- 1Institute of Immunology, Zhejiang University School of Medicine, Hangzhou, China
- 2Department of Pharmacology, China Medical University School of Pharmacy, Shenyang, China
Innate lymphoid cells (ILCs), defined as a heterogeneous population of lymphocytes, have received much attention over recent years. They can be categorized into three subsets according to the expression profiles of transcription factors and differing levels of cytokine production. These cells are widely distributed in human organs and tissues, especially in mucosal tissue. The ILCs are involved in various physiological and pathological processes, including inflammation, worm expulsion, autoimmune disease and tumor progression, many of which have been investigated and clarified in recent studies. In the tumor microenvironment, group 2 innate lymphoid cells (ILC2s) have been proved to be able to either promote or inhibit tumor progression by producing different cytokines, recruiting diverse types of immune cells, expressing immunosuppressive molecules and by regulating the expression of certain inflammatory factors. This review summarizes recent research progress on the immunomodulatory functions of ILC2s in the tumor microenvironment and puts forward some perspectives for future study.
Introduction
Innate lymphoid cells (ILCs) are heterogeneous and highly conserved immune cell populations that occur during organism development. These cells develop with negative lineage markers (Lin−). They lack phenotypical markers, antigen specificity and recombination-activating gene (Rag)-dependent rearrangement of antigen receptors (1, 2). A consensus has been reached in more recent studies that ILCs can be generally divided into non-cytotoxic ILCs and cytotoxic ILCs. Cytotoxic ILCs are named as conventional natural killer (NK) cells (3). Based on the differential requirements for transcriptional factors and cytokine production, the non-cytotoxic ILCs can be further categorized into three groups: group 1 innate lymphoid cells (ILC1s); group 2 innate lymphoid cells (ILC2s); and group 3 innate lymphoid cells (ILC3s) (4, 5). Main phenotypic markers of the different subsets of murine and human ILCs are well-summarized by Vivier et al. (6).
Non-cytotoxic ILCs exhibit similar functions to CD4+ helper T (Th) cells (7) including ILC1's constitutive expression of the transcriptional factor T-bet mirroring the function of Th1 cells. ILC1s can secret interferon-γ (IFN-γ) and tumor necrosis factor (TNF-α) when stimulated by interleukin 12 (IL-12). ILC2s consist of nuocytes, natural helper cells (NH cells) and innate helper 2 cells (IH2s). The development of ILC2s depends on the transcriptional factors GATA-3 and retinoic acid receptor-related orphan receptor-α (RORα), mirroring the nature of Th2 cells. Furthermore, these cells produce type 2 cytokines such as IL-4, IL-5, IL-9, IL-13, IL-15 in response to IL-33, IL-25, and thymic stromal lymphopoietin (TSLP). ILC3s, resembling Th17 cells, are comprised of lymphoid-tissue inducer cells (LTi cells), natural cytotoxicity receptors− (NCR−s) ILC3s as well as NCR+ ILC3s. Collectively, ILC3s secrete cytokines such as IL-17 and IL-22. The RORγ is expressed in ILC3s when stimulated by IL-7 (4). The expanding family of ILC, like ILC22, ILC17 have been identified (8). Due to their plasticity (9), conversion of ILCs occur when they encounter distinct cytokines, transcription factors or distribute diversely. Human ILC1s show the potential capacity to convert into ILC3s in the presence of IL-23. IL-1β and retinoic acid have both been shown to accelerate that process (10). IL-25-responsive ILC2s populations in the lungs can differentiate into ILC3-like cells and produce IL-17 (11). On the contrary, the conversion of ILC3s to ILC1s can be induced by IL-2, IL-12, or IL-15 (12).
ILCs can be composed of cells of fetal, postnatal, or adult origins (13), the transcriptional factors they depend on are different (14). Adult ILCs are derived from the common lymphoid progenitors (CLPs) expressing CD127 in the bone marrow. CLPs give rise to early innate lymphoid progenitors (EILPs), which express integrin α4β7 under the participation of Notch signaling, transcription factors T cell factor 1 (TCF-1), thymocyte selection-associated HMG box protein (TOX) or nuclear factor interleukin-3 (Nfil3, also known as E4BP4). Through GATA-binding protein 3 (GATA3) and the inhibitor of DNA binding 2 (Id2) dependent pathways, EILPs develop into the common helper-like innate lymphoid progenitors (ChILPs), which are defined as lineage−IL-7R+Flt-3−integrin α4β7+CD25−Id2high cells and have been shown to express a high level of Id2 and promyelocytic leukemia zinc finger (PLZF). ChILPs subsequently differentiate into lineage−IL-7R+/−−Flt-3−integrin α4β7+Id2+GATA-3+PLZF+ common innate lymphoid progenitors (CILPs), and then give rise to ILC precursors (ILCPs). NK progenitors are directly derived from EILPs and LTi progenitors not expressing PLZF come from ChILPs in a RORγt-dependent manner. In addition, LTi progenitors express a lower level of Id2, thymocyte selection-associated HMG box protein (TOX) and TCF-1, in contrast to CILPs. ILCPs generate different branches of the ILC family (15, 16). Human ILCs are more abundant in fetal tissues and cord blood than those in adults, while in ILC2s from bone marrow, compared with fetal and perinatal, development may differ considerably (17, 18). Also, fetal ILC2s are distributed to tissues before birth, postnatal ILC2s are generated in the period from birth through weaning, and adult-derived ILC2s slowly dilute the preexisting ILC2s (13). Fetal ILC precursors reside in the intestine during Peyer's patch development, and become a localized source of ILCs (17). ILC2s colonize tissues perinatally and are exceedingly long lived (19, 20), most of the ILC2s produced in bone marrow only are colonized in peripheral tissues in response to inflammatory signals (21–23).
ILC2s have received much attention in areas of allergies, worm expulsion, tissue repair and adipose metabolism homeostasis (4, 24). In recent years, increasing studies have focused on the roles of ILC2s in regulating tumor growth and cancer's inflammatory microenvironment. Here, we summarize the research progress and new findings in clarifying the functions and significance of ILC2s in the tumor microenvironment (TME).
The Physiological Characters of ILC2s
ILC2s are mainly distributed in the mucosal tissues of the skin, lungs and the gastrointestinal tract, with a small amount of ILC2s also existing in peripheral blood. ILC2s are involved in the regulation of many physiological and pathological processes including asthma, allergies, atopic dermatitis, worm expulsion, tissue repair, adipose tissue regulation, metabolic homeostasis, and tumor growth (4). Moreover, ILC2s can be further classified into natural ILC2 cells (nILC2) (which are sensitive to IL-33), and inflammatory ILC2 cells (iILC2) (which are sensitive to IL-25) (11). Immunological memory has long been described in the adaptive immune system, and now has been related to ILC2s and termed “trained immunity.” In this, naïve ILC2s become memory ILC2s upon their encounter with alarmins, where they remember previous activations and respond more vigorously upon subsequent reactivations (25, 26).
The principal transcriptional factors, implicated in the differentiation and development of ILC2s, are GATA3 and RORα, which share the similar function acting in parallel (27). Other transcription factors also take part in the regulation of the development of ILCs, including B-cell CLL/lymphoma 11b (Bcl-11b) (28–30), TCF-1 (15), growth factor independent 1 transcription repressor (GFI1) (31) and E-twenty six 1 (ETS-1) (32).
ILC2s express surface molecules, including MHC II (33), inducible co-stimulatory molecule ligand/inducible co-stimulatory molecule (ICOSL/ICOS) (34), programmed death-ligand 1 (PD-L1) (35), CRTH2 (36), NKp30 (37), killer cell lectin-like receptor subfamily G member 1 (KLRG1) (11) and OX40 (38). In response to IL-25, IL-33, and TSLP, which are mostly derived from epithelial cells, ILC2s are activated to produce the type 2 cytokines IL-4, IL-5, IL-9, IL-13, and other factors such as methionine-enkephalin (Met-Enk) and Amphiregulin (Areg), which suggests that ILC2s exhibit similar functions as CD4+ Th2 cells (5). Some studies have reported that ILC2s role in antigen presentation is having a dialog with CD4+T cells via the expression of MHC II on the surface of ILC2s (39).
Moreover, recent studies have described many new molecules which play important roles in promoting the maturation and development of ILC2s. E3 ubiquitin ligase Von Hippel-Lindau (VHL)-deficiency in innate lymphoid progenitors cause a decrease of mature ILC2s in peripheral non-lymphoid tissues, and VHL-deficient ILC2s exhibit enhanced expression of hypoxia-inducible factor 1α (HIF-1α) and subsequently caused enhanced glycolysis. VHL-deficiency results in low expressions of the IL-33 receptor ST2 and attenuated IL-33-induced ILC2 development has also been described. HIF-1α drives the expression of the glycolytic enzyme pyruvate kinase M2, which downregulates the expression of ST2 via reduced H3K4me3 modification at the Gata3 promoter and Il5 gene loci in VHL-deficient ILC2s (40). The Intercellular adhesion molecule-1 (ICAM-1) is required for the development of ILC2s, where ICAM-1−/− ILC2s show impaired ERK signaling which leads to the diminished expression of GATA3 and production of type 2 cytokines (41). In the gastrointestinal tract, murine ILC2s were demonstrated to be co-localized with cholinergic neurons expressing the neuropeptide neuromedin U (NMU) that could be recognized by the receptor NMUR1 on ILC2s. NMUR1 is important for the stimulation of the secretion of the type 2 cytokines IL-5, IL-9, and IL-13 in ILC2s (42–44). In addition, the aryl hydrocarbon receptor (Ahr) is expressed in gut ILC2s and has been implicated in controlling chromatin accessibility at the Ahr loci, playing a role in restricting the production of IL-5, IL-13, and amphiregulin via intestinal ILC2s in a cell-intrinsic manner. Ahr inhibits the expression of the IL-33 receptor ST2 in ILC2s, thereby suppressing the transcription factor—growth factor independent 1 transcription repressor (GFI1) (45). Type I interferon was reported to restrict type 2 immunopathology by diminishing type 2 signature cytokine production, cell proliferation and increasing cell death of ILC2s dependent on the interferon stimulated gene factor 3 (ISGF3) (46).
The Functions of ILC2s in the Tumor Microenvironment
ILC2s-Derived Cytokines Regulate the Growth of Tumors
As shown above, ILC2s can secrete cytokines IL-4, IL-5, IL-9, and IL-13. When ILC2s respond to IL-33, the release of high levels of IL-13 can lead to the occurrence of cholangiocyte hyperplasia and epithelial repair. In the bile duct of mice, the induction of the IL-33/ILC2/IL-13 pathway by activated AKT and Yes-associated protein (YAP) further induces cholangiocarcinoma (47). In addition, IL-13 from ILC2s plays a critical role in recruiting myeloid-derived suppressor cells (MDSCs) and M2 phenotype macrophages, which establish an immunosuppressive microenvironment to restrain anti-tumor responses (48). Dendritic Cells (DCs) are potent professional antigen-presentation cells, linking innate immunity with adaptive immunity by presenting tumor antigens to T cells to elicit a specific anti-tumor immunity. IL-13 production by ILC2s is critical for the migration of CD40+ activated DCs from the lung to drainage in the lymph nodes inducing Th2 immunity (49, 50).
During tumor development, the IL-4 receptor α (IL-4α) is overexpressed in many epithelial cancers. Recent studies have found that the production of IL-4 by ILC2s enhances the proliferation and metastasis of epithelial cancer cells (51–55). Other cytokines derived from ILC2s such as IL-5, have also been described to elicit an anti-tumor effect by recruiting eosinophils, which further attract CD4+ T cells and CD8+ T cells by producing chemokine (C-X-C motif) ligand 9 (CXCL9), CXCL10, chemokine (C-C motif) ligand 5 (CCL5) (via STAT1), CCL17 and CCL22 (via STAT6) (48, 56).
The role of IL-9 in tumors is controversial. Deficiency or neutralization of IL-9 appears to promote melanoma growth (57, 58). In contrast, overexpression of IL-9 is related to poor prognosis in some hematopoietic carcinomas (59). Recent study suggests that IL-9, as released by ILC2s, can induce specific cytotoxic T lymphocyte (CTL) responses by enhancing the cross-presentation of DCs and reduce tumor metastasis (60).
Epidermal growth factor receptor (EGFR) antagonists are used in treating epithelial-derived cancers. Interestingly, the ILC2-derived EGFR ligand, Areg, was found to support the growth of lung cancer cells and to inhibit cell apoptosis (12, 61). In the peripheral blood of gastric cancer patients, the increased mRNA expression levels of arginase 1 (ARG1) and inducible nitric oxide synthase (iNOS), which represent immunosuppressive factors, are also correlated with the increased expression of the ILC2-related markers RORα, T1/ST2, IL-17RB, CRTH2, ICOS, CD45, and the signature cytokines IL-13 and IL-5 (62). ILC2-derived Arg1 was also reported to inhibit T cell responses (63). The role of IL-33 in promoting hypoxic TME by inducing aberrant angiogenesis has also been recently highlighted (64). Subsequent to this, the high production of reactive oxygen species (ROS) elicits the expression of chemokine (C-X-C motif) receptor 2 (CXCR2) on tumor cells. Notably, ILC2s-derived CXCL2 has been shown to induce the strong apoptosis of EL4 lymphoma cells via CXCR2 (64).
ILC2s-Mediated Recruitment of Immunosuppressive Cell Subsets
The number of ILC2s increases in TME, and increased IL-13 derived from ILC2s leads to the accumulation of monocytic myeloid-derived suppressor cells (M-MDSCs) to support cancer progression (36, 65). MDSCs can promote tumor growth by expressing multiple pro-angiogenesis characteristics, and they suppress T cells and NK cells by expressing a high level of Arg1, iNOS, and reactive Oxygen species (ROS). IL-13 derived from ILC2s helps to activate Monocytic-MDSCs (M-MDSCs) after stimulation by IL-33 [8, 33], whilst, IL-33 itself can directly elicit the expansion of M-MDSC (66–68). In addition, ILC2s can also induce MDSCs to produce transforming growth factor-β (TGF-β), which contributes to the alternative activation of macrophages (69).
Regulatory T cells (Tregs) manifest their suppressive characteristics by secreting immunosuppressive factors such as IL-10, IL-35, and TGF-β (70), inducing the expression of inhibitory receptors like cytotoxic T lymphocyte antigen 4 (CTLA-4) (70), killing effector cells via granzyme B (71, 72) and perforin (73), interfering with the cellular metabolism, and down-modulating expression of CD80 and CD86 on DCs to support tumor metastasis and evasion from the immune system (74). Previous studies have shown that ILC2s activate Tregs to establish and maintain an immunosuppressive microenvironment through producing Areg, the ligand of epidermal growth factor receptor (EGFR) on Tregs (12, 48, 75). Importantly, ILC2s promote the accumulation of Tregs via the interaction of ICOSL and ICOS, which are both expressed on the surface of ILC2s (76). Nonetheless, Tregs conversely inhibits the function of ILC2s through restraining the binding of ICOS/ICOSL (34, 77).
Macrophages have critical functions in the stroma milieu of tumors. They show clear heterogeneity with different phenotypes appearing upon exposure to different stimuli. Generally, macrophages can be classified into M1 and M2 subsets. Infiltration of M2-like tumor-associated macrophages (TAMs) promotes tumor progression via the suppression of anti-tumor immune responses. Activated ILC2s produce IL-13 which play a key role in the induction of the polarization of the M2 phenotype via the STAT6 signaling pathways, and which contribute to the survival of tumors (12, 66, 77, 78).
Programmed Death-1 (PD-1)/PD-L1 Signaling Restrains the Activation of ILC2s
PD-1 is an inhibitory receptor expressed on the surface of T cells, it is also expressed on the surface of ILC2s (79). The increased PD-1 expression on ILC2s correlates with responses to IL-33. The combination of PD-1 and PD-L1 provides a negative signal which limits the proliferation and cytokine secretion of mature ILC2s by inhibiting the phosphorylation of STAT5. In this way, the immunoregulation functions mediated by mature ILC2s are impaired (35, 80, 81). However, far too little attention has been paid to ILC2-mediated immunomodulation in tumors by PD-1 and PD-L1. More detailed studies are required to investigate such relationships.
Prostaglandin Regulates the Functions of ILC2s
The prostaglandin (PG) D2 receptor, chemoattractant receptor-homologous molecule (CRTH2), is expressed on Th2 lymphocytes and also exists on the surface of human ILC2s (7). The activation and migration of ILC2s, together with the production of type 2 cytokines by ILC2s, can be ascribed to the binding of PGD2 to CRTH2 (24). In addition, ST2 (IL-33 receptor) and IL-17RA (IL-25 receptor) are also expressed on ILC2s (82). Tumor-derived PGD2 and the natural cytotoxicity receptor 3 (NCR3) ligand B7H6, respectively react with CRTH2 and NCR NKp30 expressed on ILC2s (83), inducing ILC2s, to activate M-MDSC by secreting IL-13 which exhibits the immunosuppressive functions mentioned above (4, 37). As for patients with acute promyelocytic leukemia (APL), specifically impairing the immunosuppressive axis PGD2/IL-13/NKp30 can partially decrease the numbers of ILC2s and M-MDSC to ameliorate survival (36). However, PGE2 reduces the proliferation of ILC2s by interacting with the receptors EP2 and EP4 expressed on ILC2s, and so the expression of GATA3 and the secretion of type 2 cytokines can also be restrained (84). As discussed above, different prostaglandins act uniquely on ILC2s to render distinct effects on tumor progression. Thus, prostaglandins have emerged as the potential targets of cancer therapy. However, the function of prostaglandins to control the regulating effect of ILC2s on tumors still needs further in-depth investigation.
Perspectives
In recent years, ILC2s have attracted much attention. They are important players in the modulation of the tumor microenvironment and regulate the host's antitumor immunity (Figure 1). Of note, tumor-associated ILC2 phenotypes have been reported in various types of cancer. However, a better understanding of the interaction between these ILC2s and the TME is required (3). The expression of surface markers on ILC2s is diverse in the context of the distinct TME, indicating a high heterogeneity in ILC2s. It is essential to identify the reliable and specific cell surface markers and to understand how the TME influences the phenotypic heterogeneity and functional diversity of ILC2s. Furthermore, some issues related to the interaction of ILCs with the TME remain to be addressed including those of distinct origins and periods of tumor progression, metabolic cross-talks between tumor and ILC2s, and the interaction between ILC2s and other immune cells in the TME, especially CD8+ T cells.
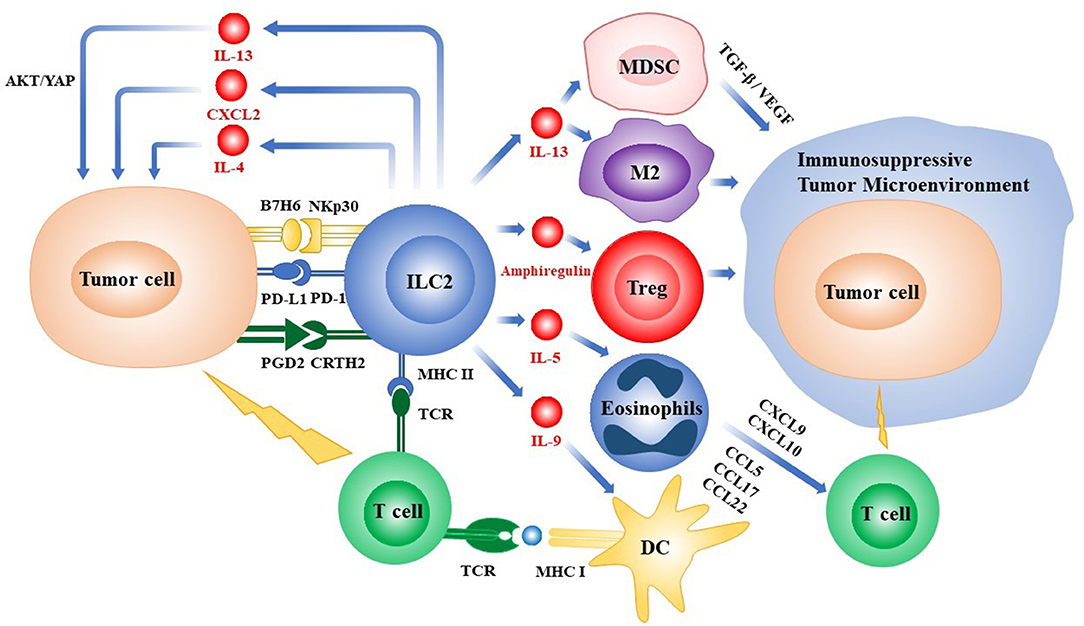
Figure 1. Overview of the roles of ILC2s in the tumor microenvironment. ILC2s can directly regulate the growth and metastasis of tumor cells by secreting cytokines or via the interactions of receptors and ligands expressed on the various types of cells, respectively. ILC2s can also indirectly influence the fates of tumors by recruiting immune cells into the tumor microenvironment or promoting the process of tumor-antigen presentation. ILC2s, group 2 innate lymphoid cells; PD-1/PD-L1, programmed death-1/programmed death-ligand 1; Treg, regulatory T cell; IL, interleukin; MHC, major histocompatibility complex; CXCL, chemokine (C-X-C motif) ligand; CCL, chemokine (C-C motif) ligand; TCR, T cell receptor; DC, dendritic cell; YAP, Yes-associated protein; MDSC, myeloid-derived suppressor cell; M2, M2-like macrophage; TGF-β, transforming growth factor-β; VEGF, vascular endothelial growth factor; PGD2, prostaglandin D2; CRTH2, chemoattractant receptor homologous to the T helper 2 Cells.
Understanding the phenotypic plasticity of ILC2s will be of benefit toward answering many questions relating to conversions among the three groups of the ILC family. When does the ILC conversion occur? Do epigenetic modification mechanisms exist during the process? Will the conversion be clinically meaningful if tumor-promoting ILC2s can adopt an anti-tumorigenic phenotype? Overall, little is known regarding how epigenetic modifications control the unique gene expression patterns and biological functions of ILC2s in the TME. Whether tumor-associated ILC2-derived cytokines or exosomes lead to the formation of the TME or pre-metastatic niche also still remains ambiguous. Future work in the field is required to address these key issues.
Given the preferential distribution of ILC2s in mucosal tissue, and the important role the microbiota plays in homeostasis of immunity, further research relating to crosstalk between ILC2s and the microbiota that reside in the microenvironment of lung cancer, colorectal cancer and gastric cancer may also pave the way for a new strategies of cancer immunotherapy (85).
With the deepening of research, ILC2s may have the potential to be a feasible immunotherapeutic target for optimizing the tumor immunotherapy strategies. This highlights the need to further identify their critical features in the inflammatory tumor microenvironment.
Author Contributions
JX, HW, and JH have participated in editing the article. QW provided intellectual guidance. All the authors have read the final version of the paper and approved it for publication.
Funding
This work was supported by grants from the National Program on Key Basic Research Project (2014CB542101), National Natural Science Foundation of China (81230074, 31870907), Zhejiang Public Welfare Technology Research Project (LGF19H160029).
Conflict of Interest Statement
The authors declare that the research was conducted in the absence of any commercial or financial relationships that could be construed as a potential conflict of interest.
References
1. Eberl G, Colonna M, Di Santo JP, McKenzie AN. Innate lymphoid cells. Innate lymphoid cells: a new paradigm in immunology. Science. (2015) 348:aaa6566. doi: 10.1126/science.aaa6566
2. Diefenbach A, Colonna M, Koyasu S. Development, differentiation, and diversity of innate lymphoid cells. Immunity. (2014) 41:354–65. doi: 10.1016/j.immuni.2014.09.005
3. Chiossone L, Dumas PY, Vienne M, Vivier E. Natural killer cells and other innate lymphoid cells in cancer. Nat Rev Immunol. (2018) 18:671–88. doi: 10.1038/s41577-018-0077-4
4. Artis D, Spits H. The biology of innate lymphoid cells. Nature. (2015) 517:293–301. doi: 10.1038/nature14189
5. Klose CSN, Artis D. Innate lymphoid cells as regulators of immunity, inflammation and tissue homeostasis. Nat Immunol. (2016) 17:765–74. doi: 10.1038/ni.3489
6. Vivier E, Artis D, Colonna M, Diefenbach A, Di Santo JP, Eberl G, et al. Innate lymphoid cells: 10 years on. Cell. (2018) 174:1054–66. doi: 10.1016/j.cell.2018.07.017
7. Spits H, Artis D, Colonna M, Diefenbach A, Di Santo JP, Eberl G, et al. Innate lymphoid cells–a proposal for uniform nomenclature. Nat Rev Immunol. (2013) 13:145–9. doi: 10.1038/nri3365
8. Spits H, Di Santo JP. The expanding family of innate lymphoid cells: regulators and effectors of immunity and tissue remodeling. Nat Immunol. (2011) 12:21–7. doi: 10.1038/ni.1962
9. Wagner M, Moro K, Koyasu S. Plastic heterogeneity of innate lymphoid cells in cancer. Trends Cancer. (2017) 3:326–35. doi: 10.1016/j.trecan.2017.03.008
10. Bernink JH, Krabbendam L, Germar K, de Jong E, Gronke K, Kofoed-Nielsen M, et al. Interleukin-12 and−23 control plasticity of CD127(+) group 1 and group 3 innate lymphoid cells in the intestinal lamina propria. Immunity. (2015) 43:146–60. doi: 10.1016/j.immuni.2015.06.019
11. Huang Y, Guo L, Qiu J, Chen X, Hu-Li J, Siebenlist U, et al. IL-25-responsive, lineage-negative KLRG1(hi) cells are multipotential 'inflammatory' type 2 innate lymphoid cells. Nat Immunol. (2015) 16:161–9. doi: 10.1038/ni.3078
12. Mattner J, Wirtz S. Friend or foe? The ambiguous role of innate lymphoid cells in cancer development. Trends Immunol. (2017) 38:29–38. doi: 10.1016/j.it.2016.10.004
13. Schneider C, Lee J, Koga S, Ricardo-Gonzalez RR, Nussbaum JC, Smith LK, et al. Tissue-resident group 2 innate lymphoid cells differentiate by layered ontogeny and in situ perinatal priming. Immunity. (2019) 50:1425–38.e5. doi: 10.1016/j.immuni.2019.04.019
14. Robinette ML, Fuchs A, Cortez VS, Lee JS, Wang Y, Durum SK, et al. Transcriptional programs define molecular characteristics of innate lymphoid cell classes and subsets. Nat Immunol. (2015) 16:306–17. doi: 10.1038/ni.3094
15. Zhong C, Zhu J. Transcriptional regulators dictate innate lymphoid cell fates. Protein Cell. (2017) 8:242–54. doi: 10.1007/s13238-017-0369-7
16. Serafini N, Vosshenrich CA, Di Santo JP. Transcriptional regulation of innate lymphoid cell fate. Nat Rev Immunol. (2015) 15:415–28. doi: 10.1038/nri3855
17. Bando JK, Liang HE, Locksley RM. Identification and distribution of developing innate lymphoid cells in the fetal mouse intestine. Nat Immunol. (2015) 16:153–60. doi: 10.1038/ni.3057
18. Vivier E, van de Pavert SA, Cooper MD, Belz GT. The evolution of innate lymphoid cells. Nat Immunol. (2016) 17:790–4. doi: 10.1038/ni.3459
19. Gasteiger G, Fan X, Dikiy S, Lee SY, Rudensky AY. Tissue residency of innate lymphoid cells in lymphoid and nonlymphoid organs. Science. (2015) 350:981–5. doi: 10.1126/science.aac9593
20. Huang Y, Mao K, Chen X, Sun MA, Kawabe T, Li W, et al. S1P-dependent interorgan trafficking of group 2 innate lymphoid cells supports host defense. Science. (2018) 359:114–9. doi: 10.1126/science.aam5809
21. Lim AI, Di Santo JP. ILC-poiesis: ensuring tissue ILC differentiation at the right place and time. Eur J Immunol. (2019) 49:11–8. doi: 10.1002/eji.201747294
22. Kotas ME, Locksley RM. Why innate lymphoid cells? Immunity. (2018) 48:1081–90. doi: 10.1016/j.immuni.2018.06.002
23. Bando JK, Colonna M. Innate lymphoid cell function in the context of adaptive immunity. Nat Immunol. (2016) 17:783–9. doi: 10.1038/ni.3484
24. Doherty TA. At the Bench: understanding group 2 innate lymphoid cells in disease. J Leukoc Biol. (2015) 97:455–67. doi: 10.1189/jlb.5BT0814-374R
25. Martinez-Gonzalez I, Matha L, Steer CA, Takei F. Immunological memory of group 2 innate lymphoid cells. Trends Immunol. (2017) 38:423–31. doi: 10.1016/j.it.2017.03.005
26. Martinez-Gonzalez I, Ghaedi M, Steer CA, Matha L, Vivier E, Takei F. ILC2 memory: recollection of previous activation. Immunol Rev. (2018) 283:41–53. doi: 10.1111/imr.12643
27. Mjosberg J, Bernink J, Golebski K, Karrich JJ, Peters CP, Blom B, et al. The transcription factor GATA3 is essential for the function of human type 2 innate lymphoid cells. Immunity. (2012) 37:649–59. doi: 10.1016/j.immuni.2012.08.015
28. Califano D, Cho JJ, Uddin MN, Lorentsen KJ, Yang Q, Bhandoola A, et al. Transcription factor Bcl11b controls identity and function of mature type 2 innate lymphoid cells. Immunity. (2015) 43:354–68. doi: 10.1016/j.immuni.2015.07.005
29. Yu Y, Wang C, Clare S, Wang JX, Lee SC, Brandt C, et al. The transcription factor Bcl11b is specifically expressed in group 2 innate lymphoid cells and is essential for their development. J Exp Med. (2015) 212:865–74. doi: 10.1084/jem.20142318
30. Walker JA, Oliphant CJ, Englezakis A, Yu Y, Clare S, Rodewald HR, et al. Bcl11b is essential for group 2 innate lymphoid cell development. J Exp Med. (2015) 212:875–82. doi: 10.1084/jem.20142224
31. Spooner CJ, Lesch J, Yan D, Khan AA, Abbas A, Ramirez-Carrozzi V, et al. Specification of type 2 innate lymphocytes by the transcriptional determinant Gfi1. Nat Immunol. (2013) 14:1229–36. doi: 10.1038/ni.2743
32. Zook EC, Ramirez K, Guo X, van der Voort G, Sigvardsson M, Svensson EC, et al. The ETS1 transcription factor is required for the development and cytokine-induced expansion of ILC2. J Exp Med. (2016) 213:687–96. doi: 10.1084/jem.20150851
33. Oliphant CJ, Hwang YY, Walker JA, Salimi M, Wong SH, Brewer JM, et al. MHCII-mediated dialog between group 2 innate lymphoid cells and CD4(+) T cells potentiates type 2 immunity and promotes parasitic helminth expulsion. Immunity. (2014) 41:283–95. doi: 10.1016/j.immuni.2014.06.016
34. Maazi H, Patel N, Sankaranarayanan I, Suzuki Y, Rigas D, Soroosh P, et al. ICOS:ICOS-ligand interaction is required for type 2 innate lymphoid cell function, homeostasis, and induction of airway hyperreactivity. Immunity. (2015) 42:538–51. doi: 10.1016/j.immuni.2015.02.007
35. Taylor S, Huang YF, Mallett G, Stathopoulou C, Felizardo TC, Sun MA, et al. PD-1 regulates KLRG1(+) group 2 innate lymphoid cells. J Exp Med. (2017) 214:1663–78. doi: 10.1084/jem.20161653
36. Trabanelli S, Chevalier MF, Martinez-Usatorre A, Gomez-Cadena A, Salome B, Lecciso M, et al. Tumour-derived PGD2 and NKp30-B7H6 engagement drives an immunosuppressive ILC2-MDSC axis. Nat Commun. (2017) 8:593. doi: 10.1038/s41467-017-00678-2
37. Salimi M, Xue LZ, John H, Hardman C, Cousins DJ, McKenzie ANJ, et al. Group 2 innate lymphoid cells express functional NKp30 receptor inducing type 2 cytokine production. J Immunol. (2016) 196:45–54. doi: 10.4049/jimmunol.1501102
38. Halim TYF, Rana BMJ, Walker JA, Kerscher B, Knolle MD, Jolin HE, et al. Tissue-Restricted Adaptive Type 2 Immunity Is Orchestrated by Expression of the Costimulatory Molecule OX40L on Group 2 Innate Lymphoid Cells. Immunity. (2018) 48:1195-1207. doi: 10.1016/j.immuni.2018.05.003
39. Schuijs MJ, Hammad H, Lambrecht BN. Professional and 'amateur' antigen-presenting cells in type 2 immunity. Trends Immunol. (2018)40:22–24. doi: 10.1016/j.it.2018.11.001
40. Li Q, Li DL, Zhang X, Wan QQ, Zhang W, Zheng MK, et al. E3 Ligase VHL promotes group 2 innate lymphoid cell maturation and function via glycolysis inhibition and induction of interleukin-33 receptor. Immunity. (2018) 48:258–70. doi: 10.1016/j.immuni.2017.12.013
41. Lei AH, Xiao Q, Liu GY, Shi K, Yang Q, Li X, et al. ICAM-1 controls development and function of ILC2. J Exp Med. (2018) 215:2157. doi: 10.1084/jem.20172359
42. Wallrapp A, Riesenfeld SJ, Burkett PR, Abdulnour REE, Nyman J, Dionne D, et al. The neuropeptide NMU amplifies ILC2-driven allergic lung inflammation. Nature. (2017) 549:351–6. doi: 10.1038/nature24029
43. Cardoso V, Chesne J, Ribeiro H, Garcia-Cassani B, Carvalho T, Bouchery T, et al. Neuronal regulation of type 2 innate lymphoid cells via neuromedin U. Nature. (2017) 549:277–81. doi: 10.1038/nature23469
44. Klose CSN, Mahlakoiv T, Moeller JB, Rankin LC, Flamar AL, Kabata H, et al. The neuropeptide neuromedin U stimulates innate lymphoid cells and type 2 inflammation. Nature. (2017) 549:282–6. doi: 10.1038/nature23676
45. Li S, Bostick JW, Ye J, Qiu J, Zhang B, Urban JF Jr, et al. Aryl hydrocarbon receptor signaling cell intrinsically inhibits intestinal group 2 innate lymphoid cell function. Immunity. (2018) 49:915–28 e5. doi: 10.1016/j.immuni.2018.09.015
46. Duerr CU, McCarthy CD, Mindt BC, Rubio M, Meli AP, Pothlichet J, et al. Type I interferon restricts type 2 immunopathology through the regulation of group 2 innate lymphoid cells. Nat Immunol. (2016) 17:65–75. doi: 10.1038/ni.3308
47. Li J, Razumilava N, Gores GJ, Walters S, Mizuochi T, Mourya R, et al. Biliary repair and carcinogenesis are mediated by IL-33-dependent cholangiocyte proliferation. J Clin Invest. (2014) 124:3241–51. doi: 10.1172/JCI73742
48. Carrega P, Campana S, Bonaccorsi I, Ferlazzo G. The Yin and Yang of innate lymphoid cells in cancer. Immunol Lett. (2016) 179:29–35. doi: 10.1016/j.imlet.2016.06.003
49. Halim TY, Steer CA, Matha L, Gold MJ, Martinez-Gonzalez I, McNagny KM, et al. Group 2 innate lymphoid cells are critical for the initiation of adaptive T helper 2 cell-mediated allergic lung inflammation. Immunity. (2014) 40:425–35. doi: 10.1016/j.immuni.2014.01.011
50. Saranchova I, Han J, Zaman R, Arora H, Huang H, Fenninger F, et al. Type 2 innate lymphocytes actuate immunity against tumours and limit cancer metastasis. Sci Rep. (2018) 8:2924. doi: 10.1038/s41598-018-20608-6
51. Hallett MA, Venmar KT, Fingleton B. Cytokine stimulation of epithelial cancer cells: the similar and divergent functions of IL-4 and IL-13. Cancer Res. (2012) 72:6338–43. doi: 10.1158/0008-5472.CAN-12-3544
52. Zhang WJ, Li BH, Yang XZ, Li PD, Yuan Q, Liu XH, et al. IL-4-induced Stat6 activities affect apoptosis and gene expression in breast cancer cells. Cytokine. (2008) 42:39–47. doi: 10.1016/j.cyto.2008.01.016
53. Todaro M, Lombardo Y, Francipane MG, Alea MP, Cammareri P, Iovino F, et al. Apoptosis resistance in epithelial tumors is mediated by tumor-cell-derived interleukin-4. Cell Death Differ. (2008) 15:762–72. doi: 10.1038/sj.cdd.4402305
54. Roca H, Craig MJ, Ying C, Varsos ZS, Czarnieski P, Alva AS, et al. IL-4 induces proliferation in prostate cancer PC3 cells under nutrient-depletion stress through the activation of the JNK-pathway and survivin up-regulation. J Cell Biochem. (2012) 113:1569–80. doi: 10.1002/jcb.24025
55. Koller FL, Hwang DG, Dozier EA, Fingleton B. Epithelial interleukin-4 receptor expression promotes colon tumor growth. Carcinogenesis. (2010) 31:1010–7. doi: 10.1093/carcin/bgq044
56. Salome B, Jandus C. Innate lymphoid cells in antitumor immunity. J Leukoc Biol. (2018) 103:479–83. doi: 10.1189/JLB.5MR0617-266R
57. Purwar R, Schlapbach C, Xiao S, Kang HS, Elyaman W, Jiang X, et al. Robust tumor immunity to melanoma mediated by interleukin-9-producing T cells. Nat Med. (2012) 18:1248–53. doi: 10.1038/nm.2856
58. Lu Y, Hong S, Li H, Park J, Hong B, Wang L, et al. Th9 cells promote antitumor immune responses in vivo. J Clin Invest. (2012) 122:4160–71. doi: 10.1172/JCI65459
59. Lange K, Uckert W, Blankenstein T, Nadrowitz R, Bittner C, Renauld JC, et al. Overexpression of NPM-ALK induces different types of malignant lymphomas in IL-9 transgenic mice. Oncogene. (2003) 22:517–27. doi: 10.1038/sj.onc.1206076
60. Kim IK, Kim BS, Koh CH, Seok JW, Park JS, Shin KS, et al. Glucocorticoid-induced tumor necrosis factor receptor-related protein co-stimulation facilitates tumor regression by inducing IL-9-producing helper T cells. Nat Med. (2015) 21:1010–7. doi: 10.1038/nm.3922
61. Busser B, Sancey L, Brambilla E, Coll JL, Hurbin A. The multiple roles of amphiregulin in human cancer. Bba-Rev Cancer. (2011) 1816:119–31. doi: 10.1016/j.bbcan.2011.05.003
62. Bie Q, Zhang P, Su Z, Zheng D, Ying X, Wu Y, et al. Polarization of ILC2s in peripheral blood might contribute to immunosuppressive microenvironment in patients with gastric cancer. J Immunol Res. (2014) 2014:923135. doi: 10.1155/2014/923135
63. Monticelli LA, Buck MD, Flamar AL, Saenz SA, Wojno EDT, Yudanin NA, et al. Arginase 1 is an innate lymphoid-cell-intrinsic metabolic checkpoint controlling type 2 inflammation. Nat Immunol. (2016) 17:656–65. doi: 10.1038/ni.3421
64. Kim J, Kim W, Moon UJ, Kim HJ, Choi HJ, Sin JI, et al. Intratumorally establishing type 2 innate lymphoid cells blocks tumor growth. J Immunol. (2016) 196:2410–23. doi: 10.4049/jimmunol.1501730
65. Chevalier MF, Trabanelli S, Racle J, Salome B, Cesson V, Gharbi D, et al. ILC2-modulated T cell-to-MDSC balance is associated with bladder cancer recurrence. J Clin Invest. (2017) 127:2916–29. doi: 10.1172/JCI89717
66. Jovanovic IP, Pejnovic NN, Radosavljevic GD, Pantic JM, Milovanovic MZ, Arsenijevic NN, et al. Interleukin-33/ST2 axis promotes breast cancer growth and metastases by facilitating intratumoral accumulation of immunosuppressive and innate lymphoid cells. Int J Cancer. (2014) 134:1669–82. doi: 10.1002/ijc.28481
67. Cao XT. Integrative strategy for improving cancer immunotherapy. J Mol Med. (2016) 94:485–7. doi: 10.1007/s00109-016-1424-1
68. Wu YM, Yan YL, Su ZL, Bie QL, Chen XB, Barnie PA, et al. Enhanced circulating ILC2s and MDSCs may contribute to ensure maintenance of Th2 predominant in patients with lung cancer. Mol Med Rep. (2017) 15:4374–81. doi: 10.3892/mmr.2017.6537
69. Gong D, Shi W, Yi SJ, Chen H, Groffen J, Heisterkamp N. TGFbeta signaling plays a critical role in promoting alternative macrophage activation. BMC Immunol. (2012) 13:31. doi: 10.1186/1471-2172-13-31
70. Togashi Y, Shitara K, Nishikawa H. Regulatory T cells in cancer immunosuppression - implications for anticancer therapy. Nat Rev Clin Oncol. (2019) 16:356–71. doi: 10.1038/s41571-019-0175-7
71. Grossman WJ, Verbsky JW, Tollefsen BL, Kemper C, Atkinson JP, Ley TJ. Differential expression of granzymes A and B in human cytotoxic lymphocyte subsets and T regulatory cells. Blood. (2004) 104:2840–8. doi: 10.1182/blood-2004-03-0859
72. Cao X, Cai SF, Fehniger TA, Song J, Collins LI, Piwnica-Worms DR, et al. Granzyme B and perforin are important for regulatory T cell-mediated suppression of tumor clearance. Immunity. (2007) 27:635–46. doi: 10.1016/j.immuni.2007.08.014
73. Grossman WJ, Verbsky JW, Barchet W, Colonna M, Atkinson JP, Ley TJ. Human T regulatory cells can use the perforin pathway to cause autologous target cell death. Immunity. (2004) 21:589–601. doi: 10.1016/j.immuni.2004.09.002
74. Tanaka A, Sakaguchi S. Regulatory T cells in cancer immunotherapy. Cell Res. (2017) 27:109–18. doi: 10.1038/cr.2016.151
75. Zaiss DMW, van Loosdregt J, Gorlani A, Bekker CPJ, Grone A, Sibilia M, et al. Amphiregulin enhances regulatory T cell-suppressive function via the epidermal growth factor receptor. Immunity. (2013) 38:275–84. doi: 10.1016/j.immuni.2012.09.023
76. Molofsky AB, Van Gool F, Liang HE, Van Dyken SJ, Nussbaum JC, Lee J, et al. Interleukin-33 and interferon-gamma counter-regulate group 2 innate lymphoid cell activation during immune perturbation. Immunity. (2015) 43:161–74. doi: 10.1016/j.immuni.2015.05.019
77. Ebbo M, Crinier A, Vely F, Vivier E. Innate lymphoid cells: major players in inflammatory diseases. Nat Rev Immunol. (2017) 17:665–78. doi: 10.1038/nri.2017.86
78. Symowski C, Voehringer D. Interactions between innate lymphoid cells and cells of the innate and adaptive immune system. Front Immunol. (2017) 8:1422. doi: 10.3389/fimmu.2017.01422
79. Mariotti FR, Quatrini L, Munari E, Vacca P, Moretta L. Innate lymphoid cells: expression of PD-1 and other checkpoints in normal and pathological conditions. Front Immunol. (2019) 10:910. doi: 10.3389/fimmu.2019.00910
80. Schwartz C, Khan AR, Floudas A, Saunders SP, Hams E, Rodewald HR, et al. ILC2s regulate adaptive Th2 cell functions via PD-L1 checkpoint control. J Exp Med. (2017) 214:2507–21. doi: 10.1084/jem.20170051
81. Chiossone L, Vivier E. Immune checkpoints on innate lymphoid cells. J Exp Med. (2017) 214:1561–3. doi: 10.1084/jem.20170763
82. Duffin R, O'Connor RA, Crittenden S, Forster T, Yu CJ, Zheng XZ, et al. Prostaglandin E2 constrains systemic inflammation through an innate lymphoid cell-IL-22 axis. Science. (2016) 351:1333–8. doi: 10.1126/science.aad9903
83. Brandt CS, Baratin M, Yi EC, Kennedy J, Gao Z, Fox B, et al. The B7 family member B7-H6 is a tumor cell ligand for the activating natural killer cell receptor NKp30 in humans. J Exp Med. (2009) 206:1495–503. doi: 10.1084/jem.20090681
84. Maric J, Ravindran A, Mazzurana L, Bjorklund AK, Van Acker A, Rao A, et al. Prostaglandin E2 suppresses human group 2 innate lymphoid cell function. J Allergy Clin Immunol. (2018) 141:1761–73 e6. doi: 10.1016/j.jaci.2017.09.050
Keywords: group 2 innate lymphoid cells, tumor microenvironment, cytokines, immunomodulation, cancer immunotherapy
Citation: Xiong J, Wang H, He J and Wang Q (2019) Functions of Group 2 Innate Lymphoid Cells in Tumor Microenvironment. Front. Immunol. 10:1615. doi: 10.3389/fimmu.2019.01615
Received: 05 April 2019; Accepted: 28 June 2019;
Published: 10 July 2019.
Edited by:
Khashayarsha Khazaie, Mayo Clinic College of Medicine & Science, United StatesReviewed by:
Kelly M. Mcnagny, University of British Columbia, CanadaPaula D. Bos, Virginia Commonwealth University, United States
Copyright © 2019 Xiong, Wang, He and Wang. This is an open-access article distributed under the terms of the Creative Commons Attribution License (CC BY). The use, distribution or reproduction in other forums is permitted, provided the original author(s) and the copyright owner(s) are credited and that the original publication in this journal is cited, in accordance with accepted academic practice. No use, distribution or reproduction is permitted which does not comply with these terms.
*Correspondence: Qingqing Wang, d3FxQHpqdS5lZHUuY24=