- Research Department, Sidra Medicine, Doha, Qatar
Vitamin D is a fat soluble secosteroid that is primarily synthesized in the skin upon exposure to Ultraviolet B (UVB) sun rays. Vitamin D is essential for the growth and development of bones and helps in reducing inflammation by strengthening muscles and the immune system. Despite the endless supply of sunlight in the Gulf Cooperation Council (GCC) countries which includes United Arab Emirates, Qatar, Kuwait, Bahrain, Saudi Arabia, and Oman, Vitamin D deficiency in the (GCC) general population at various age groups remains alarmingly high. In parallel runs the increasing prevalence of acute and chronic illnesses including, autoimmune diseases, cancer, type 1 diabetes mellitus, cardiovascular disease and Inflammatory bowel disease in the adult as well as the pediatric population of these countries. The exact association between Vitamin D deficiency and chronic disease conditions remains unclear; however, studies have focused on the mechanism of Vitamin D regulation by assessing the role of the Vitamin D associated genes/proteins such as VDR (Vitamin D receptor), VDBP (Vitamin D Binding protein), CYP27B1 as these are integral parts of the Vitamin D signaling pathway. VDR is known to regulate the expression of more than 200 genes across a wide array of tissues in the human body and may play a role in controlling the Vitamin D levels. Moreover, reduced Vitamin D level and downregulation of VDR have been linked to gut dysbiosis, highlighting an intriguing role for the gut microbiome in the Vitamin D metabolism. However, this role is not fully described yet. In this review, we aim to expand our understanding of the causes of Vitamin D deficiency in the GCC countries and explore the potential relationship between the genetic predisposition, Vitamin D levels, immune system and the gut microbiome composition. Trying to unravel this complex interaction may aid in understanding the mechanism by which Vitamin D contributes to various disease conditions and will pave the way toward new therapeutics treatments for Vitamin D deficiency and its associated outcomes.
Introduction
Vitamin D is well-known for its many health benefits. The role of Vitamin D in the elimination of rickets remains one of the most notable discoveries in medicine (1). Calcium and phosphate are two minerals that are required for normal bone formation. Upon demand, Vitamin D stimulates the intestines, bones, and kidneys to maintain calcium and phosphorus levels in the blood, and thus promotes the mineralization of the bone matrix and osteoclasts differentiation (2). Hypovitaminosis D or severe Vitamin D deficiency results in poor mineralization and bone loss, leading to osteoporosis, fractures, muscle weakness, and frank hypocalcemia (3). Vitamin D mediates its biological function via metabolizing into its active steroid form 1α,25-dihydroxyvitamin D3 [1α,25(OH)2D3 or simply 1,25(OH)2D], often referred to as a hormone, influencing many genes across various tissues in the human body, such as kidneys (4–6), intestines (7), bones (8–11), as well as cancer and immune cells (12–14).
Vitamin D is also known to interact with cells from both the innate and adaptive immune system, where it plays an important role in antigen presentation, immune regulation and antibacterial response (15). Historical Vitamin D supplementation has been used to treat lupus and mycobacterial infections such as tuberculosis and leprosy (16, 17). Studies examining the immunomodulatory properties of Vitamin D have linked its deficiency to a higher incidence of autoimmune diseases, such as type I diabetes (T1D), multiple sclerosis (MS), systemic lupus erythematosus (SLE), rheumatoid arthritis (RA), and inflammatory bowel disease (IBD) and also to an increased risk of developing various types of cancers including breast, ovarian, colon, and prostate cancer (18–21). Vitamin D is also a potent effector of vascular endothelial cells and thus has a role in cardio-protection (22), as it regulates blood pressure by stimulating the renin angiotensin system, vascular calcification and smooth muscle cell proliferation (23). Hypovitaminosis D is directly linked to the development of hypertension, and a reduction in blood pressure has been noted to be a consequence of Vitamin D supplementation (24, 25). Evidence also supports the role of Vitamin D in neuroprotection, brain development and maintenance of cognitive functions via immunomodulation, neuronal calcium regulation, antioxidative mechanisms, enhanced nerve conduction, and detoxification mechanisms (26). There have been some reports linking decreased Vitamin D levels with depression (27), cognitive delays and an increased risk of Alzheimer's disease (28). Cerebrovascular events such as the risk of vessel thrombosis, cerebrovascular infracts and strokes have also been associated with deficiency of Vitamin D (29).
Insufficient levels of Vitamin D may also be a contributing factor for other abnormalities such as poor diet, short stature, liver disease, and diabetes (30–32). The role of Vitamin D during pregnancy is also of great consideration since maternal nutritional status determines the health of fetus and newborn. Maternal Vitamin D deficiency has been associated with increased risk of preeclampsia, calcium malabsorption, bone loss, and other myopathies (33). It is suggested that the development of the fetus in a state of hypovitaminosis D can have significant impact on innate immune functions. In vitro study conducted with Monocytes cultured in Vitamin D deficient plasma, showed significantly decreased in a TLR-dependent expression of cathelicidin compared to the control (34) Vitamin D status in the cord blood was found to be associated with the risk of lower respiratory tract infection in the first year of life consistent with the in vitro study results (35). Severe deficiency can also contribute toward abnormalities such as small stature for gestational age, neonatal hypocalcemia, hypocalcemic seizures, infantile heart failure, enamel defects, large fontanelle, congenital rickets, among others (36, 37). The role of Vitamin D goes above and beyond the traditionally ascribed ones and its significance in human physiology is undeniable. In the most recent years, several papers have addressed the importance of Vitamin D and its intracellular receptor VDR in regulation of gut hemostasis and immune response (38–41), here we aim to go further and present a comprehensive review examining the epidemic of Vitamin D deficiency in Gulf Cooperation Council (GCC) which is an alliance of six Middle Eastern countries—Saudi Arabia, Qatar, Kuwait, the United Arab Emirates, Bahrain, and Oman. We would also like to study the possible role of specific genes in predisposing the gulf population to Vitamin D deficiency, and how this increasing epidemic leads to disturbed microbial balance in the intestines and manifestation of various immune mediated diseases such as IBD.
Methods Used to Review the Literature in the Field
A comprehensive literature search was carried out in PubMed, ScienceDirect, Google Scholar and SpringerLink databases using keywords like “Vitamin D,” “Human microbiome,” “Vitamin D metabolism,” “Vitamin D and Gut microbiome,” “Vitamin D deficiency in Gulf countries,” “VDR and Immune regulation,” “Immune related disease in Gulf countries,” “25-hydroxy vitamin D.” Only articles published in English and related to the study topic were included in this review. The search was not restricted to the type of study i.e., species, meta-analysis, case-control, randomized control trials, cohort studies, reviews, sample size, or year of publication. Bibliographies and citation of the included reviews were scanned for additional studies that may have been missed by the database searches. The exclusion criteria comprised the following: unpublished data, conference publications, articles available only in the abstract form, and doctoral or master's thesis. Endnote software (Thomas Reuters, Philadelphia, PA) was used to create library and manage the findings of the search as recognized by the above-mentioned strategies. The selected articles were read and organized under the following headings: (1) Vitamin D, importance, metabolism, status and supplementation (2) Prevalence of Vitamin D deficiency or insufficiency and immune related disorders in GCC countries (3). Possible relationship between disturbances of the gut microbiota and/or Vitamin D deficiency, VDR dysfunction, and role of Vitamin D in immune system and related disorders.
Vitamin D Metabolism
In humans, Vitamin D originates from three potential sources: Ultraviolet B (UVB) dependent exogenous synthesis, nutritional sources and supplements (18, 42, 43). However, it is primarily synthesized in the skin by the action of sunlight (UV dependent) as very few naturally occurring food sources have adequate amounts of Vitamin D (18, 42, 43). Dietary sources such as fish, milk, orange juice, and cereals contain one of the two forms, cholecalciferol (Vitamin D3) or ergocalciferol (Vitamin D2) (18, 42, 43). Regardless of the source, the Vitamin D synthesis pathway follows several common steps as detailed in Figure 1.
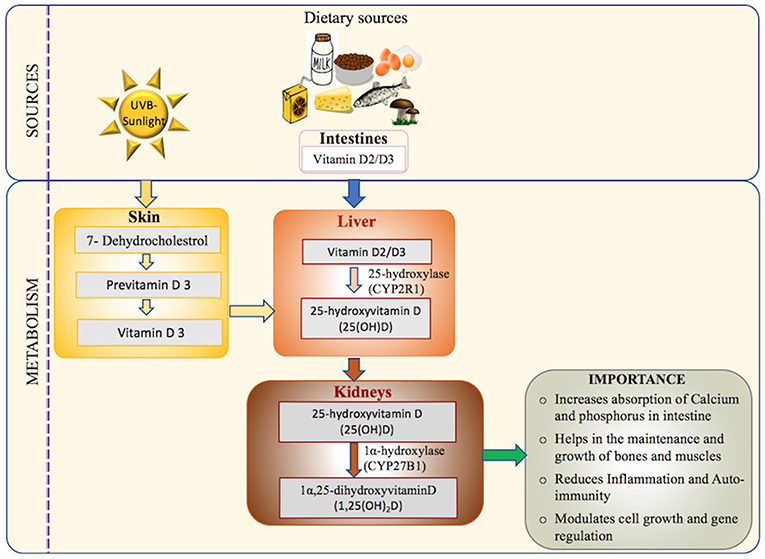
Figure 1. Vitamin D: sources, activation pathway, and importance in the human body: the major source of Vitamin D (UVB-sunlight) and the minor source (Dietary Vitamin D) are transported to liver and metabolized to its main circulating form (25-hydroxyvitamin D) which is measured in the serum in most assays (18, 42, 43). The activated form of Vitamin D is then synthesized in the kidney via hydroxylation to form 1,25-dihydroxyvitamin D, also known as calcitriol (44, 45). Calcitriol has many functions including mineralization of bone matrix, enhancing absorption of calcium and phosphorous from small intestines, reducing autoimmunity and inflammation, and gene regulation (20, 46).
Dermal synthesis starts with the conversion of cutaneously derived cholesterol precursor 7-dehydroxycholestrol to previtamin D3 by the action of UV sunlight (44, 47). Previtamin D undergoes a temperature dependent isomerization to Vitamin D3. Vitamin D-binding protein (VDBP) carries the Vitamin D3 synthesized in the skin and Vitamin D2/Vitamin D3 absorbed via intestine from the dietary sources to the liver (45). In the liver, Vitamin D2/Vitamin D3 is hydrolyzed to 25-hydroxyvitamin D[25(OH)D] by the action of enzyme 25-hydroxylase (CYP2R1) (44). The final step of activation occurs in the kidney with the conversion of 25-hydroxyvitamin D[25(OH)D] to 1,25(OH)2D by the 1α-hydroxylase enzyme (CYP27B1) (48). Finally, 1,25(OH)2D binds to its principal receptor VDR through which it regulates the expression of a large number of genes across various tissues in the human body (46). VDR plays a central role in mediating the biological functions of Vitamin D, via both genomic and non-genomic pathways as elucidated in Figure 2.
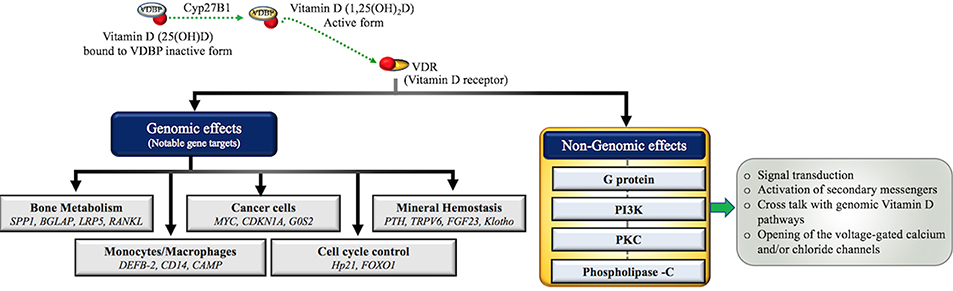
Figure 2. Molecular actions of the Vitamin D/VDR axis. Inactive Vitamin D (25(OH)D) circulates in the blood stream bound to Vitamin D binding protein (VDBP) and undergoes hydroxylation by the renal mitochondrial 1-hydroxylase (CYP27B1) enzyme to convert into active form (1α,25(OH)2D). Active Vitamin D binds to its primary receptor VDR to modulate the expression of more than 200 genes in human body. Some notable ones are grouped into various biological processes including (1) bone metabolism (8–11) (2) immune cell regulation (14, 49) (3) cancer (12) (4) cell cycle (8) (5) metabolism (4–7) demonstrating the wide range of VDR dependent genomic actions. Non-genomic actions include the activation of one or more intracellular signaling molecules, such as G protein-coupled receptors (G-protein), Protein kinase C (PKC), phosphatidylinositol-3′-kinase (PI3K), and Phospholipase C (PLC) resulting in opening of the voltage-gated channels, generation of the specific second messengers and cross talk with genomic pathways (8).
Vitamin D Status and Dosage
Levels of Vitamin D are measured in the serum as 25(OH)D, reflecting the Vitamin D status. The results of this blood test help with the clinical decision as to whether to take Vitamin D supplement or expose the skin to the sun. However, there is no international guidelines for reading the Vitamin D levels, as different organizations interpret the levels differently. Levels indicated as normal by one method may be interpreted differently by the other. The Vitamin D Council guidelines recommends 40–80 ng/ml as the ideal level, with levels of 0–30 ng/ml being considered deficient and 31–39 ng/ml considered insufficient (50). According to the Endocrine Society (51) the recommended preferred range falls between 40 and 60 ng/ml, levels below 20 ng/ml should indicate deficiency, 20–29 ng/ml defines insufficiency and ≥30 ng/ml is considered sufficient, all these guidelines along with the ones from the Food and Nutrition Board (52) are summarized in Figure 3.
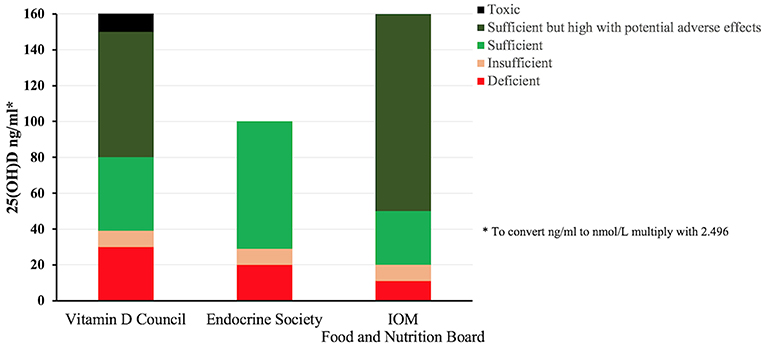
Figure 3. Vitamin D status classification according to different internationally recognized organizations. The lack of a consensus about the ranges for deficiency and sufficiency among various international groups is apparent, Vitamin D council (1) defines Vitamin D deficiency in the range of 0–30 ng/ml whereas Endocrine Society (2) and Institute of Medicine (3) defines it as 0–20 and 0–11 ng/ml, respectively. Similarly, sufficiency is defined as 40–80, 30–100, and >20 ng/ml by (1), (2), (3), respectively. Vitamin D serum levels measured above 150 ng/ml is considered toxic by (1) where as there is no such interpretation by (2) and (3) (50–52).
Measurement of 25(OH)D can be performed with a number of different analytical techniques. Automated immunoassay's is used by most pathology lab to measure total serum 25(OH) D (25(OH) D2 + 25(OH) D3). Because of the clinical importance of Vitamin D testing it is important to note the existing discrepancies in Vitamin D total immunoassays as reported by several studies (53, 54). Various causes of the discrepancies have been noted (55) including the cross-reactivities of various Vitamin D metabolites (56). Therefore, the assays for Vitamin D testing need to be standardized so there is less variability in the results, the Vitamin D Standardization Program (VDSP), was launched in 2010 in collaboration with the National Institutes of Health, the Centers for Disease Control and Prevention (CDC), the National Institute for Standards and Technology (NIST), and Ghent University in Belgium to correct the disparity and ensure reliable Vitamin D measurement. However, we are still a long way to go to attain the goal of standardization of assay and results, till then we have to rely on the judgement of physicians and experts in the field. The researchers who publish in this area will likely continue to point toward such disparities.
To overcome the deficiency, Vitamin D supplementation is usually advised, however there is no consensus on the optimal dose to be prescribed, as many factors such as age and serum levels of 25(OH)D must be taken into account before recommending the best possible dose for instance for obese people (BMI > 30 kg/m2), a daily Vitamin D dose was set as “three times” greater than the recommended dose for subjects with normal body weight (51). The most common form of Vitamin D supplementation is Cholecalciferol and Ergocalciferol with both considered highly effective and safe (57). Vitamin D toxicity generally results from having serum levels of 25(OH)D > 150 ng/ml, which in most cases is attributed to prolonged and unintended daily intakes of >40,000 IU of supplementation (58). Table 1 details the group-wise recommendations for daily dose of Vitamin D supplementation from different organizations.
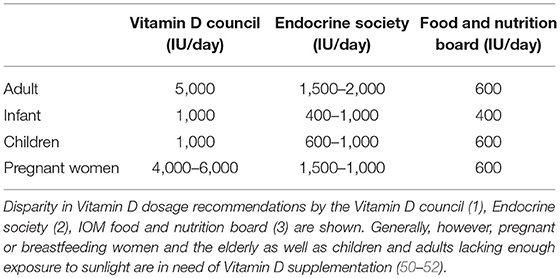
Table 1. Group-wise recommendations for daily dose of Vitamin D supplementation from different organizations.
Vitamin D Deficiency: a Health Problem Worldwide and in the GCC Countries
Vitamin D deficiency is a major public health problem worldwide, affecting more than a billion people across the globe from both the developing and industrialized countries (59, 60). Studies have suggested that more than 70% of USA and 50% of UK adults may have insufficient Vitamin D levels (61, 62). Among other European populations, 1 in 8 adults have low circulating levels of 25-hydroxyvitamin D (63) and a similar pattern has also been reported in India, Australia and New Zealand (64, 65).
Despite the ample amount of year-round sunlight in the GCC region, pandemic levels of Vitamin D deficiency have been observed in recent years as represented in Figure 4. The 2016–2017 annual report from the Qatar Biobank highlights that close to 86% of the total Qatar biobank population (comprising of adults above 18–85 year-old, 80% of them Qatari national and the rest were long-term residents) suffers from Vitamin D inadequacy and more interestingly, 14% (more women 65% than men 35%) remained deficient despite taking supplementation, 70% of the participants were also obese (71). A review examining mixed population in Saudi Arabia (e.g., pregnant/ lactating women, children, adults) found that in 81% of all the groups the levels of 25(OH) D was <20 ng/mL (<50 nmol/L) (67). The numbers run high in UAE as well, a study conducted with a large cohort of patients (60,979) including UAE nationals and visitors of other nationalities, showed that up to 82.5% of the population suffers from deficiency or insufficiency of Vitamin D. Around 86.1% UAE nationals and 78.9% visitors had serum levels of 25(OH)D < 75 nmol/L. The study also showed that the extreme cases of deficiency were higher in females (26.4%) than males (18.4%) (68). Comparable percentages have also been reported in different study cohorts' in other countries in the gulf peninsula (69, 70).
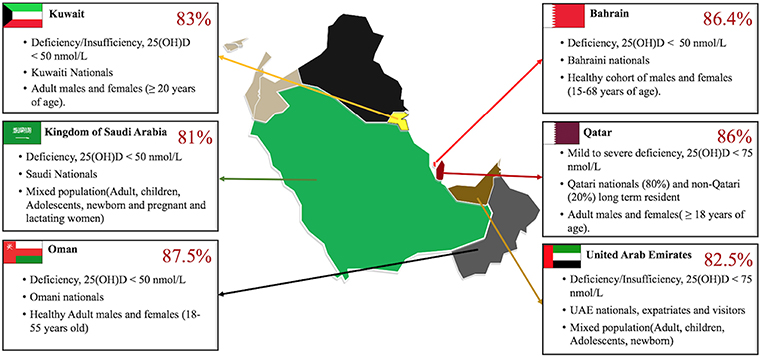
Figure 4. Vitamin D deficiency reported in GCC countries. Gulf Cooperation Council (GCC), alliance of six Middle Eastern countries—Saudi Arabia (KSA), Kuwait, the United Arab Emirates, Qatar, Bahrain, and Oman. The Vitamin D deficiency runs high in the multi-ethnic population of these countries with percentages as high as 86% in Qatar (66) other countries such as KSA (67), UAE (68), Oman (69), and Kuwait (70) follow closely similar alarmingly high rates of Vitamin D deficiency/Insufficiency. The GCC map used was obtained with copyright approval from: https://yourfreetemplates.com/free-middle-east-map/.
Recently many studies have associated high levels of Vitamin D deficiency with immune-mediated and inflammatory diseases. IBD is the term used to describe disorders that involve chronic inflammation of the GI tract. The main types of IBD include: ulcerative colitis and Crohn's disease. Recently the Epi-IBD study reported high prevalence of low Vitamin D levels in treatment-naive European IBD populations, with 79% of the patients showing either insufficient or deficient levels of Vitamin D (72). Vitamin D supplementation was associated with reduced intestinal inflammation in patients with active UC (73) and also in controlling the relapse rate of IBD (74).
Systemic lupus erythematosus (SLE) is a chronic autoimmune inflammatory disease which involves the connective tissue effecting multiple organs such as the brain, lungs, kidneys, heart, blood vessels, muscles, skin etc., and is more common among women (75). Vitamin D deficiency was significantly higher in SLE patients when compared to healthy controls in a study conducted with a cohort of Bahraini patients (76). Similar results were observed in a Saudi cohort (71) with high prevalence of Vitamin D inadequacy was observed in Saudi patients with SLE.
T1DM prevalence/incidence is increasing worldwide (77) the rise seems much steeper in gulf countries. A study conducted to determine the association between Vitamin D status and T1DM along with other factors in the young population of the State of Qatar found out that the incidences of severe Vitamin D deficiency was considerably higher in T1DM (28.8%) compared with healthy children (17.1%) (78), similarly in the Saudi cohort 84% of the T1DM children, and 59% of the healthy children were Vitamin D deficient (79). Low serum Vitamin D status was found to be associated with high prevalence and early onset of type-1 diabetes mellitus in Kuwaiti children as well (80). In a case control study done with Multiple sclerosis patient in Kuwait, VDR variants TaqI and BsmI were found to be associated with MS risk (81).The therapeutic effect of Vitamin D in immune diseases should be further assessed in interventional studies.
A comparative associative study between Vitamin D deficiency and IBS (Irritable bowel syndrome) was conducted with patients visiting the gastroenterology clinic in Saudi Arabia, Vitamin D deficiency was detected in 82% of the patients in the IBS group and 31% in the control group (82).
The potential limitation to the findings of this review should be considered, relatively small number of studies were available to be included in the review and also there is limited data to prove causal relationship between Vitamin D deficiency and risk of Immune system related illness. The studies included employ various definitions of hypovitaminosis D, and these variations may result in the over or underestimation of Vitamin D deficiency in GCC population. Thus, development of global or local standard and guidelines will help in better screening to define the candidates and treating those who are at most risk for Vitamin D deficiency.
As we previously discussed, synthesis of Vitamin D occurs in the skin and it depends on several factors such as time of sun exposure, season, latitude, altitude, clothing, veiling, use of sunscreen, old age, and skin color (83). It was previously shown that limited exposure to sunlight results in poor Vitamin D synthesis (84), thus even in sunny climates like the GCC countries, high rates of Vitamin D deficiency is found because of cultural and social habits that limit exposure to sunlight (85). The association between reduced 25D concentrations and obesity is also well-established with several large-scale studies that found obesity to be associated with lower 25D, 1,25D concentrations (86, 87). A bi-directional genetic study revealed that though the effect of lower 25D on BMI may not be significant but higher BMI leads to lower 25D (88) various explanations have been proposed to define this association, such as reduced cutaneous synthesis (89) and altered metabolism (90). Dietary sources make up for <10% of the total Vitamin D synthesized in the body (91), so even with a varied balanced diet, individuals will not be able to achieve the recommended Vitamin D levels.
However, the insufficiency of Vitamin D may not be solely due to diet or lack of sunlight. Several studies have suggested that various genes can define the Vitamin D status, thus large-scale genome-wide association studies have identified selected genes mainly those involved in the synthesis, metabolism or transport of Vitamin D to be associated with a variation in the Vitamin D status (92, 93). The SNPs associated with these genes were initially singled out in a European population (92) and were later replicated in the African American (94) and Asian populations (95). We utilized the GWAS catalog to generate the list of 49 gene variants or risk allele associated with Vitamin D Levels as shown in Table 2 below. After literature review we identified two gene-association studies conducted in the GCC region, one in the Saudi populations (104) and the other in the Kuwaiti population (105). The study performed in the Saudi population identified a significant risk of Vitamin D deficiency and insufficiency associated with the SNP rs2228570 [Chromosome: 12, Position: 47879112, Gene: Vitamin D Receptor (VDR)], rs4588 [Chromosome: 4, Position: 71752606, Gene: GC] and rs10741657[Chromosome: 11, Position: 14,893,332, Gene: Vitamin D 25-hydroxylase (CYP2R1)] (104), while the study conducted in Kuwait showed that the polymorphism in the GC gene coding for the Vitamin D Binding protein (VDBP) may play a major role in determining Vitamin D levels in this population (105).
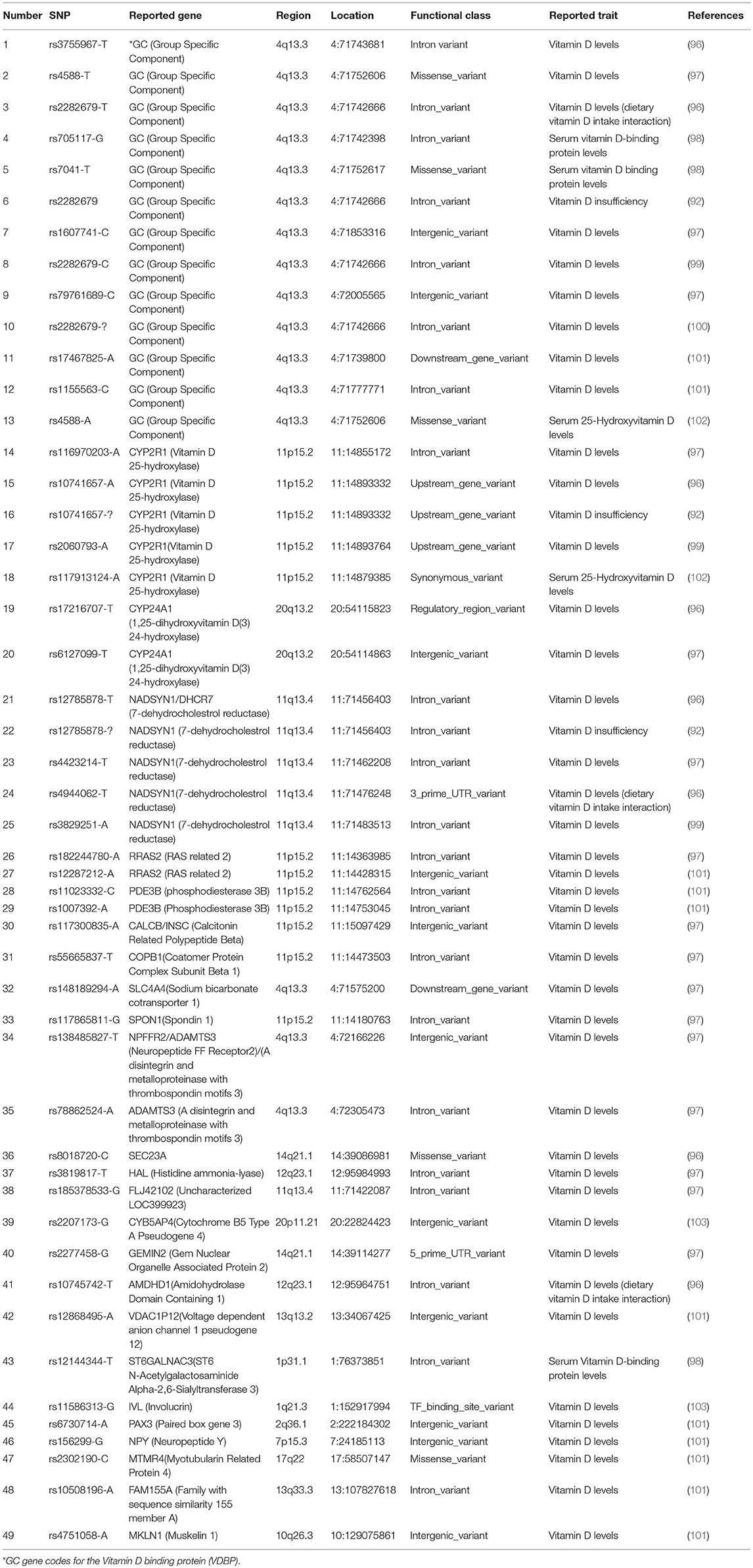
Table 2. List of key variants or risk allele associated with Vitamin D level as reported by the GWAS catalog.
Vitamin D and the Gut Microbiome: is it a Bidirectional Relationship
The human gut microbiota (the microbial taxa associated with humans) is home to an estimated 1014 microorganisms, with around 500–1,000 species of bacteria (106). The vastness of the human microbiome (the catalog of human microbiota and their genes) can be imagined in terms that it contains 10 times more cells and 100 times more genes than the human (107). These microorganisms create a “mini-ecosystem” inside our bodies, and they work together as biochemical factories performing a wide array of activities such as acquisition of nutrients, Vitamins production, degradation of toxins and enhancing host immune responses by functioning as a barrier from pathogenic microorganisms (108).
Gut microbiota exists in a symbiotic relationship with the host. The composition of the gut microbiome plays a crucial role in maintaining host homeostasis, as the wrong combination of microbes contribute to an array of chronic digestive or immune disorders such as IBD, obesity, diabetes mellitus, metabolic syndrome, atherosclerosis, alcoholic liver disease, nonalcoholic fatty liver disease, cirrhosis, and hepatocellular carcinoma (109, 110).
The compositions of the gut microbiota is determined by several factors as summarized in Figure 5, which include age, host genomics, immune health, exercise, intake of medication specifically antibiotics, and dietary habits (111). This imbalance or maladaptation of microbial communities is often referred to as Dysbiosis (also called dysbacteriosis) which has been categorized into three different types: (1) loss of beneficial organisms, (2) excessive growth of potentially harmful organisms, and (3) loss of overall microbial diversity. These types can occur in combination and are not mutually exclusive (112, 113). In such cases, the normally dominant species become underrepresented and the resulting void is filled by increasing numbers of opportunistic or pathogenic species resulting in overall decrease in overall bacterial diversity (112). Dysbiosis is commonly reported in the gastrointestinal tract (112) and is associated with a loss of the integrity of the intestinal mucosa, which may result in gut impairment and inflammation (114–116).
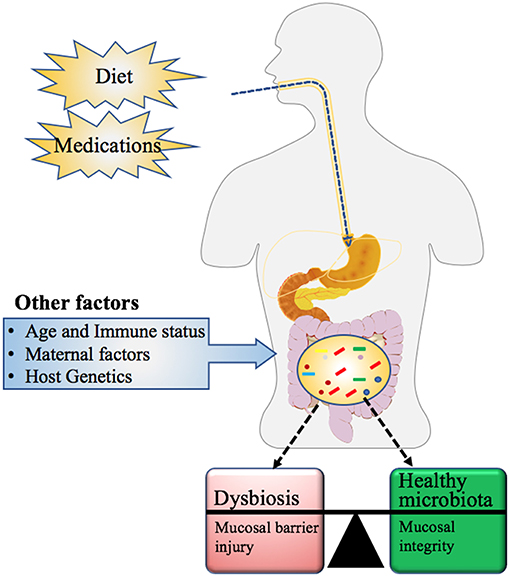
Figure 5. Factors influencing the microbial community composition in Humans. Maternal factors such as mother's nutritional status (use of pre or probiotics), mode of delivery (vaginal versus Cesarean section), gestation age at birth (full or preterm), feeding type (formula feeding or breastfeeding), and other exogenous factors such as diet and medication history, influence not only the gut microbial community but also its metabolic capacity. Other determinants of microbial composition include the age and immune health of the individual along with their genetic makeup (111).
Several studies have demonstrated the role of Vitamin D and its receptor VDR in regulating host-microbial interactions. The active and inactive forms of Vitamin D circulate in the bloodstream bound to VDBP. The active form known as Calcitriol 1,25(OH)2D is known to bind to the calcitriol receptor, commonly known as the VDR (117). Calcitriol binds to the VDR, which then forms a heterodimer with the retinoid-X receptor and other co-activators. This transcriptional complex then binds to discrete sites on DNA known as Vitamin D responsive elements (VDREs) resulting in the expression or repression of specific gene products (summarized in Figure 6) (131). It is also known that Vitamin D plays a role in various microRNA-directed post-transcriptional mechanisms (132).
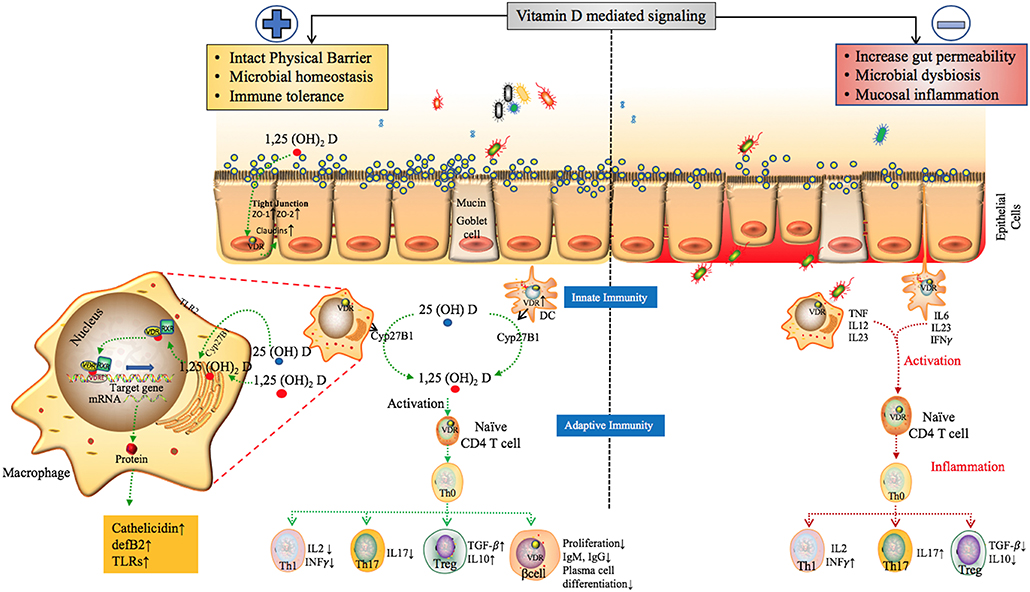
Figure 6. The proposed role of Vitamin D mediated signaling in epithelial cell barrier function, Gut microbial hemostasis and modulation of Innate and adaptive immune responses. VDR regulates the level of tight junction proteins ZO1, ZO2 via claudin2 and 12 (39, 118) and helps maintain the structural integrity of epithelial barrier. Vitamin D deficiency have also been shown to disrupt gut homeostasis leading to systemic inflammation (119). Effects of Vitamin D on different players of the innate and adaptive immune system. In response to an antigenic challenge, macrophages, dendritic cells, and lymphocytes express the Vitamin D receptor (VDR), thereby becoming targets for the active Vitamin D metabolite, 1,25(OH)2D (120). Macrophages and dendritic cells also express the CYP27B1 that synthesizes 1,25(OH)2D leading to intra and paracrine responses such as stimulates enhancing the phagocytotic responses of macrophages and production of antimicrobial proteins such as cathelicidin (49, 121, 122). Active Vitamin D also modulates adaptive immunity. At the level of the APCs (like DCs), 1,25(OH)2D inhibits the surface expression of MHC-II-complexed antigen. In addition, 1,25(OH)2D directly affects T cell responses, by inhibiting the production of Th1 cytokines (IL-2 and IFN-γ), Th17 cytokines (IL-17 and IL-21), and by stimulating Th2 cytokine production (IL-4) and Treg cell development (123–129). Finally, 1,25(OH)2D blocks plasma-cell differentiation, IgG and IgM production and B-cell proliferation (130). The above factors can trigger inflammatory immune responses such as TNF-α and IFN-γ leading to intestinal permeability and susceptibility to pathogenic infections, microbial dysbiosis and manifestation of immune related inflammatory diseases (19).
The Interplay between Vitamin D, VDBP, Cyp27B1, and VDR not only regulate the transcriptional and post-transcriptional responses, but a growing evidence supports the concept that Vitamin D metabolism impacts the intestinal microbial balance and gut homeostasis (132, 133). As previously mentioned, VDR is expressed in a variety of cell types such as kidney, muscles, prostate, immune cells; and high levels of expression in the cells of the gastrointestinal tract (GI) have been shown (134). Moreover, the Vitamin D activating enzyme Cyp27B1 is also expressed in various immune cells, as well as intestinal epithelial cells (135, 136). These two vital players of Vitamin D metabolism have also been colocalized in different cells of the GI tract suggesting the need for active Vitamin D in those cells (137).
In addition, the type of bacterial communities in the gut have been shown to regulate the expression of both VDR and Cyp27B1 (138, 139). VDR expression is inversely related to the presence of pathogenic bacteria, and correlate with probiotic bacteria with the former decreasing (138) and the latter increasing VDR expression (139). Animal studies done with germ-free and antibiotics-treated mice have reported reduced levels of Cyp27B1 expression in those animal models (140). This suggests that the intestinal bacteria regulate Vitamin D metabolism and, as a result, they regulate the innate immune response. The synthesis of active Vitamin D is modulated according to the local needs by Cyp27B1 and VDR enzymes whose expression is dependent on the “nature of local microbiota.”
The VDR, Cyp27B1, and VDBP genes have also been studied for their association with autoimmune diseases such as IBD and its forms Crohn's disease (CD) and Ulcerative colitis (UC) (72, 141, 142). Several VDR polymorphisms such as ApaI, TaqI, and FokI have been associated with the development of UC (143, 144). VDR and Cyp27B1 gene knockout mice have shown increased susceptibility to the development of intestinal colitis, elevated expression of proinflammatory cytokines, and microbial dysbiosis, with more Proteobacteria and less firmicutes, a condition commonly observed in patients with IBD (145). Vitamin D supplementation in human and 1,25D treatment of CYP27B1 knockout mice decreased Proteobacteria and increased beneficial organism including members of the Firmicutes phyla (132, 146).
Gut mucosal integrity is a crucial barrier, playing a vital role in protection against pathogenic microorganisms, as disruption in mucosal barrier function and hyperpermeability can predispose to various diseases such as asthma, tuberculosis, cystic fibrosis and intestinal lung diseases (147–149). Vitamin D and its associated molecules provide protection for the epithelial barriers in various tissues including the gut mucosa, by increasing the expression of several tight and adherent junction proteins (150–152). VDR signaling specifically has been known to regulate the gut mucosal cell inflammation by suppressing intestinal epithelial cell apoptosis (153). The increasing awareness of the role of Vitamin D as an immunomodulator was prompted by the discovery of VDR and production of active Vitamin D (1,25(OH)2 D) in almost all immune cells, including activated CD4+ and CD8+ T cells, B cells, neutrophils and antigen-presenting cells (APCs) such as macrophages and dendritic cells. The details of this regulation are described in the section below.
Vitamin D in Innate and Adaptive Immune System
The innate immune system is the first and immediate line of the defense against invading pathogens and is an alliance of components both from the host and resident microbiota. The host defense comprises of diverse components such as physical defense (such as epithelial cells of the skin, mucous membrane, and microbiome), cellular defense (as mast cells, dendritic cells, macrophages, neutrophils, natural killer cells etc.), cell receptors that recognize pathogens (as Toll-like Receptors), antimicrobial peptides and proteins (as defensins, cathelicidins).
Vitamin D is able to modulate many of the above listed components of the innate immune system. It is known to reinforce the physical barrier function of epithelial cells. The active Vitamin D and VDR are important in regulating the genes of proteins required for the tight, adheres, and gap junctions such as zonulin occluden-1, zonulin occluden-2 through the up regulation of claudin 2 and 12 (154). Studies in transgenic mice demonstrated that over-expression of VDR in the intestinal epithelium decreases mucosal inflammation suppressing epithelial cell apoptosis and boosting the function of tight junction (119, 155). The importance of active Vitamin D in maintaining healthy gut microbiota has been discussed in the earlier section.
Monocytes and macrophages are crucial members of the innate immunity, Vitamin D stimulates the differentiation of precursor monocytes to mature phagocytic macrophage, the high expression of VDR by monocytes ensures sensitivity of these cells to the differentiating effects of active Vitamin D (120). Mature macrophages sense pathogen-associated molecular patterns (PAMPs) by means of pattern-recognition receptors, such as Toll-like receptors (TLRs). The presence of CYP27B1 (Vitamin D activating enzyme) in macrophages is important for the physiological action of host defense against infection, activation of 25(OH) D to 1, 25(OH)2 D via CYP27B1 in macrophages leads to regulation of TLR2 (121), enhanced production of defensin β2, cathelicidin antimicrobial peptide (CAMP) (49) leading to induction of autophagy (122). The ability of 1,25(OH)2D to increase the production of other antimicrobial peptides, has been demonstrated both in vitro by monocytes stimulation (156) and in vivo in pediatric patients' blood (157).
At the same time, the active Vitamin D can inhibit the dendritic cell (DCs) differentiation and maturation. In human and murine DC cultures, the activation of VDR signaling pathways inhibited DC-maturation as shown by downregulation of DC markers, MHC-class II, co-stimulatory molecules (CD40, CD80, and CD86), and other maturation molecules (e.g., CD1a, CD83), chemokine (CXCL10) which is involved in the recruitment of T helper 1 (Th1) cells. Furthermore, active Vitamin D also modulates DC-derived cytokine and chemokine expression, by inhibiting the production of pro-inflammatory cytokine (IL-12 and IL-23) and enhancing the release of anti-inflammatory cytokine (IL-10) and the chemokine [CCL22, involved in the recruitment regulatory T cells (Tregs)] (123–125).
However, this interaction between active Vitamin D and cells from innate immunity also have downstream effects on cells from the adaptive immune system. The adaptive immune system or acquired immune system is the second line of defense against infection. It comes into action upon exposure to pathogens and uses immunological memory to learn about the pathogens and enhance the immune response accordingly. The adaptive immune system is composed of T and B cells and is also responsible for autoimmune reaction. 1,25(OH)2 D suppresses the immune responses mediated by Type 1 T helper (Th1) cells, by inhibiting the inflammatory cytokines IL-2 and interferon gamma (IFNγ) (125, 126) On the other hand, it has been reported to enhance cytokines associated with Th2 cells (127). The 1,25(OH)2 D also regulate Treg cells (known for their role in the inhibition of inflammation) by induction of Foxp3 (the transcription factor involved in the development and function of Treg cells) (128). In addition to Th1, Th2, and Treg cells (subsets from the CD4 + T cell lineage) there are distinct subset termed Th17 cells that produce IL-17, which has been implicated in the pathogenesis of a number of autoimmune diseases. Th17 is inhibited by 1,25(OH)2 D (129). 1,25(OH)2 D prevents the activation of nuclear factor kappa-B (NF-κB), a component of proinflammatory signaling pathway at the level of transcription [VDR/RXR has been reported to bind to NF-κB promotor (IL-12p20) thus preventing its activation] (158).
The proliferation of VDR-expressing B-cells is also suppressed by active Vitamin D negatively impacting Ig production and also inhibiting the differentiation of plasma cells and class-switched memory cells highlighting a potential role for Vitamin D in B-cell-related disorders such as SLE (130). Figure 6 details the proposed model of the overall impact of Vitamin D in the regulation of the epithelial barrier function, gut microbiota, and the immune system.
The above data elucidate the role of Vitamin D/VDR signaling in not only maintaining the microbial landscape in the gut in check and keeping the gut mucosal integrity intact but also as a potent immunomodulator. However, to fully understand the extent to which Vitamin D impact these complex systems, the multidirectional interaction between them needs to be considered. The importance of the interplay presents an encouraging field for further research with potential clinical implications.
Discussion
Vitamin D deficiency is a major health problem in GCC countries including Qatar, indicating its importance as a regional and national health problem (67–71). Vitamin D deficiency affects various age groups and threatens the GCC populations with increased prevalence for chronic diseases such as IBD, cancer, diabetes among others. The reasons for the deficiency include limited exposure to sunlight, full body covering and low consumption of food rich in Vitamin D (83–85). The deficiency is treated with Vitamin D supplementation or intramuscular injections, however, despite being treated in a similar manner; there are interracial and interindividual variations in response to Vitamin D intake (159). The differences in serum Vitamin D levels among diverse ethnic groups have been previously reported in GWAS studies that have identified SNPs associated with genes from the Vitamin D pathway (92–95). These studies have indicated that certain ethnic groups may be predisposed to lower serum Vitamin D levels. We believe the same may be true for the population residing in GCC countries. These genetic variations may also impact the response to Vitamin D supplementation in these populations.
Originally known to cure rickets, our understanding on how Vitamin D impacts human health has broadened. The discovery of VDRs and Vitamin D associated enzymes in a variety of cells including cells of the innate and adaptive immune system was crucial in appreciating its role as a potent immunomodulator. Immune cells are not only a target for Vitamin D, but are also able to activate the hormone locally, thus postulating for an autocrine and paracrine role for the active Vitamin D (160). Inadequate amounts of Vitamin D could be linked to defective functioning of the associated autocrine and paracrine circuits eventually leading to various immune abnormalities.
Among other extra-skeletal effects, we have also come to realize its role in maintaining a healthy gut microbiota. The GI tract is home to a plethora of microorganisms that program the immune system preventing colonization by harmful bacteria and viruses. Several studies referenced in this review have suggested that Vitamin D signaling influences the microbial load and composition and ensures adequate innate and adaptive immune responses to pathogenic threats; though the questions still linger about the bidirectional and multidirectional interaction between these intricate systems. Vitamin D deficiency may result in abnormal immune functions causing inflammation, which, when unresolved, may lead to chronic conditions.
In conclusion, Vitamin D signaling mediated by key players, such as VDR, VDBP, and Cyp27B1, has a diverse physiological impact, enhancing the intestinal barrier function and contributing to enteric homeostasis. Studies have shown that low serum Vitamin D levels might be linked to several diseases and given the fact that the Vitamin D status is a modifiable factor, the potential therapeutic benefits from Vitamin D supplementation in the prevention of various diseases and maintenance of a healthy microbiota are undeniable. Therefore, the widespread Vitamin D deficiency in the GCC countries demands discussion about including Vitamin D testing as part of routine clinical care practices.
Author Contributions
All authors listed have made a substantial, direct and intellectual contribution to the work, and approved it for publication.
Conflict of Interest Statement
The authors declare that the research was conducted in the absence of any commercial or financial relationships that could be construed as a potential conflict of interest.
Acknowledgments
This work has been supported by Sidra Internal Research Funds SDR200006. The authors thank Dr. Bernice Lo for her assistance in proofreading the review.
References
1. Steenbock H. The induction of growth promoting and calcifying properties in a ration by exposure to light. Science. (1924) 60:224–5. doi: 10.1126/science.60.1549.224
2. Lieben L, Carmeliet G. Vitamin D signaling in osteocytes: effects on bone and mineral homeostasis. Bone. (2013) 54:237–43. doi: 10.1016/j.bone.2012.10.007
3. Hewison M. An update on vitamin D and human immunity. Clin Endocrinol. (2012) 76:315–25. doi: 10.1111/j.1365–2265.2011.04261.x
4. Haussler MR, Whitfield GK, Kaneko I, Forster R, Saini R, Hsieh JC, et al. The role of vitamin D in the FGF23, klotho, and phosphate bone-kidney endocrine axis. Rev Endocr Metab Disord. (2012) 13:57–69. doi: 10.1007/s11154-011-9199-8
5. Forster RE, Jurutka PW, Hsieh JC, Haussler CA, Lowmiller CL, Kaneko I, et al. Vitamin D receptor controls expression of the anti-aging klotho gene in mouse and human renal cells. Biochem Biophys Res Commun. (2011) 414:557–62. doi: 10.1016/j.bbrc.2011.09.117
6. Liu SM, Koszewski N, Lupez M, Malluche HH, Olivera A, Russell J. Characterization of a response element in the 5'-flanking region of the avian (chicken) PTH gene that mediates negative regulation of gene transcription by 1,25-dihydroxyvitamin D3 and binds the vitamin D3 receptor. Mol Endocrinol. (1996) 10:206–15. doi: 10.1210/mend.10.2.8825560
7. Meyer MB, Watanuki M, Kim S, Shevde NK, Pike JW. The human transient receptor potential vanilloid type 6 distal promoter contains multiple vitamin D receptor binding sites that mediate activation by 1,25-dihydroxyvitamin D3 in intestinal cells. Mol Endocrinol. (2006) 20:1447–61. doi: 10.1210/me.2006-0031
8. Haussler MR, Jurutka PW, Mizwicki M, Norman AW. Vitamin D receptor (VDR)-mediated actions of 1alpha,25(OH)(2)vitamin D(3): genomic and non-genomic mechanisms. Best Pract Res Clin Endocrinol Metab. (2011) 25:543–59. doi: 10.1016/j.beem.2011.05.010
9. Barthel TK, Mathern DR, Whitfield GK, Haussler CA, Hopper HAt, Hsieh JC, et al. 1,25-Dihydroxyvitamin D3/VDR-mediated induction of FGF23 as well as transcriptional control of other bone anabolic and catabolic genes that orchestrate the regulation of phosphate and calcium mineral metabolism. J Steroid Biochem Mol Biol. (2007) 103:381–8. doi: 10.1016/j.jsbmb.2006.12.054
10. Haussler MR, Haussler CA, Whitfield GK, Hsieh JC, Thompson PD, Barthel TK, et al. The nuclear vitamin D receptor controls the expression of genes encoding factors which feed the “Fountain of Youth” to mediate healthful aging. J Steroid Biochem Mol Biol. (2010) 121:88–97. doi: 10.1016/j.jsbmb.2010.03.019
11. Kim S, Yamazaki M, Zella LA, Shevde NK, Pike JW. Activation of receptor activator of NF-kappaB ligand gene expression by 1,25-dihydroxyvitamin D3 is mediated through multiple long-range enhancers. Mol Cell Biol. (2006) 26:6469–86. doi: 10.1128/MCB.00353-06
12. Ryynanen J, Seuter S, Campbell MJ, Carlberg C. Gene regulatory scenarios of primary 1,25-dihydroxyvitamin d3 target genes in a human myeloid leukemia cell line. Cancers. (2013) 5:1221–41. doi: 10.3390/cancers5041221
13. Heikkinen S, Vaisanen S, Pehkonen P, Seuter S, Benes V, Carlberg C. Nuclear hormone 1alpha,25-dihydroxyvitamin D3 elicits a genome-wide shift in the locations of VDR chromatin occupancy. Nucleic Acids Res. (2011) 39:9181–93. doi: 10.1093/nar/gkr654
14. Gombart AF, Borregaard N, Koeffler HP. Human cathelicidin antimicrobial peptide (CAMP) gene is a direct target of the vitamin D receptor and is strongly up-regulated in myeloid cells by 1,25-dihydroxyvitamin D3. FASEB J. (2005) 19:1067–77. doi: 10.1096/fj.04-3284com
15. Skrobot A, Demkow U, Wachowska M. Immunomodulatory role of vitamin D: a review. Adv Exp Med Biol. (2018) 1108:13–23. doi: 10.1007/5584_2018_246
17. Herrera G. Vitamin D in massive doses as an adjuvant to the sulfones in the treatment of tuberculoid leprosy. Int J Lepr. (1949) 17:35–42.
19. Rosen CJ, Adams JS, Bikle DD, Black DM, Demay MB, Manson JE, et al. The nonskeletal effects of vitamin D: an endocrine society scientific statement. Endocr Rev. (2012) 33:456–92. doi: 10.1210/er.2012-1000
20. Giovannucci E. Expanding roles of vitamin D. J Clin Endocrinol Metab. (2009) 94:418–20. doi: 10.1210/jc.2008-2695
21. Liu W, Zhang L, Xu HJ, Li Y, Hu CM, Yang JY, et al. The anti-inflammatory effects of vitamin D in tumorigenesis. Int J Mol Sci. (2018) 19:E2736. doi: 10.3390/ijms19092736
22. Perez-Hernandez N, Aptilon-Duque G, Nostroza-Hernandez MC, Vargas-Alarcon G, Rodriguez-Perez JM, Blachman-Braun R. Vitamin D and its effects on cardiovascular diseases: a comprehensive review. Korean J Intern Med. (2016) 31:1018–29. doi: 10.3904/kjim.2015.224
23. Zittermann A, Schleithoff SS, Koerfer R. Putting cardiovascular disease and vitamin D insufficiency into perspective. Br J Nutr. (2005) 94:483–92. doi: 10.1079/BJN20051544
24. Wang TJ, Pencina MJ, Booth SL, Jacques PF, Ingelsson E, Lanier K, et al. Vitamin D deficiency and risk of cardiovascular disease. Circulation. (2008) 117:503–11. doi: 10.1161/CIRCULATIONAHA.107.706127
25. Schwarz N, Nicholls SJ, Psaltis PJ. Vitamin D and cardiovascular disease. Heart Lung Circ. (2018) 27:903–6. doi: 10.1016/j.hlc.2018.05.098
26. Buell JS, Dawson-Hughes B. Vitamin D and neurocognitive dysfunction: preventing “D”ecline? Mol Aspects Med. (2008) 29:415–22. doi: 10.1016/j.mam.2008.05.001
27. Fond G, Godin O, Schurhoff F, Berna F, Bulzacka E, Andrianarisoa M, et al. Hypovitaminosis D is associated with depression and anxiety in schizophrenia: Results from the national FACE-SZ cohort. Psychiatry Res. (2018) 270:104–10. doi: 10.1016/j.psychres.2018.09.024
28. Balion C, Griffith LE, Strifler L, Henderson M, Patterson C, Heckman G, et al. Vitamin D, cognition, and dementia: a systematic review and meta-analysis. Neurology. (2012) 79:1397–405. doi: 10.1212/WNL.0b013e31826c197f
29. Judd SE, Morgan CJ, Panwar B, Howard VJ, Wadley VG, Jenny NS, et al. Vitamin D deficiency and incident stroke risk in community-living black and white adults. Int J Stroke. (2016) 11:93–102. doi: 10.1177/1747493015607515
30. Thomas MK, Lloyd-Jones DM, Thadhani RI, Shaw AC, Deraska DJ, Kitch BT, et al. Hypovitaminosis D in medical inpatients. N Engl J Med. (1998) 338:777–83. doi: 10.1056/NEJM199803193381201
31. Jawa A, Riaz SH, Khan Assir MZ, Afreen B, Riaz A, Akram J. Causes of short stature in Pakistani children found at an Endocrine Center. Pak J Med Sci. (2016) 32:1321–5. doi: 10.12669/pjms.326.11077
32. Kim HS, Rotundo L, Kothari N, Kim SH, Pyrsopoulos N. Vitamin D is associated with severity and mortality of non-alcoholic fatty liver disease: a US population-based study. J Clin Transl Hepatol. (2017) 5:185–92. doi: 10.14218/JCTH.2017.00025
33. Hollis BW, Wagner CL. Nutritional vitamin D status during pregnancy: reasons for concern. CMAJ. (2006) 174:1287–90. doi: 10.1503/cmaj.060149
34. Walker VP, Zhang X, Rastegar I, Liu PT, Hollis BW, Adams JS, et al. Cord blood vitamin D status impacts innate immune responses. J Clin Endocrinol Metab. (2011) 96:1835–43. doi: 10.1210/jc.2010-1559
35. Belderbos ME, Houben ML, Wilbrink B, Lentjes E, Bloemen EM, Kimpen JL, et al. Cord blood vitamin D deficiency is associated with respiratory syncytial virus bronchiolitis. Pediatrics. (2011) 127:e1513–20. doi: 10.1542/peds.2010-3054
36. Mulligan ML, Felton SK, Riek AE, Bernal-Mizrachi C. Implications of vitamin D deficiency in pregnancy and lactation. Am J Obstet Gynecol. (2010) 202:429 e1–9. doi: 10.1016/j.ajog.2009.09.002
37. Javaid MK, Crozier SR, Harvey NC, Gale CR, Dennison EM, Boucher BJ, et al. Maternal vitamin D status during pregnancy and childhood bone mass at age 9 years: a longitudinal study. Lancet. (2006) 367:36–43. doi: 10.1016/S0140-6736(06)67922-1
38. Wang J, Thingholm LB, Skiecevičienė J, Rausch P, Kummen M, Hov JR, et al. Genome-wide association analysis identifies variation in vitamin D receptor and other host factors influencing the gut microbiota. Nat Genet. (2016) 48:1396–406. doi: 10.1038/ng.3695
39. Clark A, Mach N. Role of vitamin D in the hygiene hypothesis: the interplay between vitamin D, Vitamin D receptors, gut microbiota, and immune response. Front Immunol. (2016) 7:627. doi: 10.3389/fimmu.2016.00627
40. Jin D, Wu S, Zhang YG, Lu R, Xia Y, Dong H, et al. Lack of vitamin D receptor causes dysbiosis and changes the functions of the murine intestinal microbiome. Clin Ther. (2015) 37:996–1009 e7. doi: 10.1016/j.clinthera.2015.04.004
41. Chirumbolo S, Bjorklund G, Sboarina A, Vella A. The role of vitamin D in the immune system as a pro-survival molecule. Clin Ther. (2017) 39:894–916. doi: 10.1016/j.clinthera.2017.03.021
42. Lamberg-Allardt C. Vitamin D in foods and as supplements. Prog Biophys Mol Biol. (2006) 92:33–8. doi: 10.1016/j.pbiomolbio.2006.02.017
43. Wacker M, Holick MF. Vitamin D—effects on skeletal and extraskeletal health and the need for supplementation. Nutrients. (2013) 5:111–48. doi: 10.3390/nu5010111
44. Deluca HF. History of the discovery of vitamin D and its active metabolites. Bonekey Rep. (2014) 3:479. doi: 10.1038/bonekey.2013.213
45. Dusso AS, Brown AJ, Slatopolsky E. Vitamin D. Am J Physiol Renal Physiol. (2005) 289:F8–28. doi: 10.1152/ajprenal.00336.2004
46. Gombart AF. The vitamin D-antimicrobial peptide pathway and its role in protection against infection. Future Microbiol. (2009) 4:1151–65. doi: 10.2217/fmb.09.87
47. Holick MF, Frommer JE, McNeill SC, Richtand NM, Henley JW, Potts JTJr. Photometabolism of 7-dehydrocholesterol to previtamin D3 in skin. Biochem Biophys Res Commun. (1977) 76:107–14. doi: 10.1016/0006-291X(77)91674-6
48. Fraser DR, Kodicek E. Unique biosynthesis by kidney of a biological active vitamin D metabolite. Nature. (1970) 228:764–6. doi: 10.1038/228764a0
49. Wang TT, Nestel FP, Bourdeau V, Nagai Y, Wang Q, Liao J, et al. Cutting edge: 1,25-dihydroxyvitamin D3 is a direct inducer of antimicrobial peptide gene expression. J Immunol. (2004) 173:2909–12. doi: 10.4049/jimmunol.173.5.2909
50. Aloia JF. Clinical review: the 2011 report on dietary reference intake for vitamin D: where do we go from here? J Clin Endocrinol Metab. (2011) 96:2987–96. doi: 10.1210/jc.2011-0090
51. Holick MF, Binkley NC, Bischoff-Ferrari HA, Gordon CM, Hanley DA, Heaney RP, et al. Evaluation, treatment, and prevention of vitamin D deficiency: an Endocrine Society clinical practice guideline. J Clin Endocrinol Metab. (2011) 96:1911–30. doi: 10.1210/jc.2011-0385
52. Ross AC, Taylor CL, Yaktine AL, Del Valle HB. Dietary Reference Intakes for Calcium and Vitamin D. Institute of Medicine report. Washington, DC: The National Academies Press (2011). p. 1–1132.
53. Carter GD. Accuracy of 25-hydroxyvitamin D assays: confronting the issues. Curr Drug Targets. (2011) 12:19–28. doi: 10.2174/138945011793591608
54. Farrell C, Soldo J, Williams P, Herrmann M. 25-Hydroxyvitamin D testing: challenging the performance of current automated immunoassays. Clin Chem Lab Med. (2012) 50:1953–63. doi: 10.1515/cclm-2012-0522
55. Fuleihan Gel H, Bouillon R, Clarke B, Chakhtoura M, Cooper C, McClung M, et al. Serum 25-hydroxyvitamin D Levels: variability, knowledge gaps, and the concept of a desirable range. J Bone Miner Res. (2015) 30:1119–33. doi: 10.1002/jbmr.2536
56. Lee JH, Choi JH, Kweon OJ, Park AJ. Discrepancy between vitamin D total immunoassays due to various cross-reactivities. J Bone Metab. (2015) 22:107–12. doi: 10.11005/jbm.2015.22.3.107
57. Zittermann A, Prokop S, Gummert JF, Borgermann J. Safety issues of vitamin D supplementation. Anticancer Agents Med Chem. (2013) 13:4–10. doi: 10.2174/187152013804487290
58. Lowe H, Cusano NE, Binkley N, Blaner WS, Bilezikian JP. Vitamin D toxicity due to a commonly available “over the counter” remedy from the Dominican Republic. J Clin Endocrinol Metab. (2011) 96:291–5. doi: 10.1210/jc.2010-1999
59. Papandreou D, Malindretos P, Karabouta Z, Rousso I. Possible health implications and low vitamin D Status during childhood and adolescence: an updated mini review. Int J Endocrinol. (2010) 2010:472173. doi: 10.1155/2010/472173
60. Hassan-Smith ZK, Hewison M, Gittoes NJ. Effect of vitamin D deficiency in developed countries. Br Med Bull. (2017) 122:79–89. doi: 10.1093/bmb/ldx005
61. Kennel KA, Drake MT, Hurley DL. Vitamin D deficiency in adults: when to test and how to treat. Mayo Clin Proc. (2010) 85:752–7; quiz 757–8. doi: 10.4065/mcp.2010.0138
62. Pearce SH, Cheetham TD. Diagnosis and management of vitamin D deficiency. BMJ. (2010) 340:b5664. doi: 10.1136/bmj.b5664
63. Hayes A, Cashman KD. Food-based solutions for vitamin D deficiency: putting policy into practice and the key role for research. Proc Nutr Soc. (2017) 76:54–63. doi: 10.1017/S0029665116000756
64. Marwaha RK, Tandon N, Reddy DR, Aggarwal R, Singh R, Sawhney RC, et al. Vitamin D and bone mineral density status of healthy schoolchildren in northern India. Am J Clin Nutr. (2005) 82:477–82. doi: 10.1093/ajcn.82.2.477
65. Nowson CA, McGrath JJ, Ebeling PR, Haikerwal A, Daly RM, Sanders KM, et al. Vitamin D and health in adults in Australia and New Zealand: a position statement. Med J Aust. (2012) 196:686–7. doi: 10.5694/mja11.10301
67. Al-Daghri NM. Vitamin D in Saudi Arabia: prevalence,distribution and disease associations. J Steroid Biochem Mol Biol. (2018) 175:102–7. doi: 10.1016/j.jsbmb.2016.12.017
68. Haq A, Svobodova J, Imran S, Stanford C, Razzaque MS. Vitamin D deficiency: a single centre analysis of patients from 136 countries. J Steroid Biochem Mol Biol. (2016) 164:209–13. doi: 10.1016/j.jsbmb.2016.02.007
69. Abiaka C, Delghandi M, Kaur M, Al-Saleh M. Vitamin D status and anthropometric indices of an omani study population. Sultan Qaboos Univ Med J. (2013) 13:224–31. doi: 10.12816/0003227
70. Zhang FF, Al Hooti S, Al Zenki S, Alomirah H, Jamil KM, Rao A, et al. Vitamin D deficiency is associated with high prevalence of diabetes in Kuwaiti adults: results from a national survey. BMC Public Health. (2016) 16:100. doi: 10.1186/s12889-016-2758-x
71. Damanhouri LH. Vitamin D deficiency in Saudi patients with systemic lupus erythematosus. Saudi Med J. (2009) 30:1291–5. doi: 10.5001/omj.2013.10
72. Chetcuti Zammit S, Ellul P, Girardin G, Valpiani D, Nielsen KR, Olsen J, et al. Vitamin D deficiency in a European inflammatory bowel disease inception cohort: an Epi-IBD study. Eur J Gastroenterol Hepatol. (2018) 30:1297–303. doi: 10.1097/MEG.0000000000001238
73. Garg M, Hendy P, Ding JN, Shaw S, Hold G, Hart A. The effect of vitamin D on intestinal inflammation and faecal microbiota in patients with ulcerative colitis. J Crohns Colitis. (2018) 12:963–72. doi: 10.1093/ecco-jcc/jjy052
74. Li J, Chen N, Wang D, Zhang J, Gong X. Efficacy of vitamin D in treatment of inflammatory bowel disease: a meta-analysis. Medicine. (2018) 97:e12662. doi: 10.1097/MD.0000000000012662
75. Tsokos GC. Systemic lupus erythematosus. N Engl J Med. (2011) 365:2110–21. doi: 10.1056/NEJMra1100359
76. Farid E, Jaradat AA, Al-Segai O, Hassan AB. Prevalence of vitamin D deficiency in adult patients with systemic lupus erythematosus in kingdom of Bahrain. Egypt J Immunol. (2017) 24:1–8. doi: 10.4172/2153-0645-C1-016
77. Emerging Risk Factors Collaboration, Sarwar N, Gao P, Seshasai SR, Gobin R, Kaptoge S, et al. Diabetes mellitus, fasting blood glucose concentration, and risk of vascular disease: a collaborative meta-analysis of 102 prospective studies. Lancet. (2010) 375:2215–22. doi: 10.1016/S0140-6736(10)60484-9
78. Bener A, Alsaied A, Al-Ali M, Hassan AS, Basha B, Al-Kubaisi A, et al. Impact of lifestyle and dietary habits on hypovitaminosis D in type 1 diabetes mellitus and healthy children from Qatar, a sun-rich country. Ann Nutr Metab. (2008) 53:215–22. doi: 10.1159/000184439
79. Bin-Abbas BS, Jabari MA, Issa SD, Al-Fares AH, Al-Muhsen S. Vitamin D levels in Saudi children with type 1 diabetes. Saudi Med J. (2011) 32:589–92.
80. Rasoul MA, Al-Mahdi M, Al-Kandari H, Dhaunsi GS, Haider MZ. Low serum vitamin-D status is associated with high prevalence and early onset of type-1 diabetes mellitus in Kuwaiti children. BMC Pediatr. (2016) 16:95. doi: 10.1186/s12887-016-0629-3
81. Al-Temaimi RA, Al-Enezi A, Al-Serri A, Alroughani R, Al-Mulla F. The association of vitamin D receptor polymorphisms with multiple sclerosis in a case-control study from Kuwait. PLoS ONE. (2015) 10:e0142265. doi: 10.1371/journal.pone.0142265
82. Khayyat Y, Attar S. Vitamin D deficiency in patients with irritable bowel syndrome: does it exist? Oman Med J. (2015) 30:115–8. doi: 10.5001/omj.2015.25
83. Plotnikoff GA. Weather or not: the importance of vitamin D status monitoring and supplementation. Minn Med. (2009) 92:43–6.
84. Glerup H, Mikkelsen K, Poulsen L, Hass E, Overbeck S, Thomsen J, et al. Commonly recommended daily intake of vitamin D is not sufficient if sunlight exposure is limited. J Intern Med. (2000) 247:260–8. doi: 10.1046/j.1365–2796.2000.00595.x
85. Al-Mogbel ES. Vitamin D status among Adult Saudi females visiting primary health care clinics. Int J Health Sci. (2012) 6:116–26. doi: 10.12816/0005987
86. Parikh SJ, Edelman M, Uwaifo GI, Freedman RJ, Semega-Janneh M, Reynolds J, et al. The relationship between obesity and serum 1,25-dihydroxy vitamin D concentrations in healthy adults. J Clin Endocrinol Metab. (2004) 89:1196–9. doi: 10.1210/jc.2003-031398
87. Lagunova Z, Porojnicu AC, Lindberg F, Hexeberg S, Moan J. The dependency of vitamin D status on body mass index, gender, age and season. Anticancer Res. (2009) 29:3713–20. doi: 10.14341/2071-8713-4886
88. Vimaleswaran KS, Berry DJ, Lu C, Tikkanen E, Pilz S, Hiraki LT, et al. Causal relationship between obesity and vitamin D status: bi-directional Mendelian randomization analysis of multiple cohorts. PLoS Med. (2013) 10:e1001383. doi: 10.1371/journal.pmed.1001383
89. Wortsman J, Matsuoka LY, Chen TC, Lu Z, Holick MF. Decreased bioavailability of vitamin D in obesity. Am J Clin Nutr. (2000) 72:690–3. doi: 10.1093/ajcn/72.3.690
90. Kong J, Li YC. Molecular mechanism of 1,25-dihydroxyvitamin D3 inhibition of adipogenesis in 3T3-L1 cells. Am J Physiol Endocrinol Metab. (2006) 290:E916–24. doi: 10.1152/ajpendo.00410.2005
91. Holick MF. Vitamin D: a millenium perspective. J Cell Biochem. (2003) 88:296–307. doi: 10.1002/jcb.10338
92. Wang TJ, Zhang F, Richards JB, Kestenbaum B, van Meurs JB, Berry D, et al. Common genetic determinants of vitamin D insufficiency: a genome-wide association study. Lancet. (2010) 376:180–8. doi: 10.1016/S0140-6736(10)60588-0
93. Bouillon R. Genetic and environmental determinants of vitamin D status. Lancet. (2010) 376:148–9. doi: 10.1016/S0140-6736(10)60635-6
94. Engelman CD, Fingerlin TE, Langefeld CD, Hicks PJ, Rich SS, Wagenknecht LE, et al. Genetic and environmental determinants of 25-hydroxyvitamin D and 1,25-dihydroxyvitamin D levels in Hispanic and African Americans. J Clin Endocrinol Metab. (2008) 93:3381–8. doi: 10.1210/jc.2007-2702
95. Zhang Z, He JW, Fu WZ, Zhang CQ, Zhang ZL. An analysis of the association between the vitamin D pathway and serum 25-hydroxyvitamin D levels in a healthy Chinese population. J Bone Miner Res. (2013) 28:1784–92. doi: 10.1002/jbmr.1926
96. Jiang X, O'Reilly PF, Aschard H, Hsu YH, Richards JB, Dupuis J, et al. Genome-wide association study in 79,366 European-ancestry individuals informs the genetic architecture of 25-hydroxyvitamin D levels. Nat Commun. (2018) 9:260. doi: 10.1038/s41467-017-02662-2
97. Manousaki D, Dudding T, Haworth S, Hsu YH, Liu CT, Medina-Gomez C, et al. Low-frequency synonymous coding variation in CYP2R1 has large effects on vitamin D levels and risk of multiple sclerosis. Am J Hum Genet. (2017) 101:227–38. doi: 10.1016/j.ajhg.2017.06.014
98. Moy KA, Mondul AM, Zhang H, Weinstein SJ, Wheeler W, Chung CC, et al. Genome-wide association study of circulating vitamin D-binding protein. Am J Clin Nutr. (2014) 99:1424–31. doi: 10.3945/ajcn.113.080309
99. Ahn J, Yu K, Stolzenberg-Solomon R, Simon KC, McCullough ML, Gallicchio L, et al. Genome-wide association study of circulating vitamin D levels. Hum Mol Genet. (2010) 19:2739–45. doi: 10.1093/hmg/ddq155
100. Lasky-Su J, Lange N, Brehm JM, Damask A, Soto-Quiros M, Avila L, et al. Genome-wide association analysis of circulating vitamin D levels in children with asthma. Hum Genet. (2012) 131:1495–505. doi: 10.1007/s00439-012-1185-z
101. Anderson D, Holt BJ, Pennell CE, Holt PG, Hart PH, Blackwell JM. Genome-wide association study of vitamin D levels in children: replication in the Western Australian Pregnancy Cohort (Raine) study. Genes Immun. (2014) 15:578–83. doi: 10.1038/gene.2014.52
102. O'Brien KM, Sandler DP, Shi M, Harmon QE, Taylor JA, Weinberg CR. Genome-wide association study of serum 25-hydroxyvitamin D in US women. Front Genet. (2018) 9:67. doi: 10.3389/fgene.2018.00067
103. Sapkota BR, Hopkins R, Bjonnes A, Ralhan S, Wander GS, Mehra NK, et al. Genome-wide association study of 25(OH) vitamin D concentrations in Punjabi Sikhs: results of the Asian Indian diabetic heart study. J Steroid Biochem Mol Biol. (2016) 158:149–56. doi: 10.1016/j.jsbmb.2015.12.014
104. Sadat-Ali M, Al-Turki HA, Azam MQ, Al-Elq AH. Genetic influence on circulating vitamin D among Saudi Arabians. Saudi Med J. (2016) 37:996–1001. doi: 10.15537/smj.2016.9.14700
105. Mezzavilla M, Tomei S, Alkayal F, Melhem M, Ali MM, Al-Arouj M, et al. Investigation of genetic variation and lifestyle determinants in vitamin D levels in Arab individuals. J Transl Med. (2018) 16:20. doi: 10.1186/s12967-018-1396-8
106. Mazmanian SK, Round JL, Kasper DL. A microbial symbiosis factor prevents intestinal inflammatory disease. Nature. (2008) 453:620–5. doi: 10.1038/nature07008
107. Sommer F, Backhed F. The gut microbiota–masters of host development and physiology. Nat Rev Microbiol. (2013) 11:227–38. doi: 10.1038/nrmicro2974
108. Ubeda C, Pamer EG. Antibiotics, microbiota, and immune defense. Trends Immunol. (2012) 33:459–66. doi: 10.1016/j.it.2012.05.003
109. Ley RE, Turnbaugh PJ, Klein S, Gordon JI. Microbial ecology: human gut microbes associated with obesity. Nature. (2006) 444:1022–3. doi: 10.1038/4441022a
110. Wang B, Jiang X, Cao M, Ge J, Bao Q, Tang L, et al. Altered fecal microbiota correlates with liver biochemistry in nonobese patients with non-alcoholic fatty liver disease. Sci Rep. (2016) 6:32002. doi: 10.1038/srep32002
111. Nicholson JK, Holmes E, Kinross J, Burcelin R, Gibson G, Jia W, et al. Host-gut microbiota metabolic interactions. Science. (2012) 336:1262–7. doi: 10.1126/science.1223813
112. Tamboli CP, Neut C, Desreumaux P, Colombel JF. Dysbiosis in inflammatory bowel disease. Gut. (2004) 53:1–4. doi: 10.1136/gut.53.1.1
113. Moos WH, Faller DV, Harpp DN, Kanara I, Pernokas J, Powers WR, et al. Microbiota and neurological disorders: a gut feeling. Biores Open Access. (2016) 5:137–45. doi: 10.1089/biores.2016.0010
114. Jang SE, Lim SM, Jeong JJ, Jang HM, Lee HJ, Han MJ, et al. Gastrointestinal inflammation by gut microbiota disturbance induces memory impairment in mice. Mucosal Immunol. (2018) 11:369–79. doi: 10.1038/mi.2017.49
115. Saito T, Hayashida H, Furugen R. Comment on: Cani et al. (2007) Metabolic endotoxemia initiates obesity and insulin resistance: diabetes. (2007) 56:1761–1772. Diabetes 56:e20; author reply e21. doi: 10.2337/db07-1181
116. Creely SJ, McTernan PG, Kusminski CM, Fisher FM, Da Silva NF, Khanolkar M, et al. Lipopolysaccharide activates an innate immune system response in human adipose tissue in obesity and type 2 diabetes. Am J Physiol Endocrinol Metab. (2007) 292:E740–7. doi: 10.1152/ajpendo.00302.2006
117. Moore DD, Kato S, Xie W, Mangelsdorf DJ, Schmidt DR, Xiao R, et al. International Union of Pharmacology. LXII. The NR1H and NR1I receptors: constitutive androstane receptor, pregnene X receptor, farnesoid X receptor alpha, farnesoid X receptor beta, liver X receptor alpha, liver X receptor beta, and vitamin D receptor. Pharmacol Rev. (2006) 58:742–59. doi: 10.1124/pr.58.4.6
118. Zhang YG, Wu S, Sun J. Vitamin D, vitamin D receptor, and tissue barriers. Tissue Barriers. (2013) 1:e23118. doi: 10.4161/tisb.23118
119. He L, Liu T, Shi Y, Tian F, Hu H, Deb DK, et al. Gut epithelial vitamin D receptor regulates microbiota-dependent mucosal inflammation by suppressing intestinal epithelial cell apoptosis. Endocrinology. (2018) 159:967–79. doi: 10.1210/en.2017-00748
120. Reichel H, Koeffler HP, Bishop JE, Norman AW. 25-Hydroxyvitamin D3 metabolism by lipopolysaccharide-stimulated normal human macrophages. J Clin Endocrinol Metab. (1987) 64:1–9. doi: 10.1210/jcem-64-1-1
121. Proal AD, Albert PJ, Marshall TG. The human microbiome and autoimmunity. Curr Opin Rheumatol. (2013) 25:234–40. doi: 10.1097/BOR.0b013e32835cedbf
122. Campbell GR, Spector SA. Vitamin D inhibits human immunodeficiency virus type 1 and Mycobacterium tuberculosis infection in macrophages through the induction of autophagy. PLoS Pathog. (2012) 8:e1002689. doi: 10.1371/journal.ppat.1002689
123. von Essen MR, Kongsbak M, Schjerling P, Olgaard K, Odum N, Geisler C. Vitamin D controls T cell antigen receptor signaling and activation of human T cells. Nat Immunol. (2010) 11:344–9. doi: 10.1038/ni.1851
124. Almerighi C, Sinistro A, Cavazza A, Ciaprini C, Rocchi G, Bergamini A. 1Alpha,25-dihydroxyvitamin D3 inhibits CD40L-induced pro-inflammatory and immunomodulatory activity in human monocytes. Cytokine. (2009) 45:190–7. doi: 10.1016/j.cyto.2008.12.009
125. Baeke F, Takiishi T, Korf H, Gysemans C, Mathieu C. Vitamin D: modulator of the immune system. Curr Opin Pharmacol. (2010) 10:482–96. doi: 10.1016/j.coph.2010.04.001
126. Lemire JM, Adams JS, Kermani-Arab V, Bakke AC, Sakai R, Jordan SC. 1,25-Dihydroxyvitamin D3 suppresses human T helper/inducer lymphocyte activity in vitro. J Immunol. (1985) 134:3032–5.
127. Cantorna MT, Snyder L, Lin YD, Yang L. Vitamin D and 1,25(OH)2D regulation of T cells. Nutrients. (2015) 7:3011–21. doi: 10.3390/nu7043011
128. Jeffery LE, Burke F, Mura M, Zheng Y, Qureshi OS, Hewison M, et al. 1,25-Dihydroxyvitamin D3 and IL-2 combine to inhibit T cell production of inflammatory cytokines and promote development of regulatory T cells expressing CTLA-4 and FoxP3. J Immunol. (2009) 183:5458–67. doi: 10.4049/jimmunol.0803217
129. Joshi S, Pantalena LC, Liu XK, Gaffen SL, Liu H, Rohowsky-Kochan C, et al. 1,25-dihydroxyvitamin D(3) ameliorates Th17 autoimmunity via transcriptional modulation of interleukin-17A. Mol Cell Biol. (2011) 31:3653–69. doi: 10.1128/MCB.05020-11
130. Chen S, Sims GP, Chen XX, Gu YY, Chen S, Lipsky PE. Modulatory effects of 1,25-dihydroxyvitamin D3 on human B cell differentiation. J Immunol. (2007) 179:1634–47. doi: 10.4049/jimmunol.179.3.1634
131. Christakos S, Dhawan P, Verstuyf A, Verlinden L, Carmeliet G. Vitamin D: metabolism, molecular mechanism of action, and pleiotropic effects. Physiol Rev. (2016) 96:365–408. doi: 10.1152/physrev.00014.2015
132. Ooi JH, Li Y, Rogers CJ, Cantorna MT. Vitamin D regulates the gut microbiome and protects mice from dextran sodium sulfate-induced colitis. J Nutr. (2013) 143:1679–86. doi: 10.3945/jn.113.180794
133. Proal AD, Albert PJ, Marshall T. Autoimmune disease in the era of the metagenome. Autoimmun Rev. (2009) 8:677–81. doi: 10.1016/j.autrev.2009.02.016
134. Shang M, Sun J. Vitamin D/VDR, probiotics, and gastrointestinal diseases. Curr Med Chem. (2017) 24:876–87. doi: 10.2174/0929867323666161202150008
135. Veldman CM, Cantorna MT, DeLuca HF. Expression of 1,25-dihydroxyvitamin D(3) receptor in the immune system. Arch Biochem Biophys. (2000) 374:334–8. doi: 10.1006/abbi.1999.1605
136. Battault S, Whiting SJ, Peltier SL, Sadrin S, Gerber G, Maixent JM. Vitamin D metabolism, functions and needs: from science to health claims. Eur J Nutr. (2013) 52:429–41. doi: 10.1007/s00394-012-0430-5
137. Chun RF, Liu PT, Modlin RL, Adams JS, Hewison M. Impact of vitamin D on immune function: lessons learned from genome-wide analysis. Front Physiol. (2014) 5:151. doi: 10.3389/fphys.2014.00151
138. Waterhouse JC, Perez TH, Albert PJ. Reversing bacteria-induced vitamin D receptor dysfunction is key to autoimmune disease. Ann N Y Acad Sci. (2009) 1173:757–65. doi: 10.1111/j.1749–6632.2009.04637.x
139. Appleyard CB, Cruz ML, Isidro AA, Arthur JC, Jobin C, De Simone C. Pretreatment with the probiotic VSL#3 delays transition from inflammation to dysplasia in a rat model of colitis-associated cancer. Am J Physiol Gastrointest Liver Physiol. (2011) 301:G1004–13. doi: 10.1152/ajpgi.00167.2011
140. Mukherji A, Kobiita A, Ye T, Chambon P. Homeostasis in intestinal epithelium is orchestrated by the circadian clock and microbiota cues transduced by TLRs. Cell. (2013) 153:812–27. doi: 10.1016/j.cell.2013.04.020
141. Cantorna MT. IBD: vitamin D and IBD: moving towards clinical trials. Nat Rev Gastroenterol Hepatol. (2016) 13:322–3. doi: 10.1038/nrgastro.2016.72
142. Abdo J, Rai V, Agrawal DK. Interplay of immunity and vitamin D: interactions and implications with current IBD therapy. Curr Med Chem. (2017) 24:852–67. doi: 10.2174/0929867323666161026124951
143. Wang L, Wang ZT, Hu JJ, Fan R, Zhou J, Zhong J. Polymorphisms of the vitamin D receptor gene and the risk of inflammatory bowel disease: a meta-analysis. Genet Mol Res. (2014) 13:2598–610. doi: 10.4238/2014.April.8.2
144. Xue LN, Xu KQ, Zhang W, Wang Q, Wu J, Wang XY. Associations between vitamin D receptor polymorphisms and susceptibility to ulcerative colitis and Crohn's disease: a meta-analysis. Inflamm Bowel Dis. (2013) 19:54–60. doi: 10.1002/ibd.22966
145. Kim JH, Yamaori S, Tanabe T, Johnson CH, Krausz KW, Kato S, et al. Implication of intestinal VDR deficiency in inflammatory bowel disease. Biochim Biophys Acta. (2013) 1830:2118–28. doi: 10.1016/j.bbagen.2012.09.020
146. Bashir M, Prietl B, Tauschmann M, Mautner SI, Kump PK, Treiber G, et al. Effects of high doses of vitamin D3 on mucosa-associated gut microbiome vary between regions of the human gastrointestinal tract. Eur J Nutr. (2016) 55:1479–89. doi: 10.1007/s00394-015-0966-2
147. Finklea JD, Grossmann RE, Tangpricha V. Vitamin D and chronic lung disease: a review of molecular mechanisms and clinical studies. Adv Nutr. (2011) 2:244–53. doi: 10.3945/an.111.000398
148. Agrawal T, Gupta GK, Agrawal DK. Vitamin D deficiency decreases the expression of VDR and prohibitin in the lungs of mice with allergic airway inflammation. Exp Mol Pathol. (2012) 93:74–81. doi: 10.1016/j.yexmp.2012.04.004
149. Mak G, Hanania NA. Vitamin D and asthma. Curr Opin Pulm Med. (2011) 17:1–5. doi: 10.1097/MCP.0b013e3283411440
150. Li YC, Chen Y, Du J. Critical roles of intestinal epithelial vitamin D receptor signaling in controlling gut mucosal inflammation. J Steroid Biochem Mol Biol. (2015) 148:179–83. doi: 10.1016/j.jsbmb.2015.01.011
151. Barbachano A, Fernandez-Barral A, Ferrer-Mayorga G, Costales-Carrera A, Larriba MJ, Munoz A. The endocrine vitamin D system in the gut. Mol Cell Endocrinol. (2017) 453:79–87. doi: 10.1016/j.mce.2016.11.028
152. Stio M, Retico L, Annese V, Bonanomi AG. Vitamin D regulates the tight-junction protein expression in active ulcerative colitis. Scand J Gastroenterol. (2016) 51:1193–9. doi: 10.1080/00365521.2016.1185463
153. Liu W, Chen Y, Golan MA, Annunziata ML, Du J, Dougherty U, et al. Intestinal epithelial vitamin D receptor signaling inhibits experimental colitis. J Clin Invest. (2013) 123:3983–96. doi: 10.1172/JCI65842
154. Lagishetty V, Liu NQ, Hewison M. Vitamin D metabolism and innate immunity. Mol Cell Endocrinol. (2011) 347:97–105. doi: 10.1016/j.mce.2011.04.015
155. Du J, Chen Y, Shi Y, Liu T, Cao Y, Tang Y, et al. 1,25-dihydroxyvitamin D protects intestinal epithelial barrier by regulating the myosin light chain kinase signaling pathway. Inflamm Bowel Dis. (2015) 21:2495–506. doi: 10.1097/MIB.0000000000000526
156. Liu PT, Schenk M, Walker VP, Dempsey PW, Kanchanapoomi M, Wheelwright M, et al. Convergence of IL-1beta and VDR activation pathways in human TLR2/1-induced antimicrobial responses. PLoS ONE. (2009) 4:e5810. doi: 10.1371/journal.pone.0005810
157. Georgieva V, Kamolvit W, Herthelius M, Luthje P, Brauner A, Chromek M. Association between vitamin D, antimicrobial peptides and urinary tract infection in infants and young children. Acta Paediatr. (2018). doi: 10.1111/apa.14499
158. D'Ambrosio D, Cippitelli M, Cocciolo MG, Mazzeo D, Di Lucia P, Lang R, et al. Inhibition of IL-12 production by 1,25-dihydroxyvitamin D3. Involvement of NF-kappaB downregulation in transcriptional repression of the p40 gene. J Clin Invest. (1998) 101:252–62. doi: 10.1172/JCI1050
159. Aloia JF, Patel M, Dimaano R, Li-Ng M, Talwar SA, Mikhail M, et al. Vitamin D intake to attain a desired serum 25-hydroxyvitamin D concentration. Am J Clin Nutr. (2008) 87:1952–8. doi: 10.1093/ajcn/87.6.1952
Keywords: hypovitaminosis D, microbial dysbiosis, VDR, VDBP, CYP27B1, GCC
Citation: Singh P, Kumar M and Al Khodor S (2019) Vitamin D Deficiency in the Gulf Cooperation Council: Exploring the Triad of Genetic Predisposition, the Gut Microbiome and the Immune System. Front. Immunol. 10:1042. doi: 10.3389/fimmu.2019.01042
Received: 30 December 2018; Accepted: 24 April 2019;
Published: 10 May 2019.
Edited by:
Susu M. Zughaier, Qatar University, QatarReviewed by:
Michael F. Holick, Boston Medical Center, United StatesJun Sun, University of Illinois at Chicago, United States
Copyright © 2019 Singh, Kumar and Al Khodor. This is an open-access article distributed under the terms of the Creative Commons Attribution License (CC BY). The use, distribution or reproduction in other forums is permitted, provided the original author(s) and the copyright owner(s) are credited and that the original publication in this journal is cited, in accordance with accepted academic practice. No use, distribution or reproduction is permitted which does not comply with these terms.
*Correspondence: Souhaila Al Khodor, c2Fsa2hvZG9yQHNpZHJhLm9yZw==