- 1Department of Anatomy and Anthropology, Sackler Faculty of Medicine, Tel Aviv University, Tel Aviv, Israel
- 2Department of Prosthodontics, Goldschleger School of Dental Medicine, Sackler Faculty of Medicine, Tel Aviv University, Tel Aviv, Israel
- 3Department of Pathology, Sackler Faculty of Medicine, Tel Aviv University, Tel Aviv, Israel
The worldwide number of dental implants and orthopedic prostheses is steadily increasing. Orthopedic implant loosening, in the absence of infection, is mostly attributable to the generation of wear debris. Dental peri-implantitis is characterized by a multifactorial etiology and is the main cause of implant failure. It consists of a peri-implant inflammatory lesion that often results in loss of supporting bone. Disease management includes cleaning the surrounding flora by hand instruments, ultrasonic tips, lasers, or chemical agents. We recently published a paper indicating that US scaling of titanium (Ti) implants releases particles that provoke an inflammatory response and osteolysis. Here we show that a strong inflammatory response occurs; however, very few of the titanium particles are phagocytosed by the macrophages. We then measured a dramatic Ti particle-induced stimulation of IL1β, IL6, and TNFα secretion by these macrophages using multiplex immunoassay. The particle-induced expression profile, examined by FACS, also indicated an M1 macrophage polarization. To assess how the secreted cytokines contributed to the paracrine exacerbation of the inflammatory response and to osteoclastogenesis, we treated macrophage/preosteoclast cultures with neutralizing antibodies against IL1β, IL6, or TNFα. We found that anti-TNFα antibodies attenuated the overall expression of both the inflammatory cytokines and osteoclastogenesis. On the other hand, anti-IL1β antibodies affected osteoclastogenesis but not the paracrine expression of inflammatory cytokines, whereas anti-IL6 antibodies did the opposite. We then tested these neutralizing antibodies in vivo using our mouse calvarial model of Ti particle-induced osteolysis and microCT analysis. Here, all neutralizing antibodies, administered by intraperitoneal injection, completely abrogated the particle-induced osteolysis. This suggests that blockage of paracrine inflammatory stimulation and osteoclastogenesis are similarly effective in preventing bone resorption induced by Ti particles. Blocking both the inflammation and osteoclastogenesis by anti-TNFα antibodies, incorporated locally into a slow-release membrane, also significantly prevented osteolysis. The osteolytic inflammatory response, fueled by ultrasonic scaling of Ti implants, results from an inflammatory positive feedback loop and osteoclastogenic stimulation. Our findings suggest that blocking IL1β, IL6, and/or TNFα systemically or locally around titanium implants is a promising therapeutic approach for the clinical management of peri-implant bone loss.
Introduction
The prevalence of orthopedic and dental titanium (Ti) implants has increased steadily worldwide. Despite high success rates during the first 10 years (1, 2), orthopedic loosening and oral peri-implantitis remain a major problem for clinicians and constitute a major health problem for the profession. As mentioned, dental peri-implantitis is the main cause of implant failure. The risk of peri-prosthetic complications after 10 years ranges from 20 to 56% (3, 4); however, currently there are no acceptable, standardized protocols for treatment and consequently, recurrence rates remain high (5). Increasing the life expectancy of Ti prostheses is thus a major challenge in orthopedics and oral rehabilitation.
Oral peri-implantitis is believed to have a microbial etiology. However, a strong body of evidence links implant failure to aseptic inflammation around implants due to shedding of debris, Ti ions, and particles (1). Similarly, aseptic loosening of orthopedic implants has been attributed to Ti debris and particles (6). Although Ti prostheses are very biocompatible, these Ti byproducts are far from being bio-inert. Soft tissue biopsies around failing orthopedic (7, 8) and oral (9–11) implants revealed severe inflammatory reactions around aggregates of Ti particles.
We have previously shown that Ti particles released from ultrasonic (US) scaling around dental implants induce a marked inflammatory response in macrophages, with increased expression of pro-inflammatory cytokines, mainly IL1β, IL6, and TNFα. These Ti particles activate osteoclastogenesis in vitro and trigger inflammatory bone resorption in vivo (12).
Our previous results led us to further investigate the mechanism by which Ti particles entrain bone resorption and to investigate the therapeutic potential of neutralizing antibodies against IL1β, IL6, or TNFα in preventing Ti particle-induced osteolysis.
Materials and Methods
All procedures involving animals were carried out in accordance with the guidelines of Tel Aviv University and were approved by the Institutional Animal Care and Use Committee (permit number M-015–047).
Cell Culture
Primary bone marrow-derived macrophages (BMDMs) were isolated from the femora and tibiae of adult C57BL/6J mice (Envigo, Israel), as previously described (13). Briefly, cells were cultured overnight in 6-well dishes at 37°C in a humidified atmosphere with 5% CO2 in our “standard medium” consisting of alpha-modified Eagle's medium (αMEM, Life Science Technology, NY, USA) and 10% fetal bovine serum (FBS, Rhenium, Ltd, Modi'in, Israel). After 24 h, the non-adherent fraction was cultured in 10-cm non-culture-treated dishes containing standard medium and 100 ng/ml macrophage colony stimulating factor (M-CSF), prepared as previously described (14). The resulting adherent BMDMs were collected after 3 days for the specific assays described below.
Particle Generation
To obtain Ti particles that correspond to the particles shedding from oral implants during routine scaling, we subjected Ti discs that were made from Ti6Al4V (AlphaBio Tec., Petah-Tikva, Israel) to ultrasonic scaling (Newtron Led, Satelec, Acteon, Marignac, France), adjusted to a frequency of 32 kHz. Particles were obtained from discs with a machined (M), sand-blasted and acid-etched (SLA) or sand-blasted (SB) surface topography as described previously (12). When not specified, SLA-derived particles were used. All particles were generated in a sterile environment. Each disc was subjected to US scaling for 60 s in distilled water (ddH2O), then cleaned twice with ethanol, and finally resuspended in distilled water. We previously showed that each 6 mm diameter disc generates ~2.54 million particles on average. In all our in vitro assays and for the preparation of the fibrinogen-thrombin membranes (see below) we used a particle density of 1,293 particles/mm2.
Environmental Scanning Electron Microscopy (E-SEM)
To examine the cellular response of macrophages to Ti particles, BMDM were seeded on glass slides in a 10-cm plate (106 cells per well) and cultured for 24 h in the presence of Ti particles released by the US scaling of SLA-treated discs. Cultures were then visualized by E-SEM. Each field was taken either in backscattered electrons mode (BSE) or secondary electrons (SE) mode to distinguish between extracellular vs. total Ti particles in the culture, respectively.
Protein and Nitric Oxide (NO) Measurements in Conditioned Medium, RNA Isolation, and RT-qPCR
Following a 24–48 h incubation with Ti particles (or LPS or vehicle only), the supernatant was collected and referred to as conditioned medium (CM). Secreted protein amounts of pro- and anti-inflammatory cytokines in CM were measured using a multiplex assay and expressed in MFI units (Multiplex Fluorescent Immunoassay, ProcartaPlex Multiplex Immunoassay, eBioscience, San Diego, CA, USA). NO content was measured using the Griess Reagent System kit (Promega, Madison, WI, USA). After collecting the supernatant, macrophages were washed with sterile PBS, and RNA was extracted using Tri-RNA Reagent (Favorgen Biotech Corp, Kaohsiung, Taiwan). The 260/280 absorbance ratio was measured to verify the RNA purity and concentration. cDNA was produced using a high-capacity cDNA reverse transcription kit (Invitrogen, Grand Island, NY, USA), and real-time PCR was performed using Kapa SYBR Fast qPCR (Kapa Biosystems, Wilmington, MA, USA) on a StepOne real-time PCR machine (Applied Biosystems, Grand Island, NY, USA). We examined the expression of IL1β, IL6, and TNFα, which are established markers of macrophage inflammation. The primer sets were as follows: F-GAA ATG CCA CCT TTT GAC AGTG and R-TGG ATG CTC TCA TCA GGA CAG for mouse IL1β; F-TAG TCC TTC CTA CCC CAA TTT CC and R-TTG GTC CTT AGC CAC TCC TTC for mouse IL6; and F-TCT TCT CAT TCC TGC TTG TGG and R-GGT CTG GGC CAT AGA ACT GA for mouse TNFα. The reaction was subjected to 40 cycles of amplification using the following program: 95°C for 20 s, 60°C for 20 s, and 72°C for 25 s. The relative mRNA expression levels of the selected genes were normalized to the level of GPDH, which was amplified using the following primers: F-ACC CAG AAG ACT GTG GATG G and R-CAC ATT GGG GGTA GG AACAC.
Osteoclastogenesis Assay
Preosteoclasts, prepared like the BMDMs, were plated in 96-well plates (7,000 cells per well) in standard medium supplemented with 20 ng/ml M-CSF and 50 ng/ml Receptor Activator of Nuclear Factor Kappa-B Ligand (RANKL) (R&D Systems, Minneapolis, MN, USA). On the 3rd day (after 48 h), the medium was replaced by the CM of BMDM, supplemented with RANKL and M-CSF. Where indicated, we also added 2 μg/ml of neutralizing antibodies (Ab) against IL1β (Kineret, anakinra, SOBI, Stockholm, Sweden), IL6-receptor (Actemra, tocilizumab, Roche, San Francisco, CA, USA), or TNFα (Humira, adalimumab, AbbVie Inc., Chicago, IL, USA). These neutralizing Ab, effective in both humans and mice (15–19), are referred to as anti-IL1β, IL6, and TNFα Ab, respectively. On the 4th day, cells were stained using a TRAP kit (Sigma-Aldrich, St. Louis, MO, USA), and multinucleated (>3 nuclei) TRAP-positive cells were defined as osteoclasts. Images were acquired at an original magnification of × 4 (Evos FLC, Life Technologies, MS, USA). The number of osteoclasts and the total osteoclast area were measured using ImageJ software (National Institutes of Health, Bethesda, MD, USA).
Animal Model and Micro-Computed Tomography (μCT)
We used our calvarial model, which was described before (12). Briefly, US-released Ti particles (from M/SLA/SB-treated discs) were incorporated into a fibrinogen-thrombin degradable membrane used as a scaffold to localize the Ti particles. Membranes with no particles or only LPS were prepared as positive and negative controls. As indicated, neutralizing antibodies against TNFα (or saline as control) were incorporated into the membrane together with the Ti particles.
After anesthesia, the skin of 10-week-old C57Bl/6J female mice was shaved and disinfected. The parietal bones of the mice were exposed via a 10-mm incision in the nape area, and the periosteum was removed using a periosteal elevator. The fibrinogen-thrombin membranes were inserted to cover both parietal bones. The surgical incision was then closed using nylon monofilament surgical sutures (5/0). In the sham controls, no membranes were inserted and the incisions were closed. Another control group consisted of inserting an empty fibrinogen-thrombin membrane (with no Ti particles).
To test the therapeutic potential of systemic administration of neutralizing antibodies, we injected antibodies against IL1β, IL6, or TNFα (or saline as control) intraperitoneally 1 day before and once a week (2 mg/kg IL6 and 10 mg/kg TNFα) or daily (10 mg/kg IL1β) after membrane insertion, in accordance with the established clinical procedures (20–23).
All groups comprised a minimum of 6 animals. Animals were euthanized at the indicated post-operative time, and the skull of each mouse was removed, fixed for 24 h in 4% phosphate-buffered formalin, followed by 70% ethanol. All specimens were scanned and analyzed using a μCT system (μCT 50, Scanco Medical AG, Switzerland). Scans were performed at a 10-μm resolution in all three spatial dimensions, with 90 kV energy, 88 μA intensity, and 1,000 projections at a 1,000 m s integration time. The region of interest (ROI) was defined as two 3.7-mm circles in the center of the parietal bones. A custom-made algorithm, based on Image-Processing Language (IPL, Scanco Medical), was developed to isolate the resorption pits, defined as unmineralized pits that were 10 to 40-μm deep on the bone surface (12). Morphometric parameters were determined at the 3D level and included the total volume of bone pits (Pit Resorption Volume, PRV, mm3) and the bone tissue volume inside ROI (TV, mm3), which was used to determine the PRV/TV (%).
FACS Analyses
Using our calvarial model, we also determined the effect of Ti particles on macrophage polarization, in vivo. The mice received either control membranes (fibrinogen-thrombin only) or membranes including LPS/Ti particles. Mice were euthanized 3 weeks post-op. This time point was chosen to be after the acute inflammatory response induced by the surgery but before the potential resolution of the inflammatory response to the Ti particles. The soft tissue covering the parietal bones was collected and processed using collagenase. Cells were labeled using specific markers (CD11b, Ly6-C, Ly6-G, F4/80, and MHC-II). This panel was used to define macrophages and to differentiate between M1 (CD11b+, Ly6C+, Ly6G+, F4/80+, MHC-II+) and M2 (CD11b+, Ly6C-, Ly6G+, F4/80+, and MHC-II+) polarization (24, 25). Cells were fixed with 1% paraformaldehyde and analyzed on a Gallios flow cytometer (Beckman Coulter, Brea, CA, USA).
Results
Environmental Scanning Electron Microscopy (E-SEM)
To determine whether particles are internalized by the cells, a culture of BMDM and Ti particles was examined by E-SEM (Figure 1). Each field was taken either in BSE or SE mode. BSE provides a topographic view of the upper-most layer, which means that the Ti particles seen in this mode were not internalized by the cells but rather, were laid on top of the cell membrane (Figures 1A,B). The SE mode is a deep penetrating mode, able to detect all metals in the sample, within or outside the cells (Figures 1C,D). Based on the similarity between the two scanning modes (the total number of Ti particles and particles in the upper-most layer), it can be concluded that BMDM failed to internalize most of the US-released Ti particles.
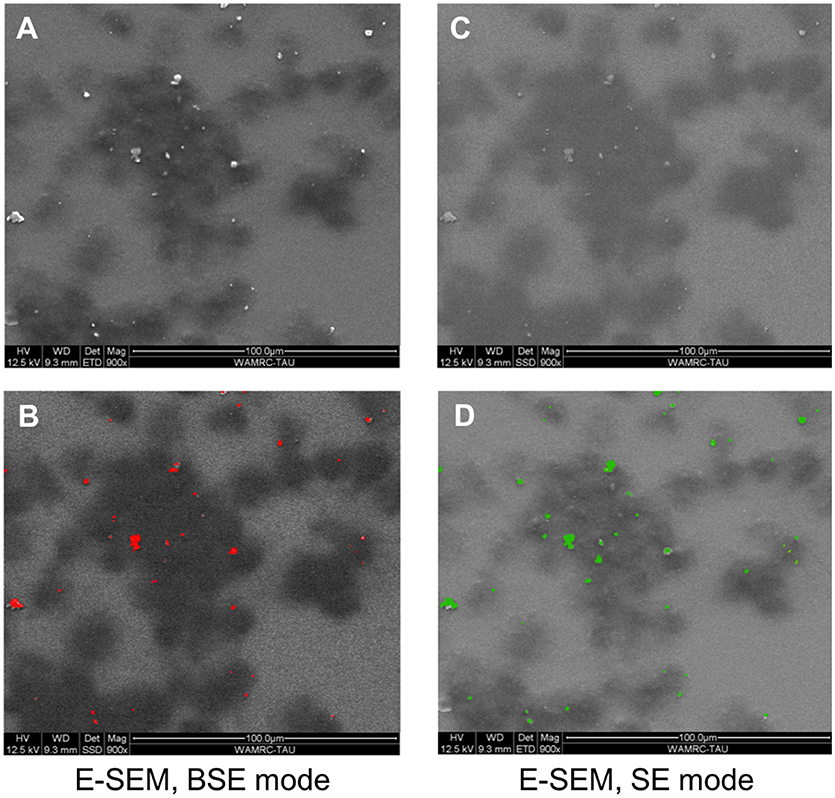
Figure 1. BMDM in the presence of Ti particles, visualized using E-SEM. BMDM were cultured for 24 h in the presence of SLA-derived Ti particles. Each field was taken either in backscattered electrons (BSE) mode or secondary electrons (SE) mode. Dark patches represent macrophages; light-bright dots (A,C) are Ti particles. (B,D) Color representation of the Ti particles (red in BSE mode, green in SE mode). Note that most particles are outside the cells, as indicated by the similarity between the BSE and SE modes.
Effect of Ti Particles on Macrophage Polarization
The effect of Ti particles on macrophage polarization was examined in vivo, using a mouse calvarial model (12). Either Ti particles (from M/SLA/SB-treated discs) or LPS was incorporated into a fibrinogen-thrombin degradable membrane. Mice were euthanized 3 weeks post-op to examine the macrophages inside the soft tissue covering the parietal bones. Our FACS analysis first gated a CD11b+/F480+/MHC-II+ monocyte/macrophage population, which was further divided into Ly6G-/Ly6C-(Gr1-) non-inflammatory monocytes/macrophages (26), Ly6G+/Ly6C+ M1 and Ly6G+/Ly6C- M2 macrophages (24, 25). Our results show no appreciable difference in the M2 population in either group (Figure 2). However, the M1 population was significantly higher in animals exposed to either machined or SLA surface-derived Ti particles. Surprisingly, milder differences were recorded for the SB particles. LPS alone also did not cause an appreciable increase in the M1 macrophage population (Figure 2). It is reasonable to assume that the fibrinogen membrane by itself caused some inflammatory changes that masked the differences between LPS and the empty membrane (control).
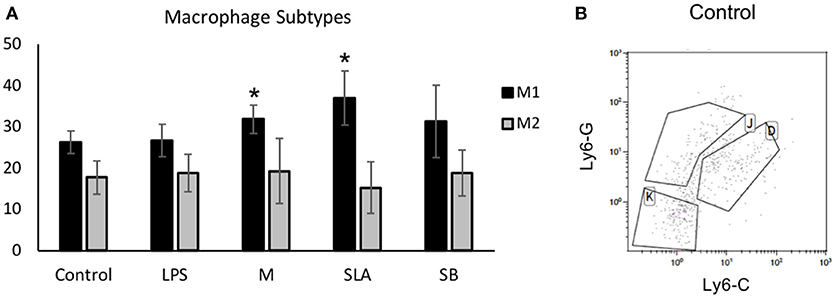
Figure 2. Ti particles induce macrophage polarization in vivo: Control fibrin membranes or membranes with either LPS or Ti particles were implanted onto mice calvaria (n = 5). Ti particles were obtained from machine (M), SLA or sand-blasted (SB) discs. Mice were euthanized after 3 weeks for soft tissue FACS analysis of macrophage polarization, using specific markers. Results show (A) the percentage of gated cells in the macrophages' sub-types, presented as mean ± SD; *p < 0.05 vs. control, 1-way ANOVA. (B) Representative FACS plot (K: monocytes, J: M2 and D: M1 macrophages).
Cytokine Secretion Profile of Macrophages Exposed to Ti Particles
To further reveal the mechanism underlying the particle-induced inflammatory response, the proteomic profile of macrophages exposed to Ti particles was assessed using the multiplex cytokine kit, after 24 h exposure to either LPS or SLA-derived particles. For most cytokines, the presence of Ti particles induced a 2- to 10-fold elevation in the proinflammatory cytokine levels (Figure 3). A modest (~2-fold) elevation in the anti-inflammatory IL1α and IL10 levels was also found, as well as in IFNγ. With respect to IL1β, IL6, and TNFα, our multiplex analysis showed a marked increase in their levels in the supernatant of macrophages cultured with Ti particles, in line with our previously published RT-qPCR analysis (12). Overall, this cytokine profile is characteristic of an M1 polarization, supporting our findings in vivo (Figure 2). We also observed a significant increase in NO secretion, which is a hallmark of M1 macrophage polarization (27).
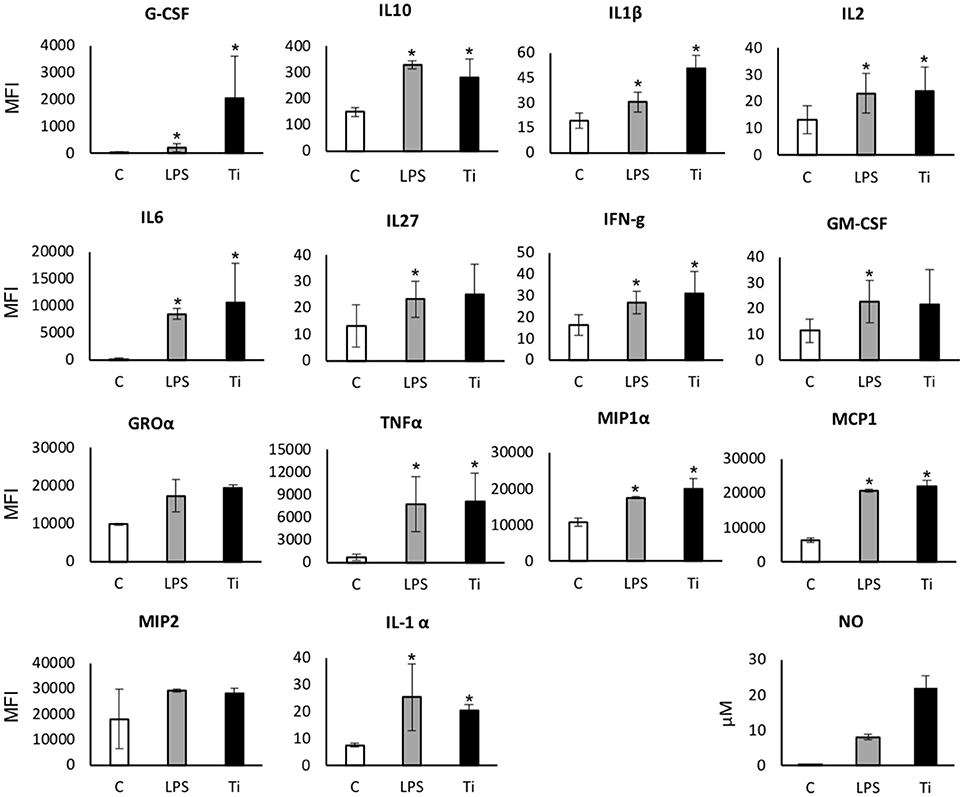
Figure 3. Secreted protein and NO levels in the supernatant of macrophages exposed to LPS/Ti particles. BMDM were cultured in the presence of either LPS or SLA-derived Ti particles. After 24 h, the supernatant was collected and analyzed using multiplex cytokine assay for secreted protein levels, and Griess Reagent System kit for NO. Results are presented as mean Melt Flow Index (MFI) units ±SD, and μM (for NO). n = 4. Experiment was repeated once with similar results. *p < 0.05 vs. control; non-parametric ANOVA.
Anti-inflammatory and Anti-osteoclastogenic Effects of Neutralizing Antibodies in BMDM and Preosteoclast Cultures
The concomitant increase in all inflammatory cytokines (Figure 3) raised a question regarding a positive paracrine feedback loop that fuels the inflammatory response of the macrophages. To determine whether blocking the paracrine effect of the cytokines secreted in response to Ti particles attenuates the overall inflammatory response of the macrophages, BMDM were cultured in the presence of Ti particles and treated with neutralizing antibodies for IL1β, IL6, or TNFα. Cells were collected after 24 h and changes in the levels of pro-inflammatory cytokines were measured using RT-qPCR. The secretion of IL1β was not affected by either of the neutralizing antibodies in the culture (Figure 4). On the other hand, IL6 expression in macrophages reduced significantly in the presence of each of the neutralizing antibodies. In contrast, TNFα expression in the culture was only affected by anti-TNFα antibodies. These findings suggest that the overall inflammatory response to Ti particles not only results from direct contacts between macrophages and Ti particles, but also results from positive feedback induced by paracrine signals.
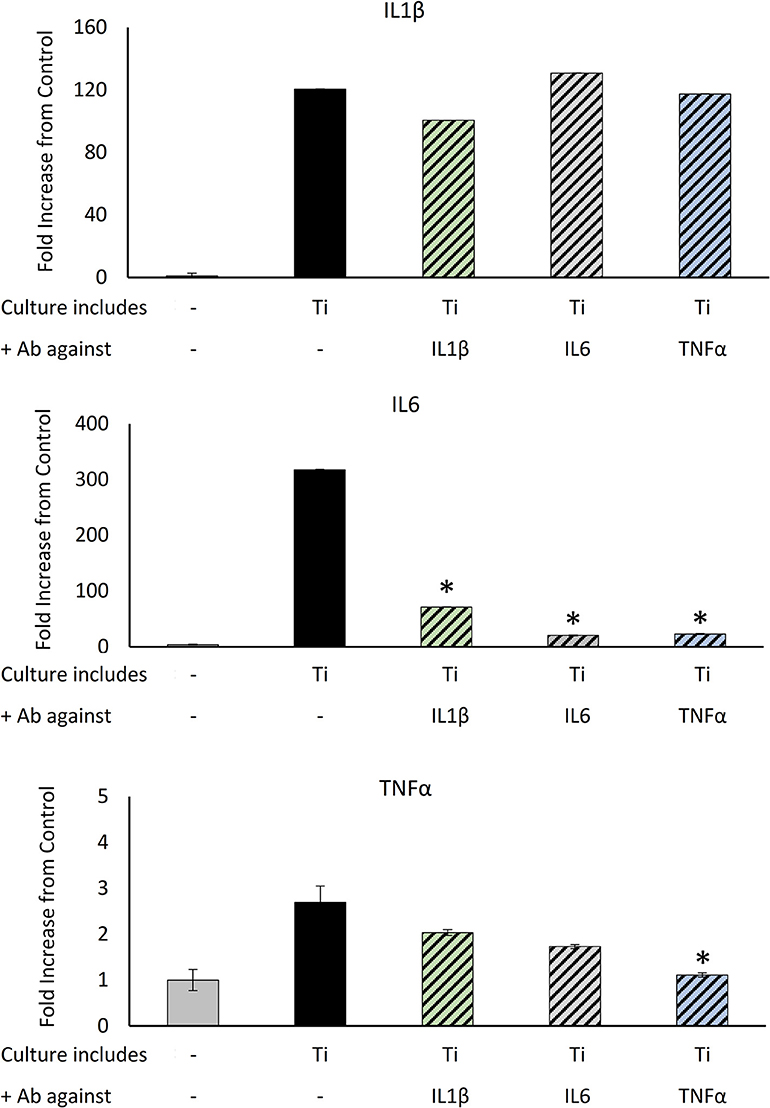
Figure 4. The effect of neutralizing Ab on Ti particle-induced inflammatory response: BMDM were cultured for 24 h in the presence of SLA-derived Ti particles released by US with neutralizing Ab for IL1β, IL6, or TNFα. IL1β, IL6, and TNFα expression levels were measured using RT-qPCR, normalized to GAPDH, and expressed as a fold change relative to the control (no particles). Data are shown as the mean ± SD. *p < 0.05 vs. Ti, non-parametric ANOVA, n = 3.
Next, we tested the potential antagonizing effect of the same neutralizing antibodies on the osteoclastogenic signals emitted by macrophages in the presence of Ti particles. To this end, preosteoclasts cultured under osteoclastogenic conditions for 48 h were supplemented with the CM of BMDM cultured with or without Ti particles. Concomitantly, neutralizing antibodies against IL1β, IL6, or TNFα were added as indicated (Figure 5). Cultures were stopped on the 4th day, and the total area covered by multinucleated (>3 nuclei) TRAP-positive osteoclasts was calculated. Treatment with anti-IL6 antibodies did not affect osteoclastogenesis in our culture of isolated preosteoclasts. However, neutralizing antibodies against either IL1β or TNFα suppressed osteoclastogenesis in the presence of CM from BMDM exposed to Ti particles (Figure 5).
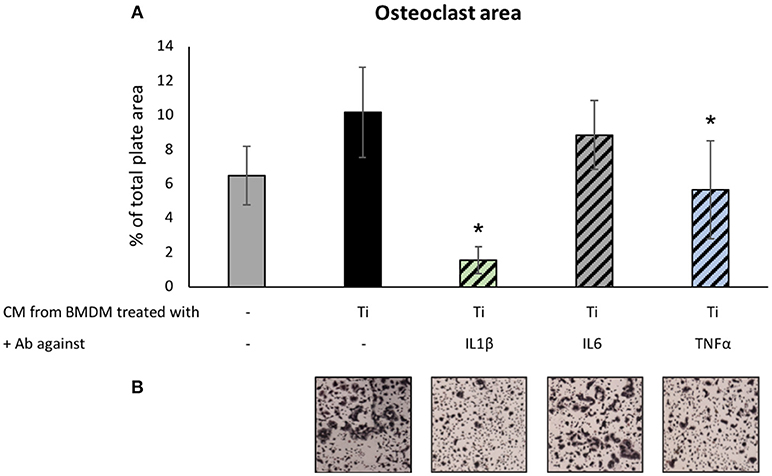
Figure 5. The effect of neutralizing Ab on Ti-particle-induced osteoclastogenesis: Preosteoclast cultures under osteoclastogenic conditions for 48 h were supplemented with condition medium (CM, supernatant) of BMDM cultured with or without SLA-derived Ti particles. At the same time, neutralizing antibodies against IL1β, IL6, or TNFα were added as indicated. Cultures were stopped after 36 h. (A) Average osteoclast area expressed as mean ± SD, n = 8. *p < 0.05 vs. Ti, 1-way ANOVA. (B) Representative images of TRAP-stained osteoclasts for each condition. Original magnification × 4.
Dynamics of Ti Particle-Induced Osteolysis
To account for the dynamics of the inflammatory response, we first established the time course of particle-induced osteolysis in our calvarial model. Ti particles released by the US scaling of SLA-treated discs were incorporated into the inserted membranes. A sham group consisted of mice undergoing the same surgical procedure without membrane insertion, which were euthanized after 4 weeks. Mice carrying a Ti particle-loaded membrane were euthanized every 2 weeks to monitor the extent of calvarial resorption using μCT. The results indicate that a dramatic resorption occurred during the first 5 weeks, along with a recovery from the 6th week onward. Of note, the membrane was totally degraded within 4 to 5 weeks; however, the presence of Ti particles was still detected even after 10 weeks (Figure 6C). This suggests that the osteolytic response is self-contained and somewhat recovers within 8 weeks after the last insult of Ti particles. Importantly, despite this recovery, the PR volume after 8 and 10 weeks remained significantly higher in the Ti than in the sham group.
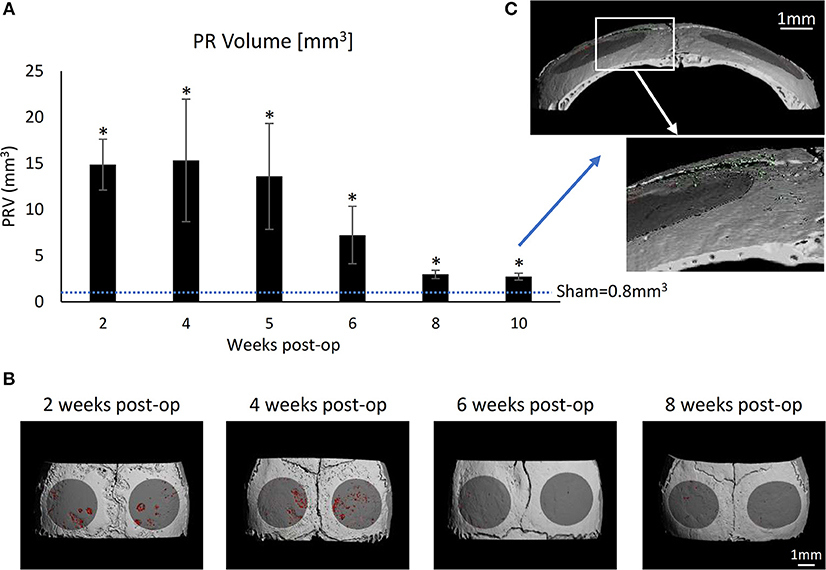
Figure 6. Time-lapse development of particle-induced bone resorption in vivo: Fibrin membranes loaded with SLA-derived Ti particles, were implanted onto mouse calvaria. Mice were euthanized every 2 weeks for μCT analysis to evaluate the dynamic progression of particle-induced osteolysis. (A) Pit Resorption Volume (PRV, mm3); data are expressed as mean ± SD, n = 5, *p < 0.05 vs. sham (no membrane), non-parametric ANOVA. (B) Representative μCT images of the calvaria are shown. The region of interest (ROI) is denoted in dark gray, and the resorption pits are denoted in red. (C) High magnification of a sample from the 10-week group indicating the residual Ti particles (green) despite the complete resorption of the membrane.
Based on these results, further treatments were given for 4 weeks. After 4 weeks, the calvariae were collected and processed for μCT.
Therapeutic Potential of Neutralizing Antibodies on Ti Particle-Induced Osteolysis
We repeated the same calvaria model with membranes loaded with SLA-derived Ti-particles. Neutralizing antibodies for IL1β, IL6, or TNFα (or saline as control) were injected intraperitoneally 1 day before, once a week (IL6 and TNFα), or daily (IL1β) after the membrane insertion. As shown in Figure 7, each of the 3 neutralizing antibodies had a dramatic, statistically significant effect in preventing particle-induced osteolysis.
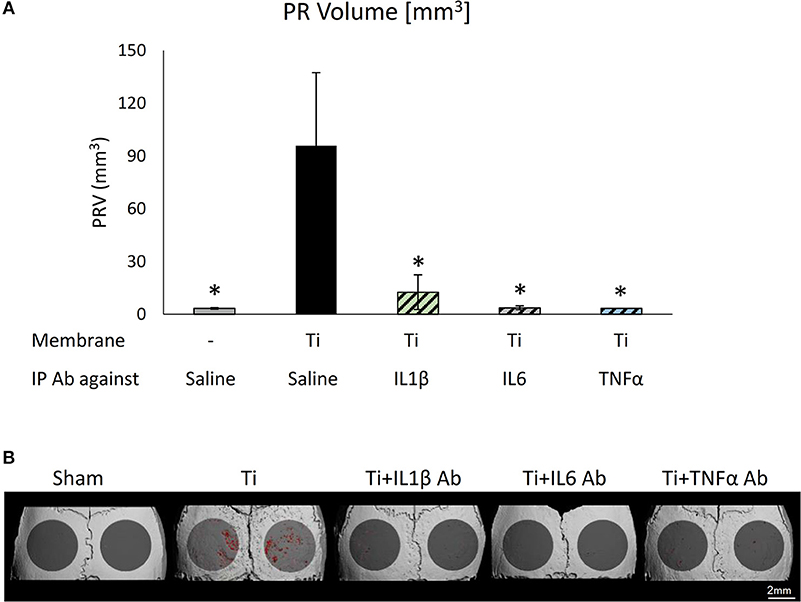
Figure 7. The effect of systemically administering anti-inflammatory neutralizing antibodies on particle-induced osteolysis in vivo: Fibrin membranes including SLA-derived Ti particles were implanted onto mouse calvaria. Neutralizing antibodies (Ab) against IL1β, IL6, or TNFα (or saline as control) were injected intraperitoneally (IP) 1 day before, once a week (IL6 and TNFα), or daily (IL1β) after membrane insertion. Mice were euthanized 4 weeks post-op for μCT analysis. (A) Pit Resorption Volume (PRV, mm3), data are expressed as mean ± SD, n = 6; *p < 0.05 vs. Ti, 1-way ANOVA. (B) Representative μCT images of the calvaria, color-coded as above.
Next, we tested the therapeutic potential of topically administered neutralizing antibodies. As a proof-of-concept, we elected to test anti-TNFα neutralizing antibodies incorporated into the membrane together with the Ti particles (Figure 8). Importantly, we found that topically-administered neutralizing antibodies significantly suppressed the particle-induced osteolysis (Figure 8).
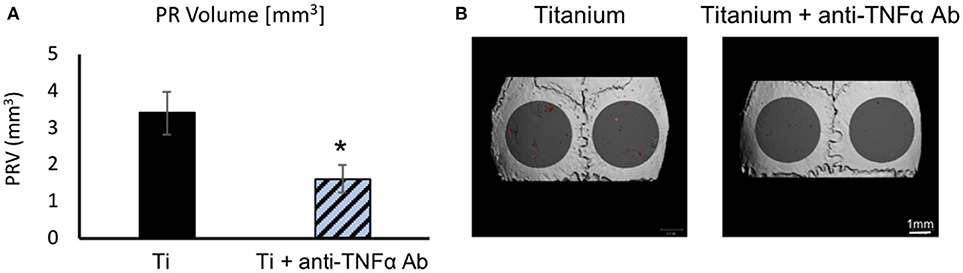
Figure 8. The effect of topically-administered anti-inflammatory neutralizing antibodies on particle-induced osteolysis in vivo: Fibrin membranes including Ti particles, together with neutralizing antibodies against TNFα (or saline as control), were implanted onto mouse calvaria. Mice were euthanized 4 weeks post-op for μCT analysis. (A) Pit Resorption Volume (PRV, mm3), data are expressed as mean ± SD, n = 6; *p = 0.029 vs. Ti, t-test. (B) Representative μCT images of the calvaria color-coded as above.
Discussion
In this study, we examined the mechanism by which Ti particles lead to inflammation and bone resorption and tested the therapeutic potential of using specific neutralizing antibodies in preventing particle-induced osteolysis.
Our multiplex analysis revealed an interesting secretion profile of macrophages cultured in the presence of Ti particles. There was a dramatic elevation in the levels of most pro-inflammatory cytokines relative to the modest rise in the anti-inflammatory IL1α and IL10 levels, which may be a compensatory restraining response (28, 29). IFNγ also displayed a significant rise; however, its contribution to the inflammatory response remains unclear, since both pro- and anti-inflammatory actions have been reported for this cytokine (30–32). MIP1α and MCP1 are chemokine proteins (CCL3 and CCL2, respectively). MIP1α is involved in the acute inflammatory state and is responsible for recruitment of polymorphonuclear cells, whereas MCP1 was found in the vicinity of bone resorption sites (33). The latter chemokine may drive osteoclast differentiation in the absence of RANKL (34). With respect to IL1β, IL6, and TNFα, our multiplex analysis revealed a trend very similar to our RT-qPCR analysis reported recently (12), with significantly elevated levels in the supernatant of macrophages cultured with Ti particles. In general, Ti particles induce in macrophages a response similar to that of LPS. The resulting inflammatory response drives the bone tissue damage mediated by osteoclasts. Together with the FACS, gene expression and secretome profiling on macrophages in vitro and in vivo, these changes indicate that macrophages undergo an “M1-like” polarization in response to Ti particles. It should be noted however that all our assays were conducted at the early stages of the inflammatory response. The resolving inflammation manifested in the partial tissue repair observed in vivo after 6–8 weeks (Figure 6), suggests a more dynamic and complex spatio-temporal distribution of M1 and M2 macrophages (27).
Next, we examined how neutralizing antibodies against IL1β, IL6, and TNFα affected Ti particle-induced inflammatory response in macrophages. Importantly, we found that (i) the secretion of IL1β was not affected by either of the neutralizing antibodies, (ii) IL6 expression was significantly decreased by the presence of each of the neutralizing antibodies, and (iii) TNFα expression in culture was only affected by anti-TNFα antibodies. This is in accordance with the notion that both TNFα and IL1β are upstream factors in the inflammatory cascade (35). Indeed, our findings suggest that IL1β expression is independent of the secretion of IL1β, IL6, and TNFα by neighboring macrophages. In contrast, most IL6 expression by macrophages depends on paracrine signals, including IL1β, IL6, and TNFα. TNFα expression is only partly dependent on these signals, since only blockade of TNFα partly suppressed its own expression. Importantly, we also observed that in vivo, blocking any of these 3 cytokines significantly attenuated bone resorption. Based on these observations we propose a model to describe this additive and synergistic interrelationship between these 3 cytokines and the resulting stimulation of osteoclasts, thus inducing bone resorption (Figure 9). In this model, blocking IL1β does not affect TNFα expression and vice versa, and blocking IL6 does not affect either IL1β or TNFα expression. Moreover, both IL1β and TNFα directly stimulate osteoclasts while IL6 does so indirectly via the osteoblasts. The latter is suggested by the significant blockade of osteolysis by anti-IL6 antibodies administered in vivo, but not in vitro in the absence of stromal cells in the cultures. This conclusion is also in line with another study demonstrating that IL6 stimulates osteoclastogenesis via osteoblasts (36). Overall, our model suggests that Ti particles stimulate osteoclastogenesis via 3 pathways, the predominant one being the synergistically increased expression of IL6 by IL1β and TNFα, which in turn stimulates osteoblast-mediated osteoclastogenesis. It is reasonable to assume that both in vitro and in vivo, not all macrophages are in direct contact with Ti particles. The paracrine effect, depicted here, portrays a chain reaction that could provide the inflammatory signals with an extended range.
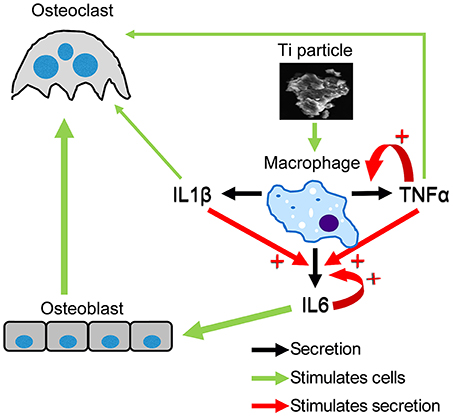
Figure 9. Putative model of the hierarchical roles of IL1β, IL6, and TNFα in particle-induced osteoclastogenesis. This model describes the paracrine and autocrine induction of cytokine secretion by macrophages exposed to SLA-derived Ti particles. The thickness of the green arrows reflects the importance of one pathway over the others.
We conducted here a time-lapse experiment and evaluated the long-term effects of Ti particles on bone resorption in vivo (Figure 6). Our data show that the osteolytic response is self-contained and somewhat recovers, although not entirely. After 8 and 10 weeks, the extent of bone residual defects remained significantly higher than in the sham group. Moreover, in clinical settings, a Ti prosthesis is likely to continuously release ions, debris, and particles, thus fueling the inflammatory response, and further aggravating osteolysis.
Previous articles studied different approaches to block the progression of bone resorption. These approaches included promoting apoptosis of osteoclasts, genetic intervention (37, 38) or using bisphosphonates, which caused pathological fractures (39).
In our previous publication, we characterized the size, number, physical and chemical properties of the titanium particles shedding from the surface of rough titanium implant commonly used in contemporary dentistry following ultrasonic scaling (12). Regarding the size, we used an automated cell counter to measure the number and size of the particles shedding from one 6-mm diameter titanium disc with an SLA surface. We found that ultrasonic scaling of each SLA disc generates 2.54 million titanium particles of an average size of 6 to 12 μm. A previous study established that the range of wear debris generated from orthopedic prostheses is between 1 and 30 μm (40). It is thus likely to assume that the cellular and inflammatory responses described in the current study with ultrasonic-generated particles are similar to those observed with wear debris surrounding orthopedic prostheses.
Three main biological strategies were tested to block the chronic inflammatory reaction to orthopedic wear particles, including (i) interference with systemic macrophage trafficking to the local implant site, (ii) modulation of macrophages from an M1 to an M2 phenotype in periprosthetic tissues, and (iii) local inhibition of the transcription factor nuclear factor-kappa B, thereby interfering with the production of pro-inflammatory mediators (38). All three approaches showed promising results in preclinical studies but have not yet been evaluated clinically.
Here we tested three clinically-approved neutralizing antibodies that are prescribed for the management of auto-immune and inflammatory diseases. Anti-IL1β antibodies (Anakinra) are prescribed to rheumatoid arthritis and neonatal-onset multisystem inflammatory disease, anti-IL6 receptor antibodies (Tocilizumab) are administered to treat arthritis and anti-TNFα antibodies (Adalimumab) are effective against rheumatoid arthritis, psoriasis and Crohn's disease (41–43). Our in vivo and in vitro results showed a clear association between each of these cytokines and the macrophage response to Ti particles. Using our mouse calvarial model, we demonstrated that blocking each of these cytokines prevents Ti particle-induced osteolysis. We further showed that both systemic and local administration are conceptually possible approaches. This preclinical study therefore advocates that neutralizing antibodies be further tested against IL1β, IL6, or TNFα in clinical settings for managing the aseptic loosening of orthopedic prostheses and oral peri-implantitis.
Author Contributions
ME, SH-B, TL, NS, YC, DK, and YG: contributed to the design of the research; ME, SH-B, and TL: performed research and contributed to the obtained results; YG and DK: supervised the project; ME and YG: wrote the paper and prepared it for publication. All authors read and approved the final manuscript.
Funding
This work was supported by Israel Science Foundation (ISF) Grants No. 1822/12, 1367/12, and 1086/17 to YG, and a Rothstein-Foundation grant to DK, NS, and YG.
Conflict of Interest Statement
The authors declare that the research was conducted in the absence of any commercial or financial relationships that could be construed as a potential conflict of interest.
Acknowledgments
We acknowledge our deepest appreciation to Prof. Sandu Pitaru (Tel Aviv University) for his helpful advices and discussion during this study. This work was carried out in partial fulfillment of the requirements for a Ph.D. degree for ME from the Sackler Faculty of Medicine, Tel Aviv University, Tel Aviv, Israel.
References
1. Revell PA. The combined role of wear particles, macrophages and lymphocytes in the loosening of total joint prostheses. J R Soc Interface (2008) 5:1263–78. doi: 10.1098/rsif.2008.0142
2. Moraschini V, Poubel LA, Ferreira VF, Barboza Edos S. Evaluation of survival and success rates of dental implants reported in longitudinal studies with a follow-up period of at least 10 years: a systematic review. Int J Oral Maxillofac Surg. (2015) 44:377–88. doi: 10.1016/j.ijom.2014.10.023
3. Landgraeber S, Jager M, Jacobs JJ, Hallab NJ. The pathology of orthopedic implant failure is mediated by innate immune system cytokines. Mediators Inflamm. (2014) 2014:185150. doi: 10.1155/2014/185150
4. Derks J, Tomasi C. Peri-implant health and disease. A systematic review of current epidemiology. J Clin Periodontol. (2015) 42 (Suppl. 16):S158–71. doi: 10.1111/jcpe.12334
5. Esposito M, Grusovin MG, Worthington HV. Interventions for replacing missing teeth: treatment of peri-implantitis. Cochrane Database Syst Rev. (2012) 1:CD004970. doi: 10.1002/14651858
6. Fretwurst T, Nelson K, Tarnow DP, Wang HL, Giannobile WV. Is metal particle release associated with peri-implant bone destruction? an emerging concept. J Dent Res. (2018) 97:259–65. doi: 10.1177/0022034517740560
7. Scales JT. Black staining around titanium alloy prostheses–an orthopaedic enigma. J Bone Joint Surg Br. (1991) 73:534–6.
8. Witt JD, Swann M. Metal wear and tissue response in failed titanium alloy total hip replacements. J Bone Joint Surg Br. (1991) 73:559–63.
9. Schlegel KA, Eppeneder S, Wiltfang J. Soft tissue findings above submerged titanium implants–a histological and spectroscopic study. Biomaterials (2002) 23:2939–44. doi: 10.1016/S0142-9612(01)00423-9
10. Olmedo DG, Paparella ML, Spielberg M, Brandizzi D, Guglielmotti MB, Cabrini RL. Oral mucosa tissue response to titanium cover screws. J Periodontol. (2012) 83:973–80. doi: 10.1902/jop.2011.110392
11. Wilson TGJr, Valderrama P, Burbano M, Blansett J, Levine R, Kessler H, et al. Foreign bodies associated with peri-implantitis human biopsies. J Periodontol. (2015) 86:9–15. doi: 10.1902/jop.2014.140363
12. Eger M, Sterer N, Liron T, Kohavi D, Gabet Y. Scaling of titanium implants entrains inflammation-induced osteolysis. Sci Rep. (2017) 7:39612. doi: 10.1038/srep39612.
13. Hiram-Bab S, Liron T, Deshet-Unger N, Mittelman M, Gassmann M, Rauner M, et al. Erythropoietin directly stimulates osteoclast precursors and induces bone loss. FASEB J. (2015) 29:1890–900. doi: 10.1096/fj.14-259085
14. Takeshita S, Kaji K, Kudo A. Identification and characterization of the new osteoclast progenitor with macrophage phenotypes being able to differentiate into mature osteoclasts. J Bone Miner Res. (2000) 15:1477–88. doi: 10.1359/jbmr.2000.15.8.1477
15. Goh AX, Bertin-Maghit S, Ping Yeo S, Ho AW, Derks H, Mortellaro A, et al. A novel human anti-interleukin-1beta neutralizing monoclonal antibody showing in vivo efficacy. MABS (2014) 6:765–73. doi: 10.4161/mabs.28614
16. Yu D, Ye X, Che R, Wu Q, Qi J, Song L, et al. FGF21 exerts comparable pharmacological efficacy with Adalimumab in ameliorating collagen-induced rheumatoid arthritis by regulating systematic inflammatory response. Biomed Pharmacother. (2017) 89:751–60. doi: 10.1016/j.biopha.2017.02.059
17. Zafir-Lavie I, Miari R, Sherbo S, Krispel S, Tal O, Liran A, et al. Sustained secretion of anti-tumor necrosis factor alpha monoclonal antibody from ex vivo genetically engineered dermal tissue demonstrates therapeutic activity in mouse model of rheumatoid arthritis. J Gene Med. (2017) 19. doi: 10.1002/jgm.2965
18. Hu J, Feng X, Valdearcos M, Lutrin D, Uchida Y, Koliwad SK, et al. Interleukin-6 is both necessary and sufficient to produce perioperative neurocognitive disorder in mice. Br J Anaesth. (2018) 120:537–45. doi: 10.1016/j.bja.2017.11.096
19. Wu R, Liu X, Yin J, Wu H, Cai X, Wang N, et al. IL-6 receptor blockade ameliorates diabetic nephropathy via inhibiting inflammasome in mice. Metabolism (2018) 83:18–24. doi: 10.1016/j.metabol.2018.01.002
20. Machold KP, Smolen JS. Adalimumab-a new TNF-alpha antibody for treatment of inflammatory joint disease. Expert Opin Biol Ther. (2003) 3:351–60. doi: 10.1517/14712598.3.2.351
21. Palframan R, Airey M, Moore A, Vugler A, Nesbitt A. Use of biofluorescence imaging to compare the distribution of certolizumab pegol, adalimumab, and infliximab in the inflamed paws of mice with collagen-induced arthritis. J Immunol Methods (2009) 348:36–41. doi: 10.1016/j.jim.2009.06.009
22. Martinez-Fernandez de la Camara C, Hernandez-Pinto AM, Olivares-Gonzalez L, Cuevas-Martin C, Sanchez-Arago M, Hervas D, et al. Adalimumab reduces photoreceptor cell death in a mouse model of retinal degeneration. Sci Rep. (2015) 5:11764. doi: 10.1038/srep11764
23. Benny Klimek ME, Sali A, Rayavarapu S, Van der Meulen JH, Nagaraju K. Effect of the IL-1 receptor antagonist kineret(R) on disease phenotype in mdx mice. PLoS ONE (2016) 11:e0155944. doi: 10.1371/journal.pone.0155944
24. Nahrendorf M, Swirski FK, Aikawa E, Stangenberg L, Wurdinger T, Figueiredo JL, et al. The healing myocardium sequentially mobilizes two monocyte subsets with divergent and complementary functions. J Exp Med. (2007) 204:3037–47. doi: 10.1084/jem.20070885
25. Rider P, Carmi Y, Guttman O, Braiman A, Cohen I, Voronov E, et al. IL-1alpha and IL-1beta recruit different myeloid cells and promote different stages of sterile inflammation. J Immunol. (2011) 187:4835–43. doi: 10.4049/jimmunol.1102048
26. Geissmann F, Jung S, Littman DR. Blood monocytes consist of two principal subsets with distinct migratory properties. Immunity (2003) 19:71–82. doi: 10.1016/S1074-7613(03)00174-2
27. Murray PJ. Macrophage polarization. Annu Rev Physiol. (2017) 79:541–66. doi: 10.1146/annurev-physiol-022516-034339
28. Fiorentino DF, Zlotnik A, Mosmann TR, Howard M, O'Garra A. IL-10 inhibits cytokine production by activated macrophages. J Immunol. (1991) 147:3815–22.
29. Baseler WA, Davies LC, Quigley L, Ridnour LA, Weiss JM, Hussain SP, et al. Autocrine IL-10 functions as a rheostat for M1 macrophage glycolytic commitment by tuning nitric oxide production. Redox Biol. (2016) 10:12–23. doi: 10.1016/j.redox.2016.09.005
30. Allen JB, Bansal GP, Feldman GM, Hand AO, Wahl LM, Wahl SM. Suppression of bacterial cell wall-induced polyarthritis by recombinant gamma interferon. Cytokine (1991) 3:98–106.
31. Wahl SM, Allen JB, Ohura K, Chenoweth DE, Hand AR. IFN-gamma inhibits inflammatory cell recruitment and the evolution of bacterial cell wall-induced arthritis. J Immunol. (1991) 146:95–100.
32. Muhl H, Pfeilschifter J. Anti-inflammatory properties of pro-inflammatory interferon-gamma. Int Immunopharmacol. (2003) 3:1247–55. doi: 10.1016/S1567-5769(03)00131-0
33. Cook DN. The role of MIP-1 alpha in inflammation and hematopoiesis. J Leukoc Biol. (1996) 59:61–6.
34. Kim MS, Day CJ, Morrison NA. MCP-1 is induced by receptor activator of nuclear factor-{kappa}B ligand, promotes human osteoclast fusion, and rescues granulocyte macrophage colony-stimulating factor suppression of osteoclast formation. J Biol Chem. (2005) 280:16163–9. doi: 10.1074/jbc.M412713200
35. Rider P, Carmi Y, Cohen I. Biologics for targeting inflammatory cytokines, clinical uses, and limitations. Int J Cell Biol. (2016) 2016:9259646. doi: 10.1155/2016/9259646
36. Udagawa N, Takahashi N, Katagiri T, Tamura T, Wada S, Findlay DM, et al. Interleukin (IL)-6 induction of osteoclast differentiation depends on IL-6 receptors expressed on osteoblastic cells but not on osteoclast progenitors. J Exp Med. (1995) 182:1461–8.
37. Wang H, Jia TH, Zacharias N, Gong W, Du HX, Wooley PH, et al. Combination gene therapy targeting on interleukin-1beta and RANKL for wear debris-induced aseptic loosening. Gene Ther. (2013) 20:128–35. doi: 10.1038/gt.2012.1
38. Goodman SB, Gibon E, Pajarinen J, Lin TH, Keeney M, Ren PG, et al. Novel biological strategies for treatment of wear particle-induced periprosthetic osteolysis of orthopaedic implants for joint replacement. J R Soc Interface (2014) 11:20130962. doi: 10.1098/rsif.2013.0962
39. Orozco C, Maalouf NM. Safety of bisphosphonates. Rheum Dis Clin North Am. (2012) 38:681–705. doi: 10.1016/j.rdc.2012.09.001
40. Schmiedberg SK, Chang DH, Frondoza CG, Valdevit AD, Kostuik JP. Isolation and characterization of metallic wear debris from a dynamic intervertebral disc prosthesis. J Biomed Mater Res. (1994) 28:1277–88. doi: 10.1002/jbm.820281105
41. Mertens M, Singh JA. Anakinra for rheumatoid arthritis. Cochrane Database Syst Rev. (2009) CD005121. doi: 10.1002/14651858.CD005121.pub3
42. Kearsley-Fleet L, Beresford MW, Davies R, De Cock D, Baildam E, Foster HE, et al. Short-term outcomes in patients with systemic juvenile idiopathic arthritis treated with either tocilizumab or anakinra. Rheumatology (2018). doi: 10.1093/rheumatology/key262. [Epub ahead of print].
Keywords: dental implants, peri-implantitis, macrophages, cytokines, osteoclasts
Citation: Eger M, Hiram-Bab S, Liron T, Sterer N, Carmi Y, Kohavi D and Gabet Y (2018) Mechanism and Prevention of Titanium Particle-Induced Inflammation and Osteolysis. Front. Immunol. 9:2963. doi: 10.3389/fimmu.2018.02963
Received: 03 September 2018; Accepted: 03 December 2018;
Published: 18 December 2018.
Edited by:
Asaf Wilensky, Hadassah Medical Center, IsraelReviewed by:
Gustavo Pompermaier Garlet, University of São Paulo, BrazilKeith Kirkwood, University at Buffalo, United States
Copyright © 2018 Eger, Hiram-Bab, Liron, Sterer, Carmi, Kohavi and Gabet. This is an open-access article distributed under the terms of the Creative Commons Attribution License (CC BY). The use, distribution or reproduction in other forums is permitted, provided the original author(s) and the copyright owner(s) are credited and that the original publication in this journal is cited, in accordance with accepted academic practice. No use, distribution or reproduction is permitted which does not comply with these terms.
*Correspondence: Yankel Gabet, yankel@tau.ac.il