- 1Institute of Clinical Chemistry and Pathobiochemistry, School of Medicine, Technical University of Munich, Munich, Germany
- 2German Cancer Consortium (DKTK), Heidelberg, Germany
- 3German Center for Infection Research (DZIF), Munich, Germany
Intestinal homeostasis requires a balanced interaction between the host innate immune system and the gut microbiota. A dysregulation of this interdependency can result in inflammatory bowel diseases (IBDs), and this dysregulation is a key pathogenic factor during the development of colorectal cancer. CARD9 is a central signaling molecule in the innate immune system, which is essential for host defense against infection. Moreover, polymorphisms in CARD9 are key risk factors for IBD development, indicating that CARD9 signaling is critical for intestinal immune homeostasis. This review summarizes recent insights into the regulation of CARD9 signaling, its pathophysiological role during IBD development via effects on the microbiota and epithelial regeneration and the pro- and antitumor immune functions of CARD9 during intestinal carcinogenesis.
Introduction
Homeostasis in the gastrointestinal tract depends on balanced interactions between the host immune system and the gut microbiome. The microbiome encompasses bacteria, fungi, and other microorganisms, and its composition is dynamically shaped by the host immune system, which continuously senses microbes and their metabolites and reacts with tolerogenic or stimulatory signals (1). Dysregulation of the immune-microbial balance due to the host's genetic composition, microbial dysbiosis, or environmental factors can cause inflammatory bowel diseases (IBDs), such as ulcerative colitis and Crohn's disease (2). Moreover, both overt chronic inflammation and smoldering inflammation are key risk factors for the development of gastrointestinal cancers (3). Therefore, understanding the reciprocal interaction between the host immune system and the intestinal microbiome is of great biomedical importance.
To sense microbial structures in the gut, innate immune cells are located in the lamina propria underneath the intestinal epithelium and in organized lymphoid structures. These cells are equipped with germline-encoded pattern recognition receptors (PRRs) that survey the intestinal environment and engage intracellular signaling pathways to induce the production of effector molecules that orchestrate local immunity. Importantly, a majority of genetic susceptibility loci that modulate the risk of developing IBD lie within genes involved in immune responses (4), which underscores the importance of immune signaling in IBD development. One very prominent IBD susceptibility gene whose key function in intestinal pathophysiology is being increasingly understood is CARD9, which encodes the innate immune signaling adapter protein CARD9 (4–8). This review will summarize recent insights into the molecular functions of CARD9 signaling and its role in intestinal inflammation and oncogenesis.
Molecular Mechanisms of CARD9 Signaling
CARD9 is selectively expressed in myeloid immune cells, including dendritic cells, macrophages, and neutrophils, and it is composed of an N-terminal caspase-activating and recruitment domain (CARD), a coiled-coil domain and a C-terminal tail with no specific domain structure (9) (Figure 1A). CARD9 can be activated by several plasma membrane-associated and intracellular PRRs (10, 11), and it is critical for the function of SYK-coupled C-type lectin receptors (CLRs). These CLRs, including Dectin-1 (12), Dectin-2 (13), and Mincle (14, 15), are key PRRs for the detection of commensal and pathogenic fungi but can also sense certain bacteria or danger signals released from dying host cells (16). The CLR-mediated activation of SYK leads to the activation of PKCδ via intermediate steps (Figure 1B), and PKCδ then directly phosphorylates CARD9 at T231 in the coiled-coil domain (17) in cooperation with VAV proteins (18). These molecular events enable CARD9 activation and the assembly of a BCL10- and MALT1-containing signaling platform, termed the CARD9-BCL10-MALT1 (CBM) complex, which serves as a scaffold to place ubiquitin modifiers and protein kinases in proximity to activate downstream effector pathways (9).
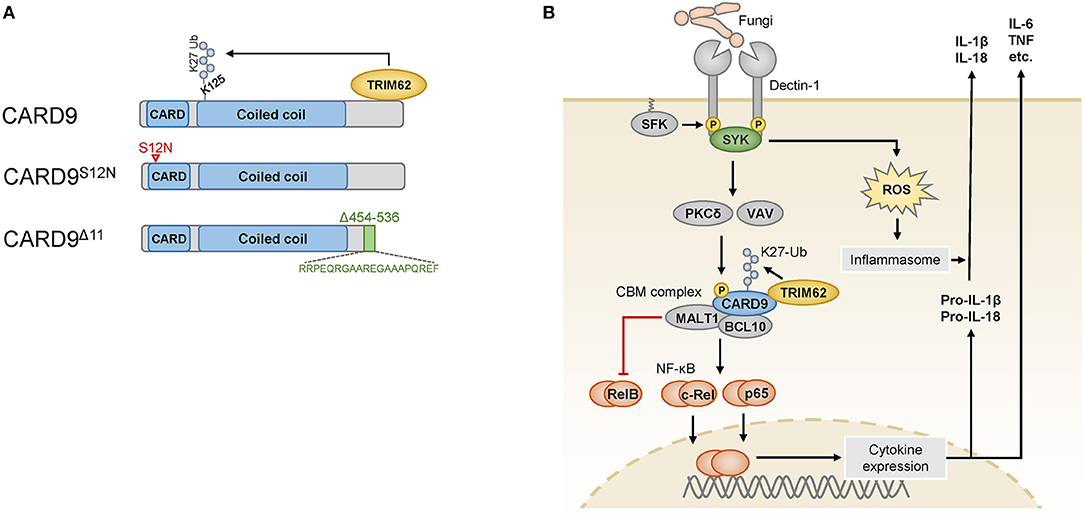
Figure 1. (A) The wild-type CARD9 protein consists of an N-terminal caspase activation and recruitment domain (CARD) followed by a coiled-coil domain and a C-terminal tail. The C-terminus mediates binding to the ubiquitin ligase TRIM62, which ubiquitinates CARD9 at K125. The CARD9S12N variant encoded by an IBD risk-associated SNP carries an amino acid substitution in the N-terminal portion of the CARD domain (indicated by the red arrowhead). The rare variant CARD9Δ11 results from the skipping of exon 11, and instead of the amino acids 454–536, it bears a shortened and altered C-terminus (indicated in green) that is unable to bind to TRIM62. (B) Fungi are sensed by myeloid cells through Dectin-1, which is phosphorylated by SRC-family kinases (SFK) in its cytoplasmic portion upon ligand binding. The spleen tyrosine kinase (SYK) can recognize these phosphosites and activate the protein kinase C (PKC) δ and VAV proteins, which cooperate to phosphorylate CARD9, enabling it to assemble the CARD9-BCL10-MALT1 (CBM) complex. K27 ubiquitination of CARD9 by TRIM62 enables the activation of NF-κB, which consists of the transcriptionally active subunits c-Rel, p65 and RelB. RelB is counterregulated by the proteolytic activity of MALT1. Cytokines produced after stimulation of this pathway include IL-6, TNF and pro-IL-1β, which is further processed and released by inflammasomes that are triggered in a SYK- and reactive oxygen species (ROS)-dependent manner. Pro-IL-18 is similarly processed and released by the inflammasome.
One major effector pathway of the CBM complex is the canonical IκB kinase (IKK)-mediated NF-κB pathway, which controls the expression of a variety of immune-regulatory factors. The NF-κB family consists of several subunits, of which p65, c-Rel, and RelB are transcriptionally active and form homodimers and heterodimers with other NF-κB proteins to initiate gene expression (19). To mediate NF-κB signaling, CARD9 recruits the ubiquitin ligase TRIM62, which binds to the C-terminal tail of CARD9 and mediates K27-linked ubiquitination of CARD9 on K125 in the coiled-coil domain (20) (Figure 1A). This modification is dispensable for the formation of the CBM complex but critical for the downstream activation of NF-κB (20). The CBM complex activates NF-κB dimers containing the subunits p65 or c-Rel (18), while MALT1 also functions as a protease that can inactivate the subunit RelB (21) to control distinct NF-κB subprograms. RelB is a subunit that is primarily activated by the noncanonical NF-κB pathway, and it can bind to the IL5 promoter to activate IL5 transcription in myeloid cells (22). Factors regulated by the canonical NF-κB pathway include pro-inflammatory cytokines, including TNF, IL-6 and the pro-inflammatory cytokine precursor pro-IL-1β, which is processed by inflammasomes into mature IL-1β. Inflammasomes are activated in response to fungal recognition in a SYK- and reactive oxygen species-dependent manner (23), and they can process additional cytokines of the IL-1 family, e.g., IL-18.
CARD9 signaling can also be activated by intracellular PRRs, such as the nucleotide-binding oligomerization domain-containing protein 2 (NOD2) (11), which is encoded by another important IBD risk gene, NOD2 (4). In this pathway, NF-κB is activated independently of CARD9 by the interaction of NOD2 with RIPK2, whereas the interaction of NOD2 with CARD9 promotes the activation of the MAP kinases p38 and JNK, supporting pro-inflammatory cytokine production in response to stimulation with the bacterial cell wall component peptidoglycan or Listeria monocytogenes infection (11). Moreover, CARD9 is activated in response to the ligation of the nucleic acid receptors RIG-I (24) and RAD50 (25) after recognition of bacterial or viral nucleic acids, which also trigger the activation of the NF-κB pathway and the subsequent production of pro-inflammatory cytokines.
These CARD9-mediated signaling pathways are critical for host defense, particularly for protection against fungi, as CARD9-deficient mice and humans with CARD9 loss-of-function mutations exhibit severe susceptibility to fungal infections caused by species such as Candida albicans (26) or dermatophytes (27), with invasive fungal growth into tissues (28). These protective functions are in part mediated by the critical role of CARD9 in inducing adaptive TH17 cell responses (26, 29), which exert defense mechanisms against fungi and other extracellular pathogens. Moreover, during fungal infection, CARD9 controls the induction of neutrophilic myeloid-derived suppressor cells (MDSCs) (30), which prevent damaging hyperinflammation by counterbalancing T and NK cell responses.
CARD9 Genetic Variants in Intestinal Inflammation
While a complete loss of CARD9 results in immunodeficiency, several single-nucleotide polymorphisms (SNPs) in the human CARD9 gene are associated with inflammatory diseases, particularly with IBD (rs10781499 (4), rs10870077 (5), and rs4077515 (6, 7)). Non-coding or synonymous variants presumably influence the level of CARD9 expression (31, 32) and thereby modulate the strength of CARD9 signaling. In addition, the IBD-associated SNP rs4077515 encodes a missense CARD9 variant that carries an asparagine instead of a serine residue in the CARD domain in position 12 (CARD9S12N) (Figure 1A). Experiments with knock-in mice that carry the CARD9S12N-encoding allele have demonstrated that CARD9S12N leads to aberrant activation of RelB and subsequent excessive production of IL-5 by myeloid cells in response to A. fumigatus, which skews T cell responses toward TH2 differentiation (22). These findings indicate that CARD9S12N deviates physiological CARD9 signaling and suggest that the respective SNP in human IBD could potentially contribute to intestinal inflammation through related mechanisms, although this possibility remains to be investigated.
While the above-mentioned CARD9 risk SNPs are relatively frequent and only moderately increase IBD risk (odds ratio (OR) ≈ 1.2), a rare splice site variant of CARD9 confers strong protection from IBD (OR ≈ 0.3–0.4) (8, 33). This specific variant, IVS11+1C>G, results in the skipping of exon 11, which leads to a CARD9 protein with a shortened C-terminal tail (CARD9Δ11) (Figure 1A). CARD9Δ11 is unable to bind TRIM62 and consequently fails to induce pro-inflammatory cytokines in myeloid cells after stimulation with fungal particles (20), which is thought to be responsible for the protective activity of CARD9Δ11 during IBD pathogenesis.
CARD9 Signaling in Murine Models of Intestinal Inflammation
Based on the association of CARD9 SNPs with IBD susceptibility, several research groups have started to investigate the role of CARD9 in murine models of experimental colitis (34, 35). In these studies, colitis was triggered by the administration of the chemical compound dextran sodium sulfate (DSS) via the drinking water (34, 35) or by oral inoculation with Citrobacter rodentium (34, 36). Both regimens damage the colonic epithelium and enable the entry of intestinal contents including bacteria and fungi into submucosal layers, triggering intestinal inflammation that leads to weight loss in the animals, followed by a recovery phase after DSS discontinuance in the DSS model (37, 38) or upon bacterial clearance in the C. rodentium model (34, 36).
CARD9-deficient mice exhibited a defect in epithelial regeneration and a significant delay in weight gain during the recovery phase after acute colitis (34, 35). The deficiency of CARD9 affects primarily myeloid cells and cytokines produced by these cells, which secondarily act on other cell types in the intestinal environment. Based on this interplay, CARD9 deficiency results in reduced production of pro-inflammatory cytokines (IL-6, TNFα, and IFNγ) and TH17- and innate lymphoid cell (ILC)-related cytokines (IL-17A, IL-17F, and importantly IL-22) by leukocytes in the lamina propria and mesenteric lymph nodes (34, 35). This phenotype is in part caused by defective production of the chemokine CCL20, which is required to recruit TH17 cells and ILCs to sites of epithelial damage (34), and by the failure of CARD9-deficient myeloid cells to secrete optimal amounts of IL-1β, which is necessary to stimulate IL-22 production by ILCs (Figure 2) (35). IL-22 is a key cytokine that promotes recovery from DSS-induced or C. rodentium-induced inflammation. IL-22 binds to its receptor on intestinal epithelial cells (IECs), which drives their regenerative proliferation (39) and stimulates the expression of antimicrobial peptides for innate defense against intruding microbes. As a consequence of impaired IL-22 and IL-17 signals, the IECs in CARD9-deficient mice do not receive the proper pro-proliferative signals for recovery (35), and they fail to produce sufficient amounts of antimicrobial peptides (34). Apart from controlling gut microbes through the epithelial compartment, CARD9-expressing myeloid cells also provide signals that support humoral responses to C. rodentium (36), although the exact mechanisms of this interaction remain to be investigated.
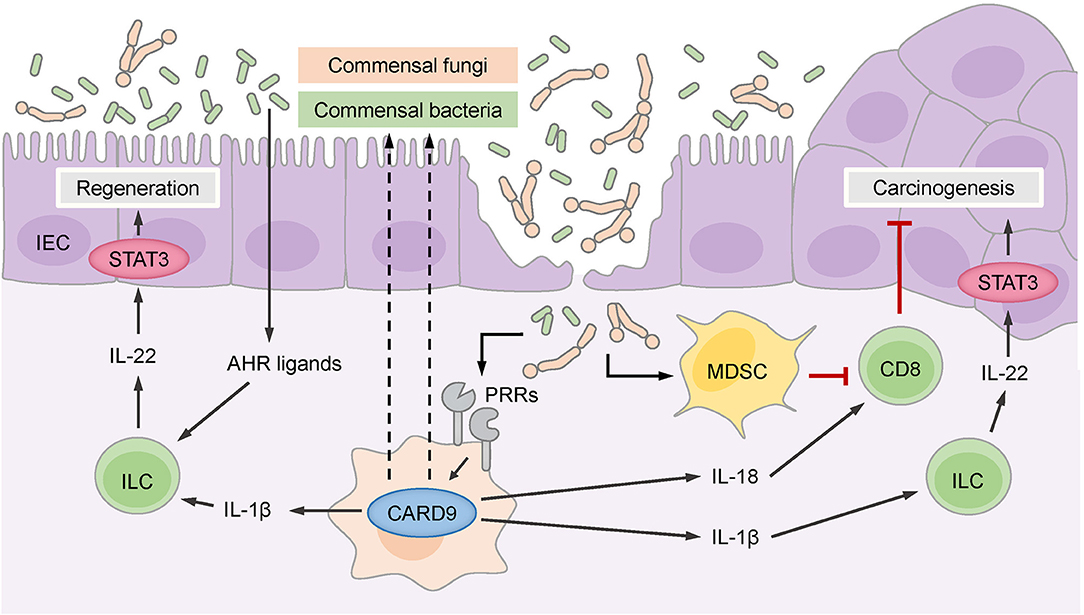
Figure 2. CARD9 controls the intestinal microbiome, which consists of commensal bacteria and fungi. Commensal gut bacteria supported by CARD9 provide aryl hydrocarbon receptor (AHR) ligands for the stimulation of innate lymphoid cells (ILCs) that secrete IL-22 to promote the regeneration of intestinal epithelial cells (IECs) through signal transducer and activator of transcription 3 (STAT3). ILCs are also stimulated by IL-1β, which is produced in a CARD9-dependent manner. CARD9 activated by pattern recognition receptors (PRRs) regulates commensal gut fungi that enter the damaged epithelium and promotes the accumulation of myeloid-derived suppressor cells (MDSCs). MDSCs inhibit CD8+ cytotoxic T cells that keep malignant IECs in check and thereby prevent carcinogenesis. CD8+ T cells are also stimulated by IL-18, which is secreted in response to commensal fungi in a CARD9-dependent manner. The CARD9-dependent IL-1β-IL-22 axis can support carcinogenesis through the stimulation of STAT3 in malignant IECs.
Additional work demonstrated that CARD9 also shapes the composition of the gut microbiome and affects both fungal and bacterial communities (40). Under steady-state conditions, the fungal burden is increased in CARD9-deficient mice, and during colitis, the fungal microbiome changes more substantially in CARD9-deficient mice than in wild-type mice. Similarly, at baseline, the composition of the bacterial microbiome is disturbed in CARD9-deficient mice and exhibits decreased stability during DSS colitis. Underrepresented bacteria in CARD9-deficient mice include, for example, species from the Adlercreutzia genus and Lactobacillus reuteri. Under steady-state conditions, this dysbiotic microbiota of CARD9-deficient mice exhibits a striking failure to metabolize tryptophan into ligands of the aryl hydrocarbon receptor (AHR) transcription factor (Figure 2), which is crucial for the expression of IL-22 by TH17 cells (39). This failure to produce AHR ligands has also been linked to impaired IL-22 production and delayed epithelial restitution in CARD9-deficient mice (40). Together, these experimental studies revealed that CARD9 signaling within the myeloid compartment affects epithelial regeneration during the recovery from colitis by triggering ILC and TH17 responses, in part by directing the microbiota toward AHR ligand-producing species. These pathways are presumably also important for the pathogenesis of IBD in patients with CARD9 risk variants, as a deficiency in AHR ligands is observed in the microbiota of IBD patients with the CARD9 risk SNP rs10781499 (40).
CARD9 in Immune Environments in Colorectal Cancer
The inflammatory pathways that stimulate the regenerative expansion of normal IECs are also frequent drivers of malignant proliferation and survival in transformed epithelial cells during colorectal cancer pathogenesis (3). However, in addition to providing pro-inflammatory, tumor-promoting effects, immune cells also play a protective role in colorectal cancer, which is largely executed via the antitumor activity of cytotoxic CD8+ T cells, whose presence within the tumor tissue is one of the strongest prognostic markers for a positive clinical outcome in colorectal cancer patients (41). Recent studies revealed that CARD9 signaling can contribute to both tumor-promoting and tumor-suppressive immunity in the intestine, and these distinct effects are strongly influenced by the composition of the microbiota (35, 42, 43).
To explore the role of CARD9 in colitis-associated cancer (CAC) independent groups used CARD9-deficient mice in the AOM/DSS model (35, 42–44). In this model, the administration of the carcinogen azoxymethane (AOM) initiates mutations in IECs, and subsequent cycles of DSS administration trigger inflammation that promotes epithelial dysplasia through a variety of immune cell-derived mediators, growth factors and cytokines. In wild-type mice, this treatment protocol provokes the formation of multiple polyploid lesions in the colon and rectum, with histological scores from low to high grade, but invasive dysplasia rarely occurs in this context.
To minimize the effects of the microbiota on tumor development, Bergmann et al. co-housed CARD9-deficient mice and littermate controls from birth on and during the AOM/DSS treatment protocol (35). Under these conditions, CARD9-deficient mice displayed smaller polyps in the distal colon than wild-type mice, although the total number of polyps did not differ between CARD9-deficient and wild-type mice. Moreover, the rate of tumor cell proliferation was decreased in CARD9-deficient mice, and the tumor cell-intrinsic activation of STAT3 was substantially reduced. STAT3 is a key transcription factor that drives the proliferation and survival of malignant cells during CAC pathogenesis (45) and is activated by several cytokines and growth factors, including IL-22 (39). These experiments indicate that CARD9 signaling in the immune environment of developing colorectal cancers can trigger the tumor-promoting STAT3 pathway within tumor cells, presumably via ILC-mediated IL-22 production, comparable to its role during epithelial regeneration after acute colitis (34, 35).
Two subsequent studies single-housed CARD9-deficient and wild-type animals to dissect the microbiota-dependent influences on CAC development using the AOM/DSS model (42, 43). Under these conditions, CARD9-deficient mice develop more and larger tumors than wild-type mice, and these effects were attributed to an aberrant fungal microbiome and secondary effects of fungi on antitumor T cells (42, 43). Wang et al. found that several fungal species, particularly Candida tropicalis, were enriched in the intestinal flora of CARD9-deficient mice (43) and interestingly also in the feces of colorectal cancer patients (43). Moreover, monocolonizing germ-free mice with C. tropicalis, but not with the irrelevant fungal species Saccharomycopsis fibuligera, increased their susceptibility to CAC in the AOM/DSS model, indicating that this species can support cancer development (43). Mechanistically, Wang et al. proposed that specific fungi promote the accumulation and activation of granulocytic MDSCs in the colonic lamina propria, which suppress the proliferation and effector functions of T cells and thereby negatively interfere with T cell-mediated antitumor immunity and enable enhanced colorectal cancer growth (Figure 2). Since the fungal load in the feces of colorectal cancer patients positively correlates with MDSC frequencies in the tumor, this pathway could also play an important role in clinical settings (43).
Similarly, Malik et al. observed an increased tumor burden in CARD9-deficient mice using the AOM/DSS model under single-housing conditions (42), but they propose an additional mechanism. While this group also demonstrates that CARD9 signaling shapes the composition of fungal communities and T cell immunity in the colon, they show that CARD9 controls the IL-1 family cytokine IL-18 through a canonical SYK-dependent pathway in myeloid cells in the early phase of the AOM/DSS treatment protocol (42) (Figure 2). IL-18 can maintain intestinal epithelial integrity during inflammation and stimulate IFNγ and FASL expression by T cells (42, 46), supporting antitumor immunity during CAC development. Consistent with this model, supplementation with exogenous IL-18 restored T cell function in CARD9-deficient mice during CAC, ameliorated the exacerbated intestinal inflammation and reduced tumor development (42).
Together, these two studies reveal previously unknown functions of CARD9 in the control of fungal communities that initiate MDSCs and stimulate IL-18 secretion, both of which regulate the function of antitumor CD8+ T cells during CAC development (Figure 2). Nevertheless, it remains unclear to what extent the overall fungal load determines CAC outcome, as the pharmacological depletion of fungi can on one hand reduce MDSC frequencies and thus enhance antitumor T cell responses in CARD9-deficient mice (43) but on the other hand result in a lack of IL-18 signals for stimulation of antitumor T cells (42).
Conclusion and Perspective
The human CARD9 locus has been a known IBD susceptibility factor for more than 10 years, and during that time, the basic mechanisms of CARD9 signaling have been elucidated (9). In IBD, both risk-promoting and protective CARD9 variants have been identified, and based on an understanding of the protective CARD9 variant, which is defective in the CARD9-TRIM62 interaction, small-molecule inhibitors that block the CARD9-TRIM62 interaction have been developed that might be useful for IBD treatment (47). Additional pathomechanisms of CARD9 signaling during IBD are alterations in regenerative IL-22 signaling via the effects of CARD9-expressing myeloid cells on ILCs (34, 35) or via the effects of CARD9 on the control of the microbiota (40, 42, 43), which could also provide new strategies for IBD treatment. The key role of CARD9 in intestinal immune homeostasis is also underscored by the influence of CARD9 signaling on colorectal cancer development. Here, depending on the composition of the microbiota, particularly the fungal communities, CARD9 can play a pro-tumorigenic role by triggering inflammation-induced oncogenic STAT3 activation within malignant cells or a tumor-suppressive function by promoting antitumor T cell responses through various mechanisms. How the balance between these CARD9-mediated pro- and antitumor immune functions is precisely tuned requires further investigation. Such studies are expected to provide further insights into the complex interplay among the immune system, the microbiota, tissue homeostasis and cancer development, as well as potential rational targets for therapeutic interventions.
Author Contributions
All authors listed have made a substantial, direct and intellectual contribution to the work, and approved it for publication.
Funding
Work in the authors' laboratory is supported by research grants from the Deutsche Forschungsgemeinschaft (SFB 1054/B01, SFB 1335/P01 and P08, TRR 237/A10, and RU 695/9-1) and the European Research Council (FP7, Grant Agreement No. 322865) to JR and the international doctoral programme i-Target: Immunotargeting of Cancer, funded by the Elite Network of Bavaria.
Conflict of Interest Statement
The authors declare that the research was conducted in the absence of any commercial or financial relationships that could be construed as a potential conflict of interest.
References
1. Thaiss CA, Zmora N, Levy M, Elinav E. The microbiome and innate immunity. Nature. (2016) 535:65–74. doi: 10.1038/nature18847
2. Ananthakrishnan AN, Bernstein CN, Iliopoulos D, Macpherson A, Neurath MF, Ali RAR, et al. Environmental triggers in IBD: a review of progress and evidence. Nat Rev Gastroenterol Hepatol. (2017) 15:39–49. doi: 10.1038/nrgastro.2017.136
3. Terzic J, Grivennikov S, Karin E, Karin M. Inflammation and colon cancer. Gastroenterology. (2010) 138:2101–14.e2105. doi: 10.1053/j.gastro.2010.01.058
4. Jostins L, Ripke S, Weersma RK, Duerr RH, McGovern DP, Hui KY, et al. Host-microbe interactions have shaped the genetic architecture of inflammatory bowel disease. Nature. (2012) 491:119–24. doi: 10.1038/nature11582
5. Zhernakova A, Festen EM, Franke L, Trynka G, van Diemen CC, Monsuur AJ, et al. Genetic analysis of innate immunity in Crohn's disease and ulcerative colitis identifies two susceptibility loci harboring CARD9 and IL18RAP. Am J Hum Genet. (2008) 82:1202–10. doi: 10.1016/j.ajhg.2008.03.016
6. Franke A, McGovern DP, Barrett JC, Wang K, Radford-Smith GL, Ahmad T, et al. Meta-analysis increases to 71 the tally of confirmed Crohn's disease susceptibility loci. Nat Genet. (2010) 42:1118–25. doi: 10.1038/ng.717
7. McGovern DP, Gardet A, Törkvist L, Goyette P, Essers J, Taylor KD, et al. Genome-wide association identifies multiple ulcerative colitis susceptibility loci. Nat Genet. (2010) 42:332–7. doi: 10.1038/ng.549
8. Rivas MA, Beaudoin M, Gardet A, Stevens C, Sharma Y, Zhang CK, et al. Deep resequencing of GWAS loci identifies independent rare variants associated with inflammatory bowel disease. Nat Genet. (2011) 43:1066–73. doi: 10.1038/ng.952
9. Ruland J, Hartjes L. CARD–BCL-10–MALT1 signalling in protective and pathological immunity. Nat Rev Immunol. (2018) 19:118–34. doi: 10.1038/s41577-018-0087-2
10. Hara H, Ishihara C, Takeuchi A, Imanishi T, Xue L, Morris SW, et al. The adaptor protein CARD9 is essential for the activation of myeloid cells through ITAM-associated and Toll-like receptors. Nat Immunol. (2007) 8:619–29. doi: 10.1038/ni1466
11. Hsu YM, Zhang Y, You Y, Wang D, Li H, Duramad O, et al. The adaptor protein CARD9 is required for innate immune responses to intracellular pathogens. Nat Immunol. (2007) 8:198–205. doi: 10.1038/ni1426
12. Gross O, Gewies A, Finger K, Schäfer M, Sparwasser T, Peschel C, et al. Card9 controls a non-TLR signalling pathway for innate anti-fungal immunity. Nature. (2006) 442:651–6. doi: 10.1038/nature04926
13. Robinson MJ, Osorio F, Rosas M, Freitas RP, Schweighoffer E, Gross O, et al. Dectin-2 is a Syk-coupled pattern recognition receptor crucial for Th17 responses to fungal infection. J Exp Med. (2009) 206:2037–51. doi: 10.1084/jem.20082818
14. Dorhoi A, Desel C, Yeremeev V, Pradl L, Brinkmann V, Mollenkopf HJ, et al. The adaptor molecule CARD9 is essential for tuberculosis control. J Exp Med. (2010) 207:777–92. doi: 10.1084/jem.20090067
15. Shenderov K, Barber DL, Mayer-Barber KD, Gurcha SS, Jankovic D, Feng CG, et al. Cord factor and peptidoglycan recapitulate the Th17-promoting adjuvant activity of mycobacteria through mincle/CARD9 signaling and the inflammasome. J Immunol. (2013) 190:5722–30. doi: 10.4049/jimmunol.1203343
16. Brown GD, Willment JA, Whitehead L. C-type lectins in immunity and homeostasis. Nat Rev Immunol. (2018) 18:374–89. doi: 10.1038/s41577-018-0004-8
17. Strasser D, Neumann K, Bergmann H, Marakalala MJ, Guler R, Rojowska A, et al. Syk kinase-coupled C-type lectin receptors engage protein kinase C-sigma to elicit Card9 adaptor-mediated innate immunity. Immunity. (2012) 36:32–42. doi: 10.1016/j.immuni.2011.11.015
18. Roth S, Bergmann H, Jaeger M, Yeroslaviz A, Neumann K, Koenig PA, et al. Vav proteins are key regulators of card9 signaling for innate antifungal immunity. Cell Rep. (2016) 17:2572–83. doi: 10.1016/j.celrep.2016.11.018
19. Hayden MS, Ghosh S. NF-kappaB, the first quarter-century: remarkable progress and outstanding questions. Genes Dev. (2012) 26:203–34. doi: 10.1101/gad.183434.111
20. Cao Z, Conway KL, Heath RJ, Rush JS, Leshchiner ES, Ramirez-Ortiz ZG, et al. Ubiquitin ligase TRIM62 regulates CARD9-mediated anti-fungal immunity and intestinal inflammation. Immunity. (2015) 43:715–26. doi: 10.1016/j.immuni.2015.10.005
21. Hailfinger S, Nogai H, Pelzer C, Jaworski M, Cabalzar K, Charton JE, et al. Malt1-dependent RelB cleavage promotes canonical NF-kappaB activation in lymphocytes and lymphoma cell lines. Proc Natl Acad Sci USA. (2011) 108:14596–601. doi: 10.1073/pnas.1105020108
22. Xu X, Xu J-F, Zheng G, Lu H-W, Duan J-L, Rui W. CARD9(S12N) facilitates the production of IL-5 by alveolar macrophages for the induction of type 2 immune responses. Nat Immunol. (2018) 19:547–60. doi: 10.1038/s41590-018-0112-4
23. Gross O, Poeck H, Bscheider M, Dostert C, Hannesschläger N, Endres S, et al. Syk kinase signalling couples to the Nlrp3 inflammasome for anti-fungal host defence. Nature. (2009) 459:433–6. doi: 10.1038/nature07965
24. Poeck H, Bscheider M, Gross O, Finger K, Roth S, Rebsamen M, et al. Recognition of RNA virus by RIG-I results in activation of CARD9 and inflammasome signaling for interleukin 1 beta production. Nat Immunol. (2010) 11:63–9. doi: 10.1038/ni.1824
25. Roth S, Rottach A, Lotz-Havla AS, Laux V, Muschaweckh A, Gersting SW, et al. Rad50-CARD9 interactions link cytosolic DNA sensing to IL-1beta production. Nat Immunol. (2014) 15:538–45. doi: 10.1038/ni.2888
26. Glocker EO, Hennigs A, Nabavi M, Schäffer AA, Woellner C, Salzer U, et al. A homozygous CARD9 mutation in a family with susceptibility to fungal infections. N Engl J Med. (2009) 361:1727–35. doi: 10.1056/NEJMoa0810719
27. Lanternier F, Mahdaviani SA, Barbati E, Chaussade H, Koumar Y, Levy R, et al. Inherited CARD9 deficiency in otherwise healthy children and adults with Candida species-induced meningoencephalitis, colitis, or both. J Allergy Clin Immunol. (2015) 135:1558–68 e1552. doi: 10.1016/j.jaci.2014.12.1930
28. Lanternier F, Pathan S, Vincent QB, Liu L, Cypowyj S, Prando C, et al. Deep dermatophytosis and inherited CARD9 deficiency. N Engl J Med. (2013) 369:1704–14. doi: 10.1056/NEJMoa1208487
29. LeibundGut-Landmann S, Gross O, Robinson MJ, Osorio F, Slack EC, Tsoni SV, et al. Syk- and CARD9-dependent coupling of innate immunity to the induction of T helper cells that produce interleukin 17. Nat Immunol. (2007) 8:630–8. doi: 10.1038/ni1460
30. Rieber N, Singh A, Öz H, Carevic M, Bouzani M, Amich J, et al. Pathogenic fungi regulate immunity by inducing neutrophilic myeloid-derived suppressor cells. Cell Host Microbe. (2015) 17:507–14. doi: 10.1016/j.chom.2015.02.007
31. Pointon JJ, Harvey D, Karaderi T, Appleton LH, Farrar C, Stone MA, et al. Elucidating the chromosome 9 association with AS; CARD9 is a candidate gene. Genes Immun. (2010) 11:490–6. doi: 10.1038/gene.2010.17
32. Fairfax BP, Humburg P, Makino S, Naranbhai V, Wong D, Lau E, et al. Innate immune activity conditions the effect of regulatory variants upon monocyte gene expression. Science. (2014) 343:1246949. doi: 10.1126/science.1246949
33. Beaudoin M, Goyette P, Boucher G, Lo KS, Rivas MA, Stevens C, et al. Deep resequencing of GWAS loci identifies rare variants in CARD9, IL23R and RNF186 that are associated with ulcerative colitis. PLoS Genet. (2013) 9:e1003723. doi: 10.1371/journal.pgen.1003723
34. Sokol H, Conway KL, Zhang M, Choi M, Morin B, Cao Z, et al. Card9 mediates intestinal epithelial cell restitution, T-helper 17 responses, and control of bacterial infection in mice. Gastroenterology. (2013) 145:591–601 e593. doi: 10.1053/j.gastro.2013.05.047
35. Bergmann H, Roth S, Pechloff K, Kiss EA, Kuhn S, Heikenwälder M, et al. Card9-dependent IL-1β regulates IL-22 production from group 3 innate lymphoid cells and promotes colitis-associated cancer. Eur J Immunol. (2017) 47:1342–53. doi: 10.1002/eji.201646765
36. Lamas B, Michel ML, Waldschmitt N, Pham HP, Zacharioudaki V, Dupraz L, et al. Card9 mediates susceptibility to intestinal pathogens through microbiota modulation and control of bacterial virulence. Gut. (2018) 67:1836–44. doi: 10.1136/gutjnl-2017-314195
37. Mizoguchi A, Mizoguchi E. Animal models of IBD: linkage to human disease. Curr Opin Pharm. (2010) 10:578–87. doi: 10.1016/j.coph.2010.05.007
38. Collins JW, Keeney KM, Crepin VF, Rathinam VA, Fitzgerald KA, Finlay BB, et al. Citrobacter rodentium: infection, inflammation and the microbiota. Nat Rev Microbiol. (2014) 12:612–23. doi: 10.1038/nrmicro3315
39. Eyerich K, Dimartino V, Cavani A. IL-17 and IL-22 in immunity: driving protection and pathology. Eur J Immunol. (2017) 47:607–14. doi: 10.1002/eji.201646723
40. Lamas B, Richard ML, Leducq V, Pham HP, Michel ML, Da Costa G, et al. CARD9 impacts colitis by altering gut microbiota metabolism of tryptophan into aryl hydrocarbon receptor ligands. Nat Med. (2016) 22:598–605. doi: 10.1038/nm.4102
41. Fridman WH, Pagès F, Sautès-Fridman C, Galon J, Fridman WH, Pages F, Sautes-Fridman C, Galon J. The immune contexture in human tumours: impact on clinical outcome. Nat Rev Cancer. (2012) 12:298–306. doi: 10.1038/nrc3245
42. Malik A, Sharma D, Malireddi RKS, Guy CS, Chang TC, Olsen SR, et al. SYK-CARD9 signaling axis promotes gut fungi-mediated inflammasome activation to restrict colitis and colon cancer. Immunity. (2018) 49:515–30.e515. doi: 10.1016/j.immuni.2018.08.024
43. Wang T, Fan C, Yao A, Xu X, Zheng G, You Y, et al. The adaptor protein card9 protects against colon cancer by restricting mycobiota-mediated expansion of myeloid-derived suppressor cells. Immunity. (2018) 49:504–14.e504. doi: 10.1016/j.immuni.2018.08.018
44. Okayasu I, Ohkusa T, Kajiura K, Kanno J, Sakamoto S. Promotion of colorectal neoplasia in experimental murine ulcerative colitis. Gut. (1996) 39:87–92. doi: 10.1136/gut.39.1.87
45. Yu H, Pardoll D, Jove R. STATs in cancer inflammation and immunity: a leading role for STAT3. Nat Rev Cancer. (2009) 9:798–809. doi: 10.1038/nrc2734
46. Dinarello C, Novick D, Kim S, Kaplanski G. Interleukin-18 and IL-18 binding protein. (2013) 4:289. doi: 10.3389/fimmu.2013.00289
Keywords: CARD9, intestinal immune homeostasis, inflammatory bowel disease, intestinal inflammation, intestinal carcinogenesis, gut microbiome
Citation: Hartjes L and Ruland J (2019) CARD9 Signaling in Intestinal Immune Homeostasis and Oncogenesis. Front. Immunol. 10:419. doi: 10.3389/fimmu.2019.00419
Received: 04 December 2018; Accepted: 18 February 2019;
Published: 11 March 2019.
Edited by:
Frederic Bornancin, Novartis, SwitzerlandReviewed by:
Harry Sokol, Saint Antoine Hospital, APHP, FranceSuryasarathi Dasgupta, University of California, San Diego, United States
Copyright © 2019 Hartjes and Ruland. This is an open-access article distributed under the terms of the Creative Commons Attribution License (CC BY). The use, distribution or reproduction in other forums is permitted, provided the original author(s) and the copyright owner(s) are credited and that the original publication in this journal is cited, in accordance with accepted academic practice. No use, distribution or reproduction is permitted which does not comply with these terms.
*Correspondence: Jürgen Ruland, ai5ydWxhbmRAdHVtLmRl orcid.org/0000-0002-8381-3597