- 1Deeley Research Centre, BC Cancer Agency, Victoria, BC, Canada
- 2Department of Biochemistry and Microbiology, University of Victoria, Victoria, BC, Canada
Resident memory T cells (TRM) are a recently identified subset of long-lived memory T cells that are characterized in terms of their unique surface phenotype combined with a non-recirculating pattern of localization to non-lymphoid, peripheral tissues. TRM have quickly become a key area of focus in understanding immune responses to microbial infection in so-called “barrier” tissues, and appear to be particularly critical for protection against repeat exposure at the same site. More recently, tumor-infiltrating T cells with canonical TRM features are being identified in human cancers, in particular cancers of epithelial origin, and their presence is broadly found to be associated with favorable long-term prognosis. Moreover, recent studies have shown that these “resident memory-like” tumor-infiltrating lymphocytes (referred to herein as TILRM) are uniquely activated in melanoma patients undergoing PD-1 directed checkpoint blockade therapy. Accordingly, there is much interest at present regarding the biology of these cells and their precise role in anti-cancer immunity. Herein, we review the current state of the literature regarding TILRM with a specific emphasis on their specificity, origins, and relationship to conventional pathogen-specific TRM and speculate upon the way(s) in which they might contribute to improved prognosis for cancer patients. We discuss the growing body of evidence that suggests TILRM may represent a population of bona-fide tumor-reactive T cells and the attractive possibility of leveraging this cell population for future immunotherapy.
Brief Introduction to Resident Memory T Cells (TRM) and the TRM-Defining Surface Marker CD103
In recent years, there has been growing recognition of the importance of a peripheral, non-recirculating component of the immune system known as TRM [for review see Ref. (1–3)]. TRM have historically been defined by their peripheral tissue localization and lack of circulatory activity. More recently, there is increasing understanding of the unique surface phenotype(s) of TRM and how the specific molecules that comprise this phenotype contribute to their (non-)circulatory nature. Although this phenotype can vary somewhat between tissues, disease states, and CD4 versus CD8 subsets, most TRM in skin, lung, and GI tract typically express CD69, a molecule widely considered to be an indicator of recent activation, but which is also involved in downregulation of the receptor for sphingosine 1 phosphate (S1P1), thereby inhibiting the ability of TRM to traffic out from peripheral tissue in response to S1P1 gradients (4). Likewise, TRM frequently lack surface expression of CCR7, preventing them from trafficking in response to gradients of CCL19 and CCL21 (5). In addition, surface expression of CD103 (the αE component of the αE/β7 integrin molecule) (6, 7) is now widely considered to be a canonical marker of TRM, and although TRM populations can be comprised of variable proportions of both CD4 and CD8 cells, CD103 appears to be uniquely overexpressed by CD8 TRM (8). CD103 expression is also biologically relevant to the non-recirculating phenotype of TRM, as the ligand for αE(CD103)/β7 integrin is E-cadherin expressed on epithelial cells (9). Although chemokines are thought to be the initial mediator of T cell recruitment into peripheral sites of inflammation, adhesive interactions between αE(CD103)/β7 and E-cadherin is thought to be responsible for the long-term “retention” of antigen-specific TRM at relevant sites (10, 11). This phenomenon is particularly well studied in the context of mucosal tissue infection, where the long-term retention of TRM at the site of an initial infection is thought to provide durable and rapid protection against repeat attack by the same organism. Indeed, once TRM populations are established, they can be retained at the original site of infection for months or even years, even in the complete absence of relevant antigen (12–14). This TRM phenomenon can also be exploited by vaccination strategies that involve delivery of vaccine to the relevant mucosal tissue (15). Indeed, the historical field of “mucosal” immunity and the newer field of “TRM-mediated” immunity are rapidly merging in terms of the memory T cell components.
In addition to mediating adhesion and TRM formation, both αE(CD103)/β7 and E-cadherin are also capable of intracellular signaling. For example, the intracellular domain of E-cadherin interacts with β-catenin which in turn interacts with the actin cytoskeleton, affecting cell shape and motility (16). Likewise, cross-linking of surface-expressed αE(CD103)/β7 impacts the shape and motility of lymphocytes (17), enhances T cell proliferation and induces lysis of target cells (18). Thus through the combination of “inside-out” and “outside-in” signals, αE(CD103)/β7 has the potential to profoundly impact TRM effector function, in addition to augmenting peripheral memory formation.
Mechanism of CD103 Upregulation on TRM
TGF-β has long been known to play a key role in the regulation of αE(CD103)/β7 surface expression on T lymphocytes (19, 20). Although TGF-β is often considered solely as an immunosuppressive factor, it is, in reality, a highly pleiotropic cytokine that is expressed in a multitude of (primarily peripheral) tissue types and has biological activities that are context specific (21). Interestingly, although TGF-β is required for upregulation of αE(CD103)/β7 surface expression, TGF-β exposure alone is not sufficient (18, 22). Rather, it is the combination of TGF-β plus concurrent signaling through the TCR that results in dramatic and rapid αE(CD103)/β7 expression. Indeed, the combination of these two signals makes perfect sense biologically as it would allow for large numbers of lymphocytes (with diverse specificities) to transiently traffic through TGF-β-rich sites of peripheral infection, but result in the αE(CD103)/β7-mediated retention of only those T cells with relevant specificity. This model of TRM formation is supported by the finding that in CD103 knockout mice, numbers of TRM are substantially reduced (10). Likewise, dysregulation of the SMAD signaling pathway downstream of the TGF-β-receptor results in reduced numbers of TRM (23).
Although TGF-β-mediated upregulation of CD103 clearly plays an important role in the establishment of TRM, it is certainly not the only mechanism. For example, it has also been reported that the formation of TRM populations can be enhanced through signaling via the homeostatic cytokine, interleukin-15 (IL-15) (24, 25). However, dependency upon IL-15 for TRM formation varies from tissue to tissue (26), implying that the requirement for IL-15 is not absolute and may be more complex than that of TGF-β. Moreover, as described above, CD4+ TRM populations, in general, express much lower levels of CD103 than do CD8+ TRM, thus they must maintain residency in a CD103-independent manner (27, 28).
TRM in the Cancer Setting
In recent years, there has been growing appreciation that TRM biology/immunology is not unique to the infectious disease setting. Indeed, it has long been speculated that TRM play a key role in both allograft rejection and autoimmunity. For example, αE(CD103)/β7 is expressed on the majority of tissue-infiltrating CD8+ T cells during transplant rejection (20, 29, 30) and graft versus host disease (22). In CD103-deficient mice, T cells are not able to infiltrate allogeneic islet cell transplants and allografts persist for long periods in vivo (30, 31) often surrounded by a characteristic “halo” of CD103-deficient CD8 T cells. In the autoimmune disease setting, islet infiltrating cells in both human diabetic patients (32, 33) and mouse models of autoimmune diabetes (34) are enriched for αE(CD103)/β7-expressing TRM. Presumably, in each of these settings TRM are derived via the same TGF-β plus concurrent TCR signaling mechanism described above for infectious diseases.
αE(CD103)/β7-expressing tumor-infiltrating T cells (TIL) are also now turning up, with increasing regularity, in various cancer settings, particularly in cancers of epithelial origin. This should really not be surprising considering the relationship between TGF-β and αE(CD103)/β7 and the frequent expression of TGF-β in cancers of various types. TGF-β overexpression in cancer has been broadly considered as an immunosuppressive mechanism of tumor escape from immunological pressure (21, 35). However, an alternate hypothesis could be that TGF-β production by tumors is not so much an acquired trait as it is an amplification of the TGF-β that is expressed as part of the “normal” biology of epithelial tissues. Regardless of the mechanism, when tumor-reactive T cells enter these TGF-β-rich environments and then become activated through the TCR, there is full reason to assume they would upregulate αE(CD103)/β7 on the cell surface, in the same manner that conventional TRM do.
However, as described above, CD103 expression is only one part the larger phenotypic profile that defines TRM. Whether CD103-expressing TIL are phenotypically identical to conventional pathogen-specific TRM, or whether they are simply closely related cousins is an issue that remains to be determined. For example, the phenotypic features that are known to be shared among conventional TRM populations, regardless of their specificity and/or tissue location, are reported to be driven by the TRM master transcriptional regulators Blimp-1 and Hobit (36). However, the expression of Blimp-1 and Hobit in tumor-infiltrating TRM is yet to be reported. By contrast, the transcription factor Runx3, which influences the downregulation of mRNA transcripts associated with cellular migration (S1pr1, Klf2, and Ccr7) appears to be expressed in both conventional and tumor-infiltrating TRM (37). Moreover, conventional pathogen-specific TRM are thought to be retained in peripheral tissue after resolution of infection, acting as a vanguard against future re-exposure. In this context, a large proportion of conventional TRM are likely persisting in peripheral tissue in an antigen-free manner, until such time as they become re-challenged through re-exposure. By contrast, tumor-infiltrating TRM (assuming they are tumor-specific) are resident within active tumor tissue and would thus be continuously exposed to antigen, which would likely result in a phenotype distinct from conventional “resting” TRM. For these reasons and because the precise relationship between conventional TRM and tumor-infiltrating TRM is yet to be well-defined in the literature, in our laboratory we have adopted the term “TILRM” (resident memory-like TIL) to delineate these CD103-expressing tumor resident cells from conventional pathogen-specific TRM.
Until recently, broader investigation into the global nature of TILRM infiltration in human tumors was severely hampered by the lack of an anti-human CD103 antibody that was suitable for IHC of formalin-fixed tissues. This situation changed in 2013 when a new antibody was, ironically, developed for diagnosis of hairy cell leukemia (38), a setting where CD103 is ectopically overexpressed. Since the introduction of this reagent, TILRM have now been reported to be present in at least eight different tumor settings including lung, breast, ovarian, endometrial, cervical, melanoma, colorectal, pancreatic, and bladder cancer (39–53) (see Table 1). In the majority of these reports, CD103 is used as a marker to delineate “intraepithelial” TIL, and more importantly, the presence of CD103+ TIL is associated with favorable prognosis.
TILRM Cells in the Gynecologic Cancer Setting
Our group first noted the presence of TILRM cells in the ovarian cancer (OvCa) setting during a flow cytometry-based survey of immune cells present in OvCa patient ascites (54). Interestingly, some but not all, ascites specimens contained CD103-expressing T cells, specifically within the CD8 subset and sometimes comprising as much as 80% of the cells in that compartment. The presence of these cells in a fluid-based tissue (ascites) initially seemed inconsistent with them being a TRM population as TRM are normally restricted to solid tissues. However, the ascites compartment in ovarian patients can contain large numbers of free-floating tumor cells plus abundant amounts of TGF-β. Thus it should not be surprising that tumor-specific T cells present in this fluid compartment could adopt a TRM phenotype more typical of solid tissues. We have also found that these cells have a unique phenotype that includes upregulation of HLA-DR, Ki67, and PD-1, but a lack of CD69, CD137, or intracellular cytokines suggesting that they have been recently activated, but are not actively “engaging” with targets at the time of analysis. Although the cells were PD-1 positive (41), they lacked other markers of exhaustion and were capable of robust cytokine production after stimulation with PMA/ionomycin, ex vivo, suggesting that they were not terminally exhausted. These initial findings regarding CD103-expressing TILRM in OvCa were limited to flow cytometric analysis of small numbers of ascites specimens. However, once an IHC-suitable antibody was available, we followed up by analyzing larger cohorts of patients using tissue microarray technology and showed that CD103-expressing TILRM cells were also present in the solid tumors of some, but not all, OvCa patients (55). Moreover, we also demonstrated that infiltration of tumors by TILRM correlated strongly with a favorable 5-year disease-specific survival advantage in high-grade serous cancer (HGSC), the most lethal of OvCas (55, 56). This finding has now been replicated in three additional cohorts of OvCa patients (43, 57, 58) as well as in endometrial (42) and cervical cancers (44). Clearly, TILRM cells are playing an important role in the gynecologic tumor setting, as they are in other epithelial tumor settings.
Evidence in Support of TILRM Cells Being “Tumor-Specific”
Based upon their significant prognostic benefit and unique surface phenotype, we and others speculate that TILRM in OvCa as well as other cancers are highly likely to be tumor-specific (56). Unfortunately, at present there is a paucity of well-characterized tumor antigens in the HGSC setting to directly test this hypothesis. Nonetheless, our group has previously characterized the cellular immune response to the cancer/testis tumor antigen (NY-ESO-1) in a small cohort of HGSC patients (59) by IFN-γ ELISPOT. The specificity of one such patient was mapped to a well-known HLA-A2-restricted epitope (NY-ESO-1157–165) for which MHC tetramer reagents are available. Combining tetramer staining with CD103 staining revealed that NY-ESO-1-specific CD8+ cells in this tumor sample were indeed CD103+ (54), confirming that tumor-specific cells fell within the TILRM compartment in this patient. However, the NY-ESO-1-specific cells in this sample comprised only a tiny proportion of the entire TILRM population, which had otherwise unknown specificity.
Similar results regarding tumor specificity of TILRM have been obtained in other cancer settings. One of the first studies to demonstrate tumor specificity of TILRM was in the non-small cell lung cancer setting wherein the authors found that CD8+CD103+ TIL selectively upregulated CD107a and granzyme B in the presence of autologous tumor cells and also specifically lysed autologous tumor cells when co-cultured in the presence of an anti-PD-1 blocking antibody (39). More recently, TILRM populations have been identified in the melanoma setting and have been shown to contain cells that stain with melan A-specific tetramers (45), again confirming the presence of tumor-specific T cells in the TILRM subset. Likewise, TILRM have been demonstrated to play a role in anti-tumor immunity in various murine tumor models. For example, using a murine model of melanoma it was reported that CD103 was required for establishment of gp100-specific TILRM populations at the tumor site (60). Interestingly, in this model gp100-specific TILRM cells even remained at the site after tumor resolution and provided long-term immunity against rechallenge, but also caused permanent vitiligo in the dermis. On a somewhat related note CD103+ TRM have also recently been reported to be abundant in human vitiligo specimens (61).
Despite the abundance of evidence supporting the likely tumor specificity of TILRM, one should also consider the alternate hypothesis, that because many of these epithelial tumor types originate from a tissue that could be directly or indirectly considered a mucosal barrier tissue, the TILRM populations could actually be conventional pathogen-specific TRM “bystander” populations that have been amplified during tumor outgrowth. Indeed, this possibility has been raised in a very recent study designed to characterize the phenotypes of authentic tumor-specific TIL versus bystander virus-specific TIL present in human colorectal and lung tumors (62). Interestingly, in this study both the tumor-specific and bystander T cells were found to express features of TRM, including CD103, whereas CD39 was found to be a more reliable marker for distinguishing between the two. Although this study does not contradict earlier findings demonstrating CD103 expression by tumor-reactive TIL, if correct, it suggests that TILRM populations may actually be more heterogeneous than previously thought. Indeed this might be particularly relevant in the gynecologic cancer setting as HSV-2 reactive T cells with a typical TRM phenotype have been reported to be present in the cervical tissue of women with known HSV-2 infection (63) and the numbers of typical TRM in the fallopian tube are reported to increase with age (64). Perhaps these pathogen-specific TILRM populations in previously healthy gynecologic barrier tissues simply “come along for the ride” once the tissue becomes cancerous, and perhaps even co-exist with nascent tumor-specific TILRM populations. Clearly, it remains a challenge to the field to more precisely define the specificity of TILRM.
The “Paradox” of the Prognostic Effect of TILRM
As described above, the significant prognostic benefit conferred by TILRM in HGSC and other cancers implies that they are likely to be tumor-specific or at least encompass tumor-specific populations. However, at the same time, this interpretation is somewhat paradoxical as these cells are present in tumor specimens that have been obtained from patients who have required clinical intervention (in the form of surgical de-bulking in the case of HGSC). This scenario suggests that if TILRM are indeed tumor-specific, they have ultimately lost the ability to control growth of the primary tumor. In recent years, it has become readily apparent that this paradox can be explained, at least in part, by various mechanisms of immune suppression and/or immune exhaustion. Indeed, the tumor microenvironment in OvCa, much like other cancers, has long been considered to be highly immunosuppressive due to the presence of soluble immune-inhibitory factors including IL-10, TGF-β, IDO, and PGE-2 (65). Likewise, the master immune-inhibitory switch molecule CTLA-4 has also been shown to be upregulated in the OvCa setting (66). In addition, inhibitory cells such as CD4+ Foxp3+ regulatory T cells (67), immunosuppressive B7-H4+ tumor-associated macrophages (68), and myeloid-derived suppressor cells (69) have all been reported to be present in OvCa. More recently, the PD-1 immune checkpoint pathway has also been found to play a potential role in OvCa (70), as it has in many other cancer settings.
As mentioned above, our group has recently made the observation that the CD103+ TILRM in HGSC tumors (and ascites) are almost universally positive for PD-1 surface expression (41). By contrast, PD-1 surface expression does not seem to be a universal characteristic of conventional TRM where expression of PD-1 is reported to be dynamic and perhaps even restricted to certain tissue types (71, 72). This finding would suggest that unlike conventional TRM, intra-tumoral TILRM may have become partially (or permanently) exhausted likely due to chronic stimulation with tumor antigen over a period of weeks to months. Indeed, we speculate that although CD103 expression may initially be beneficial to TILRM function by promoting retention within the tumor, CD103 may actually be detrimental in the longer term by causing T cells to become “trapped” within the tumor, thereby exacerbating the phenomenon of chronic Ag stimulation (see Figure 1). This scenario is supported by a recent finding in melanoma, wherein CD103+ TILRM selectively and specifically became activated and started expanding in patients who were undergoing anti-PD-1 immunotherapy (45). This finding suggests that TILRM may be critical players in dictating responsiveness to checkpoint blockade therapy, a topic which is currently undergoing intense scrutiny. Thus, more fully understanding the biology of TILRM becomes paramount in that context.
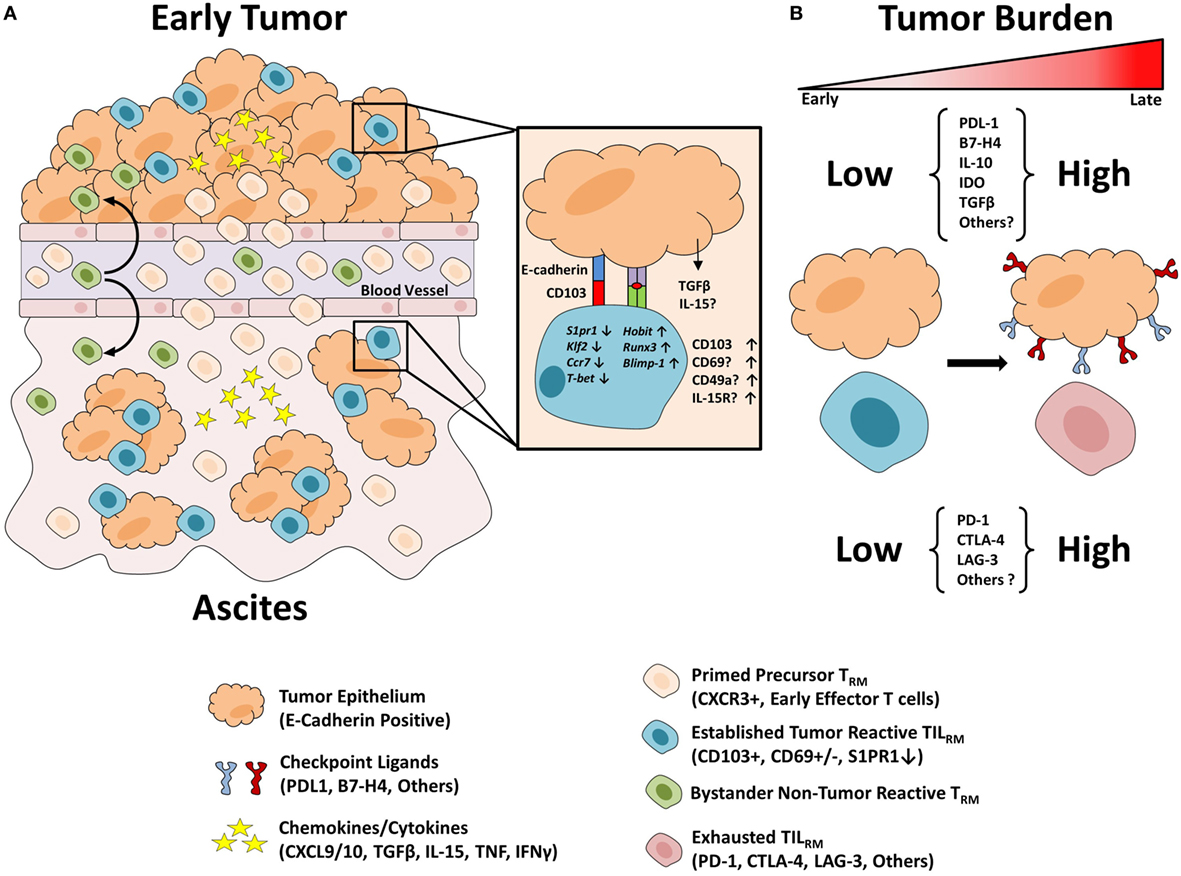
Figure 1. Proposed model of TILRM formation. (A) Precursor resident memory T cells (TRM) populations are composed of previously activated CXCR3+ T cells which are attracted to the chemokines CXCL9/10 in the inflamed tumor environment. Within the epithelial tumor tissue, cells encounter TGF-β which promotes CD103 expression. In response to TCR engagement cells may express increased CD69 which in turn disrupts S1PR1 expression leading to a breakdown in the chemoattractant signal from S1P concentrations in the blood. TILRM cells bind to their target tumor cells with increased strength due to CD103 binding to its ligand E-cadherin, thus promoting their residency in the epithelial tissue. Similarly, precursor TRM may traffic to the inflamed ascites environment and interact with epithelial tumor cells leading to TILRM formation. Finally, bystander precursor TRM populations may traffic to the inflamed tumor and/or ascites environment and develop TRM-like characteristics but with irrelevant antigen specificity. (B) Throughout cancer progression, the tumor microenvironment becomes increasingly inhospitable with increased tumor burden. Tumor cells upregulate immunosuppressive checkpoint receptors to avoid immune eradication. Following T cell activation and prolonged antigen stimulation T cells upregulate a variety of immune checkpoints which act to suppress anti-tumor immunity. TILRM may be inhibited due to the high expression of such checkpoint receptors and thus are likely candidates to respond to immune checkpoint blockade therapy.
Conclusion and Future Perspectives
Resident memory T cells have rapidly gained a reputation as sentinels of peripheral immunity, primed to prevent infection via re-exposure to a previously encountered pathogen. However, the biology of TRM is now spilling over into the field of oncology where TRM are being detected in an increasing number of tumor settings. Whether all the functions and characteristics of conventional TRM directly translate into the unique, dynamic and often hostile microenvironment of tumors has yet to be fully elucidated. Furthermore, what role TILRM play in preventing disease recurrence after standard treatments such as radiation and chemotherapy is essentially unknown territory. Clearly much remains to be learned about these cells. What is certain is the prognostic benefit that comes along with the presence of TILRM, implying that at best, they play a direct role in anti-tumor immunity, or at minimum, they are a surrogate indicator of a separate phenomenon that leads to favorable outcomes for patients with TILRM positive tumors. Future studies should explore the potential utility of these cells in cancer immunotherapy strategies, including checkpoint blockade, cancer vaccination, and cellular therapies. Of particular interest would be understanding methodologies to convert immunologically “cold” tumors to “warm” ones by coaxing the formation and putative anti-tumor activity of TILRM. One can even imagine that the TILRM phenomenon could be applied to the rapid growing field of chimeric antigen receptor (CAR) T cell technology as it transitions into the solid tumor setting, by facilitating the retention of CAR T cells in solid tumor targets. Clearly, we are still in the early days of understanding TILRM biology, but the potential implications for immuno-oncology are significant.
Author Contributions
All authors listed have made a substantial, direct, and intellectual contribution to the work and approved it for publication.
Conflict of Interest Statement
The authors declare that the research was conducted in the absence of any commercial or financial relationships that could be construed as a potential conflict of interest.
References
1. Schenkel JM, Masopust D. Tissue-resident memory T cells. Immunity (2014) 41(6):886–97. doi:10.1016/j.immuni.2014.12.007
2. Park CO, Kupper TS. The emerging role of resident memory T cells in protective immunity and inflammatory disease. Nat Med (2015) 21(7):688–97. doi:10.1038/nm.3883
3. Clark RA. Resident memory T cells in human health and disease. Sci Transl Med (2015) 7(269):269rv261. doi:10.1126/scitranslmed.3010641
4. Mackay LK, Braun A, Macleod BL, Collins N, Tebartz C, Bedoui S, et al. Cutting edge: CD69 interference with sphingosine-1-phosphate receptor function regulates peripheral T cell retention. J Immunol (2015) 194(5):2059–63. doi:10.4049/jimmunol.1402256
5. Watanabe R, Gehad A, Yang C, Scott LL, Teague JE, Schlapbach C, et al. Human skin is protected by four functionally and phenotypically discrete populations of resident and recirculating memory T cells. Sci Transl Med (2015) 7(279):279ra239. doi:10.1126/scitranslmed.3010302
6. Cerf-Bensussan N, Jarry A, Brousse N, Lisowska-Grospierre B, Guy-Grand D, Griscelli C. A monoclonal antibody (HML-1) defining a novel membrane molecule present on human intestinal lymphocytes. Eur J Immunol (1987) 17(9):1279–85. doi:10.1002/eji.1830170910
7. Kruschwitz M, Fritzsche G, Schwarting R, Micklem K, Mason DY, Falini B, et al. Ber-ACT8: new monoclonal antibody to the mucosa lymphocyte antigen. J Clin Pathol (1991) 44(8):636–45. doi:10.1136/jcp.44.8.636
8. Sheridan BS, Lefrancois L. Regional and mucosal memory T cells. Nat Immunol (2011) 12(6):485–91. doi:10.1038/ni.2029
9. Cepek KL, Shaw SK, Parker CM, Russell GJ, Morrow JS, Rimm DL, et al. Adhesion between epithelial cells and T lymphocytes mediated by E-cadherin and the alpha E beta 7 integrin. Nature (1994) 372(6502):190–3. doi:10.1038/372190a0
10. Schön MP, Arya A, Murphy EA, Adams CM, Strauch UG, Agace WW, et al. Mucosal T lymphocyte numbers are selectively reduced in integrin alpha E (CD103)-deficient mice. J Immunol (1999) 162(11):6641–9.
11. Gorfu G, Rivera-Nieves J, Ley K. Role of beta7 integrins in intestinal lymphocyte homing and retention. Curr Mol Med (2009) 9(7):836–50. doi:10.2174/156652409789105525
12. Mackay LK, Stock AT, Ma JZ, Jones CM, Kent SJ, Mueller SN, et al. Long-lived epithelial immunity by tissue-resident memory T (TRM) cells in the absence of persisting local antigen presentation. Proc Natl Acad Sci U S A (2012) 109(18):7037–42. doi:10.1073/pnas.1202288109
13. Casey KA, Fraser KA, Schenkel JM, Moran A, Abt MC, Beura LK, et al. Antigen-independent differentiation and maintenance of effector-like resident memory T cells in tissues. J Immunol (2012) 188(10):4866–75. doi:10.4049/jimmunol.1200402
14. Jiang X, Clark RA, Liu L, Wagers AJ, Fuhlbrigge RC, Kupper TS. Skin infection generates non-migratory memory CD8+ T(RM) cells providing global skin immunity. Nature (2012) 483(7388):227–31. doi:10.1038/nature10851
15. Lycke N. Recent progress in mucosal vaccine development: potential and limitations. Nat Rev Immunol (2012) 12(8):592–605. doi:10.1038/nri3251
16. Wheelock MJ, Johnson KR. Cadherin-mediated cellular signaling. Curr Opin Cell Biol (2003) 15(5):509–14. doi:10.1016/S0955-0674(03)00101-7
17. Schlickum S, Sennefelder H, Friedrich M, Harms G, Lohse MJ, Kilshaw P, et al. Integrin alpha E(CD103)beta 7 influences cellular shape and motility in a ligand-dependent fashion. Blood (2008) 112(3):619–25. doi:10.1182/blood-2008-01-134833
18. Le Floc’h A, Jalil A, Vergnon I, Le Maux Chansac B, Lazar V, Bismuth G, et al. Alpha E beta 7 integrin interaction with E-cadherin promotes antitumor CTL activity by triggering lytic granule polarization and exocytosis. J Exp Med (2007) 204(3):559–70. doi:10.1084/jem.20061524
19. Parker CM, Cepek KL, Russell GJ, Shaw SK, Posnett DN, Schwarting R, et al. A family of beta 7 integrins on human mucosal lymphocytes. Proc Natl Acad Sci U S A (1992) 89(5):1924–8. doi:10.1073/pnas.89.5.1924
20. Hadley GA, Bartlett ST, Via CS, Rostapshova EA, Moainie S. The epithelial cell-specific integrin, CD103 (alpha E integrin), defines a novel subset of alloreactive CD8+ CTL. J Immunol (1997) 159(8):3748–56.
21. Li MO, Flavell RA. TGF-beta: a master of all T cell trades. Cell (2008) 134(3):392–404. doi:10.1016/j.cell.2008.07.025
22. El-Asady R, Yuan R, Liu K, Wang D, Gress RE, Lucas PJ, et al. TGF-{beta}-dependent CD103 expression by CD8(+) T cells promotes selective destruction of the host intestinal epithelium during graft-versus-host disease. J Exp Med (2005) 201(10):1647–57. doi:10.1084/jem.20041044
23. Suzuki R, Nakao A, Kanamaru Y, Okumura K, Ogawa H, Ra C. Localization of intestinal intraepithelial T lymphocytes involves regulation of alphaEbeta7 expression by transforming growth factor-beta. Int Immunol (2002) 14(4):339–45. doi:10.1093/intimm/14.4.339
24. Mackay LK, Wynne-Jones E, Freestone D, Pellicci DG, Mielke LA, Newman DM, et al. T-box transcription factors combine with the cytokines TGF-beta and IL-15 to control tissue-resident memory T cell fate. Immunity (2015) 43(6):1101–11. doi:10.1016/j.immuni.2015.11.008
25. Strutt TM, Dhume K, Finn CM, Hwang JH, Castonguay C, Swain SL, et al. IL-15 supports the generation of protective lung-resident memory CD4 T cells. Mucosal Immunol (2018) 11(3):668–80. doi:10.1038/mi.2017.101
26. Schenkel JM, Fraser KA, Casey KA, Beura LK, Pauken KE, Vezys V, et al. IL-15-independent maintenance of tissue-resident and boosted effector memory CD8 T cells. J Immunol (2016) 196(9):3920–6. doi:10.4049/jimmunol.1502337
27. Sathaliyawala T, Kubota M, Yudanin N, Turner D, Camp P, Thome JJ, et al. Distribution and compartmentalization of human circulating and tissue-resident memory T cell subsets. Immunity (2013) 38(1):187–97. doi:10.1016/j.immuni.2012.09.020
28. Turner DL, Farber DL. Mucosal resident memory CD4 T cells in protection and immunopathology. Front Immunol (2014) 5:331. doi:10.3389/fimmu.2014.00331
29. Hadley GA, Charandee C, Weir MR, Wang D, Bartlett ST, Drachenberg CB. CD103+ CTL accumulate within the graft epithelium during clinical renal allograft rejection. Transplantation (2001) 72(9):1548–55. doi:10.1097/00007890-200111150-00013
30. Feng Y, Wang D, Yuan R, Parker CM, Farber DL, Hadley GA. CD103 expression is required for destruction of pancreatic islet allografts by CD8(+) T cells. J Exp Med (2002) 196(7):877–86. doi:10.1084/jem.20020178
31. Kilshaw PJ, Higgins JM. Alpha E: no more rejection? J Exp Med (2002) 196(7):873–5. doi:10.1084/jem.20021404
32. Kuric E, Seiron P, Krogvold L, Edwin B, Buanes T, Hanssen KF, et al. Demonstration of tissue resident memory CD8 T cells in insulitic lesions in adult patients with recent-onset type 1 diabetes. Am J Pathol (2017) 187(3):581–8. doi:10.1016/j.ajpath.2016.11.002
33. Radenkovic M, Uvebrant K, Skog O, Sarmiento L, Avartsson J, Storm P, et al. Characterization of resident lymphocytes in human pancreatic islets. Clin Exp Immunol (2017) 187(3):418–27. doi:10.1111/cei.12892
34. Barrie ES, Lodder M, Weinreb PH, Buss J, Rajab A, Adin C, et al. Role of ITGAE in the development of autoimmune diabetes in non-obese diabetic mice. J Endocrinol (2015) 224(3):235–43. doi:10.1530/JOE-14-0396
35. Pickup M, Novitskiy S, Moses HL. The roles of TGFbeta in the tumour microenvironment. Nat Rev Cancer (2013) 13(11):788–99. doi:10.1038/nrc3603
36. Mackay LK, Minnich M, Kragten NA, Liao Y, Nota B, Seillet C, et al. Hobit and Blimp1 instruct a universal transcriptional program of tissue residency in lymphocytes. Science (2016) 352(6284):459–63. doi:10.1126/science.aad2035
37. Milner JJ, Toma C, Yu B, Zhang K, Omilusik K, Phan AT, et al. Runx3 programs CD8(+) T cell residency in non-lymphoid tissues and tumours. Nature (2017) 552(7684):253–7. doi:10.1038/nature24993
38. Morgan EA, Yu H, Pinkus JL, Pinkus GS. Immunohistochemical detection of hairy cell leukemia in paraffin sections using a highly effective CD103 rabbit monoclonal antibody. Am J Clin Pathol (2013) 139(2):220–30. doi:10.1309/AJCPHW7RULIZT2GB
39. Djenidi F, Adam J, Goubar A, Durgeau A, Meurice G, de Montpréville V, et al. CD8+CD103+ tumor-infiltrating lymphocytes are tumor-specific tissue-resident memory T cells and a prognostic factor for survival in lung cancer patients. J Immunol (2015) 194(7):3475–86. doi:10.4049/jimmunol.1402711
40. Wang ZQ, Milne K, Derocher H, Webb JR, Nelson BH, Watson PH. CD103 and intratumoral immune response in breast cancer. Clin Cancer Res (2016) 22(24):6290–7. doi:10.1158/1078-0432.CCR-16-0732
41. Webb JR, Milne K, Nelson BH. PD-1 and CD103 are widely coexpressed on prognostically favorable intraepithelial CD8 T cells in human ovarian cancer. Cancer Immunol Res (2015) 3(8):926–35. doi:10.1158/2326-6066.CIR-14-0239
42. Workel HH, Komdeur FL, Wouters MC, Plat A, Klip HG, Eggink FA, et al. CD103 defines intraepithelial CD8+ PD1+ tumour-infiltrating lymphocytes of prognostic significance in endometrial adenocarcinoma. Eur J Cancer (2016) 60:1–11. doi:10.1016/j.ejca.2016.02.026
43. Komdeur FL, Wouters MC, Workel HH, Tijans AM, Terwindt AL, Brunekreeft KL, et al. CD103+ intraepithelial T cells in high-grade serous ovarian cancer are phenotypically diverse TCRalphabeta+ CD8alphabeta+ T cells that can be targeted for cancer immunotherapy. Oncotarget (2016) 7(46):75130–44. doi:10.18632/oncotarget.12077
44. Komdeur FL, Prins TM, van de Wall S, Plat A, Wisman GBA, Hollema H, et al. CD103+ tumor-infiltrating lymphocytes are tumor-reactive intraepithelial CD8+ T cells associated with prognostic benefit and therapy response in cervical cancer. Oncoimmunology (2017) 6(9):e1338230. doi:10.1080/2162402X.2017.1338230
45. Edwards J, Wilmott JS, Madore J, Gide TN, Quek C, Tasker A, et al. CD103(+) tumor-resident CD8(+) T cells are associated with improved survival in immunotherapy-naive melanoma patients and expand significantly during anti-PD-1 treatment. Clin Cancer Res (2018) 24(13):3036–45. doi:10.1158/1078-0432.CCR-17-2257
46. Ganesan AP, Clarke J, Wood O, Garrido-Martin EM, Chee SJ, Mellows T, et al. Tissue-resident memory features are linked to the magnitude of cytotoxic T cell responses in human lung cancer. Nat Immunol (2017) 18(8):940–50. doi:10.1038/ni.3775
47. Quinn E, Hawkins N, Yip YL, Suter C, Ward R. CD103+ intraepithelial lymphocytes – a unique population in microsatellite unstable sporadic colorectal cancer. Eur J Cancer (2003) 39(4):469–75. doi:10.1016/S0959-8049(02)00633-0
48. Boddupalli CS, Bar N, Kadaveru K, Krauthammer M, Pornputtapong N, Mai Z, et al. Interlesional diversity of T cell receptors in melanoma with immune checkpoints enriched in tissue-resident memory T cells. JCI Insight (2016) 1(21):e88955. doi:10.1172/jci.insight.88955
49. Ling KL, Dulphy N, Bahl P, Salio M, Maskell K, Piris J, et al. Modulation of CD103 expression on human colon carcinoma-specific CTL. J Immunol (2007) 178(5):2908–15. doi:10.4049/jimmunol.178.5.2908
50. Cresswell J, Robertson H, Neal DE, Griffiths TR, Kirby JA. Distribution of lymphocytes of the alpha(E)beta(7) phenotype and E-cadherin in normal human urothelium and bladder carcinomas. Clin Exp Immunol (2001) 126(3):397–402. doi:10.1046/j.1365-2249.2001.01652.x
51. Lohneis P, Sinn M, Bischoff S, Jühling A, Pelzer U, Wislocka L, et al. Cytotoxic tumour-infiltrating T lymphocytes influence outcome in resected pancreatic ductal adenocarcinoma. Eur J Cancer (2017) 83:290–301. doi:10.1016/j.ejca.2017.06.016
52. Guo X, Zhang Y, Zheng L, Zheng C, Song J, Zhang Q, et al. Global characterization of T cells in non-small-cell lung cancer by single-cell sequencing. Nat Med (2018) 24(7):978–85. doi:10.1038/s41591-018-0045-3
53. Savas P, Virassamy B, Ye C, Salim A, Mintoff CP, Caramia F, et al. Single-cell profiling of breast cancer T cells reveals a tissue-resident memory subset associated with improved prognosis. Nat Med (2018) 24(7):986–93. doi:10.1038/s41591-018-0078-7
54. Webb JR, Wick DA, Nielsen JS, Tran E, Milne K, McMurtrie E, et al. Profound elevation of CD8+ T cells expressing the intraepithelial lymphocyte marker CD103 (alphaE/beta7 Integrin) in high-grade serous ovarian cancer. Gynecol Oncol (2010) 118(3):228–36. doi:10.1016/j.ygyno.2010.05.016
55. Webb JR, Milne K, Watson P, Deleeuw RJ, Nelson BH. Tumor-infiltrating lymphocytes expressing the tissue resident memory marker CD103 are associated with increased survival in high-grade serous ovarian cancer. Clin Cancer Res (2014) 20(2):434–44. doi:10.1158/1078-0432.CCR-13-1877
56. Webb JR, Milne K, Nelson BH. Location, location, location: CD103 demarcates intraepithelial, prognostically favorable CD8+ tumor-infiltrating lymphocytes in ovarian cancer. Oncoimmunology (2014) 3(1):e27668. doi:10.4161/onci.27668
57. Bösmüller HC, Wagner P, Peper JK, Schuster H, Pham DL, Greif K, et al. Combined immunoscore of CD103 and CD3 identifies long-term survivors in high-grade serous ovarian cancer. Int J Gynecol Cancer (2016) 26(4):671–9. doi:10.1097/IGC.0000000000000672
58. Santoiemma PP, Reyes C, Wang LP, McLane MW, Feldman MD, Tanyi JL, et al. Systematic evaluation of multiple immune markers reveals prognostic factors in ovarian cancer. Gynecol Oncol (2016) 143(1):120–7. doi:10.1016/j.ygyno.2016.07.105
59. Milne K, Barnes RO, Girardin A, Mawer MA, Nesslinger NJ, Ng A, et al. Tumor-infiltrating T cells correlate with NY-ESO-1-specific autoantibodies in ovarian cancer. PLoS One (2008) 3(10):e3409. doi:10.1371/journal.pone.0003409
60. Malik BT, Byrne KT, Vella JL, Zhang P, Shabaneh TB, Steinberg SM, et al. Resident memory T cells in the skin mediate durable immunity to melanoma. Sci Immunol (2017) 2(10). doi:10.1126/sciimmunol.aam6346
61. Boniface K, Jacquemin C, Darrigade AS, Dessarthe B, Martins C, Boukhedouni N, et al. Vitiligo skin is imprinted with resident memory CD8 T cells expressing CXCR3. J Invest Dermatol (2018) 138(2):355–64. doi:10.1016/j.jid.2017.08.038
62. Simoni Y, Becht E, Fehlings M, Loh CY, Koo SL, Teng KWW, et al. Bystander CD8(+) T cells are abundant and phenotypically distinct in human tumour infiltrates. Nature (2018) 557(7706):575–9. doi:10.1038/s41586-018-0130-2
63. Posavad CM, Zhao L, Dong L, Jin L, Stevens CE, Magaret AS, et al. Enrichment of herpes simplex virus type 2 (HSV-2) reactive mucosal T cells in the human female genital tract. Mucosal Immunol (2017) 10(5):1259–69. doi:10.1038/mi.2016.118
64. Rodriguez-Garcia M, Fortier JM, Barr FD, Wira CR. Aging impacts CD103(+) CD8(+) T cell presence and induction by dendritic cells in the genital tract. Aging Cell (2018) 17(3):e12733. doi:10.1111/acel.12733
65. Lavoué V, Thédrez A, Levêque J, Foucher F, Henno S, Jauffret V, et al. Immunity of human epithelial ovarian carcinoma: the paradigm of immune suppression in cancer. J Transl Med (2013) 11:147. doi:10.1186/1479-5876-11-147
66. Melichar B, Nash MA, Lenzi R, Platsoucas CD, Freedman RS. Expression of costimulatory molecules CD80 and CD86 and their receptors CD28, CTLA-4 on malignant ascites CD3+ tumour-infiltrating lymphocytes (TIL) from patients with ovarian and other types of peritoneal carcinomatosis. Clin Exp Immunol (2000) 119(1):19–27. doi:10.1046/j.1365-2249.2000.01105.x
67. Curiel TJ, Coukos G, Zou L, Alvarez X, Cheng P, Mottram P, et al. Specific recruitment of regulatory T cells in ovarian carcinoma fosters immune privilege and predicts reduced survival. Nat Med (2004) 10(9):942–9. doi:10.1038/nm1093
68. Kryczek I, Zou L, Rodriguez P, Zhu G, Wei S, Mottram P, et al. B7-H4 expression identifies a novel suppressive macrophage population in human ovarian carcinoma. J Exp Med (2006) 203(4):871–81. doi:10.1084/jem.20050930
69. Obermajer N, Muthuswamy R, Odunsi K, Edwards RP, Kalinski P. PGE(2)-induced CXCL12 production and CXCR4 expression controls the accumulation of human MDSCs in ovarian cancer environment. Cancer Res (2011) 71(24):7463–70. doi:10.1158/0008-5472.CAN-11-2449
70. Zhu X, Lang J. The significance and therapeutic potential of PD-1 and its ligands in ovarian cancer: a systematic review. Gynecol Oncol (2016) 142(1):184–9. doi:10.1016/j.ygyno.2016.04.002
71. Hombrink P, Helbig C, Backer RA, Piet B, Oja AE, Stark R, et al. Programs for the persistence, vigilance and control of human CD8(+) lung-resident memory T cells. Nat Immunol (2016) 17(12):1467–78. doi:10.1038/ni.3589
Keywords: resident memory T cells, CD8, CD103, tumor-infiltrating lymphocytes, prognosis
Citation: Smazynski J and Webb JR (2018) Resident Memory-Like Tumor-Infiltrating Lymphocytes (TILRM): Latest Players in the Immuno-Oncology Repertoire. Front. Immunol. 9:1741. doi: 10.3389/fimmu.2018.01741
Received: 25 May 2018; Accepted: 13 July 2018;
Published: 26 July 2018
Edited by:
Fathia Mami-Chouaib, Institut National de la Santé et de la Recherche Médicale (INSERM), FranceReviewed by:
Karl Kai McKinstry, University of Central Florida, United StatesTara Marlene Strutt, University of Central Florida, United States
Copyright: © 2018 Smazynski and Webb. This is an open-access article distributed under the terms of the Creative Commons Attribution License (CC BY). The use, distribution or reproduction in other forums is permitted, provided the original author(s) and the copyright owner(s) are credited and that the original publication in this journal is cited, in accordance with accepted academic practice. No use, distribution or reproduction is permitted which does not comply with these terms.
*Correspondence: John R. Webb, andlYmJAYmNjcmMuY2E=