm0103002j0004).
References
1. Reed SG, Orr MT, Fox CB. Key roles of adjuvants in modern vaccines. Nat Med (2013) 19(12):1597–608. doi:10.1038/nm.3409
2. Mond JJ, Lees A, Snapper CM. T cell-independent antigens type 2. Annu Rev Immunol (1995) 13:655–92. doi:10.1146/annurev.iy.13.040195.003255
3. Vinuesa CG, Linterman MA, Yu D, MacLennan IC. Follicular helper T cells. Annu Rev Immunol (2016) 34:335–68. doi:10.1146/annurev-immunol-041015-055605
4. Fujii S, Shimizu K, Hemmi H, Steinman RM. Innate Vα14+ natural killer T cells mature dendritic cells, leading to strong adaptive immunity. Immunol Rev (2007) 220(1):183–98. doi:10.1111/j.1600-065X.2007.00561.x
5. Dhodapkar MV, Kumar V. Type II NKT cells and their emerging role in health and disease. J Immunol (2017) 198(3):1015–21. doi:10.4049/jimmunol.1601399
6. Brennan PJ, Brigl M, Brenner MB. Invariant natural killer T cells: an innate activation scheme linked to diverse effector functions. Nat Rev Immunol (2013) 13(2):101–17. doi:10.1038/nri3369
7. Yang JQ, Zhou Y, Singh RR. Effects of invariant NKT cells on parasite infections and hygiene hypothesis. J Immunol Res (2016) 2016:2395645. doi:10.1155/2016/2395645
8. Tessmer MS, Fatima A, Paget C, Trottein F, Brossay L. NKT cell immune responses to viral infection. Expert Opin Ther Targets (2009) 13(2):153–62. doi:10.1517/14712590802653601
9. Paget C, Ivanov S, Fontaine J, Blanc F, Pichavant M, Renneson J, et al. Potential role of invariant NKT cells in the control of pulmonary inflammation and CD8+ T cell response during acute influenza A virus H3N2 pneumonia. J Immunol (2011) 186(10):5590–602. doi:10.4049/jimmunol.1002348
10. Opasawatchai A, Matangkasombut P. iNKT cells and their potential lipid ligands during viral infection. Front Immunol (2015) 6:378. doi:10.3389/fimmu.2015.00378
11. Gao Y, Williams AP. Role of innate T cells in anti-bacterial immunity. Front Immunol (2015) 6:302. doi:10.3389/fimmu.2015.00302
12. Crosby CM, Kronenberg M. Invariant natural killer T cells: front line fighters in the war against pathogenic microbes. Immunogenetics (2016) 68(8):639–48. doi:10.1007/s00251-016-0933-y
13. Anderson CK, Brossay L. The role of MHC class Ib-restricted T cells during infection. Immunogenetics (2016) 68(8):677–91. doi:10.1007/s00251-016-0932-z
14. Kohlgruber AC, Donado CA, LaMarche NM, Brenner MB, Brennan PJ. Activation strategies for invariant natural killer T cells. Immunogenetics (2016) 68(8):649–63. doi:10.1007/s00251-016-0944-8
15. Lang GA, Exley MA, Lang ML. The CD1d-binding glycolipid alpha-galactosylceramide enhances humoral immunity to T-dependent and T-independent antigen in a CD1d-dependent manner. Immunology (2006) 119(1):116–25. doi:10.1111/j.1365-2567.2006.02413.x
16. Leadbetter EA, Brigl M, Illarionov P, Cohen N, Luteran MC, Pillai S, et al. NK T cells provide lipid antigen-specific cognate help for B cells. Proc Natl Acad Sci U S A (2008) 105(24):8339–44. doi:10.1073/pnas.0801375105
17. Barral P, Eckl-Dorna J, Harwood NE, De Santo C, Salio M, Illarionov P, et al. B cell receptor-mediated uptake of CD1d-restricted antigen augments antibody responses by recruiting invariant NKT cell help in vivo. Proc Natl Acad Sci U S A (2008) 105(24):8345–50. doi:10.1073/pnas.0802968105
18. Dellabona P, Abrignani S, Casorati G. iNKT-cell help to B cells: a cooperative job between innate and adaptive immune responses. Eur J Immunol (2014) 44(8):2230–7. doi:10.1002/eji.201344399
19. Vomhof-DeKrey EE, Yates J, Leadbetter EA. Invariant NKT cells provide innate and adaptive help for B cells. Curr Opin Immunol (2014) 28:12–7. doi:10.1016/j.coi.2014.01.007
20. King IL, Fortier A, Tighe M, Dibble J, Watts GF, Veerapen N, et al. Invariant natural killer T cells direct B cell responses to cognate lipid antigen in an IL-21-dependent manner. Nat Immunol (2012) 13(1):44–50. doi:10.1038/ni.2172
21. Chang PP, Barral P, Fitch J, Pratama A, Ma CS, Kallies A, et al. Identification of Bcl-6-dependent follicular helper NKT cells that provide cognate help for B cell responses. Nat Immunol (2012) 13(1):35–43. doi:10.1038/ni.2166
22. Tonti E, Fedeli M, Napolitano A, Iannacone M, von Andrian UH, Guidotti LG, et al. Follicular helper NKT cells induce limited B cell responses and germinal center formation in the absence of CD4(+) T cell help. J Immunol (2012) 188(7):3217–22. doi:10.4049/jimmunol.1103501
23. Gaya M, Barral P, Burbage M, Aggarwal S, Montaner B, Warren Navia A, et al. Initiation of antiviral B cell immunity relies on innate signals from spatially positioned NKT cells. Cell (2018) 172(3):517–33.e20. doi:10.1016/j.cell.2017.11.036
24. Lee YJ, Wang H, Starrett GJ, Phuong V, Jameson SC, Hogquist KA. Tissue-specific distribution of iNKT cells impacts their cytokine response. Immunity (2015) 43(3):566–78. doi:10.1016/j.immuni.2015.06.025
25. Detre C, Keszei M, Garrido-Mesa N, Kis-Toth K, Castro W, Agyemang AF, et al. SAP expression in invariant NKT cells is required for cognate help to support B-cell responses. Blood (2012) 120(1):122–9. doi:10.1182/blood-2011-11-395913
26. Devera TS, Shah HB, Lang GA, Lang ML. Glycolipid-activated NKT cells support the induction of persistent plasma cell responses and antibody titers. Eur J Immunol (2008) 38(4):1001–11. doi:10.1002/eji.200738000
27. Lang GA, Devera TS, Lang ML. Requirement for CD1d expression by B cells to stimulate NKT cell-enhanced antibody production. Blood (2008) 111(4):2158–62. doi:10.1182/blood-2007-10-117309
28. Shah HB, Joshi SK, Rampuria P, Devera TS, Lang GA, Stohl W, et al. BAFF- and APRIL-dependent maintenance of antibody titers after immunization with T-dependent antigen and CD1d-binding ligand. J Immunol (2013) 191(3):1154–63. doi:10.4049/jimmunol.1300263
29. Bai L, Deng S, Reboulet R, Mathew R, Teyton L, Savage PB, et al. Natural killer T (NKT)-B-cell interactions promote prolonged antibody responses and long-term memory to pneumococcal capsular polysaccharides. Proc Natl Acad Sci U S A (2013) 110(40):16097–102. doi:10.1073/pnas.1303218110
30. Fujii S, Shimizu K, Smith C, Bonifaz L, Steinman RM. Activation of natural killer T cells by alpha-galactosylceramide rapidly induces the full maturation of dendritic cells in vivo and thereby acts as an adjuvant for combined CD4 and CD8 T cell immunity to a coadministered protein. J Exp Med (2003) 198(2):267–79. doi:10.1084/jem.20030324
31. Liu K, Idoyaga J, Charalambous A, Fujii S, Bonito A, Mordoh J, et al. Innate NKT lymphocytes confer superior adaptive immunity via tumor-capturing dendritic cells. J Exp Med (2005) 202(11):1507–16. doi:10.1084/jem.20050956
32. Padte NN, Li X, Tsuji M, Vasan S. Clinical development of a novel CD1d-binding NKT cell ligand as a vaccine adjuvant. Clin Immunol (2011) 140(2):142–51. doi:10.1016/j.clim.2010.11.009
33. Osmond TL, Farrand KJ, Painter GF, Ruedl C, Petersen TR, Hermans IF. Activated NKT cells can condition different splenic dendritic cell subsets to respond more effectively to TLR engagement and enhance cross-priming. J Immunol (2015) 195(3):821–31. doi:10.4049/jimmunol.1401751
34. Fujii S, Liu K, Smith C, Bonito AJ, Steinman RM. The linkage of innate to adaptive immunity via maturing dendritic cells in vivo requires CD40 ligation in addition to antigen presentation and CD80/86 costimulation. J Exp Med (2004) 199(12):1607–18. doi:10.1084/jem.20040317
35. Shimizu K, Kurosawa Y, Taniguchi M, Steinman RM, Fujii S. Cross-presentation of glycolipid from tumor cells loaded with α-galactosylceramide leads to potent and long-lived T cell mediated immunity via dendritic cells. J Exp Med (2007) 204(11):2641–53. doi:10.1084/jem.20070458
36. Fujii SI, Shimizu K. Exploiting antitumor immunotherapeutic novel strategies by deciphering the cross talk between invariant NKT cells and dendritic cells. Front Immunol (2017) 8:886. doi:10.3389/fimmu.2017.00886
37. Tonti E, Galli G, Malzone C, Abrignani S, Casorati G, Dellabona P. NKT-cell help to B lymphocytes can occur independently of cognate interaction. Blood (2009) 113(2):370–6. doi:10.1182/blood-2008-06-166249
38. Galli G, Pittoni P, Tonti E, Malzone C, Uematsu Y, Tortoli M, et al. Invariant NKT cells sustain specific B cell responses and memory. Proc Natl Acad Sci U S A (2007) 104(10):3984–9. doi:10.1073/pnas.0700191104
39. Yamasaki S, Shimizu K, Kometani K, Sakurai M, Kawamura M, Fujii SI. In vivo dendritic cell targeting cellular vaccine induces CD4(+) Tfh cell-dependent antibody against influenza virus. Sci Rep (2016) 6:35173. doi:10.1038/srep35173
40. Shin C, Han JA, Koh H, Choi B, Cho Y, Jeong H, et al. CD8alpha(-) dendritic cells induce antigen-specific T follicular helper cells generating efficient humoral immune responses. Cell Rep (2015) 11(12):1929–40. doi:10.1016/j.celrep.2015.05.042
41. Kastenmuller W, Torabi-Parizi P, Subramanian N, Lammermann T, Germain RN. A spatially-organized multicellular innate immune response in lymph nodes limits systemic pathogen spread. Cell (2012) 150(6):1235–48. doi:10.1016/j.cell.2012.07.021
42. Calabro S, Gallman A, Gowthaman U, Liu D, Chen P, Liu J, et al. Bridging channel dendritic cells induce immunity to transfused red blood cells. J Exp Med (2016) 213(6):887–96. doi:10.1084/jem.20151720
43. Calabro S, Liu D, Gallman A, Nascimento MS, Yu Z, Zhang TT, et al. Differential intrasplenic migration of dendritic cell subsets tailors adaptive immunity. Cell Rep (2016) 16(9):2472–85. doi:10.1016/j.celrep.2016.07.076
44. Barral P, Sanchez-Nino MD, van Rooijen N, Cerundolo V, Batista FD. The location of splenic NKT cells favours their rapid activation by blood-borne antigen. EMBO J (2012) 31(10):2378–90. doi:10.1038/emboj.2012.87
45. King IL, Amiel E, Tighe M, Mohrs K, Veerapen N, Besra G, et al. The mechanism of splenic invariant NKT cell activation dictates localization in vivo. J Immunol (2013) 191(2):572–82. doi:10.4049/jimmunol.1300299
46. MacLennan IC. Germinal centers. Annu Rev Immunol (1994) 12:117–39. doi:10.1146/annurev.iy.12.040194.001001
47. Matsumoto M, Lo SF, Carruthers CJ, Min J, Mariathasan S, Huang G, et al. Affinity maturation without germinal centres in lymphotoxin-alpha-deficient mice. Nature (1996) 382(6590):462–6. doi:10.1038/382462a0
48. Banks TA, Rouse BT, Kerley MK, Blair PJ, Godfrey VL, Kuklin NA, et al. Lymphotoxin-alpha-deficient mice. Effects on secondary lymphoid organ development and humoral immune responsiveness. J Immunol (1995) 155(4):1685–93.
49. Kato J, Motoyama N, Taniuchi I, Takeshita H, Toyoda M, Masuda K, et al. Affinity maturation in Lyn kinase-deficient mice with defective germinal center formation. J Immunol (1998) 160(10):4788–95.
50. Kaji T, Ishige A, Hikida M, Taka J, Hijikata A, Kubo M, et al. Distinct cellular pathways select germline-encoded and somatically mutated antibodies into immunological memory. J Exp Med (2012) 209(11):2079–97. doi:10.1084/jem.20120127
51. Choi YS, Yang JA, Crotty S. Dynamic regulation of Bcl6 in follicular helper CD4 T (Tfh) cells. Curr Opin Immunol (2013) 25(3):366–72. doi:10.1016/j.coi.2013.04.003
52. De Silva NS, Klein U. Dynamics of B cells in germinal centres. Nat Rev Immunol (2015) 15(3):137–48. doi:10.1038/nri3804
53. Semmling V, Lukacs-Kornek V, Thaiss CA, Quast T, Hochheiser K, Panzer U, et al. Alternative cross-priming through CCL17-CCR4-mediated attraction of CTLs toward NKT cell-licensed DCs. Nat Immunol (2010) 11(4):313–20. doi:10.1038/ni.1848
54. Fujii S, Goto A, Shimizu K. Antigen mRNA-transfected, allogeneic fibroblasts loaded with NKT-cell ligand confer antitumor immunity. Blood (2009) 113(18):4262–72. doi:10.1182/blood-2008-08-176446
55. Shimizu K, Mizuno T, Shinga J, Asakura M, Kakimi K, Ishii Y, et al. Vaccination with antigen-transfected, NKT cell ligand-loaded, human cells elicits robust in situ immune responses by dendritic cells. Cancer Res (2013) 73(1):62–73. doi:10.1158/0008-5472.CAN-12-0759
56. Shimizu K, Yamasaki S, Shinga J, Sato Y, Watanabe T, Ohara O, et al. Systemic DC activation modulates the tumor microenvironment and shapes the long-lived tumor-specific memory mediated by CD8+ T cells. Cancer Res (2016) 76(13):3756–66. doi:10.1158/0008-5472.CAN-15-3219
57. Houser K, Subbarao K. Influenza vaccines: challenges and solutions. Cell Host Microbe (2015) 17(3):295–300. doi:10.1016/j.chom.2015.02.012
58. Gerhard W, Mozdzanowska K, Furchner M, Washko G, Maiese K. Role of the B-cell response in recovery of mice from primary influenza virus infection. Immunol Rev (1997) 159:95–103. doi:10.1111/j.1600-065X.1997.tb01009.x
59. Wiley DC, Wilson IA, Skehel JJ. Structural identification of the antibody-binding sites of Hong Kong influenza haemagglutinin and their involvement in antigenic variation. Nature (1981) 289:373. doi:10.1038/289373a0
60. Kamijuku H, Nagata Y, Jiang X, Ichinohe T, Tashiro T, Mori K, et al. Mechanism of NKT cell activation by intranasal coadministration of alpha-galactosylceramide, which can induce cross-protection against influenza viruses. Mucosal Immunol (2008) 1(3):208–18. doi:10.1038/mi.2008.2
61. Ko SY, Ko HJ, Chang WS, Park SH, Kweon MN, Kang CY. α-Galactosylceramide can act as a nasal vaccine adjuvant inducing protective immune responses against viral infection and tumor. J Immunol (2005) 175(5):3309–17. doi:10.4049/jimmunol.175.5.3309
Keywords: NKT, Tfh, NKTfh, dendritic cell, vaccines
Citation: Fujii S, Yamasaki S, Sato Y and Shimizu K (2018) Vaccine Designs Utilizing Invariant NKT-Licensed Antigen-Presenting Cells Provide NKT or T Cell Help for B Cell Responses. Front. Immunol. 9:1267. doi: 10.3389/fimmu.2018.01267
Received: 31 March 2018; Accepted: 22 May 2018;
Published: 04 June 2018
Edited by:
Luc Van Kaer, Vanderbilt University, United StatesReviewed by:
Mark L. Lang, University of Oklahoma Health Sciences Center, United StatesTonya J. Webb, University of Maryland, Baltimore, United States
Copyright: © 2018 Fujii, Yamasaki, Sato and Shimizu. This is an open-access article distributed under the terms of the Creative Commons Attribution License (CC BY). The use, distribution or reproduction in other forums is permitted, provided the original author(s) and the copyright owner are credited and that the original publication in this journal is cited, in accordance with accepted academic practice. No use, distribution or reproduction is permitted which does not comply with these terms.
*Correspondence: Shin-ichiro Fujii, c2hpbi1pY2hpcm8uZnVqaWlAcmlrZW4uanA=