- 1Department of Microbiology and Immunology, Kunming Medical University, Kunming, China
- 2Department of Biochemistry and Molecular Biology, Kunming Medical University, Kunming, China
- 3Yunnan Province Key Laboratory for Tropical Infectious Diseases in Universities, Kunming Medical University, Kunming, China
- 4The Institute for Tropical Medicine, Kunming Medical University, Kunming, China
- 5Yunnan Province Key Laboratory for Major Childhood Diseases, Kunming Medical University, Kunming, China
Background: Currently, there is no tuberculosis (TB) vaccine recommended for use in latent TB infections and healthy adults. M72/AS01E is a new peptide vaccine currently under development, which may improve protection against TB disease. This vaccine has been investigated in several phase I/II clinical trials. We conducted a meta-analysis to clarify the immunogenicity and safety of the M72/AS01E peptide vaccine.
Methods: We searched the PubMed, Embase, and Cochrane Library databases for published studies (until December 2018) investigating this candidate vaccine. A meta-analysis was performed using the standard methods and procedures established by the Cochrane Collaboration.
Results: Seven eligible studies—involving 4,590 participants—were selected. The analysis revealed a vaccine efficacy was 57.0%, significantly higher abundance of polyfunctional M72-specific CD4+ T cells [standardized mean difference (SMD) = 2.58] in the vaccine group vs. the control group, the highest seropositivity rate [relative risk (RR) = 74.87] at 1 month after the second dose of vaccination (Day 60), and sustained elevated anti-M72 IgG geometric mean concentration at study end (Day 210) (SWD = 4.94). Compared with the control, participants who received vaccination were at increased risk of local injection site redness [relative risk (RR) = 5.99], local swelling (RR = 7.57), malaise (RR = 3.01), and fatigue (RR = 3.17). However, they were not at increased risk of headache (RR = 1.57), myalgia (RR = 0.97), and pain (RR = 3.02).
Conclusion: The M72/AS01E vaccine against TB is safe and effective. Although the vaccine is associated with a mild adverse reaction, it is promising for the prevention of TB in healthy adults.
Introduction
Tuberculosis (TB) is the deadliest infectious disease worldwide. In 2017, there were an estimated 10 million new cases of TB and 1.6 million deaths caused by the disease (1). Treatment of latent TB infections (LTBI) and vaccination of children with the bacillus Calmette–Guérin (BCG) vaccine are the currently available effective interventions for the prevention of new infections with Mycobacterium tuberculosis (M. tuberculosis) and progression to TB disease. The only licensed BCG vaccine against TB induces protective memory that lasts for 10–20 years (1–3). However, BCG does not offer substantial protection against M. tuberculosis in adolescents and adults. Notably, the timeframe for the waning of BCG-induced protection throughout childhood and early adult life coincides with a gradual increase in the incidence of TB (4). In recent years, the incidence of TB has increased due to human immunodeficiency virus (HIV)/TB co-infection, and the emergence of multidrug-resistant TB and extremely drug-resistant TB. Therefore, the development of a novel safe and effective TB vaccine is urgently warranted.
The M72/AS01E candidate vaccine is composed of the M72 antigen—a recombinant fusion protein derived from the two highly immunogenic M. tuberculosis antigens Mtb32A (Rv0125 encoding PepA) (5) and Mtb39A (Rv 1196 encoding PPE 18) (6)—and the liposome-based AS01E. Mtb32A and Mtb39A—present in both M. tuberculosis and BCG—were selected based on their ability to stimulate T-cell responses (5–9). AS01E contains two immunostimulants, namely monophosphoryl lipid A and Quillaja saponaria Molina fraction 21, which known to mediate cytotoxic lymphocyte cells and TLR4 receptors (10). The M72/AS01E has shown promising results in clinical trials involving adults and adolescents infected with M. tuberculosis. These studies demonstrated that the vaccine exhibited a clinically acceptable safety profile and elicited high magnitude M72-specific humoral and CD4+ T-cell responses (11–17). The purpose of the present analysis was to evaluate the safety and immunogenicity of two doses of the M72/AS01E vaccine in HIV-positive (HIV+) or HIV-negative (HIV−) M. tuberculosis-infected adults and adolescents, as well as BCG-vaccinated infants.
Methodology
Eligibility Criteria
In this analysis, we evaluated randomized clinical trials (RCTs) of all phases investigating the M72/AS01E candidate TB vaccine and including a control group (e.g., placebo, adjuvant, or other vaccines). The selection criteria for studies included the evaluation of at least one outcome related to efficacy, immunogenicity, and safety of the vaccine in different populations, and the intramuscular administration of two doses of M72/AS01E or control (1 month apart). Simultaneously, our populations of interest involved HIV-infected or TB-infected individuals. Of note, we excluded studies that did not report outcomes of interest and other types of research. The first outcome of interest was the serotype-specific M72/AS01E antibody response which was considered protective. The secondary outcome was the occurrence of adverse effects related to the candidate vaccine.
Literature Search and Data Extraction
A sensitive and systematic search was performed by two independent investigators (Z.H.J. and M.M.J.). A comprehensive literature search of the PubMed, Embase, and Cochrane Library databases was conducted to identify articles published until December 2018. The key search terms were “M72/AS01E” or “M72/AS01” or “tuberculosis vaccine” and “immunogenicity” or “safety.” The language was restricted to English (Supplementary Material 1).
Data extraction was performed by two investigators based on the predefined inclusion and exclusion criteria. A data extraction sheet was developed based on the Cochrane Handbook for Systematic Reviews of Intervention (18) and Meta-analyses (PRISMA) guidelines (Supplementary Material 2) (19). A third investigator (Z.D.) analyzed any dissimilar results to resolve discrepancies. The data extracted included the name of the first author, date of publication, experimental design, country, study population, age, gender, follow-up, groups, immunogenicity, and safety.
Quality of Evidence and Risk of Bias
We assessed the risk of bias for each included study using the methodology established by the Cochrane Collaboration (20). The extent to which a Cochrane review can draw conclusions regarding the effects of an intervention depends on the validity of the data obtained from the included studies. This comprises a judgement and support of the judgement for each entry in a “risk of bias table,” in which each entry addresses a specific feature of the study. The judgement for each entry determines the risk of bias as “low risk,” “high risk,” or “unclear risk.” The last category indicates either lack of information or uncertainty over the potential for bias.
Statistical Analysis
The data were collected using Microsoft Excel (Microsoft Corp. Albuquerque, NM, USA). The Stata/SE (StataCorp, College Station, TX, USA), RevMan 5.2 (The Cochrane Collaboration, Cochrane organizations, London, UK) and GraphPad Prism 6 (GraphPad Software Inc., San Diego, CA, USA) software were used for the statistical analyses. Stata/SE was used for the meta-analysis and assessment of heterogeneity. The results were reported as relative risk (RR) and standardized mean difference (SMD) with 95% confidence intervals (95%CI). The Q and I2 tests were used to evaluate the statistical heterogeneity among studies. A P < 0.1 or I2 > 50% indicated statistically significant heterogeneity, which could be explored through regression analysis. A forest plot and funnel plot were generated to judge the overall effect size and determine the presence of publication bias. The Begg's funnel plot and Egger's test were employed for the assessment of potential publication bias. An Egger's test P < 0.05 was interpreted as statistically significant. Influence analysis was performed to determine the robustness of the meta-analysis. For trials including more than one treatment/control group, we used the data obtained for the combined treatment/control groups. Publication bias was evaluated using the RevMan 5.2 software and shown in the risk of bias summary diagram. Application of GraphPad Prism 6 to difference Statistics and drawing figures among groups.
Role of the Funding Source
The funder had no role in study design, data collection, data analysis, data interpretation, or writing of the report. The corresponding author had full access to all data in the study and had final responsibility for the decision to submit for publication.
Results
Study Characteristics
Of the 3,676 reports identified through the systematic search, 2,441 duplicates were removed. Subsequently, we screened the titles and abstracts of the remaining studies. Of the 156 titles screened, the abstracts of 14 reports were assessed. Eventually, seven studies satisfied the standard eligibility criteria (double-blinded, two- or three-arm RCTs) (11–17). The process of study selection is illustrated in Figure 1. The main characteristics of the included studies are presented in Table 1.
Methodological Quality and Risk of Bias
Methodological quality and risk of bias were evaluated using the tool established by the Cochrane Collaboration for assessing the risk of bias. This is a two-part tool, addressing the following seven specific domains: sequence generation, allocation concealment, blinding of participants and personnel, blinding of outcome assessment, incomplete outcome data, selective outcome reporting, and “other issues.” The Cochrane Handbook provides criteria for judging the risk of bias based on the seven domains included in the tool. All included trials presented a low risk of bias for important aspects, such as random sequence generation, allocation concealment, and selective reporting. All studies followed this protocol. Unfortunately, one study did not report complete data, which resulted in a high risk of bias (Supplementary Material 3).
Evaluation of Immunogenicity
Polyfunctional M72-Specific CD4+ T-Cell
Polyfunctional M72-specific CD4+ T-cells were defined as cells expressing more than two immune markers among cytokines IFN-γ, IL-2, TNF-α, CD40L, IL-17, and IL-13. The meta-analysis was performed by comparing the polyfunctional CD4+ T-cells of the vaccine group with those of the control group. The overall mean value of CD4+ T-cells was transformed using the natural logarithm (ln) form at different times (Figure 2A). Significant heterogeneity was reported (I2 > 50% and P < 0.1); thus, a random effects model was used.
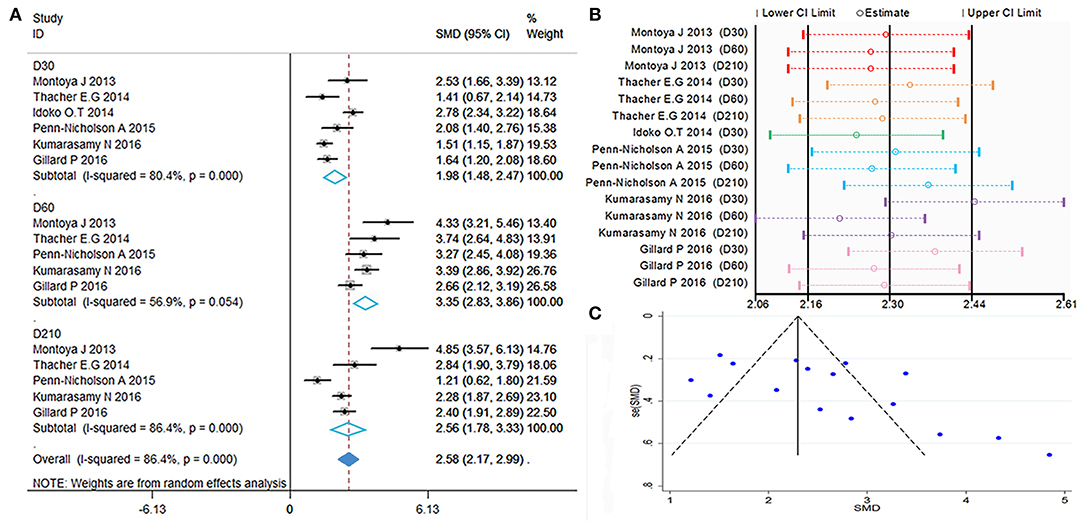
Figure 2. Polyfunctional M72-specific CD4+ T-cell evaluation. (A) Forest plot: a SMD > 0 indicates that vaccine can effectively stimulate the growth of polyfunctional CD4+ T-cells. SMD = 0, invalid result. Point estimates and 95%CI are shown for each study and for the pooled results. (B) Influence analysis, examine the impact of a study on the total combined effect. The vertical solid line in the middle indicates the total combined effect, while the left and right vertical solid lines indicate 95%CI. (C) Funnel plot: the publication bias was independently assessed by determining the symmetrical distribution of the studies.
The results showed a significant difference between the vaccinated and non-vaccinated groups in the number of polyfunctional CD4+ T cells at 30 days (D30) after the first dose, 60 days (D60) after the first dose (i.e., 30 days after the second dose), and 210 days (D210) after the first dose. The abundance of polyfunctional CD4+ T cells was higher than that reported pre-vaccination at D30 (SMD = 1.98, 95%CI = 1.48–2.47, I2 = 80.4%, P = 0.000), with significant boosting D60 (SMD = 3.35, 95%CI = 2.83–3.86, I2 = 56.9%, P = 0.054). Furthermore, the vaccinated group maintained higher levels of cytokine expression at D210 (SMD = 2.56, 95%CI = 1.78–3.33, I2 = 86.4%, P = 0.000). With the exception of D60, the remaining time points presented high heterogeneity.
The forest plot and funnel plot showed that the studies were symmetrically distributed (Figures 2A,C). In addition, the publication bias test showed that there was no statistically significant publication bias (Table 2). However, based on the results of the influence analysis, several articles affecting the stability of the results were identified. After removing these studies, the combined effect point estimates fell outside the total combined effect value (Figure 2B). Moreover, the regression analysis explained the main heterogeneity observed at D60 due to differences among studies. In addition, the meta-regression analysis revealed a relationship between different ages and heterogeneity at D30. The I2 was reduced to 20.41%, while the covariate was age (Table 3).
Polyfunctional M72-Specific CD8+ T-Cell
As previously defined, polyfunctional M72-specific CD8+ T-cells also express at least two immune markers of IFN-γ, IL-2, TNF-α, CD40L, IL-17, or IL-13. The initial data of polyfunctional CD8+ T-cells were transformed using the natural logarithm (ln), then SMD calculated, the polyfunctional CD8+ T-cells of the vaccine group with those of the control group were compared. The random effects model was applied, because significant heterogeneity was reported (I2 > 50% and P < 0.1).
The results are showed in Figure 3A, SMDs all contains zero at every time point, which means the number of polyfunctional CD8+ T-cells has no change between the vaccinated and non-vaccinated groups. Our influence analysis to the study by Idoko et al. pointed out a great influence on the total combined effect (Figure 3B), publication bias analysis showed that several studies are biased from symmetrical in the funnel plot (Figure 3C). Therefore, we did the Begg's test and Egger's test analysis, but results did not show the publication bias (Table 2). In order to find out the source of heterogeneity, we did regression analysis, the results showed that age (D30) could reduce I2 value in all kinds of covariables (Table 3).
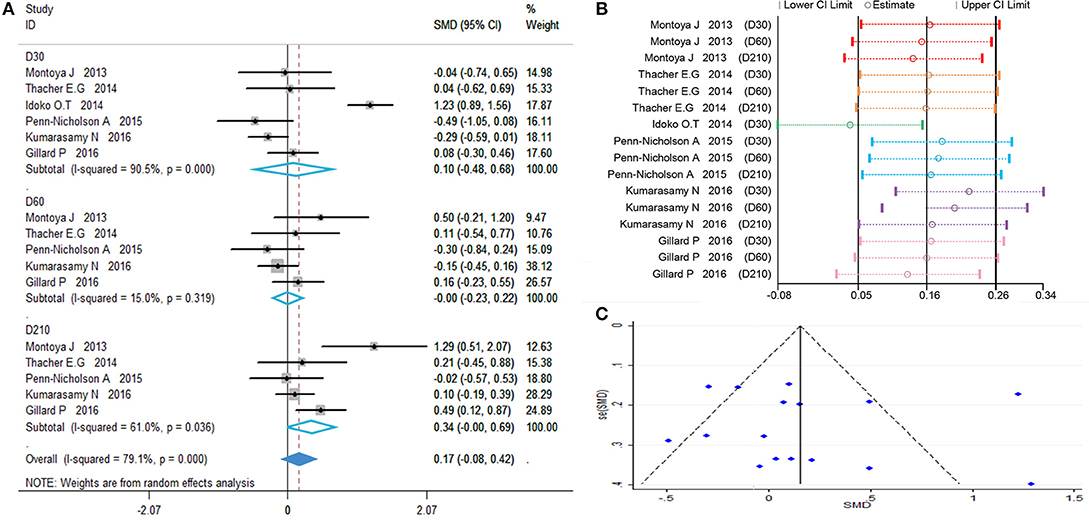
Figure 3. Polyfunctional M72-specific CD8+ T-cell evaluation. (A) Forest plot: a SMD > 0 indicates that vaccine can effectively stimulate the growth of polyfunctional CD8+ T-cells. SMD = 0, invalid result. Point estimates and 95%CI are shown for each study and for the pooled results. (B) Influence analysis, examine the impact of a study on the total combined effect. The vertical solid line in the middle indicates the total combined effect, while the left and right vertical solid lines indicate 95%CI. (C) Funnel plot: the publication bias was independently assessed by determining the symmetrical distribution of the studies.
Anti-M72 IgG Seropositivity Rate
Anti-M72 IgG seropositive subjects were defined as titers ≥2.8 enzyme-linked heterogeneity among the included studies (I2 < 50%, P > 0.1). Therefore, the fixed effect model was applied for the meta-analysis. The best results for the serotype-specific immune response were obtained 30 days after the second dose of M72/AS01E (D60) (RR = 74.87, 95%CI = 33.04–169.81, I2 = 36.2%, P = 0.166), while the seropositivity rate RR at D60 was 74.87% (Figure 4A). These findings revealed that the probability of publication bias was exist (Table 2, Figures 4B,C). In addition, the funnel plot showed that the studies were not generally symmetrically distributed (Figure 4C).
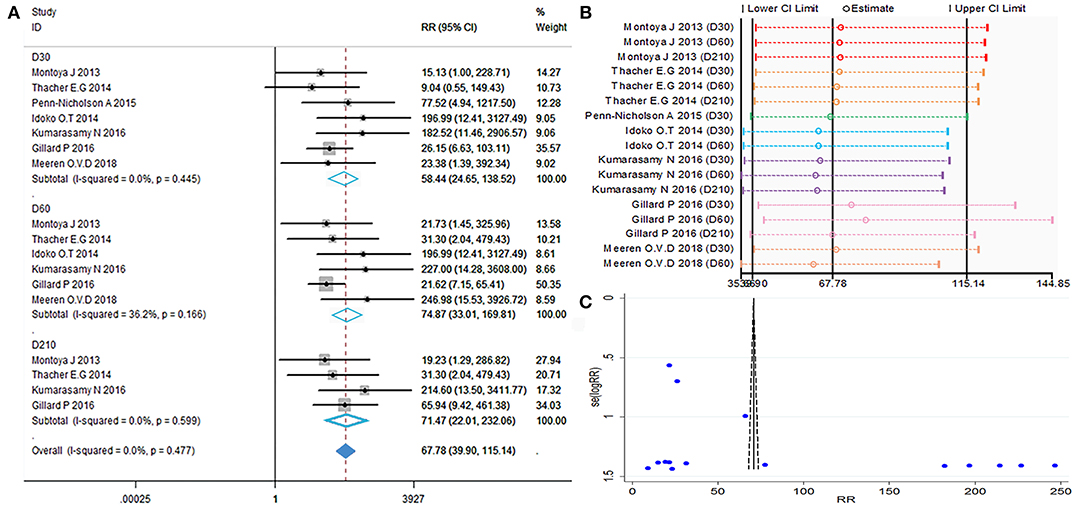
Figure 4. Anti-M72 IgG seropositivity rate. (A) Forest plot: a RR >1 indicates that the vaccine was protective. RR = 1, invalid result. Point estimates and 95%CI are shown for each study and for the pooled results. (B) Influence analysis, examine the impact of a study on the total combined effect. The vertical solid line in the middle indicates the total combined effect, while the left and right vertical solid lines indicate 95%CI. (C) Funnel plot: the publication bias was independently assessed by determining the symmetrical distribution of the studies.
Anti-M72 IgG Geometric Mean Concentration (GMC)
Calculations of the anti-M72 IgG GMC were performed by taking the anti-ln of the mean of the concentration transformations. Because the heterogeneity test showed P = 0.000 with I2> 50%, the random effects model was applied (Figure 5). The results showed that all time point effect size was high contrast and that the second dose was the highest titer (D30 SMD = 3.41, 95%CI = 1.67–5.16, I2 = 97.9%, P = 0.000; D60 SMD = 5.99, 95%CI = 4.62–7.73, I2 = 87.6%, P = 0.000; D210 SMD = 4.94, 95%CI = 3.53–6.34, I2 = 90.6%, P = 0.000).
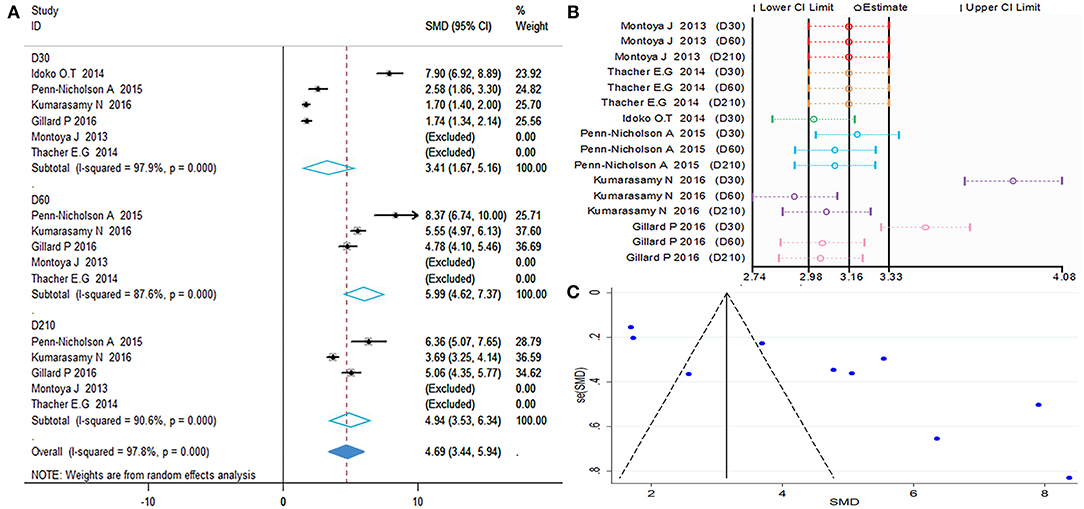
Figure 5. Anti-M72 IgG geometric mean concentration(GMC). (A) Forest plot: SMD >0 indicates that the vaccine was protective. SMD = 0, invalid result. Point estimates and 95%CI are shown for each study and for the pooled results. (B) Influence analysis, examine the impact of a study on the total combined effect. The vertical solid line in the middle indicates the total combined effect, while the left and right vertical solid lines indicate 95%CI. (C) Funnel plot: the publication bias was independently assessed by determining the symmetrical distribution of the studies.
As shown in Figures 5A,C, the data presented by Kumarasamy N (D30, D60) (15) and Gillard P (D30) (16) significantly affected the stability of the results. The Begg and Egger tests did not show significant publication bias for the studies included in the summary analysis (Table 2). Although the regression analysis did not identify the main causes of high heterogeneity (Table 3), negligible publication bias is not a major factor. Finally, in this study, we included control subjects who received a non-adjuvanted vaccine, alum-adjuvanted vaccine, or placebo. Thus, the level of reactogenicity in the control groups may be affected.
Evaluation of Safety
The local and systemic toxicity associated with the M72/AS01E vaccine were evaluated in six studies (11–16). Grade 1 local and systemic toxicities were observed in most participants, whereas grade 3 toxicities were noted in some cases. Injection site reactions observed after vaccination with M72/AS01E were redness (RR = 5.99, 95%CI = 1.45–24.85, I2 = 11.9%, P = 0.287), swelling (RR = 7.57, 95%CI = 2.19–26.81, I2 = 0.0%, P = 0.594). Adverse events (AEs) occurred more frequently in the vaccine group compared with control. The most frequently reported toxicity AEs were malaise (RR = 3.01, 95%CI = 1.02–8.92, I2 = 0.0%, P = 0.997) and fatigue (RR = 3.17, 95%CI = 1.43–7.05, I2 = 0.0%, P = 0.651). There were no differences detected between the M72/AS01E and control arms in terms of headache (RR = 1.57, 95%CI = 0.71–3.50, I2 = 51.7%, P = 0.102), myalgia (RR = 0.97, 95%CI = 0.14–6.62, I2 = 61.0%, P = 0.077) and pain (RR = 3.02, 95%CI = 0.64–14.14, I2 = 87.4%, P = 0.000).
The meta-analysis of safety is shown in Figures 6A,B. The funnel plot (Figure 6C) and Table 2 show that evaluation of safety publication bias was excluded. Although the source of heterogeneity could not be definitively identified, it may be related to age or gender (Table 3). Individuals of different ages and genders exhibit different subjective judgments of headache and myalgia, and normal incidence is also different. This needs to be taken into consideration when interpreting the results of such evaluations.
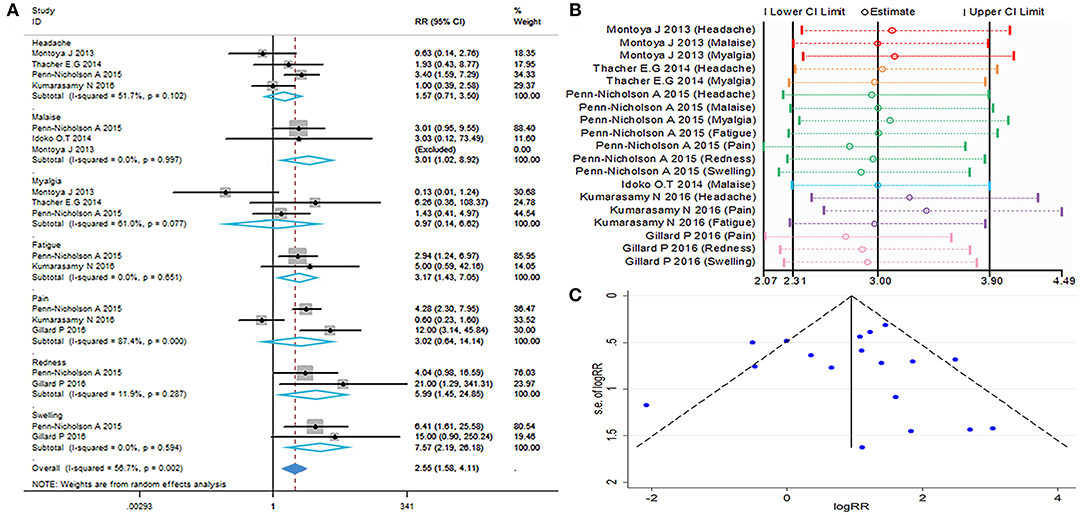
Figure 6. Safety evaluation. (A) Forest plot: a RR >1 indicates that the vaccine was protective, the result of intersection with the intermediate invalid line was invalid. RR = 1, invalid result. Point estimates and 95%CI are shown for each study and for the pooled results. (B) Influence analysis, examine the impact of a study on the total combined effect. The vertical solid line in the middle indicates the total combined effect, while the left and right vertical solid lines indicate 95%CI. (C) Funnel plot: the publication bias was independently assessed by determining the symmetrical distribution of the studies.
Subgroup Analysis
We considered that HIV-positive and HIV-negative individuals have different immunity responses to vaccines and may have an impact on the results, so we performed a subgroup analyses. Six publications included in this meta-analysis compared immune responses after vaccination in HIV-positive and HIV-negative patients, among them, two studies are related to the HIV-positive population.
We examined the kinetic changes of polyfunctional CD4+ T-cell and polyfunctional CD8+ T-cell counts both in HIV-positive and HIV-negative individuals (Figure 7G). At D30 after the first dose, compared with contrast, polyfunctional CD4+ T-cell counts in HIV-positive and HIV-negative populations both were higher (p < 0.05). The elevation in polyfunctional CD4+ T-cell counts was most prominent at D60 after the first dose, polyfunctional CD4+ T-cell counts both in HIV-positive and HIV-negative were higher than contrast (p < 0.01). At D210 after the first dose, polyfunctional CD4+ T-cell counts became relatively stable (p < 0.01), the count of polyfunctional CD4+ T-cell both in HIV-positive and HIV-negative were always higher than contrast. Importantly, there is no statistical difference in the count of polyfunctional CD4+ T-cell between HIV-positive and HIV-negative during vaccination (p > 0.05). At every time point, the number of polyfunctional CD8+ T-cell were no statistical difference between HIV-positive and HIV-negative individuals (p > 0.05). The corresponding forest map also showed no significant difference between the two groups, and the heterogeneity did not decrease after subgrouping (Figures 7A–F).
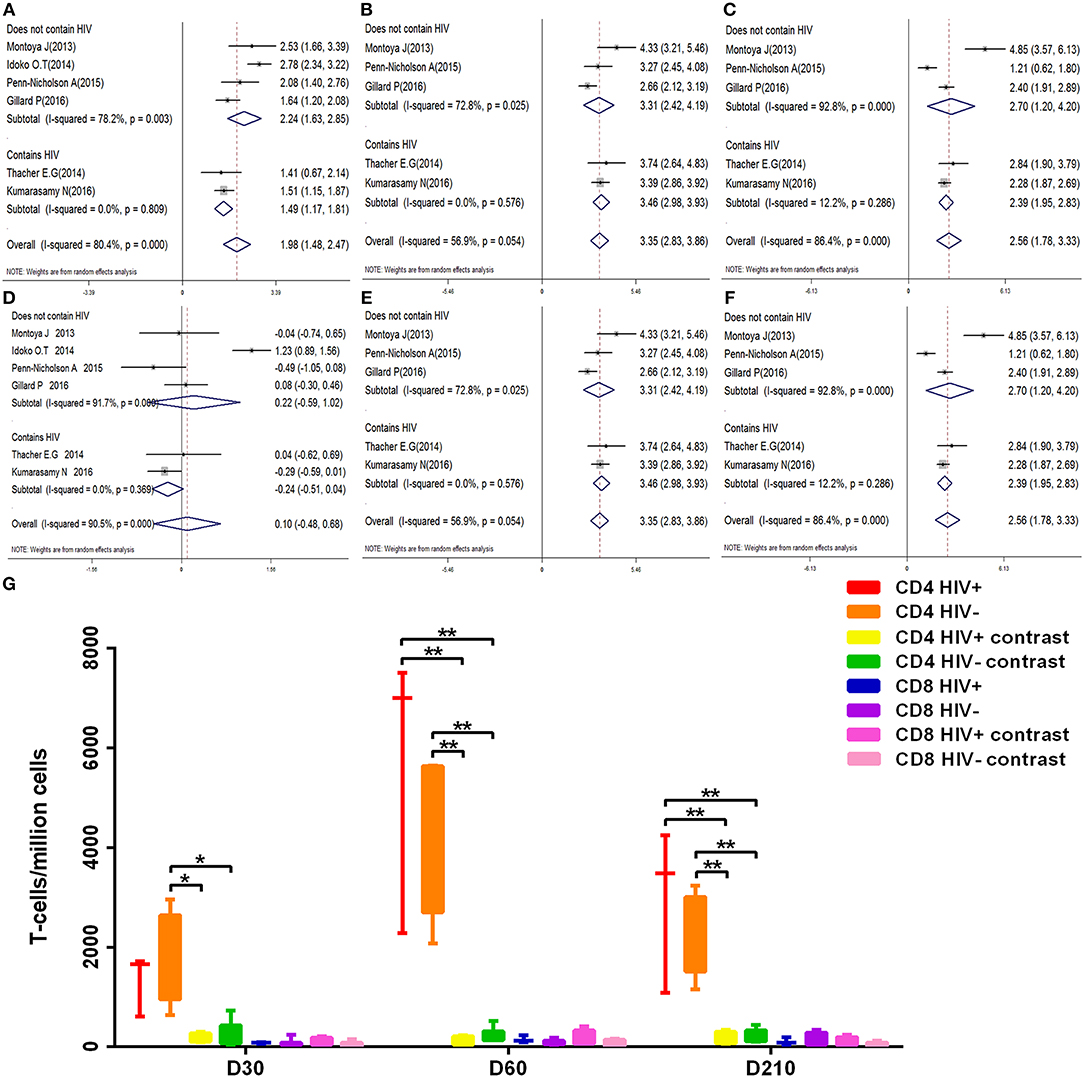
Figure 7. Subgroup analysis polyfunctional CD4+ and CD8+ T-cell. (A–F) Forest plot: a SMD > 0 indicates that vaccine can effectively stimulate the growth of polyfunctional CD4+ or CD8+ T-cells. SMD = 0, invalid result. (A–C) are the result of polyfunctional CD4+ T-cell analysis of D30, D60, and D210, respectively. (D–F) are the result of polyfunctional CD4+ T-cell analysis of D30, D60, and D210, respectively. (G) Box diagram: The transverse coordinates are the date of vaccination. The longitudinal coordinates are the number of polyfunctional CD4+ or CD8+ T-cells. Statistically significant differences between the two groups are indicated by star symbols: *p < 0.05; **p < 0.001.
Similarly, the anti-M72 IgG GMC was most prominent at D60 after the first dose, anti-M72 IgG GMC both in HIV-positive and HIV-negative groups were higher (p < 0.01) (Figure 8D) in comparison with contrast. At every time point, there is no statistical difference in the anti-M72 IgG GMC between HIV-positive and HIV-negative groups. In short, The results of the subgroup analyses were also similar (Figures 8A–C).
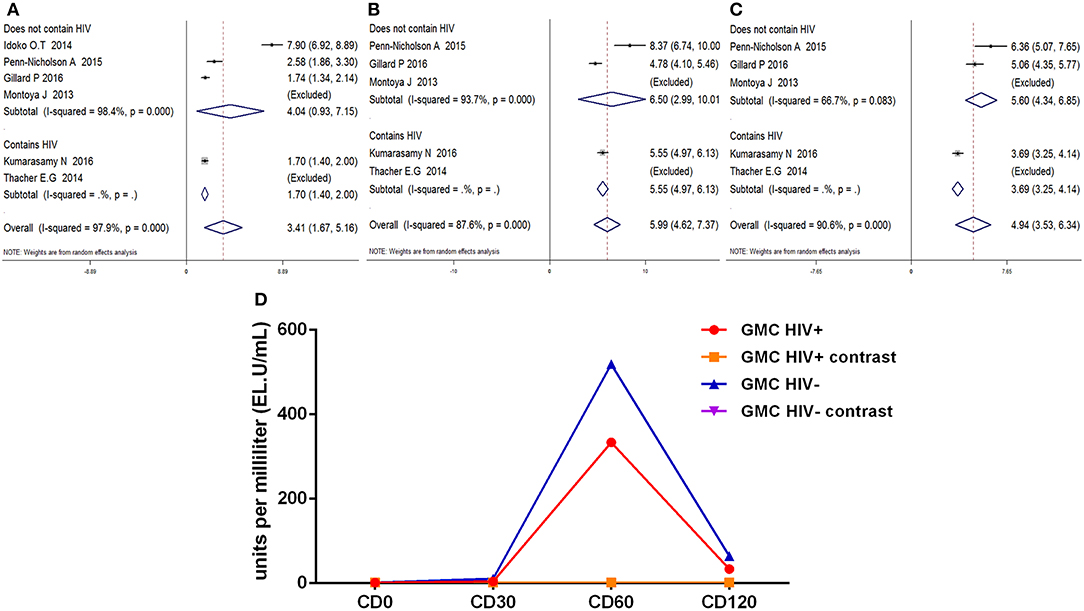
Figure 8. Subgroup analysis Anti-M72 antibody IgG geometric mean concentration(GMC). (A–C) Forest plot: SMD >0 indicates that the vaccine was protective. SMD = 0, invalid result. Point estimates and 95%CI are shown for each study and for the pooled results. (D) Line chart: the anti-M72 IgG GMC varies (Ordinate) with time in different time points (Abscissa) after vaccination.
Discussion
The elimination of TB has been partly hindered by the ability of M. tuberculosis to remain dormant in the human body for years without causing disease—a state referred to as LTBI (21). It is estimated that approximately one quarter of the global population are infected with M. tuberculosis. Of those, 5–10% will develop TB disease during their lifetime (1). Although the majority of infected individuals are asymptomatic, they develop a strong acquired immune response to the pathogen (22). Thus, the prevention and treatment of LTBI is currently the focus of ongoing research. Eradication of TB requires a highly efficacious TB vaccine. The evaluation of immunogenicity and safety of the M72/AS01E candidate vaccine may be provide rationale for further large-scale efficacy trials of M72/AS01E vaccine.
This study is the first systematic review and meta-analysis evaluating the safety and immunogenicity of candidate vaccine M72/AS01E in complex individuals. In the examined data, we did not identify serious AEs related to the M72/AS01E vaccine or safety concerns. The results of our meta-analysis showed that the incidence of local AEs (i.e., pain) or general AEs (i.e., headache and myalgia) were low during a 6-month follow-up study. However, redness, swelling, fatigue, and malaise were significantly higher in the vaccinated group vs. the placebo group. For the M72/AS01E vaccine, solicited AEs were primarily mild to moderate in intensity and transient. These local or general AEs were limited in duration (most resolved within a few days) and there was no requirement for specific diagnostic or therapeutic interventions. The AE profiles associated with M72/AS01E were clinically acceptable in antiretroviral therapy (ART)-stable and ART-naive HIV-infected individuals. Therefore, the use of M72/AS01E vaccine for the prevention of TB is safe.
In vaccine clinical trials, the abundance of antigen-specific T cells and antibody concentrations are often used to explain the response to vaccination (23). Various kinds of preclinical studies have demonstrated that humoral immunity may play a part in the protection against M. tuberculosis (24–26). Our results showed that two-dose M72/AS01E vaccination induced higher seroconversion rates vs. placebo. Meanwhile, there was a sharp increase in the seroconversion rates after the second vaccine dose compared with the first. Kumarasamy et al. reported that M72-specific antibodies induced through vaccination with M72/AS01E persisted for a maximum of 3 years (27). Notably, two-dose vaccination offered more durable long-term protection. Moreover, the AS01 adjuvant system is a component of the RTS, S/AS01 malaria vaccine (28–30) and recombinant zoster vaccine (31) (both currently investigated in phase III studies). Due to its association with improvement in adaptive immune responses (humoral and cellular), AS01E may promote increases in Ag-specific antibody responses, cytokine release and levels of costimulatory molecules in humans (32). Use of adjuvants is required to induce the most potent immune responses. Therefore, the use of a potent adjuvant such as AS01E may allow the reduction of antigen doses (i.e., antigen sparing effect).
Based on preclinical studies, the protection against M. tuberculosis is mediated by antigen-specific polyfunctional CD4+ T cells (33–37). Although immune correlates of protection against TB have not been defined (38), IFN-γ, TNF-a, IL-2, and IL-17 are important for the control of mycobacterial infection (39–41). IFN-γ and TNF-α can activates infected macrophages, respectively, which in turn inhibit M. tuberculosis growth by inducing iNOS and autophagy (42, 43). Moreover, IFN-γ and TNF-α synergistically mediate killing of pathogens (42). IL-2 induces CD4+ and CD8+ T cells proliferation and differentiation, and promotes the development of memory T cells during primary infection. IL-17 plays an important antimicrobial pro-inflammatory role in the stages of M. tuberculosis infection by inducing neutrophil generation, stimulate cytokine production (44). Studies have shown that polyfunctional IFN-γ+ IL-2+ TNF-α+ CD4+ T cells may produce higher levels of each cytokine on a per-cell basis, compared with other T cells (37, 45).
A notable feature of M72/AS01E is the complexity of the induced T-cell responses. A variety of distinct cytokine-producing populations of M72-specific CD4+ T-cell responses were induced after vaccination, including a discrete population of Th17 cells (46). The M72/AS01E vaccine-induced CD4+ T-cell responses were strongly Th1-biased (7, 11, 47). Thus, the immunogenicity of TB candidate vaccines is typically expressed in terms of magnitudes of the induced specific CD4+ T-cell responses expressing Th1-type cytokines. Similarly, evidence showed that vaccination with M72/AS01E induced robust polyfunctional M72-specific CD4+ T cells, which persisted for 3 years. This response was significantly boosted through a second vaccination, indicating the presence of effector memory T cells. By comparing it with other candidate vaccines, M72/AS01E induced higher memory Th1 cytokine-expressing CD4 T cell responses than other novel vaccine (48).
In a small study, the abundance of polyfunctional M72-specific CD4+ T cells expressing CD40L+ TNF-α+ IFN-γ+, CD40L+ IL-2+ TNF-α+ IFN-γ+, or CD40L+ IFN-γ+ profiles was higher in the TB-treatment (adults receiving treatment for TB disease who have completed the intensive phase of treatment) and TB-treated (adults with previous history of successfully treated pulmonary TB disease groups) vs. the TB-naïve (adults who never had TB) group (16). This finding is consistent with results showing that patients with sputum smear+ TB exhibited decreased polyfunctional IFN-γ+ IL-2+ TNF-α+ and IL-2-producing specific CD4+ T cells and recovery of the number of T cells during therapy vs. those with sputum smear− TB and LTBI (49). These data indicate that the functional capacity of M. tuberculosis-specific T cells elicited by the M72/AS01E vaccine may also be progressively impaired with an increased mycobacterial load—subsequently recovering during therapy.
The abundance of M72-specific CD4+ T cells expressing IL-17 was low and did not co-express Th1 cytokines. In this analysis, almost no M72-specific IL-13+CD4+ T-cell responses were detected (data not shown). In humans, Th2-type responses (i.e., IL-4, IL-5, and IL-13) correlate with TB immunopathology and suppress the production of IFN-γ and TNF-α. Maintaining the balance between the Th1/Th2 responses may contribute to the control of M. tuberculosis infection. Of note, M72/AS01E vaccine-induced M72-specific CD8+ T-cell responses were low and did not increase significantly over time in M72/AS01E recipients (50).
The clinically acceptable safety, the potent and sustained T-cell responses, and the induction of a M72-specific IgG antibody after vaccination suggest that M72/AS01E is a good candidate to advance into efficacy trials. A study found that—among healthy M. tuberculosis-infected, largely BCG-vaccinated, HIV-negative adults—M72/AS01E was linked to a significantly lower incidence of pulmonary TB vs. placebo. After a mean follow-up period of 2.3 years, the efficacy of M72/AS01E in preventing active pulmonary TB (based on two positive sputum tests) was 54%. Moreover, polymerase chain reaction data indicated that—in individuals with LTBI—the total vaccine efficacy was 57% (17). However, this trial focused exclusively on LTBI populations. Therefore, studies with larger sample sizes are warranted to demonstrate the efficacy of the M72/AS01E vaccine in a wider population. Populations of interest may include healthy individuals without previous exposure to TB, individuals with an impaired immune system (HIV+ patients, diabetics, users of injectable drugs, etc.), household contacts of TB patients who with a higher risk of infection, and individuals across various geographical settings. In addition, it is important to compare the efficacy and duration of protection provided by the M72/AS01E vaccine against all forms of TB in adolescents and adults vs. those associated with BCG. The TB vaccine clinical pipeline is being diversified, and new models of delivery by the pulmonary and mucosal routes examined (For example, the MVA85A is re-entering phase I trials as an aerosol vaccine), that holds promise for future development (51, 52).
Finally, the immunological analysis of study data may assist in better understanding the protective immune mechanisms against TB. The development of vaccines has been an iterative process. International collaborations are of crucial importance to offer renewed hope that effective new vaccines against TB can be developed (53, 54).
Data Availability
All datasets generated for this study are included in the manuscript and/or the Supplementary Files.
Author Contributions
FB, AL, ZJ, and MJ conceived and designed the study. ZJ and MJ did the database search and screening. YB, SW, GZ, and LLi did the data extraction. TC, LLu, XD, RB, and M-EA did the quality assessment. ZJ and MJ did the analysis, and in conjunction with SW and ZD interpreted the data and drafted the manuscript, in collaboration with FB and AL.
Conflict of Interest Statement
The authors declare that the research was conducted in the absence of any commercial or financial relationships that could be construed as a potential conflict of interest.
Acknowledgments
The authors gratefully acknowledge the support provided by grants from the National Natural Science Foundation of China (Grant Nos. 81560596, 81860644, and 31560051) and the Scientific and Technological Development Project of Yunnan Province of China (Grant Nos. 2017FE467-001 and 2014FA011).
Supplementary Material
The Supplementary Material for this article can be found online at: https://www.frontiersin.org/articles/10.3389/fimmu.2019.02089/full#supplementary-material
References
1. World Health Organization. Executive Summary. Global Tuberculosis Report 2018. Available online at: https://www.who.int/tb/publications/global_report/en/
2. Hart PD, Sutherland I. BCG and vole bacillus vaccines in the prevention of tuberculosis in adolescence and early adult life. Br Med J. (1977) 2: 293–95. doi: 10.1136/bmj.2.6082.293
3. Comstock GW, Woolpert SF, Livesay VT. Tuberculosis studies in Muscogee County, Georgia. Twenty-year evaluation of a community trial of BCG vaccination. Public Health Rep. (1976) 91: 276–280.
4. Dockrell HM, Smith SG. What have we learnt about BCG vaccination in the last 20 years? Front Immunol. (2017) 8:1134. doi: 10.3389/fimmu.2017.01134
5. Skeiky YA, Lodes MJ, Guderian JA, Mohamath R, Bement T, Alderson MR, et al. Cloning, expression, and immunological evaluation of two putative secreted serine protease antigens of Mycobacterium tuberculosis. Infect Immun. (1999) 67:3998–4007.
6. Dillon DC, Alderson MR, Day CH, Lewinsohn DM, Coler R, Bement T, et al. Molecular characterization and human T-cell responses to a member of a novel Mycobacterium tuberculosis mtb39 gene family. Infect Immun. (1999) 67:2941–50.
7. Leroux-Roels I, Forgus S, De Boever F, Clement F, Demoitié MA, Mettens P, et al. Improved CD4(+) T cell responses to Mycobacterium tuberculosis in PPD-negative adults by M72/AS01 as compared to the M72/AS02 and Mtb72F/AS02 tuberculosis candidate vaccine formulations: a randomized trial. Vaccine. (2013) 31:2196–206. doi: 10.1016/j.vaccine.2012.05.035
8. Skeiky YAW, Alderson MR, Ovendale PJ, Guderian JA, Brandt L, Dillon DC, et al. Differential immune responses and protective efficacy induced by components of a tuberculosis polyprotein vaccine, Mtb72F, delivered as naked DNA or recombinant protein. J Immunol. (2004) 172: 7618–28. doi: 10.4049/jimmunol.172.12.7618
9. Nathalie GO, Marcelle VM. Recent clinical experience with vaccines using MPL- and QS-21-containing adjuvant systems. Expert Rev Vaccines. (2011) 10:471. doi: 10.1586/erv.11.29
10. Baldridge J, Myers K, Johnson D, Persing D, Cluff C, Hershberg R. Monophosphoryl Lipid A and Synthetic Lipid A Mimetics as TLR4-Based Adjuvants and Immunomodulators. Clifton: Humana Press (2006).
11. Montoya J, Solon JA, Cunanan SRC, Acosta L, Bollaerts A, Moris P, et al. A randomized, controlled dose-finding phase II study of the M72/AS01 candidate tuberculosis vaccine in healthy PPD-positive adults. J Clin Immunol. (2013) 33:1360–75. doi: 10.1007/s10875-013-9949-3
12. Penn-Nicholson A, Geldenhuys H, Burny W, Most R, Day CL, Jongert E, et al. Safety and immunogenicity of candidate vaccine M72/AS01E in adolescents in a TB endemic setting. Vaccine. (2015) 33: 4025–34. doi: 10.1016/j.vaccine.2015.05.088
13. Thacher EG, Cavassini M, Audran R, Thierry AC, Bollaerts A, Cohen J, et al. Safety and immunogenicity of the M72/AS01 candidate tuberculosis vaccine in HIV-infected adults on combination antiretroviral therapy: a phase I/II, randomized trial. AIDS. (2014) 28: 1769–81. doi: 10.1097/QAD.0000000000000343
14. Idoko OT, Owolabi OA, Owiafe PK, Moris P, Odutola A, Bollaerts A, et al. Safety and immunogenicity of the M72/AS01 candidate tuberculosis vaccine when given as a booster to BCG in Gambian infants: an open-label randomized controlled trial. Tuberculosis. (2014) 94:564–78. doi: 10.1016/j.tube.2014.07.001
15. Kumarasamy N, Poongulali S, Bollaerts A, Moris P, Beulah FE, Ayuk LN, et al. A randomized, controlled safety, and immunogenicity trial of the M72/AS01 candidate tuberculosis vaccine in HIV-positive Indian adults. Medicine. (2016) 95:e2459. doi: 10.1097/MD.0000000000002459
16. Gillard P, Yang PC, Danilovits M, Su WJ, Cheng SL, Pehme L, et al. Safety and immunogenicity of the M72/AS01E candidate tuberculosis vaccine in adults with tuberculosis: a phase II randomized study. Tuberculosis. (2016) 100:118–27. doi: 10.1016/j.tube.2016.07.005
17. Van Der Meeren O, Hatherill M, Nduba V, Wilkinson RJ, Muyoyeta M, Van Brakel E, et al. Phase 2b controlled trial of M72/AS01E vaccine to prevent tuberculosis. N Engl J Med. (2018) 379:1621–34. doi: 10.1056/NEJMoa1803484
18. Higgins JPT, Deeks JJ. Chapter 7: Selecting studies and collecting data. Cochrane Handbook for Systematic Reviews of Interventions Version 5.1.0. The Cochrane Collaboration (2011). Available online at: https://training.cochrane.org/handbook
19. Moher D, Liberati A, Tetzlaff J, Altman DG, The PG. Preferred reporting items for systematic reviews and meta-analyses: the PRISMA statement. PLoS Med. (2009) 6:e1000097. doi: 10.1371/journal.pmed.1000097
20. Higgins JPT, Altman DG, Sterne JAC. Chapter 8: Assessing risk of bias in included studies. Cochrane Handbook for Systematic Reviews of Interventions Version 5.1.0. The Cochrane Collaboration (2011). Available online at: https://training.cochrane.org/handbook
21. Velayati AA, Abeel T, Shea T, Konstantinovich ZG, Birren B, Cassell GH, et al. Populations of latent Mycobacterium tuberculosis lack a cell wall: isolation, visualization, and whole-genome characterization. Int J Mycobacteriol. (2016) 5:66–73. doi: 10.1016/j.ijmyco.2015.12.001
22. Gideon HP, Flynn JL. Latent tuberculosis: what the host “sees”? Immunol Res. (2011) 50: 202–12. doi: 10.1007/s12026-011-8229-7
23. Van RDB, Laurane DM, Geert LR, Bechtold V, Clement F, Coccia M, et al. Adjuvant-associated peripheral blood mRNA profiles and kinetics induced by the adjuvanted recombinant protein candidate tuberculosis vaccine M72/AS01 in bacillus Calmette-Guerin-vaccinated adults. Front immunol. (2018) 9:564. doi: 10.3389/fimmu.2018.00564
24. Glatman-Freedman A. The role of antibody-mediated immunity in defense against Mycobacterium tuberculosis: advances toward a novel vaccine strategy. Tuberculosis. (2006) 86:191–7. doi: 10.1016/j.tube.2006.01.008
25. Phuah JY, Mattila JT, Lin PL, Flynn JL. Activated B cells in the granulomas of nonhuman primates infected with Mycobacterium tuberculosis. Am J Pathol. (2012) 181:508–14. doi: 10.1016/j.ajpath.2012.05.009
26. Pethe K, Alonso S, Biet F, Delogu G, Brennan MJ, Locht C, et al. The heparin-binding haemagglutinin of M. tuberculosis is required for extrapulmonary dissemination. Nature. (2001) 412:190–4. doi: 10.1038/35084083
27. Kumarasamy N, Poongulali S, Beulah FE, Akite EA, Ayuk LN, Bollaerts A, et al. Long-term safety and immunogenicity of the M72/AS01E candidate tuberculosis vaccine in HIV-positive and -negative Indian adults: results from a phase II randomized controlled trial. Medicine. (2018) 97:e13120. doi: 10.1097/MD.0000000000013120
28. Agnandji ST, Lell B, Soulanoudjingar SS, Fernandes JF, Abossolo BP, Conzelmann C, et al. First results of phase 3 trial of RTS, S/AS01 malaria vaccine in African children. N Engl J Med. (2011) 365:1863–75. doi: 10.1056/NEJMoa1102287
29. Agnandji ST, Lell B, Fernandes JF, Abossolo BP, Methogo BG, Kabwende AL, et al. A phase 3 trial of RTS, S/AS01 malaria vaccine in African infants. N Engl J Med. (2012) 367:2284–95. doi: 10.1056/NEJMoa1208394
30. Moncunill G, De Rosa SC, Ayestaran A, Nhabomba AJ, Mpina M, Cohen KW, et al. RTS, S/AS01E malaria vaccine induces memory and polyfunctional T Cell responses in a pediatric African phase III trial. Front immunol. (2017) 8:1008. doi: 10.3389/fimmu.2017.01008
31. Dendouga N, Fochesato M, Lockman L, Mossman S, Giannini SL. Cell-mediated immune responses to a varicella-zoster virus glycoprotein E vaccine using both a TLR agonist and QS21 in mice. Vaccine. (2012) 30:3126–35. doi: 10.1016/j.vaccine.2012.01.088
32. Livingston PO, Adluri S, Helling F, Yao TJ, Kensil CR, Newman MJ, et al. Phase 1 trial of immunological adjuvant QS-21 with a GM2 ganglioside-keyhole limpet haemocyanin conjugate vaccine in patients with malignant melanoma. Vaccine. (1994) 12: 1275–80. doi: 10.1016/S0264-410X(94)80052-2
33. Stenger S. Immunological control of tuberculosis: role of tumour necrosis factor and more. Ann Rheum Dis. (2005) 64:iv24–28. doi: 10.1136/ard.2005.042531
34. Flynn JL. Immunology of tuberculosis and implications in vaccine development. Tuberculosis. (2004) 84: 93–101. doi: 10.1016/j.tube.2003.08.010
35. Henrik M, Detjen AK, Schuck SD, Gutschmidt A, Wahn U, Magdorf K, et al. Mycobacterium tuberculosis-specific CD4+, IFNgamma+, and TNFalpha+ multifunctional memory T cells coexpress GM-CSF. Cytokine. (2008) 43:143–8. doi: 10.1016/j.cyto.2008.05.002
36. Aagaard CS, Hoang TL, Vingsbo-Lundberg C, Dietrich J, Andersen P. Quality and vaccine efficacy of CD4+ T cell responses directed to dominant and subdominant epitopes in ESAT-6 from Mycobacterium tuberculosis. J Immunol. (2009) 183:2659–68. doi: 10.4049/jimmunol.0900947
37. Lindenstrøm T, Agger EM, Korsholm KS, Darrah PA, Aagaard C, Seder RA, et al. Tuberculosis subunit vaccination provides long-term protective immunity characterized by multifunctional CD4 memory T cells. J Immunol. (2009) 182:8047. doi: 10.4049/jimmunol.0801592
38. Kagina BM, Abel B, Scriba TJ, Hughes EJ, Keyser A, Soares A, et al. Specific T cell frequency and cytokine expression profile do not correlate with protection against tuberculosis after bacillus Calmette-Guerin vaccination of newborns. Am J Respir Crit Care Med. (2010) 182:1073–9. doi: 10.1164/rccm.201003-0334OC
39. Keane J, Gershon S, Wise RP, Mirabile-Levens E, Kasznica J, Schwieterman WD, et al. Tuberculosis associated with infliximab, a tumor necrosis factor alpha-neutralizing agent. N Engl J Med. (2001) 345:1098–104. doi: 10.1056/NEJMoa011110
40. Khader SA, Bell GK, Pearl JE, Fountain JJ, Rangel-Moreno J, Cilley GE, et al. IL-23 and IL-17 in the establishment of protective pulmonary CD4+ T cell responses after vaccination and during Mycobacterium tuberculosis challenge. Nat Immunol. (2007) 8:369–77. doi: 10.1038/ni1449
41. Millington KA, Innes JA, Hackforth S, Hinks TS, Deeks JJ, Dosanjh DP, et al. Dynamic relationship between IFN-gamma and IL-2 profile of Mycobacterium tuberculosis-specific T cells and antigen load. J Immunol. (2007) 178:5217–26. doi: 10.4049/jimmunol.178.8.5217
42. Lewinsohn DA, Gold MC, Lewinsohn DM. Views of immunology: effector T cells. Immunol Rev. (2011) 240:25–39. doi: 10.1111/j.1600-065X.2010.00997.x
43. Allie N, Grivennikov SI, Keeton R, Hsu NJ, Bourigault ML, Court N, et al. Prominent role for T cell-derived tumour necrosis factor for sustained control of Mycobacterium tuberculosis infection. Sci Rep. (2013) 3:1809. doi: 10.1038/srep01809
44. Lyadova IV, Panteleev AV. T1 and T17 cells in tuberculosis: protection, pathology, and biomarkers. Mediators Inflamm. (2015) 2015:854507. doi: 10.1155/2015/854507
45. Darrah PA, Patel DT, De Luca PM, Lindsay RW, Davey DF, Flynn BJ, et al. Multifunctional TH1 cells defne a correlate of vaccine-mediated protection against Leishmania major. Nat Med. (2007) 13:843–50. doi: 10.1038/nm1592
46. Day CL, Tameris M, Mansoor N, Rooyen M, Kock M, Geldenhuys H, et al. Induction and regulation of T-cell immunity by the novel tuberculosis vaccine M72/AS01 in South African adults. Am J Respir Crit Care Med. (2013) 188:492–502. doi: 10.1164/rccm.201208-1385OC
47. Spertini F, Audran R, Lurati F, Ofori-Anyinam O, Zysset F, Vandepapelière P, et al. The candidate tuberculosis vaccine Mtb72F/AS02 in PPD positive adults: a randomized controlled phase I/II study. Tuberculosis. (2013) 93:179–88. doi: 10.1016/j.tube.2012.10.011
48. Rodo MJ, Rozot V, Nemes E, Dintwe O, Hatherill M, Little F, et al. A comparison of antigen-specific T cell responses induced by six novel tuberculosis vaccine candidates. PLoS Pathog. (2019) 15:e1007643. doi: 10.1371/journal.ppat.1007643
49. Day CL, Abrahams DA, Lerumo L, Janse van Rensburg E, Stone L, O'rie T, et al. Functional capacity of Mycobacterium tuberculosis-specific T cell responses in humans is associated with mycobacterial load. J immunol. (2011) 187:2222–32. doi: 10.4049/jimmunol.1101122
50. Li Q, Zhang H, Yu L, Wu C, Luo X, Sun H, et al. Down-regulation of Notch signaling pathway reverses the Th1/Th2 imbalance in tuberculosis patients. Int Immunopharmacol. (2018) 54:24–32. doi: 10.1016/j.intimp.2017.10.026
51. Counoupas C, Triccas JA, Britton WJ. Deciphering protective immunity against tuberculosis: implications for vaccine development. Expert Rve Vaccines. (2019) 4:1–12. doi: 10.1080/14760584.2019.1585246
52. Satti I, Meyer J, Harris SA, Manjaly Thomas ZR, Griffiths K, Antrobus RD, et al. Safety and immunogenicity of a candidate tuberculsis vaccine MVA85A delivered by aerosol in BCG-vaccinated healthy adults: a phase 1, double-blind, randomised controlled trial. Lancet Infect Dis. (2014) 14:939–46. doi: 10.1016/S1473-3099(14)70845-X
53. Bloom B R. New Promise for Vaccines against Tuberculosis. N Engl J Med. (2018) 379:1672–4. doi: 10.1056/NEJMe1812483
Keywords: vaccine, tuberculosis, M72/AS01E, immunogenicity, safety
Citation: Ji Z, Jian M, Chen T, Luo L, Li L, Dai X, Bai R, Ding Z, Bi Y, Wen S, Zhou G, Abi M-E, Liu A and Bao F (2019) Immunogenicity and Safety of the M72/AS01E Candidate Vaccine Against Tuberculosis: A Meta-Analysis. Front. Immunol. 10:2089. doi: 10.3389/fimmu.2019.02089
Received: 24 March 2019; Accepted: 19 August 2019;
Published: 03 September 2019.
Edited by:
Denise Doolan, James Cook University, AustraliaReviewed by:
Carmen Alvarez-Dominguez, Marqués de Valdecilla Health Research Institute (IDIVAL), SpainYoshihiko Hoshino, National Institute of Infectious Diseases (NIID), Japan
Copyright © 2019 Ji, Jian, Chen, Luo, Li, Dai, Bai, Ding, Bi, Wen, Zhou, Abi, Liu and Bao. This is an open-access article distributed under the terms of the Creative Commons Attribution License (CC BY). The use, distribution or reproduction in other forums is permitted, provided the original author(s) and the copyright owner(s) are credited and that the original publication in this journal is cited, in accordance with accepted academic practice. No use, distribution or reproduction is permitted which does not comply with these terms.
*Correspondence: Aihua Liu, bGl1YWlodWFAa21tdS5lZHUuY24=; Fukai Bao, YmFvZnVrYWlAa21tdS5lZHUuY24=
†Joint first authors