- 1Division of Clinical Infectious Diseases, Research Center Borstel, Borstel, Germany
- 2German Center for Infection Research (DZIF), Hamburg, Germany
- 3International Health/Infectious Diseases, University of Lübeck, Lübeck, Germany
- 4Division of Microbial Interface Biology, Research Center Borstel, Borstel, Germany
- 5National Reference Center for Mycobacteria, Research Center Borstel, Borstel, Germany
- 6Department of Medicine, Karolinska Institute, Stockholm, Sweden
Background: In order to eliminate tuberculosis (TB), an effective vaccine is urgently needed to prevent infection with Mycobacterium tuberculosis. A key obstacle for the development of novel TB vaccines is the lack of surrogate markers for immune protection against M. tuberculosis.
Methods: We investigated growth rates of M. tuberculosis in the mycobacterial growth inhibition assay (MGIA) as a marker for mycobacterial growth control of human bronchoalveolar lavage (BALC) and peripheral blood mononuclear cells (PBMC) before and after vaccination with Mycobacterium bovis Bacille Calmette–Guérin (BCG) of healthy adult volunteers.
Results: Vaccination induced a positive response (p < 0.001) to purified protein derivate (PPD) in 58.8% of the individuals in an interferon-γ release assay-ELISpot. Intraindividual evaluation of the MGIA growth rates before and after M. bovis BCG-vaccination revealed no significant difference in time to culture positivity before and after vaccination in BALC (p = 0.604) and PBMC (p = 0.199). The magnitude of the PPD-response induced by M. bovis BCG-vaccination did not correlate with growth control in BALC and PBMC (correlation = 0.468, 95% CI: −0.016 to 0.775).
Conclusion: In conclusion, M. bovis BCG-vaccination-induced mycobacterial-specific cytokine immune response does not result in functional immune control against M. tuberculosis in the MGIA.
Introduction
Tuberculosis (TB) is a leading cause of morbidity and mortality worldwide (1). In the year 2016, the World Health Organization reported 10.4 million new cases of TB. Elimination of TB appears to be an unrealistic goal in the near future unless TB prevention can be dramatically improved. Prevention of TB can be achieved by vigorous infection control measures, treatment of individuals that are latently infected with Mycobacterium tuberculosis (LTBI) and most effectively by a preventive vaccine. Almost 100 years ago, Albert Calmette and Camille Guérin developed a vaccine based on attenuated Mycobacterium bovis [Bacillus Calmette-Guérin (BCG)], which is still the only available anti-TB vaccine in clinical use today (2, 3). Although M. bovis BCG is among the most commonly used of all vaccines worldwide, its effect is largely on the attenuation of severe forms of the disease in children and it does not prevent TB in adults (4, 5).
In view of this important limitation, there is an urgent need for the development of a novel TB vaccine, with the property to prevent active TB in adults and children (6–8). None of the candidates have shown a significant protective effect against TB infection (7–11). The main obstacle to validate a new vaccine candidate’s ability to protect against M. tuberculosis infection is the lack of a robust correlate of protection (12).
The in vitro mycobacterial growth inhibition assay (MGIA) appears to be a promising marker to detect protective immunity of vaccine-induced cells toward M. tuberculosis (13–17). Improved immune control of mycobacterial growth was demonstrated following primary M. bovis BCG-vaccination when compared to individuals who had been M. bovis BCG-vaccinated as a child and revaccinated (15). In another human challenge trial, improved immune control was reported in individuals with past M. bovis BCG-vaccination, but no additional protective effect was seen after MVA85A-vaccination (16). And H56:CAF01-vaccination revealed an enhanced immune-control in the MGIA corresponding to in vivo protection in mice (17). So far, the MGIA has been evaluated in the following cell types and species: splenocytes (17–19) and bone-marrow-derived macrophages in mice (20), whole blood of Rhesus macaques (21), whole blood cells, frozen and fresh peripheral blood mononuclear cells (PBMC) (15, 16, 22, 23), and antigen-expanded T-cells (24) in humans.
In order to test the MGIA as a pulmonary correlate of immune protection from mycobacterial infection, we recruited M. bovis BCG-naïve healthy adult volunteers and analyzed mycobacteria-specific immune responses and growth of M. tuberculosis in an ex vivo growth inhibition model in bronchoalveolar lavage cells (BALCs) and PBMC before and after vaccination with M. bovis BCG.
Materials and Methods
Study Design
Healthy M. bovis BCG-vaccine-naïve subjects underwent phlebotomy and bronchoscopy before and 8 weeks after intracutaneously administered M. bovis BCG-vaccination. At both time points, BALC and PBMC were in vitro infected with M. tuberculosis (H37Rv) and cultured for 96 h. M. tuberculosis growth rate was determined by the time to culture positivity (TTP) in the MGIA in liquid cultures [Mycobacterium growth indicator tube (MGIT)], Becton, Dickinson and Company (BD), Franklin Lakes, NJ, USA, and colony forming units (CFU) on solid cultures (Middlebrook 7H10, BD). BALC and PBMC were characterized by flow cytometry for CD3, CD4, CD8, Granulysin, Granzyme B, and Perforin expression. T-cell immune responses of PBMC were further analyzed in an interferon-γ release assay (IGRA) enzyme-linked immunospot assay (ELISpot).
Study Approval
This study complied with the declaration of Helsinki (2008) and ethics approval was obtained from the Ethics Committee of the University of Lübeck (14-091). Reporting follows the “Standards for Reporting of Diagnostic Accuracy” (STARD).
Inclusion and Exclusion Criteria
Participants had no history of TB or M. bovis BCG-vaccination and negative IGRA results in PBMC to exclude LTBI at the beginning of the study.
To reduce potential adverse events of M. bovis BCG-vaccination, volunteers were investigated for their health-status and immune-competence by testing for HIV, Hepatitis B or C-infection, analysis of the CD4/CD8 T-cell quotient and the differential blood cell-count and immunoglobulin status by serum electrophoresis. Pregnancy was excluded in female participants. Before bronchoscopy, the differential blood cell-count and coagulation parameters were analyzed repeatedly and symptom screen for any on-going viral or bacterial infection and physical examination were performed. The study flow diagram is shown in Figure 1.
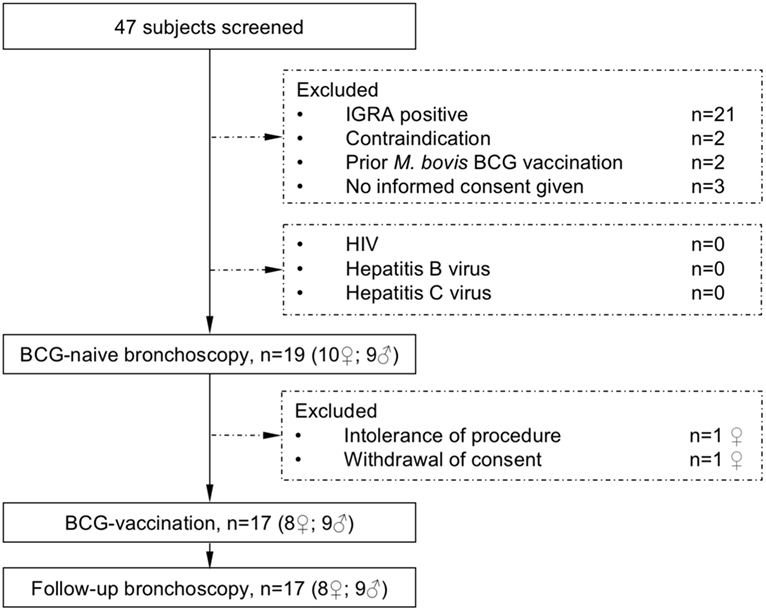
Figure 1. The study flow diagram describes participant screening and study interventions. The interferon-γ release assay (IGRA) was performed to exclude previous contact to mycobacteria. All subjects were healthy and not infected with HIV (human immunodeficiency virus) or hepatitis B or C virus before receiving Mycobacterium bovis Bacille Calmette–Guérin (BCG) vaccination.
Cell Sampling and Preparation
Flexible bronchoscopy including bronchoalveolar lavage (BAL) of the middle lobe was performed with 300 mL saline by a pulmonologist according to German national recommendations (25). Sedation was achieved by the application of intravenous midazolam and propofol.
Bronchoalveolar lavage was processed immediately to isolate the BALC as previously described (26, 27). In brief, after centrifugation (10 min, 578 g, 4°C) and washing steps, cells were counted and adjusted to 10 Mio/mL in cell culture medium (Rosewell Park Memorial Institute; RPMI with 5 µg/mL Amphotericin B, 100 U/mL Penicillin G and 5% human serum—Sigma-Aldrich Corp., St. Louis, MO, USA). Lavage differential cell counts were performed on cytocentrifuged preparations stained by “hemacolor rapid staining of blood smear” (May-Grünwald-Giemsa; Merck, Germany). Thousand cells per sample were counted.
Peripheral blood mononuclear cells were isolated from venous lithium-heparin blood by ficoll density centrifugation, washed, counted, and adjusted for 10 Mio/mL in cell culture medium as previously described (28, 29).
Interferon-γ Release Assay
As antigen-specific interferon gamma release assay, an ELISpot (T-Spot.TB® test, Oxford Immunotec, Abingdon, UK) was performed on PBMC as recommended for blood according to the manufacturer’s recommendation (30). 200,000 PBMCs were cultured in RPMI medium containing 1% penicillin–streptomycin and 5%-fetal-calf-serum (FCS) at 37°C on a precoated 96-well T-spot.TB® test plate (Oxford Immunotec). Negative controls were left unstimulated, positive controls were stimulated with anti-CD3-antibody (10 ng/mL, Beckmann Coulter, Brea, CA, USA). Purified protein derivative (PPD) (10 µg/mL, Statens Serum Institute, Copenhagen, Denmark) was utilized to detect cellular immune-responses toward M. tuberculosis, M. bovis BCG, or non-tuberculous mycobacteria (31, 32). Spot forming cells (SCF) were counted after 18–24 h incubation.
ELISpot assay results were considered conclusive if the number of SFCs in the positive control well was more than 20 SFCs after subtracting the number of spots in the negative control well and had less than twice the number of spots of the negative control well, positive if more than five spots were counted in the ESAT-6 or CFP-10 well after subtracting the number of the SFC in the negative control and if the total number of SFC was at least twice the number of the negative control, and negative in any other case.
M. tuberculosis Inoculum
Mycobacterium tuberculosis H37Rv strain ATCC 27294 was grown in Middlebrook 7H9 broth (BD) supplemented with Middlebrook OADC enrichment medium consisting of 600 µg/mL oleic acid, 50 mg/mL bovine albumin, 20 mg/mL dextrose, and 30 µg/mL catalase (OADC, Life Technologies, Gaithersburg, MI, USA), as well as 0.002% glycerol, and 0.05% tyloxapol. Midlog phase cultures were harvested, aliquoted, and frozen at −80°C.
A new vial was thawed for every experiment and dilution series of the bacterial suspension were prepared in MGIA assay medium for M. tuberculosis. Viable M. tuberculosis counts were determined by plating serial dilutions of the cultures on Middlebrook 7H10 agar plates. This enabled us to calculate the absolute number of CFU as inoculum per experiment. Furthermore, the infection inoculum was directly suspended into MGIT and tubes were placed in a BACTEC 960 instrument until tubes were detected positive. For each test series, the TTP and the concentration of M. tuberculosis measured by CFU were paired and the coefficient of variation was calculated between the experiments.
To determine the necessary bacterial inoculum to reach 156 h in TTP, which fits the midlog phase of the mycobacterial growth and is said to be the widest window of growth inhibition (19), several pre-experiments with increasing concentrations of M. tuberculosis and different cell numbers were performed (Figure S1 in Supplementary Material).
Mycobacterial Growth Inhibition Assay
One million of each of PBMC or BALC were infected with the dosage multiplicity of infection (MOI) of 0.058 (58,000 M. tuberculosis in CFU with 1 Mio cells) per well on a 24-well plate (Nunclon™ Delta surface, Apogent, Roskilde, Denmark) in 600 µL RPMI without HEPES and 5% (0.05 mL/mL) human serum. Since lavage is not physiologically sterile (33), all cells were cultured in the presence of antibiotics (100 U/mL penicillin G, and 5 µg/mL amphotericin B, both Biochrom AG, Berlin, Germany). As control group, M. tuberculosis was cultured for 96 h in cell culture medium without cells. Infection experiments in the MGIA were performed in duplicates, plates were incubated at 37°C and 5% CO2 atmosphere. After 96 h, the mycobacterial growth in PBMC or BALC was stopped by cell lysis with hypotonic aqua destillata. The mycobacterial suspension was transferred into the two different bacterial growth systems: MGIT and agar plates.
Time to Culture Positivity (TTP) in MGIT
After the infection assay, the MGIA-lysate was transferred to the MGIT system, containing liquid 7H9 medium (Middlebrook Bouillon, BD, Franklin Lakes, NJ, USA) with OADC Supplement and PANTA (polymyxin B 400 U/mL, amphotericin B 40 µg/mL, nalidixic 160 µg/mL, trimethoprim 40 µg/mL, and 40 µg Azlocillin/mL). The MGIT device recorded electronically the start time of incubation and the time at which the culture turned positive. Each infection inoculum from day 0 was processed in the same manner.
Colony Forming Units (CFU) on Middlebrook 7H10 Agar Plates
Three serial decimal dilution steps of the MGIA-lysate of day 4 and the infection inoculum on day 0 were plated in triplicates on Middlebrook 7H10 agar plates to determine mycobacterial growth in CFU. Plates were incubated airtight with 5% CO2 for 2 weeks. CFUs were counted manually.
MGIA With In Vitro Supplementation of Vitamin D
In a subset of experiments, the MGIA with PBMC or BAL was performed in the presence of 30 µmol/L vitamin D3 (1α,25(OH)2-cholecalciferol solved in dimethyl sulfoxide), which was added to the plain mycobacterial well, as well as to the infection wells with PBMC plus M. tuberculosis or BALC plus M. tuberculosis.
Flow Cytometry
For phenotypic analysis, freshly isolated PBMC and BALC were stained (20 min, 4°C, light-protection) with the following surface antibodies (all BioLegend; SanDiego; CA, USA): anti-CD3 PerCP Cy5.5 (Clone UCHT1), anti-CD4 Brilliant Violet 510 (Clone OKT4), anti-CD8a APC-Cy7 (Clone RPA-T8). Afterward, cells were permeabilized with 250 µL Cytofix/Cytoperm (BD) for 30 min at 20°C, washed twice, centrifuged (7 min, 912 g, 4°C), and decanted. Intracellular staining was performed with anti-Granzyme B Pacific blue (Clone GB11), anti-Perforin PE (Clone dG9), and anti-Granulysin Alexa Fluor 647 (Clone DH2) for 30 min at 4°C. Acquisition was performed on a FACSCanto II® flow cytometer (BD). Gating strategy is shown in Figure S2 in Supplementary Material. Data were analyzed with FlowJo software version 10.2 (TreeStar, Ashland, TX, USA).
Statistical Analysis
General linear mixed-effects models were used to describe and graph the estimated effects of all predictors (except eosinophils and PPD outliners, described later) and provided p-values for differences between groups, while adjusting for the possible confounding effects of the other predictors. Because multiple observations for each individual in the study were present, the models were corrected for the correlation between observations on the same individual by including the individual identities in the model as random effects. The predictors were several groupings (such as M. bovis BCG vaccination: after/before and vitamin D: no/yes), and the numerical predictor, dilution of the outcome. The CFU counts and the fluorescence data were log-transformed to approximate normality for all analyses. As each outcome was modeled once only, all estimates, including p-values, for a specific outcome, will be adjusted for each other. McNemar tests were used to estimate the p-values for differences in eosinophils and PPD outliners in Table 1. Notched boxplots are used to illustrate the distribution of outcomes in groups. The notches represent approximate 95% confidence intervals for the medians. If the notches do not overlap, the medians of the groups probably differ significantly, and vice versa. All statistics was done in base R: a language and environment for statistical computing, and R packages lmer and lmerTest (https://www.r-project.org/).
Results
Demographics and Patient Characteristics
Nineteen healthy volunteers (nine male, ten female) with a median age of 25 years (range 19–56 years) were recruited for this intervention study. After the first bronchoscopy, one study participant withdrew her consent and one participant was excluded due to hypotension side effects following the first bronchoscopy. Seventeen participants were vaccinated with M. bovis BCG and received a follow-up bronchoscopy 8 weeks after vaccination (median 56 days, range 54–70 days, Figure 1). Figure 2 gives an overview for the different sets of experiments performed.
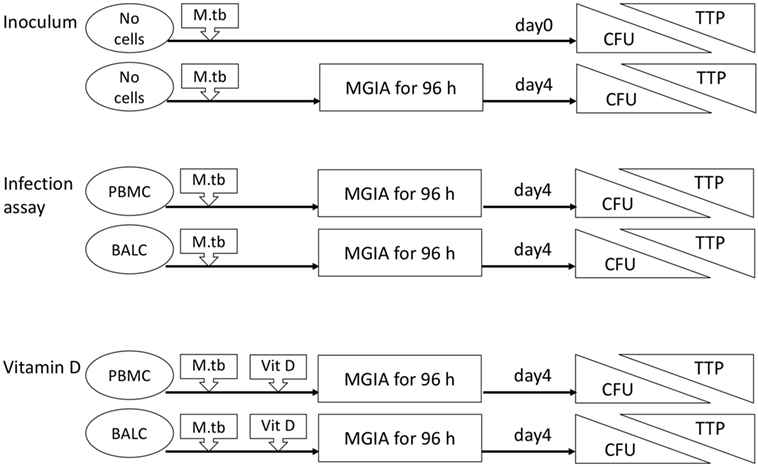
Figure 2. Flow diagram of the different sets of experiments. The inoculum experiment contained no cells but only Mycobacterium tuberculosis H37Rv in dilution. Colony forming units (CFU) and time to culture positivity (TTP) were measured as readings in all mycobacterial growth inhibition assays (MGIA) after 96 h and also immediately in the inoculum experiments. Peripheral blood mononuclear cells (PBMC), bronchoalveolar lavage cells, respectively, were added in the infection assay with M. tuberculosis. In addition to these cells, vitamin D (Vit D) was supplemented in the vitamin D experiments.
Cell Differentiation and IGRA-Immune Responses
Differentiation in the cell composition of BAL did not change before and after vaccination. M. bovis BCG-vaccination induced positive blood IGRA immune responses against PPD in 10/17 participants (58.8%, Table 1).
M. tuberculosis Inoculum
Reproducibility of the inoculum was illustrated by TTP dilution curves repeated for each experiment on day 0 and day 4 (Figure 3): each dilution unit step resulted in an estimated Δ 43.5 h increase in TTP (95% CI: 40.7–46.2 h, p < 0.0001). The inoculum at day 4 represented the growth of the M. tuberculosis inoculum, after 96 h MGIA in cell culture medium without cells, and was used as blank control. Due to the decreased growth of M. tuberculosis in RPMI without cells, TTP values were on average 17.1 h lower in the inoculum on day 4 than on day 0 (95% CI: 15 to 19.1 h, p < 0.0001).
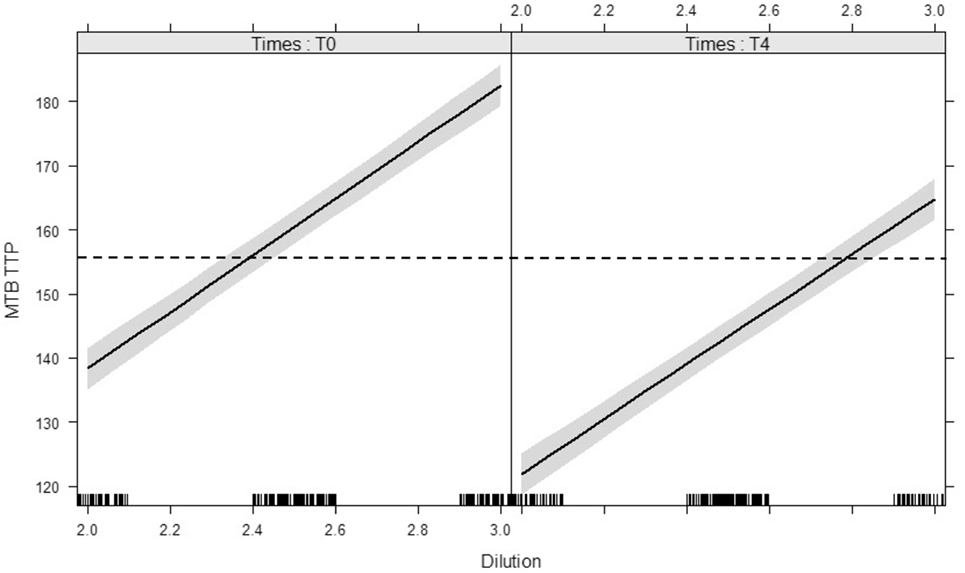
Figure 3. Modeled lines between dilution of Mycobacterium tuberculosis H37Rv inocula and the time to culture positivity (TTP) on day 0 and day 4. The time target of 156 h is marked with a dotted line, the 95% confidence bands are gray. There is no interaction between time (day 0 vs. day 4) and dilution on TTP. Both main effects (slope and intercept) are significant. The modeled lines with their 95% confidence bands illustrates that the slopes of the lines are significant but not different and the intercepts differ significantly.
Infection Assays
The growth of M. tuberculosis was tested in PBMC and BALC, before and after M. bovis BCG-vaccination (Figure 4A). Comparing the capability of cells to restrict M. tuberculosis-growth before and after vaccination, no significant difference in TTP in PBMC (after–before = 2.2 h, p = 0.1990) nor in BALC (after–before = 0.9 h, p = 0.6040) was shown. Irrespective of M. bovis BCG-vaccination, growth (TTP) in PBMC was an estimated 7.6 h longer than in BALC before vaccination (95% CI: 4.3–10.9 h; p < 0.0001) and 9.0 h longer after vaccination (95% CI: 5.6–12.4 h; p < 0.0001).
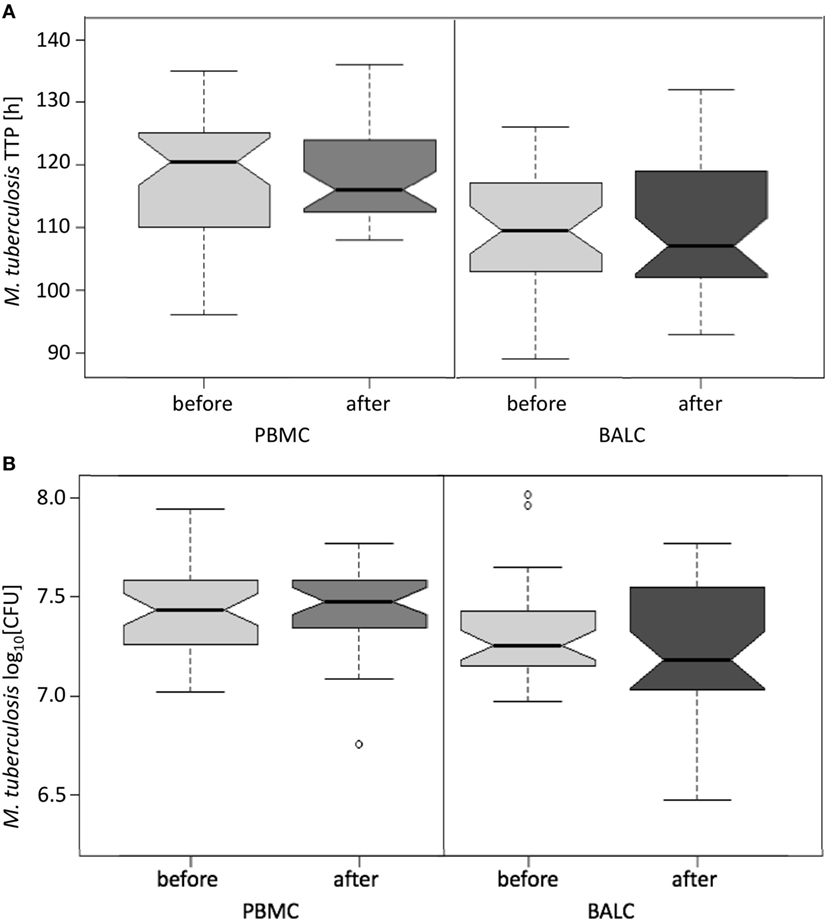
Figure 4. Notched boxplots of the result of Mycobacterium bovis Bacille Calmette–Guérin (BCG) vaccination on healthy human adults in peripheral blood mononuclear cells (PBMC) and Bronchoalveolar lavage cells (BALC) in an in vitro infection assay with Mycobacterium tuberculosis H37Rv. M. tuberculosis growth was measured (A) by time to culture positivity (TTP) or (B) colony forming units (CFU).
In PBMC, the CFU counts did not differ between before and after M. bovis BCG-vaccination (estimate 0.01 log10, p = 0.7512) (Figure 4B). After M. bovis BCG-vaccination, the BALC had an estimated 0.1 lower log10 CFU count (95% CI: 0 to 0.2 h p = 0.0231) than BALC before vaccination. But this effect had to be relativized by the deviation of the infection inoculum, when each TTP of the M. tuberculosis inoculum per experiment was plotted against the corresponding TTP of the M. tuberculosis infected cell type (Figure 5A shows TTP data, Figure 5B CFU data, respectively). M. tuberculosis growth measured as TTP or CFU was not affected by BCG-vaccination.
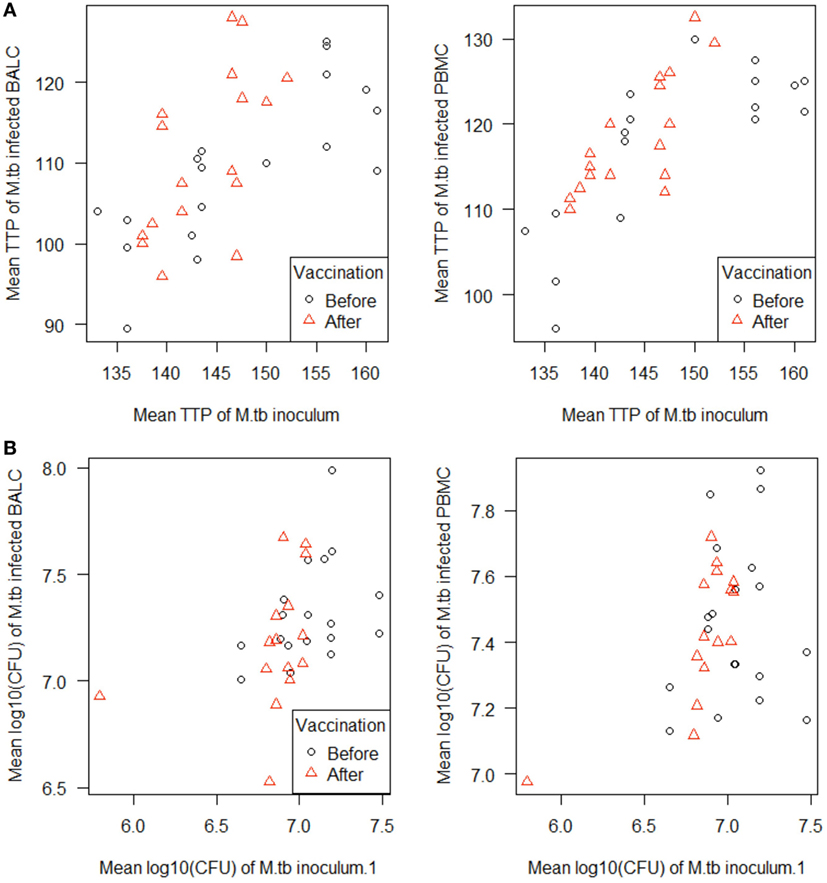
Figure 5. Scatterplots of the in vitro Mycobacterium tuberculosis H37Rv-infected cells [peripheral blood mononuclear cells (PBMC) and bronchoalveolar lavage cells (BALC), respectively] plotted against the M. tuberculosis inoculum. M. tuberculosis growth was measured as (A) time to culture positivity (TTP) or (B) colony forming units (CFU) at both time points before (γ) and after vaccination (Δ).
Vitamin D Supplementation
A test series with vitamin D proved that mycobacterial growth control by human immune cells can be measured by the MGIA: in PBMC and BALC, M. tuberculosis grew slower in the presence of vitamin D, which is reflected by the estimated 17.2 h higher TTP in PBMC (95% CI: 13.4–21.0 h, p < 0.0001) and 20.6 h higher TTP in BALC (95% CI: 16.8–24.3 h, p < 0.0001, Figure 6A), as well as in the 0.441 lower log10 CFU count in PBMC (95% CI: −0.524 to −0,359, p < 0.0001) and 0.226 log10 CFU count in BALC (95% CI: −0.348 to −0.184, p < 0.0001, Figure 6B).
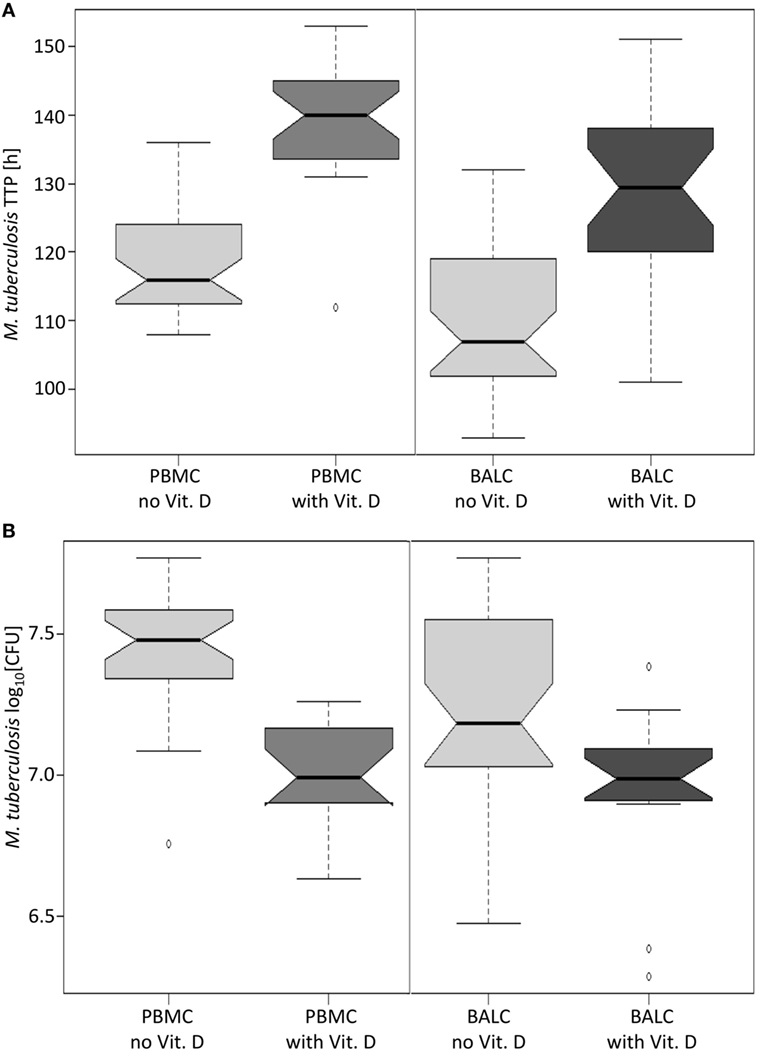
Figure 6. Notched boxplots of the result of vitamin D in peripheral blood mononuclear cells (PBMC) and bronchoalveolar lavage cellss (BALC) of healthy human adults in an in vitro infection assay with Mycobacterium tuberculosis H37Rv. M. tuberculosis growth was measured as (A) time to culture positivity (TTP) or (B) colony forming units (CFU).
Correlation Between IGRA Response and Growth Control in MGIA
To answer the key question of whether growth control after vaccination was influenced by T-cell response, M. tuberculosis-growth (TTP) was stratified by IGRA-response. No correlation between the changes of TTP before and after M. bovis BCG-vaccination and the induced IGRA T-cell immune response was found (correlation = 0.468, 95% CI: −0.016 to 0.775). M. bovis BCG-vaccination-induced IGRA response did not predict the direction of possible changes of any effect on M. tuberculosis-growth control in PBMC nor BALC (Figure 7).
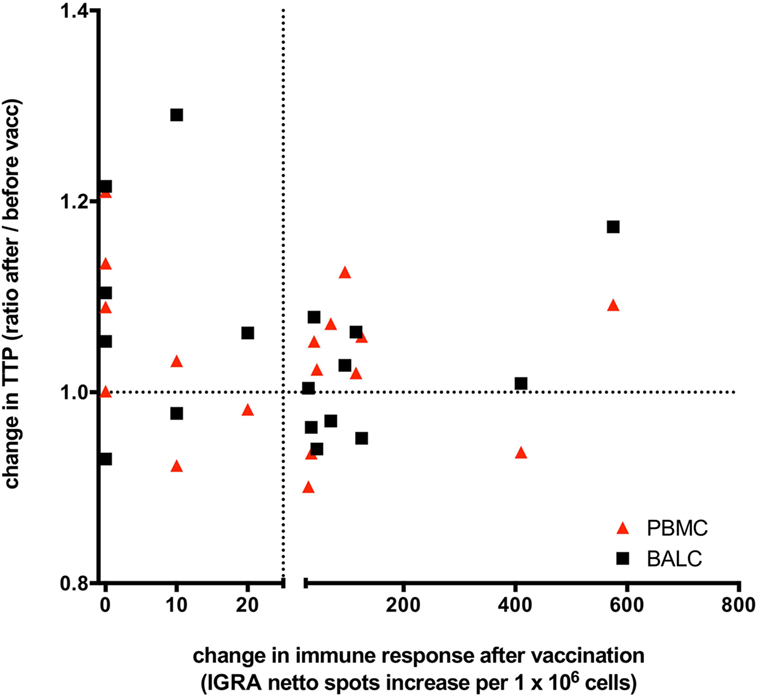
Figure 7. Changes of Mycobacterium tuberculosis growth rate plotted against Mycobacterium bovis Bacille Calmette–Guérin (BCG) vaccination induced T-cell immune response: the change in time to culture positivity (TTP) in the mycobacterial growth inhibition assay (MGIA) is calculated as TTP ratio after vaccination to before vaccination. The dotted line on the y-axis depicts a ratio of 1, indicating the same TTP for M. tuberculosis-growth before and after vaccination. A ratio >1 represents a reduced mycobacterial growth (increase in TTP in the second MGIA after BCG-vaccination) and a ratio <1 represents an increased mycobacterial growth (decrease in TTP in the second MGIA after BCG vaccination). TTP in bronchoalveolar lavage cells (BALC) is given in black squares, TTP in peripheral blood mononuclear cells (PBMC) in red triangles. Before vaccination, all participants had a negative interferon-γ release assay (IGRA) enzyme-linked immunospot (ELISpot) response to purified protein derivate from M. bovis BCG. On the x-axis, a change in the immune response before and after M. bovis BCG-vaccination is given as increase of netto spots per 1 million PBMC in the IGRA-ELISpot 8 weeks after vaccination. The dotted line depicts an increase of 25 netto spots per 1 million PBMC. An increase of less than 25 netto spots in the IGRA means that the immune response after BCG-vaccination stayed negative in that individual, whereas BCG-vaccination had induced an IGRA-response in these individuals, who had an increase >25 netto SFC in the second IGRA. Patients presented in the upper right quadrant have gained a successful innate immune response following vaccination (better growth control of M. tuberculosis and a new positive cytokine immune response), whereas patients situated in the right lower quadrant are having a new positive cytokine response but miss an enhanced growth control. The upper left quadrant shows better growth control without any adaptive immune response. This overall spread depicts the lack of correlation of immune responses and growth control measured by the MGIA.
FACS Analysis
Phenotype characterization (Table 2) revealed a significant lower percentage of lymphocytes in BALC than in PBMC (2.06 vs. 43.90% before vaccination and 0.80 vs. 41.50% after vaccination, p < 0.0001 for difference between cell-types, p = 0.4190 for difference between time points) with a slightly lower CD4/CD8-quotient in BALC than in PBMC (1.66 vs. 3.44% before vaccination and 1.77 vs. 2.52% after vaccination, p = 0.0045 for difference between cell-types, p = 0.3273 for difference between time points).
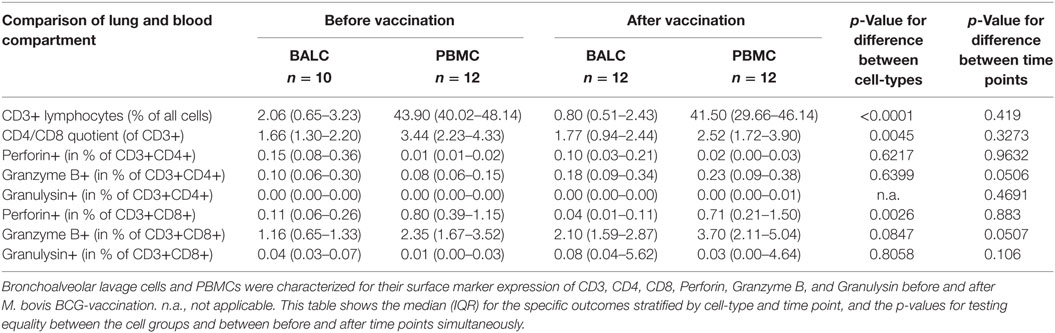
Table 2. Phenotypical characterization of bronchoalveolar lavage cells (BALC) and peripheral blood mononuclear cells (PBMCs) before and after Mycobacterium bovis Bacille Calmette–Guérin (BCG) vaccination by FACS analysis.
Less CD8+ T-cells expressed Perforin in BALC than in PBMC (0.11 vs. 0.80% before vaccination and 0.04 vs. 0.71% after vaccination, p = 0.0026 for difference between cell-types, p = 0.8830 for difference between time points). The expression of Granzyme B was almost affected by vaccination: granzyme B on CD8+ T-cells was elevated from 1.16 to 2.10% on CD8 + BALC and from 2.35 to 3.70% on CD8 + PBMC (p = 0.0507). The expression of Perforin and Granzyme B on CD4+ T-cells was so low, that we would not overestimate any effects seen, the expression of Granulysin on CD4+ T-cells was not detectable.
Discussion
We evaluated the performance of the MGIA as a marker for immune protection following M. bovis BCG-vaccination of healthy adult volunteers. M. bovis BCG-vaccination did not have a significant impact on the mycobacterial growth rate in BALC or PBMC and M. bovis BCG-induced T-cell immune response did not correlate with the mycobacterial growth rate in pulmonary or systemic mononuclear cells.
Due to the absence of an effective vaccine, M. bovis BCG is still applied in new-borns from high-incidence countries to reduce the severity of disease (4, 34). Although a promising vaccine candidate MVA85A induced T-cell mediated immune responses, these signals failed to correlate with protective immunity in a clinical trial (12). In the present study, 58.8% of the participants developed a conversion of their PPD-specific IGRA-ELISpot immune response in blood following M. bovis BCG-vaccination. Nevertheless, M. bovis BCG-vaccination did not result in improved immune control in the M. tuberculosis infection assays (neither TTP nor CFU), suggesting that M. bovis BCG-vaccination does not improve M. tuberculosis growth control. This finding cannot be explained by a failing MGIA but indicates a lack of protective immunity following M. bovis BCG vaccination (4, 5). The missing immune response after M. bovis BCG-vaccination fits to epidemiological data, showing that M. bovis BCG-vaccination does not protect from tuberculosis infection and can only reduce the rates of miliary tuberculosis and tuberculosis meningitis in children (4, 35).
Our results support previous findings that a positive vaccine-antigen-specific IGRA immune response does not correlate with functional immune control for mycobacterial infection in vivo and is, therefore, an inappropriate marker to predict immune protection (12).
The MGIA was presented as a promising surrogate for immune protection in previous studies (16, 18). Vitamin D3 [1α,25(OH)2-Cholecalciferol] is known for its immune-modulating effects on M. tuberculosis-control (36–38) and for inducing an antimicrobial activity of human monocytes/macrophages toward M. tuberculosis. In a vulnerable population with genetic variations in the vitamin D pathway, an adjuvant treatment with high-dose vitamin D was shown to accelerate the time to sputum culture conversion (39). Possible cellular mechanisms were observed to be associated with expression of cathelicidin (40). In our study, this vitamin D-effect is used as a model to monitor improved immune control as expected in an effective vaccine. In the presence of vitamin D, mycobacterial growth rates decreased (measured as increase in TTP or lower CFU counts) confirming the functionality of the MGIA. Our findings corroborate previous studies with regard to the antimycobacterial activity of vitamin D (38, 41).
Our findings differ from previously published results, where M. bovis BCG-vaccination was associated with improved mycobacterial growth control in humans (15, 16). We avoided the potent antituberculosis drug streptomycin and used freshly isolated BALC or PBMC instead of cryopreserved human PBMC or whole blood, third, in our setting, the infection was performed with M. tuberculosis and not with M. bovis BCG. So far, M. tuberculosis has only been used in murine splenocytes (17–19) or murine bone-marrow-derived macrophages (20) to investigate vaccine-induced changes of immune control against M. tuberculosis. With regard to vaccine-induced growth inhibition in human cells, the MGIA was successfully performed with M. bovis BCG in either PBMC and/or whole blood (15, 16, 22). To our knowledge, the current study represents the first description of the MGIA as a surrogate marker for vaccine-induced antimycobacterial activity using M. tuberculosis in human cells (in PBMC and especially in human lung cells), an aspect that has not been addressed so far.
Compared to the mentioned previous studies, which have used MOIs between 0.0001 and 0.0002, it needs to be stated that the MOI of 0.058 used in the current study is significantly higher. In order to make sure that this MOI would allow the measurement of an enhanced antituberculosis response, we included vitamin D to our experiments. As we observed a vitamin D-dependent average TTP increase by 17.2 h in PBMC and 20.6 h in BALC (Figure 6A), we concluded that even at an MOI of 0.058, a given vaccination-dependent antituberculosis directed response leading to prolonged TTP values should have been detectable. This was not the case. Nevertheless, we cannot exclude, that different experimental conditions such as the use of a lower MOI could have led to different results. The current study may, however, indicate that the type of bacteria used in the MGIA needs to be considered. Several authors have shown that M. bovis BCG-vaccination of human individuals induces growth inhibition of M. bovis BCG, whereas our experimental data suggest that M. bovis BCG-vaccination does not lead to growth control of M. tuberculosis by human PBMC and BALC.
The TTP in BALC was significantly lower than TTP in PBMC, implicating higher growth rate in BALC. This effect was not seen in CFU read-outs, which may indicate that CFU and TTP measure different conditions. The MGIT-device detects fluorescence, if actively respiring micro-organisms consume the oxygen. In comparison, CFU documents mycobacteria in colonies on agar plates, where M. tuberculosis grows typically eugone in firmly attached, beige, dry crumbles, but may differ in colony size. Latter observation may indicate a substantially reduced replication status of a given tiny/small colony. Microbiologists have described these small cell populations as persisters or dormant, non-growing bacteria with the capacity to regrow or viable but not-culturable (42). Regarding to M. tuberculosis, it was shown that the replication rate is reduced in small persister-type M. tuberculosis in comparison to a population of larger, culture-viable mycobacteria (43). In vivo replicating and dormant bacterial populations exist within the same patient (43–45). We assume that small, slow-replicating colonies will be counted in the CFU analysis, but may be left underestimated in the MGIA assay, which documents rates of metabolism and growth by oxygen consumption and explain the observed differences between the TTP and CFU analysis.
Another reason to explain this difference in growth behavior might be cell differences between the lung and the blood compartment (46). Lavage consisted of significantly less lymphocytes than PBMC and additionally a lower number of cytotoxic CD8 + T-cells expressing Perforin was found in BALC in comparison with PBMC. These results once more demonstrate that in vitro experiments with PBMC may not imitate the cell-based immune responses and control in human TB (47–49).
Our study is limited by the number of participants and by the lack of an alternative and more promising vaccine candidate for the immune intervention. Although other candidate markers such as mRNA transcripts (50, 51) or cytokines (52) may also appear promising as correlates for protection from M. tuberculosis infection, our findings may serve as valuable proof-of-concept for future vaccine evaluations.
In conclusion, vaccination with M. bovis BCG did not result in ex vivo growth inhibition of M. tuberculosis in human alveolar macrophages from healthy volunteers despite the development of antimycobacterial immune responses in peripheral blood.
Ethics Statement
This study was carried out in accordance with the recommendations of the Declaration of Helsinki (2008) and the Ethics Committee of the University of Lübeck. The protocol was approved by the Ethics Committee of the University Lübeck (14-091). All subjects gave written informed consent in accordance with the Declaration of Helsinki.
Author Contributions
Conceived and designed the study: JH, CL, and BK. Performed the experiments: JR, ER, and BK. Analyzed the data: JR, LM, PSC, NR, and BK. Wrote the manuscript: JR, JH, LM, PSC, NR, ER, CL, and BK.
Conflict of Interest Statement
The authors declare that the research was conducted in the absence of any commercial or financial relationships that could be construed as a potential conflict of interest.
Acknowledgments
The authors thank Andrea Zelmer at the London School of Hygiene and Tropical Medicine (London, United Kingdom) for initial instructions concerning MGIA conduction. Parts of the present work have been presented in the following international conference: European Congress of Clinical Microbiology and Infectious Diseases (ECCMID), April 2017, Vienna, Austria: Oral session 197, Presentation number 6737.
Funding
JR, JH, PSC, NR, CL, and BK receive funding from the German Center for Infection Research (DZIF), Germany.
Supplementary Material
The Supplementary Material for this article can be found online at https://www.frontiersin.org/articles/10.3389/fimmu.2018.01708/full#supplementary-material.
Figure S1. In our pre-experiments, the optimal dosage multiplicity of infection was determined. One million peripheral blood mononuclear cells of three different healthy donors were infected with increasing concentrations [colony forming units (CFU)] of the inoculum of Mycobacterium tuberculosis H37Rv. The concentration of CFU/mL is plotted against the correlating time to culture positivity (TTP) in Mycobacterium growth indicator tubes (MGIT) given in hours (h).
Figure S2. The gating strategy used to identify CD4+ and CD8+ T-cells from peripheral blood mononuclear cells (PBMC) and bronchoalveolar lavage cells (BALCs) is shown in representative density plots from a single person. From left to right, lymphocytes were selected using forward scatter/side scatter (FSC-A/SSC-A)-area, subsequently followed by gating on lymphocyte-singlets (SSC-H/SSC-A), thereafter selecting CD3+ T-cells and stratifying for CD4+ and CD8+ T-cells.
References
1. World Helath organization. Global Tuberculosis Report. Geneva: World Health Organization (2017). Licence: CC BY-NC- SA 3.0 IGO.
2. Kaufmann SH, Lange C, Rao M, Balaji KN, Lotze M, Schito M, et al. Progress in tuberculosis vaccine development and host-directed therapies-a state of the art review. Lancet Respir Med (2014) 2(4):301–20. doi:10.1016/S2213-2600(14)70033-5
3. Andersen P, Doherty TM. The success and failure of BCG– implications for a novel tuberculosis vaccine. Nat Rev Microbiol (2005) 3(8):656–62. doi:10.1038/nrmicro1211
4. Fine PEM. Variation in protection by BCG: implications of and for heterologues immunity. Lancet (1995) 346:1339–45. doi:10.1016/S0140-6736(95)92348-9
5. Rodrigues LC, Pereira SM, Cunha SS, Genser B, Ichihara MY, Brito SCD, et al. Effect of BCG revaccination on incidence of tuberculosis in school-aged children in Brazil: the BCG-REVAC cluster-randomised trial. Lancet (2005) 366(9493):1290–5. doi:10.1016/s0140-6736(05)67503-4
6. Andersen P, Kaufmann SH. Novel vaccination strategies against tuberculosis. Cold Spring Harb Perspect Med (2014) 4(6):a018523. doi:10.1101/cshperspect.a018523
7. Ahsan MJ. Recent advances in the development of vaccines for tuberculosis. Ther Adv Vaccines (2015) 3(3):66–75. doi:10.1177/2051013615593891
8. Zhu B, Dockrell HM, Ottenhoff THM, Evans TG, Zhang Y. Tuberculosis vaccines: opportunities and challenges. Respirology (2018) 23(4):359–68. doi:10.1111/resp.13245
9. Clark S, Lanni F, Marinova D, Rayner E, Martin C, Williams A. Revaccination of guinea pigs with the live attenuated Mycobacterium tuberculosis vaccine MTBVAC improves BCG’s protection against tuberculosis. J Infect Dis (2017) 216(5):525–33. doi:10.1093/infdis/jix030
10. Loxton AG, Knaul JK, Grode L, Gutschmidt A, Meller C, Eisele B, et al. Safety and immunogenicity of the recombinant Mycobacterium bovis BCG vaccine VPM1002 in HIV-unexposed newborn infants in South Africa. Clin Vaccine Immunol (2017) 24(2):e439–416. doi:10.1128/CVI.00439-16
11. Gillard P, Yang PC, Danilovits M, Su WJ, Cheng SL, Pehme L, et al. Safety and immunogenicity of the M72/AS01E candidate tuberculosis vaccine in adults with tuberculosis: a phase II randomised study. Tuberculosis (Edinb) (2016) 100:118–27. doi:10.1016/j.tube.2016.07.005
12. Tameris MD, Hatherill M, Landry BS, Scriba TJ, Snowden MA, Lockhart S, et al. Safety and efficacy of MVA85A, a new tuberculosis vaccine, in infants previously vaccinated with BCG: a randomised, placebo-controlled phase 2b trial. Lancet (2013) 381(9871):1021–8. doi:10.1016/s0140-6736(13)60177-4
13. Brennan MJ, Tanner R, Morris S, Scriba TJ, Achkar JM, Zelmer A, et al. The cross-species mycobacterial growth inhibition assay (MGIA) project, 2010–2014. Clin Vaccine Immunol (2017) 24(9):e142–117. doi:10.1128/CVI.00142-17
14. Tanner R, O’Shea MK, Fletcher HA, McShane H. In vitro mycobacterial growth inhibition assays: a tool for the assessment of protective immunity and evaluation of tuberculosis vaccine efficacy. Vaccine (2016) 34(39):4656–65. doi:10.1016/j.vaccine.2016.07.058
15. Fletcher HA, Tanner R, Wallis RS, Meyer J, Manjaly ZR, Harris S, et al. Inhibition of mycobacterial growth in vitro following primary but not secondary vaccination with Mycobacterium bovis BCG. Clin Vaccine Immunol (2013) 20(11):1683–9. doi:10.1128/cvi.00427-13
16. Harris SA, Meyer J, Satti I, Marsay L, Poulton ID, Tanner R, et al. Evaluation of a human BCG challenge model to assess antimycobacterial immunity induced by BCG and a candidate tuberculosis vaccine, MVA85A, alone and in combination. J Infect Dis (2014) 209(8):1259–68. doi:10.1093/infdis/jit647
17. Jensen C, Holm LL, Svensson E, Aagaard C, Ruhwald M. Optimisation of a murine splenocyte mycobacterial growth inhibition assay using virulent Mycobacterium tuberculosis. Sci Rep (2017) 7(1):2830–41. doi:10.1038/s41598-017-02116-1
18. Zelmer A, Tanner R, Stylianou E, Damelang T, Morris S, Izzo A, et al. A new tool for tuberculosis vaccine screening: ex vivo mycobacterial growth inhibition assay indicates BCG-mediated protection in a murine model of tuberculosis. BMC Infect Dis (2016) 16:412. doi:10.1186/s12879-016-1751-4
19. Marsay L, Matsumiya M, Tanner R, Poyntz H, Griffiths KL, Stylianou E, et al. Mycobacterial growth inhibition in murine splenocytes as a surrogate for protection against Mycobacterium tuberculosis (M. tb). Tuberculosis (Edinb) (2013) 93(5):551–7. doi:10.1016/j.tube.2013.04.007
20. Parra M, Yang AL, Lim J, Kolibab K, Derrick S, Cadieux N, et al. Development of a murine mycobacterial growth inhibition assay for evaluating vaccines against Mycobacterium tuberculosis. Clin Vaccine Immunol (2009) 16(7):1025–32. doi:10.1128/CVI.00067-09
21. Tanner R, O’Shea MK, White AD, Muller J, Harrington-Kandt R, Matsumiya M, et al. The influence of haemoglobin and iron on in vitro mycobacterial growth inhibition assays. Sci Rep (2017) 7:43478. doi:10.1038/srep43478
22. Smith SG, Zelmer A, Blitz R, Fletcher HA, Dockrell HM. Polyfunctional CD4 T-cells correlate with in vitro mycobacterial growth inhibition following Mycobacterium bovis BCG-vaccination of infants. Vaccine (2016) 34(44):5298–305. doi:10.1016/j.vaccine.2016.09.002
23. Joosten SA, van Meijgaarden KE, Arend SM, Prins C, Oftung F, Korsvold GE, et al. Mycobacterial growth inhibition is associated with trained innate immunity. J Clin Invest (2018) 128(5):1837–51. doi:10.1172/JCI97508
24. Hoft D, Worku S, Kampmann B, Whalen C, Ellner J, Hirsch C, et al. Investigation of the relationships between immune-mediated inhibition of mycobacterial growth and other potential surrogate markers of protective Mycobacterium tuberculosis immunity. J Infect Dis (2002) 186(10):1448–57. doi:10.1086/344359
25. Haussinger K, Ballin A, Becker HD, Bolcskei P, Dierkesmann R, Dittrich I, et al. Recommendations for quality standards in bronchoscopy. Pneumologie (2004) 58(5):344–56. doi:10.1055/s-2004-818406
26. Jafari C, Ernst M, Kalsdorf B, Greinert U, Diel R, Kirsten D, et al. Rapid diagnosis of smear-negative tuberculosis by bronchoalveolar lavage enzyme-linked immunospot. Am J Respir Crit Care Med (2006) 174(9):1048–54. doi:10.1164/rccm.200604-465OC
27. Herzmann C, Ernst M, Ehlers S, Stenger S, Maertzdorf J, Sotgiu G, et al. Increased frequencies of pulmonary regulatory T-cells in latent Mycobacterium tuberculosis infection. Eur Respir J (2012) 40(6):1450–7. doi:10.1183/09031936.00214611
28. Brakke MK. Density gradient centrifugation: a new separation technique. J Am Chem Soc (1951) 73:1847–8. doi:10.1021/ja01148a508
29. Brakke MK. Zonal separations by density-gradient centrifugation. Arch Biochem Biophys (1953) 45(2):275–90. doi:10.1016/S0003-9861(53)80005-6
30. Oxford Immunotec Ltd. Manufacturer’s Instructions for T-SPOT.TB 8. Oxfordshire, Great Britain: (2013).
31. Harboe M. Antigens of PPD, old tuberculin, and autoclaved Mycobacterium bovis BCG studied by crossed immunoelectrophoresis. Am Rev Respir Dis (1981) 124:80–7.
32. Weir RE, Black GF, Nazareth B, Floyd S, Stenson S, Stanley C, et al. The influence of previous exposure to environmental mycobacteria on the interferon-gamma response to bacille Calmette-Guérin vaccination in southern England and northern Malawi. Clin Exp Immunol (2006) 146:390–9. doi:10.1111/j.1365-2249.2006.03222.x
33. Charlson ES, Bittinger K, Haas AR, Fitzgerald AS, Frank I, Yadav A, et al. Topographical continuity of bacterial populations in the healthy human respiratory tract. Am J Respir Crit Care Med (2011) 184(8):957–63. doi:10.1164/rccm.201104-0655OC
34. Zwerling A, Behr M, Verma A, Brewer T, Menzies D. The BCG World Atlas: a database of global BCG vaccination policies and practices. PLoS Med (2011) 8(3):e1001012. doi:10.1371/journal.pmed.1001012
35. Mangtani P, Abubakar I, Ariti C, Beynon R, Pimpin L, Fine PE, et al. Protection by BCG vaccine against tuberculosis: a systematic review of randomized controlled trials. Clin Infect Dis (2014) 58(4):470–80. doi:10.1093/cid/cit790
36. Fabri M, Stenger S, Shin DM, Yuk JM, Liu PT, Realegeno S, et al. Vitamin D is required for IFN-gamma-mediated antimicrobial activity of human macrophages. Sci Transl Med (2011) 3(104):104ra102. doi:10.1126/scitranslmed.3003045
37. Martineau AR, Jolliffe DA, Hooper RL, Greenberg L, Aloia JF, Bergman P, et al. Vitamin D supplementation to prevent acute respiratory tract infections: systematic review and meta-analysis of individual participant data. BMJ (2017) 356:i6583. doi:10.1136/bmj.i6583
38. Coussens A, Timms PM, Boucher BJ, Venton TR, Ashcroft AT, Skolimowska KH, et al. 1alpha,25-dihydroxyvitamin D3 inhibits matrix metalloproteinases induced by Mycobacterium tuberculosis infection. Immunology (2009) 127(4):539–48. doi:10.1111/j.1365-2567.2008.03024.x
39. Ganmaa D, Munkhzul B, Fawzi W, Spiegelman D, Willett WC, Bayasgalan P, et al. High-dose vitamin D3 during tuberculosis treatment in Mongolia. A randomized controlled trial. Am J Respir Crit Care Med (2017) 196(5):628–37. doi:10.1164/rccm.201705-0936OC
40. Liu PT, Stenger S, Tang DH, Modlin RL. Cutting edge: vitamin D-mediated human antimicrobial activity against Mycobacterium tuberculosis is dependent on the induction of cathelicidin. J Immunol (2007) 179(4):2060–3. doi:10.4049/jimmunol.179.4.2060
41. Liu PT, Stenger S, Li H, Wenzel L, Tan BH, Krutzik SR, et al. Toll-like receptor triggering of a vitamin D–mediated human antimicrobial response. Science (2006) 311(5768):1770–3. doi:10.1126/science.1123933
42. Zhang Y. Persisters, persistent infections and the Yin-Yang model. Emerg Microbes Infect (2014) 3(1):e3. doi:10.1038/emi.2014.3
43. Kayigire XA, Friedrich SO, van der Merwe L, Donald PR, Diacon AH. Simultaneous staining of sputum smears for acid-fast and lipid-containing Myobacterium tuberculosis can enhance the clinical evaluation of antituberculosis treatments. Tuberculosis (Edinb) (2015) 95(6):770–9. doi:10.1016/j.tube.2015.08.001
44. Peddireddy V, Doddam SN, Ahmed N. Mycobacterial dormancy systems and host responses in tuberculosis. Front Immunol (2017) 8:84. doi:10.3389/fimmu.2017.00084
45. Horsburgh CR, Barry CE, Lange C. Treatment of tuberculosis. N Engl J Med (2015) 373(22):2149–60. doi:10.1056/NEJMra1413919
46. Srivastava S, Ernst JD, Desvignes L. Beyond macrophages: the diversity of mononuclear cells in tuberculosis. Immunol Rev (2014) 262(1):179–92. doi:10.1111/imr.12217
47. White D, Sibley L, Dennis MJ, Gooch K, Betts G, Edwards N, et al. Evaluation of the safety and immunogenicity of a candidate tuberculosis vaccine, MVA85A, delivered by aerosol to the lungs of macaques. Clin Vaccine Immunol (2013) 20(5):663–73. doi:10.1128/CVI.00690-12
48. Perdomo C, Zedler U, Kuhl AA, Lozza L, Saikali P, Sander LE, et al. Mucosal BCG vaccination induces protective lung-resident memory T cell populations against tuberculosis. MBio (2016) 7(6):e1686–1616. doi:10.1128/mBio.01686-16
49. Aguilo N, Alvarez-Arguedas S, Uranga S, Marinova D, Monzon M, Badiola J, et al. Pulmonary but not subcutaneous delivery of BCG vaccine confers protection to tuberculosis-susceptible mice by an interleukin 17-dependent mechanism. J Infect Dis (2016) 213(5):831–9. doi:10.1093/infdis/jiv503
50. Berry MP, Graham CM, McNab FW, Xu Z, Bloch SA, Oni T, et al. An interferon-inducible neutrophil-driven blood transcriptional signature in human tuberculosis. Nature (2010) 466(7309):973–7. doi:10.1038/nature09247
51. Zak DE, Penn-Nicholson A, Scriba TJ, Thompson E, Suliman S, Amon LM, et al. A blood RNA signature for tuberculosis disease risk: a prospective cohort study. Lancet (2016) 387(10035):2312–22. doi:10.1016/S0140-6736(15)01316-1
Keywords: tuberculosis, vaccine, mycobacterial growth inhibition assay, Bacille Calmette–Guérin, vitamin D, interferon-γ release assay immune response
Citation: Radloff J, Heyckendorf J, van der Merwe L, Sanchez Carballo P, Reiling N, Richter E, Lange C and Kalsdorf B (2018) Mycobacterium Growth Inhibition Assay of Human Alveolar Macrophages as a Correlate of Immune Protection Following Mycobacterium bovis Bacille Calmette–Guérin Vaccination. Front. Immunol. 9:1708. doi: 10.3389/fimmu.2018.01708
Received: 16 March 2018; Accepted: 11 July 2018;
Published: 24 July 2018
Edited by:
Pere-Joan Cardona, Institut d’Investigació en Ciències de la Salut Germans Trias i Pujol (IGTP), SpainReviewed by:
Simone Joosten, Leiden University Medical Center, NetherlandsMichael Schotsaert, Icahn School of Medicine at Mount Sinai, United States
Copyright: © 2018 Radloff, Heyckendorf, van der Merwe, Sanchez Carballo, Reiling, Richter, Lange and Kalsdorf. This is an open-access article distributed under the terms of the Creative Commons Attribution License (CC BY). The use, distribution or reproduction in other forums is permitted, provided the original author(s) and the copyright owner(s) are credited and that the original publication in this journal is cited, in accordance with accepted academic practice. No use, distribution or reproduction is permitted which does not comply with these terms.
*Correspondence: Barbara Kalsdorf, YmthbHNkb3JmQGZ6LWJvcnN0ZWwuZGU=
†Present address: Elvira Richter, MVZ Laboratory Dr. Limbach, Heidelberg, Germany