- 1Department of Obstetrics and Gynaecology, Medical University of Vienna, Vienna, Austria
- 2British Columbia's Children's Hospital Research Institute, Vancouver, BC, Canada
- 3Department of Obstetrics and Gynecology, University of British Columbia, Vancouver, BC, Canada
During placentation invasive extravillous trophoblasts (EVTs) migrate into the maternal uterus and modify its vessels. In particular, remodeling of the spiral arteries by EVTs is critical for adapting blood flow and nutrient transport to the developing fetus. Failures in this process have been noticed in different pregnancy complications such as preeclampsia, intrauterine growth restriction, stillbirth, or recurrent abortion. Upon invasion into the decidua, the endometrium of pregnancy, EVTs encounter different maternal cell types such as decidual macrophages, uterine NK (uNK) cells and stromal cells expressing a plethora of growth factors and cytokines. Here, we will summarize development of the EVT lineage, a process occurring independently of the uterine environment, and formation of its different subtypes. Further, we will discuss interactions of EVTs with arteries, veins and lymphatics and illustrate how the decidua and its different immune cells regulate EVT differentiation, invasion and survival. The present literature suggests that the decidual environment and its soluble factors critically modulate EVT function and reproductive success.
Introduction
Development of the human placenta, its distinct epithelial trophoblast subtypes and their interplay with maternal cells and growth factors of the pregnant uterus are crucial for a successful pregnancy. After implantation the trophectoderm, the outermost cell layer of the blastocyst, gives rise to mononuclear cytotrophoblasts (CTBs) forming placental villi through branching morphogenesis. During the first weeks of gestation primary villi, consisting of proliferative CTBs, transform into secondary mesenchymal villi and mature tertiary villi, the latter undergoing vasculogenesis and angiogenesis (1–3). At term these tree-like structures of the human placenta display a surface area of ~15 m2, completely covered with multinuclear syncytiotrophoblasts (STBs). STBs are generated by cell fusion of villous CTBs (vCTBs) and fulfill a vast range of functions such as production of pregnancy hormones, transport of oxygen and nutrients from the maternal blood stream to the growing fetus and clearance of fetal waste products (4, 5). However, early placental development and fetal growth occurs in the absence of maternal blood and oxygen and are likely supported by growth factors and proteins secreted from endometrial glands (6). As soon as the utero-placental circulation is established between 10th and 12th week of pregnancy placental villi are bathed in maternal blood, and hence are termed floating villi.
Whereas, STBs of floating villi represent the transport units of the human placenta, anchoring villi of the placental basal plate form another differentiated trophoblast type, the so called invasive extravillous trophoblast (EVT). Upon attachment of villi to the maternal decidua, the endometrium of the pregnant uterus, proliferative proximal cell column trophoblasts (pCCTs) develop which further differentiate into distal CCTs (dCCTs) ceasing their mitotic activity (Figure 1). EVTs are formed upon detachment from the distal cell column. These cells deeply migrate into the maternal decidua and the first third of the underlying myometrium (7). Already 2 weeks after fertilization two types of EVTs can be discerned within the maternal uterine compartment, interstitial CTBs (iCTBs), colonizing the decidual stroma, and endovascular CTBs (eCTBs), penetrating the maternal spiral arteries (8). Stepwise modification of these vessels is regarded as a critical step in placentation. In the first weeks of pregnancy, EVTs plug the spiral arteries, likely to prevent precocious onset of blood flow to the developing placenta, hence protecting against early placental damage through oxidative stress and fetal loss (6, 9). However, as the embryo switches from histiotrophic to haemotrophic nutrition after the 10th week gestation, plugs dissolve and the endothelial layer of the spiral arteries is replaced by eCTBs (8, 10). The latter are thought to arise by luminal migration into the myometrial segments of spiral arteries (11). Moreover, iCTBs accumulate in the muscular vessel wall promoting its elastolysis and degradation, where decidual macrophages and uterine natural killer cells (uNKs) also contribute to this process (12). Notably, uNK cells, increasing in numbers during the secretory phase of the menstrual cycle and early pregnancy (13), initiate remodeling by inducing apoptosis of vascular smooth muscle cells, whereas iCTBs are thought to complete this process (14). These modifications transform the spiral arteries into highly dilated vessels ensuring low-pressure blood flow to the placenta and the developing fetus. Both iCTBs and eCTBs upregulate adhesion molecules mimicking an endothelial phenotype which could be instrumental during invasion and for the replacement of maternal endothelial cells (15). Defects in vessel remodeling, in particular in the myometrial part of the spiral arteries, have been reported in various pregnancy complications, such as preeclampsia, fetal growth restriction, preterm labor, abortions, and stillbirth (9, 10, 16–19). Failures in immunological acceptance of the placenta, decidual function and/or abnormal trophoblast invasion and differentiation could be underlying causes (20–23). During the first weeks of gestation EVTs, originating from the trophoblastic shell (24), also migrate into decidual lymphatics and veins, already before arterial remodeling occurs (25–27). Number of EVTs in lymphatic and venous vessels is lower in recurrent abortions suggesting that, along with defects in spiral artery remodeling, failed interactions of EVTs with other types of uterine vessels could contribute to pregnancy complications (26). Similarly, EVTs also invade into the decidual glands which could promote early histiotrophic nutrition (28).
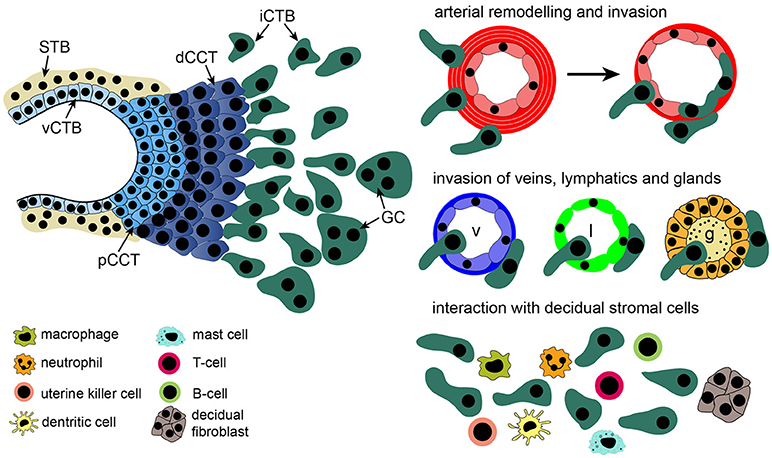
Figure 1. Structure of a placental anchoring villus and its different trophoblast subtypes. Precursors, residing in the villous cytotrophoblast (vCTB) layer either differentiate into multinuclear syncytiotrophoblasts (STBs), surrounded by maternal blood, or give rise to proliferative proximal cell column trophoblasts (pCCTs) upon attachment of villi to the maternal decidua. After differentiation into distal cell column trophoblasts (dCCTs) extravillous trophoblasts (EVTs) develop, breaking through the overlying STB layer. EVTs detach from distal cell columns, migrate into the decidual stroma and the maternal spiral arteries replacing maternal endothelial cells. In the decidual stroma interstitial CTBs (iCTBs) interact with macrophages, B- and T-cells, mast cells, dendritic cells, neutrophils, decidual stromal cells, and uterine natural killer (uNK) cells. Moreover, iCTBs approach the vessel walls of spiral arteries and promote remodeling from outside. Also, veins (v), lymphatics (l) and glands (g) are invaded by these cells forming multinucleated trophoblast giant cells (GC) as an end-stage of differentiation.
In the decidua basalis, iCTBs communicate with diverse cell types of the fetal-maternal interface, such as decidual stromal cells (DSCs) and different immune cells (Figure 1). Amongst those, uNK cells and macrophages have been delineated as the most abundant cell types (29). The role of uNK cells has been extensively investigated throughout the years. Besides their role in the immunological tolerance of the semi-allogenic fetus, uNK cells are thought to affect decidual angiogenesis and EVT function (22, 30). Expression of human leukocyte antigen C (HLA-C) on EVTs, interacting with killer cell immunoglobulin-like (KIR) receptors on uNK cells, could play a role in pregnancy outcome as certain combinations of fetal HLA-C and maternal KIR alleles might increase the risk of developing preeclampsia and recurrent miscarriage (31, 32). It is anticipated that unfavorable HLA-C/KIR interactions impair trophoblast invasion and as a consequence spiral artery remodeling. The role of KIRs and their effects in allorecognition of EVTs has been subject of numerous reviews (22, 33–36) and will be only briefly discussed herein. Instead, we review how uNK cells influence EVTs in a paracrine manner. Further, we will also focus on the other maternal cell types of the decidua and summarize how they might affect cell column growth of anchoring villi, EVT formation and motility. Factors secreted by EVTs, controlling trophoblast migration and invasion in an autocrine manner, have been extensively discussed elsewhere (37, 38), and will not be presented herein. Likewise, the paracrine effects of EVT-secreted factors on decidual immune cell function will not be a topic of this review.
Development of the Evt Lineage and Its Different Subtypes
EVTs originate from distal cell columns of anchoring villi at distinct contact sites with the maternal decidua. Numbers of the latter are a consequence of the frequency of villous branching (5). Different to growth of vCTBs, that form a double-rowed epithelium after lateral cell division and fuse into STBs, pCCTs break through the overlying STB layer and form multiple layers of proliferative trophoblasts (Figure 1). Similar to early phases of tumor formation, pCCTs detach from the basal membrane and lose their polarity. However, in contrast to cancer cells, growth and invasion of trophoblasts is highly organized and precisely controlled in a spatiotemporal manner. At distal sites of anchoring villi, pCCTs differentiate into non-proliferative dCCTs. Similar to iCTBs which have deeply migrated into the decidua, dCCTs express numerous EVT markers such as HLA-G (39), T-cell factor 4 (TCF-4) (40), integrin α5 (ITGA5) and β1 (41), Notch2 (42), proteoglycan 2 (26). and ErbB2 (43). Hence, formation of dCCTs represents the first step of EVT differentiation. In comparison to dCCTs, iCTBs undergo further differentiation by inducing/upregulating specific proteins, for example ITGA1 (41), matrix metalloproteinase (MMP) 12 (44, 45) or diamine oxidase (DAO) (46). The latter is predominantly expressed in EVTs surrounding decidual vessels and was shown to be decreased in serum samples of early-onset preeclamptic women (46). In vivo, DAO is only detected in ~20 and 45% of iCTBs and perivascular CTBs, respectively, providing some evidence for the existence of different iCTB subtypes. Similarly, different EVT populations, identified by single-cell RNA-Seq, have recently been suggested (47). However, it remains largely unknown if variations between iCTBs are specified by the intrinsic genetic program of the placental anchoring villus or determined by the diverse decidual structures. Likewise, the exact route of eCTB migration and the mechanisms specifying these cells have not been unraveled (11). The different phenotypes of EVTs could eventually be influenced by the decidual environment. For example, it was shown that abnormal gene expression of preeclamptic CTBs was reverted back to normal physiological levels when cultured in vitro (21). On the other hand, EVT development per se occurs independently of the decidual environment and its growth factors. Purified CTBs and villous explant cultures, seeded on extracellular matrix, undergo spontaneous EVT differentiation upregulating dCCT, and iCTB markers in a kinetic manner (48–50). In preeclampsia this endogenous EVT differentiation program could be disturbed (51). Anchoring villi and detaching EVTs of tubal pregnancies show the same pattern in integrin switching as EVTs invading the decidua basalis (52). Similarly, EVTs migrating from implanted villous explants and invading the kidney capsule of SCID mice, were shown to induce HLA-G expression (53).
Although the genome-wide expression profiles of non-migratory CTBs and invasive EVTs have been unraveled (54, 55), mechanisms promoting cell column formation and CTB commitment toward the EVT lineage have been poorly elucidated. Recently, Notch1 has been detected in a subset of proliferative pCCTs, indicating that this particular receptor could mark EVT progenitors (56). Indeed, the active Notch1 intracellular domain promoted pCCT survival and marker expression, but suppressed stemness markers of vCTBs suggesting that Notch1 could convert CTB precursors into EVT progenitors (57). Low oxygen levels, occurring during early phases of placental development (58), were shown to trigger Notch1 expression in primary CTBs (57). Hence, low oxygen could promote expansion of EVT progenitors and promote early stages of EVT differentiation and invasion (59). However, the current literature about the specific role of oxygen in trophoblast biology is controversial, has been extensively discussed (60–63), and will not be subject of the present review. Moreover, changes of the self-renewing conditions of long-term expanding 3-dimensional cytotrophoblast organoid cultures promoted outgrowth of Notch1-positive progenitors and EVT formation (64), further supporting the view that development of different trophoblast subtypes is largely determined by the intrinsic differentiation program of the placenta.
The Impact of the Decidua on Extravillous Trophoblasts: General Aspects
In a few species, spontaneous uterine transformation commences during the second half of the menstrual cycle. This process, preceding implantation, is exclusively observed in mammals with menstruation and deep, haemochorial placentation, such as humans and higher primates (65, 66). Shortly after implantation the pregnant uterus undergoes dramatic morphological changes including extracellular matrix remodeling, vascularization, increase in uNK cell numbers and secretory activity of glands as well as transformation of stromal fibroblasts into polygonal decidual cells (67). Decidual glands secrete glycoproteins, such as glycodelin A, carbohydrates and other metabolites nourishing the embryo during the first weeks of pregnancy (68–70). During this phase of histiotrophic nutrition glandular cells also produce various growth factors likely promoting early placental development such as leukemia-inhibitory factors (LIF), epidermal growth factor (EGF), vascular endothelial growth factor (VEGF) and endocrine gland-derived vascular endothelial growth factor (EG-VEGF) (69, 71–74). Indeed, EGF (see below) and EG-VEGF were shown to augment proliferation of vCTBs/CCTs in villous explant cultures (75). Similarly, VEGF was shown to stimulate growth of trophoblast cell lines and primary cultures (76). In contrast, LIF may be mainly involved in the regulation of implantation and trophoblast invasion (77–79).
Differentiation of uterine fibroblasts, commonly referred to as decidualization, initiates during the luteal phase of the menstrual cycle and requires the combined action of cAMP and progesterone on the estrogen-primed endometrium (80). Besides secretion of growth- and- invasion-controlling factors (see below) numerous other functions have been assigned to decidual fibroblasts (DFs). For example, DFs secrete enzymes clearing reactive oxygen species (67, 81) and thereby might protect the decidua and/or EVTs from adverse stress response when local oxygen levels rise between 10th and 12th week of gestation. Trophoblast-derived human chorionic gonadotrophin (hCG) could further increase resistance of DFs against oxidative tissue damage (82). DFs also express various extracellular matrix proteins, such as fibronectin, emilin-1, decorin, fibulins, collagens and laminins (83–86), potentially controlling EVT motility by binding to trophoblast-expressed adhesion molecules and receptors (87).
In women with placenta accreta, EVTs excessively invade the maternal uterus, mostly as a consequence of implantation onto or close to a scar after preceding cesarean section. It is anticipated that the local absence of decidua facilitates trophoblast invasion into the underlying myometrium (88, 89). From this pathology, one might conclude that the decidua restricts migration of trophoblasts thereby controlling depth of invasion in a temporal manner and preventing aberrant, tumor-like expansion of the placenta. Indeed, former concepts suggested that trophoblast-derived MMPs, known to promote invasiveness, are counter-balanced by tissue-inhibitors of metalloproteinases (TIMPs) present in the decidua (90, 91). Similarly, decidual plasminogen activator inhibitors (PAI) 1 and 2 could control timing and depth of trophoblast invasion by inhibiting the plasminogen activator (PA) system expressed by migratory EVTs. However, physiology of trophoblast invasion is more complex since both EVTs and DFs express MMPs, TIMPs, PAs, PAIs as well as urokinase plasminogen activator receptor (uPAR) (90, 92–98). Hence, the decreasing rate of EVT invasion during pregnancy cannot be merely explained by the reciprocal expression of MMPs/uPA and TIMPs/PAI in EVTs and the decidua, respectively. Moreover, potential changes of inhibitor expression throughout gestation or in superficial vs. deeper regions of the decidua have not been measured. Diminished EVT migration at later stages of pregnancy might also be a consequence of the decreasing growth rates of cell columns. As follows, defects in invasion/remodeling, as observed in IUGR, could at least partly be the result of reduced trophoblast growth in this condition (99).
Other data do not support the concept that the decidua restricts trophoblast invasion. In contrast to anchoring villi of normal uterine pregnancies, distal cell columns of ectopic placental villi, isolated from tubal pregnancies, were extended in size. This suggests that the decidua could facilitate EVT detachment from anchoring villi during physiological development of the placental basal plate (52). Indeed, decidualized endometrial stromal cells express a tissue-specific variant of fibronectin favoring trophoblast invasion (100). Whereas, EVTs, generated by first trimester villous explant culture, migrated superficially on dermal fibroblasts, their co-culture with DFs resulted in promotion of interstitial invasion (100). Therefore, the specific features of the decidua may adapt to different stages of pregnancy and precisely control invasion of EVTs by expressing pro- and anti-migratory matrix proteins and factors (37, 38). In return, DFs have a high migratory capacity and could promote implantation by actively moving toward the blastocyst and provoking encapsulation of the conceptus (101). Indeed, EVT supernatants contain chemotactic signals that promote endometrial stromal cell migration (102). Genome-wide expression analyses revealed that trophoblast-conditioned medium of cultivated CTB preparations, containing a mixture of different CTB subtypes, could induce mRNAs encoding chemokines and angiogenic factors in decidualized endometrial fibroblasts (103). However, compared to EVTs, mixed CTB isolates may elicit different responses. In a recent study EVTs increased numbers of resting FoxP3-positive regulatory T cells (Tregs) upon co-cultivation with CD4+ T cells, whereas vCTBs were ineffective (104).
Caveats of in vitro Studies With Extravillous Trophoblasts
Decidua-derived growth factors and their role in trophoblast motility have been investigated in numerous publications. However, many of these studies have drawbacks limiting their scientific value. Access to primary trophoblasts of early pregnancy is generally restricted, hence different trophoblast-like cell lines were utilized in invasion and migration assays. Yet, the specific origin of these cell lines is uncertain and their genome-wide gene expression profiles and HLA status differ considerably from purified CTBs (105, 106). Cell lines proliferate in culture whereas EVTs are non-mitotic cells. Hence, discordant results were obtained between cell lines and primary cells, for example in migration assays under hypoxic conditions (107, 108). Similarly, transforming growth factor β (TGFB), expressed by DFs, uNK cells and uterine glands (109, 110), was shown to either promote or inhibit trophoblast proliferation or invasion (111–115). Besides divergence between cell lines and first trimester CTBs, contaminations with highly migratory placental fibroblasts and variations between different cell isolations and primary model systems might account for multiple discrepancies and the high variances observed in trophoblast-related studies. Invasion assays are often performed with pooled fractions of trypsinized primary CTBs representing a mixture of CCTs, EVTs and vCTBs. The latter also invade through 8 μm transwells in vitro, whilst undergoing cell fusion in vivo. Moreover, nuclear size is a limiting factor in invasion/migration assays (116). Indeed, EVTs become polyploid during differentiation displaying increased nuclear diameter (117, 118). Therefore, EVTs hardly pass membranes with 8 μm pores (50), a fact which has not been considered by the majority of trophoblast invasion/migration studies. Moreover, the high complexity of decidual cell types cannot be mimicked in vitro. In addition, local concentrations of soluble factors in the tissue and their variations during pregnancy and between uterine cell types are poorly studied. Therefore, in vitro assays are usually performed with saturating levels of recombinant factors. As follows, the prime target cell of many decidual proteins remains uncertain since the respective receptors have been identified on several uterine cell types. As a consequence, opposing roles for particular factors were suggested. For example, IL10 was shown to directly impair CTB invasion, but also to abolish the adverse effects of LPS-treated macrophages on trophoblastic cell migration (119, 120). Additionally, results obtained with first trimester villous explant cultures recapitulating attachment, outgrowth and EVT migration in vitro (121) are often interpreted differently by authors. For example, depending on the specific analyses, outgrowth was suggested to be indicative of both increased trophoblast motility and elevated proliferative capacity.
Herein, we focus on the abundant decidual factors, cytokines, and chemokines which have been most convincingly proven to affect EVT formation and function in reliable trophoblast in vitro model systems. However, these studies should also be interpreted in the light of the above-mentioned limitations.
Regulation of Extravillous Trophoblasts by Decidual Fibroblasts
During decidualization fibroblasts upregulate key markers of the pregnant endometrium of which prolactin (PRL) and insulin growth factor binding protein-1 (IGFBP-1) are amongst the most abundantly expressed proteins (122–124). Both proteins likely exert pleiotropic effects on different uterine cell types including regulation of decidualization and EVT migration (125–128). Although PRL, also involved in differentiation of the decidual glandular epithelium (129), was shown to promote motility of first trimester CTBs (130, 131), the role of IGFBP-1 is less clear due to the high complexity of the IGF/IGFBP system. Both migration-activating and -inhibiting effects were attributed to IGFBP-1 upon binding to the EVT-expressed fibronectin receptor ITGA5B1 (132–134). However, one of the main functions of IGFBP-1 could be the regulation of IGF bioavailability at the fetal-maternal interface, possibly triggered by EVT-derived IGF-II (135). Upon secretion of IGF-II from these cells decidual IGFBP-1 might get dephosphorylated and further cleaved by EVT-specific MMP-3 and MMP-9 thereby increasing unbound IGFs, the latter stimulating trophoblast migration (126, 136–139). Yet, proteolytic fragments, generated by trophoblast-derived MMPs, may also restrain trophoblast invasion. Endostatin, a cleavage product of decidual collagen XVIII, was shown to impair IGF-II-induced EVT-motility (50, 140).
Besides the prime markers IGFBP-1 and PRL, other classes of soluble DF-secreted factors were suggested to control EVT motility including chemokines, cytokines and ligands of the EGF and Wingless (WNT) signaling pathways (38, 141). Different CXCL and CCL chemokines have been identified in DFs. Their respective receptors are present on uterine leukocytes and EVTs suggesting a role in immune cell trafficking as well as trophoblast migration, respectively (142, 143). For example, CXCL14 was shown to reduce invasiveness (144), whereas CXCL12 promoted CTB migration and suppressed apoptosis of term trophoblasts through its receptor CXCR4 (145–147). CCL2, expressed by DFs, macrophages and EVTs (148, 149), may recruit T helper 17 cells into the decidua, and interleukin (IL) 17 expressed by these cells could promote trophoblast growth and invasion (150). Other interleukins, shown to stimulate CTB invasion, are IL1B (151, 152) and IL8, secreted from uterine NK cells and DFs (153, 154), whereas IL11 had inhibitory effects (155).
While EGF and heparin-binding EGF (HB-EGF), expressed by the decidua, were shown to stimulate trophoblast invasion and outgrowth from villous explants cultures, proliferation of primary EVTs and trophoblastic HTR-8/SVneo cells was unaffected (156–159). Recently, however, we could demonstrate that these factors increased proliferation of vCTBs and CCTs in villous explant cultures of early placentae (160). Moreover, in contrast to vCTBs and CCTs, EVTs largely lack the EGF/HB-EGF-specific receptors EGFR and ErbB4, and induce ErbB2 and ErbB3 during differentiation (43, 161). Heterodimers of ErbB2 and ErbB3 were shown to interact with neuregulin 1, expressed by DFs, protecting EVTs from apoptosis and thereby retaining their differentiation program (43). Therefore, upregulation of EVT invasion/differentiation by EGF/HB-EGF could largely be a consequence of increased CCT proliferation, while direct effects of these factors on EVTs might be negligible.
Like in other developing tissues WNT signaling has been suggested to play a pivotal role in placental morphogenesis and differentiation (141, 162). Activation of the pathway by secreted ligands stabilizes the key mediator of WNT signaling, β-catenin, and promotes its nuclear recruitment (163). In the nucleus β-catenin binds to DNA-binding proteins of the T-cell factor (TCF) family thereby inducing TCF-mediated gene transcription. Invasive trophoblasts, the secretory endometrium and first trimester DFs express a variety of WNT ligands suggesting autocrine as well as paracrine effects of the particular pathway (164, 165). EVT formation and differentiation is strongly associated with activation of canonical Wnt signaling and nuclear expression of β-catenin, TCF-3 and TCF-4 (40, 166). Indeed, migration and differentiation of EVTs requires TCF-4, whereas survival and proliferation of CCTs is induced by WNT5A involving non-canonical mitogen-activated protein kinase (MAPK) activity (166, 167). Moreover, canonical Wnt signaling might play a dual role in early placental development controlling both long-term expansion of vCTB progenitors and EVT differentiation (64).
Immune Cell Distribution in the Decidua
The pregnant uterus is mainly colonized by cells of the innate immune system, of which the most abundant and by far best characterized cell types are macrophages and uNK cells. Most available literature refers to numbers ranging from 50 to 70% uNK cells, 20–30 % macrophages and 10–15 % T cells of the total CD45+ immune cells in the decidua; only 2 % account toward the less abundant leukocyte populations including dendritic cells or Tregs (30, 168–170). However, the vast majorities of comparative analyses do not consider regional differences (parietalis vs. basalis) in decidual immune distribution and may also miss certain cell populations due to pre-selective isolation methods or due to the lack of appropriate markers to distinguish certain immune cell populations from each other. Hence, before describing uNK cell and macrophage function in detail, we will shortly discuss decidual immune cell populations which in our opinion have widely been ignored in the context of reproductive biology.
Mast cells have been previously described to mainly colonize the uterine myometrium and were shown to localize around decidual vessels. Interestingly, mast cell depletion in a mouse model results in diminished spiral artery remodeling and as a consequence leads to IUGR. The same study demonstrated a close spatial distribution of mast cells and EVTs in the human decidua basalis and reported a mast cell-dependent positive effect on EVT migration (171). Another study showed a role for neutrophils in placentation and trophoblastic giant cell invasion in mice (172). Interestingly, neutrophils seem to accumulate around spiral arteries and develop a pro-angiogenic phenotype toward the second trimester of pregnancy (173). The reason for the oversight of neutrophils might be explained by methodological issues as most protocols to obtain tissue leukocytes involve density gradient centrifugation eliminating all non-mononucleated immune cells, including neutrophils. Nevertheless, it should be taken into consideration that neutrophil accumulation in the decidua may at least be partly a response to blood coagulation and tissue damage occurring during tissue collection. Although mostly ignored, some scientific papers describe the presence of B cells in human term decidua (174). Moreover, a recent study shows that term decidua basalis contains more B cells when compared to decidua parietalis tissues (175). The authors of this study further found that decidua parietalis contains a higher proportion of mature/naive B cells whereas transitional B cells were enriched in decidua basalis. Since altered B cell distributions have recently been associated with preterm labor (174), more studies are needed to determine the role of B cells during pregnancy. In addition, distribution and characterization of B cells in first trimester decidua tissues has not been studied so far. While macrophages are considered to be the main phagocytic and antigen-presenting cell type in the human decidua little is known about dendritic cell distribution and function during pregnancy. One reason for the scarce information concerning decidual dendritic cells is the lack of marker combinations, which could reliably segregate macrophages from dendritic cells, since they develop from a common myeloid progenitor and therefore express common cell surface markers (176, 177). A good example for the problem to distinguish between macrophages and dendritic cells are Langerhans cells. These cells have long been referred to as long-lived dermal dendritic cells and are now considered tissue-resident macrophages with features of dendritic cells such as T cell-stimulation in lymph nodes (178). Despite overlapping cell marker expression and functional similarities in the skin, dendritic cells have unique properties. For instance, DCs homeostatically migrate to draining lymph nodes and are much more potent in antigen cross-presentation to CD8+ T cells (179). Consequently, DCs are likely involved in shaping host immune responses toward the invading EVTs.
Proposed Functions of Uterine Natural Killer Cells
Unlike conventional peripheral blood (pb) NK that are efficient killers, uNK cells in rodents and humans do not normally mount cytotoxic responses against fetal or placental tissues. Instead, growing evidence highlights the importance of uNK in controlling uterine neo-angiogenesis, spiral artery remodeling, the immune response against fetal antigen, and trophoblast function (30, 180–182). However, recent work shows that aberrant inflammation in pregnancy resulting from infection or fetal-driven alloimmunity programs uNK cells to acquire cytotoxic properties that promote fetal death and/or placental dysfunction (183, 184). Therefore, a contemporary view suggests that appropriate uNK activation is important for promoting healthy placentation, where inadequate (not enough) or inappropriate (too much) uNK activity contributes to defective placentation and related disorders of pregnancy that may include recurrent miscarriage, preterm birth, and preeclampsia (185, 186). In women, uNK cell numbers rapidly expand during the progesterone-dominant luteal phase of the menstrual cycle (22). Evidence suggests that the decidual environment, enriched with factors like progesterone and transforming growth factor TGFB1, promote the differentiation of NK cell progenitors into mature uNK cells that are defined phenotypically as CD56superbright/CD16− cells (187, 188). By contrast, the phenotype of conventional pbNK cells is predominantly CD56dim/CD16+. Other distinctive features of uNK include the expression of tissue-residency markers (i.e., CD9, CD69, CD49a) (189, 190) and cytolytic proteins (i.e., perforin, granzyme, and granulysin) (191), and the expression of a distinctive natural killer receptor repertoire (192). In particular, killer immunoglobulin-like receptor (KIR) and natural killer group 2 (NKG2) receptors are robustly expressed by uNK cells (189, 192). These and other natural killer cell receptors, are thought to modulate maternal-fetal recognition, but may also play important roles in controlling aspects of trophoblast biology.
Communication Between Invasive Trophoblasts and Uterine Natural Killer Cells
In vivo evidence shows that EVTs directly interact with uNK cells (193), indicating that by EVT- uNK cell interactions these potentially modulate each other's functions (194–196). EVTs, unlike vCTBs that do not express major histocompatibility complex (MHC) type-I molecules, express a unique combination of classical HLA-C and non-classical HLA-E, HLA-F, and HLA-G class-I ligands playing a role in immunological acceptance of the placenta/fetus (30, 197, 198). This unique MHC composition enables EVT to directly interact with and modulate uNK cell processes through specific combinations of natural killer receptors. However, hard evidence for in vivo or ex vivo natural killer cell receptor-EVT interactions has been challenging to generate, due in large part to the ethical boundaries of working with human samples of pregnancy and to the logistical hurdles of working with primary CTB cultures and uNK cells isolated from tissues of the same pregnancy. As a surrogate for primary EVTs, co-cultivation of trophoblastic HTR-8/SVneo cells with uNK cells were performed demonstrating elevated uNK cell survival and downregulation of the activating NKG2D receptor (199). However, these data have to be interpreted with caution since HTR-8/SVneo cells express a different repertoire of HLA proteins, including HLA-A and HLA-B, which are absent from EVTs (105).
MHC class-I molecules expressed on EVTs can interact with multiple natural killer receptors that transmit inhibitory or activating signals to dampen or promote uNK cytotoxicity and production of cytokines, respectively (30). Perhaps the best-studied uNK cell receptors are the family of polymorphic KIRs that are defined by the presence of either 2 (2D) or 3 (3D) immunoglobulin-like domains and long (L) or short (S) cytoplasmic tails that help initiate inhibitory (L) or activating (S) signals. Inhibitory KIRs expressed on uNK cells include KIR2DL1, KIR2DL2, and KIR2DL3, and these receptors transmit strong inhibitory signals through their immunoreceptor Tyr-based inhibitory motif (ITIM) (35). Activating uNK KIRs include KIR2DS1 and KIR2DS4 (186, 200), however the receptor KIR2DL4, an unconventional KIR that predominantly localizes to endosomes and not the cell membrane, is also capable of transmitting activating signals (104, 201, 202). KIRs bind mainly to HLA-C, expressed by multiple cell types within decidual tissue, including EVTs (203). The number of KIR genes in the genome of any given individual varies within the population, as does the expression of haplotypic specific HLA-C, making the immunogenic complexities of uNK cell-EVT responses unique for any given pregnancy (204).
To date, most research has examined the importance of HLA-C in controlling uNK cell-related processes through either uNK-target cell or antibody cross-linking experiments. It is important to note that, the directionality of uNK cell response to HLA-C depends largely on the epitope type, designated broadly as C1 or C2. This designation is based on a dimorphism at position 80 of the α1 domain of HLA-C (205). Overall, in mixed uNK cell populations that express high levels of inhibitory KIR, HLA-C challenge promotes an inhibitory signal regardless of the co-presence of activating KIRs (186). KIR-directed inhibitory signals associate with impaired or blunted degranulation (206) and reduced secretion of EVT-regulatory factors (i.e. IL8, VEGF, placental growth factor (PGF) and CXCL10, also known as IP-10) (153). In uNK cells, expressing activating KIR2DS1 or KIR2DS4, HLA-C promotes uNK cell degranulation and secretion of granulocyte-macrophage colony-stimulating factor (GM-CSF) and tumor necrosis factor (TNF) (186, 200, 207). Production of these factors potentially impacts EVT biology (discussed below). However, the direct effect of endogenous HLA-C expressed by EVTs on uNK cell processes has yet to be determined. Nonetheless, ex vivo EVTs physically interact with HLA-C-specific KIR2DL1 and KIR2DS1 (204), indicating that biological functions for these KIR-EVT interaction likely do exist.
HLA-E protein is present in EVTs at the 5th week of gestation but absent from these cells after the 7th week, suggesting a predominant role in implantation and/or early trophoblast development (198). Inhibitory signals elicited by HLA-E are mediated through the dimeric CD94/NKG2A receptor (208). Previous work shows that CD94/NKG2A elicits strong suppressive signals that generally override most activating inputs (209). However, whether EVT-derived HLA-E's sole purpose is to restrain uNK cell cytotoxicity in pregnancy is currently not well understood. For example, CD94 function-blocking experiments do not potentiate trophoblast killing (208), suggesting that CD94/NKG2A may serve other roles within the maternal-fetal interface that have yet to be elucidated.
The role of HLA-G in controlling uNK cells has been studied more so than other MHC class-I ligands, due in part to the relevance of its unique and restricted expression within EVT (197). Previous studies have identified two receptors expressed on uNK cells that interact with HLA-G: leukocyte immunoglobulin-like subfamily B member 1 (LILRB1) (210) and KIR2DL4 (211, 212). Interpretation of previous HLA-G-related findings necessitates caution due to the nature and design of the experimental systems used. For example, most approaches implement forced ectopic expression of HLA-G in HLA-null target cell lines, designed to synthesize either of two major HLA-G isoforms expressed by EVTs, namely membrane-bound HLA-G1 (213) or the truncated soluble HLA-G5 (211, 214). Further, many studies have interchangeably used peripheral blood NK cells or NK-like cell lines as surrogate readouts of HLA-G-uNK cell interaction within the maternal-fetal interface. These assumptions have perhaps contributed to over-interpretation of the role of HLA-G in controlling uNK cell-related processes in pregnancy. Conflicting findings have arisen from these studies, indicating that both membrane-bound and soluble HLA-G enhance the production of pro-inflammatory (IFN-γ, TNF, IL1B, IL6) and angiogenic (IL8) factors in endometrial (i.e., not decidual) (213, 215) or pbNK cells (202, 216). In support of the above findings using pbNK cells, Li et al. demonstrated that HLA-G treatment of ex vivo-derived uNK cells induces IL6, IL8, and TNF through processes dependent upon KIR2DL4 (212). In contrast to this, ex vivo uNK cells, exposed to membrane-bound HLA-G, resulted in inhibition or had marginal impact on uNK degranulation and cytokine production (214). Two recent studies, directly examining the function of HLA-G in primary EVTs and uNK cells, indicated that HLA-G does not induce cytokine secretion from uNK cells but instead dampens uNK cell activity (104, 193), a finding that is consistent with the original paradigm that HLA-G likely promotes immune cell tolerance within the fetal-maternal interface.
Regulation of Extravillous Trophoblasts by Uterine Natural Killer Cells
Uterine NK are robust producers of cytokines and growth factors, and due to their close proximity to EVTs within the maternal-fetal interface, these cells likely play roles in regulating the diverse functions of trophoblasts. In support of this, uNK cell-generated conditioned media modifies specific biological processes of primary trophoblast, including the promotion of cell invasion (153, 154, 217). Notably, the pro-invasive effect of uNK cell-derived conditioned media is solely attributed to soluble factors produced by uNK cells harvested from later gestational age time-points within the first trimester of pregnancy (i.e., 10–13 weeks' gestation). By contrast, soluble factors produced by uNK cells from the earlier first trimester time-points do not elicit pro-invasive characteristics on EVTs, indicating that the composition of uNK cell factors is influenced by processes related to gestational age, and that the impact of uNK cells on EVT biology is relative to stage of development.
Multiple factors produced by uNK cells have been identified, and importantly, receptors for many of these substances are expressed on primary EVTs. For example, uNK cells produce high levels of IL8, TNF, interferon (INF) γ, TGFB1, CXCL10, as well as the angiogenic factors such as vascular endothelial growth factor A (VEGF-A), VEGF-C and PGF (153). Reciprocally, immunolocalization studies on implantation sites and ex vivo studies using primary EVTs provide evidence that invasive trophoblasts populating the maternal-fetal interface produce receptors for these ligands. For example, EVTs express CXCR1 (an IL8 receptor), CXCR3 (an CXCL10 receptor), TNFR1, as well as VEGFR-1 and VEGFR-3, the latter binding VEGF-A and VEGF-C, respectively (110, 153, 218, 219). Notably, supplementation of IL8 and CXCL10 promoted migration of primary CTBs (153). Likewise, inhibition of ligand binding to VEGFR-1 and VEGFR-3 diminished trophoblast invasion (220). Moreover, downregulation of VEGF in uNK cell-conditioned media impaired EVT outgrowth compared to controls (221). In contrast, TNF and IFN-γ inhibited trophoblast migration and invasion by increasing PAI expression and impacting MMP-directed proteolysis, respectively (222, 223). Taken together, factors produced by uNK cells do have the ability to control EVT-related processes in vivo. Given the interplay between promoting and restraining invasive characteristics in EVTs, uNK cells could be an important cellular component of the decidua that controls depth of EVT invasion as well as extent of trophoblast-mediated spiral artery remodeling.
Can the Breakdown of Maternal-Fetal Tolerance be Related to Uterine Natural Killer Cells Dysfunction?
Much research related to uNK-trophoblast interactions has centered on the possibility that uNK cells, activated by infection or inflammation, may mount cytotoxic responses toward the semi-allogeneic fetus and trophoblast, thus contributing to infection-related miscarriage and other pregnancy disorders with aberrant inflammation. Surprisingly, most well designed studies, utilizing primary syngeneic uNK cell and trophoblast co-cultures, provided convincing evidence that trophoblasts (both HLA-G- and non-HLA-G-expressing trophoblasts) are highly resistant to uNK-directed killing (193, 224). Although uNK cells do not target trophoblasts for killing, even when artificially activated, uNK still retain pro-cytotoxic features (i.e. granzyme, perforin) that enable efficient cellular immune responses against virally-infected maternal uterine stromal cells, highlighting that trophoblasts are immuno-privileged (193, 225). Nonetheless, research in mice suggests that uNK cells can adopt anti-trophoblast characteristics in the right context. For example, aberrantly activated uNK cells in response to inflammation induced by bacterial endotoxin (183) or alloimmunogenic responses (184) target fetal tissues, including the placenta, and induce fetal resorption. These uNK-driven processes lead to impairments in uterine artery remodeling and placental sufficiency, and can be reversed through genetic ablation strategies (i.e., IL15−/−) (183) or antibody-directed inhibition of uNK cells (184). It has also been suggested that uNK cell numbers are altered in pregnancy complications such as PE and IUGR, although contradictory data have been published. Reduced numbers of uNK cells in pregnancies with IUGR have been consistently demonstrated using different methods (226–229). Some studies also suggest a decrease of uNK cells (229) or of the CD56+/CD16+ uNK subset (228) in PE compared to healthy pregnancies. In contrast, others describe an elevated number of total uNK cells or of the CD56+/CD16+ subset in PE (230, 231). More recently, research has identified pre-existing health conditions of the mother that associate with low-grade inflammation, like obesity, that potentiate uNK cell activity and modify how uNK cells interact with fetal MHC ligand (207). However, although the in utero environment likely shapes uNK cell processes, to date, hard evidence showing that aberrantly activated uNK cells in humans directly target trophoblasts for killing has yet to be clearly demonstrated.
Regulation of Extravillous Trophoblasts by Decidual Macrophages
Next to uterine natural killer cells, macrophages are thought to comprise the second largest leukocyte population within the decidua (168, 232). Besides suggested contributions to spiral artery remodeling and immune modulation (12, 233), the function of decidual macrophages, in particular their effect on EVT activity, remains largely unknown. Although historically described for their function in immune defense, inflammation, and clearance of apoptotic cells, macrophages have also been recognized to play important roles in the development, homeostasis, and repair of various tissues (234).
Macrophages are usually classified into M1, representing a classical pro-inflammatory, anti-microbial activation or into M2, referring to an anti-inflammatory phenotype promoting wound healing. A body of growing evidence however suggests that M1 and M2 rather represent two extreme poles of a broad spectrum of macrophage polarization (235). The non-exclusivity of M1 and M2 macrophage phenotypes in vivo has likely several reasons. Firstly, it has been shown that macrophage activation statuses are reversible when specific stimuli change. Secondly, macrophages are often exposed to opposing activating signals in vivo (236). For instance, the co-existence of pro-inflammatory M1 and anti-inflammatory M2 profiles has been demonstrated in various mouse models and during tumor progression (237, 238). In light of these data it is not surprising that decidual macrophages also show a unique activation status with a dominating but not exclusive M2 phenotype. While decidual macrophages express typical M2 markers such as CD209 and CD206 and secrete the anti-inflammatory cytokines IL10 and TGFB, they were also shown to secrete pro-inflammatory cytokines including IL6 and TNF and the neutrophil chemoattractant CXCL8 (IL8) (239, 240). Nevertheless, the anti-inflammatory M2-related cytokines IL10 and M-CSF were shown to be important for inducing a decidual macrophage phenotype in peripheral blood monocytes (240). In addition, decidual macrophages were able to suppress T cell activity and induce Tregs in vitro (194, 241), which further strengthens the notion of an M2-dominated function. Two independent studies suggested the presence of two distinct decidual macrophage subpopulations, characterized by the absence or presence of the cell surface markers CD11c and ICAM3 (239, 240). Expression of these markers may at least partly relate to immature macrophages or blood contamination, as high levels of CD11c and ICAM3 are also found in blood monocytes. More recently, a study described three decidual macrophage subtypes, based on the expression or absence of CCR2 and CD11c. Using RNA sequencing and functional assays, the authors identified distinct functional states, including differences in phagocytosis, anti-oxidative, and anti-inflammatory activities, and proximity to EVTs (242). Whether these differences indeed account for unique subpopulations or reflect cellular plasticity and thus relate to phenotypical alterations awaits further clarification.
Due to their high abundance in the decidua, it is conceivable that macrophages markedly influence the local paracrine environment and thus EVT function. As follows, it is interesting to note that decidual macrophages are more abundant at the site of implantation and accumulate at the invasive front of EVTs (243, 244). There is growing evidence for a macrophage-guided growth-promoting function in various epithelia (245–247). Interestingly, both placental and decidual macrophages could promote proximal cell column proliferation by secreting the M2-associated (248, 249) factors IL33 and Wnt5a (167, 250). These data suggest that paracrine activity of decidual macrophages could be important for the initial steps in EVT formation. Furthermore, decidual macrophages have been shown to secrete a range of factors known to alter EVT motility (239), albeit with conflicting evidence as to whether they promote or restrict EVT invasion. For instance, while IL8 (153) was described as pro-invasive factor in the context of trophoblast migration, TNF (222) and IL10 (119) have been shown to inhibit EVT motility. The net effect of these factors on EVTs may be pro-invasive, anti-invasive, or neither. In addition, it is unclear under which circumstances decidual macrophages produce these opposing cytokines in terms of macrophage polarization and EVT response. Phenotypic macrophage polarization is controlled by a complex array of soluble factors provided by the local microenvironment and even dictated by extracellular matrix-dependent cell morphology (251). Moreover, ligand distribution within tissues is limited by a wide variety of factors, including limited diffusion capacities, endocytosis, and interaction with extracellular matrix proteins. It is therefore also important to consider the spatial relationship between macrophages and EVTs.
Unfortunately, there is limited information on the difference in macrophage distribution between decidua basalis and parietalis, and especially on whether these different tissue compartments harbor specific macrophage phenotypes. Immunohistochemical studies provided evidence for an enrichment of macrophages in the decidua basalis (243, 244), which has recently been confirmed via flow cytometric analysis (175), suggesting that macrophages preferentially accumulate in the vicinity of EVTs. In a similar context, it has been shown that binding between HLA-G homodimers and macrophage-associated leukocyte immunoglobulin-like receptor B1 (LILRB1) upregulates secretion of IL6, IL8, and TNF (212). Although it is not clear whether these effects relate to membrane-bound or soluble HLA-G, the presence of EVTs likely influences the macrophage phenotype and thus could substantially influence the paracrine activity of decidual macrophages.
Are Decidual Macrophages Altered in Complicated Pregnancies?
Complicated pregnancies with IUGR or early-onset PE have repeatedly been associated with compromised EVT function. Several studies have tried to decipher whether aberrations in the decidual macrophage population could mediate these EVT defects, albeit with conflicting results. Some studies suggest an increase in macrophage numbers in preeclampsia compared to healthy pregnancies (252, 253). Further, an inverse relationship between macrophage infiltration and invasion of trophoblasts into arteries, with a shift toward macrophage infiltration in preeclamptic pregnancies, was shown (252). On the contrary, other studies point toward a decrease in the number of macrophages in IUGR and PE, compared to healthy pregnancies (229, 254). Still some additional studies found no significant differences in macrophage distribution and activation patterns between preeclamptic women and preterm labor controls (255).
In addition, it is unclear whether aberrant macrophage polarization could be associated with EVT defects in the development of placental pathologies. In detail, some evidence exists that macrophages could display a more M1-like polarization in cases of PE, resulting in an exacerbated production of pro-inflammatory cytokines adversely affecting EVT function (256, 257). For instance, TNF has been shown to inhibit trophoblast motility in villous explant cultures (222). A study utilizing a rat model, demonstrating that systematic LPS injection results in IUGR and PE-like symptoms, also reported increased levels of TNF and exacerbated numbers of uteroplacental macrophages (258). Unfortunately, the authors did not further elucidate whether LPS-induced systemic inflammation also changes the phenotype of decidual macrophages. Nevertheless, whether a shift toward a pro-inflammatory M1-like macrophage phenotype indeed adversely affects EVT function has not been proven. As mentioned above, M2-related anti-inflammatory cytokines such as TGFB or IL10 were also reported to exert adverse effects on EVTs by restricting their migratory potential. Conversely, macrophages isolated from miscarriages showed reduced expression of IL6 and IL8, the latter with pro-invasive potential toward EVTs (153). In summary, studies investigating the functional interplay between primary macrophage and EVT cultures are scarce. Thus, the particular role of macrophages in the context of EVT function remains unclear both in healthy and complicated pregnancies. Unfortunately, there is also limited availability of suitable human in vitro systems to study this interaction. Immortalized monocytic cell lines, such as THP-1 cells, do not represent a useful model system for decidual macrophages due to their massive genomic rearrangements and their phenotypic and functional differences (259). It is still unclear whether the decidua-specific macrophage phenotype can be sustained in isolated primary cells or mimicked by controlled differentiation of peripheral blood monocytes in vitro. Moreover, more information is needed on whether macrophages change their polarization and secretory profile depending on their location within the human decidua. Finally, the long lasting paradigm of macrophage differentiation from recruited monocytes has been challenged by numerous studies demonstrating that macrophages are also maintained throughout adult life by a tissue-resident, proliferative population originating from embryonic or yolk sac-derived precursors (260, 261). In mice, tissue-resident macrophage populations, such as liver Kupffer cells (262), epidermal Langerhans cells (263), microglia (264), and pleural macrophages (265), were shown to be able to proliferate and renew independently from the bone marrow. Although very few data have been generated so far to confirm the existence of tissue-resident macrophages in humans, it is interesting to note that decidual, tissue-resident CD34+ stromal cells were described to differentiate into functional CD56+ uNK cells (266). Moreover, a recent study shows that continuous pregnancies induce a pregnancy-promoting memory uNK subset which differentiates from progenitors residing in the post-gravid endometrium (267). Additional studies, confirming the existence of tissue-resident NK cells and T cells in other tissues, strengthen the idea of an organ-specific immunity that is maintained independently of the bone marrow and secondary lymph nodes (268, 269). Intriguingly, several reports describe the proliferative signature of decidual macrophages (270, 271), supporting the idea that tissue-resident macrophages could be maintained in the uterus by local proliferation. On the other hand, both EVTs and decidual macrophages produce monocyte-recruiting chemokines, such as CCL2 (148, 149) or CCL4 (148, 239) suggesting a contribution of monocytes to the pool of decidual macrophages in early pregnancy. In light of these data it is still not clear whether the endometrium is continuously populated by bone marrow-derived monocytes or whether local progenitors mainly repopulate within the tissue.
Summary
The influence of soluble decidual factors on trophoblast proliferation, migration and invasion has been intensively investigated using different trophoblast cell models including cell lines and primary CTBs containing a mixture of different trophoblast subtypes. However, studies about the effects of secreted proteins on cell column proliferation of the anchoring villus are scarce. Moreover, isolated primary CCTs rapidly cease proliferation in culture and undergo differentiation (57), impairing their usability for proliferation assays. Hence, only few factors were convincingly shown to promote CCT expansion using first trimester villous explant cultures (Figure 2). Recently however, self-renewing trophoblast stem cells and organoids have been developed (64, 272). These culture systems should allow more reliable investigations on the role of decidual growth factors in trophoblast progenitor growth, EVT formation and differentiation. Furthermore, DFs, macrophages and uNK cells express a plethora of cytokines, chemokines and soluble factors, some of which are detectable in more than one cell type (Figure 3). Although the majority of these proteins likely control trophoblast invasion and/or migration the prime decidual target cell of an individual factor remains elusive. Besides their presumptive role in trophoblast motility, chemokines and cytokines could regulate immune cell recruitment and mutual activation of macrophages, uNK cells and DFs as well as other less abundant immune cells of the fetal-maternal interface.
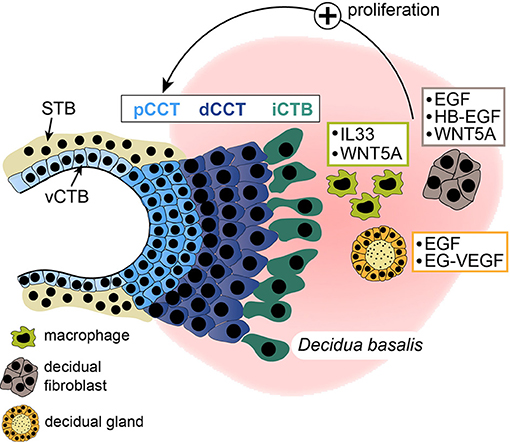
Figure 2. Schematical depiction of soluble factors secreted from decidual macrophages, stromal cells, or glands. Mediators, stimulating proliferation of proximal cell column trophoblasts (pCCTs) in villous explant cultures are illustrated. dCCT, distal cell column trophoblast; STB, syncytiotrophoblast; vCTB, villous cytotrophoblast; iCTB, interstitial cytotrophoblast.
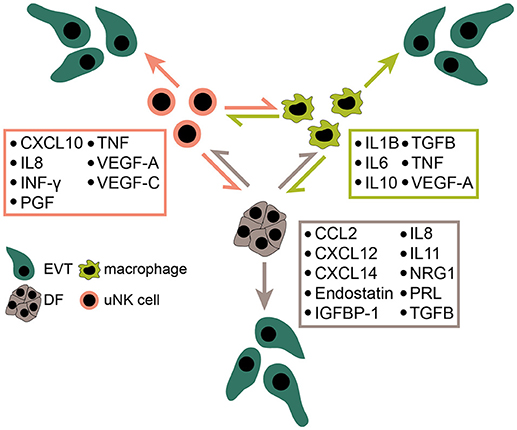
Figure 3. Expression of decidual chemokines, cytokines and other soluble mediators affecting trophoblast migration and/or invasion. Some of the depicted factors likely play numerous roles at the fetal-maternal interface including activation and maturation of immune cells, as well as decidual angiogenesis and spiral artery remodeling. EVT, extravillous trophoblast; uNK cell, uterine natural killer cell; DF, decidual fibroblast.
Author Contributions
The manuscript was written by JP, AGB, and MK and edited by SV and JB. JP provided the graphical illustrations.
Funding
The present work was supported by Austrian Science Fund (grant P-28417-B30 to MK), the Austrian National Bank (grant 17613 to JP) and a Canadian Institutes of Health Research (CIHR) Open Operating Grant (201403MOP-325905-CIA-CAAA) to AGB.
Conflict of Interest Statement
The authors declare that the research was conducted in the absence of any commercial or financial relationships that could be construed as a potential conflict of interest.
References
1. Hamilton WJ, Boyd JD. Development of the human placenta in the first three months of gestation. J Anat (1960) 94:297–328.
2. Castellucci M, Scheper M, Scheffen I, Celona A, Kaufmann P. The development of the human placental villous tree. Anat Embryol. (1990) 181:117–28. doi: 10.1007/BF00198951
3. Jones CJ, Fox H. Ultrastructure of the normal human placenta. Electron Microsc Rev. (1991) 4:129–78. doi: 10.1016/0892-0354(91)90019-9
4. Evain-Brion D, Malassine A. Human placenta as an endocrine organ. Growth Horm IGF Res (2003) 13 (Suppl. A):S34–37. doi: 10.1016/S1096-6374(03)00053-4
5. Aplin JD. Developmental cell biology of human villous trophoblast: current research problems. Int J Dev Biol. (2010) 54:323–9. doi: 10.1387/ijdb.082759ja
6. Burton GJ, Jauniaux E, Charnock-Jones DS. The influence of the intrauterine environment on human placental development. Int J Dev Biol. (2010) 54:303–12. doi: 10.1387/ijdb.082764gb
7. Pijnenborg R, Bland JM, Robertson WB, Brosens I. Uteroplacental arterial changes related to interstitial trophoblast migration in early human pregnancy. Placenta (1983) 4:397–413. doi: 10.1016/S0143-4004(83)80043-5
8. Pijnenborg R, Dixon G, Robertson WB, Brosens I. Trophoblastic invasion of human decidua from 8 to 18 weeks of pregnancy. Placenta (1980) 1:3–19. doi: 10.1016/S0143-4004(80)80012-9
9. Hustin J, Jauniaux E, Schaaps JP. Histological study of the materno-embryonic interface in spontaneous abortion. Placenta (1990) 11:477–86. doi: 10.1016/S0143-4004(05)80193-6
10. Brosens I, Robertson WB, Dixon HG. The physiological response of the vessels of the placental bed to normal pregnancy. J Pathol Bacteriol.(1967) 93:569–79. doi: 10.1002/path.1700930218
11. Pijnenborg R, Vercruysse L, Hanssens M. The uterine spiral arteries in human pregnancy: facts and controversies. Placenta (2006) 27:939–58. doi: 10.1016/j.placenta.2005.12.006
12. Harris LK. IFPA gabor than award lecture: transformation of the spiral arteries in human pregnancy: key events in the remodelling timeline. Placenta (2011) 32 (Suppl. 2):S154–8. doi: 10.1016/j.placenta.2010.11.018
13. King A, Wellings V, Gardner L, Loke YW. Immunocytochemical characterization of the unusual large granular lymphocytes in human endometrium throughout the menstrual cycle. Hum Immunol. (1989) 24:195–205. doi: 10.1016/0198-8859(89)90060-8
14. Smith SD, Dunk CE, Aplin JD, Harris LK, Jones RL. Evidence for immune cell involvement in decidual spiral arteriole remodeling in early human pregnancy. Am J Pathol. (2009) 174:1959–71. doi: 10.2353/ajpath.2009.080995
15. Zhou Y, Fisher SJ, Janatpour M, Genbacev O, Dejana E, Wheelock M, et al. Human cytotrophoblasts adopt a vascular phenotype as they differentiate. A strategy for successful endovascular invasion? J Clin Invest. (1997) 99:2139–51.
16. Khong TY, De Wolf F, Robertson WB, Brosens I. Inadequate maternal vascular response to placentation in pregnancies complicated by pre-eclampsia and by small-for-gestational age infants. Br J Obstet Gynaecol. (1986) 93:1049–59. doi: 10.1111/j.1471-0528.1986.tb07830.x
17. Pijnenborg R, Anthony J, Davey DA, Rees A, Tiltman A, Vercruysse L, et al. Placental bed spiral arteries in the hypertensive disorders of pregnancy. Br J Obstet Gynaecol. (1991) 98:648–55. doi: 10.1111/j.1471-0528.1991.tb13450.x
18. Romero R, Kusanovic JP, Chaiworapongsa T, Hassan SS. Placental bed disorders in preterm labor, preterm PROM, spontaneous abortion and abruptio placentae. Best Pract Res Clin Obstet Gynaecol. (2011) 25:313–27. doi: 10.1016/j.bpobgyn.2011.02.006
19. Fisher SJ. Why is placentation abnormal in preeclampsia? Am J Obstet Gynecol.(2015) 213:S115–122. doi: 10.1016/j.ajog.2015.08.042
20. Zhou Y, Damsky CH, Fisher SJ. Preeclampsia is associated with failure of human cytotrophoblasts to mimic a vascular adhesion phenotype. One cause of defective endovascular invasion in this syndrome? J Clin Invest (1997) 99:2152–64.
21. Zhou Y, Gormley MJ, Hunkapiller NM, Kapidzic M, Stolyarov Y, Feng V, et al. Reversal of gene dysregulation in cultured cytotrophoblasts reveals possible causes of preeclampsia. J Clin Invest. (2013) 123:2862–72. doi: 10.1172/JCI66966
22. Moffett A, Colucci F. Uterine NK cells: active regulators at the maternal-fetal interface. J Clin Invest. (2014) 124:1872–9. doi: 10.1172/JCI68107
23. Schatz F, Guzeloglu-Kayisli O, Arlier S, Kayisli UA, Lockwood CJ. The role of decidual cells in uterine hemostasis, menstruation, inflammation, adverse pregnancy outcomes and abnormal uterine bleeding. Hum Reprod Update (2016) 22:497–515. doi: 10.1093/humupd/dmw004
24. Burton GJ, Jauniaux E. The cytotrophoblastic shell and complications of pregnancy. Placenta (2017) 60:134–9. doi: 10.1016/j.placenta.2017.06.007
25. He N, van Iperen L, de Jong D, Szuhai K, Helmerhorst FM, van der Westerlaken LA, et al. Human extravillous trophoblasts penetrate decidual veins and lymphatics before remodeling spiral arteries during early pregnancy. PLoS ONE (2017) 12:e0169849. doi: 10.1371/journal.pone.0169849
26. Windsperger K, Dekan S, Pils S, Golletz C, Kunihs V, Fiala C, et al. Extravillous trophoblast invasion of venous as well as lymphatic vessels is altered in idiopathic, recurrent, spontaneous abortions. Hum Reprod. (2017) 32:1208–17. doi: 10.1093/humrep/dex058
27. Moser G, Weiss G, Sundl M, Gauster M, Siwetz M, Lang-Olip I, et al. Extravillous trophoblasts invade more than uterine arteries: evidence for the invasion of uterine veins. Histochem Cell Biol. (2017) 147:353–66. doi: 10.1007/s00418-016-1509-5
28. Moser G, Weiss G, Gauster M, Sundl M, Huppertz B. Evidence from the very beginning: endoglandular trophoblasts penetrate and replace uterine glands in situ and in vitro. Hum Reprod (2015) 30:2747–57. doi: 10.1093/humrep/dev266
29. Bulmer JN, Williams PJ, Lash GE. Immune cells in the placental bed. Int J Dev Biol. (2010) 54:281–94. doi: 10.1387/ijdb.082763jb
30. Wallace AE, Fraser R, Cartwright JE. Extravillous trophoblast and decidual natural killer cells: a remodelling partnership. Hum Reprod Update (2012) 18:458–71. doi: 10.1093/humupd/dms015
31. Hiby SE, Walker JJ, O'Shaughnessy KM, Redman CW, Carrington M, Trowsdale J, et al. Combinations of maternal KIR and fetal HLA-C genes influence the risk of preeclampsia and reproductive success. J Exp Med. (2004) 200:957–965. doi: 10.1084/jem.20041214
32. Hiby SE, Regan L, Lo W, Farrell L, Carrington M, Moffett A. Association of maternal killer-cell immunoglobulin-like receptors and parental HLA-C genotypes with recurrent miscarriage. Hum Reprod. (2008) 23:972–6. doi: 10.1093/humrep/den011
33. Hanna J, Mandelboim O. When killers become helpers. Trends Immunol. (2007) 28:201–6. doi: 10.1016/j.it.2007.03.005
34. Manaster I, Mandelboim O. The unique properties of uterine NK cells. Am J Reprod Immunol. (2010) 63:434–44. doi: 10.1111/j.1600-0897.2009.00794.x
35. Moffett A, Colucci F. Co-evolution of NK receptors and HLA ligands in humans is driven by reproduction. Immunol Rev. (2015) 267:283–97. doi: 10.1111/imr.12323
36. Moffett A, Chazara O, Colucci F. Maternal allo-recognition of the fetus. Fertil Steril. (2017) 107:1269–72. doi: 10.1016/j.fertnstert.2017.05.001
37. Knofler M. Critical growth factors and signalling pathways controlling human trophoblast invasion. Int J Dev Biol. (2010) 54:269–80. doi: 10.1387/ijdb.082769mk
38. Knofler M, Pollheimer J. IFPA Award in Placentology lecture: molecular regulation of human trophoblast invasion. Placenta (2012) 33 (Suppl.):S55–62. doi: 10.1016/j.placenta.2011.09.019
39. Kovats S, Main EK, Librach C, Stubblebine M, Fisher SJ, DeMars R. A class I antigen, HLA-G, expressed in human trophoblasts. Science (1990) 248:220–3. doi: 10.1126/science.2326636
40. Pollheimer J, Loregger T, Sonderegger S, Saleh L, Bauer S, Bilban M, et al. Activation of the canonical wingless/T-cell factor signaling pathway promotes invasive differentiation of human trophoblast. Am J Pathol. (2006) 168:1134–47. doi: 10.2353/ajpath.2006.050686
41. Damsky CH, Fitzgerald ML, Fisher SJ. Distribution patterns of extracellular matrix components and adhesion receptors are intricately modulated during first trimester cytotrophoblast differentiation along the invasive pathway, in vivo. J Clin Invest. (1992) 89:210–22. doi: 10.1172/JCI115565
42. Plessl K, Haider S, Fiala C, Pollheimer J, Knofler M. Expression pattern and function of Notch2 in different subtypes of first trimester cytotrophoblast. Placenta (2015) 36:365–71. doi: 10.1016/j.placenta.2015.01.009
43. Fock V, Plessl K, Draxler P, Otti GR, Fiala C, Knofler M, et al. Neuregulin-1-mediated ErbB2-ErbB3 signalling protects human trophoblasts against apoptosis to preserve differentiation. J Cell Sci. (2015) 128:4306–16. doi: 10.1242/jcs.176933
44. Aghababaei M, Perdu S, Irvine K, Beristain AG. A disintegrin and metalloproteinase 12 (ADAM12) localizes to invasive trophoblast, promotes cell invasion and directs column outgrowth in early placental development. Mol Hum Reprod. (2013) 20:235–49. doi: 10.1093/molehr/gat084
45. Biadasiewicz K, Fock V, Dekan S, Proestling K, Velicky P, Haider S, et al. Extravillous trophoblast-associated ADAM12 exerts pro-invasive properties, including induction of integrin beta 1-mediated cellular spreading. Biol Reprod. (2014) 90:101. doi: 10.1095/biolreprod.113.115279
46. Velicky P, Windsperger K, Petroczi K, Pils S, Reiter B, Weiss T, et al. Pregnancy-associated diamine oxidase originates from extravillous trophoblasts and is decreased in early-onset preeclampsia. Sci Rep. (2018) 8:6342. doi: 10.1038/s41598-018-24652-0
47. Liu Y, Fan X, Wang R, Lu X, Dang YL, Wang H, et al. Single-cell RNA-seq reveals the diversity of trophoblast subtypes and patterns of differentiation in the human placenta. Cell Res (2018) 28:819–32. doi: 10.1038/s41422-018-0066-y
48. Genbacev O, Jensen KD, Powlin SS, Miller RK. In vitro differentiation and ultrastructure of human extravillous trophoblast (EVT) cells. Placenta (1993) 14:463–75. doi: 10.1016/S0143-4004(05)80466-7
49. Vicovac L, Jones CJ, Aplin JD. Trophoblast differentiation during formation of anchoring villi in a model of the early human placenta in vitro. Placenta (1995) 16:41–56. doi: 10.1016/0143-4004(95)90080-2
50. Pollheimer J, Haslinger P, Fock V, Prast J, Saleh L, Biadasiewicz K, et al. Endostatin suppresses IGF-II-mediated signaling and invasion of human extravillous trophoblasts. Endocrinology (2011) 152:4431–42. doi: 10.1210/en.2011-1196
51. Lim KH, Zhou Y, Janatpour M, McMaster M, Bass K, Chun SH, et al. Human cytotrophoblast differentiation/invasion is abnormal in pre-eclampsia. Am J Pathol. (1997) 151:1809–18.
52. Goffin F, Munaut C, Malassine A, Evain-Brion D, Frankenne F, Fridman V, et al. Evidence of a limited contribution of feto-maternal interactions to trophoblast differentiation along the invasive pathway. Tissue Antigens (2003) 62:104–16. doi: 10.1034/j.1399-0039.2003.00085.x
53. Red-Horse K, Rivera J, Schanz A, Zhou Y, Winn V, Kapidzic M, et al. Cytotrophoblast induction of arterial apoptosis and lymphangiogenesis in an in vivo model of human placentation. J Clin Invest. (2006) 116:2643–52. doi: 10.1172/JCI27306
54. Bilban M, Haslinger P, Prast J, Klinglmuller F, Woelfel T, Haider S, et al. Identification of novel trophoblast invasion-related genes: heme oxygenase-1 controls motility via peroxisome proliferator-activated receptor gamma. Endocrinology (2009) 150:1000–13. doi: 10.1210/en.2008-0456
55. Apps R, Sharkey A, Gardner L, Male V, Trotter M, Miller N, et al. Genome-wide expression profile of first trimester villous and extravillous human trophoblast cells. Placenta (2011) 32:33–43. doi: 10.1016/j.placenta.2010.10.010
56. Haider S, Meinhardt G, Velicky P, Otti GR, Whitley G, Fiala C, et al. Notch signaling plays a critical role in motility and differentiation of human first-trimester cytotrophoblasts. Endocrinology (2014) 155:263–74. doi: 10.1210/en.2013-1455
57. Haider S, Meinhardt G, Saleh L, Fiala C, Pollheimer J, Knofler M. Notch1 controls development of the extravillous trophoblast lineage in the human placenta. Proc Natl Acad Sci USA. (2016) 113:E7710–E7719. doi: 10.1073/pnas.1612335113
58. Rodesch F, Simon P, Donner C, Jauniaux E. Oxygen measurements in endometrial and trophoblastic tissues during early pregnancy. Obstet Gynecol. (1992) 80:283–5.
59. Wakeland AK, Soncin F, Moretto-Zita M, Chang CW, Horii M, Pizzo D, et al. Hypoxia directs human extravillous trophoblast differentiation in a hypoxia-inducible factor-dependent manner. Am J Pathol. (2017) 187:767–80. doi: 10.1016/j.ajpath.2016.11.018
60. James JL, Stone PR, Chamley LW. The regulation of trophoblast differentiation by oxygen in the first trimester of pregnancy. Hum Reprod Update (2006) 12:137–44. doi: 10.1093/humupd/dmi043
61. Tuuli MG, Longtine MS, Nelson DM. Review: oxygen and trophoblast biology–a source of controversy. Placenta (2011) 32 (Suppl. 2):S109–18. doi: 10.1016/j.placenta.2010.12.013
62. Soares MJ, Iqbal K, Kozai K. Hypoxia and placental development. Birth Defects Res. (2017) 109:1309–29. doi: 10.1002/bdr2.1135
63. Chang CW, Wakeland AK, Parast MM. Trophoblast lineage specification, differentiation and their regulation by oxygen tension. J Endocrinol. (2018) 236:R43–56. doi: 10.1530/JOE-17-0402
64. Haider S, Meinhardt G, Saleh L, Kunihs V, Gamperl M, Kaindl U, et al. Self-renewing trophoblast organoids recapitulate the developmental program of the early human placenta. Stem Cell Reports (2018) 11:537–51. doi: 10.1016/j.stemcr.2018.07.004
65. Ramsey EM, Houston ML, Harris JW. Interactions of the trophoblast and maternal tissues in three closely related primate species. Am J Obstet Gynecol. (1976) 124:647–52. doi: 10.1016/0002-9378(76)90068-5
66. Emera D, Romero R, Wagner G. The evolution of menstruation: a new model for genetic assimilation: explaining molecular origins of maternal responses to fetal invasiveness. Bioessays (2012) 34:26–35. doi: 10.1002/bies.201100099
67. Gellersen B, Brosens IA, Brosens JJ. Decidualization of the human endometrium: mechanisms, functions, and clinical perspectives. Semin Reprod Med. (2007) 25:445–53. doi: 10.1055/s-2007-991042
68. Burton GJ, Watson AL, Hempstock J, Skepper JN, Jauniaux E. Uterine glands provide histiotrophic nutrition for the human fetus during the first trimester of pregnancy. J Clin Endocrinol Metab. (2002) 87:2954–9. doi: 10.1210/jcem.87.6.8563
69. Hempstock J, Cindrova-Davies T, Jauniaux E, Burton GJ. Endometrial glands as a source of nutrients, growth factors and cytokines during the first trimester of human pregnancy: a morphological and immunohistochemical study. Reprod Biol Endocrinol. (2004) 2:58. doi: 10.1186/1477-7827-2-58
70. Burton GJ, Jauniaux E, Charnock-Jones DS. Human early placental development: potential roles of the endometrial glands. Placenta (2007) 28 (Suppl. A):S64–69. doi: 10.1016/j.placenta.2007.01.007
71. Charnock-Jones DS, Sharkey AM, Fenwick P, Smith SK. Leukaemia inhibitory factor mRNA concentration peaks in human endometrium at the time of implantation and the blastocyst contains mRNA for the receptor at this time. J Reprod Fertil. (1994) 101:421–6. doi: 10.1530/jrf.0.1010421
72. Cullinan EB, Abbondanzo SJ, Anderson PS, Pollard JW, Lessey BA, Stewart CL. Leukemia inhibitory factor (LIF) and LIF receptor expression in human endometrium suggests a potential autocrine/paracrine function in regulating embryo implantation. Proc Natl Acad Sci U S A (1996) 93:3115–20. doi: 10.1073/pnas.93.7.3115
73. Hornung D, Lebovic DI, Shifren JL, Vigne JL, Taylor RN. Vectorial secretion of vascular endothelial growth factor by polarized human endometrial epithelial cells. Fertil Steril. (1998) 69:909–15. doi: 10.1016/S0015-0282(98)00044-2
74. Ngan ES, Lee KY, Yeung WS, Ngan HY, Ng EH, Ho PC. Endocrine gland-derived vascular endothelial growth factor is expressed in human peri-implantation endometrium, but not in endometrial carcinoma. Endocrinology (2006) 147:88–95. doi: 10.1210/en.2005-0543
75. Brouillet S, Murthi P, Hoffmann P, Salomon A, Sergent F, De Mazancourt P, et al. EG-VEGF controls placental growth and survival in normal and pathological pregnancies: case of fetal growth restriction (FGR). Cell Mol Life Sci. (2013) 70:511–25. doi: 10.1007/s00018-012-1141-z
76. Athanassiades A, Hamilton GS, Lala PK. Vascular endothelial growth factor stimulates proliferation but not migration or invasiveness in human extravillous trophoblast. Biol Reprod. (1998) 59:643–54. doi: 10.1095/biolreprod59.3.643
77. Bischof P, Haenggeli L, Campana A. Effect of leukemia inhibitory factor on human cytotrophoblast differentiation along the invasive pathway. Am J Reprod Immunol. (1995) 34:225–30. doi: 10.1111/j.1600-0897.1995.tb00945.x
78. Nachtigall MJ, Kliman HJ, Feinberg RF, Olive DL, Engin O, Arici A. The effect of leukemia inhibitory factor (LIF) on trophoblast differentiation: a potential role in human implantation. J Clin Endocrinol Metab. (1996) 81:801–6.
79. Paiva P, Menkhorst E, Salamonsen L, Dimitriadis E. Leukemia inhibitory factor and interleukin-11: critical regulators in the establishment of pregnancy. Cytokine Growth Factor Rev. (2009) 20:319–28. doi: 10.1016/j.cytogfr.2009.07.001
80. Gellersen B, Brosens JJ. Cyclic decidualization of the human endometrium in reproductive health and failure. Endocr Rev. (2014) 35:851–905. doi: 10.1210/er.2014-1045
81. Kajihara T, Jones M, Fusi L, Takano M, Feroze-Zaidi F, Pirianov G, et al. Differential expression of FOXO1 and FOXO3a confers resistance to oxidative cell death upon endometrial decidualization. Mol Endocrinol. (2006) 20:2444–55. doi: 10.1210/me.2006-0118
82. Kajihara T, Uchino S, Suzuki M, Itakura A, Brosens JJ, Ishihara O. Human chorionic gonadotropin confers resistance to oxidative stress-induced apoptosis in decidualizing human endometrial stromal cells. Fertil Steril. (2011) 95:1302–7. doi: 10.1016/j.fertnstert.2010.05.048
83. Aplin JD, Charlton AK, Ayad S. An immunohistochemical study of human endometrial extracellular matrix during the menstrual cycle and first trimester of pregnancy. Cell Tissue Res. (1988) 253:231–40. doi: 10.1007/BF00221758
84. Church HJ, Vicovac LM, Williams JD, Hey NA, Aplin JD. Laminins 2 and 4 are expressed by human decidual cells. Lab Invest. (1996) 74:21–32.
85. Xu G, Guimond MJ, Chakraborty C, Lala PK. Control of proliferation, migration, and invasiveness of human extravillous trophoblast by decorin, a decidual product. Biol Reprod. (2002) 67:681–9. doi: 10.1095/biolreprod67.2.681
86. Winship A, Cuman C, Rainczuk K, Dimitriadis E. Fibulin-5 is upregulated in decidualized human endometrial stromal cells and promotes primary human extravillous trophoblast outgrowth. Placenta (2015) 36:1405–11. doi: 10.1016/j.placenta.2015.10.008
87. Harris LK, Jones CJ, Aplin JD. Adhesion molecules in human trophoblast - a review. II extravillous trophoblast. Placenta (2009) 30:299–304. doi: 10.1016/j.placenta.2008.12.003
88. Tantbirojn P, Crum CP, Parast MM. Pathophysiology of placenta creta: the role of decidua and extravillous trophoblast. Placenta (2008) 29:639–45. doi: 10.1016/j.placenta.2008.04.008
89. Hannon T, Innes BA, Lash GE, Bulmer JN, Robson SC. Effects of local decidua on trophoblast invasion and spiral artery remodeling in focal placenta creta - an immunohistochemical study. Placenta (2012) 33:998–1004. doi: 10.1016/j.placenta.2012.09.004
90. Lala PK, Graham CH. Mechanisms of trophoblast invasiveness and their control: the role of proteases and protease inhibitors. Cancer Metastasis Rev. (1990) 9:369–79. doi: 10.1007/BF00049525
91. Bischof P, Meisser A, Campana A. Paracrine and autocrine regulators of trophoblast invasion–a review. Placenta (2000) 21 (Suppl. A):S55–60. doi: 10.1053/plac.2000.0521
92. Queenan JTJr, Kao LC, Arboleda CE, Ulloa-Aguirre A, Golos TG, Cines DB, et al. Regulation of urokinase-type plasminogen activator production by cultured human cytotrophoblasts. J Biol Chem (1987) 262:10903–6.
93. Feinberg RF, Kao LC, Haimowitz JE, Queenan JT Jr, Wun TC, Strauss JF III, et al. Plasminogen activator inhibitor types 1 and 2 in human trophoblasts. PAI-1 is an immunocytochemical marker of invading trophoblasts. Lab Invest. (1989) 61:20–6.
94. Fisher SJ, Cui TY, Zhang L, Hartman L, Grahl K, Zhang GY, et al. Adhesive and degradative properties of human placental cytotrophoblast cells in vitro. J Cell Biol (1989) 109:891–902. doi: 10.1083/jcb.109.2.891
95. Zini JM, Murray SC, Graham CH, Lala PK, Kariko K, Barnathan ES, et al. Characterization of urokinase receptor expression by human placental trophoblasts. Blood (1992) 79:2917–29.
96. Schatz F, Lockwood CJ. Progestin regulation of plasminogen activator inhibitor type 1 in primary cultures of endometrial stromal and decidual cells. J Clin Endocrinol Metab. (1993) 77:621–5.
97. Bogic LV, Ohira RH, Yamamoto SY, Okazaki KJ, Millar K, Bryant-Greenwood GD. Tissue plasminogen activator and its receptor in the human amnion, chorion, and decidua at preterm and term. Biol Reprod. (1999) 60:1006–12. doi: 10.1095/biolreprod60.4.1006
98. Plaisier M, Koolwijk P, Willems F, Helmerhorst FM, van Hinsbergh VW. Pericellular-acting proteases in human first trimester decidua. Mol Hum Reprod. (2008) 14:41–51. doi: 10.1093/molehr/gam085
99. Chen CP, Bajoria R, Aplin JD. Decreased vascularization and cell proliferation in placentas of intrauterine growth-restricted fetuses with abnormal umbilical artery flow velocity waveforms. Am J Obstet Gynecol. (2002) 187:764–9. doi: 10.1067/mob.2002.125243
100. Lopez-Mejia IC, De Toledo M, Della Seta F, Fafet P, Rebouissou C, Deleuze V, et al. Tissue-specific and SRSF1-dependent splicing of fibronectin, a matrix protein that controls host cell invasion. Mol Biol Cell (2013) 24:3164–76. doi: 10.1091/mbc.e13-03-0142
101. Weimar CH, Macklon NS, Post Uiterweer ED, Brosens JJ, Gellersen B. The motile and invasive capacity of human endometrial stromal cells: implications for normal and impaired reproductive function. Hum Reprod Update (2013) 19:542–57. doi: 10.1093/humupd/dmt025
102. Schwenke M, Knofler M, Velicky P, Weimar CH, Kruse M, Samalecos A, et al. Control of human endometrial stromal cell motility by PDGF-BB, HB-EGF and trophoblast-secreted factors. PLoS ONE (2013) 8:e54336. doi: 10.1371/journal.pone.0054336
103. Hess AP, Hamilton AE, Talbi S, Dosiou C, Nyegaard M, Nayak N, et al. Decidual stromal cell response to paracrine signals from the trophoblast: amplification of immune and angiogenic modulators. Biol Reprod. (2007) 76:102–17. doi: 10.1095/biolreprod.106.054791
104. Tilburgs T, Crespo AC, van der Zwan A, Rybalov B, Raj T, Stranger B, et al. Human HLA-G+ extravillous trophoblasts: Immune-activating cells that interact with decidual leukocytes. Proc Natl Acad Sci USA (2015) 112:7219–24. doi: 10.1073/pnas.1507977112
105. Apps R, Murphy SP, Fernando R, Gardner L, Ahad T, Moffett A. Human leucocyte antigen (HLA) expression of primary trophoblast cells and placental cell lines, determined using single antigen beads to characterize allotype specificities of anti-HLA antibodies. Immunology (2009) 127:26–39. doi: 10.1111/j.1365-2567.2008.03019.x
106. Bilban M, Tauber S, Haslinger P, Pollheimer J, Saleh L, Pehamberger H, et al. Trophoblast invasion: assessment of cellular models using gene expression signatures. Placenta (2010) 31:989–96. doi: 10.1016/j.placenta.2010.08.011
107. Genbacev O, Joslin R, Damsky CH, Polliotti BM, Fisher SJ. Hypoxia alters early gestation human cytotrophoblast differentiation/invasion in vitro and models the placental defects that occur in preeclampsia. J Clin Invest. (1996) 97:540–50. doi: 10.1172/JCI118447
108. Lash GE, Hornbuckle J, Brunt A, Kirkley M, Searle RF, Robson SC, et al. Effect of low oxygen concentrations on trophoblast-like cell line invasion. Placenta (2007) 28:390–8. doi: 10.1016/j.placenta.2006.06.001
109. Selick CE, Horowitz GM, Gratch M, Scott RTJr, Navot D, Hofmann GE. Immunohistochemical localization of transforming growth factor-beta in human implantation sites. J Clin Endocrinol Metab. (1994) 78:592–6.
110. Lash GE, Schiessl B, Kirkley M, Innes BA, Cooper A, Searle RF, et al. Expression of angiogenic growth factors by uterine natural killer cells during early pregnancy. J Leukoc Biol. (2006) 80:572–80. doi: 10.1189/jlb.0406250
111. Graham CH, Lysiak JJ, McCrae KR, Lala PK. Localization of transforming growth factor-beta at the human fetal-maternal interface: role in trophoblast growth and differentiation. Biol Reprod. (1992) 46:561–72. doi: 10.1095/biolreprod46.4.561
112. Forbes K, Souquet B, Garside R, Aplin JD, Westwood M. Transforming growth factor-{beta} (TGF{beta}) receptors I/II differentially regulate TGF{beta}1 and IGF-binding protein-3 mitogenic effects in the human placenta. Endocrinology (2010) 151:1723–31. doi: 10.1210/en.2009-0896
113. Cheng JC, Chang HM, Leung PC. Transforming growth factor-beta1 inhibits trophoblast cell invasion by inducing Snail-mediated down-regulation of vascular endothelial-cadherin protein. J Biol Chem. (2013) 288:33181–92. doi: 10.1074/jbc.M113.488866
114. Prossler J, Chen Q, Chamley L, James JL. The relationship between TGFbeta, low oxygen and the outgrowth of extravillous trophoblasts from anchoring villi during the first trimester of pregnancy. Cytokine (2014) 68:9–15. doi: 10.1016/j.cyto.2014.03.001
115. Xu J, Sivasubramaniyam T, Yinon Y, Tagliaferro A, Ray J, Nevo O, et al. Aberrant TGFbeta signaling contributes to altered trophoblast differentiation in preeclampsia. Endocrinology (2016) 157:883–99. doi: 10.1210/en.2015-1696
116. Wolf K, Te Lindert M, Krause M, Alexander S, Te Riet J, Willis AL, et al. Physical limits of cell migration: control by ECM space and nuclear deformation and tuning by proteolysis and traction force. J Cell Biol. (2013) 201:1069–84. doi: 10.1083/jcb.201210152
117. Zybina TG, Kaufmann P, Frank HG, Freed J, Kadyrov M, Biesterfeld S. Genome multiplication of extravillous trophoblast cells in human placenta in the course of differentiation and invasion into endometrium and myometrium. I Dynamics of polyploidization. Tsitologiia (2002) 44:1058–67.
118. Zybina TG, Frank HG, Biesterfeld S, Kaufmann P. Genome multiplication of extravillous trophoblast cells in human placenta in the course of differentiation and invasion into endometrium and myometrium. II Mechanisms of polyploidization. Tsitologiia (2004) 46:640–8.
119. Roth I, Fisher SJ. IL-10 is an autocrine inhibitor of human placental cytotrophoblast MMP-9 production and invasion. Dev Biol. (1999) 205:194–204. doi: 10.1006/dbio.1998.9122
120. Renaud SJ, Macdonald-Goodfellow SK, Graham CH. Coordinated regulation of human trophoblast invasiveness by macrophages and interleukin 10. Biol Reprod. (2007) 76:448–54. doi: 10.1095/biolreprod.106.055376
121. Genbacev O, Schubach SA, Miller RK. Villous culture of first trimester human placenta–model to study extravillous trophoblast (EVT) differentiation. Placenta (1992) 13:439–61. doi: 10.1016/0143-4004(92)90051-T
122. Riddick DH, Luciano AA, Kusmik WF, Maslar IA. De novo synthesis of prolactin by human decidua. Life Sci. (1978) 23:1913–21. doi: 10.1016/0024-3205(78)90557-X
123. Golander A, Hurley T, Barrett J, Handwerger S. Synthesis of prolactin by human decidua in vitro. J Endocrinol. (1979) 82:263–7. doi: 10.1677/joe.0.0820263
124. Rutanen EM, Koistinen R, Wahlstrom T, Bohn H, Ranta T, Seppala M. Synthesis of placental protein 12 by human decidua. Endocrinology (1985) 116:1304–9. doi: 10.1210/endo-116-4-1304
125. Giudice LC, Mark SP, Irwin JC. Paracrine actions of insulin-like growth factors and IGF binding protein-1 in non-pregnant human endometrium and at the decidual-trophoblast interface. J Reprod Immunol. (1998) 39:133–48. doi: 10.1016/S0165-0378(98)00018-7
126. Gibson JM, Aplin JD, White A, Westwood M. Regulation of IGF bioavailability in pregnancy. Mol Hum Reprod. (2001) 7:79–87. doi: 10.1093/molehr/7.1.79
127. Jabbour HN, Critchley HO. Potential roles of decidual prolactin in early pregnancy. Reproduction (2001) 121:197–205. doi: 10.1530/rep.0.1210197
128. Marano RJ, Ben-Jonathan N. Minireview: extrapituitary prolactin: an update on the distribution, regulation, and functions. Mol Endocrinol. (2014) 28:622–33. doi: 10.1210/me.2013-1349
129. Turco MY, Gardner L, Hughes J, Cindrova-Davies T, Gomez MJ, Farrell L, et al. Long-term, hormone-responsive organoid cultures of human endometrium in a chemically defined medium. Nat Cell Biol. (2017) 19:568–77. doi: 10.1038/ncb3516
130. Stefanoska I, Jovanovic Krivokuca M, Vasilijic S, Cujic D, Vicovac L. Prolactin stimulates cell migration and invasion by human trophoblast in vitro. Placenta (2013) 34:775–83. doi: 10.1016/j.placenta.2013.06.305
131. Garrido-Gomez T, Dominguez F, Quinonero A, Diaz-Gimeno P, Kapidzic M, Gormley M, et al. Defective decidualization during and after severe preeclampsia reveals a possible maternal contribution to the etiology. Proc Natl Acad Sci USA (2017) 114:E8468–E8477. doi: 10.1073/pnas.1706546114
132. Irwin JC, Giudice LC. Insulin-like growth factor binding protein-1 binds to placental cytotrophoblast alpha5beta1 integrin and inhibits cytotrophoblast invasion into decidualized endometrial stromal cultures. Growth Horm IGF Res. (1998) 8:21–31. doi: 10.1016/S1096-6374(98)80318-3
133. Gleeson LM, Chakraborty C, McKinnon T, Lala PK. Insulin-like growth factor-binding protein 1 stimulates human trophoblast migration by signaling through alpha 5 beta 1 integrin via mitogen-activated protein Kinase pathway. J Clin Endocrinol Metab. (2001) 86:2484–93. doi: 10.1210/jcem.86.6.7532
134. Tanaka K, Sakai K, Matsushima M, Matsuzawa Y, Izawa T, Nagashima T, et al. Branched-chain amino acids regulate insulin-like growth factor-binding protein 1 (IGFBP1) production by decidua and influence trophoblast migration through IGFBP1. Mol Hum Reprod. (2016) 22:890–9. doi: 10.1093/molehr/gaw032
135. Han VK, Bassett N, Walton J, Challis JR. The expression of insulin-like growth factor (IGF) and IGF-binding protein (IGFBP) genes in the human placenta and membranes: evidence for IGF-IGFBP interactions at the feto-maternal interface. J Clin Endocrinol Metab. (1996) 81:2680–93.
136. Hamilton GS, Lysiak JJ, Han VK, Lala PK. Autocrine-paracrine regulation of human trophoblast invasiveness by insulin-like growth factor (IGF)-II and IGF-binding protein (IGFBP)-1. Exp Cell Res. (1998) 244:147–56. doi: 10.1006/excr.1998.4195
137. Lacey H, Haigh T, Westwood M, Aplin JD. Mesenchymally-derived insulin-like growth factor 1 provides a paracrine stimulus for trophoblast migration. BMC Dev Biol. (2002) 2:5. doi: 10.1186/1471-213X-2-5
138. Coppock HA, White A, Aplin JD, Westwood M. Matrix metalloprotease-3 and−9 proteolyze insulin-like growth factor-binding protein-1. Biol Reprod. (2004) 71:438–43. doi: 10.1095/biolreprod.103.023101
139. Husslein H, Haider S, Meinhardt G, Prast J, Sonderegger S, Knofler M. Expression, regulation and functional characterization of matrix metalloproteinase-3 of human trophoblast. Placenta (2009) 30:284–91. doi: 10.1016/j.placenta.2008.12.002
140. Pollheimer J, Husslein P, Knofler M. Invasive trophoblasts generate regulatory collagen XVIII cleavage products. Placenta (2005) 26 (Suppl. A):S42–45. doi: 10.1016/j.placenta.2004.12.005
141. Knofler M, Pollheimer J. Human placental trophoblast invasion and differentiation: a particular focus on Wnt signaling. Front Genet. (2013) 4:190. doi: 10.3389/fgene.2013.00190
142. Red-Horse K, Drake PM, Gunn MD, Fisher SJ. Chemokine ligand and receptor expression in the pregnant uterus: reciprocal patterns in complementary cell subsets suggest functional roles. Am J Pathol. (2001) 159:2199–213. doi: 10.1016/S0002-9440(10)63071-4
143. Red-Horse K, Drake PM, Fisher SJ. Human pregnancy: the role of chemokine networks at the fetal-maternal interface. Expert Rev Mol Med. (2004) 6:1–14. doi: 10.1017/S1462399404007720
144. Kuang H, Chen Q, Zhang Y, Zhang L, Peng H, Ning L, et al. The cytokine gene CXCL14 restricts human trophoblast cell invasion by suppressing gelatinase activity. Endocrinology (2009) 150:5596–605. doi: 10.1210/en.2009-0570
145. Hanna J, Wald O, Goldman-Wohl D, Prus D, Markel G, Gazit R, et al. CXCL12 expression by invasive trophoblasts induces the specific migration of CD16- human natural killer cells. Blood (2003) 102:1569–77. doi: 10.1182/blood-2003-02-0517
146. Jaleel MA, Tsai AC, Sarkar S, Freedman PV, Rubin LP. Stromal cell-derived factor-1 (SDF-1) signalling regulates human placental trophoblast cell survival. Mol Hum Reprod. (2004) 10:901–9. doi: 10.1093/molehr/gah118
147. Zhou WH, Du MR, Dong L, Yu J, Li DJ. Chemokine CXCL12 promotes the cross-talk between trophoblasts and decidual stromal cells in human first-trimester pregnancy. Hum Reprod. (2008) 23:2669–79. doi: 10.1093/humrep/den308
148. Svensson-Arvelund J, Mehta RB, Lindau R, Mirrasekhian E, Rodriguez-Martinez H, Berg G, et al. The human fetal placenta promotes tolerance against the semiallogeneic fetus by inducing regulatory T cells and homeostatic M2 macrophages. J Immunol. (2015) 194:1534–44. doi: 10.4049/jimmunol.1401536
149. Choudhury RH, Dunk CE, Lye SJ, Aplin JD, Harris LK, Jones RL. Extravillous trophoblast and endothelial cell crosstalk mediates leukocyte infiltration to the early remodeling decidual spiral arteriole wall. J Immunol. (2017) 198:4115–28. doi: 10.4049/jimmunol.1601175
150. Wu HX, Jin LP, Xu B, Liang SS, Li DJ. Decidual stromal cells recruit Th17 cells into decidua to promote proliferation and invasion of human trophoblast cells by secreting IL-17. Cell Mol Immunol. (2014) 11:253–62. doi: 10.1038/cmi.2013.67
151. Librach CL, Feigenbaum SL, Bass KE, Cui TY, Verastas N, Sadovsky Y, et al. Interleukin-1 beta regulates human cytotrophoblast metalloproteinase activity and invasion in vitro. J Biol Chem. (1994) 269:17125–31.
152. Prutsch N, Fock V, Haslinger P, Haider S, Fiala C, Pollheimer J, et al. The role of interleukin-1beta in human trophoblast motility. Placenta (2012) 33:696–703. doi: 10.1016/j.placenta.2012.05.008
153. Hanna J, Goldman-Wohl D, Hamani Y, Avraham I, Greenfield C, Natanson-Yaron S, et al. Decidual NK cells regulate key developmental processes at the human fetal-maternal interface. Nat Med. (2006) 12:1065–74. doi: 10.1038/nm1452
154. De Oliveira LG, Lash GE, Murray-Dunning C, Bulmer JN, Innes BA, Searle RF, et al. Role of interleukin 8 in uterine natural killer cell regulation of extravillous trophoblast cell invasion. Placenta (2010) 31:595–601. doi: 10.1016/j.placenta.2010.04.012
155. Paiva P, Salamonsen LA, Manuelpillai U, Dimitriadis E. Interleukin 11 inhibits human trophoblast invasion indicating a likely role in the decidual restraint of trophoblast invasion during placentation. Biol Reprod. (2009) 80:302–10. doi: 10.1095/biolreprod.108.071415
156. Maruo T, Matsuo H, Murata K, Mochizuki M. Gestational age-dependent dual action of epidermal growth factor on human placenta early in gestation. J Clin Endocrinol Metab (1992) 75:1362–7.
157. Bass KE, Morrish D, Roth I, Bhardwaj D, Taylor R, Zhou Y, et al. Human cytotrophoblast invasion is up-regulated by epidermal growth factor: evidence that paracrine factors modify this process. Dev Biol. (1994) 164:550–61. doi: 10.1006/dbio.1994.1223
158. Leach RE, Kilburn B, Wang J, Liu Z, Romero R, Armant DR. Heparin-binding EGF-like growth factor regulates human extravillous cytotrophoblast development during conversion to the invasive phenotype. Dev Biol. (2004) 266:223–37. doi: 10.1016/j.ydbio.2003.09.026
159. Biadasiewicz K, Sonderegger S, Haslinger P, Haider S, Saleh L, Fiala C, et al. Transcription factor AP-2alpha promotes EGF-dependent invasion of human trophoblast. Endocrinology (2011) 152:1458–69. doi: 10.1210/en.2010-0936
160. Fock V, Plessl K, Fuchs R, Dekan S, Milla SK, Haider S, et al. Trophoblast subtype-specific EGFR/ERBB4 expression correlates with cell cycle progression and hyperplasia in complete hydatidiform moles. Hum Reprod. (2015) 30:789–99. doi: 10.1093/humrep/dev027
161. Wright JK, Dunk CE, Amsalem H, Maxwell C, Keating S, Lye SJ. HER1 signaling mediates extravillous trophoblast differentiation in humans. Biol Reprod. (2010) 83:1036–45. doi: 10.1095/biolreprod.109.083246
162. Sonderegger S, Pollheimer J, Knofler M. Wnt signalling in implantation, decidualisation and placental differentiation–review. Placenta (2010) 31:839–47. doi: 10.1016/j.placenta.2010.07.011
163. Gordon MD, Nusse R. Wnt signaling: multiple pathways, multiple receptors, and multiple transcription factors. J Biol Chem. (2006) 281:22429–33. doi: 10.1074/jbc.R600015200
164. Tulac S, Nayak NR, Kao LC, Van Waes M, Huang J, Lobo S, et al. Identification, characterization, and regulation of the canonical Wnt signaling pathway in human endometrium. J Clin Endocrinol Metab. (2003) 88:3860–6. doi: 10.1210/jc.2003-030494
165. Sonderegger S, Husslein H, Leisser C, Knofler M. Complex expression pattern of Wnt ligands and frizzled receptors in human placenta and its trophoblast subtypes. Placenta (2007) 28 (Suppl. A):S97–102. doi: 10.1016/j.placenta.2006.11.003
166. Meinhardt G, Haider S, Haslinger P, Proestling K, Fiala C, Pollheimer J, et al. Wnt-dependent T-cell factor-4 controls human etravillous trophoblast motility. Endocrinology (2014) 155:1908–20. doi: 10.1210/en.2013-2042
167. Meinhardt G, Saleh L, Otti GR, Haider S, Velicky P, Fiala C, et al. Wingless ligand 5a is a critical regulator of placental growth and survival. Sci Rep. (2016) 6:28127. doi: 10.1038/srep28127
168. Bulmer JN, Johnson PM. Macrophage populations in the human placenta and amniochorion. Clin Exp Immunol. (1984) 57:393–403.
169. Heikkinen J, Mottonen M, Alanen A, Lassila O. Phenotypic characterization of regulatory T cells in the human decidua. Clin Exp Immunol. (2004) 136:373–8. doi: 10.1111/j.1365-2249.2004.02441.x
170. Tilburgs T, Claas FH, Scherjon SA. Elsevier trophoblast research award lecture: unique properties of decidual T cells and their role in immune regulation during human pregnancy. Placenta (2010) 31 (Suppl.) S82–6. doi: 10.1016/j.placenta.2010.01.007
171. Meyer N, Woidacki K, Knofler M, Meinhardt G, Nowak D, Velicky P, et al. Chymase-producing cells of the innate immune system are required for decidual vascular remodeling and fetal growth. Sci Rep. (2017) 7:45106. doi: 10.1038/srep45106
172. Nadkarni S, Smith J, Sferruzzi-Perri AN, Ledwozyw A, Kishore M, Haas R, et al. Neutrophils induce proangiogenic T cells with a regulatory phenotype in pregnancy. Proc Natl Acad Sci USA. (2016) 113:E8415–E8424. doi: 10.1073/pnas.1611944114
173. Amsalem H, Kwan M, Hazan A, Zhang J, Jones RL, Whittle W, et al. Identification of a novel neutrophil population: proangiogenic granulocytes in second-trimester human decidua. J Immunol. (2014) 193:3070–9. doi: 10.4049/jimmunol.1303117
174. Huang B, Faucette AN, Pawlitz MD, Pei B, Goyert JW, Zhou JZ, et al. Interleukin-33-induced expression of PIBF1 by decidual B cells protects against preterm labor. Nat Med. (2017) 23:128–35. doi: 10.1038/nm.4244
175. Solders M, Gorchs L, Gidlof S, Tiblad E, Lundell AC, Kaipe H. Maternal adaptive immune cells in decidua parietalis display a more activated and coinhibitory phenotype compared to decidua basalis. Stem Cells Int. (2017) 2017:8010961. doi: 10.1155/2017/8010961
176. Hume DA. Macrophages as APC and the dendritic cell myth. J Immunol. (2008) 181:5829–35. doi: 10.4049/jimmunol.181.9.5829
177. Murray PJ, Wynn TA. Protective and pathogenic functions of macrophage subsets. Nat Rev Immunol. (2011) 11:723–37. doi: 10.1038/nri3073
178. Doebel T, Voisin B, Nagao K. Langerhans cells - the macrophage in dendritic cell clothing. Trends Immunol. (2017) 38:817–28. doi: 10.1016/j.it.2017.06.008
179. Hashimoto D, Miller J, Merad M. Dendritic cell and macrophage heterogeneity in vivo. Immunity (2011) 35:323–35. doi: 10.1016/j.immuni.2011.09.007
180. Chakraborty D, Rumi MA, Konno T, Soares MJ. Natural killer cells direct hemochorial placentation by regulating hypoxia-inducible factor dependent trophoblast lineage decisions. Proc Natl Acad Sci USA. (2011) 108:16295–300. doi: 10.1073/pnas.1109478108
181. Lima PD, Zhang J, Dunk C, Lye SJ, Croy BA. Leukocyte driven-decidual angiogenesis in early pregnancy. Cell Mol Immunol. (2014) 11:522–37. doi: 10.1038/cmi.2014.63
182. Sharkey AM, Xiong S, Kennedy PR, Gardner L, Farrell LE, Chazara O, et al. Tissue-specific education of decidual NK cells. J Immunol. (2015) 195:3026–32. doi: 10.4049/jimmunol.1501229
183. Lee AJ, Kandiah N, Karimi K, Clark DA, Ashkar AA. Interleukin-15 is required for maximal lipopolysaccharide-induced abortion. J Leukoc Biol. (2013) 93:905–12. doi: 10.1189/jlb.0912442
184. Yougbare I, Tai WS, Zdravic D, Oswald BE, Lang S, Zhu G, et al. Activated NK cells cause placental dysfunction and miscarriages in fetal alloimmune thrombocytopenia. Nat Commun. (2017) 8:224. doi: 10.1038/s41467-017-00269-1
185. Ball E, Bulmer JN, Ayis S, Lyall F, Robson SC. Late sporadic miscarriage is associated with abnormalities in spiral artery transformation and trophoblast invasion. J Pathol. (2006) 208:535–42. doi: 10.1002/path.1927
186. Xiong S, Sharkey AM, Kennedy PR, Gardner L, Farrell LE, Chazara O, et al. Maternal uterine NK cell-activating receptor KIR2DS1 enhances placentation. J Clin Invest. (2013) 123:4264–72. doi: 10.1172/JCI68991
187. Eriksson M, Meadows SK, Wira CR, Sentman CL. Unique phenotype of human uterine NK cells and their regulation by endogenous TGF-beta. J Leukoc Biol. (2004) 76:667–75. doi: 10.1189/jlb.0204090
188. Male V, Sharkey A, Masters L, Kennedy PR, Farrell LE, Moffett A. The effect of pregnancy on the uterine NK cell KIR repertoire. Eur J Immunol. (2011) 41:3017–27. doi: 10.1002/eji.201141445
189. Cerdeira AS, Rajakumar A, Royle CM, Lo A, Husain Z, Thadhani RI, et al. Conversion of peripheral blood NK cells to a decidual NK-like phenotype by a cocktail of defined factors. J Immunol. (2013) 190:3939–48. doi: 10.4049/jimmunol.1202582
190. Montaldo E, Vacca P, Chiossone L, Croxatto D, Loiacono F, Martini S, et al. Unique Eomes(+) NK cell subsets are present in uterus and decidua during early pregnancy. Front Immunol. (2015) 6:646. doi: 10.3389/fimmu.2015.00646
191. King A, Wooding P, Gardner L, Loke YW. Expression of perforin, granzyme A and TIA-1 by human uterine CD56+ NK cells implies they are activated and capable of effector functions. Hum Reprod. (1993) 8:2061–7. doi: 10.1093/oxfordjournals.humrep.a137982
192. Chazara O, Xiong S, Moffett A. Maternal KIR and fetal HLA-C: a fine balance. J Leukoc Biol. (2011) 90:703–16. doi: 10.1189/jlb.0511227
193. Tilburgs T, Evans JH, Crespo AC, Strominger JL. The HLA-G cycle provides for both NK tolerance and immunity at the maternal-fetal interface. Proc Natl Acad Sci USA (2015) 112:13312–7. doi: 10.1073/pnas.1517724112
194. Vacca P, Cantoni C, Vitale M, Prato C, Canegallo F, Fenoglio D, et al. Crosstalk between decidual NK and CD14+ myelomonocytic cells results in induction of Tregs and immunosuppression. Proc Natl Acad Sci USA. (2010) 107:11918–23. doi: 10.1073/pnas.1001749107
195. Co EC, Gormley M, Kapidzic M, Rosen DB, Scott MA, Stolp HA, et al. Maternal decidual macrophages inhibit NK cell killing of invasive cytotrophoblasts during human pregnancy. Biol Reprod. (2013) 88:155. doi: 10.1095/biolreprod.112.099465
196. Gaynor LM, Colucci F. Uterine natural killer cells: functional distinctions and influence on pregnancy in humans and mice. Front Immunol. (2017) 8:467. doi: 10.3389/fimmu.2017.00467
197. Lee CQ, Gardner L, Turco M, Zhao N, Murray MJ, Coleman N, et al. What is trophoblast? a combination of criteria define human first-trimester trophoblast. Stem Cell Rep. (2016) 6:257–72. doi: 10.1016/j.stemcr.2016.01.006
198. Hackmon R, Pinnaduwage L, Zhang J, Lye SJ, Geraghty DE, Dunk CE. Definitive class I human leukocyte antigen expression in gestational placentation: HLA-F, HLA-E, HLA-C, and HLA-G in extravillous trophoblast invasion on placentation, pregnancy, and parturition. Am J Reprod Immunol. (2017) 77:e12643. doi: 10.1111/aji.12643
199. Zhang J, Dunk CE, Kwan M, Jones RL, Harris LK, Keating S, et al. Human dNK cell function is differentially regulated by extrinsic cellular engagement and intrinsic activating receptors in first and second trimester pregnancy. Cell Mol Immunol. (2017) 14:203–13. doi: 10.1038/cmi.2015.66
200. Kennedy PR, Chazara O, Gardner L, Ivarsson MA, Farrell LE, Xiong S, et al. Activating KIR2DS4 is expressed by uterine NK cells and contributes to successful pregnancy. J Immunol. (2016) 197:4292–300. doi: 10.4049/jimmunol.1601279
201. Rajagopalan S, Long EO. KIR2DL4 (CD158d): An activation receptor for HLA-G. Front Immunol. (2012) 3:258. doi: 10.3389/fimmu.2012.00258
202. Rajagopalan S, Long EO. Cellular senescence induced by CD158d reprograms natural killer cells to promote vascular remodeling. Proc Natl Acad Sci U S A (2012) 109:20596–601. doi: 10.1073/pnas.1208248109
203. Sharkey AM, Gardner L, Hiby S, Farrell L, Apps R, Masters L, et al. Killer Ig-like receptor expression in uterine NK cells is biased toward recognition of HLA-C and alters with gestational age. J Immunol. (2008) 181:39–46. doi: 10.4049/jimmunol.181.1.39
204. Hiby SE, Apps R, Sharkey AM, Farrell LE, Gardner L, Mulder A, et al. Maternal activating KIRs protect against human reproductive failure mediated by fetal HLA-C2. J Clin Invest. (2010) 120:4102–10. doi: 10.1172/JCI43998
205. Apps R, Gardner L, Hiby SE, Sharkey AM, Moffett A. Conformation of human leucocyte antigen-C molecules at the surface of human trophoblast cells. Immunology (2008) 124:322–8. doi: 10.1111/j.1365-2567.2007.02789.x
206. Crespo AC, Strominger JL, Tilburgs T. Expression of KIR2DS1 by decidual natural killer cells increases their ability to control placental HCMV infection. Proc Natl Acad Sci USA. (2016) 113:15072–7. doi: 10.1073/pnas.1617927114
207. Castellana B, Perdu S, Kim Y, Chan K, Atif J, Marziali M, et al. Maternal obesity alters uterine NK activity through a functional KIR2DL1/S1 imbalance. Immunol Cell Biol. (2018). doi: 10.1111/imcb.12041
208. King A, Allan DS, Bowen M, Powis SJ, Joseph S, Verma S, et al. HLA-E is expressed on trophoblast and interacts with CD94/NKG2 receptors on decidual NK cells. Eur J Immunol. (2000) 30:1623–31. doi: 10.1002/1521-4141(200006)30:6<1623::AID-IMMU1623>3.0.CO;2-M
209. El Costa H, Casemayou A, Aguerre-Girr M, Rabot M, Berrebi A, Parant O, et al. Critical and differential roles of NKp46- and NKp30-activating receptors expressed by uterine NK cells in early pregnancy. J Immunol. (2008) 181:3009–17. doi: 10.4049/jimmunol.181.5.3009
210. Apps R, Gardner L, Sharkey AM, Holmes N, Moffett A. A homodimeric complex of HLA-G on normal trophoblast cells modulates antigen-presenting cells via LILRB1. Eur J Immunol. (2007) 37:1924–37. doi: 10.1002/eji.200737089
211. Rajagopalan S, Long EO. A human histocompatibility leukocyte antigen (HLA)-G-specific receptor expressed on all natural killer cells. J Exp Med. (1999) 189:1093–100. doi: 10.1084/jem.189.7.1093
212. Li C, Houser BL, Nicotra ML, Strominger JL. HLA-G homodimer-induced cytokine secretion through HLA-G receptors on human decidual macrophages and natural killer cells. Proc Natl Acad Sci USA. (2009) 106:5767–72. doi: 10.1073/pnas.0901173106
213. van der Meer A, Lukassen HG, van Lierop MJ, Wijnands F, Mosselman S, Braat DD, et al. Membrane-bound HLA-G activates proliferation and interferon-gamma production by uterine natural killer cells. Mol Hum Reprod. (2004) 10:189–95. doi: 10.1093/molehr/gah032
214. Apps R, Sharkey A, Gardner L, Male V, Kennedy P, Masters L, et al. Ex vivo functional responses to HLA-G differ between blood and decidual NK cells. Mol Hum Reprod. (2011) 17:577–86. doi: 10.1093/molehr/gar022
215. van der Meer A, Lukassen HG, van Cranenbroek B, Weiss EH, Braat DD, van Lierop MJ, et al. Soluble HLA-G promotes Th1-type cytokine production by cytokine-activated uterine and peripheral natural killer cells. Mol Hum Reprod. (2007) 13:123–33. doi: 10.1093/molehr/gal100
216. Rajagopalan S, Bryceson YT, Kuppusamy SP, Geraghty DE, van der Meer A, Joosten I, et al. Activation of NK cells by an endocytosed receptor for soluble HLA-G. PLoS Biol (2006) 4:e9. doi: 10.1371/journal.pbio.0040009
217. Lash GE, Otun HA, Innes BA, Bulmer JN, Searle RF, Robson SC. Inhibition of trophoblast cell invasion by TGFB1:2, and 3 is associated with a decrease in active proteases. Biol Reprod. (2005) 73:374–81. doi: 10.1095/biolreprod.105.040337
218. Athanassiades A, Lala PK. Role of placenta growth factor (PIGF) in human extravillous trophoblast proliferation, migration and invasiveness. Placenta (1998) 19:465–73. doi: 10.1016/S0143-4004(98)91039-6
219. Knofler M, Mosl B, Bauer S, Griesinger G, Husslein P. TNF-alpha/TNFRI in primary and immortalized first trimester cytotrophoblasts. Placenta (2000) 21:525–35. doi: 10.1053/plac.1999.0501
220. Zhou Y, McMaster M, Woo K, Janatpour M, Perry J, Karpanen T, et al. Vascular endothelial growth factor ligands and receptors that regulate human cytotrophoblast survival are dysregulated in severe preeclampsia and hemolysis, elevated liver enzymes, and low platelets syndrome. Am J Pathol. (2002) 160:1405–23. doi: 10.1016/S0002-9440(10)62567-9
221. Zhang J, Dunk CE, Lye SJ. Sphingosine signalling regulates decidual NK cell angiogenic phenotype and trophoblast migration. Hum Reprod. (2013) 28:3026–37. doi: 10.1093/humrep/det339
222. Bauer S, Pollheimer J, Hartmann J, Husslein P, Aplin JD, Knofler M. Tumor necrosis factor-alpha inhibits trophoblast migration through elevation of plasminogen activator inhibitor-1 in first-trimester villous explant cultures. J Clin Endocrinol Metab. (2004) 89:812–22. doi: 10.1210/jc.2003-031351
223. Lash GE, Otun HA, Innes BA, Kirkley M, De Oliveira L, Searle RF, et al. Interferon-gamma inhibits extravillous trophoblast cell invasion by a mechanism that involves both changes in apoptosis and protease levels. FASEB J. (2006) 20:2512–8. doi: 10.1096/fj.06-6616com
224. Kopcow HD, Allan DS, Chen X, Rybalov B, Andzelm MM, Ge B, et al. Human decidual NK cells form immature activating synapses and are not cytotoxic. Proc Natl Acad Sci USA. (2005) 102:15563–8. doi: 10.1073/pnas.0507835102
225. Crespo AC, van der Zwan A, Ramalho-Santos J, Strominger JL, Tilburgs T. Cytotoxic potential of decidual NK cells and CD8+ T cells awakened by infections. J Reprod Immunol. (2017) 119:85–90. doi: 10.1016/j.jri.2016.08.001
226. Stallmach T, Hebisch G, Orban P, Lu X. Aberrant positioning of trophoblast and lymphocytes in the feto-maternal interface with pre-eclampsia. Virchows Arch. (1999) 434:207–11. doi: 10.1007/s004280050329
227. Eide IP, Rolfseng T, Isaksen CV, Mecsei R, Roald B, Lydersen S, et al. Serious foetal growth restriction is associated with reduced proportions of natural killer cells in decidua basalis. Virchows Arch. (2006) 448:269–76. doi: 10.1007/s00428-005-0107-z
228. Rieger L, Segerer S, Bernar T, Kapp M, Majic M, Morr AK, et al. Specific subsets of immune cells in human decidua differ between normal pregnancy and preeclampsia–a prospective observational study. Reprod Biol Endocrinol. (2009) 7:132. doi: 10.1186/1477-7827-7-132
229. Williams PJ, Bulmer JN, Searle RF, Innes BA, Robson SC. Altered decidual leucocyte populations in the placental bed in pre-eclampsia and foetal growth restriction: a comparison with late normal pregnancy. Reproduction (2009) 138:177–84. doi: 10.1530/REP-09-0007
230. Wilczynski JR, Tchorzewski H, Banasik M, Glowacka E, Wieczorek A, Lewkowicz P, et al. Lymphocyte subset distribution and cytokine secretion in third trimester decidua in normal pregnancy and preeclampsia. Eur J Obstet Gynecol Reprod Biol. (2003) 109:8–15. doi: 10.1016/S0301-2115(02)00350-0
231. Bachmayer N, Rafik Hamad R, Liszka L, Bremme K, Sverremark-Ekstrom E. Aberrant uterine natural killer (NK)-cell expression and altered placental and serum levels of the NK-cell promoting cytokine interleukin-12 in pre-eclampsia. Am J Reprod Immunol. (2006) 56:292–301. doi: 10.1111/j.1600-0897.2006.00429.x
232. Lessin DL, Hunt JS, King CR, Wood GW. Antigen expression by cells near the maternal-fetal interface. Am J Reprod Immunol Microbiol. (1988) 16:1–7. doi: 10.1111/j.1600-0897.1988.tb00169.x
233. Ning F, Liu H, Lash GE. The role of decidual macrophages during normal and pathological pregnancy. Am J Reprod Immunol. (2016) 75:298–309. doi: 10.1111/aji.12477
234. Okabe Y, Medzhitov R. Tissue biology perspective on macrophages. Nat Immunol. (2016) 17:9–17. doi: 10.1038/ni.3320
235. Mosser DM, Edwards JP. Exploring the full spectrum of macrophage activation. Nat Rev Immunol. (2008) 8:958–69. doi: 10.1038/nri2448
236. Piccolo V, Curina A, Genua M, Ghisletti S, Simonatto M, Sabo A, et al. Opposing macrophage polarization programs show extensive epigenomic and transcriptional cross-talk. Nat Immunol. (2017) 18:530–40. doi: 10.1038/ni.3710
237. Helm O, Held-Feindt J, Grage-Griebenow E, Reiling N, Ungefroren H, Vogel I, et al. Tumor-associated macrophages exhibit pro- and anti-inflammatory properties by which they impact on pancreatic tumorigenesis. Int J Cancer (2014) 135:843–61. doi: 10.1002/ijc.28736
238. Kratochvill F, Neale G, Haverkamp JM, Van de Velde LA, Smith AM, Kawauchi D, et al. TNF Counterbalances the Emergence of M2 Tumor Macrophages. Cell Rep. (2015) 12:1902–14. doi: 10.1016/j.celrep.2015.08.033
239. Houser BL, Tilburgs T, Hill J, Nicotra ML, Strominger JL. Two unique human decidual macrophage populations. J Immunol.(2011) 186:2633–42. doi: 10.4049/jimmunol.1003153
240. Svensson J, Jenmalm MC, Matussek A, Geffers R, Berg G, Ernerudh J. Macrophages at the fetal-maternal interface express markers of alternative activation and are induced by M-CSF and IL-10. J Immunol. (2011) 187:3671–82. doi: 10.4049/jimmunol.1100130
241. Sayama S, Nagamatsu T, Schust DJ, Itaoka N, Ichikawa M, Kawana K, et al. Human decidual macrophages suppress IFN-gamma production by T cells through costimulatory B7-H1:PD-1 signaling in early pregnancy. J Reprod Immunol. (2013) 100:109–17. doi: 10.1016/j.jri.2013.08.001
242. Jiang X, Du MR, Li M, Wang H. Three macrophage subsets are identified in the uterus during early human pregnancy. Cell Mol Immunol. (2018). doi: 10.1038/s41423-018-0008-0. [Epub ahead of print].
243. Kabawat SE, Mostoufi-Zadeh M, Driscoll SG, Bhan AK. Implantation site in normal pregnancy. A study with monoclonal antibodies. Am J Pathol.(1985) 118:76–84.
244. Helige C, Ahammer H, Moser G, Hammer A, Dohr G, Huppertz B, et al. Distribution of decidual natural killer cells and macrophages in the neighbourhood of the trophoblast invasion front: a quantitative evaluation. Hum Reprod. (2014) 29:8–17. doi: 10.1093/humrep/det353
245. Pull SL, Doherty JM, Mills JC, Gordon JI, Stappenbeck TS. Activated macrophages are an adaptive element of the colonic epithelial progenitor niche necessary for regenerative responses to injury. Proc Natl Acad Sci USA (2005) 102:99–104. doi: 10.1073/pnas.0405979102
246. Mussar K, Tucker A, McLennan L, Gearhart A, Jimenez-Caliani AJ, Cirulli V, et al. Macrophage/epithelium cross-talk regulates cell cycle progression and migration in pancreatic progenitors. PLoS ONE (2014) 9:e89492. doi: 10.1371/journal.pone.0089492
247. Dang T, Liou GY. Macrophage cytokines enhance cell proliferation of normal prostate epithelial cells through activation of ERK and Akt. Sci Rep. (2018) 8:7718. doi: 10.1038/s41598-018-26143-8
248. Bergenfelz C, Medrek C, Ekstrom E, Jirstrom K, Janols H, Wullt M, et al. Wnt5a induces a tolerogenic phenotype of macrophages in sepsis and breast cancer patients. J Immunol. (2012) 188:5448–58. doi: 10.4049/jimmunol.1103378
249. Seo DH, Che X, Kwak MS, Kim S, Kim JH, Ma HW, et al. Interleukin-33 regulates intestinal inflammation by modulating macrophages in inflammatory bowel disease. Sci Rep. (2017) 7:851. doi: 10.1038/s41598-017-00840-2
250. Fock V, Mairhofer M, Otti GR, Hiden U, Spittler A, Zeisler H, et al. Macrophage-derived IL-33 is a critical factor for placental growth. J Immunol (2013) 191:3734–43. doi: 10.4049/jimmunol.1300490
251. McWhorter FY, Wang T, Nguyen P, Chung T, Liu WF. Modulation of macrophage phenotype by cell shape. Proc Natl Acad Sci USA (2013) 110:17253–8. doi: 10.1073/pnas.1308887110
252. Reister F, Frank HG, Heyl W, Kosanke G, Huppertz B, Schroder W, et al. The distribution of macrophages in spiral arteries of the placental bed in pre-eclampsia differs from that in healthy patients. Placenta (1999) 20:229–33. doi: 10.1053/plac.1998.0373
253. Schonkeren D, van der Hoorn ML, Khedoe P, Swings G, van Beelen E, Claas F, et al. Differential distribution and phenotype of decidual macrophages in preeclamptic versus control pregnancies. Am J Pathol. (2011) 178:709–17. doi: 10.1016/j.ajpath.2010.10.011
254. Burk MR, Troeger C, Brinkhaus R, Holzgreve W, Hahn S. Severely reduced presence of tissue macrophages in the basal plate of pre-eclamptic placentae. Placenta (2001) 22:309–16. doi: 10.1053/plac.2001.0624
255. Kim JS, Romero R, Cushenberry E, Kim YM, Erez O, Nien JK, et al. Distribution of CD14+ and CD68+ macrophages in the placental bed and basal plate of women with preeclampsia and preterm labor. Placenta (2007) 28:571–6. doi: 10.1016/j.placenta.2006.07.007
256. Saito S, Umekage H, Sakamoto Y, Sakai M, Tanebe K, Sasaki Y, et al. Increased T-helper-1-type immunity and decreased T-helper-2-type immunity in patients with preeclampsia. Am J Reprod Immunol. (1999) 41:297–306. doi: 10.1111/j.1600-0897.1999.tb00442.x
257. Wilczynski JR, Tchorzewski H, Glowacka E, Banasik M, Lewkowicz P, Szpakowski M, et al. Cytokine secretion by decidual lymphocytes in transient hypertension of pregnancy and pre-eclampsia. Mediators Inflamm. (2002) 11:105–11. doi: 10.1080/09629350220131962
258. Cotechini T, Komisarenko M, Sperou A, Macdonald-Goodfellow S, Adams MA, Graham CH. Inflammation in rat pregnancy inhibits spiral artery remodeling leading to fetal growth restriction and features of preeclampsia. J Exp Med. (2014) 211:165–79. doi: 10.1084/jem.20130295
259. Odero MD, Zeleznik-Le NJ, Chinwalla V, and Rowley JD. Cytogenetic and molecular analysis of the acute monocytic leukemia cell line THP-1 with an MLL-AF9 translocation. Genes Chromosomes Cancer (2000) 29:333–8. doi: 10.1002/1098-2264(2000)9999:9999<::AID-GCC1040>3.0.CO;2-Z
260. Gomez Perdiguero E, Klapproth K, Schulz C, Busch K, Azzoni E, Crozet L, et al. Tissue-resident macrophages originate from yolk-sac-derived erythro-myeloid progenitors. Nature (2015) 518:547–51. doi: 10.1038/nature13989
261. Ginhoux F, Guilliams M. Tissue-resident macrophage ontogeny and homeostasis. Immunity (2016) 44:439–49. doi: 10.1016/j.immuni.2016.02.024
262. Klein I, Cornejo JC, Polakos NK, John B, Wuensch SA, Topham DJ, et al. Kupffer cell heterogeneity: functional properties of bone marrow derived and sessile hepatic macrophages. Blood (2007) 110:4077–85. doi: 10.1182/blood-2007-02-073841
263. Merad M, Manz MG, Karsunky H, Wagers A, Peters W, Charo I, et al. Langerhans cells renew in the skin throughout life under steady-state conditions. Nat Immunol. (2002) 3:1135–41. doi: 10.1038/ni852
264. Ginhoux F, Greter M, Leboeuf M, Nandi S, See P, Gokhan S, et al. Fate mapping analysis reveals that adult microglia derive from primitive macrophages. Science (2010) 330:841–5. doi: 10.1126/science.1194637
265. Jenkins SJ, Ruckerl D, Cook PC, Jones LH, Finkelman FD, van Rooijen N, et al. Local macrophage proliferation, rather than recruitment from the blood, is a signature of TH2 inflammation. Science (2011) 332:1284–8. doi: 10.1126/science.1204351
266. Vacca P, Vitale C, Montaldo E, Conte R, Cantoni C, Fulcheri E, et al. CD34+ hematopoietic precursors are present in human decidua and differentiate into natural killer cells upon interaction with stromal cells. Proc Natl Acad Sci USA (2011) 108:2402–7. doi: 10.1073/pnas.1016257108
267. Gamliel M, Goldman-Wohl D, Isaacson B, Gur C, Stein N, Yamin R, et al. Trained memory of human uterine NK cells enhances their function in subsequent pregnancies. Immunity (2018) 48:951–62 e955. doi: 10.1016/j.immuni.2018.03.030
268. Carbone FR. Tissue-resident memory T cells and fixed immune surveillance in nonlymphoid organs. J Immunol. (2015) 195:17–22. doi: 10.4049/jimmunol.1500515
269. Bjorkstrom NK, Ljunggren HG, Michaelsson J. Emerging insights into natural killer cells in human peripheral tissues. Nat Rev Immunol. (2016) 16:310–20. doi: 10.1038/nri.2016.34
270. Kammerer U, Eggert AO, Kapp M, McLellan AD, Geijtenbeek TB, Dietl J, et al. Unique appearance of proliferating antigen-presenting cells expressing DC-SIGN (CD209) in the decidua of early human pregnancy. Am J Pathol. (2003) 162:887–96. doi: 10.1016/S0002-9440(10)63884-9
271. Gustafsson C, Mjosberg J, Matussek A, Geffers R, Matthiesen L, Berg G, et al. Gene expression profiling of human decidual macrophages: evidence for immunosuppressive phenotype. PLoS ONE (2008) 3:e2078. doi: 10.1371/journal.pone.0002078
Keywords: placental development, extravillous trophoblast, decidual immune cells, trophoblast invasion, uterine natural killer cells, decidual macrophages
Citation: Pollheimer J, Vondra S, Baltayeva J, Beristain AG and Knöfler M (2018) Regulation of Placental Extravillous Trophoblasts by the Maternal Uterine Environment. Front. Immunol. 9:2597. doi: 10.3389/fimmu.2018.02597
Received: 28 August 2018; Accepted: 22 October 2018;
Published: 13 November 2018.
Edited by:
Nandor Gabor Than, Hungarian Academy of Sciences (MTA), HungaryReviewed by:
Caroline Dunk, Lunenfeld-Tanenbaum Research Institute, CanadaJoanna James, University of Auckland, New Zealand
Copyright © 2018 Pollheimer, Vondra, Baltayeva, Beristain and Knöfler. This is an open-access article distributed under the terms of the Creative Commons Attribution License (CC BY). The use, distribution or reproduction in other forums is permitted, provided the original author(s) and the copyright owner(s) are credited and that the original publication in this journal is cited, in accordance with accepted academic practice. No use, distribution or reproduction is permitted which does not comply with these terms.
*Correspondence: Martin Knöfler, bWFydGluLmtub2VmbGVyQG1lZHVuaXdpZW4uYWMuYXQ=