- 1Department of Biochemistry and Molecular Biology, College of Medicine, National Cheng Kung University, Tainan, Taiwan
- 2Kaohsiung Chang Gung Memorial Hospital, Institute for Translational Research in Biomedicine, Kaohsiung, Taiwan
- 3Institute of Microbiology & Immunology, College of Medicine, National Cheng Kung University, Tainan, Taiwan
- 4Department of Pediatrics, College of Medical, National Cheng Kung University, Tainan, Taiwan
Asthma is a chronic inflammatory disease of the airway. Its major symptoms are reversible breathing problems causing airway narrowing and obstruction. IL-19 is a member of the IL-10 family cytokines. We previously showed that IL-19 induces T-helper 2 (Th2) cytokines and that asthma patients had higher serum IL-19 levels. To further examine whether inhibiting IL-19 and its receptor (IL-20R1) protected rodents against asthma, we used Dermatophagoides pteronyssinus (Der p; house dust mites) to induce chronic airway inflammation in wild-type C57BL/6 and IL-20R1-deficient mice and then analyzed the effect of the IL-20R1 deficiency on the pathogenesis of asthma. We also examined whether inhibiting IL-19 and IL-20R1 ameliorated Der p-induced chronic asthma. Der p induced IL-19 in lung airway epithelial cells, type 2 alveolar cells, and alveolar macrophages. An IL-20R1 deficiency abolished IL-19-induced Th2 cell differentiation in vitro. Th2 cytokine expression, immune cell infiltration in the bronchoalveolar lavage, airway hyperresponsiveness (AHR), and bronchial wall thickening were lower in Der p-challenged IL-20R1-deficient mice. Anti-IL-20R1 monoclonal antibody (mAb) 51D and IL-19 polyclonal antibody (pAb) both ameliorated Der p-induced AHR, lung immune cell infiltration, bronchial wall thickening, and Th2 cytokine expression. Moreover, we confirmed that anti-IL-19 mAb (1BB1) attenuated lung inflammation in a rat ovalbumin-induced asthma model. This is the first report to show that inhibition of IL-19 by targeting IL-19 or IL-20R1 protected rodents from allergic lung inflammation. Our study suggests that targeting IL-19 signaling might be a novel therapeutic strategy for treating allergic asthma.
Introduction
Asthma is a chronic inflammatory disease of the airways characterized primarily by T-helper 2 (Th2) lymphocyte-mediated immune responses and associated with bronchial hyperresponsiveness, airflow obstruction, and airway remodeling (1). The global incidence of asthma is increasing, and it leads to significant use of healthcare resources (2). The protease activity of two major group-1 allergens (Dermatophagoides pteronyssinus [Der p] 1 and Dermatophagoides Farinae [Der f ] 1) from house dust mites (HDMs), two of the most important allergens worldwide, causes barrier dysfunction, induces the production of proinflammatory cytokines in epithelial cells, and induces Th2 responses (3–5).
The importance of type 2 immune responses, characterized by the production of interleukin-4 (IL-4), IL-5, and IL-13, has received much attention in the pathogenesis of asthma (6, 7). IgE was the first successful biological target used in patients with allergic disease and asthma (8). Recently, therapies targeting IL-4, IL-5, and IL-13 have shown potential efficacy for treating asthma (9). Although the majority of asthma patients benefit from current commercial therapies to control the symptoms, some patients do not respond well to these therapies (9). Thus, new asthma therapies that can inhibit not only airway hyper-responsiveness (AHR), but also mucus hyper-secretion and variable airflow obstruction, are needed.
IL-19 is a member of the IL-10 family, which includes IL-10, IL-19, IL-20, IL-22, melanoma differentiation-associated gene (MDA)-7 (IL-24), and AK155 (IL-26) (10, 11). IL-19 binds to IL-20 receptor (R)1/IL-20R2, a heterodimer complex mediating its signal transduction, and an activator of transcription (STAT)3 (12). IL-19 is produced primarily by monocytes, in which lipopolysaccharide (LPS) and granulocyte macrophage colony-stimulating factor (GM-CSF) upregulate IL-19 expression (13). Treating monocytes with IL-19 stimulates IL-6 and tumor necrosis factor (TNF)-α expression and induces monocyte apoptosis and the production of reactive oxygen species (ROS) (14). IL-19 is involved in inflammatory diseases such as rheumatoid arthritis (15), kidney injury (16), psoriasis (17), and breast cancer (18), and induces angiogenesis in endothelial cells (19). Acutely induced IL-19 in systemic inflammation promotes neutrophil chemotaxis and causes lung injury in mice undergoing endotoxin shock (20).These together suggest the potential roles of IL-19 as a tissue-derived inflammatory mediator.
We previously reported higher IL-19 expression in asthma patients and that patients with high IL-19 expression also have high IL-4 and IL-13 expression (21). We also found that IL-19 upregulated IL-13 and IgE production in asthmatic mice and that IL-19 induced Th2 cytokines in vitro, which suggested that IL-19 is substantially involved in allergic asthma (21). However, little is known about the molecular mechanism of IL-19 signaling in the pathogenesis of asthma. In this study, we investigated the role of IL-19 in asthmatic animal models in order to determine the therapeutic potential of IL-19-signaling antagonists for treating allergic asthma.
Materials and Methods
Animals
All animal experiments were done using the protocols of the National Institutes of Health standards and guidelines for the care and use of experimental animals. The research procedures were approved by the Animal Ethics Committee of National Cheng Kung University (IACUC NO. 107272 and 105077).
Generating IL-20R1 Knockout Mice
IL-20R1 knockout(−/−) mice were generated and maintained on a C57BL/6 genetic background, as previously described (22). Female wild-type (WT) C57BL/6 and IL-20R1−/− mice were used for the Der p-induced-asthma model and for the in vitro Th2 differentiation experiments.
Der p-Induced Asthmatic Mouse Model
The allergen Der p (1 g of lyophilized whole body extract in diethyl ether [Allergon, Engelholm, Sweden]) was dissolved in pyrogen-free isotonic saline, filtered with a 0.22-μm filter, and stored at −80°C before it was used (23). The LPS concentration of the Der p preparations was <0.96 endotoxin U/mg/Der p (E-Toxate [Limulus amebocyte lysate] test kit; Sigma-Aldrich, St. Louis, MO, USA). Groups of specific pathogen-free, 6–8 week-old C57BL/6 female mice (Laboratory Animal Center, National Cheng Kung University, Tainan, Taiwan) were intranasally (i.n.) inoculated with Der p (10 μl: 2.5 mg/ml) for 10 days. Control mice were inoculated with saline instead of with Der p. Seventy-two hours after the last Der p inoculation, the mice were killed, and blood samples were collected. Lung tissue was removed from control mice and asthmatic mice, and bronchoalveolar lavage fluid (BALF) was isolated and analyzed for immune cell infiltration. For antibody neutralization experiments, control isotype mIgG (6 mg/kg), 51D (6 mg/kg), pre-immune rabbit IgG, or IL-19 pAb were given 1 h after Der p treatment on day 0, 2, 4, 6, 8, and 10.
Measuring Airway Resistance and Hyperresponsiveness
Mice were anesthetized, steel cannulae were inserted into their tracheas, and then they were individually placed in a chamber to measure, using the Buxco FinePointe system [Data Sciences International (DSI), St. Paul, MN, USA], their lung resistance (RL) while they were exposed to increasing doses of acetyl-β-methylcholine chloride (methacholine; Sigma-Aldrich, St. Louis, MO, USA). Dynamic airway resistance (Penh value) was noninvasively measured using unrestrained whole body plethysmography (Buxco Electronics, Wilmington, NC, USA) while they were exposed to increasing aerosol concentrations of methacholine.
Histology and Immunohistochemistry
Lung tissues were embedded in paraffin, cut into 4 μm sections, and stained with hematoxylin and eosin (H&E). Inflammatory cell infiltration and lung architecture were assessed by light microscopy. The mucus secretion level was detected by periodic acid-Schiff (PAS; Sigma-Aldrich) staining. Lung sections were deparaffinized, hydrated in water, and then stained with periodic acid for 5 min. For immunohistochemistry, samples were paraffin embedded, sectioned, and stained by standard immunohistochemistry methods as previously described (24, 25). An additional antigen retrieval step was applied by heating samples in a Tris-based buffer (pH 9.0) to 95°C for 20 min. After blocking in 5% bovine serum albumin for 30 min at room temperature, tissues were incubated overnight at 4°C with primary antibodies against IL-19 (1:100; ab14106, Abcam), IL-20R1 (1:100; ab203196, Abcam), IL-20R2 (1:100; ab95824, Abcam), and SPC (1:100; ab211326, Abcam). The immune-reactivity of positive staining was developed by using the AEC chromogen kit (Romulin AEC Chromogen Kit; Biocare Medical, Walnut Creek, CA) and counterstained with Mayer's hematoxylin (J. T. Baker, Phillipsburg, NJ). Images were captured using an immunofluorescence microscope (Olympus BX51, Tokyo, Japan) or scanned using 3D Histech Pannoramic MIDI (3DHISTECH).
Lung Histopathology
The degree of lung inflammation was determined as described previously (26–28). Serial sections (4 μm) were stained with hematoxylin and eosin (H&E), Alcian blue/periodic acid-Schiff, and Picro Sirius Red (Abcam). Three to four randomly selected H&E-stained lung tissue sections per group were analyzed per sample for quantification of lung inflammation scores. The tissue inflammation score was evaluated by two independent pathologists who were blinded to the sample codes as previously described (28): 0, no inflammatory cell infiltration; 1, little inflammatory cell infiltration; 2, 1 layer of inflammatory cells around the airway; 3, 2–4 layers of inflammatory cells around the airway; 4, 4 or more layers of inflammatory cells around the airway. For quantification of bronchial wall thickness, the thicknesses of airway walls from 5 to 10 random airways per slide were measured by using Olympus Viewer 3 image software (Olympus, Tokyo, Japan). For quantification of airway fibrosis, the percentage of Sirius Red-stained area in 5–10 random airways per slide (high power field) was measured by using ImageJ v1.48 (NIH, USA).
Generating Anti-mIL-19 Polyclonal Antibody
Mouse (m) IL-19 polyclonal antibody (pAb) was generated using the standard procedure to immunize rabbits with mIL-19 recombinant protein. After the rabbits had been immunized, serum samples were collected and anti-IL-19 pAb was purified using IL-19-bound affinity chromatography. Antibodies purified from rabbit pre-immune serum using protein A chromatography (GE Healthcare, Illinois, USA) were used as control antibodies.
Antibody Preparation
Anti-IL-19 monoclonal antibody (mAb) (1BB1) was prepared as previously described (21). Anti-IL-20 receptor (IL-20R1) mAb (51D) was generated using standard protocols as previously described (29).
Analyzing IL-19, Stem Cell Factor, and Thymic Stromal Lymphopoietin Expression in Epithelial Cells
A549 and BEAS-2B cells (ATCC, Manassas, VA, USA) were plated for 12 h in Dulbecco's Modified Eagle's Medium (DMEM) with 10% fetal bovine serum. Epithelial cells were kept in serum-free DMEM for 8 h, and then incubated with Der p (500 ng/ml, Allergon, Engelholm, Sweden) or IL-19 (200 ng/ml) for 24 h. The culture media was collected, and stem cell factor (SCF), IL-19, and thymic stromal lymphopoietin (TSLP) protein levels were analyzed using enzyme-linked immunosorbent assay (ELISA). Mouse SCF and mouse TSLP ELISA kits were purchased from eBioscience, San Diego, USA. Mouse IL-19 protein was detected by homemade sandwich ELISA as previous described (21).
Detecting Cytokines in Serum, BALF, and Lung Tissue Lysates
Protein levels of IL-4 (50-1128931, eBioscience), IL-5 (88-7054-22, eBioscience), IL-9 (50-112-5217, eBioscience), IL-13 (88-7137, eBioscience), SCF, TSLP, IgE, and IgA (88-50450, Invitrogen) in serum, BALF, lung tissue lysates, and culture medium were detected using ELISA kits according to manufactures' instructions. For analysis of protein levels in lung tissues, 50 μg of tissue lysates were analyzed by using the following ELISA kits: IL-33 (DY3626, R&D Systems), IL-17 (DY421, R&D Systems), CCL11 (DY420, R&D Systems), IL-22 (DY582, R&D Systems), and Amphiregulin (DY989, R&D Systems).
In vitro Activity of IL-19 on CD4+ T Cells
Single-cell suspensions were prepared from splenocytes depleted of red blood cells (RBCs). CD4+ T cells were subsequently isolated to >97% purity with positive selection using anti-CD4 (L3T4) magnetic beads (Miltenyi Biotec, Auburn, CA, USA) (21). For in vitro differentiation assays, CD4+ T cells were incubated for 2 days with 1 μg/ml of plate-bound anti-CD3 and 1 μg/ml of plate-bound anti-CD28 mAbs with IL-2 (20 U/ml), IL-4 (10 ng/ml), anti-IL-12 mAb (1 μg/ml), and anti-interferon (IFN)-γ Ab (10 μg/ml) for Th2 differentiation. Three days after the primary incubation, the cells were washed by PBS and then incubated with or without IL-19 (200 ng/ml) added in the Th2 differentiation medium containing IL-2 (20 U/ml), IL-4 (10 ng/ml), anti-IL-12 mAb (1 μg/ml), and anti-interferon (IFN)-γ Ab (10 μg/ml) for 7 days. Fresh cytokines and Abs were added every 2 days. On day 7, cell culture supernatants were collected for ELISA of Th2 cytokine production (IL-5, IL-9, IL-13, and IL-4). All the cytokines and Abs used were purchased from PeproTech, Rocky Hill, NJ, USA. ELISA kits for human cytokines were purchased from eBiosciences.
Ovalbumin-Induced Asthmatic Rat Model
Ovalbumin (OVA) (Sigma-Aldrich) was prepared in pyrogen-free PBS and precipitated at a 1:1 ratio with Al(OH)3 (Sigma-Aldrich). Six 6- to 8-week-old female Sprague-Dawley rats (250–300 g) were sensitized with 2 mg of OVA (1 ml of OVA-Al(OH)3 suspension) given intraperitoneally on day 0, 7, and 14. Another 6 rats were given sham immunizations using PBS. Twenty-five days after the rats had been sensitized, for 5 days they were intranasally challenged daily with 1% OVA in 100 μl of PBS. Experimental rats (n = 6 per group) were given 1BB1 (10 mg/kg) and control rats were given isotype mouse immunogammaglobulin G1 (mIgG1) (10 mg/kg) 1 h before each intranasal OVA challenge. The rats were sacrificed on day 30 and the BALF and lung tissues were collected for histological analysis and mRNA preparation for qRT-PCR analysis.
Quantitative Real-Time Polymerase Chain Reaction
Total RNA was isolated using Trizol reagent (Invitrogen, Carlsbad, CA, USA) followed by reverse transcription to generate cDNA (PrimeScript RT-PCR kit; Clontech, Palo Alto, CA, USA). The expression of transcripts was analyzed using polymerase chain reaction (PCR) with gene-specific primers. Glyceraldehyde phosphate dehydrogenase (GAPDH) was used as an internal control. The following sequence-specific primers were used in real-time PCR: hIL-19, F: 5′-CGC GGA TCC ACT CAG GAG ATG TCT GAT TTC-3′, R: 5′-AGC TGA GAA CAT TAC TTC ATG A-3′; hIL-20R1, F: 5′-AAC GCT TCT ATC CTT TCT TGG A-3′, R: 5′-GAT GAC TTT AGC CTT CCA TGC-3′; hIL-20R2, F: 5′-GTG CAC CTA GAA ACC ATG GA-3′, R': CCA TCT TCC AGA CGG AGA G-3′; hHPRT, F: 5′-TGA CCA GTC AAC AGG GGA CA-3′, R: 5′-TGC CTG ACC AAG GAA AGC AA-3′; rat IL13, F: 5′-CCA CAG GAC CCA GAG GAT ATT GA-3′, R: 5′-TAG CGG AAA AGT TGC TTG GAG TAA-3′; rat IL19, F: 5′-GCC ATG CAA ACT AAG GAC ACC-3′, R: 5′-TTG GTC ATG CAG CAC ACA TC-3′; rat GAPDH: F: 5′-ACA TGC CGC CTG GAG AAA CCT-3′, R: 5'-TCC ACC ACC CTG TTG CTG TAG-3′. The expression levels were amplified using a thermal cycler (LightCycler 480; Roche Diagnostics, Indianapolis, IN, USA) with SYBR Green I (Roche, Basel, Switzerland) for quantitative analysis normalized with GAPDH as an input control. Relative changes in mRNA expression were determined by calculating 2−ΔΔCt.
Statistical Analysis
Prism 5.0 (GraphPad Software, San Diego, CA, USA) was used for all statistical analyses. The one-way analysis of variance (ANOVA) non-parametric test (Kruskal-Wallis test) or student t-test was used to compare the data between groups. Post hoc comparisons were done using Dunn's multiple comparison test. Results are mean ± standard deviation (SD). Significance was set at P < 0.05.
Results
IL-19 Expression Was Induced in Der p-Treated Lung Epithelial Cells
Bronchial epithelial cells are critical for the pathogenesis of asthma. HDM allergens cause bronchial constriction in asthma patients and induce an inflammatory response in the lungs because of the expression of cytokines, chemokines, and some inflammatory mediators (30, 31). We first confirmed whether bronchial epithelial cells express the transcripts of IL-19 and its receptor subunits, IL-20R1 and IL-20R2. Using RT-PCR, we found that human A549 lung epithelial cells and human BEAS-2B bronchial epithelial cells expressed IL-19 and its receptors subunits (Figure 1A). We next tested whether Der p induces IL-19 production in vitro. We co-cultured human A549 lung epithelial cells with Der p and collected the medium for ELISA analyses. IL-19 expression was induced in Der p-stimulated A549 cells (Figure 1B) in a time-dependent manner.
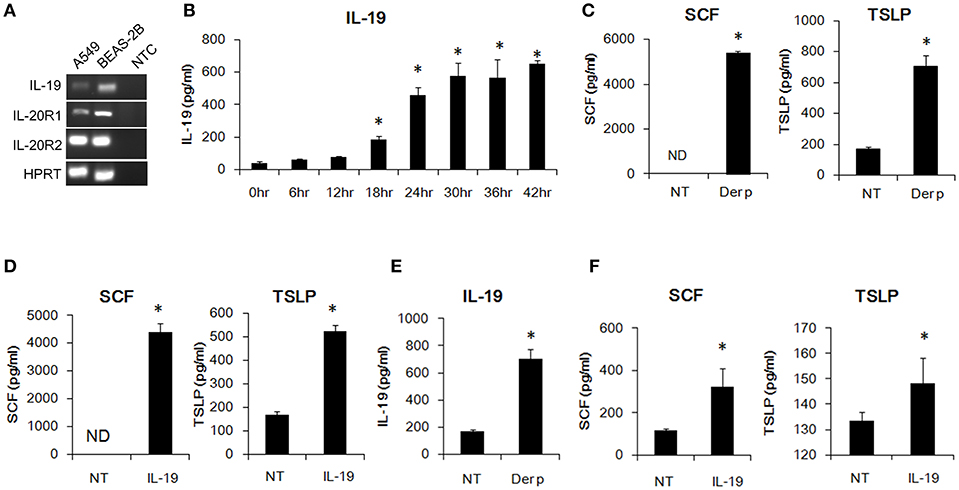
Figure 1. IL-19 was induced by Der p and upregulated SCF and TSLP production in lung epithelial cells in vitro. (A) RT-PCR analysis for the transcripts of IL-19, IL-20R1, and IL-20R2 in A549 and BEAS-2B cells. (B) IL-19 protein levels in the media of Der p (500 ng/ml)-treated A549 cells for different time courses. *P < 0.05 by one-way non-parametric ANOVA. (C) Stem cell factor (SCF) and thymic stromal lymphopoietin (TSLP) protein levels in the culture media of A549 cells treated with Der p (500 ng/ml) for 24 h. (D) A549 cells were treated with IL-19 (200 ng/ml) for 24 h and the culture media were collected for ELISA analysis of SCF and TSLP levels. (E) IL-19 levels in the culture media from BEAS-2B cells treated with Der p (500 ng/ml) for 24 h. (F) SCF and TSLP protein levels in the culture medium from BEAS-2B cells treated with IL-19 (200 ng/ml) for 24 h. NT, no template control; ND, not detectable. *P < 0.05 by t-test.
SCF and TSLP Protein Expression Was Higher in Der p-Treated Lung Epithelial Cells in vitro
In addition to IL-19, we also detected higher SCF and TSLP protein levels in Der p-treated A549 cells than in control cells (Figure 1C). Epithelial-derived SCF supports the development and survival of mucosal mast cells at the airway surface (32). Allergens are processed by myeloid dendritic cells, which are conditioned by the TSLP secreted by epithelial cells and mast cells to attract Th2 cells (33, 34). To test whether IL-19 alone activates lung epithelial cells and induces cytokine production, we treated the A549 cells with IL-19 and collected the media for ELISA analysis. SCF and TSLP protein was induced in IL-19-treated A549 cells (Figure 1D). Similarly, IL-19 was induced by Der p and upregulated SCF, and TSLP production in the BEAS-2B lung epithelial cells in vitro. (Figures 1E,F). These data together showed that Der p induced IL-19 production and that IL-19 activated lung epithelial cells to produce SCF and TSLP in vitro.
IL-20R1-Deficiency Attenuated Th2 Cell Differentiation in vitro
Der p induces proinflammatory cytokines in epithelial cells and induces Th2 responses (3–5). The importance of type 2 immune responses in the pathogenesis of asthma has received much attention (6, 7). We previously reported that IL-19 promoted Th2 polarization in vitro (21). We next hypothesized that IL-19 signaling through the IL-20R1/IL-20R2 receptor complex promotes Th2 differentiation from naïve T cells and induces Th2 cytokine production. To test this hypothesis, we isolated CD4+ naïve T cells from WT and IL-20R1−/− mice and differentiated the cells into Th2 phenotypes with and without IL-19 treatment (Figure 2). We collected and analyzed the culture media from the differentiated Th2 cells on day 7. IL-19 upregulated the production of IL-5 (Figure 2A), IL-9 (Figure 2B), and IL-13 (Figure 2C), but not IL-4 (Figure 2D), in differentiated Th2 cells from WT mice. IL-20R1 deficiency abolished IL-19-induced IL-5, IL-9, and IL-13 production in IL-19-treated CD4+ naïve T cells, which suggested that IL-20R1-mediated IL-19 signaling is crucial for IL-19-induced Th2 polarization in vitro.
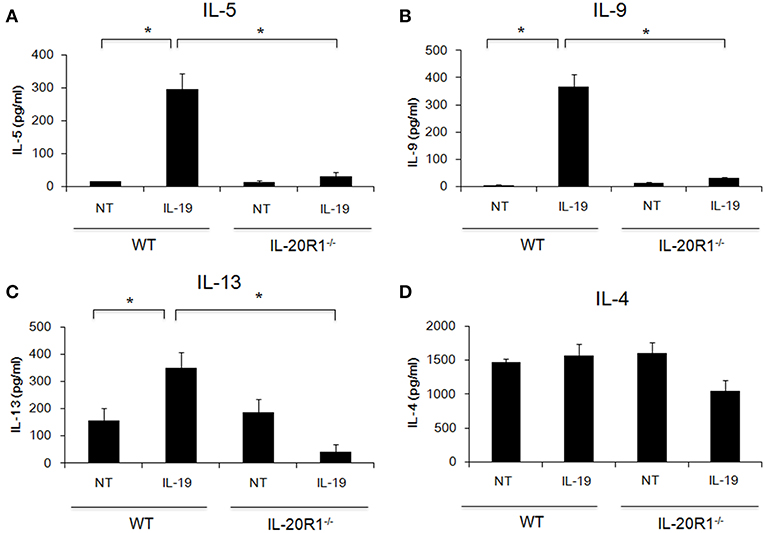
Figure 2. Deficiency of IL-20R1 attenuated IL-19-induced Th2 cell polarization in vitro. A negative selection of CD4+ T cells were isolated from the spleens of wild-type C57BL/6 (WT) and IL-20R1−/− mice and then incubated on a plate coated with anti-CD3 and anti-CD28 monoclonal antibodies (mAbs), and then the CD4+ T cells were incubated in Th2-differentiaion condition with PBS or IL-19 (200 ng/ml) for another 7 days as described in the methods section. Conditioned medium was collected on day 7, and (A) IL-5, (B) IL-9, (C) IL-13, and (D) IL-4 expression levels were analyzed using ELISA. The expression of T-helper 2 (Th2) cytokines on IL-19-treated CD4+ T cells was upregulated. Data are the average of duplicate samples. NT, not treated (PBS controls). *P < 0.05 by one-way non-parametric ANOVA.
IL-19 Expression Was Upregulated in Der p-Induced Allergic Asthmatic Mice
We next examined whether IL-19 is involved in the pathogenesis of HDM-induced allergic asthma. Chronic asthmatic mice were induced by repeated intranasal administration of Der p for 10 days (Figure 3A). The serum IL-19 and IgE levels were higher after 5 days of Der p treatment (Figure 3B). Serum IL-13, a well-known critical mediator in the pathogenesis of asthma (35), was upregulated after day 7 (Figure 3B). This result indicated that Der p-induced allergic lung inflammation associated with the elevation of IL-19, IL-13, and IgE serum levels, and that IL-19 was induced earlier (at day 5) than the upregulation of IL-13 after Der p challenges.
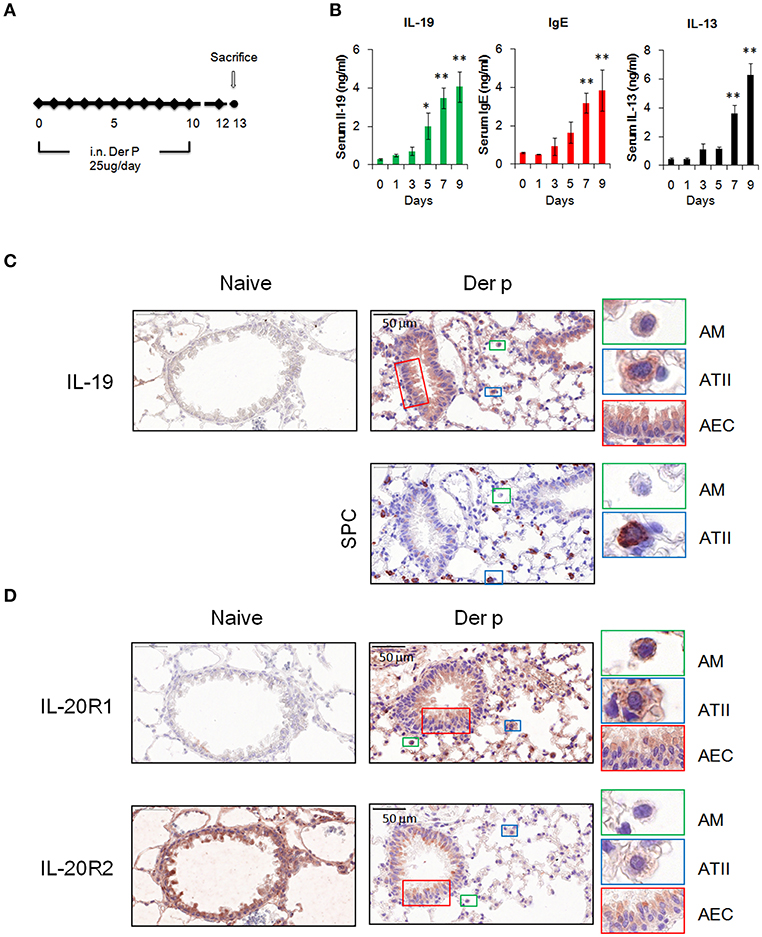
Figure 3. Expression of IL-19 and IL-19 receptors in lung tissues. (A) C57BL/6 mice were intranasally (i.n.) challenged with Der p extract (25 μg/mouse/day) for 10 days to induce asthma. (B) Dynamic serum levels of IL-19, IL-13, and immunoglobulin E (IgE) in Der p-sensitized mice (n = 5). *P < 0.05, **P < 0.01 compared with day 0 control by one-way non-parametric ANOVA. (C) Lung tissues were collected on day 13 and paraffin-sectioned for IHC staining for IL-19. Detection of the expression of surfactant protein C (SPC) in adjacent slide was used as marker for type II alveolar cells. (D) IHC staining for the expression of IL-20R1 and IL-20R2 with specific antibodies. Nuclei were counterstained with hematoxylin. Bars represented 50 μm. AM, alveolar macrophages; ATII, type II alveolar cells; AEC, airway epithelial cells.
Upregulation of IL-19 and IL-19 Receptors in Lung Tissues From Der p-Treated Mice
To further confirm the source and target of IL-19 in vivo, we performed immunohistochemical staining to detect the protein expression of IL-19, IL-20R1, and IL-20R2 with specific antibodies. IL-19 expression was not detected in naïve lung tissues (Figure 3C). In lung tissues from Der p challenged mice, IL-19 was detected in alveolar macrophages (AM) that were distributed the alveolar space and in surfactant protein C (SPC)-producing type II alveolar cells (ATII). IL-19 was also upregulated in the airway epithelial cells (AEC) (Figure 3D). The expression of IL-20R1 receptor subunit was upregulated in AM, ATII, and AEC following Der p challenge, and minimal to undetectable levels were found in normal lung tissues (Figure 3D). In contrast to the induction of IL-19 and IL-20R1 by Der p, basal level of IL-20R2 expression was detected in AM, ATII, and AEC in the lungs from healthy and Der p-treated mice (Figure 3D). Taken together, the results of IHC staining revealed that the lung epithelial cells (AEC and ATII) and AM could be the sources and target cells of IL-19 in the context of Der p-induced lung inflammation.
IL-20R1 Deficiency Protected the Mice Against Der p-Induced Chronic Asthma
IL-20R1 and IL-20R2 could form functional receptor complex for IL-19 (12). Since IL-19 and IL-20R1 were induced in the lung following Der p challenge, it was hypothesized that IL-19 signaling via IL-20R1 axis contributes to the progression of lung inflammation. To verify the role of IL-20R1-mediated IL-19 signaling in the development of allergic lung inflammation, we compared the pathological outcomes between WT and IL-20R1−/− mice in the Der p-induced chronic asthma model. The airway hyperresponsiveness (AHR) was lower in IL-20R1−/− mice than in WT mice, indicating improved lung function in IL-20R1−/− mice (Figure 4A). Histopathological analysis by using H&E, PAS, and Sirius Red staining were performed to evaluate the lung structure changes, pulmonary inflammation, and collagen deposition in the lung tissues (Figure 4B). The degree of lung inflammation (Figure 4C), bronchial wall thickening (Figure 4D), and airway fibrosis (Figure 4E) were lower in IL-20R1−/− mice than in WT mice (Figures 4B–E). In addition, IL-20R1−/− mice treated with Der p had fewer immune cells in their BALF than did WT mice (Figure 4F). The serum levels of IL-13 and IL-19 were lower in IL-20R1−/− mice than in WT mice (Figure 4G). The serum IgE level, however, was not altered by IL-20R1-deficiency in Der p-challenged mice (63.09 ± 4.85 ng/ml in WT vs. 65.07 ± 7.01 ng/ml in IL-20R1−/− mice, respectively) (Figure 4G). The IgA levels, which also associated with asthma onset (36), were lower in the BALF from IL-20R1−/− mice than from WT mice (Figure 4H). We analyzed the levels of pro-inflammatory cytokines associated with immune cell infiltration and type 2 immune responses and found that CCL11 (37), and IL-33 (38) levels were lower in lung tissues of IL-20R1−/− mice than in WT mice (Figure 4I). Levels of IL-13 and IL-4 were also slightly lower in IL-20R1−/− but were not statistically significantly different (Figure 4I). We also analyzed the protein levels of IL-5, IL-17, IL-22, and Amphiregulin, and we did not find significant differences on the expression of these proteins between groups (data not shown). These data suggested that IL-20R1 deficiency protected the mice from Der p-induced chronic asthma and reduced proinflammatory cytokines associated with immune cell infiltration.
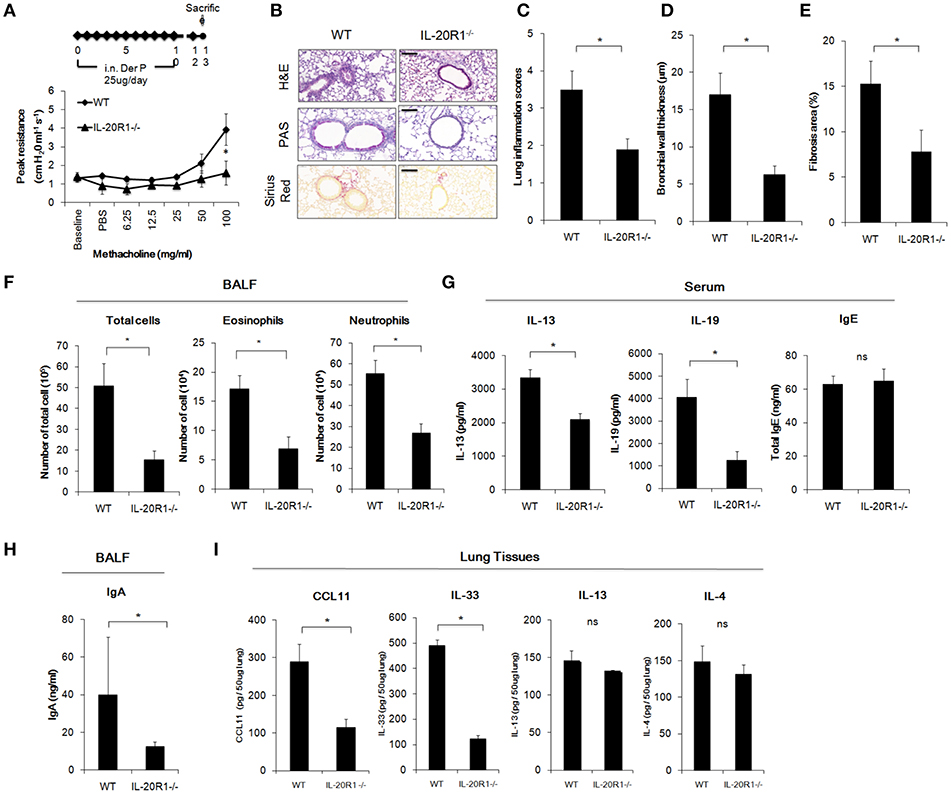
Figure 4. Der p-induced chronic asthma was attenuated in IL-20R1−/− mice. Wild-type C57BL/6 and IL-20R1−/− mice (n = 6 in each group) were intranasally (i.n.) challenged with Der p extract (25 μg/mouse/day) for 10 days to induce asthma. (A) Airway hyperresponsiveness (AHR) was analyzed on day 13. IL-20R1−/− mice had lower peak airway resistance with increasing doses of methacholine than did wild-type mice. (B) Histopathology assessments for lung inflammation, airway wall thickness by Hematoxylin and Eosin (H&E) and PAS staining. Degree of collagen deposition was evaluated by Sirius Red staining. Bars represented 50 μm. (C) Quantification of lung inflammation scores. (D) Quantification of bronchial wall thickness. (E) Quantification of Fibrosis area. (F) The number of infiltrated immune cells in mouse bronchoalveolar lavage fluid (BALF). (G) Serum IL-13, IL-19, and IgE levels. (H) BALF IgA levels. (I) Inflammatory cytokine levels (CCL11, IL-33, IL-13, and IL-4) in the lung tissues. *P < 0.05 by t-test. ns, not significant.
Anti-IL-20R1 mAb (51D) Reduced the Severity of Asthma in Asthmatic Mice Model
We next examined whether 51D, an IL-20R1 blocking mAb previously shown to inhibit liver fibrosis in mice model (29), would also protect Der p-treated mice from developing chronic asthma by preventing lung inflammation. In this model, 51D or control mIgG were given intraperitoneally (i.p.) on day 0, 2, 4, 6, and 8 (Figure 5A). The AHR of the mice was measured on day 13. The AHR of the 51D-treated mice was significantly lower compared with Der p-treated or Der p plus mIgG groups (p < 0.05) (Figure 5B). H&E and PAS staining showed reduced lung inflammation, bronchial wall thickening, and mucus-secreting goblet cell areas in 51D-treated mice (Figures 5C–E). There were also fewer infiltrated immune cells, eosinophils, and neutrophils in the BALF of 51D-treated mice than in the BALF of Control group mice (Figure 5F). ELISA analysis showed significantly lower serum IL-13, IL-19, and IgE levels, as well as lower BALF IgA levels in 51D-treated mice (Figures 5G,H). Tissue protein levels of CCL11 and IL-33 were also lower in 51D-treated group (Figure 5I). These data together demonstrated that IL-20R1 neutralizing mAb 51D ameliorated Der p-induced allergic lung inflammation in vivo.
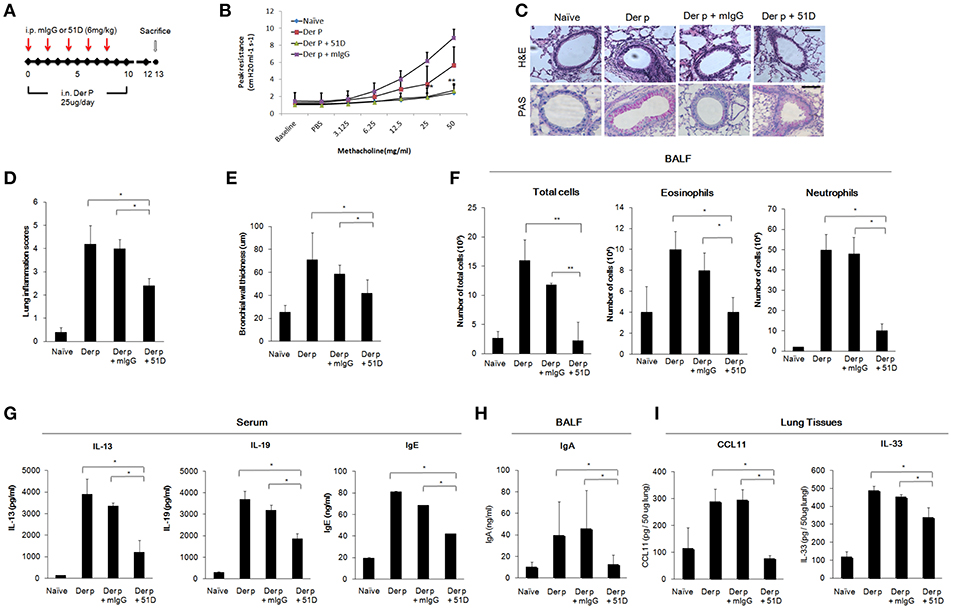
Figure 5. Anti-IL-20R1 mAb (51D) reduced airway inflammation in Der p-induced asthmatic mice. (A) Protocol of Der p-induced asthmatic mouse model with anti-IL-20R1 mAb (51D) treatment. C57BL/6 mice were intranasally (i.n.) challenged with Der p extract (25 μg/mouse/day) for 10 days to induce asthma. 51D (6 mg/kg) or control mIgG (6 mg/kg) was intraperitoneally injected into the Der p-sensitized mice on day 0, 2, 4, 6, and 8 (n = 6 per group). (B) AHR was analyzed in the mice 3 days after the last Der p treatment. Peak AHR was methacholine dose-dependently lower in 51D-treated mice. (C) Hematoxylin and eosin (H&E) staining showed less airway thickening and periodic acid-Schiff (PAS) staining showed fewer goblet cell areas in 51D-treated mice. (D) Lung inflammation scores of the mice. (E) Bronchial wall thickness. (F) Number of infiltrated immune cells, eosinophils, and neutrophils in mouse BALF. (G) Serum IL-13, IL-19, and IgE levels in the mice. (H) BALF IgA levels. (I) CCL11 and IL-33 levels in the lung tissues. *P < 0.05, **P < 0.01 by one-way non-parametric ANOVA.
Der p-Induced Chronic Asthma Was Attenuated in mIL-19 pAb-Treated Mice
To further confirm whether direct inhibition of IL-19 would attenuate chronic asthma Der p-treated mice, we treated mice with mIL-19 neutralizing antibodies. We previously generated the anti-human IL-19 mAb 1BB1, which showed specific neutralization activity against human IL-19 in a mouse model of breast cancer (39, 40), but 1BB1 did not effectively neutralize mouse IL-19 protein in vivo (data not shown). Thus, we generated rabbit polyclononal antibodies against mIL-19 and treated the Der p-induced asthmatic mice with the anti-mIL-19 pAb. The pre-immune serum-derived pAb was used as control (Figure 6A). AHR analysis showed that IL-19 blockade improved lung function in the Der p-challenged mice (Figure 6B). H&E staining revealed that IL-19 inhibition also reduced the degree of lung inflammation and the levels of bronchial wall thickening (Figures 6C–E). In addition, the number of infiltrated immune cells, eosinophils, and neutrophils in their BALF (Figure 6F), and the serum levels of IL-13, IL-19, IgE (Figure 6G), BALF IgA (Figure 6H), and lung tissue CCL11 and IL-33 levels (Figure 6I) were significantly lower in mIL-19 pAb-treated group than those of pre-immune pAb treated control group mice. These data together indicated that inhibition of IL-19 signaling through blocking IL-19 or IL-20R1 were both effective to attenuate Der p-induced pulmonary inflammation in the mouse model.
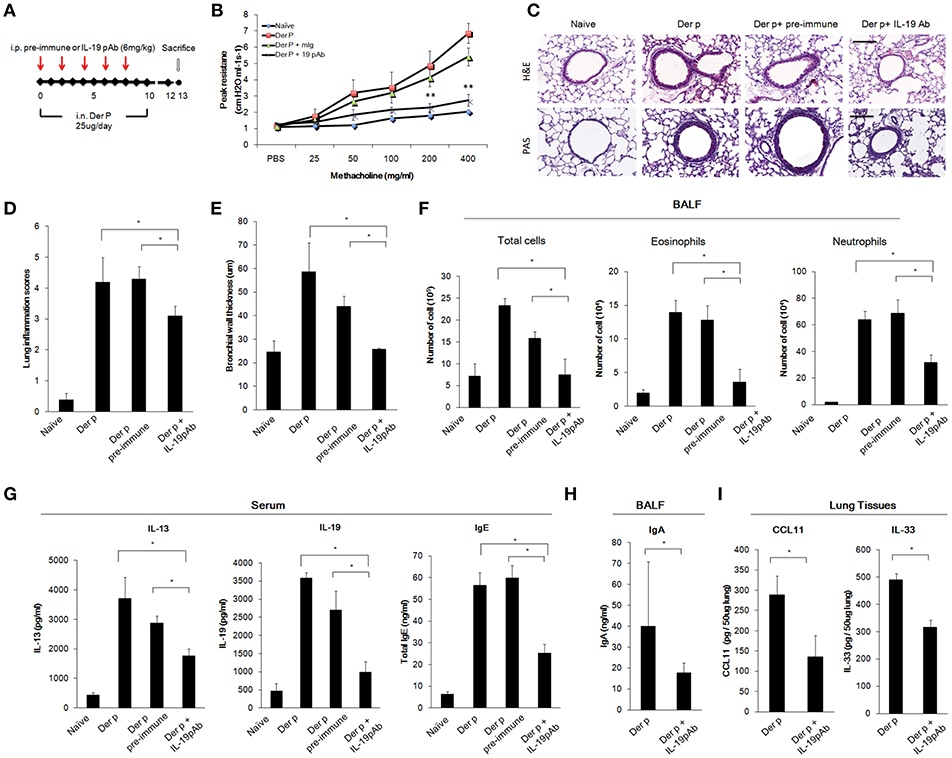
Figure 6. Anti-IL-19 antibody attenuated Der p-induced chronic asthma. (A) Protocol of Der p-induced asthmatic mouse model with anti-IL-19 pAb treatment. C57BL/6 mice were intranasally (i.n.) challenged with Der p (25 μg/mice/day) for 10 days to induce chronic airway inflammation. Anti-IL-19 pAb (6 mg/kg) or a control pre-immune IgG (6 mg/kg) was intraperitoneally injected into the Der p sensitized mice (n = 6 in each group). (B) AHR was analyzed in the mice 3 days after the last Der p treatment. Peak AHR was methacholine dose-dependently lower in 51D-treated mice. (C) Hematoxylin and eosin (H&E) staining showed reduced airway thickening and PAS staining showed fewer goblet cell areas in anti-IL-19 pAb-treated mice. (D) Lung inflammation scores of the mice. (E) Airway wall thickness. (F) Number of infiltrated immune cells, eosinophils, and neutrophils in mouse BALF. (G) Serum IL-13, IL-19, and IgE levels in the mice. (H) BALF IgA levels. (I) CCL11 and IL-33 levels in the lung tissues. *P < 0.05, **P < 0.01 by one-way non-parametric ANOVA.
Airway Inflammation Was Less Severe in Ovalbumin-Induced Asthmatic Rats Treated With Anti-IL-19 mAb 1BB1
Our results demonstrated that targeting IL-19 or IL-20R1 could ameliorate Der p-induced asthma in the mouse model (Figures 5, 6). We thought to further confirm whether targeting IL-19 could ameliorate allergen-induced allergic airway inflammation in rats. In contrast to the use of Der p in mouse model, we established the asthmatic rat model by repeated immunization of rats with ovalbumin (OVA), which is derived from chicken eggs and is a frequently used allergen to induce a robust allergic pulmonary inflammation in laboratory rodents (41). In addition, our previous study demonstrated that our anti-human IL-19 mAb 1BB1 could cross-react with rat IL-19 and attenuated collagen-induced arthritis in rats (41). Thus, we tested whether IL-19 specific mAb 1BB1 would attenuate pulmonary inflammation in the OVA-induced asthma model in rats. To establish the rat asthmatic model, WT Sprague-Dawley rats were sensitized by repeated immunization of OVA 3 times on day 0, 7, and 14. The rats were then challenged by daily OVA intranasally from day 25 for 5 days to induce allergic lung inflammation (Figure 7A). For the antibody treatment groups, 1BB1 or control mIgG were intraperitoneally injected 1 h before OVA treatments (Figure 7A). The OVA-sensitized rats developed pathological airway inflammation outcomes similar to those in Der p-treated mice (Figure 7B). Histopathological analysis by H&E and PAS staining showed less lung inflammation and bronchial wall thickening and fewer mucus-secreting goblet cell areas in 1BB1-treated rats (Figures 7C,D). The number of infiltrated immune cells in their BALF (Figure 7E), and the mRNA levels of IL-19 and IL-13 (Figure 7F) in the lung tissues from 1BB1-treated rats were lower than in control group rats. These data together indicated that blocking IL-19 by polyclonal and monoclonal antibodies were effective to attenuate the pathological outcomes of allergen-induced asthma both in the mouse and rat models.
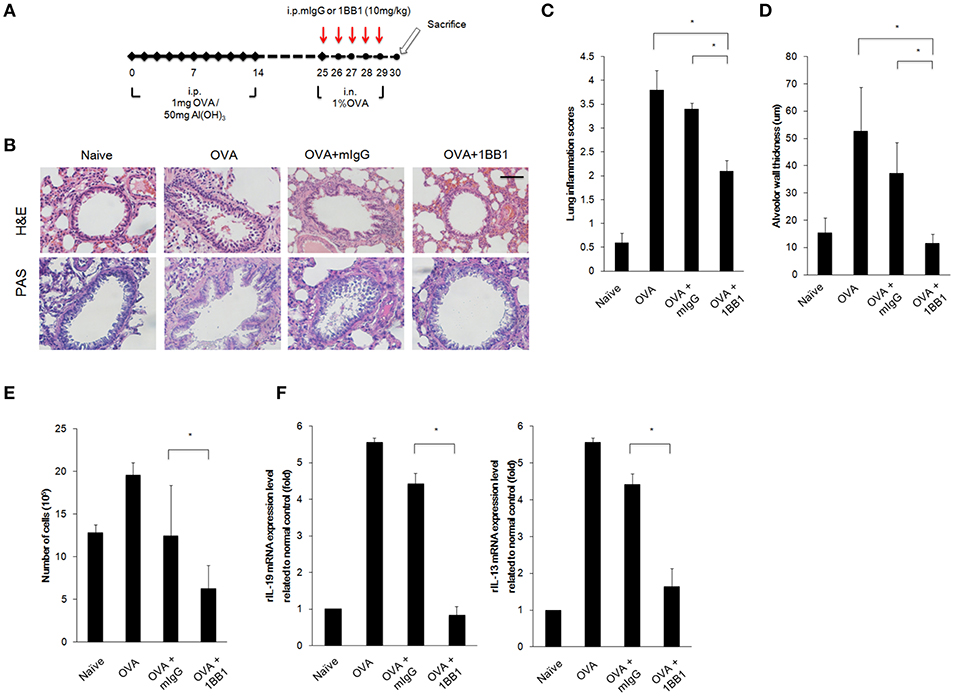
Figure 7. OVA-induced asthma was ameliorated in 1BB1-treated rats. (A) Protocol of ovalbumin (OVA)-induced asthmatic mouse model with anti-IL-19 mAb (1BB1) treatment. Wild-type Sprague-Dawley rats (n = 6 per group) were intraperitoneally (i.p.) injected with 1 mg of OVA adsorbed on 50 mg of gelatinous Al(OH)3 on days 0, 7, and 14. The rats were treated intranasally (i.n.) daily with 1% OVA in 100 μL of PBS from day 25 for 5 days. They were then dosed via i.p. injection with 1BB1 (10 mg/kg) or immunogammaglobulin (mIgG) (10 mg/kg) (control) 1 h before OVA treatment. (B) H&E and PAS staining showed less airway thickening in 1BB1-treated1 asthmatic rats. (C) Lung inflammation scores of the mice. (D) Airway wall thickness. (E) Number of infiltrated immune cells in rat BALF. (F) qRT-PCR analysis for the mRNA levels of IL-13 and IL-19 in rat lung tissue. *P < 0.05 by one-way non-parametric ANOVA.
Discussion
In this study, we established the mouse asthma models by repeatedly exposing the airways of mice to Der p and injecting rats with OVA and analyzed the mechanisms of the inflammatory responses. The Der p-induced asthma model was associated with increased AHR and bronchial wall thickening, elevated serum IL-19, IL-13, IgE levels, BALF IgA levels, increased immune cell infiltration, and tissue IL-33 and CCL11 in the lungs. Results from IL-20R1−/− mice suggested that IL-19 signaling through IL-20R1 contributed to Der p-induced asthma. The IL-19 receptor has two subunits: IL-20R1 and IL-20R2. IL-20R2 is constitutively expressed in lung epithelium and other types of tissue (42). IL-20R1 is also expressed in lung epithelium; thus, lung epithelium could be the target of IL-19 (42). In this study, our results further confirmed that IL-20R2 was constitutively expressed while IL-19 and IL-20R1 were upregulated in the AM, ATII, and AECs in the lung tissues of mice with Der p challenge. These results together suggested that Der p-induced IL-19 could mediate lung inflammation via targeting to the cells expressing both of the IL-19 receptor subunits (IL-20R1 and IL-20R2) in the autocrine and paracrine manner.
IL-19 was shown to autoregulate IL-19 production in vitro (43). In the current study, Der p-induced IL-19 expression in A549 and BEAS-2B lung epithelial cells (Figure 1) and upregulated serum IL-19 levels in mice (Figure 3). In asthmatic mice, the degree of airway inflammation and IL-19 serum levels were lower in IL-20R1−/− mice and 51D-treated mice, which suggested that IL-19 levels are associated with the outcomes of airway inflammation. The mechanism of the auto-amplification loop might explain why IL-19 levels were lower in the IL-20R1−/− and 51D-treated mice. Autoregulation of IL-19, however, was not observed in IL-19-treated A549 cells (16), which likely imply that other immune cells, such as activated alveolar macrophages, contribute to the expression of IL-19 during airway inflammation.
In our mouse model, IL-20R1 deficiency reduced Der p-induced lung inflammation, bronchial wall thickening, and the levels of Th2 cytokines (IL-13, IL-33, CCL11), IgA, suggesting that IL-20R1 downstream signaling contribute to exacerbation of type 2 immune responses in the context of Der p-induced lung inflammation. We thus hypothesize that IL-20R1 is crucial for the effecter function of IL-19 to regulate T cell differentiation. In vitro, we confirmed that IL-19 promoted Th2 differentiation from CD4+ naïve T cells and that the Th2 differentiation was abolished in CD4+ T cells derived from IL-20R1 mice (Figure 2). In vivo, we found that using IL-20R1 mAb 51D to inhibit IL-20R1 attenuated Der p-induced asthma and Th2 cytokine expression. Additionally, IL-19 pAb treatment had a similar protective effect against Der p-induced pulmonary inflammation. These data together indicated that targeting IL-19 signaling might be a potential therapeutic strategy for treating or preventing the development of chronic asthma. IL-20, another IL-10 family cytokine, also binds to the IL-20R1/IL-20R2 receptor complex. However, the expression level of IL-20 was not altered after Der p treatment (data not shown), which suggested that IL-19 plays more dominant roles in IL-20R1-mediated allergic airway inflammation.
Allergic asthma is a hypersensitivity reaction initiated by immunologic mechanisms mediated by IgE antibodies (1). IgE is important for the initiation and propagation of the inflammatory cascade and allergic responses (9). Thus, IgE-mediated immunologic pathways have long been an attractive target for therapeutic agents in asthma and other allergic diseases (8). In the current study, we found that using antibodies to inhibit IL-19 or IL-20R1 in asthmatic mice lowered their serum IgE levels. This indicates that targeting IL-19 signaling might attenuate or even prevent the early development of airway remodeling and inflammation. Thus, IL-19 inhibitors might be novel and potent therapeutics for asthma because IL-19 likely mediates early allergic response after allergen exposure and attribute to the development of Th2-dominant airway inflammation. It is interesting to note that IL-20R1 deficiency did not reduce the serum IgE levels following Der p challenge compared with WT mice, which might attribute to higher basal serum IgE levels in naïve IL-20R1−/− mice than did in WT mice (23.50 ± 16.4 ng/ml and 9.38 ± 3.64, respectively). Despite the unaltered serum IgE levels in IL-20R1−/− mice upon Der p challenge, IL-20R1−/− mice were more resistant to Der p-induced allergic airway inflammation compared to WT mice. An unidentified role of IL-20R1 downstream signaling on the regulation of IgE synthesis may exist in the context of genetic deficiency of IL-20R1 signaling and requires further investigation.
We previously reported that IL-19 upregulated IL-1β and TNF-α in A549 lung epithelial cells, which might promote an inflammatory response and cause pulmonary injury (16). Moreover, overexpression of IL-19 upregulated serum IL-13 levels only in asthmatic mice but not healthy mice, which suggested that IL-19-mediated allergic response might be dependent upon the tissue microenvironment (21). In the current study, we found that IL-19 induced lung epithelial cells to express TSLP, which is involved in allergic airway inflammation by activating group 2 innate lymphoid cells (ILC2) (38). TSLP released from injured epithelium after antigenic stimulation of allergic asthmatic airways is a key initiator of type-2 inflammation (38). It is possible that IL-19 activates ILC2 through TSLP and leads to elevated IL-13 levels. Our in vivo study also showed that the protein levels of IL-33 and CCL11 were reduced by IL-20R1 deficiency, IL-19 inhibition, or IL-20R1 blockades, suggesting that blocking IL-19 signaling inhibits allergic lung inflammation via reduction of the pro-inflammatory cytokines and reduced immune cell infiltration. IL-33 is also a key mediator for activation of ILC2 and eosinophils (38). CCL11 has been shown to promote eosinophil infiltration in allergen-induced lung inflammation (37). Although we did not analyze whether IL-19 could directly activate these immune cells (eosinophils, neutrophils, and ILCs), we did not exclude the possibility that IL-19 contributes to the amplification of type 2 immune responses via activation of the tissue-derived inflammatory mediators such as TSLP and IL-33. Whether IL-19 directly activates ILC2 and whether ILC2 activation is involved in IL-19-mediated airway inflammation require additional investigation. Comprehensive profiling of the direct target cells of IL-19 during the progression of allergic pulmonary inflammation is needed.
Rodent models of experimental allergic asthma have contributed greatly to our current understanding of the pathogenesis of this disease. In the present study, we established the Der p- or OVA-induced allergic airway inflammation models in mice and rats, respectively. The major difference between these two models is that allergens derived from HDMs exhibit intrinsic enzymatic protease activity and mimic the route of allergen exposure and allergic responses that are observed during human allergic airway inflammation (41). Conventional OVA-models, however, in rodents require co-administration of adjuvants to establish successful sensitization. The OVA model may not be the most disease-relevant model for allergic airway inflammation (44). The use of HDMs as allergens in rats is limited owing to the higher amount and cost of house dust mite preparation. Nevertheless, our data provided evidence that blocking IL-19 signaling may be a potential treatment strategy in these two commonly used preclinical rodent models of experimental allergic asthma.
In summary, this is the first report demonstrating that inhibition of IL-19 signaling by targeting IL-19 or IL-20R1 protected mice and rats from developing asthma in the rodent models. These findings provide new evidence that IL-19 is involved in the development of allergen-induced airway remodeling and inflammation and also indicates that IL-19 signaling is a promising target for anti-asthmatic intervention.
Ethics Statement
This study was carried out in accordance with the recommendations of the National Institutes of Health standards and guidelines for the care and use of experimental animals. The protocol was approved by the Animal Ethics Committee of National Cheng Kung University (IACUC NO. 107272 and 105077).
Author Contributions
J-YW and M-SC designed the study. Y-HW and Y-LL performed the experiments. Y-HW and W-YC analyzed the data. Y-HW, W-YC, and M-SC wrote the manuscript. W-YC and J-YW provided critical technical and scientific guidance and discussion.
Funding
This work was supported by research grant from the Ministry of Science and Technology, Taiwan (105-2320-B-006-040-).
Conflict of Interest Statement
The authors declare that the research was conducted in the absence of any commercial or financial relationships that could be construed as a potential conflict of interest.
References
1. Busse WW, Lemanske RF Jr. Asthma. N Engl J Med. (2001) 344:350–62. doi: 10.1056/NEJM200102013440507
2. Nieminen MM, Kaprio J, Koskenvuo M. A population-based study of bronchial asthma in adult twin pairs. Chest. (1991) 100:70–5. doi: 10.1378/chest.100.1.70
3. King C, Brennan S, Thompson PJ, Stewart GA. Dust mite proteolytic allergens induce cytokine release from cultured airway epithelium. J Immunol. (1998) 161:3645–51.
4. Gough L, Schulz O, Sewell HF, Shakib F. The cysteine protease activity of the major dust mite allergen Der p 1 selectively enhances the immunoglobulin E antibody response. J Exp Med. (1999) 190:1897–902. doi: 10.1084/jem.190.12.1897
5. Shakib F, Ghaemmaghami AM, Sewell HF. The molecular basis of allergenicity. Trends Immunol. (2008) 29:633–42. doi: 10.1016/j.it.2008.08.007
6. Sokol CL, Barton GM, Farr AG, Medzhitov R. A mechanism for the initiation of allergen-induced T helper type 2 responses. Nat Immunol. (2008) 9:310–8. doi: 10.1038/ni1558
7. Wynn TA. Type 2 cytokines: mechanisms therapeutic strategies. Nat Rev Immunol. (2015) 15:271–82. doi: 10.1038/nri3831
8. Poole JA, Matangkasombut P, Rosenwasser LJ. Targeting the IgE molecule in allergic and asthmatic diseases: review of the IgE molecule and clinical efficacy. J Allergy Clin Immunol. (2005) 115:S376–85. doi: 10.1016/j.jaci.2004.12.1124
9. Fajt ML, Wenzel SE. Asthma phenotypes and the use of biologic medications in asthma and allergic disease: the next steps toward personalized care. J Allergy Clin Immunol. (2015) 135:299–310; quiz 311. doi: 10.1016/j.jaci.2014.12.1871
10. Ouyang W, Rutz S, Crellin NK, Valdez PA, Hymowitz SG. Regulation and functions of the IL-10 family of cytokines in inflammation and disease. Annu Rev Immunol. (2011) 29:71–109. doi: 10.1146/annurev-immunol-031210-101312
11. Sabat R. IL-10 family of cytokines. Cytokine Growth Factor Rev. (2010) 21:315–24. doi: 10.1016/j.cytogfr.2010.11.001
12. Autieri MV. IL-19 and other IL-20 family member cytokines in vascular inflammatory diseases. Front Immunol. (2018) 9:700. doi: 10.3389/fimmu.2018.00700
13. Gallagher G, Dickensheets H, Eskdale J, Izotova LS, Mirochnitchenko OV, Peat JD, et al. Cloning, expression and initial characterization of interleukin-19 (IL-19), a novel homologue of human interleukin-10 (IL-10). Genes Immun. (2000) 1:442–50. doi: 10.1038/sj.gene.6363714
14. Liao YC, Liang WG, Chen FW, Hsu JH, Yang JJ, Chang MS. IL-19 induces production of IL-6 and TNF-alpha and results in cell apoptosis through TNF-alpha. J Immunol. (2002) 169:4288–97. doi: 10.4049/jimmunol.169.8.4288
15. Hsu YH, Hsieh PP, Chang MS. Interleukin-19 blockade attenuates collagen-induced arthritis in rats. Rheumatology. (2012) 51:434–42. doi: 10.1093/rheumatology/ker127
16. Hsu YH, Li HH, Sung JM, Chen WT, Hou YC, Chang MS. Interleukin-19 mediates tissue damage in murine ischemic acute kidney injury. PLoS ONE. (2013) 8:e56028. doi: 10.1371/journal.pone.0056028
17. Li HH, Lin YC, Chen PJ, Hsiao CH, Lee JY, Chen WC, et al. Interleukin-19 upregulates keratinocyte growth factor and is associated with psoriasis. Br J Dermatol. (2005) 153:591–5. doi: 10.1111/j.1365–2133.2005.06665.x
18. Hsing CH, Li HH, Hsu YH, Ho CL, Chuang SS, Lan KM, et al. The distribution of interleukin-19 in healthy and neoplastic tissue. Cytokine. (2008) 44:221–8. doi: 10.1016/j.cyto.2008.06.007
19. Jain S, Gabunia K, Kelemen SE, Panetti TS, Autieri MV. The anti-inflammatory cytokine interleukin 19 is expressed by and angiogenic for human endothelial cells. Arterioscler Thromb Vasc Biol. (2011) 31:167–75. doi: 10.1161/ATVBAHA.110.214916
20. Hsing CH, Chiu CJ, Chang LY, Hsu CC, Chang MS. IL-19 is involved in the pathogenesis of endotoxic shock. Shock. (2008) 29:7–15. doi: 10.1097/SHK.0b013e318067de40
21. Liao SC, Cheng YC, Wang YC, Wang CW, Yang SM, Yu CK, et al. IL-19 induced Th2 cytokines and was up-regulated in asthma patients. J Immunol. (2004) 173:6712–8. doi: 10.4049/jimmunol.173.11.6712
22. Hsu YH, Chen WY, Chan CH, Wu CH, Sun ZJ, Chang MS. Anti-IL-20 monoclonal antibody inhibits the differentiation of osteoclasts and protects against osteoporotic bone loss. J Exp Med. (2011) 208:1849–61. doi: 10.1084/jem.20102234
23. Lin IP, Hsu YS, Kang SW, Hsieh MH, Wang JY. Escherichia coli heat-labile detoxified enterotoxin modulates dendritic cell function and attenuates allergic airway inflammation. PLoS ONE. (2014) 9:e90293. doi: 10.1371/journal.pone.0090293
24. Chen WY, Hong J, Gannon J, Kakkar R, Lee RT. Myocardial pressure overload induces systemic inflammation through endothelial cell IL-33. Proc Natl Acad Sci USA. (2015) 112:7249–54. doi: 10.1073/pnas.1424236112
25. Chen WY, Chang YJ, Su CH, Tsai TH, Chen SD, Hsing CH, et al. Upregulation of Interleukin-33 in obstructive renal injury. Biochem Biophys Res Commun. (2016) 473:1026–32. doi: 10.1016/j.bbrc.2016.04.010
26. Zeldin DC, Wohlford-Lenane C, Chulada P, Bradbury JA, Scarborough PE, Roggli V, et al. Airway inflammation and responsiveness in prostaglandin H synthase-deficient mice exposed to bacterial lipopolysaccharide. Am J Respir Cell Mol Biol. (2001) 25:457–65. doi: 10.1165/ajrcmb.25.4.4505
27. Klopfleisch R. Multiparametric and semiquantitative scoring systems for the evaluation of mouse model histopathology–a systematic review. BMC Vet Res. (2013) 9:123. doi: 10.1186/1746-6148-9-123
28. Bandukwala HS, Clay BS, Tong J, Mody PD, Cannon JL, Shilling RA, et al. Signaling through Fc gamma RIII is required for optimal T helper type (Th)2 responses and Th2-mediated airway inflammation. J Exp Med. (2007) 204:1875–89. doi: 10.1084/jem.20061134
29. Chiu YS, Wei CC, Lin YJ, Hsu YH, Chang MS. IL-20 and IL-20R1 antibodies protect against liver fibrosis. Hepatology. (2014) 60:1003–14. doi: 10.1002/hep.27189
30. Kauffman HF, Tamm M, Timmerman JA, Borger P. House dust mite major allergens Der p 1 and Der p 5 activate human airway-derived epithelial cells by protease-dependent and protease-independent mechanisms. Clin Mol Allergy. (2006) 4:5. doi: 10.1186/1476-7961-4-5
31. Asokananthan N, Graham PT, Stewart DJ, Bakker AJ, Eidne KA, Thompson PJ, et al. House dust mite allergens induce proinflammatory cytokines from respiratory epithelial cells: the cysteine protease allergen, Der p 1, activates protease-activated receptor (PAR)-2 and inactivates PAR-1. J Immunol. (2002) 169:4572–8. doi: 10.4049/jimmunol.169.8.4572
32. Furuta GT, Ackerman SJ, Lu L, Williams RE, Wershil BK. Stem cell factor influences mast cell mediator release in response to eosinophil-derived granule major basic protein. Blood. (1998) 92:1055–61.
33. West EE, Kashyap M, Leonard WJ. TSLP: a key regulator of asthma pathogenesis. Drug Discov Today Dis Mech. (2012) 9:e83–8. doi: 10.1016/j.ddmec.2012.09.003
34. Zhu Z, Oh MH, Yu J, Liu YJ, Zheng T. The Role of TSLP in IL-13-induced atopic march. Sci Rep. (2011) 1:23. doi: 10.1038/srep00023
35. Wills-Karp M, Luyimbazi J, Xu X, Schofield B, Neben TY, Karp CL, et al. Interleukin-13: central mediator of allergic asthma. Science. (1998) 282:2258–61. doi: 10.1126/science.282.5397.2258
36. Shima K, Koya T, Tsukioka K, Sakagami T, Hasegawa T, Fukano C, et al. Effects of sublingual immunotherapy in a murine asthma model sensitized by intranasal administration of house dust mite extracts. Allergol Int. (2017) 66:89–96. doi: 10.1016/j.alit.2016.05.012
37. Teixeira AL, Gama CS, Rocha NP, Teixeira MM. Revisiting the role of eotaxin-1/CCL11 in psychiatric disorders. Front Psychiatry. (2018) 9:241. doi: 10.3389/fpsyt.2018.00241
38. Smith SG, Gugilla A, Mukherjee M, Merim K, Irshad A, Tang W, et al. Thymic stromal lymphopoietin and IL-33 modulate migration of hematopoietic progenitor cells in patients with allergic asthma. J Allergy Clin Immunol. (2015) 135:1594–602. doi: 10.1016/j.jaci.2014.12.1918
39. Chen YY, Li CF, Yeh CH, Chang MS, Hsing CH. Interleukin-19 in breast cancer. Clin Dev Immunol. (2013) 2013:294320. doi: 10.1155/2013/294320
40. Hsing CH, Cheng HC, Hsu YH, Chan CH, Yeh CH, Li CF, et al. Upregulated IL-19 in breast cancer promotes tumor progression and affects clinical outcome. Clin Cancer Res. (2012) 18:713–25. doi: 10.1158/1078–0432.CCR-11-1532
41. Nials AT, Uddin S. Mouse models of allergic asthma: acute and chronic allergen challenge. Dis Model Mech. (2008) 1:213–20. doi: 10.1242/dmm.000323
42. Dumoutier L, Leemans C, Lejeune D, Kotenko SV, Renauld JC. Cutting edge: STAT activation by IL-19, IL-20 and mda-7 through IL-20 receptor complexes of two types. J Immunol. (2001) 167:3545–9. doi: 10.4049/jimmunol.167.7.3545
43. Jordan WJ, Eskdale J, Boniotto M, Lennon GP, Peat J, Campbell JD, et al. Human IL-19 regulates immunity through auto-induction of IL-19 and production of IL-10. Eur J Immunol. (2005) 35:1576–82. doi: 10.1002/eji.200425317
Keywords: interleukin-19, IL-20R1, asthma, airway inflammation, allergy
Citation: Weng Y-H, Chen W-Y, Lin Y-L, Wang J-Y and Chang M-S (2019) Blocking IL-19 Signaling Ameliorates Allergen-Induced Airway Inflammation. Front. Immunol. 10:968. doi: 10.3389/fimmu.2019.00968
Received: 13 November 2018; Accepted: 15 April 2019;
Published: 30 April 2019.
Edited by:
Rubén Francés, Hospital General Universitario de Alicante, SpainReviewed by:
Remo Castro Russo, Federal University of Minas Gerais, BrazilVanessa Pinho, Federal University of Minas Gerais, Brazil
Copyright © 2019 Weng, Chen, Lin, Wang and Chang. This is an open-access article distributed under the terms of the Creative Commons Attribution License (CC BY). The use, distribution or reproduction in other forums is permitted, provided the original author(s) and the copyright owner(s) are credited and that the original publication in this journal is cited, in accordance with accepted academic practice. No use, distribution or reproduction is permitted which does not comply with these terms.
*Correspondence: Jiu-Yao Wang, a122@mail.ncku.edu.tw
Ming-Shi Chang, mschang@mail.ncku.edu.tw
†These authors have contributed equally to this work