- 1Division of Vaccine Research, Institute of Human Virology, University of Maryland School of Medicine, Baltimore, MD, United States
- 2Thayer School of Engineering, Dartmouth College, Hanover, NH, United States
- 3Viral Evolution and Transmission Unit, Department of Immunology, Transplantation and Infectious Diseases, IRCCS San Raffaele Scientific Institute, Milan, Italy
- 4INSERM U1109, Fédération Hospitalo-Universitaire (FHU) OMICARE, Fédération de Médecine Translationnelle de Strasbourg (FMTS), Université de Strasbourg, Strasbourg, France
- 5Vaccine Branch, Center for Cancer Research, National Cancer Institute, National Institues of Health, Bethesda, MD, United States
- 6Department of Microbiology and Immunology, The University of Melbourne, at the Peter Doherty Institute for Infection and Immunity, Melbourne, VIC, Australia
- 7Division of Human Biology, Fred Hutchinson Cancer Research Center, Seattle, WA, United States
- 8Center for Virology and Vaccine Research, Beth Israel Deaconess Medical Center/Harvard Medical School, Boston, MA, United States
- 9Department of Surgery and Duke Human Vaccine Institute, Duke University Medical Center, Durham, NC, United States
- 10Global HIV Vaccine Enterprise, New York, NY, United States
It is now well-accepted that Fc-mediated effector functions, including antibody-dependent cellular cytotoxicity (ADCC), can contribute to vaccine-elicited protection as well as post-infection control of HIV viremia. This picture was derived using a wide array of ADCC assays, no two of which are strictly comparable, and none of which is qualified at the clinical laboratory level. An earlier comparative study of assay protocols showed that while data from different ADCC assay formats were often correlated, they remained distinct in terms of target cells and the epitopes and antigen(s) available for recognition by antibodies, the effector cells, and the readout of cytotoxicity. This initial study warrants expanded analyses of the relationships among all current assay formats to determine where they detect overlapping activities and where they do not. Here we summarize knowns and unknowns of assaying ADCC against HIV-1.
Introduction
That Fc-mediated effector function contributes to antibody-mediated protection against HIV-1 for both broadly neutralizing antibodies (bnAbs) as well as non-neutralizing antibodies has become well-accepted. Although there are several categories of Fc receptors, this report is focused on the Fc-gamma receptors (FcγR) that are expressed largely on cells of the hematopoietic lineage including, B-cells, T-cells, monocytes/macrophages, dendritic cells, NK cells, and granulocytes, as well as on follicular dendritic cells of mesenchymal origin. FcγR play a pivotal role in coupling adaptive antibody (Ab) responses with innate immune effector responses by the recognition of antigen-antibody complexes (i.e., immune complexes, IC). Effector cell recognition by FcγR of IC formed on the surfaces of viruses, bacteria, and eukaryotic cells can result in their elimination by various mechanisms. In addition, FcγR recognition of IC on follicular dendritic cells as well as on B-cells plays a key role in the regulation of Ab responses. Thus, FcγR-mediated effector functions very likely play a pivotal role in vaccine-elicited protection against HIV-1 as well as in the prophylaxis and treatment of HIV-1 infections with Abs. Despite their apparent importance, there is still no consensus about which types of FcγR-mediated effector functions contribute to vaccine-elicited protection against HIV-1.
ADCC is characterized by IC coupled interactions between an effector cell and target cell that leads to target cell death. IC coupling occurs via the interaction of the Ab Fc region and the FcR on the effector cell and the Ab Fab region with antigen on the target cell. This interaction typically triggers the release of cytotoxic granules containing perforin and granzymes from the effector cells to the target cell via an immunologic synapse resulting in target cell lysis. ADCC has been correlated with vaccine-elicited protection in non-human primates (NHPs), reduced risk of infection/mortality in the setting of mother to child transmission, and in the RV144 human vaccine trial, where it emerged as a secondary correlate of reduced infection risk [(1–5), reviewed in (6)]. These observations place ADCC at the forefront as a potential correlate and mechanism of protection against HIV-1. Although the data strongly suggest a role in Ab-mediated protection against HIV-1, questions remain about which of the many ADCC assay formats best reflects the biology of protection and therefore should be used in clinical trials of HIV-1 vaccines. This issue is complicated by the necessity of the assay to be amenable to qualification as a clinical assay in a high-throughput format.
Complexities of Measuring ADCC
At the minimum, an ADCC assay requires an effector cell, an antigen-bearing target cell, Abs, and a means to measure cytotoxicity. These nominal requirements veil the true complexity of ADCC assays and the biology they are thought to represent. For example, there are major differences among the various assay formats in effector cell type, target cell type, antigen targets, and the readout of activity (Figure 1 and Table 1).
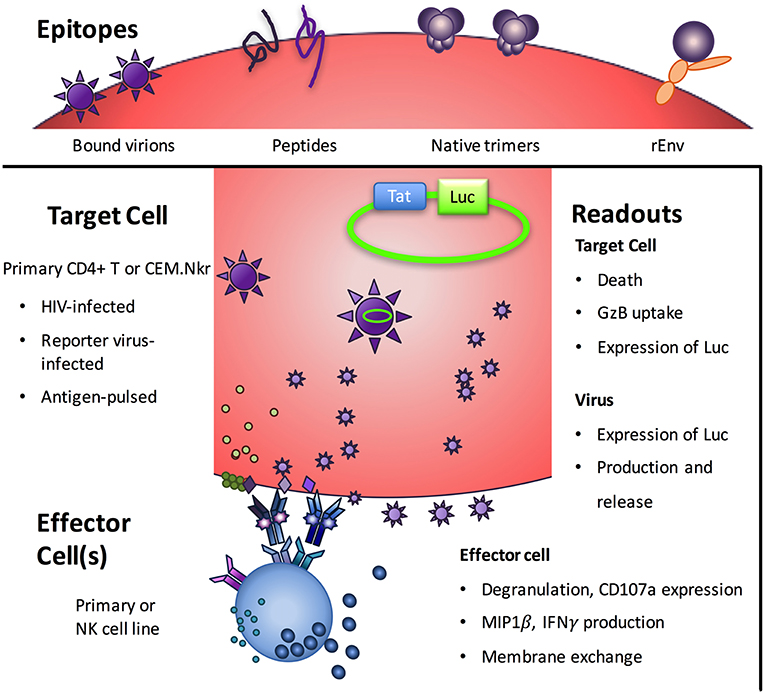
Figure 1. Notable variables among ADCC assays. Beyond the monoclonal or polyclonal antibodies being assessed, ADCC assays vary in terms of the viral epitopes presented, the target cells on which they are presented, the effector cells that will respond, and the readout of the biological activity assayed. Image adapted from (7).
A major point of differentiation among ADCC assay formats is how target cells present antigen, which has a major impact on the specificity of Ab responses detected by the assay. Some assays use target cells incubated with recombinant extracellular domains of envelope protein trimers or gp120/140 subunits, or even peptides (8, 20). While there may be more than one means of association between envelope proteins in such “coated” cells, the interaction between recombinant envelope and cells is thought to primarily be achieved via direct interaction with CD4, resulting in CD4-induced conformational changes. In contrast, assays utilizing inactivated virus, reporter viruses, and infectious virions differ in a number of additional ways with respect to their antigenic composition. Infection of target cells with unmodified infectious virus will downregulate CD4 from the cell surface, supporting presentation of “native trimer” envelope conformations, as opposed to CD4-induced (CD4i) monomeric conformational states. Relative to “coated cell” assays, those that employ reporter viruses can have the advantage of presenting full envelope glycoprotein with native transmembrane domains and associated epitopes. However, these epitopes too may differ from unmodified viruses in their level of envelope expression and their ability to drive CD4-downregulation, resulting again in presentation of different conformational states of envelope, and sensitivity to different antibodies. Further, CD4 receptor downregulation, envelope expression, virus budding, and envelope shedding are all time-dependent processes, complicating comparison of readouts from different ADCC assay protocols. It is possible that the spectrum of envelope states on infecting and/or budding virus relevant to anti-viral activity in vivo may be quite broad. As one specific example, longitudinal exposure of infected cells to Dual-Affinity Re-Targeting (DART) molecules derived from combinations of anti-HIV-1 non-neutralizing and anti-CD3 targeting antibody Fabs resulted in CD3+ T cell mediated killing even when surface expression of Env appeared low (34). Thus, it is clear that there is a rich milieu of different viral epitopes addressed across different assay types and over different time scales.
In addition to a multitude of Env-bearing target cells studied, ADCC assays commonly employ different effector cells. These range from NK cell lines to mixed populations of primary peripheral blood mononuclear cells (PBMCs). This variety of effector populations expresses different levels and compositions of antibody receptors including FcγR and FcαR, as well as accessory proteins involved in downstream signaling and biological activities. Even among NK cells, expression of higher and lower affinity polymorphic variants of FcγRIIIa, at different levels and in the context of different signaling partners (35, 36), are known to impact outcomes in ADCC assays. PBMC-based assays will include monocyte populations expressing FcγRIIa and FcγRIIb receptors that (a) have different preferences among IgG subclasses and Fc glycoforms; (b) are considered activating and inhibitory, respectively (37–39), and; (c) vary in allotypic composition of FcγR from donor to donor. Beyond inherent differences in receptor expression and activities, effector cells are present at different sites at different levels, and tissue localization can change FcγR expression levels, activation status, and functional competence (40–42). Assays are often conducted using mixed PBMCs, purified primary cell subsets, and/or cell lines; yet, these cells may not accurately reflect the activities most relevant to tissue-resident effector function in vivo, across different sites and local environmental cues. Activity can also be affected by other, less obvious factors. For example, expression of FcαR and the presence of IgA that binds to but does not activate FcαR has been reported to interfere with responses from activating FcγR (43–45). In contrast, IgA and its receptors can also drive effector function (46–48). The complexity of this biology is perhaps most simply demonstrated by the observation that both the inhibitory and activating roles of FcαR rely on the common γ chain, which is also critical to FcγR-mediated activities. However, the potential relevance of such nuanced cell biology to outcomes of HIV vaccination can be found in observations that IgA can interfere with IgG-mediated ADCC (49, 50), and that IgA and ADCC were observed to have opposing relationships to risk of infection among vaccine recipients in the RV144 vaccine trial (3) whereas IgA has shown positive associations with protection in NHP vaccine models (46, 51).
In addition, assayed outcomes differ significantly across approaches. They include readouts associated with target cells and readouts of effector cells. Among target cell endpoints, Cr51 release, dye release, and reporter loss have all been assayed. Among effector cells, dye uptake, CD107a staining, MIP1α and IFNγ production, granzyme transfer, and ligand transfer, among others have been assayed. Further, even outcomes such as reduction of virus outgrowth have been utilized (26). Overall, given this variation in (a) presentation of antigen epitopes and conformations on target cells, (b) polymorphisms, levels, and composition of expressed FcγR and downstream signaling partners, within and among distinct effector cell types with different preferences among antibody subclasses and glycoforms, and (c) endpoints alternatively focused on target or effector cells relating activities ranging from target cell death to expression of cytokines from effector cells, rich insights into these aspects of immunobiology result from analysis of various monoclonal and polyclonal antibody samples across studies.
Impact of Vaccine-Induced ADCC Activity on Protective Efficacy in Non-Human Primates; Early Studies
Early work reported consistent correlations between ADCC and vaccine-elicited protection against SIV and SHIV in NHP models. This work was principally accomplished using the rapid fluorometric antibody-dependent cellular cytotoxicity assay (RFADCC) (Figure 2) (8), in which CEM.Nkr (NK-resistant) target cells are double-stained with a membrane and a viability dye, and following incubation with antibodies and PBMCs as effector cells, target cell death is assessed by flow cytometry and quantified as the fraction of non-viable target cells. This assay continues to be used widely to evaluate ADCC. Correlations between ADCC titers and protection were observed in both single high-dose and repeat low-dose SIV challenge studies. In the single high-dose SIV challenge studies, the NHP were not protected from infection, but post-infection control of viremia, correlated with ADCC, was observed in the vaccine groups across several studies (52, 53). Using repeat low-dose challenge protocols, which are thought to more accurately reflect sexual transmission in humans, vaccinated NHP resisted infection with SIV. This protection against infection also correlated with ADCC (54). Taken together, the body of literature developed by Dr. Robert-Guroff and her colleagues strongly suggests that ADCC assessed by the RFADCC method correlates with vaccine-elicited protection in NHP models of infection and supports further exploration of ADCC in large-scale HIV-1 vaccine trials.
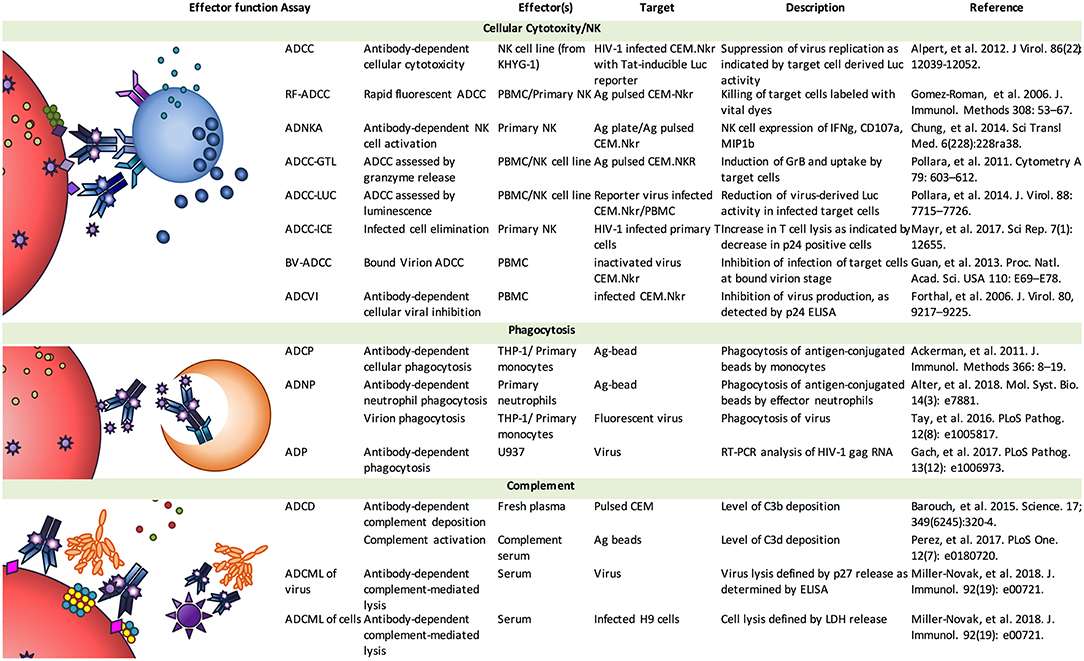
Figure 2. Effector function assays. Antibodies elicit the activities of a diverse array of effector cell types and mechanisms, leading to the generation of a variety of in vitro assays aimed at characterizing the activity of mAb and pAb samples, and defining further insights into basic mechanisms. Image adapted from (7).
Inverse Correlations Between ADCC and Breast Milk Transmission of HIV-1
Recent studies from the Nairobi breastfeeding clinical trial showed that passively transferred ADCC-mediating Abs correlated with favorable infant outcomes (5). In the absence of treatment, approximately 40% of infants exposed to HIV-1 become infected suggesting that there may be factors that protect some infants from infection. Using the RFADCC assay and target cells coated with gp120, Milligan et al. (5) showed a trend for higher passively transferred ADCC activity in infants who don't acquire HIV-1. In the subset of infants who acquired HIV-1 infection, there was a significant correlation between passively acquired ADCC-mediating Ab and the survival of infected infants. By contrast, there was no correlation between infant infection or survival outcomes and passively acquired neutralizing Abs in the same cohort (5, 55). The levels of HIV-specific IgG in the infants were also not correlated with these outcomes, suggesting the effect was specific to ADCC-mediating Abs.
Another study from the Nairobi trial showed that among mothers who would be expected to be highly infectious based on having high viral loads, non-transmitting mothers had higher ADCC titers in their breast milk as compared with transmitting mothers, lending further support that ADCC activity as measured by RFADCC predicts outcome (4). These studies are among the first to show an immune correlate of protection from HIV infection in human studies and suggest the need for a more detailed evaluation of the specificity and function of Abs that could contribute to protection in the setting of mother-to-child transmission.
Functional Cytotoxicity-Based Assays to Detect Vaccine-Induced ADCC Responses in Clinical Trial Settings
The measurement of ADCC activity in a clinical trial setting requires more rigorous standardization than is needed for a research laboratory. This problem was addressed in an ADCC comparative study (56). To date, the most experience using ADCC in a clinical trial setting resides in the NIAID sponsored HIV Vaccine Clinical Trials Network (HVTN) where extensive and specific quality control criteria have been developed and implemented to perform two ADCC assay formats that permit rigorous comparisons among independent human HIV-1 vaccine trials. One assay, denoted as the GranToxiLux (GTL) assay (57), measures the transfer of granzyme from the effector cell to the target cell as a surrogate of NK cell-mediated lysis. The platform utilizes gp120-coated target cells, which have been historically utilized as target cells to detect anti-HIV-1 ADCC responses (9). Moreover, the ADCC responses detected with gp120-coated target cells have been correlated with vaccine-induced Ab responses that can control virus replication (52, 53, 58, 59) and prevent infection (1, 2, 54, 60) in pre-clinical studies as well as with prevention from mother to infant transmission of HIV-1 (4). The assay may represent a surrogate of the CD4 T-cells targeted by ADCC-mediating Abs during virus entry at the time of gp120-CD4 receptor engagement as suggested by the correlation between results generated by this assay and an ADCC assay that utilizes virus-bound target cells (33, 56). Because the recombinant gp120 protein interacts with target cells via CD4, this assay cannot measure Ab responses recognizing the CD4 binding site (CD4bs), but it can detect those directed against CD4 inducible epitopes (CD4i). Moreover, whole PBMC were used as source of the effector population to generate data with GTL assay and area scaling analysis was applied (57) to directly quantify the contributions of NK cells vs. monocytes that recognize the target cells based on the frequency of Granzyme B+ events within singlet and doublet populations representing cells recognized by the NK cells and monocytes, respectively. Such de-convolution of effector cell types demonstrated the correlation between NK cell-mediated ADCC activity and protection in a NHP vaccination/challenge study (1, 57).
Another assay, denoted the Luciferase-based (Luc) ADCC assay (Figure 2), utilizes target cells that are infected with HIV-1 Infectious Molecular Clones (IMC), expressing a Luciferase reporter gene under the control of HIV-1 Tat, which allows for detection of target cell elimination following the infection of cells and virus replication (61). The final read-out is based on the reduction of luciferase signal upon incubation of target and effector cells in presence of a source of Ab. During virus replication, diverse conformations of the HIV-1 envelopes are presented on the membrane of the infected cells including exposure of CD4bs epitopes as well as those represented by closed Env trimers. Of note, for qualification purposes of this assay under Good Clinical Laboratory Procedures (GCLP) guidelines, it was observed that the median level of CD4 downregulation was 56% (range 39–83%) and 69% (range 34–89%) at 48 and 72 h post-infection. The levels of CD4 downregulation and frequency of CD4+ infected cells observed in these target cells were comparable to those observed in primary CD4+ T cells infected with primary HIV-1 isolates reported by different groups (62–64). The 48 and 72 h post-infection times were defined as optimal to allow for maximum virus replication before initiating the incubation of IMC-infected cells with the Ab sample of interest to detect ADCC responses. Under these experimental conditions, the lower level of Nef expression in the CEM.Nkr was compensated by the Vpu in the 2TA reporter IMCs to achieve downregulation of CD4 on the infected cells, as further discussed below. With this assay, it was shown that susceptibility to ADCC does not cluster based on Env subtype, instead, it appears that there is a tiered ranking of ADCC responses for CEM.Nkr infected with different IMCs of HIV-1 (65). Moreover, the tiered ADCC ranking was distinct from the tiered ranking widely used for neutralization of HIV-1 with the Tzm-bl assay, illustrating that these two assays detect significantly different biological responses.
Deciphering ADCC Activity on Primary Infected Cells
One recurrent question is how different ADCC assays recapitulate in vivo lysis of infected cells. Most studies of ADCC have employed various target cell lines with the most frequently used being variants of the CEM.Nkr T-cell line and diverse effector cells such as primary NK cells, primary monocytes, PBMCs, and NK cell lines (Table 1). Some studies have articulated the confounding effects of uninfected bystander cells (66), and the effect of different viral backgrounds that may or may not be fully replication competent or express fully functional Nef and Vpu accessory proteins (16, 66–71). Of note, introduction of the Luciferase reporter into the IMC construct can affect down-regulation of CD4 by Nef (69), but does not impact the Vpu-mediated down-regulation (72). Therefore, the time- and replication-dependent down-regulation of CD4 must be carefully evaluated using these assays as they are also influenced by the type of target cells used, i.e., cell line vs. activated primary CD4 T-cells.
Therefore, while at odds with attributes needed for implementation in large scale evaluation of vaccine-elicited responses, having a fully autologous assay system comprised of primary HIV-1 infected cells and primary effector cells from the same donor may give a more physiologically relevant picture of the true function of Abs with ADCC activity. To this end, an assay using autologous PBMCs infected in vitro with HIV-1 as targets and NK cells purified from these PBMC as effectors has been developed (24). This ADCC system measured the increase in lysis observed in the presence vs. absence of NK cells, and was compared with the NK-mediated ADCC assay using HIV-1 infected CEM.Nkr cells and the NK cell CD107a expression ADCC assay using monoclonal Abs and polyclonal antisera from HIV-1 infected subjects (20). Strikingly, ADCC under these potentially physiologically more relevant conditions (i.e., the primary autologous system) was distinct from that obtained with the assay format using HIV-1 infected CEM.Nkr cells.
Interestingly, non-neutralizing monoclonal Abs directed against the V2 loop, that were previously found to be associated with vaccine-elicited decreased risk of infection in the RV144 vaccine trial, showed highly efficient ADCC activity under these physiologically relevant conditions (24). A recent publication also pointed toward a superiority of ADCC functions for anti-V2 bNAbs compared to other bNAbs when primary immune cells are used (63). These results differ from previously published data obtained using infected cell lines for quantifying ADCC (25), and support the relevance of a fully autologous ADCC system with infected primary target cells and NK effector cells (24, 63). Moreover, the data indicate that V2 epitopes may be particularly accessible on primary infected cells. Notably, CD4 expression was still detected on the primary T-cells infected with primary HIV isolates for 4 days demonstrating a limited down-regulation of CD4 expression compared to its almost complete disappearance observed on CEM.Nkr cell lines infected with the same viruses (66, 73). These differential CD4 expression patterns point to distinct CD4/trimeric Env engagement suggesting that epitopes such as the V2 loop may be more accessible to Abs on infected primary cells than on CEM.Nkr cell lines. The nature of the epitopes of the viral envelope glycoproteins exposed on the surface of infected primary cells requires further investigation.
Further comparison of infected primary cell lysis with other ADCC parameters shows that there is no strong correlation between lysis and binding of Abs to infected primary PBMCs or to CD107a down-regulation (24). Of note, the Abs tested in these and many other similar experiments are variably comprised of recombinant, hybridoma-derived monoclonal IgG, and polyclonal IgG isolated from vaccinated or infected patients. For the latter, Fc domains were therefore naturally induced, which is at variance with the Fc domains of most of the recent bNAbs where the VH, Vκ, and Vλ chains were sequenced and further reconstructed with defined heterogenous heavy chains, often using new proteomics approaches (74, 75). As the combination of the immunoglobulin heavy and light chains of the HIV-specific Abs may play a decisive role in ADCC, increased attention should be paid to the characterization of the Abs Fc domains, including post-translational modifications that may be specific to the native B cell, since they are essential for the induction of ADCC.
Differing Value Propositions Offered by ADCC Assays
Collectively, these studies strongly underscore the need for additional comparative analyses (56) of all currently used ADCC assays, not only to better understand similarities and differences, but also to decipher the relevance of each assay relative to in vivo protection. For example, there is more to be learned by comparative testing in the context of vaccine and passive transfer studies in which efficacy has been observed. Indeed, there is a significant diversity of thought regarding the value of different approaches with respect to ability to support derivation of fundamental insights into host and virus interactions vs. the performance characteristics suitable for use in large-scale vaccine efficacy and immunogenicity studies. This divergence may largely reflect an inherent tradeoff between biological fidelity and practical scalability that poses a challenge to many fields. This spectrum of assays (Figure 2) and spectrum of differing utility is further intensified by conflicting observations among and differences in interpretations of data from clinical and NHP studies [reviewed in (76)]. However, continued investment in comparative and correlates studies promises the possibility of resolution.
Biophysical Assays to Monitor Antibody Functionality in HIV Vaccine Trials
There are substantial challenges inherent to applying assessment of Fc-mediated effector function in cellular assays, particularly across large clinical studies. Cell-based assays of Fc-mediated functions, especially those that use frozen/thawed primary blood cells as targets or effectors, are relatively difficult to reproduce across diverse laboratories. Polymorphisms across effector cell FcRs can influence the outcome of cell-based assays; for example, the high affinity FcγRIIIa V158 allotypic variant is associated with more potent ADCC than the F158 variant with lower affinity for IgG (77, 78). Significant effort has therefore been directed toward developing biophysical assays that serve as useful proxies of Fc-mediated functions. Toward this end, several groups have developed and standardized methods to assess the FcR-binding capacity of antigen-specific Abs present in clinical samples (79–82). It is known that the affinities of the interaction between Ab and FcR are fundamental to Ab effector function, and as such, this parameter has long been a target of numerous successful molecular engineering efforts to increase or ablate effector functions (83, 84). FcR-mediated effector functions in general, and ADCC in particular, require the aggregation of FcR on the effector cell surface by IC. Leveraging the fact that multimeric FcR has a higher affinity for antigen-bound IgG than monomeric FcR, these biophysical approaches, namely FcγR dimer/multimer assays (79, 85), aim to mimic the capacity of a given antibody sample to form ICs that can avidly interact with FcR by assessing their capacity to interact with FcR multimers. The FcγR dimer/multimer assays typically exploit antigen-coated microwells, or multiplexed antigen-conjugated microbeads, which are then probed with immune sera, and bound Abs detected using multimeric FcRs (either dimers or tetramers) (79, 81). These assays have been shown to reliably reproduce the differences apparent among natural IgG types in binding to FcR relevant to Ab effector functions. For example, across a panel of monoclonal Ab variants, despite equivalent opsonization, the receptor binding profiles that drive the differing activities of the IgG subclasses and glycovariants were recapitulated via detection with multimerized FcR (79). Further, the FcγR dimer/multimer assays have been shown to be better correlated with effector function and more accurately predictive of the effector function of polyclonal responses than Ab titer in the context of influenza (81, 86, 87), and HIV (88–91). Further these assays are useful in modeling outcomes in vivo in the context of vaccination and natural infection (1, 60, 91–93). Common polymorphisms of FcRs can be studied in isolation and such analyses are consistent with the known function of such polymorphisms (e.g., the V/F158 polymorphism of FcγRIIIa and H/R131 polymorphism of FcγRIIa) (81, 85).
Biophysical assays of FcR engagement can be more sensitive and reproducible in comparison to cell-based assays of Fc-mediated functions. The simplicity and relatively low cost of biophysical assays mean these assays have become useful in probing the breadth of antigen recognition and breadth of FcRs bound, which may be important aspects of protective ADCC responses (85). In the setting of HIV vaccine response evaluations, recombinant proteins that properly capture antigen conformations relevant during infection (94) will make these assays more biologically relevant. As the field develops standardized panels of Env protein of diverse conformations, the biophysical assays of FcR engagement can be used to screen for breadth of Fc-functional Ab responses induced by vaccination. However, it is already known that biophysical binding assays can correlate well with multiple effector activities, for example, reflecting both the killing and trogocytosis components of the RFADCC assay (23, 85, 91). Lastly, biophysical assays are highly amenable to high-throughput analyses and correlations such as those employed for systems serology (95, 96). These advanced analytical tools offer a highly nuanced view of the differences or similarities between polyclonal responses present among different subjects/cohorts.
Systematic Serology to Asses Other FcR-Mediated Effector Functions
Given this rich history of work developing ADCC assays and observations correlating these activities to outcomes in human and NHP studies, it is perhaps not surprising that effort to characterize this effector function has matured into similar efforts to assess other FcR-mediated effector functions (Figure 2). These activities include Ab-mediated phagocytosis carried out by monocytes, macrophages, and neutrophils (91, 97–101), antibody-dependent trogocytosis mediated by monocytes (23, 57, 102), as well as complement-dependent cytotoxicity (60, 82, 91, 103). Further, these activities extend all the way through to investigations of how Ab opsonization may impact antigen presentation, dendritic cell responses, and shape the development of germinal center reactions. Clearly, there is a wide spectrum of potential means by which Abs can mediate anti-viral activities, and yet, similar challenges confront assays of these activities, and because a number of these activities have also correlated with resistance to viral challenge (46, 60), similar questions as to the relevance of each in vitro assay to the processes that may contribute to in vivo outcomes exist.
In sum, the spectrum of FcγR-mediated effector function is extremely diverse as shown in recent systems serology studies that reveal the high dimensionality of interactions among FcγR classes, FcγR alleles, immunoglobulin classes, immunoglobulin subclasses, immunoglobulin glycosylation, and antigen specificity (96). By contrast, any single functional assay, such as for ADCC, samples only a subset of the many potential interactions. Thus, it is critical to reconcile observations made with this subset of interactions and a biological outcome, which underscores the importance of identifying ADCC assay formats that can be deployed in large scale HIV-1 vaccine trials that produce the essential biological data defining protection or its absence. Fortunately, the first rigorous comparative study of multiple methods to quantify different FcγR mediated effector functions, showed that four different ADCC assay formats produced data that was more highly concordant as compared with the other assays that were distinct from one another and ADCC (56). The clustering of ADCC data in that study strongly suggests the further development of assays that can be deployed in large-scale HIV-1 vaccine trials and natural history studies of Ab-mediated control of HIV-1 infection.
The Complexity of Effector Cells for ADCC: Classical and Memory NK Cells
The classical NK cell subsets engaged by ADCC Ab responses were initially identified among Lineage negative, i.e., CD3-CD19-CD20-CD14-, human cells as those cells that express high level of CD16 receptor (CD16high) and simultaneously express low levels of CD56 (CD56dim) (104). More recently, other phenotypic characteristics of these cellular subsets have been identified such as co-expressing the NKG2D receptor (105) and being more differentiated to express CD57 (106). In the rhesus macaque, a commonly used NHP model for HIV-1 research, most of the NK cell subsets share analogous characteristics with their human counterparts for their ability to serve as ADCC effector cells (107).
In addition to the classic NK cell subsets, more recently NK cells with adaptive features have been described and could play a role as effector cells for ADCC responses. Memory-like NK cells are distinguished from other NK cell subsets by the following criteria: (1) they lack the gamma signaling chain of the FcγR and the Syk adaptor protein; (2) they still require Abs to grant antigen specificity; (3) they proliferate rapidly after antigen signaling; and (4) they are more potent mediators of ADCC (35, 36). These cells, designated as FcγRΔg NK cells, are massively expanded by CMV infection. Recent data now shows that FcγRΔg NK cells are also present in rhesus macaques where they are also expanded by rhesus CMV positivity (108). Further, FcγRΔg NK cells are distributed in peripheral tissues, particularly enriched in the mucosae, and their frequencies are increased in lymphoid tissues in SIV-infected animals. The nature of FcγRIIIa signaling in FcγRΔg NK cells is clearly distinct from other NK cell subsets and is mediated through the CD3ζ chain, accounting, at least partially, for the enhanced functions. Collectively, the available data suggest that FcγRΔg NK cells are strong candidates as effector cells for ADCC in vivo setting the stage to determine how they impact Ab-mediated protection against HIV-1.
Caveats
Although a wealth of data spanning mouse and NHP models to human studies suggests the relevance of Ab effector functions, including ADCC, to anti-viral activity in vivo, it is important to note that many of these studies are often by nature associational and cannot clearly delineate mechanistic relevance. Similarly, NHP studies often rely on small cohorts resulting in limitations in the ability to confidently assess relationships (or lack thereof) between assays and outcomes; there are studies in which ADCC activity but not protective efficacy was observed (109, 110), as well as vaccines and passive antibody transfer experiments that have shown protection not associated with ADCC (111, 112). In rhesus macaques, passive monoclonal Ab transfer experiments have suggested the importance of effector function, but have not allowed conclusive determination of whether non-neutralizing Abs might be sufficient to provide protection, or indicated that enhancing the ADCC activity of a monoclonal Ab can result in improved protection (111, 113–115) as strongly as similar studies conducted in mouse models have (116–118). Further, the ways in which effector cells (119), Ab receptors (120), and Ab types (121, 122) present in model systems differ from those in humans introduce a number of potentially confounding factors. Differences in viruses and mode of challenge further compound challenges in translation. Even among human studies, it is worth noting that ADCC was identified in secondary analysis of a vaccine with a low level of efficacy, and mother to child transmission studies are few in number and need to be repeated in additional cohorts. Thus, it is worth remembering that while use of various assays allows for exciting exploration of relevant aspects of Ab and effector immunology and HIV virology at great resolution and with many nuances, considerable in vivo knowledge gaps remain.
Conclusions
The complex mechanism of ADCC makes its in vitro detection highly challenging. Its mechanistic relationships with in vivo protection are yet to be defined. Nonetheless, numerous assays have been developed to dissect this phenomenon. The data obtained by these assays has contributed to our ever-increasing knowledge on the role of ADCC in HIV/AIDS. Future studies need to investigate other potential ADCC parameters including the HIV epitopes accessible on the target cells; the role of Ab isotype, specific Fc domains, as well as the FcR counterpart expression and function on the effector cells in relevant tissues; and the potential of various effector cells to induce target cell lysis. An increased knowledge of parameters implicated in ADCC functions is a prerequisite for a better understanding of its potential role in vivo. Such information will allow us to gain insight and knowledge for future HIV vaccine development.
Author Contributions
All authors listed have made a substantial, direct and intellectual contribution to the work, and approved it for publication.
Funding
This work was supported in part by the Bill & Melinda Gates Foundation [OPP1146996] and NIH P01 AI120756.
Conflict of Interest Statement
The authors declare that the research was conducted in the absence of any commercial or financial relationships that could be construed as a potential conflict of interest.
References
1. Bradley T, Pollara J, Santra S, Vandergrift N, Pittala S, Bailey-Kellogg C, et al. Pentavalent HIV-1 vaccine protects against simian-human immunodeficiency virus challenge. Nat Commun. (2017) 8:15711. doi: 10.1038/ncomms15711
2. Fouts TR, Bagley K, Prado IJ, Bobb KL, Schwartz JA, Xu R, et al. Balance of cellular and humoral immunity determines the level of protection by HIV vaccines in rhesus macaque models of HIV infection. Proc Natl Acad Sci USA. (2015) 112:E992–9. doi: 10.1073/pnas.1423669112
3. Haynes BF, Gilbert PB, McElrath MJ, Zolla-Pazner S, Tomaras GD, Alam SM, et al. Immune-correlates analysis of an HIV-1 vaccine efficacy trial. N Engl J Med. (2012) 366:1275–86. doi: 10.1056/NEJMoa1113425
4. Mabuka J, Nduati R, Odem-Davis K, Peterson D, Overbaugh J. HIV-specific antibodies capable of ADCC are common in breastmilk and are associated with reduced risk of transmission in women with high viral loads. PLoS Pathog. (2012) 8:e1002739. doi: 10.1371/journal.ppat.1002739
5. Milligan C, Richardson BA, John-Stewart G, Nduati R, Overbaugh J. Passively acquired antibody-dependent cellular cytotoxicity (ADCC) activity in HIV-infected infants is associated with reduced mortality. Cell Host Microbe. (2015) 17:500–6. doi: 10.1016/j.chom.2015.03.002
6. Margolis DM, Koup RA, Ferrari G. HIV antibodies for treatment of HIV infection. Immunol Rev. (2017) 275:313–23. doi: 10.1111/imr.12506
7. Ackerman ME, Alter G. Opportunities to exploit non-neutralizing HIV-specific antibody activity. Curr HIV Res. (2013) 11:365–77. doi: 10.2174/1570162X113116660058
8. Gomez-Roman VR, Florese RH, Patterson LJ, Peng B, Venzon D, Aldrich K, et al. A simplified method for the rapid fluorometric assessment of antibody-dependent cell-mediated cytotoxicity. J Immunol Methods. (2006) 308:53–67. doi: 10.1016/j.jim.2005.09.018
9. Lyerly HK, Reed DL, Matthews TJ, Langlois AJ, Ahearne PA, Petteway SR Jr, et al. Anti-GP 120 antibodies from HIV seropositive individuals mediate broadly reactive anti-HIV ADCC. AIDS Res Hum Retroviruses. (1987) 3:409–22. doi: 10.1089/aid.1987.3.409
10. Weinhold KJ, Lyerly HK, Matthews TJ, Tyler DS, Ahearne PM, Stine KC, et al. Cellular anti-GP120 cytolytic reactivities in HIV-1 seropositive individuals. Lancet. (1988) 1:902–5. doi: 10.1016/S0140-6736(88)91713-8
11. Jewett A, Bonavida B. Peripheral blood monocytes derived from HIV+ individuals mediate antibody-dependent cellular cytotoxicity (ADCC). Clin Immunol Immunopathol. (1990) 54:192–9. doi: 10.1016/0090-1229(90)90081-Z
12. Baldwin GC, Fuller ND, Roberts RL, Ho DD, Golde DW. Granulocyte- and granulocyte-macrophage colony-stimulating factors enhance neutrophil cytotoxicity toward HIV-infected cells. Blood. (1989) 74:1673–7.
13. Kinne TJ, Gupta S. Antibody-dependent cellular cytotoxicity by polymorphonuclear leucocytes in patients with AIDS and AIDS-related complex. J Clin Lab Immunol. (1989) 30:153–6.
14. von Bredow B, Arias JF, Heyer LN, Moldt B, Le K, Robinson JE, et al. Comparison of antibody-dependent cell-mediated cytotoxicity and virus neutralization by HIV-1 Env-specific monoclonal antibodies. J Virol. (2016) 90:6127–39. doi: 10.1128/JVI.00347-16
15. Alpert MD, Heyer LN, Williams DE, Harvey JD, Greenough T, Allhorn M, et al. A novel assay for antibody-dependent cell-mediated cytotoxicity against HIV-1- or SIV-infected cells reveals incomplete overlap with antibodies measured by neutralization and binding assays. J Virol. (2012) 86:12039–52. doi: 10.1128/JVI.01650-12
16. Veillette M, Coutu M, Richard J, Batraville LA, Dagher O, Bernard N, et al. The HIV-1 gp120 CD4-bound conformation is preferentially targeted by antibody-dependent cellular cytotoxicity-mediating antibodies in sera from HIV-1-infected individuals. J Virol. (2015) 89:545–51. doi: 10.1128/JVI.02868-14
17. Ding S, Verly MM, Princiotto A, Melillo B, Moody AM, Bradley T, et al. Short communication: small-molecule CD4 mimetics sensitize HIV-1-infected cells to antibody-dependent cellular cytotoxicity by antibodies elicited by multiple envelope glycoprotein immunogens in nonhuman primates. AIDS Res Hum Retroviruses. (2017) 33:428–31. doi: 10.1089/aid.2016.0246
18. Blumberg RS, Paradis T, Hartshorn KL, Vogt M, Ho DD, Hirsch MS, et al. Antibody-dependent cell-mediated cytotoxicity against cells infected with the human immunodeficiency virus. J Infect Dis. (1987) 156:878–84. doi: 10.1093/infdis/156.6.878
19. Bracher M, Gould HJ, Sutton BJ, Dombrowicz D, Karagiannis SN. Three-colour flow cytometric method to measure antibody-dependent tumour cell killing by cytotoxicity and phagocytosis. J Immunol Methods. (2007) 323:160–71. doi: 10.1016/j.jim.2007.04.009
20. Stratov I, Chung A, Kent SJ. Robust NK cell-mediated human immunodeficiency virus (HIV)-specific antibody-dependent responses in HIV-infected subjects. J Virol. (2008) 82:5450–9. doi: 10.1128/JVI.01952-07
21. Pollara J, Hart L, Brewer F, Pickeral J, Packard BZ, Hoxie JA, et al. High-throughput quantitative analysis of HIV-1 and SIV-specific ADCC-mediating antibody responses. Cytometry A. (2011) 79:603–12. doi: 10.1002/cyto.a.21084
22. Chung AW, Rollman E, Center RJ, Kent SJ, Stratov I. Rapid degranulation of NK cells following activation by HIV-specific antibodies. J Immunol. (2009) 182:1202–10. doi: 10.4049/jimmunol.182.2.1202
23. Kramski M, Schorcht A, Johnston AP, Lichtfuss GF, Jegaskanda S, De Rose R, et al. Role of monocytes in mediating HIV-specific antibody-dependent cellular cytotoxicity. J Immunol Methods. (2012) 384:51–61. doi: 10.1016/j.jim.2012.07.006
24. Mayr LM, Decoville T, Schmidt S, Laumond G, Klingler J, Ducloy C, et al. Non-neutralizing antibodies targeting the V1V2 domain of HIV exhibit strong antibody-dependent cell-mediated cytotoxic activity. Sci Rep. (2017) 7:12655. doi: 10.1038/s41598-017-12883-6
25. Bruel T, Guivel-Benhassine F, Lorin V, Lortat-Jacob H, Baleux F, Bourdic K, et al. Lack of ADCC breadth of human nonneutralizing anti-HIV-1 antibodies. J Virol. (2017) 91:e02440-16. doi: 10.1128/JVI.02440-16
26. Forthal DN, Landucci G, Cole KS, Marthas M, Becerra JC, Van Rompay K. Rhesus macaque polyclonal and monoclonal antibodies inhibit simian immunodeficiency virus in the presence of human or autologous rhesus effector cells. J Virol. (2006) 80:9217–25. doi: 10.1128/JVI.02746-05
27. Tudor D, Bomsel M. The broadly neutralizing HIV-1 IgG 2F5 elicits gp41-specific antibody-dependent cell cytotoxicity in a FcgammaRI-dependent manner. AIDS. (2011) 25:751–9. doi: 10.1097/QAD.0b013e32834507bd
28. Cheng ZJ, Garvin D, Paguio A, Moravec R, Engel L, Fan F, et al. Development of a robust reporter-based ADCC assay with frozen, thaw-and-use cells to measure Fc effector function of therapeutic antibodies. J Immunol Methods. (2014) 414:69–81. doi: 10.1016/j.jim.2014.07.010
29. Tada M, Ishii-Watabe A, Suzuki T, Kawasaki N. Development of a cell-based assay measuring the activation of FcgammaRIIa for the characterization of therapeutic monoclonal antibodies. PLoS ONE. (2014) 9:e95787. doi: 10.1371/journal.pone.0095787
30. Hsieh YT, Aggarwal P, Cirelli D, Gu L, Surowy T, Mozier NM. Characterization of FcgammaRIIIA effector cells used in in vitro ADCC bioassay: comparison of primary NK cells with engineered NK-92 and Jurkat T cells. J Immunol Methods. (2017) 441:56–66. doi: 10.1016/j.jim.2016.12.002
31. Ahmad A, Yao XA, Tanner JE, Cohen E, Menezes J. Surface expression of the HIV-1 envelope proteins in env gene-transfected CD4-positive human T cell clones: characterization and killing by an antibody-dependent cellular cytotoxic mechanism. J Acquir Immune Defic Syndr. (1994) 7:789–98.
32. Lyerly HK, Matthews TJ, Langlois AJ, Bolognesi DP, Weinhold KJ. Human T-cell lymphotropic virus IIIB glycoprotein (gp120) bound to CD4 determinants on normal lymphocytes and expressed by infected cells serves as target for immune attack. Proc Natl Acad Sci USA. (1987) 84:4601–5. doi: 10.1073/pnas.84.13.4601
33. Guan Y, Pazgier M, Sajadi MM, Kamin-Lewis R, Al-Darmarki S, Flinko R, et al. Diverse specificity and effector function among human antibodies to HIV-1 envelope glycoprotein epitopes exposed by CD4 binding. Proc Natl Acad Sci USA. (2013) 110:E69–78. doi: 10.1073/pnas.1217609110
34. Sung JA, Pickeral J, Liu L, Stanfield-Oakley SA, Lam CY, Garrido C, et al. Dual-affinity re-targeting proteins direct T cell-mediated cytolysis of latently HIV-infected cells. J Clin Invest. (2015) 125:4077–90. doi: 10.1172/JCI82314
35. Hwang I, Zhang T, Scott JM, Kim AR, Lee T, Kakarla T, et al. Identification of human NK cells that are deficient for signaling adaptor FcRgamma and specialized for antibody-dependent immune functions. Int Immunol. (2012) 24:793–802. doi: 10.1093/intimm/dxs080
36. Lee J, Zhang T, Hwang I, Kim A, Nitschke L, Kim M, et al. Epigenetic modification and antibody-dependent expansion of memory-like NK cells in human cytomegalovirus-infected individuals. Immunity. (2015) 42:431–42. doi: 10.1016/j.immuni.2015.02.013
37. Hayes JM, Wormald MR, Rudd PM, Davey GP. Fc gamma receptors: glycobiology and therapeutic prospects. J Inflamm Res. (2016) 9:209–19. doi: 10.2147/JIR.S121233
38. Nimmerjahn F, Gordan S, Lux A. FcgammaR dependent mechanisms of cytotoxic, agonistic, and neutralizing antibody activities. Trends Immunol. (2015) 36:325–36. doi: 10.1016/j.it.2015.04.005
39. Nimmerjahn F, Ravetch JV. Fcgamma receptors as regulators of immune responses. Nat Rev Immunol. (2008) 8:34–47. doi: 10.1038/nri2206
40. Carrega P, Ferlazzo G. Natural killer cell distribution and trafficking in human tissues. Front Immunol. (2012) 3:347. doi: 10.3389/fimmu.2012.00347
41. Sips M, Krykbaeva M, Diefenbach TJ, Ghebremichael M, Bowman BA, Dugast AS, et al. Fc receptor-mediated phagocytosis in tissues as a potent mechanism for preventive and therapeutic HIV vaccine strategies. Mucosal Immunol. (2016) 9:1584–95. doi: 10.1038/mi.2016.12
42. Tuijnman WB, Van Wichen DF, Schuurman HJ. Tissue distribution of human IgG Fc receptors CD16, CD32 and CD64: an immunohistochemical study. APMIS. (1993) 101:319–29. doi: 10.1111/j.1699-0463.1993.tb00117.x
43. Nikolova EB, Russell MW. Dual function of human IgA antibodies: inhibition of phagocytosis in circulating neutrophils and enhancement of responses in IL-8-stimulated cells. J Leukoc Biol. (1995) 57:875–82. doi: 10.1002/jlb.57.6.875
44. Wilton JM. Suppression by IgA of IgG-mediated phagocytosis by human polymorphonuclear leucocytes. Clin Exp Immunol. (1978) 34:423–8.
45. Pasquier B, Launay P, Kanamaru Y, Moura IC, Pfirsch S, Ruffie C, et al. Identification of FcalphaRI as an inhibitory receptor that controls inflammation: dual role of FcRgamma ITAM. Immunity. (2005) 22:31–42. doi: 10.1016/S1074-7613(04)00377-2
46. Ackerman ME, Das J, Pittala S, Broge T, Linde C, Suscovich TJ, et al. Route of immunization defines multiple mechanisms of vaccine-mediated protection against SI. Nat Med. (2018) 24:1590–8. doi: 10.1038/s41591-018-0161-0
47. Black KP, Cummins JE Jr, Jackson S. Serum and secretory IgA from HIV-infected individuals mediate antibody-dependent cellular cytotoxicity. Clin Immunol Immunopathol. (1996) 81:182–90. doi: 10.1006/clin.1996.0175
48. Duchemin M, Khamassi M, Xu L, Tudor D, Bomsel M. IgA targeting human immunodeficiency virus-1 envelope gp41 triggers antibody-dependent cellular cytotoxicity cross-clade and cooperates with gp41-specific IgG to increase cell lysis. Front Immunol. (2018) 9:244. doi: 10.3389/fimmu.2018.00244
49. Ruiz MJ, Ghiglione Y, Falivene J, Laufer N, Holgado MP, Socias ME, et al. Env-specific IgA from viremic HIV-infected subjects compromises antibody-dependent cellular cytotoxicity. J Virol. (2016) 90:670–81. doi: 10.1128/JVI.02363-15
50. Tomaras GD, Ferrari G, Shen X, Alam SM, Liao HX, Pollara J, et al. Vaccine-induced plasma IgA specific for the C1 region of the HIV-1 envelope blocks binding and effector function of IgG. Proc Natl Acad Sci USA. (2013) 110:9019–24. doi: 10.1073/pnas.1301456110
51. Bomsel M, Tudor D, Drillet AS, Alfsen A, Ganor Y, Roger MG, et al. Immunization with HIV-1 gp41 subunit virosomes induces mucosal antibodies protecting nonhuman primates against vaginal SHIV challenges. Immunity. (2011) 34:269–80. doi: 10.1016/j.immuni.2011.01.015
52. Gomez-Roman VR, Patterson LJ, Venzon D, Liewehr D, Aldrich K, Florese R, et al. Vaccine-elicited antibodies mediate antibody-dependent cellular cytotoxicity correlated with significantly reduced acute viremia in rhesus macaques challenged with SIVmac251. J Immunol. (2005) 174:2185–9. doi: 10.4049/jimmunol.174.4.2185
53. Patterson LJ, Beal J, Demberg T, Florese RH, Malkevich N, Venzon D, et al. Replicating adenovirus HIV/SIV recombinant priming alone or in combination with a gp140 protein boost results in significant control of viremia following a SHIV89.6P challenge in Mamu-A*01 negative rhesus macaques. Virology. (2008) 374:322–37. doi: 10.1016/j.virol.2007.12.037
54. Xiao P, Patterson LJ, Kuate S, Brocca-Cofano E, Thomas MA, Venzon D, et al. Replicating adenovirus-simian immunodeficiency virus (SIV) recombinant priming and envelope protein boosting elicits localized, mucosal IgA immunity in rhesus macaques correlated with delayed acquisition following a repeated low-dose rectal SIV(mac251) challenge. J Virol. (2012) 86:4644–57. doi: 10.1128/JVI.06812-11
55. Lynch JB, Nduati R, Blish CA, Richardson BA, Mabuka JM, Jalalian-Lechak Z, et al. The breadth and potency of passively acquired human immunodeficiency virus type 1-specific neutralizing antibodies does not correlate with risk of infant infection. J Virol. (2011) 85:5252–61. doi: 10.1128/JVI.02216-10
56. Huang Y, Ferrari G, Alter G, Forthal DN, Kappes JC, Lewis GK, et al. Diversity of antiviral IgG effector activities observed in HIV-infected and vaccinated subjects. J Immunol. (2016) 197:4603–12. doi: 10.4049/jimmunol.1601197
57. Pollara J, Orlandi C, Beck C, Edwards RW, Hu Y, Liu S, et al. Application of area scaling analysis to identify natural killer cell and monocyte involvement in the GranToxiLux antibody dependent cell-mediated cytotoxicity assay. Cytometry A. (2018) 93:436–47. doi: 10.1002/cyto.a.23348
58. Thomas MA, Tuero I, Demberg T, Vargas-Inchaustegui DA, Musich T, Xiao P, et al. HIV-1 CD4-induced (CD4i) gp120 epitope vaccines promote B and T-cell responses that contribute to reduced viral loads in rhesus macaques. Virology. (2014) 471–3:81–92. doi: 10.1016/j.virol.2014.10.001
59. Gomez-Roman VR, Florese RH, Peng B, Montefiori DC, Kalyanaraman VS, Venzon D, et al. An adenovirus-based HIV subtype B prime/boost vaccine regimen elicits antibodies mediating broad antibody-dependent cellular cytotoxicity against non-subtype B HIV strains. J Acquir Immune Defic Syndr. (2006) 43:270–7. doi: 10.1097/01.qai.0000230318.40170.60
60. Barouch DH, Alter G, Broge T, Linde C, Ackerman ME, Brown EP, et al. HIV-1 vaccines. Protective efficacy of adenovirus/protein vaccines against SIV challenges in rhesus monkeys. Science. (2015) 349:320–4. doi: 10.1126/science.aab3886
61. Pollara J, Bonsignori M, Moody MA, Liu P, Alam SM, Hwang KK, et al. HIV-1 vaccine-induced C1 and V2 Env-specific antibodies synergize for increased antiviral activities. J Virol. (2014) 88:7715–26. doi: 10.1128/JVI.00156-14
62. Grau-Exposito J, Serra-Peinado C, Miguel L, Navarro J, Curran A, Burgos J, et al. A novel single-cell FISH-flow assay identifies effector memory CD4(+) T cells as a Major Niche for HIV-1 transcription in HIV-infected patients. MBio. (2017) 8:e00876-17. doi: 10.1128/mBio.00876-17
63. Mujib S, Liu J, Rahman A, Schwartz JA, Bonner P, Yue FY, et al. Comprehensive cross-clade characterization of antibody-mediated recognition, complement-mediated lysis and cell-mediated cytotoxicity of HIV-1 envelope specific antibodies towards the eradication of the HIV-1 reservoir. J Virol. (2017) 91:e00634-17. doi: 10.1128/JVI.00634-17
64. Lee WS, Prevost J, Richard J, van der Sluis RM, Lewin SR, Pazgier M, et al. CD4- and time-dependent susceptibility of HIV-1-infected cells to ADCC. J Virol. (2019). doi: 10.1128/JVI.01901-18. [Epub ahead of print].
65. Stanfield-Oakley S, Patel K, deCamp AC, LaBranche C, Ochsenbauer C, Greene K, et al. Identification of a Panel of IMC to define breadth and potency of ADCC responses. In: Keystone Symposia X8. Olympic Valley, CA (2016).
66. Richard J, Prevost J, Baxter AE, von Bredow B, Ding S, Medjahed H, et al. Uninfected bystander cells impact the measurement of HIV-specific antibody-dependent cellular cytotoxicity responses. MBio. (2018) 9:e00358-18. doi: 10.1128/mBio.00358-18
67. Veillette M, Desormeaux A, Medjahed H, Gharsallah NE, Coutu M, Baalwa J, et al. Interaction with cellular CD4 exposes HIV-1 envelope epitopes targeted by antibody-dependent cell-mediated cytotoxicity. J Virol. (2014) 88:2633–44. doi: 10.1128/JVI.03230-13
68. Alsahafi N, Ding S, Richard J, Markle T, Brassard N, Walker B, et al. Nef proteins from HIV-1 elite controllers are inefficient at preventing antibody-dependent cellular cytotoxicity. J Virol. (2015) 90:2993–3002. doi: 10.1128/JVI.02973-15
69. Prevost J, Richard J, Medjahed H, Alexander A, Jones J, Kappes JC, et al. Incomplete downregulation of CD4 expression affects HIV-1 env conformation and antibody-dependent cellular cytotoxicity responses. J Virol. (2018) 92:e00484-18. doi: 10.1128/JVI.00484-18
70. Alsahafi N, Richard J, Prevost J, Coutu M, Brassard N, Parsons MS, et al. Impaired downregulation of NKG2D ligands by Nef proteins from elite controllers sensitizes HIV-1-infected cells to antibody-dependent cellular cytotoxicity. J Virol. (2017) 91:e00109-17. doi: 10.1128/JVI.00109-17
71. Richard J, Prevost J, Alsahafi N, Ding S, Finzi A. Impact of HIV-1 envelope conformation on ADCC responses. Trends Microbiol. (2018) 26:253–65. doi: 10.1016/j.tim.2017.10.007
72. Alberti MO, Jones JJ, Miglietta R, Ding H, Bakshi RK, Edmonds TG, et al. Optimized replicating renilla luciferase reporter HIV-1 utilizing novel internal ribosome entry site elements for native Nef expression and function. AIDS Res Hum Retroviruses. (2015) 31:1278–96. doi: 10.1089/aid.2015.0074
73. Pham TN, Lukhele S, Hajjar F, Routy JP, Cohen EA. HIV Nef and Vpu protect HIV-infected CD4+ T cells from antibody-mediated cell lysis through down-modulation of CD4 and BST2. Retrovirology. (2014) 11:15. doi: 10.1186/1742-4690-11-15
74. Cheung WC, Beausoleil SA, Zhang X, Sato S, Schieferl SM, Wieler JS, et al. A proteomics approach for the identification and cloning of monoclonal antibodies from serum. Nat Biotechnol. (2012) 30:447–52. doi: 10.1038/nbt.2167
75. Scheid JF, Mouquet H, Feldhahn N, Seaman MS, Velinzon K, Pietzsch J, et al. Broad diversity of neutralizing antibodies isolated from memory B cells in HIV-infected individuals. Nature. (2009) 458:636–40. doi: 10.1038/nature07930
76. Forthal DN, Finzi A. Antibody-Dependent Cellular Cytotoxicity (ADCC) in HIV Infection. AIDS. (2018) 32:2439–51. doi: 10.1097/QAD.0000000000002011
77. S. Dall'Ozzo, Tartas S, Paintaud G, Cartron G, Colombat P, Bardos P, et al. Rituximab-dependent cytotoxicity by natural killer cells: influence of FCGR3A polymorphism on the concentration-effect relationship. Cancer Res. (2004) 64:4664–9. doi: 10.1158/0008-5472.CAN-03-2862
78. Wu J, Edberg JC, Redecha PB, Bansal V, Guyre PM, Coleman K, et al. A novel polymorphism of FcgammaRIIIa (CD16) alters receptor function and predisposes to autoimmune disease. J Clin Invest. (1997) 100:1059–70. doi: 10.1172/JCI119616
79. Brown EP, Dowell KG, Boesch AW, Normandin E, Mahan AE, Chu T, et al. Multiplexed Fc array for evaluation of antigen-specific antibody effector profiles. J Immunol Methods. (2017) 443:33–44. doi: 10.1016/j.jim.2017.01.010
80. Brown EP, Weiner JA, Lin S, Natarajan H, Normandin E, Barouch DH, et al. Optimization and qualification of an Fc Array assay for assessments of antibodies against HIV-1/SIV. J Immunol Methods. (2018) 455:24–33. doi: 10.1016/j.jim.2018.01.013
81. Wines BD, Vanderven HA, Esparon SE, Kristensen AB, Kent SJ, Hogarth PM. Dimeric FcgammaR ectodomains as probes of the Fc receptor function of anti-influenza virus IgG. J Immunol. (2016) 197:1507–16. doi: 10.4049/jimmunol.1502551
82. Perez LG, Martinez DR, deCamp AC, Pinter A, Berman PW, Francis D, et al. V1V2-specific complement activating serum IgG as a correlate of reduced HIV-1 infection risk in RV144. PLoS ONE. (2017) 12:e0180720. doi: 10.1371/journal.pone.0180720
83. Shields RL, Namenuk AK, Hong K, Meng YG, Rae J, Briggs J, et al. High resolution mapping of the binding site on human IgG1 for Fc gamma RI, Fc gamma RII, Fc gamma RIII, and FcRn and design of IgG1 variants with improved binding to the Fc gamma R. J Biol Chem. (2001) 276:6591–604. doi: 10.1074/jbc.M009483200
84. Lazar GA, Dang W, Karki S, Vafa O, Peng JS, Hyun L, et al. Engineered antibody Fc variants with enhanced effector function. Proc Natl Acad Sci USA. (2006) 103:4005–10. doi: 10.1073/pnas.0508123103
85. McLean MR, Madhavi V, Wines BD, Hogarth PM, Chung AW, Kent SJ. Dimeric Fcgamma receptor enzyme-linked immunosorbent assay to study HIV-specific antibodies: a new look into breadth of Fcgamma receptor antibodies induced by the RV144 vaccine trial. J Immunol. (2017) 199:816–26. doi: 10.4049/jimmunol.1602161
86. Kristensen AB, Lay WN, Ana-Sosa-Batiz F, Vanderven HA, Madhavi V, Laurie KL, et al. Antibody responses with Fc-mediated functions after vaccination of HIV-infected subjects with trivalent influenza vaccine. J Virol. (2016) 90:5724–34. doi: 10.1128/JVI.00285-16
87. Vanderven HA, Ana-Sosa-Batiz F, Jegaskanda S, Rockman S, Laurie K, Barr I, et al. What lies beneath: antibody dependent natural killer cell activation by antibodies to internal influenza virus proteins. EBio Med. (2016) 8:277–90. doi: 10.1016/j.ebiom.2016.04.029
88. Kratochvil S, McKay PF, Kopycinski JT, Bishop C, Hayes PJ, Muir L, et al. A phase 1 human immunodeficiency virus vaccine trial for cross-profiling the kinetics of serum and mucosal antibody responses to CN54gp140 modulated by two homologous prime-boost vaccine regimens. Front Immunol. (2017) 8:595. doi: 10.3389/fimmu.2017.00595
89. Madhavi V, Wines BD, Amin J, Emery S, Group ES, Lopez E, et al. HIV-1 Env- and Vpu-specific antibody-dependent cellular cytotoxicity responses associated with elite control of HIV. J Virol. (2017) 91:e00700-17. doi: 10.1128/JVI.00700-17
90. Wines BD, Billings H, McLean MR, Kent SJ, Hogarth PM. Antibody functional assays as measures of Fc receptor-mediated immunity to HIV - new technologies and their impact on the HIV vaccine field. Curr HIV Res. (2017) 15:202215. doi: 10.2174/1570162X15666170320112247
91. Richardson SI, Chung AW, Natarajan H, Mabvakure B, Mkhize NN, Garrett N, et al. HIV-specific Fc effector function early in infection predicts the development of broadly neutralizing antibodies. PLoS Pathog. (2018) 14:e1006987. doi: 10.1371/journal.ppat.1006987
92. Vaccari M, Gordon SN, Fourati S, Schifanella L, Liyanage NP, Cameron M, et al. Adjuvant-dependent innate and adaptive immune signatures of risk of SIVmac251 acquisition. Nat Med. (2016) 22:762–70. doi: 10.1038/nm.4105
93. Vanderven HA, Jegaskanda S, Wheatley AK, Kent SJ. Antibody-dependent cellular cytotoxicity and influenza virus. Curr Opin Virol. (2017) 22:89–96. doi: 10.1016/j.coviro.2016.12.002
94. Ren Y, Korom M, Truong R, Chan D, Huang SH, Kovacs CC, et al. Susceptibility to neutralization by broadly neutralizing antibodies generally correlates with infected cell binding for a panel of clade B HIV reactivated from latent reservoirs. J Virol. (2018) 92:e00895-18. doi: 10.1101/330894
95. Chung AW, Kumar MP, Arnold KB, Yu WH, Schoen MK, Dunphy LJ, et al. Dissecting polyclonal vaccine-induced humoral immunity against HIV using systems serology. Cell. (2015) 163:988–98. doi: 10.1016/j.cell.2015.10.027
96. Ackerman ME, Barouch DH, Alter G. Systems serology for evaluation of HIV vaccine trials. Immunol Rev. (2017) 275:262–70. doi: 10.1111/imr.12503
97. Ackerman ME, Moldt B, Wyatt RT, Dugast AS, McAndrew E, Tsoukas S, et al. A robust, high-throughput assay to determine the phagocytic activity of clinical antibody samples. J Immunol Methods. (2011) 366:8–19. doi: 10.1016/j.jim.2010.12.016
98. Gach JS, Bouzin M, Wong MP, Chromikova V, Gorlani A, Yu KT, et al. Human immunodeficiency virus type-1 (HIV-1) evades antibody-dependent phagocytosis. PLoS Pathog. (2017) 13:e1006793. doi: 10.1371/journal.ppat.1006793
99. Tay MZ, Liu P, Williams LD, McRaven MD, Sawant S, Gurley TC, et al. Antibody-mediated internalization of infectious HIV-1 virions differs among antibody isotypes and subclasses. PLoS Pathog. (2016) 12:e1005817. doi: 10.1371/journal.ppat.1005817
100. Ana-Sosa-Batiz F, Johnston AP, Liu H, Center RJ, Rerks-Ngarm S, Pitisuttithum P, et al. HIV-specific antibody-dependent phagocytosis matures during HIV infection. Immunol Cell Biol. (2014) 92:679–87. doi: 10.1038/icb.2014.42
101. Worley MJ, Fei K, Lopez-Denman AJ, Kelleher AD, Kent SJ, Chung AW. Neutrophils mediate HIV-specific antibody-dependent phagocytosis and ADCC. J Immunol Methods. (2018) 457:41–52. doi: 10.1016/j.jim.2018.03.007
102. Richardson SI, Crowther C, Mkhize NN, Morris L. Measuring the ability of HIV-specific antibodies to mediate trogocytosis. J Immunol Methods. (2018) 463:71–83. doi: 10.1016/j.jim.2018.09.009
103. Miller-Novak LK, Das J, Musich TA, Demberg T, Weiner JA, Venzon DJ, et al. Analysis of complement-mediated lysis of Simian Immunodeficiency Virus (SIV) and SIV-infected cells reveals sex differences in vaccine-induced immune responses in rhesus macaques. J Virol. (2018) 92:e00721-18. doi: 10.1128/JVI.00721-18
104. Cooper MA, Fehniger TA, Turner SC, Chen KS, Ghaheri BA, Ghayur T, et al. Human natural killer cells: a unique innate immunoregulatory role for the CD56(bright) subset. Blood. (2001) 97:3146–51. doi: 10.1182/blood.V97.10.3146
105. Parsons MS, Richard J, Lee WS, Vanderven H, Grant MD, Finzi A, et al. NKG2D acts as a co-receptor for natural killer cell-mediated anti-HIV-1 antibody-dependent cellular cytotoxicity. AIDS Res Hum Retroviruses. (2016) 32:1089–96. doi: 10.1089/aid.2016.0099
106. Gooneratne SL, Center RJ, Kent SJ, Parsons MS. Functional advantage of educated KIR2DL1(+) natural killer cells for anti-HIV-1 antibody-dependent activation. Clin Exp Immunol. (2016) 184:101–9. doi: 10.1111/cei.12752
107. Vargas-Inchaustegui DA, Demberg T, Robert-Guroff M. A CD8alpha(-) subpopulation of macaque circulatory natural killer cells can mediate both antibody-dependent and antibody-independent cytotoxic activities. Immunology. (2011) 134:326–40. doi: 10.1111/j.1365-2567.2011.03493.x
108. Shah SV, Manickam C, Ram DR, Kroll K, Itell H, Permar SR, et al. CMV primes functional alternative signaling in adaptive deltag NK cells but is subverted by lentivirus infection in rhesus macaques. Cell Rep. (2018) 25:2766–74 e3. doi: 10.1016/j.celrep.2018.11.020
109. Dugast AS, Chan Y, Hoffner M, Licht A, Nkolola J, Li H, et al. Lack of protection following passive transfer of polyclonal highly functional low-dose non-neutralizing antibodies. PLoS ONE. (2014) 9:e97229. doi: 10.1371/journal.pone.0097229
110. Florese RH, Van Rompay KK, Aldrich K, Forthal DN, Landucci G, Mahalanabis M, et al. Evaluation of passively transferred, nonneutralizing antibody-dependent cellular cytotoxicity-mediating IgG in protection of neonatal rhesus macaques against oral SIVmac251 challenge. J Immunol. (2006) 177:4028–36. doi: 10.4049/jimmunol.177.6.4028
111. Moldt B, Shibata-Koyama M, Rakasz EG, Schultz N, Kanda Y, Dunlop DC, et al. A nonfucosylated variant of the anti-HIV-1 monoclonal antibody b12 has enhanced FcgammaRIIIa-mediated antiviral activity in vitro but does not improve protection against mucosal SHIV challenge in macaques. J Virol. (2012) 86:6189–96. doi: 10.1128/JVI.00491-12
112. Parsons MS, Lee WS, Kristensen AB, Amarasena T, Khoury G, Wheatley AK, et al. Fc-dependent functions are redundant to efficacy of anti-HIV antibody PGT121 in macaques. J Clin Invest. (2019) 129:182–91. doi: 10.1172/JCI122466
113. Hessell AJ, Hangartner L, Hunter M, Havenith CE, Beurskens FJ, Bakker JM, et al. Fc receptor but not complement binding is important in antibody protection against HIV. Nature. (2007) 449:101–4. doi: 10.1038/nature06106
114. Astronomo RD, Santra S, Ballweber-Fleming L, Westerberg KG, Mach L, Hensley-McBain T, et al. Neutralization takes precedence over IgG or IgA isotype-related functions in mucosal HIV-1 antibody-mediated protection. EBioMedicine. (2016) 14:97–111. doi: 10.1016/j.ebiom.2016.11.024
115. Santra S, Tomaras GD, Warrier R, Nicely NI, Liao HX, Pollara J, et al. Human non-neutralizing HIV-1 envelope monoclonal antibodies limit the number of founder viruses during SHIV mucosal infection in rhesus macaques. PLoS Pathog. (2015) 11:e1005042. doi: 10.1371/journal.ppat.1005042
116. Horwitz JA, Bar-On Y, Lu CL, Fera D, Lockhart AK, Lorenzi CC, et al. Non-neutralizing antibodies alter the course of HIV-1 infection in vivo. Cell. (2017) 170:637–648 e10. doi: 10.1016/j.cell.2017.06.048
117. Bournazos S, Klein F, Pietzsch J, Seaman MS, Nussenzweig MC, Ravetch JV. Broadly neutralizing anti-HIV-1 antibodies require Fc effector functions for in vivo activity. Cell. (2014) 158:1243–53. doi: 10.1016/j.cell.2014.08.023
118. Halper-Stromberg A, Lu CL, Klein F, Horwitz JA, Bournazos S, Nogueira L, et al. Broadly neutralizing antibodies and viral inducers decrease rebound from HIV-1 latent reservoirs in humanized mice. Cell. (2014) 158:989–99. doi: 10.1016/j.cell.2014.07.043
119. Trist HM, Tan PS, Wines BD, Ramsland PA, Orlowski E, Stubbs J, et al. Polymorphisms and interspecies differences of the activating and inhibitory FcgammaRII of Macaca nemestrina influence the binding of human IgG subclasses. J Immunol. (2014) 192:792–803. doi: 10.4049/jimmunol.1301554
120. Chan YN, Boesch AW, Osei-Owusu NY, Emileh A, Crowley AR, Cocklin SL, et al. IgG binding characteristics of rhesus macaque FcgammaR. J Immunol. (2016) 197:2936–47. doi: 10.4049/jimmunol.1502252
121. Boesch AW, Osei-Owusu NY, Crowley AR, Chu TH, Chan YN, Weiner JA, et al. Biophysical and functional characterization of rhesus macaque IgG subclasses. Front Immunol. (2016) 7:589. doi: 10.3389/fimmu.2016.00589
Keywords: HIV—human immunodeficiency virus, antibodies, effector function, ADCC—antibody dependent cellular cytotoxicity, Fc receptor
Citation: Lewis GK, Ackerman ME, Scarlatti G, Moog C, Robert-Guroff M, Kent SJ, Overbaugh J, Reeves RK, Ferrari G and Thyagarajan B (2019) Knowns and Unknowns of Assaying Antibody-Dependent Cell-Mediated Cytotoxicity Against HIV-1. Front. Immunol. 10:1025. doi: 10.3389/fimmu.2019.01025
Received: 11 October 2018; Accepted: 23 April 2019;
Published: 10 May 2019.
Edited by:
Aurelio Cafaro, Istituto Superiore di Sanità (ISS), ItalyReviewed by:
Catarina E. Hioe, Icahn School of Medicine at Mount Sinai, United StatesMorgane Bomsel, Institut National de la Santé et de la Recherche Médicale (INSERM), France
Copyright © 2019 Lewis, Ackerman, Scarlatti, Moog, Robert-Guroff, Kent, Overbaugh, Reeves, Ferrari and Thyagarajan. This is an open-access article distributed under the terms of the Creative Commons Attribution License (CC BY). The use, distribution or reproduction in other forums is permitted, provided the original author(s) and the copyright owner(s) are credited and that the original publication in this journal is cited, in accordance with accepted academic practice. No use, distribution or reproduction is permitted which does not comply with these terms.
*Correspondence: Margaret E. Ackerman, margaret.e.ackerman@dartmouth.edu