- Life and Medical Sciences (LIMES) Institute, University of Bonn, Bonn, Germany
Dendritic cells have the ability to efficiently present internalized antigens on major histocompatibility complex (MHC) I molecules. This process is termed cross-presentation and is important role in the generation of an immune response against viruses and tumors, after vaccinations or in the induction of immune tolerance. The molecular mechanisms enabling cross-presentation have been topic of intense debate since many years. However, a clear view on these mechanisms remains difficult, partially due to important remaining questions, controversial results and discussions. Here, we give an overview of the current concepts of antigen cross-presentation and focus on a description of the major cross-presentation pathways, the role of retarded antigen degradation for efficient cross-presentation, the dislocation of antigens from endosomal compartment into the cytosol, the reverse transport of proteasome-derived peptides for loading on MHC I and the translocation of the cross-presentation machinery from the ER to endosomes. We try to highlight recent advances, discuss some of the controversial data and point out some of the major open questions in the field.
Introduction
Dendritic cells (DCs) scan the peripheral tissue for antigens. Upon their recognition, antigens are internalized and the DCs activated and migrate toward the draining lymph node, where they can induce an adaptive immune response (1). In order to do so, they need to process the internalized antigens and load antigen-derived peptides on major histocompatibility complex (MHC) molecules. Peptides loaded onto MHC II molecules can be recognized by antigen-specific CD4+ T helper cells. Similarly, peptides loaded on MHC I molecules can be recognized by antigen-specific CD8+ T cells, leading to their proliferation and the activation of their cytotoxic capacities.
The presentation of internalized antigens on MHC I molecules is a process termed cross-presentation. Efficient cross-presentation has been shown to be crucial in, e.g., the induction of an adaptive immune response against tumors and viruses that do not infect DCs directly and in the induction of peripheral tolerance (2–5).
The molecular mechanisms that regulate classical antigen presentation on MHC II molecules and cross-presentation, however, have been shown to be quite divers. For MHC II-restricted presentation, internalized antigens are degraded in endo/lysosomal compartments by proteases such as cathepsins. Newly synthesized MHC II molecules, which are stabilized by binding to the invariant chain (Ii), are transported from the ER toward this compartment, where Ii is degraded by lysosomal proteases, resulting in the binding of only a small peptide fragment (CLIP) to MHC II. Subsequently, CLIP is replaced by antigen-derived peptides by the chaperon HLA-DM (6).
In contrast to MHC II-restricted presentation, the molecular mechanisms regulating cross-presentation are less understood and in part discussed controversially. There seems to be a whole variety of pathways leading to antigen cross-presentation and, despite intensive investigations, the molecular mechanisms and individual contribution of each pathway are rather unclear.
In this review, we try to describe some of the recent advances in cross-presentation, focusing on the major cross-presentation pathways and highlighting some of the controversial observations in the field.
Cross-Presenting DC Subsets
Although many cells are able to present extracellular antigens on MHC I, DCs are considered to be the most prominent and most relevant cross-presenting cells.
In general, DCs are subdivided into conventional DCs (cDCs) and plasmacytoid DCs (pDCs). cDCs are further classified into cDC1 and cDC2 (7). In mice and human, cDC1 are characterized by the expression of the chemokine receptor XCR1 (7–9) and their development relies on the expression of the transcription factors IRF8 and Batf3 (10–12), whereas the development of cDC2 is mainly regulated by IRF4 (7, 13). Additionally, murine cDC1 express either CD8 (in lymphoid tissues) or CD103 (in non-lymphoid tissues), whereas human cDC1 are characterized by the expression of BDCA-3 (CD141) (8, 14–17).
The cDC1 are generally considered to be potent cross-presenting DCs in vivo. Accordingly, in murine lymphoid tissue, soluble and cell-associated OVA are cross-presented by resident CD8+ DCs (18–20), whereas soluble and cell-associated antigens in lung (21, 22), intestine, and skin (23–25) are cross-presented by migratory CD103+ DCs. Further functional properties of cDC1 are the uptake of apoptotic cells via Clec9A/DNGR1 (26–29) and the responsiveness to TLR3 stimulation (30).
cDC1 express high levels of MHC I pathway genes (31), show high intra-endosomal reactive oxygen species (ROS) production and low acidification in endosomes (32, 33), all features of efficient cross-presentation (see below). They express the small GTPase Rac2, which enables the assembly of the NAPDH oxidase complex NADPH oxidase 2 (NOX2), resulting in enhanced ROS production and active alkalization of endosomes (33). Additionally, cDC1 show only marginal expression levels of the C-type lectin Siglec-G, a potent inhibitor of NOX2 (34).
However, the cDC1 are not the only cross-presenting DC population. Many other DC subpopulations, including cDC2, have been shown to cross-present as well (35–39). For human DCs, it even has been demonstrated that BDCA3+ (cDC1s), BDCA1+ (cDC2s), and even pDCs all bear intrinsic capacities to cross-present extracellular antigens (40). The exact role of different cDC1 and cDC2 subpopulations in cross-presentation is, therefore, under debate and, especially since functional data on the physiological role of human DC subsets in cross-presentation is hard to obtain, future experiments will have to shed light on this question.
Although pDCs have been shown to be able to cross-present antigens (41), their role in cross-presentation in vivo is questionable, especially since their depletion did not affect cross-presentation and clearance of viral antigens (42).
Major Pathways of Antigen Cross-Presentation
Intensive research has clearly shown that there are a wide variety of mechanisms by which peptides derived from extracellular antigens can be presented on MHC I molecules. In general, there are two main cross-presentation pathways: the vacuolar pathway and the endosome-to-cytosol pathway (Figure 1). In the vacuolar pathway, antigen processing and loading onto MHC I molecules occurs within the endo/lysosomal compartment. After internalization, antigens are degraded by lysosomal proteases and antigen-derived peptides are loaded onto MHC class I molecules there. The lysosomal protease Cathepsin S has been demonstrated to play a crucial role in antigen degradation for the vacuolar pathway (43). In the endosome-to-cytosol pathway, internalized antigens need to be transported from the endosomal compartment into the cytosol, where they are degraded by the proteasome (44–46). Derived peptides are subsequently transported by the transporter associated with antigen processing (TAP) into the ER or back into the antigen-containing endosomes, where they can be loaded onto MHC class I (44, 45, 47–49). Although substantial evidence points out that some antigens indeed can be cross-presented independent of proteasomal degradation and TAP-mediated peptide transport by the vacuolar pathway (43, 50–53), most cross-presentation studies report of cross-presentation via the endosome-to-cytosol pathway. The dependency of cross-presentation on proteasomal degradation seems logical, since the functional outcome of cross-presentation is the activation of antigen-specific cytotoxic T cells. After migration toward to site of infection, these T cells are fully equipped to kill potential target cells, like virus-infected cells or tumor cells. In order to become functionally active, T cells must recognize the same epitope presented on MHC I by the target cells. Importantly, MHC I-loaded peptides on target cells do not emerge from cross-presentation but rather are the result of direct (classical) MHC I-restricted presentation of endogenous antigens, in which peptides are generated by the proteasome. Since it is hard to assume that for all antigens, the proteasome and lysosomal proteases generate exactly the same epitopes, the dependency of cross-presentation on proteasomal degradation for at least a substantial part of the antigens might circumvent this problem. Accordingly, DCs deficient in the LMP7 subunit of the immunoproteasome are impaired in cross-presentation in vitro and in vivo (46). However, it needs to be mentioned that very few information about the in vivo significance of the vacuolar vs. endosome-to-cytosol pathway is available, pointing out that future experiments are needed to further investigate the relative importance of both pathways in vivo.
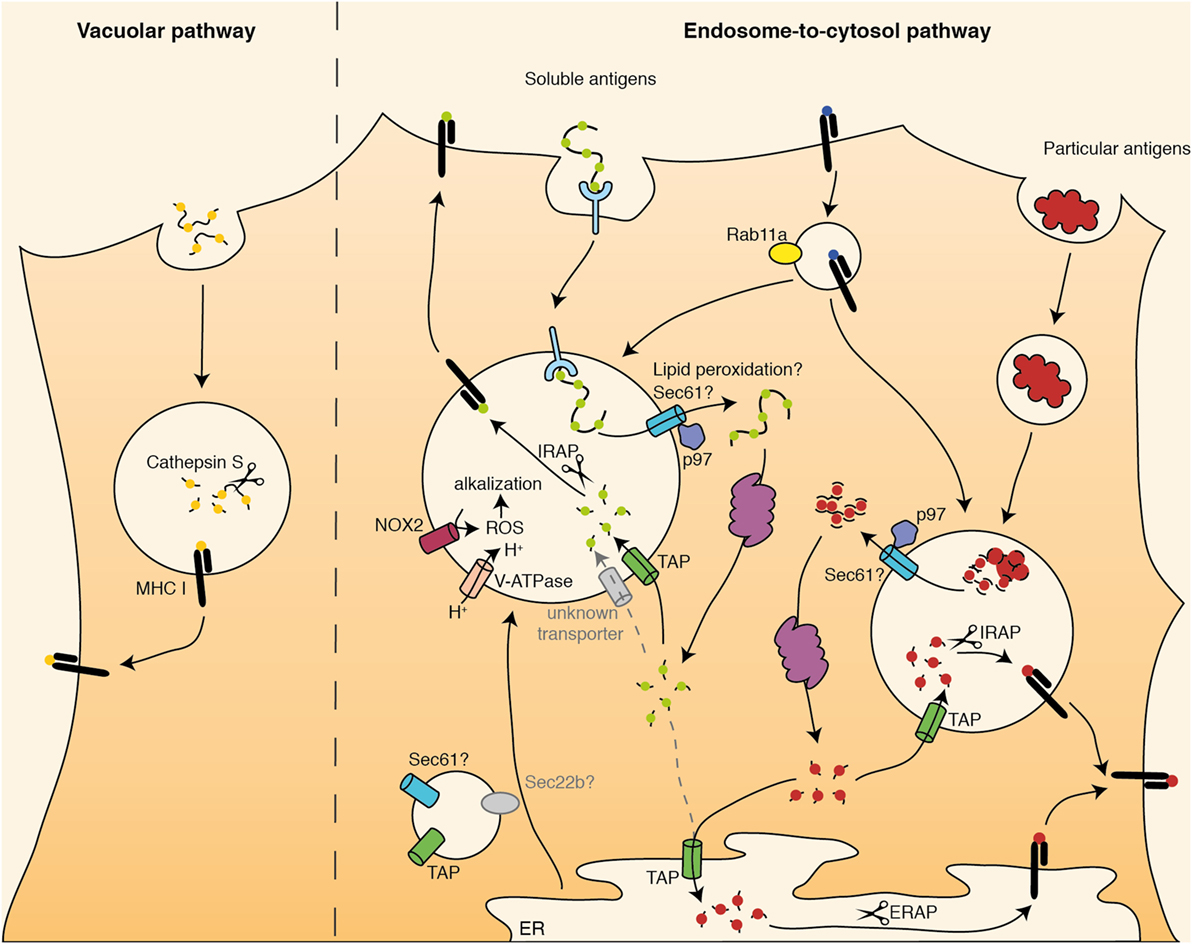
Figure 1. Schematic overview of cross-presentation pathways. Internalized antigens can be presented via the vacuolar pathway or via the endosome-to-cytosol pathway. In the vacuolar pathway, antigens are degraded in endosomes by Cathepsin S and loaded onto major histocompatibility complex (MHC) I there. In the endosome-to-cytosol pathway, antigens are transported into the cytosol for proteasomal degradation. Afterward, antigen-derived peptides are transported back into the endosomes (soluble and particular antigens) or into the ER (particular antigens) via TAP. There, they are trimmed by IRAP (endosomes) or ERAP (ER) and loaded onto MHC I. They cross-presentation machinery might be translocated toward endosomes via Sec22b.
Delayed Antigen Degradation and its Role in Cross-Presentation
Over the last years, it has become clear that intra-endosomal antigen stability critically regulates cross-presentation, which efficiency is negatively affected by rapid lysosomal degradation of internalized antigens (54). Lysosomal maturation and activation of lysosomal proteases is fine-tuned by the transcription factor TFEB, an important regulator of cross-presentation (55).
It is generally assumed that rapid antigen degradation quickly destroys a large amount of epitopes before they can be processed properly and loaded onto MHC I molecules (56, 57). Additionally, peptide-loaded MHC I molecules have a limited life span at the cell membrane (58–60). In order to enable T cell activation after migration toward the draining lymph node, however, prolonged cross-presentation seems to be essential. Therefore, limited antigen degradation in antigen-presenting cells might be a mechanism to generate a kind of intracellular antigen depot, from where continuous antigen processing and presentation might ensure the presence of peptide-loaded MHC I molecules over a longer period of time (61). Such intracellular antigen storage depots have also been shown in human monocytes, which accumulate long-peptide antigens for over 5 days in non-lysosomal compartments, where day are protected from rapid degradation (62).
Since DCs are the most efficient cross-presenting cells, these cells possess several mechanisms by which they can actively prevent rapid lysosomal antigen degradation.
First, it was demonstrated that DCs express lower levels of lysosomal proteases (63) and display a reduced velocity of endosome maturation (64) compared to other immune cells. Expression of asparagine endopeptidase and Cathepsins L, S, D, and B in phagosomes of DCs was clearly reduced compared to macrophages, resulting in impaired phagolysosomal degradation and prolonged antigen stability after internalization by DCs (63). The delivery of lysosomal proteases toward phagosomes was even further reduced after stimulation of DCs with LPS (64).
Second, in DCs, an active alkalization of endosomes prevents pH-dependent activation of lysosomal proteases. During lysosome maturation, protons are transported into the luminal space by the V-ATPase, leading to the activation of pH-dependent lysosomal proteases. Reduced V-ATPase activity in DCs might contribute to prevent a rapid drop in pH after antigen internalization (65). Additionally, DCs seem to have the unique capacity to alkalize their endosomes by the recruitment the NOX2 toward the endosomal membrane (32, 66). There, NOX2 can mediate the generation of ROS, which in turn capture protons to build hydrogen peroxide (Figure 1). Proton trapping by ROS causes an active alkalization, impairing pH-dependent activation of lysosomal proteases, which in turn prevents rapid antigen degradation and stimulates cross-presentation (32). The recruitment of NOX2 toward endosomes is mediated by Rab27a (67).
Third, DCs express endocytosis receptors that specifically target non-degradative endosomal compartments. Previous studies have demonstrated that the endocytosis receptor used to internalize an antigen critically determines its intracellular routing and degradation (68). A previous study from our group demonstrated that antigens internalized by fluid phase pinocytosis or scavenger receptor-mediated endocytosis are rapidly targeted toward lysosomes, where they are efficiently degraded by lysosomal proteases, resulting in poor cross-presentation (68). However, if the same DCs simultaneously internalized the same antigen by the mannose receptor, it was targeted toward a distinct pool of early endosomes, which did not undergo rapid fusion with lysosomes and in which antigens were protected from lysosomal degradation, resulting in efficient cross-presentation of MR-internalized antigens (68). Although the role of the MR in in vivo cross-presentation has been discussed controversially (69, 70), it now is clear that CD103+ DCs in liver and lung use this receptor for cross-presentation of, e.g., viral antigens (71). A correlation between antigen targeting into early endosomes clearly distinct from lysosomes and cross-presentation efficiency has also been confirmed in human DCs. Also in these cells, MR-mediated internalization resulted in its routing into early endosomes, retarded degradation and efficient cross-presentation, whereas uptake by DEC205 lead to antigen targeting into lysosomes, rapid lysosomal degradation, and hence poor cross-presentation (56). Interestingly, attenuating lysosomal degradation was sufficient to rescue the cross-presentation of DEC-205-internalized antigens (56), highlighting again the importance of intra-endosomal antigen stability for efficient cross-presentation. Additionally, the targeted region of the endocytosis receptor might also play a role in antigen degradation and presentation, adding even an additional degree of complexity. Figdor and colleagues demonstrated that antigen targeting toward the carbohydrate recognition domain of DC-SIGN delivers antigens to lysosomal compartments, resulting in rapid degradation and poor cross-presentation, whereas targeting the neck region of DC-SIGN causes antigen delivery in early endosomal compartments clearly distinct from lysosomes, causing prolonged stability and efficient cross-presentation (72, 73).
Antigen Translocation into the Cytosol as Critical Step in Antigen Cross-Presentation
After being internalized into a non-degradative endosomal compartment, antigens need to be processed before they can be loaded onto MHC I. In the endosome-to-cytosol pathway, internalized antigens, therefore, need to be transported across the endosomal membrane into the cytosol for proteasomal degradation. Although this is a key step in antigen cross-presentation and significant efforts have been made to shed light on this process, the underlying mechanisms mediating such intracellular antigen transport are still topic of debate.
In general, if DCs enable access of endosomal antigens to the cytosol, this must be a process, which is controlled very tightly. Uncontrolled lysosome leakage would lead to the cytosolic release of Cathepsins, which in turn would activate the NLRP3 inflammasome (74) and result in pyroptosis, an inflammatory form of cell death (75). To avoid this, total lysosomal content cannot just be released into the cytosol in an uncontrolled fashion. Accordingly, antigens need to be unfolded (76) and disulfide bridges need to be reduced by the γ-interferon-inducible lysosomal thiol reductase GILT (77) before efficient translocation and hence cross-presentation can take place. This supports the idea that antigen translocation is highly regulated, might involve dislocation through a transmembrane pore complex, and is presumably not the result of simple lysosome leakage.
It is generally assumed that members of the ER-associated degradation (ERAD) machinery contribute in enabling antigen dislocation for cross-presentation. First indirect indications for a role of ERAD in this process came from observations describing the presence of ERAD components in the phagosomal membrane (47, 48) and from experiments using the ERAD inhibitor Exotoxin A, which specifically represses cross-presentation (49, 78).
First direct evidence for the involvement of the ERAD machinery came from the Cresswell group, who demonstrated an important role of the AAA ATPase p97 in antigen dislocation (49). Whereas expression of a dominant-negative p97 mutant in DCs represses cross-presentation, the addition of purified wild-type p97 but not the dominant-negative mutant to purified phagosomes enhanced antigen translocation (49, 79–81), indicating that p97 indeed might provide the energy to pull endosomal antigens into the cytosol.
The identification of a dedicated translocon, which actually functions as a transmembrane pore complex to enable antigen dislocation across the endosomal membrane, has been (and still is) by far more difficult. One putative candidate, which has been proposed to mediate antigen dislocation into the cytosol over a decade ago, is the ERAD member Sec61 (49, 78), a trimeric protein whose downregulation has been shown to inhibit antigen translocation and cross-presentation (79, 82). However, since Sec61 plays an important role in the dislocation of proteins at the ER membrane, like, e.g., the dislocation of MHC I molecules themselves (83), it is very hard to distinguish endosome-specific effects of Sec61 from general effects at the ER. In an attempt to solve this problem, we generated Sec61-specific intracellular antibody (intrabody), which we fused to an ER retention signal (84), leading to the trapping of Sec61 in the ER and preventing its transport toward endosomes (82). By this means, we could demonstrate that the transport of Sec61 toward endosomes indeed is essential for antigen dislocation and cross-presentation. Additionally, we could demonstrate that the expression of the intrabody did not alter overall Sec61 expression and did not affected the ERAD-mediated dislocation of MHC I, TCR, CD3δ and the split venus protein at the ER membrane. This points out that ERAD activity at the ER remained unaltered by the expression of the intrabody. These data suggest that Sec61 indeed might serve as a translocon for cross-presentation (Figure 1). However, it cannot be formally excluded that the translation of another pore complex at the ER membrane is changed by manipulating intracellular Sec61 transport or that another putative pore complex is translocated toward endosomes in a complex with Sec61, being influences by intrabody expression. Additionally, Grotzke et al. demonstrated that a chemical inhibitor of Sec61, mycolactone, does not seem to influence antigen dislocation from the cytosol (85). This inhibitor has been shown to directly bind Sec61 and targets proteins that are co-translationally imported in a Sec61-mediated fashion into the ER toward proteasomal degradation (86, 87). However, whether mycolactone could possibly affect Sec61-mediated protein dislocation from the ER into the cytosol is not clear, especially since such proteins are generally ubiquitinated and targeted for proteasomal degradation also in the absence of mycolactone (80). Indeed, mycolactone was shown to have no influence on ERAD (86) and also Grotzke et al. demonstrated that mycolactone does not affect protein retranslocation from the ER into the cytosol. Whether this is due to a missing role of Sec61 in this process or to specific properties of the inhibitor needs to be determined. Especially since addition of Exotoxin A, an inhibitor that blocks Sec61 channel openings (78, 88), clearly affects antigen translocation and cross-presentation (49, 82), there seems to be a need of information on the exact working mechanism of these inhibitors and on the role of Sec61 in dislocation from the ER to finally clear a potential role of Sec61 on cross-presentation.
In addition to antigen dislocation through a pore complex, a recent study postulated that lipid peroxidation in DCs might play a crucial role in antigen transport into the cytoplasm (89). Here, the authors proposed that the specific recruitment of NOX2 might cause lipid peroxidation in endosomes. As mentioned above, NOX2 captures protons to generate hydrogen peroxide, preventing rapid acidification of the endosome. Lipid peroxidation caused by such hydrogen peroxide was suggested to result in leakiness of the endosomal membrane and hence, antigen access into the cytosol and enhanced cross-presentation. However, it remains unclear how the antigen-presenting cell in this case would prevent inflammasome-induced cell death caused by unspecific release of cathepsins. Additionally, the necessity for endosomal antigens to be unfolded (76) and reduced by GILT (77) cannot be explained by simple leackage of the endosomal membrane. Therefore, the significance of such a pathway in cross-presentation in vivo remains to be elucidated.
Transport of Proteasome-Derived Peptides for Loading Onto MHC I
After being transported into the cytosol, internalized antigens are degraded by the proteasome. Subsequently, antigen-derived peptides can be transported through the TAP transporter into the ER or alternatively, by endosomal TAP, back into the endosomal compartments (44, 45, 47, 48, 90). There, peptides are trimmed into a suited size for loading onto MHC I molecules. Such trimming can occur via the peptidases ERAP (in the ER) or IRAP (in endosomes) (Figure 1) (91). Presentation of peptides derived from soluble antigens is mainly ERAP-independent in vitro and in vivo (92), but rather occurs in endosomes after transport by endosomal TAP and IRAP-mediated peptide trimming (90, 92). Proteasome-derived peptides derived from particulate antigens, however, can be transferred into both the ER and endosomes, where they are trimmed by ERAP or IRAP, respectively, and loaded onto MHC I (91). These underlying mechanisms for these differences are unknown.
Recently, it was demonstrated that, in addition to antigen translocation through endosomal TAP, some peptides might enter endosomes in an energy-consuming but TAP-independent fashion (93), pointing out the possibility of additional (unknown) transporters involved in peptide transport into endosomes for cross-presentation. Additionally, since TAP-independency was often used to demonstrate cross-presentation via the vacuolar pathway, there is the possibility that at least in part of these studies, antigens might have entered the endosome via the endosome-to-cytosol pathway, using alternative peptide transporters.
After peptide reimport into the endosomes, they can be loaded onto MHC I molecules. In general, there are two basic possibilities how MHC I molecules can enter the endosome. First, newly synthesized MHC I molecules could be transported from the ER to the endosomes and used for peptide loading in cross-presentation. Second, MHC I from the cell surface (that are already loaded with peptides) could be transported toward endosomes during endocytosis events. The Blander group demonstrated that for particulate antigens, MHC I molecules used for cross-presentation mainly originated from the cell membrane and were translocated into an endosomal recycling compartment in a Rab11a-dependent fashion (Figure 1) (94). From these organelles, MHC I molecules can be transported toward phagosomes, a process that is mediated by the SNARE protein SNAP23 and critically depends on MyD88 signaling (94). It remains unclear, however, whether peptide exchange on recycling MHC I molecules requires the help of additional chaperon proteins (similar to the function of HLA-DM in MHC II-restricted presentation) or can occur after simple weakening of the peptide–MHC I binding in endosomes. Additionally, it has been demonstrated that for cross-presentation of elongated peptides, a substantial part of the used MHC I molecules are newly synthesized molecules recruited from the ER (95). In this case, it needs to be determined whether such MHC I molecules are loaded with peptides in the ER and undergo peptide exchange in acidic endosomes, or whether the transport of the entire peptide loading complex, which stabilizes unbound MHC I molecules and assists in peptide binding, to the endosomes is required for cross-presentation. Despite the presence of several members of the peptide loading machinery in endosomes (45, 47, 48), a functional relevance of these proteins in cross-presentation is missing.
Transport of ER Components to Endosomes
As described above, efficient cross-presentation requires the transport of ER proteins toward endosomes. The exact mechanisms, by which this transfer occurs, are not completely understood and in part contradictory data complicate a clear view on this process.
Since it is known that endosomes during their maturation directly interact with the ER to exchange a wide variety of molecules (96), such ER-endosome membrane contact sites would offer an easy explanation for the transfer of ER proteins toward endosomes. However, it has been proposed by Amigorena and Savina that the transport of cross-presentation components toward endosomes takes place from the ER-golgi intermediate compartment (ERGIC) (97). Membrane fusion between the ERGIC and the phagosomes has been postulated to be mediated by the SNARE proteins Sec22b (in the ERGIC) and syntaxin 4 (in the phagosome). Accordingly, shRNA-mediated downregulation of Sec22b resulted in impaired recruitment of ER proteins toward phagosomes, decreased antigen translocation into the cytosol, and hence reduced cross-presentation (94, 97). These observations support a critical role of the ERAD machinery in antigen dislocation into the cytosol for cross-presentation as described above. However, a recent study by Reddy and colleagues demonstrated that severe off target effects of the used shRNA might have caused the observed influence on cross-presentation (98), questioning the role of Sec22b in cross-presentation. Since such off target effects of shRNA molecules can be circumvented by the generation of Sec22b-deficient mice, one could expect that the use of conditional knockout mice would shed light on the situation and would clearly indicate whether Sec22b is indeed involved in cross-presentation. Mice bearing a conditional knockout of Sec22b in CD11c+ DCs were generated by both the Reddy and the Amigorena group. Strikingly, whereas Reddy et al. reported complete independency of cross-presentation on Sec22b (98), Amigorena et al. showed a clear impairment of cross-presentation in Sec22b-knockout DCs, hence drawing completely opposite conclusions (99). Both groups used partially different in vitro and in vivo systems to substantiate their findings, but since also opposite effects of Sec22b on cross-presentation using the same cells (BM-DCs and splenic DCs) and the same antigens (soluble and bead-bound OVA) were observed, these contractionary results cannot be explained by different experimental setups only (100). Therefore, the exact role of Sec22b and ERGIC-mediated transport of ER proteins needs to be confirmed.
The recruitment of MHC I molecules toward antigen-containing phagosomes was shown to be induced by TLR ligands. TLR-induced and MyD88-dependent signaling resulted in the activation of IKK2, which phosphorylates SNAP23, mediating fusion events between phagosomes and MHC I-containing recycling endosomes (94). Also the transport of other ER proteins toward endosomes has been shown to be stimulated by TLR ligands (82, 90). Using flow cytometric analysis of individual endosomes (101), have demonstrated before that low amounts of Sec61 are present in endosomes also in the absence of TLR ligands, and that a clear recruitment of Sec61 toward antigen-containing endosomes was induced by LPS (82). Since it is very unlikely that Sec61 is also recruited via recycling endosomes, distinct mechanisms might come into play for the transport of these molecules.
One of these mechanisms might rely on the uncoordinated 93 homolog B1 (UNC93B1), which is activated by TLR triggering and mediates the transport of TLRs from the ER toward endosomes (102–104). Interestingly, UNC91B1 has been demonstrated to be critically involved in cross-presentation (105). Although a putative role of UNC93B has also been discussed controversially (106), it now becomes clear that an essential role of UNC93B1 in cross-presentation is based on its interaction with the store-operated-Ca2+-entry regulator STIM1. UNC93B1 has been shown to be essential for oligomerization of hence activation of STIM1, which in turn alters local Calcium signaling regulating phago/endosome fusion events (107, 108). Ablation of UNC93B1 impairs antigen translocation into the cytosol and cross-presentation (107). Interestingly, antigen dislocation into the cytosol was impaired despite reduced endosomal antigen degradation, which is generally assumed to increase antigen export from the endosomes. Since UNC93B1 upon TLR stimulation mediates TLR transport from the ER toward endosomes, it, therefore, is thinkable that ER members of the cross-presentation machinery are transported from the ER toward endosomes in a similar UNC93B1-dependent fashion.
Additionally, DC activation by TLR ligands can have other effects on the cross-presentation machinery independent of ER to endosome transport, like the prevention of phagosome fusion with lysosomes and concomitant antigen stabilization (57) or increases in antigen internalization (109).
Alternative Cross-Presentation Pathways
In all cross-presentation pathways described above, cross-presented antigens entered the DC via endocytosis. However, there are some reports indicating that also distinct mechanisms can lead to cross-presentation.
One of these mechanisms is the transport of pre-processed antigens (peptides) from a donor cell to a DC. Such transport can occur via direct cell–cell contact, mediated by gap junctions (110, 111). After gap junction-mediated transport from one cell to another, antigen-derived peptides can enter the normal MHC I presentation pathway. Interestingly, the donor cell does not need to be an antigen-presenting cell, offering the possibility that DCs can obtain such peptides directly from infected cells. Infection of melanoma cells with Salmonella has been demonstrated to increase the expression of Connexin 43, an important gap junction protein, enabling efficient gap junction-mediated peptide transfer from the infected cell to the DC and hence efficient cross-presentation (112). However, given the limited stability of intracellular peptides (113), the physiological significance of such peptide transfer in cross-presentation remains unclear.
Another alternative cross-presentation pathway is termed cross-dressing, which generally implies that the cross-presenting DC becomes an MHC I molecule, which has already been loaded with an antigen-derived peptide, transferred from a donor cell (114, 115). Similar to gap junction-mediated peptide transfer, such donor cell does not necessarily need to be an antigen-presenting cell, suggesting that DCs can derive peptide-loaded MHC I molecules directly from infected cells or even apoptotic cells. The transfer of loaded MHC I molecules is thought to be mediated by cell–cell contact rather than secretory vesicles (114, 116) and overcomes the need of intracellular antigen processing within the DC. Cross-dressing has been shown to occur in vivo (114, 116) and cross-dressed DCs have been shown to activate memory T cells after viral infection (116). Remarkably, in this study, the activation of naive T cells did not depend on cross-dressing (116), offering the possibility that different cross-presentation pathways might be responsible for the activation of different T cell populations or for T cell activation under specific conditions. However, the exact physiological relevance of cross-dressing and especially its contribution compared to the other cross-presentation pathways in specific situations, however, remains to be elucidated.
Conclusion
Despite intensive research over the last decades, several questions regarding the molecular mechanisms of cross-presentation remain unsolved. How are antigens translocated into the cytosol? How are ER components recruited toward endosomes? What is the role of Sec22b and TLR ligands in this process? And probably most important: which of all these proposed mechanisms holds true in vivo? Are different cross-presentation pathways used in vivo by distinct cell types or antigens (e.g., particulate vs. soluble), or under different physiological conditions? Without any doubt, the elucidation of the molecular mechanisms underlying cross-presentation in vivo bears a high intrinsic potential to optimize various vaccination strategies. Therefore, future investigations will be required to shed more light into the exact pathways of cross-presentation and to solve remaining controversies. The publication of clearly contradicting data might suggest the need for common protocols to perform cross-presentation experiments, in particular in regard to cell culture procedures to generate the often used BM-DCs.
Author Contributions
ME and SB designed the article and wrote the manuscript.
Conflict of Interest Statement
The authors declare that the research was conducted in the absence of any commercial or financial relationships that could be construed as a potential conflict of interest.
Funding
This work was supported by the German Research Foundation consortium SFB704 project A24.
References
1. Reis e Sousa C. Toll-like receptors and dendritic cells: for whom the bug tolls. Semin Immunol (2004) 16:27–34. doi:10.1016/j.smim.2003.10.004
2. Huang AY, Golumbek P, Ahmadzadeh M, Jaffee E, Pardoll D, Levitsky H. Role of bone marrow-derived cells in presenting MHC class I-restricted tumor antigens. Science (1994) 264:961–5. doi:10.1126/science.7513904
3. Sigal LJ, Crotty S, Andino R, Rock KL. Cytotoxic T-cell immunity to virus-infected non-haematopoietic cells requires presentation of exogenous antigen. Nature (1999) 398:77–80. doi:10.1038/18038
4. den Haan JM, Bevan MJ. Antigen presentation to CD8+ T cells: cross-priming in infectious diseases. Curr Opin Immunol (2001) 13:437–41. doi:10.1016/S0952-7915(00)00238-7
5. Heath WR, Carbone FR. Cross-presentation in viral immunity and self-tolerance. Nat Rev Immunol (2001) 1:126–34. doi:10.1038/35100512
6. Mellins ED, Stern LJ. HLA-DM and HLA-DO, key regulators of MHC-II processing and presentation. Curr Opin Immunol (2014) 26:115–22. doi:10.1016/j.coi.2013.11.005
7. Guilliams M, Ginhoux F, Jakubzick C, Naik SH, Onai N, Schraml BU, et al. Dendritic cells, monocytes and macrophages: a unified nomenclature based on ontogeny. Nat Rev Immunol (2014) 14:571–8. doi:10.1038/nri3712
8. Crozat K, Guiton R, Contreras V, Feuillet V, Dutertre C-A, Ventre E, et al. The XC chemokine receptor 1 is a conserved selective marker of mammalian cells homologous to mouse CD8alpha+ dendritic cells. J Exp Med (2010) 207:1283–92. doi:10.1084/jem.20100223
9. Dalod M, Chelbi R, Malissen B, Lawrence T. Dendritic cell maturation: functional specialization through signaling specificity and transcriptional programming. EMBO J (2014) 33:1104–16. doi:10.1002/embj.201488027
10. Aliberti J, Schulz O, Pennington DJ, Tsujimura H, Reis e Sousa C, Ozato K, et al. Essential role for ICSBP in the in vivo development of murine CD8alpha + dendritic cells. Blood (2003) 101:305–10. doi:10.1182/blood-2002-04-1088
11. Edelson BT, KC W, Juang R, Kohyama M, Benoit LA, Klekotka PA, et al. Peripheral CD103+ dendritic cells form a unified subset developmentally related to CD8alpha+ conventional dendritic cells. J Exp Med (2010) 207:823–36. doi:10.1084/jem.20091627
12. Merad M, Sathe P, Helft J, Miller J, Mortha A. The dendritic cell lineage: ontogeny and function of dendritic cells and their subsets in the steady state and the inflamed setting. Annu Rev Immunol (2013) 31:563–604. doi:10.1146/annurev-immunol-020711-074950
13. Schlitzer A, McGovern N, Teo P, Zelante T, Atarashi K, Low D, et al. IRF4 transcription factor-dependent CD11b+ dendritic cells in human and mouse control mucosal IL-17 cytokine responses. Immunity (2013) 38:970–83. doi:10.1016/j.immuni.2013.04.011
14. Bachem A, Güttler S, Hartung E, Ebstein F, Schaefer M, Tannert A, et al. Superior antigen cross-presentation and XCR1 expression define human CD11c+CD141+ cells as homologues of mouse CD8+ dendritic cells. J Exp Med (2010) 207:1273–81. doi:10.1084/jem.20100348
15. Jongbloed SL, Kassianos AJ, McDonald KJ, Clark GJ, Ju X, Angel CE, et al. Human CD141+ (BDCA-3)+ dendritic cells (DCs) represent a unique myeloid DC subset that cross-presents necrotic cell antigens. J Exp Med (2010) 207:1247–60. doi:10.1084/jem.20092140
16. Poulin LF, Salio M, Griessinger E, Anjos-Afonso F, Craciun L, Chen J-L, et al. Characterization of human DNGR-1+ BDCA3+ leukocytes as putative equivalents of mouse CD8alpha+ dendritic cells. J Exp Med (2010) 207:1261–71. doi:10.1084/jem.20092618
17. Haniffa M, Shin A, Bigley V, McGovern N, Teo P, See P, et al. Human tissues contain CD141hi cross-presenting dendritic cells with functional homology to mouse CD103+ nonlymphoid dendritic cells. Immunity (2012) 37:60–73. doi:10.1016/j.immuni.2012.04.012
18. den Haan JM, Lehar SM, Bevan MJ. CD8(+) but not CD8(-) dendritic cells cross-prime cytotoxic T cells in vivo. J Exp Med (2000) 192:1685–96. doi:10.1084/jem.192.12.1685
19. Pooley JL, Heath WR, Shortman K. Cutting edge: intravenous soluble antigen is presented to CD4 T cells by CD8- dendritic cells, but cross-presented to CD8 T cells by CD8+ dendritic cells. J Immunol (2001) 166:5327–30. doi:10.4049/jimmunol.166.9.5327
20. Busche A, Jirmo AC, Welten SPM, Zischke J, Noack J, Constabel H, et al. Priming of CD8+ T cells against cytomegalovirus-encoded antigens is dominated by cross-presentation. J Immunol (2013) 190:2767–77. doi:10.4049/jimmunol.1200966
21. Becker M, Güttler S, Bachem A, Hartung E, Mora A, Jäkel A, et al. Ontogenic, phenotypic, and functional characterization of XCR1(+) dendritic cells leads to a consistent classification of intestinal dendritic cells based on the expression of XCR1 and SIRPα. Front Immunol (2014) 5:326. doi:10.3389/fimmu.2014.00326
22. Cerovic V, Houston SA, Westlund J, Utriainen L, Davison ES, Scott CL, et al. Lymph-borne CD8α+ dendritic cells are uniquely able to cross-prime CD8+ T cells with antigen acquired from intestinal epithelial cells. Mucosal Immunol (2015) 8:38–48. doi:10.1038/mi.2014.40
23. Bedoui S, Whitney PG, Waithman J, Eidsmo L, Wakim L, Caminschi I, et al. Cross-presentation of viral and self antigens by skin-derived CD103+ dendritic cells. Nat Immunol (2009) 10:488–95. doi:10.1038/ni.1724
24. Henry CJ, Grayson JM, Brzoza-Lewis KL, Mitchell LM, Westcott MM, Cook AS, et al. The roles of IL-12 and IL-23 in CD8+ T cell-mediated immunity against Listeria monocytogenes: insights from a DC vaccination model. Cell Immunol (2010) 264:23–31. doi:10.1016/j.cellimm.2010.04.007
25. Kitano M, Yamazaki C, Takumi A, Ikeno T, Hemmi H, Takahashi N, et al. Imaging of the cross-presenting dendritic cell subsets in the skin-draining lymph node. Proc Natl Acad Sci U S A (2016) 113:1044–9. doi:10.1073/pnas.1513607113
26. Caminschi I, Proietto AI, Ahmet F, Kitsoulis S, Shin Teh J, Lo JCY, et al. The dendritic cell subtype-restricted C-type lectin Clec9A is a target for vaccine enhancement. Blood (2008) 112:3264–73. doi:10.1182/blood-2008-05-155176
27. Huysamen C, Willment JA, Dennehy KM, Brown GD. CLEC9A is a novel activation C-type lectin-like receptor expressed on BDCA3+ dendritic cells and a subset of monocytes. J Biol Chem (2008) 283:16693–701. doi:10.1074/jbc.M709923200
28. Sancho D, Joffre OP, Keller AM, Rogers NC, Martínez D, Hernanz-Falcón P, et al. Identification of a dendritic cell receptor that couples sensing of necrosis to immunity. Nature (2009) 458:899–903. doi:10.1038/nature07750
29. Hanč P, Fujii T, Iborra S, Yamada Y, Huotari J, Schulz O, et al. Structure of the complex of F-actin and DNGR-1, a C-Type lectin receptor involved in dendritic cell cross-presentation of dead cell-associated antigens. Immunity (2015) 42:839–49. doi:10.1016/j.immuni.2015.04.009
30. Jelinek I, Leonard JN, Price GE, Brown KN, Meyer-Manlapat A, Goldsmith PK, et al. TLR3-specific double-stranded RNA oligonucleotide adjuvants induce dendritic cell cross-presentation, CTL responses, and antiviral protection. J Immunol (2011) 186:2422–9. doi:10.4049/jimmunol.1002845
31. Dudziak D, Kamphorst AO, Heidkamp GF, Buchholz VR, Trumpfheller C, Yamazaki S, et al. Differential antigen processing by dendritic cell subsets in vivo. Science (2007) 315:107–11. doi:10.1126/science.1136080
32. Savina A, Jancic C, Hugues S, Guermonprez P, Vargas P, Moura IC, et al. NOX2 controls phagosomal pH to regulate antigen processing during crosspresentation by dendritic cells. Cell (2006) 126:205–18. doi:10.1016/j.cell.2006.05.035
33. Savina A, Peres A, Cebrian I, Carmo N, Moita C, Hacohen N, et al. The small GTPase Rac2 controls phagosomal alkalinization and antigen crosspresentation selectively in CD8(+) dendritic cells. Immunity (2009) 30:544–55. doi:10.1016/j.immuni.2009.01.013
34. Ding Y, Guo Z, Liu Y, Li X, Zhang Q, Xu X, et al. The lectin Siglec-G inhibits dendritic cell cross-presentation by impairing MHC class I-peptide complex formation. Nat Immunol (2016) 17:1167–75. doi:10.1038/ni.3535
35. Sheng J, Chen Q, Soncin I, Ng SL, Karjalainen K, Ruedl C. A discrete subset of monocyte-derived cells among typical conventional type 2 dendritic cells can efficiently cross-present. Cell Rep (2017) 21:1203–14. doi:10.1016/j.celrep.2017.10.024
36. Ji Q, Castelli L, Goverman JM. MHC class I-restricted myelin epitopes are cross-presented by Tip-DCs that promote determinant spreading to CD8? T cells. Nat Immunol (2013) 14:254–61. doi:10.1038/ni.2513
37. Ballesteros-Tato A, León B, Lund FE, Randall TD. Temporal changes in dendritic cell subsets, cross-priming and costimulation via CD70 control CD8(+) T cell responses to influenza. Nat Immunol (2010) 11:216–24. doi:10.1038/ni.1838
38. Backer R, van Leeuwen F, Kraal G, den Haan JM. CD8- dendritic cells preferentially cross-present Saccharomyces cerevisiae antigens. Eur J Immunol (2008) 38:370–80. doi:10.1002/eji.200737647
39. Mouriès J, Moron G, Schlecht G, Escriou N, Dadaglio G, Leclerc C. Plasmacytoid dendritic cells efficiently cross-prime naive T cells in vivo after TLR activation. Blood (2008) 112:3713–22. doi:10.1182/blood-2008-03-146290
40. Segura E, Durand M, Amigorena S. Similar antigen cross-presentation capacity and phagocytic functions in all freshly isolated human lymphoid organ-resident dendritic cells. J Exp Med (2013) 210:1035–47. doi:10.1084/jem.20121103
41. Di Pucchio T, Chatterjee B, Smed-Sörensen A, Clayton S, Palazzo A, Montes M, et al. Direct proteasome-independent cross-presentation of viral antigen by plasmacytoid dendritic cells on major histocompatibility complex class I. Nat Immunol (2008) 9:551–7. doi:10.1038/ni.1602
42. GeurtsvanKessel CH, Willart MAM, van Rijt LS, Muskens F, Kool M, Baas C, et al. Clearance of influenza virus from the lung depends on migratory langerin+CD11b- but not plasmacytoid dendritic cells. J Exp Med (2008) 205:1621–34. doi:10.1084/jem.20071365
43. Shen L, Sigal LJ, Boes M, Rock KL. Important role of cathepsin S in generating peptides for TAP-independent MHC class I crosspresentation in vivo. Immunity (2004) 21:155–65. doi:10.1016/j.immuni.2004.07.004
44. Kovacsovics-Bankowski M, Rock KL. A phagosome-to-cytosol pathway for exogenous antigens presented on MHC class I molecules. Science (1995) 267:243–6. doi:10.1126/science.7809629
45. Ackerman AL, Kyritsis C, Tampé R, Cresswell P. Early phagosomes in dendritic cells form a cellular compartment sufficient for cross presentation of exogenous antigens. Proc Natl Acad Sci U S A (2003) 100:12889–94. doi:10.1073/pnas.1735556100
46. Palmowski MJ, Gileadi U, Salio M, Gallimore A, Millrain M, James E, et al. Role of immunoproteasomes in cross-presentation. J Immunol (2006) 177:983–90. doi:10.4049/jimmunol.177.2.983
47. Guermonprez P, Saveanu L, Kleijmeer M, Davoust J, van Endert P, Amigorena S. ER-phagosome fusion defines an MHC class I cross-presentation compartment in dendritic cells. Nature (2003) 425:397–402. doi:10.1038/nature01911
48. Houde M, Bertholet S, Gagnon E, Brunet S, Goyette G, Laplante A, et al. Phagosomes are competent organelles for antigen cross-presentation. Nature (2003) 425:402–6. doi:10.1038/nature01912
49. Ackerman AL, Giodini A, Cresswell P. A role for the endoplasmic reticulum protein retrotranslocation machinery during crosspresentation by dendritic cells. Immunity (2006) 25:607–17. doi:10.1016/j.immuni.2006.08.017
50. Pfeifer JD, Wick MJ, Roberts RL, Findlay K, Normark SJ, Harding CV. Phagocytic processing of bacterial antigens for class I MHC presentation to T cells. Nature (1993) 361:359–62. doi:10.1038/361359a0
51. Liu T, Chambers B, Diehl AD, van Kaer L, Jondal M, Ljunggren HG. TAP peptide transporter-independent presentation of heat-killed Sendai virus antigen on MHC class I molecules by splenic antigen-presenting cells. J Immunol (1997) 159:5364–71.
52. Campbell DJ, Serwold T, Shastri N. Bacterial proteins can be processed by macrophages in a transporter associated with antigen processing-independent, cysteine protease-dependent manner for presentation by MHC class I molecules. J Immunol (2000) 164:168–75. doi:10.4049/jimmunol.164.1.168
53. Bertholet S, Goldszmid R, Morrot A, Debrabant A, Afrin F, Collazo-Custodio C, et al. Leishmania antigens are presented to CD8+ T cells by a transporter associated with antigen processing-independent pathway in vitro and in vivo. J Immunol (2006) 177:3525–33. doi:10.4049/jimmunol.177.6.3525
54. Accapezzato D, Visco V, Francavilla V, Molette C, Donato T, Paroli M, et al. Chloroquine enhances human CD8+ T cell responses against soluble antigens in vivo. J Exp Med (2005) 202:817–28. doi:10.1084/jem.20051106
55. Samie M, Cresswell P. The transcription factor TFEB acts as a molecular switch that regulates exogenous antigen-presentation pathways. Nat Immunol (2015) 16:729–36. doi:10.1038/ni.3196
56. Chatterjee B, Smed-Sörensen A, Cohn L, Chalouni C, Vandlen R, Lee B-C, et al. Internalization and endosomal degradation of receptor-bound antigens regulate the efficiency of cross presentation by human dendritic cells. Blood (2012) 120:2011–20. doi:10.1182/blood-2012-01-402370
57. Alloatti A, Kotsias F, Pauwels A-M, Carpier J-M, Jouve M, Timmerman E, et al. Toll-like receptor 4 engagement on dendritic cells restrains phago-lysosome fusion and promotes cross-presentation of antigens. Immunity (2015) 43:1087–100. doi:10.1016/j.immuni.2015.11.006
58. Eberl G, Widmann C, Corradin G. The functional half-life of H-2Kd-restricted T cell epitopes on living cells. Eur J Immunol (1996) 26:1993–9. doi:10.1002/eji.1830260904
59. Cella M, Engering A, Pinet V, Pieters J, Lanzavecchia A. Inflammatory stimuli induce accumulation of MHC class II complexes on dendritic cells. Nature (1997) 388:782–7. doi:10.1038/42030
60. Rescigno M, Citterio S, Thèry C, Rittig M, Medaglini D, Pozzi G, et al. Bacteria-induced neo-biosynthesis, stabilization, and surface expression of functional class I molecules in mouse dendritic cells. Proc Natl Acad Sci U S A (1998) 95:5229–34. doi:10.1073/pnas.95.9.5229
61. van Montfoort N, Camps MG, Khan S, Filippov DV, Weterings JJ, Griffith JM, et al. Antigen storage compartments in mature dendritic cells facilitate prolonged cytotoxic T lymphocyte cross-priming capacity. Proc Natl Acad Sci U S A (2009) 106:6730–5. doi:10.1073/pnas.0900969106
62. Faure F, Jouve M, Lebhar-Pequillet I, Sadaka C, Sepulveda F, Lantz O, et al. Blood monocytes sample MelanA/MART1 antigen for long-lasting cross-presentation to CD8+ T cells after differentiation into dendritic cells. Int J Cancer (2018) 142:133–44. doi:10.1002/ijc.31037
63. Delamarre L, Pack M, Chang H, Mellman I, Trombetta ES. Differential lysosomal proteolysis in antigen-presenting cells determines antigen fate. Science (2005) 307:1630–4. doi:10.1126/science.1108003
64. Lennon-Duménil A-M, Bakker AH, Maehr R, Fiebiger E, Overkleeft HS, Rosemblatt M, et al. Analysis of protease activity in live antigen-presenting cells shows regulation of the phagosomal proteolytic contents during dendritic cell activation. J Exp Med (2002) 196:529–40. doi:10.1084/jem.20020327
65. Trombetta ES, Ebersold M, Garrett W, Pypaert M, Mellman I. Activation of lysosomal function during dendritic cell maturation. Science (2003) 299:1400–3. doi:10.1126/science.1080106
66. Mantegazza AR, Savina A, Vermeulen M, Pérez L, Geffner J, Hermine O, et al. NADPH oxidase controls phagosomal pH and antigen cross-presentation in human dendritic cells. Blood (2008) 112:4712–22. doi:10.1182/blood-2008-01-134791
67. Jancic C, Savina A, Wasmeier C, Tolmachova T, El-Benna J, Dang PM-C, et al. Rab27a regulates phagosomal pH and NADPH oxidase recruitment to dendritic cell phagosomes. Nat Cell Biol (2007) 9:367–78. doi:10.1038/ncb1552
68. Burgdorf S, Kautz A, Böhnert V, Knolle PA, Kurts C. Distinct pathways of antigen uptake and intracellular routing in CD4 and CD8 T cell activation. Science (2007) 316:612–6. doi:10.1126/science.1137971
69. Segura E, Albiston AL, Wicks IP, Chai SY, Villadangos JA. Different cross-presentation pathways in steady-state and inflammatory dendritic cells. Proc Natl Acad Sci U S A (2009) 106:20377–81. doi:10.1073/pnas.0910295106
70. Burgdorf S, Schuette V, Semmling V, Hochheiser K, Lukacs-Kornek V, Knolle PA, et al. Steady-state cross-presentation of OVA is mannose receptor-dependent but inhibitable by collagen fragments. Proc Natl Acad Sci U S A (2010) 107:E48–9. doi:10.1073/pnas.1000598107
71. Schuette V, Embgenbroich M, Ulas T, Welz M, Schulte-Schrepping J, Draffehn AM, et al. Mannose receptor induces T-cell tolerance via inhibition of CD45 and up-regulation of CTLA-4. Proc Natl Acad Sci U S A (2016) 113:10649–54. doi:10.1073/pnas.1605885113
72. Tacken PJ, de Vries I, Jolanda M, Gijzen K, Joosten B, Wu D, et al. Effective induction of naive and recall T-cell responses by targeting antigen to human dendritic cells via a humanized anti-DC-SIGN antibody. Blood (2005) 106:1278–85. doi:10.1182/blood-2005-01-0318
73. Tacken PJ, Ginter W, Berod L, Cruz LJ, Joosten B, Sparwasser T, et al. Targeting DC-SIGN via its neck region leads to prolonged antigen residence in early endosomes, delayed lysosomal degradation, and cross-presentation. Blood (2011) 118:4111–9. doi:10.1182/blood-2011-04-346957
74. Hornung V, Bauernfeind F, Halle A, Samstad EO, Kono H, Rock KL, et al. Silica crystals and aluminum salts activate the NALP3 inflammasome through phagosomal destabilization. Nat Immunol (2008) 9:847–56. doi:10.1038/ni.1631
75. Próchnicki T, Mangan MS, Latz E. Recent insights into the molecular mechanisms of the NLRP3 inflammasome activation. F1000Res (2016) 5:1–15. doi:10.12688/f1000research.8614.1
76. Giodini A, Cresswell P. Hsp90-mediated cytosolic refolding of exogenous proteins internalized by dendritic cells. EMBO J (2008) 27:201–11. doi:10.1038/sj.emboj.7601941
77. Singh R, Cresswell P. Defective cross-presentation of viral antigens in GILT-free mice. Science (2010) 328:1394–8. doi:10.1126/science.1189176
78. Koopmann JO, Albring J, Hüter E, Bulbuc N, Spee P, Neefjes J, et al. Export of antigenic peptides from the endoplasmic reticulum intersects with retrograde protein translocation through the Sec61p channel. Immunity (2000) 13:117–27. doi:10.1016/S1074-7613(00)00013-3
79. Imai J, Hasegawa H, Maruya M, Koyasu S, Yahara I. Exogenous antigens are processed through the endoplasmic reticulum-associated degradation (ERAD) in cross-presentation by dendritic cells. Int Immunol (2005) 17:45–53. doi:10.1093/intimm/dxh184
80. Zehner M, Chasan AI, Schuette V, Embgenbroich M, Quast T, Kolanus W, et al. Mannose receptor polyubiquitination regulates endosomal recruitment of p97 and cytosolic antigen translocation for cross-presentation. Proc Natl Acad Sci U S A (2011) 108:9933–8. doi:10.1073/pnas.1102397108
81. Ménager J, Ebstein F, Oger R, Hulin P, Nedellec S, Duverger E, et al. Cross-presentation of synthetic long peptides by human dendritic cells: a process dependent on ERAD component p97/VCP but Not sec61 and/or Derlin-1. PLoS One (2014) 9:e89897. doi:10.1371/journal.pone.0089897
82. Zehner M, Marschall AL, Bos E, Schloetel J-G, Kreer C, Fehrenschild D, et al. The translocon protein Sec61 mediates antigen transport from endosomes in the cytosol for cross-presentation to CD8(+) T cells. Immunity (2015) 42:850–63. doi:10.1016/j.immuni.2015.04.008
83. Wiertz EJ, Tortorella D, Bogyo M, Yu J, Mothes W, Jones TR, et al. Sec61-mediated transfer of a membrane protein from the endoplasmic reticulum to the proteasome for destruction. Nature (1996) 384:432–8. doi:10.1038/384432a0
84. Meli G, Lecci A, Manca A, Krako N, Albertini V, Benussi L, et al. Conformational targeting of intracellular Aβ oligomers demonstrates their pathological oligomerization inside the endoplasmic reticulum. Nat Commun (2014) 5:3867. doi:10.1038/ncomms4867
85. Grotzke JE, Kozik P, Morel J-D, Impens F, Pietrosemoli N, Cresswell P, et al. Sec61 blockade by mycolactone inhibits antigen cross-presentation independently of endosome-to-cytosol export. Proc Natl Acad Sci U S A (2017) 114:E5910–9. doi:10.1073/pnas.1705242114
86. Hall BS, Hill K, McKenna M, Ogbechi J, High S, Willis AE, et al. The pathogenic mechanism of the Mycobacterium ulcerans virulence factor, mycolactone, depends on blockade of protein translocation into the ER. PLoS Pathog (2014) 10:e1004061. doi:10.1371/journal.ppat.1004061
87. McKenna M, Simmonds RE, High S. Mechanistic insights into the inhibition of Sec61-dependent co- and post-translational translocation by mycolactone. J Cell Sci (2016) 129:1404–15. doi:10.1242/jcs.182352
88. Wirth A, Jung M, Bies C, Frien M, Tyedmers J, Zimmermann R, et al. The Sec61p complex is a dynamic precursor activated channel. Mol Cell (2003) 12:261–8. doi:10.1016/S1097-2765(03)00283-1
89. Dingjan I, Verboogen DR, Paardekooper LM, Revelo NH, Sittig SP, Visser LJ, et al. Lipid peroxidation causes endosomal antigen release for cross-presentation. Sci Rep (2016) 6:22064. doi:10.1038/srep22064
90. Burgdorf S, Schölz C, Kautz A, Tampé R, Kurts C. Spatial and mechanistic separation of cross-presentation and endogenous antigen presentation. Nat Immunol (2008) 9:558–66. doi:10.1038/ni.1601
91. Saveanu L, Carroll O, Weimershaus M, Guermonprez P, Firat E, Lindo V, et al. IRAP identifies an endosomal compartment required for MHC class I cross-presentation. Science (2009) 325:213–7. doi:10.1126/science.1172845
92. Firat E, Saveanu L, Aichele P, Staeheli P, Huai J, Gaedicke S, et al. The role of endoplasmic reticulum-associated aminopeptidase 1 in immunity to infection and in cross-presentation. J Immunol (2007) 178:2241–8. doi:10.4049/jimmunol.178.4.2241
93. Lawand M, Abramova A, Manceau V, Springer S, van Endert P. TAP-dependent and -independent peptide import into dendritic cell phagosomes. J Immunol (2016) 197:3454–63. doi:10.4049/jimmunol.1501925
94. Nair-Gupta P, Baccarini A, Tung N, Seyffer F, Florey O, Huang Y, et al. TLR signals induce phagosomal MHC-I delivery from the endosomal recycling compartment to allow cross-presentation. Cell (2014) 158:506–21. doi:10.1016/j.cell.2014.04.054
95. Ma W, Zhang Y, Vigneron N, Stroobant V, Thielemans K, van der Bruggen P, et al. Long-peptide cross-presentation by human dendritic cells occurs in vacuoles by peptide exchange on nascent MHC class I molecules. J Immunol (2016) 196:1711–20. doi:10.4049/jimmunol.1501574
96. Eden ER. The formation and function of ER-endosome membrane contact sites. Biochim Biophys Acta (2016) 1861:874–9. doi:10.1016/j.bbalip.2016.01.020
97. Cebrian I, Visentin G, Blanchard N, Jouve M, Bobard A, Moita C, et al. Sec22b regulates phagosomal maturation and antigen crosspresentation by dendritic cells. Cell (2011) 147:1355–68. doi:10.1016/j.cell.2011.11.021
98. Wu SJ, Niknafs YS, Kim SH, Oravecz-Wilson K, Zajac C, Toubai T, et al. A critical analysis of the role of SNARE protein SEC22B in antigen cross-presentation. Cell Rep (2017) 19:2645–56. doi:10.1016/j.celrep.2017.06.013
99. Alloatti A, Rookhuizen DC, Joannas L, Carpier J-M, Iborra S, Magalhaes JG, et al. Critical role for Sec22b-dependent antigen cross-presentation in antitumor immunity. J Exp Med (2017) 214:2231–41. doi:10.1084/jem.20170229
100. Montealegre S, van Endert P. MHC class I cross-presentation: stage lights on Sec22b. Trends Immunol (2017) 38:618–21. doi:10.1016/j.it.2017.07.002
101. Zehner M, Rauen J, Chasan AI, Embgenbroich M, Camps MG, Kaden S, et al. Intraendosomal flow cytometry: a novel approach to analyze the protein composition of antigen-loaded endosomes. Eur J Immunol (2012) 42:2187–90. doi:10.1002/eji.201142089
102. Latz E, Schoenemeyer A, Visintin A, Fitzgerald KA, Monks BG, Knetter CF, et al. TLR9 signals after translocating from the ER to CpG DNA in the lysosome. Nat Immunol (2004) 5:190–8. doi:10.1038/ni1028
103. Kagan JC, Su T, Horng T, Chow A, Akira S, Medzhitov R. TRAM couples endocytosis of toll-like receptor 4 to the induction of interferon-beta. Nat Immunol (2008) 9:361–8. doi:10.1038/ni1569
104. Kim Y-M, Brinkmann MM, Paquet M-E, Ploegh HL. UNC93B1 delivers nucleotide-sensing toll-like receptors to endolysosomes. Nature (2008) 452:234–8. doi:10.1038/nature06726
105. Tabeta K, Hoebe K, Janssen EM, Du X, Georgel P, Crozat K, et al. The Unc93b1 mutation 3d disrupts exogenous antigen presentation and signaling via toll-like receptors 3, 7 and 9. Nat Immunol (2006) 7:156–64. doi:10.1038/ni1297
106. Deguine J, Lee BL, Newman ZR, Barton GM. No antigen-presentation defect in Unc93b1(3d/3d) (3d) mice. Nat Immunol (2013) 14:1101–2. doi:10.1038/ni.2733
107. Maschalidi S, Nunes-Hasler P, Nascimento CR, Sallent I, Lannoy V, Garfa-Traore M, et al. UNC93B1 interacts with the calcium sensor STIM1 for efficient antigen cross-presentation in dendritic cells. Nat Commun (2017) 8:1640. doi:10.1038/s41467-017-01601-5
108. Nunes-Hasler P, Maschalidi S, Lippens C, Castelbou C, Bouvet S, Guido D, et al. STIM1 promotes migration, phagosomal maturation and antigen cross-presentation in dendritic cells. Nat Commun (2017) 8:1852. doi:10.1038/s41467-017-01600-6
109. Gil-Torregrosa BC, Lennon-Duménil AM, Kessler B, Guermonprez P, Ploegh HL, Fruci D, et al. Control of cross-presentation during dendritic cell maturation. Eur J Immunol (2004) 34:398–407. doi:10.1002/eji.200324508
110. Neijssen J, Herberts C, Drijfhout JW, Reits E, Janssen L, Neefjes J. Cross-presentation by intercellular peptide transfer through gap junctions. Nature (2005) 434:83–8. doi:10.1038/nature03290
111. Mendoza-Naranjo A, Saéz PJ, Johansson CC, Ramírez M, Mandakovic D, Pereda C, et al. Functional gap junctions facilitate melanoma antigen transfer and cross-presentation between human dendritic cells. J Immunol (2007) 178:6949–57. doi:10.4049/jimmunol.178.11.6949
112. Saccheri F, Pozzi C, Avogadri F, Barozzi S, Faretta M, Fusi P, et al. Bacteria-induced gap junctions in tumors favor antigen cross-presentation and antitumor immunity. Sci Transl Med (2010) 2:44ra57. doi:10.1126/scitranslmed.3000739
113. Reits E, Griekspoor A, Neijssen J, Groothuis T, Jalink K, van Veelen P, et al. Peptide diffusion, protection, and degradation in nuclear and cytoplasmic compartments before antigen presentation by MHC class I. Immunity (2003) 18:97–108. doi:10.1016/S1074-7613(02)00511-3
114. Dolan BP, Gibbs KD, Ostrand-Rosenberg S. Dendritic cells cross-dressed with peptide MHC class I complexes prime CD8+ T cells. J Immunol (2006) 177:6018–24. doi:10.4049/jimmunol.177.9.6018
115. Smyth LA, Harker N, Turnbull W, El-Doueik H, Klavinskis L, Kioussis D, et al. The relative efficiency of acquisition of MHC:peptide complexes and cross-presentation depends on dendritic cell type. J Immunol (2008) 181:3212–20. doi:10.4049/jimmunol.181.5.3212
Keywords: dendritic cells, cross-presentation, antigen processing, endosomes, antigen dislocation
Citation: Embgenbroich M and Burgdorf S (2018) Current Concepts of Antigen Cross-Presentation. Front. Immunol. 9:1643. doi: 10.3389/fimmu.2018.01643
Received: 22 May 2018; Accepted: 04 July 2018;
Published: 16 July 2018
Edited by:
Diana Dudziak, Universitätsklinikum Erlangen, GermanyReviewed by:
Steffen Jung, Weizmann Institute of Science, IsraelJoke M. M. Den Haan, VU University Medical Center, Netherlands
Copyright: © 2018 Embgenbroich and Burgdorf. This is an open-access article distributed under the terms of the Creative Commons Attribution License (CC BY). The use, distribution or reproduction in other forums is permitted, provided the original author(s) and the copyright owner(s) are credited and that the original publication in this journal is cited, in accordance with accepted academic practice. No use, distribution or reproduction is permitted which does not comply with these terms.
*Correspondence: Sven Burgdorf, YnVyZ2RvcmZAdW5pLWJvbm4uZGU=