- 1William Harvey Research Institute, Barts and the London School of Medicine and Dentistry, Queen Mary University of London, London, United Kingdom
- 2Department of Drug Science and Technology, University of Turin, Turin, Italy
- 3Department of Anesthesiology and Intensive Care Medicine, Jena University Hospital, Jena, Germany
- 4Septomics Research Center, Jena University Hospital, Jena, Germany
- 5Department of Operative Intensive Care and Intermediate Care, RWTH University Hospital Aachen, Aachen, Germany
Sepsis is one of the most prevalent diseases in the world. The development of cardiac dysfunction in sepsis results in an increase of mortality. It is known that Bruton's tyrosine kinase (BTK) plays a role in toll-like receptor signaling and NLRP3 inflammasome activation, two key components in the pathophysiology of sepsis and sepsis-associated cardiac dysfunction. In this study we investigated whether pharmacological inhibition of BTK (ibrutinib 30 mg/kg and acalabrutinib 3 mg/kg) attenuates sepsis associated cardiac dysfunction in mice. 10-week old male C57BL/6 mice underwent CLP or sham surgery. One hour after surgery mice received either vehicle (5% DMSO + 30% cyclodextrin i.v.), ibrutinib (30 mg/kg i.v.), or acalabrutinib (3 mg/kg i.v.). Mice also received antibiotics and an analgesic at 6 and 18 h. After 24 h, cardiac function was assessed by echocardiography in vivo. Cardiac tissue underwent western blot analysis to determine the activation of BTK, NLRP3 inflammasome and NF-κB pathway. Serum analysis of 33 cytokines was conducted by a multiplex assay. When compared to sham-operated animals, mice subjected to CLP demonstrated a significant reduction in ejection fraction (EF), fractional shortening (FS), and fractional area change (FAC). The cardiac tissue from CLP mice showed significant increases of BTK, NF-κB, and NLRP3 inflammasome activation. CLP animals resulted in a significant increase of serum cytokines and chemokines (TNF-α, IL-6, IFN-γ, KC, eotaxin-1, eotaxin-2, IL-10, IL-4, CXCL10, and CXCL11). Delayed administration of ibrutinib and acalabrutinib attenuated the decline of EF, FS, and FAC caused by CLP and also reduced the activation of BTK, NF-κB, and NLRP3 inflammasome. Both ibrutinib and acalabrutinib significantly suppressed the release of cytokines and chemokines. Our study revealed that delayed intravenous administration of ibrutinib or acalabrutinib attenuated the cardiac dysfunction associated with sepsis by inhibiting BTK, reducing NF-κB activation and the activation of the inflammasome. Cytokines associated with sepsis were significantly reduced by both BTK inhibitors. Acalabrutinib is found to be more potent than ibrutinib and could potentially prove to be a novel therapeutic in sepsis. Thus, the FDA-approved BTK inhibitors ibrutinib and acalabrutinib may be repurposed for the use in sepsis.
Introduction
Sepsis is a life-threatening organ dysfunction caused by a dysregulated host response to an infection (1), which affects approximately 30 million people worldwide (2). In the UK, sepsis is the second leading cause of death with 36,000–64,000 patients dying each year (3) costing the NHS £2.5 billion annually (4). The development of cardiac dysfunction affects 40% of septic patients (5) and is associated with an increased mortality rate of 70–90% in comparison to 20% mortality in patients who do not present with cardiac dysfunction (6). However, the mechanisms that underlie this cardiac dysfunction are not well-known. Evidence suggests that multiple factors contribute to the pathophysiology of the cardiac dysfunction associated with sepsis. These include the activation of NF-κB and NLRP3 leading to excessive formation of e.g., IL-1 and TNF-α (7, 8). There are currently no drugs for the specific treatment of the cardiac dysfunction (or indeed the multiple organ dysfunction) associated with sepsis that specifically target NF-κB and the NLRP3 inflammasome.
Bruton's tyrosine kinase (BTK) plays a role in innate immunity and is a critical component in the development of B cells (9). The FDA has approved the use of the irreversible BTK inhibitors ibrutinib (first generation) in chronic lymphatic leukemia (CLL), mantle cell lymphoma (MCL), Waldenstrom macroglobulinemia (WM), and graft vs. host disease (10) and acalabrutinib (more selective, second generation) in MCL (11). Ibrutinib is also approved by the EMA for the treatment of CLL, MCL, and WM (12), whereas acalabrutinib has received an orphan designation for CCL, MCL, and lymphoplasmacytic lymphoma (13–15). During sepsis, bacterial LPS stimulates TLR4 and BTK is directly involved in the activation of this signaling pathway. Specifically, BTK binds to the TIR domain of TLR4 and its adaptor molecules (also found in other TLR's) MyD88 and Mal, and results in downstream activation of NF-κB and the generation of proinflammatory cytokines (16). BTK also regulates the assembly and, hence, activation of the NLRP3 inflammasome by binding to the ASC component (17, 18). Inhibition of BTK by BTK inhibitors reduces NF-κB activation and the formation of NF-κB-dependent cytokines in murine models of arthritis (19).
Given the importance of TLRs and NLRP3 in the pathophysiology of sepsis, we hypothesized that BTK inhibitors, such as ibrutinib or acalabrutinib, may attenuate the cardiac dysfunction in a murine model of polymicrobial sepsis. Additionally, we set out to investigate the potential effects of BTK inhibition on (a) the activation of NF-κB and NLRP3 in the heart, and (b) the serum levels of key, pro- and anti-inflammatory cytokines and chemokines.
Methods
Ethical Statement
The Animal Welfare Ethics Review Board of Queen Mary University of London approved all experiments in accordance with the Home Office guidance on the operation of Animals (Scientific Procedure Act, 1986) published by Her Majesty's Stationary Office, and the Guide for the Care and Use of Laboratory Animals of the National Research Council. Work was conducted under U.K. home office project license number PC5F29685. All in vivo experiments are reported in accordance to ARRIVE guidelines (20).
Animals
This study was carried out on 40 10-week-old male C57BL/6 mice (Charles River Laboratories UK Ltd., Kent, UK) weighing 25–30 g and kept under standard laboratory conditions. Six mice were housed together (in each cage) with access to a chow diet and water ad libitum. They were subjected to a 12-h light and dark cycle with a temperature maintained at 19–23°C.
Drugs
Ibrutinib and acalabrutinib were purchased from Selleck Chemicals. Stock solutions were made in DMSO 5% and cyclodextrin 30% (vehicle).
Murine Model of Polymicrobial Sepsis Caused by Cecum Ligation and Puncture (CLP)
Mice were randomized to undergo either sham operation, CLP + vehicle (5% DMSO + 30% cyclodextrin), CLP + ibrutinib (30 mg/kg), or CLP + acalabrutinib (3 mg/kg). Before surgery, mice were injected with buprenorphine (0.05 mg/kg, i.p.). Mice were initially anesthetized by isoflurane (3 L/min) and oxygen (1 L/min) in an anesthetic chamber and maintained with isoflurane (2 L/min) and oxygen (1 L/min) via a face mask. Temperature was monitored via a rectal probe and kept at 37°C by a homeothermic mat. Veet® hair removal cream was used to remove the fur from the abdomen of the mouse and skin was then cleaned with 70% ethanol. The abdomen was opened with a 1.5 cm midline incision to expose the cecum. The cecum was fully ligated below the ileocecal valve, and a G-18 needle was used to puncture two holes in the top and bottom of the cecum. A small amount of feces was then squeezed out. The cecum was returned to the abdomen in its anatomical position and 5 ml/kg of saline was administered into the abdomen before its closure. Saline (10 ml/kg s.c.) was administered directly after surgery. One hour after CLP, vehicle (5% DMSO + 30% cyclodextrin), ibrutinib (30 mg/kg), or acalabrutinib (3 mg/kg) was administered intravenously. At 6 and 18 h after surgery, antibiotics (imipenem/cilastatin; 20 mg/kg dissolved in saline s.c.) and an analgesic (buprenorphine; 0.05 mg/kg i.p.) were administered. After 24 h, cardiac function was assessed by echocardiography in vivo. Mice that underwent sham surgery were not subjected to ligation or perforation of the cecum but were otherwise treated the same way, 1 h after surgery sham animals were treated with vehicle (5% DMSO + 30% cyclodextrin).
Renal Dysfunction Analysis
Renal dysfunction was analyzed in all mice. The mice were anesthetized with isoflurane (3 L/min) and oxygen (1 L/min) before being sacrificed. Cardiac puncture was performed with a G25 needle and non-heparinized syringes to obtain approximately 0.7 ml of blood. The blood was immediately decanted into 1.3 ml serum gel tubes (Sarstedt, Nürnbrecht, Germany). The heart and lungs were then removed. The blood samples were centrifuged for 3 min at 9,000 RPM to separate the serum, where 100 μL of serum was pipetted into an aliquot and snap frozen in liquid nitrogen and stored at −80°C for further analysis. The serum was then sent to an independent veterinary testing laboratory (MRC Harwell Institute, Oxford, UK) to blindly quantify serum urea and creatinine known markers of renal dysfunction.
Echocardiography
At 24 h after CLP, cardiac function was assessed with a Vevo 3100 imaging system (VisualSonics, Toronto, Ontario, Canada). Mice were fully sedated in an anesthetic chamber with isoflurane (3 L/min) and oxygen (1 L/min) and were then transferred to the thermoregulatory platform in the supine position, where their paws were taped on to the EKG leads. Anesthetic was maintained throughout the entire procedure via a nosecone with isoflurane (0.5–2.0 L/min) and oxygen (1 L/min). The fur on the chest was removed by Veet® hair removal cream and pre-warmed echo gel was placed onto the shaven chest. The heart was then imaged with the MX550D imaging probe. To measure the left ventricle in B-mode the probe was placed along the long axis of the left ventricle, and directed toward the right of the mouse, here we measured percentage fractional area change (FAC%). The probe was then rotated 90° to visualize the short axis in the M-mode where the following parameters were measured: the percentage ejection fraction (EF%) and fractional shortening (FS%).
Western Blot Analysis
Immunoblot analyses of cardiac tissue samples were carried out using a semi-quantitative western blotting analysis. The antibody used were: 1:1,000 rabbit anti-Ser176/180-IKKα/β, 1:1,000 rabbit anti-total IKKα/β, 1:1,000 mouse anti-Ser32/36-IκBα, 1:1,000 mouse anti-total IκBα, 1:1,000 rabbit anti-NF-κB, 1:1,000 rabbit anti-total BTK, 1:1,000 rabbit anti-Tyr1217 PLCγ, 1:1,000 rabbit anti-total PLCγ (from Cell Signaling), 1:1,000 rabbit anti-Tyr223-BTK, 1:5,000 rabbit anti NLRP3 inflammasome (from Abcam), 1:1,000 mouse anti-caspase 1 (p20) (from Adipogen). The apex of the heart was taken and homogenized in 1:10 of homogenization buffer at 4°C. Nuclear and cytosolic proteins were then extracted as previously described (21) and concentrations were quantified by bicinchoninic acid (BCA) protein assay (Thermo Fisher Scientific Rockford, IL). Proteins were separated by 8% sodium dodecyl sulfate (SDS)-PAGE and transferred to polyvinyldenedifluoride membranes. Membranes were blocked in 10% milk solution with TBS-Tween and then incubated with the primary antibody overnight at 4°C. The next day the secondary antibody was added for 30 min at room temperature and visualized using the ECL detection system. Tubulin and histone 3 were used as loading control. The immunoreactive bands were analyzed by the Bio-Rad Image Lab Software™ 6.0.1 and results were normalized to the sham bands.
Multiplex Flow Immunoassay
The principle of multiplex flow immunoassay technology has been reviewed previously (22, 23). Cytokines, chemokines and a growth factor were determined in serum by Bio-Plex Pro Mouse Chemokine 33-plex panel assay (Bio-Rad, Kabelsketal, Germany). The cytokines IL-1ß,−2,−4,−6,−10,−16, CCL1,−2,−3,−4,−5,−7,−11,−12,−17,−19,−20,−22,−24,−25, −27, IFN-γ, TNF-α and the chemokines CX3CL1, CXCL1,−2,−5,−10,−11,−12,−13,−16 and the growth factor GM-CSF were measured according to the manufacturer's instructions. The assays were performed in one batch, with samples randomly distributed. The lower detection limit was 3.2 pg/ml for all the analytes. Data were collected and analyzed using a Bio-Plex® 200 instrument equipped with Bio-Plex Manager software (Bio-Rad).
Statistical Analysis
All data in text and figures are expressed as mean ± standard error mean (SEM) of n observations. Measurements obtained from the intervention, control and sham were analyzed by one-way ANOVA followed by a Bonferroni's post-hoc test on GraphPad Prism 6.0 (GraphPad Software, Inc., La Jolla, CA, USA). Correlations coefficients were determined by Pearson's correlation with P-values based on two-tailed tests. Differences were considered to be statistically significant when P < 0.05.
Results
Ibrutinib or Acalabrutinib Attenuate the Cardiac Dysfunction Caused by CLP-sepsis
When compared to sham-operated animals, mice subjected to CLP for 24 h (Figure 1A) demonstrated a significant reduction in EF, FS, and FAC (P < 0.0001; Figures 1B–E) indicating the development of systolic cardiac dysfunction. The observed reduction in EF also negatively correlated with the rise of the chemokines CXCL10 and CXCL11, both of which are well-known biomarkers of left ventricular dysfunction (Figures 1F–I). When compared to CLP mice treated with vehicle (control), the administration of ibrutinib (30 mg/kg) or acalabrutinib (3 mg/kg) at 1 h after CLP significantly attenuated the decline in EF, FS and FAC caused by CLP (P < 0.01; Figures 1B–E). The rise in the serum levels of the chemokines CXCL10 and CXCL11 caused by CLP were also significantly reduced by either ibrutinib or acalabrutinib (P < 0.05; Figures 1F–I). No significant differences were observed in any of the cardiac parameters or cytokines measured in CLP animals treated with either ibrutinib or acalabrutinib (P > 0.05; Figures 1B–I). To gain a better insight into the mechanism by which the two BTK-inhibitors reduce the cardiac dysfunction associated with sepsis, we investigated the effects of ibrutinib and acalabrutinib on (a) BTK-activation and signaling, (b) NF-κB activation, and (c) NLRP3 inflammasome assembly and activation (see below).
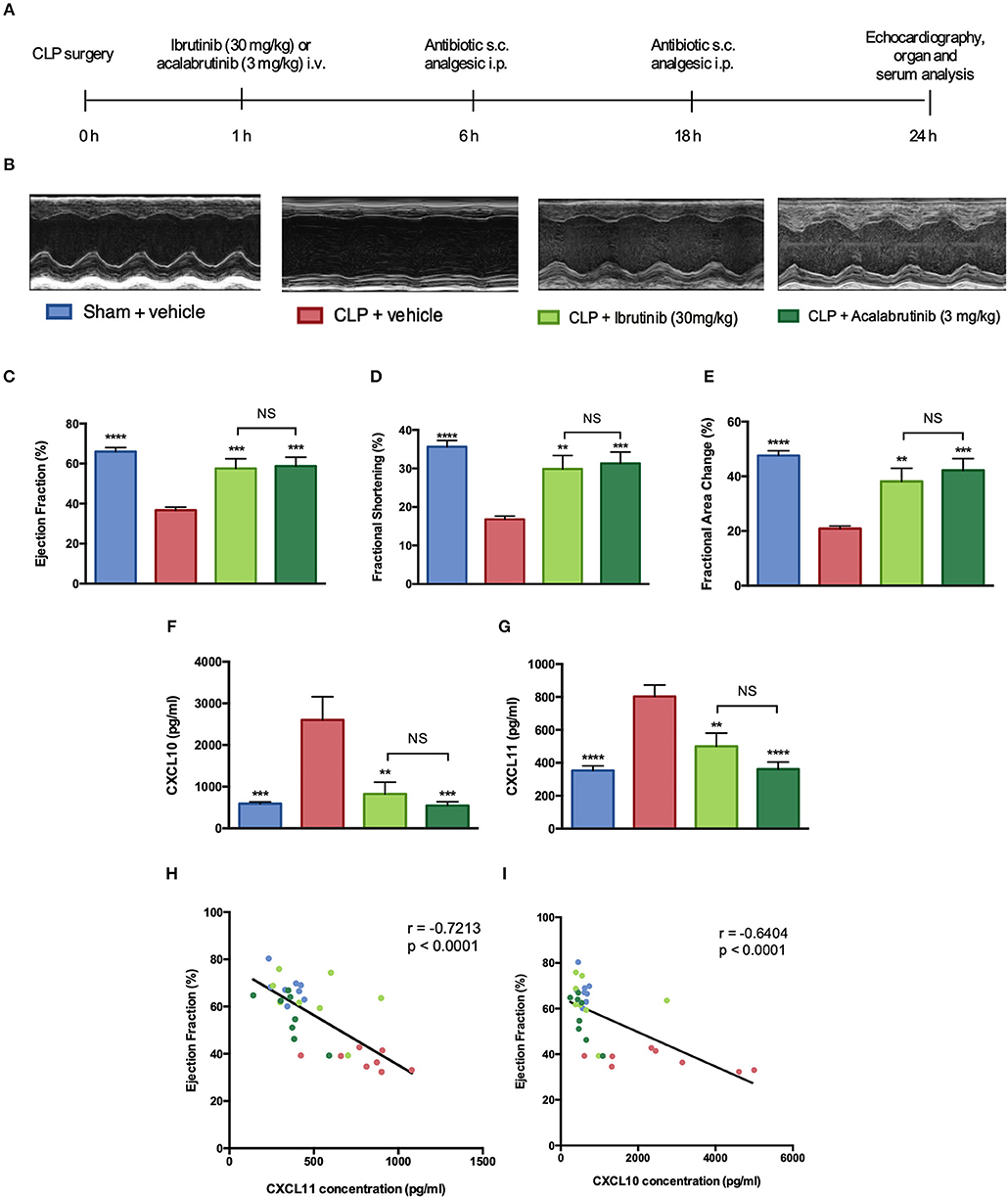
Figure 1. Ibrutinib or acalabrutinib attenuate the cardiac dysfunction caused by CLP-sepsis. Mice were randomly assigned to undergo CLP or sham surgery (n = 10). One hour later, mice were treated with ibrutinib (30 mg/kg i.v.), acalabrutinib (3 mg/kg i.v.), or vehicle (5% DMSO + 30% cyclodextrin i.v.). Cardiac function was assessed 24 h after CLP surgery (n = 10 per group). (A) Illustration of the timelines of the CLP model. (B) Representative M-mode echocardiograms. (C) Ejection fraction (%). (D) Fractional shortening (%). (E) Fractional area change (%). (F) CXCL10 serum concentration (pg/ml). (G) CXCL11 serum concentration (pg/ml). (H) correlation of ejection fraction and CXCL10 serum concentration. (I) Correlation of ejection fraction and CXCL11 serum concentration. All data are expressed as mean ± SEM for n number of observations. A value of ****P < 0.0001, ***P < 0.001, **P < 0.01 was considered to be statistically significant when compared to the control by one-way ANOVA followed by a Bonferroni's post-hoc test. Correlations coefficients were determined by Pearson's correlation with P-values based on two-tailed tests.
Ibrutinib or Acalabrutinib Attenuate the Renal Dysfunction Caused by CLP-sepsis
Urea and creatinine were measured to study the effect of CLP (in the absence and presence of BTK inhibitors) on kidney function. When compared to sham, mice subjected to CLP and treated with vehicle had a significant increase of urea and creatinine, indicating kidney dysfunction (P < 0.0001; Figure 2). Administration of ibrutinib (30 mg/kg) or acalabrutinib (3 mg/kg) to CLP mice significantly attenuated the rise in urea and creatinine when compared to CLP mice treated with vehicle (P < 0.01; Figure 2), without any significant difference between the two treatment.
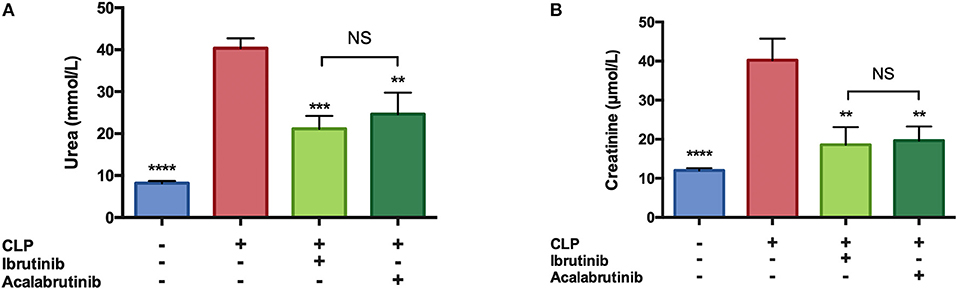
Figure 2. Ibrutinib or acalabrutinib attenuate the renal dysfunction caused by CLP-sepsis. Mice were randomly assigned to undergo CLP or sham surgery (n = 10). One hour later, mice were treated with ibrutinib (30 mg/kg i.v.), acalabrutinib (3 mg/kg i.v.), or vehicle (5% DMSO + 30% cyclodextrin i.v.). At 24 h after CLP, blood samples were collected for analyses (n = 10 per group). (A) Serum urea (mmol/L). (B) Serum creatinine (μmol/L). All data are expressed as mean ± SEM for n number of observations. A value of ****P < 0.0001, ***P < 0.001, **P < 0.01 was considered to be statistically significant when compared to the control by one-way ANOVA followed by a Bonferroni's post-hoc test.
Cardiac BTK Is Activated in CLP Mice and Reduced by Ibrutinib or Acalabrutinib
Using Western blot analysis, we investigated whether CLP-sepsis leads to an activation of BTK in the heart. The activation of BTK and the subsequent activation of BTK-signaling involves (a) phosphorylation of BTK at Tyr223 and (b) the phosphorylation of PLCγ at Tyr1217 by phosphorylated (activated) BTK as the first step in the BTK-signaling cascade. When compared to sham operated mice, CLP mice treated with vehicle demonstrated significant increases in the phosphorylation of cardiac BTK at Tyr223 and the phosphorylation of PLCγ at Tyr1217, indicating that BTK is activated in septic hearts (P < 0.0001; Figure 3A). Administration of ibrutinib (30 mg/kg) or acalabrutinib (3 mg/kg) in CLP mice resulted in a significant decrease in the phosphorylation of cardiac BTK at Tyr223 and the phosphorylation of PLCγ at Tyr1217 when compared to CLP mice treated with vehicle (P < 0.0001; Figure 3B) demonstrating that the doses of the two BTK inhibitors used in our study caused a significant inhibition of BTK-signaling in the heart. No significant differences were observed in the degree of phosphorylation of cardiac BTK at Tyr223 and the phosphorylation of PLCγ at Tyr1217 in CLP-animals treated with either ibrutinib or acalabrutinib (P > 0.05; Figures 3A,B).
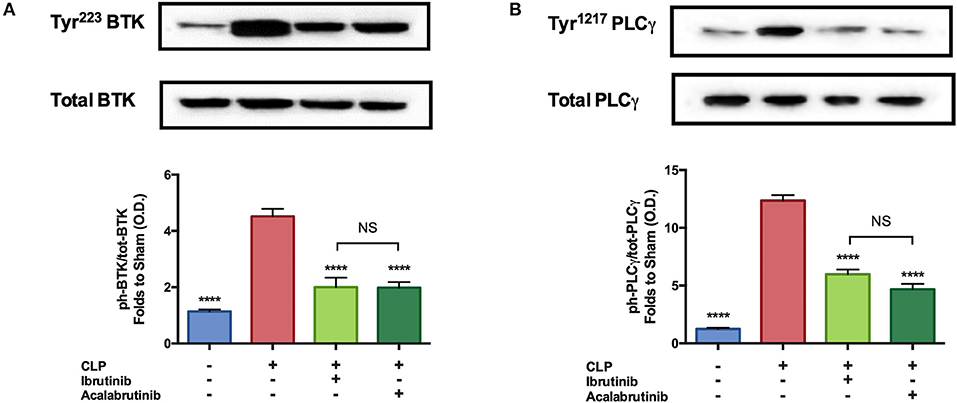
Figure 3. Cardiac BTK is activated in CLP mice and reduced by ibrutinib or acalabrutinib. Mice were randomly assigned to undergo CLP or sham surgery. One hour later, mice were treated with ibrutinib (30 mg/kg i.v.), acalabrutinib (3 mg/kg i.v.), or vehicle (5% DMSO + 30% cyclodextrin i.v.). At 24 h after CLP surgery, the activation of BTK in the heart was analyzed by western blot analysis (n = 5 per group). Specifically, densitometric analysis of the bands is expressed as relative OD of (A) phosphorylation of BTK at Tyr223 corrected for the corresponding total BTK and normalized using the related sham band. (B) Phosphorylation of PLCγ at Tyr1217 corrected for the corresponding total PLCγ. All data are expressed as mean ± SEM for n number of observations. A value of ****P < 0.0001 was considered to be statistically significant when compared to the control by one-way ANOVA followed by a Bonferroni's post-hoc test.
Cardiac NF-κB Activation in CLP Mice Is Reduced by Ibrutinib or Acalabrutinib
To understand the signaling mechanism associated with the observed cardiac dysfunction, we investigated the effect of BTK inhibition on the activation of key signaling pathways of inflammation including pathways leading to the activation of NF-κB. When compared to sham operated mice, CLP mice treated with vehicle had significant increases in the phosphorylation of IKKα/β at Ser176/180, the phosphorylation of IκBα at Ser32/36 and the translocation of p65 to the nucleus (P < 0.001; Figures 4A–C). When compared with CLP mice treated with vehicle, treatment of CLP mice with ibrutinib (30 mg/kg) or acalabrutinib (3 mg/kg) significantly attenuated the increases in cardiac phosphorylation of IKKα/β at Ser176/180 and IκBα at Ser32/36 and the nuclear translocation of p65 (P < 0.0001; Figures 4A–C). No significant differences were observed in the degree of phosphorylation of IKKα/β at Ser176/180, the phosphorylation of IκBα at Ser32/36 and the translocation of p65 to the nucleus in CLP animals treated with either ibrutinib or acalabrutinib (P > 0.05; Figures 4A–C).
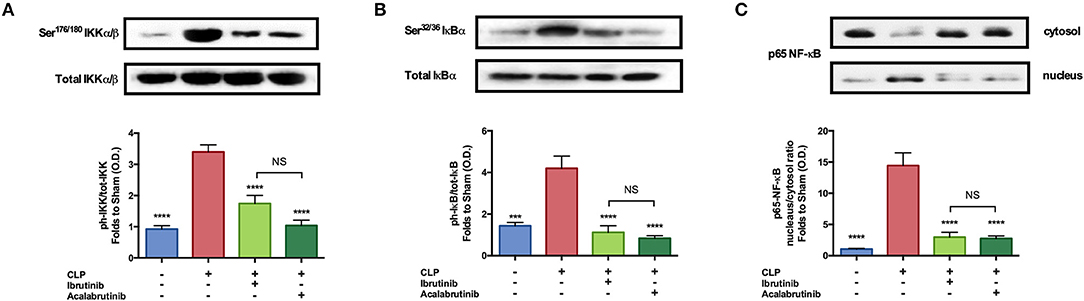
Figure 4. Cardiac NF-κB activation in CLP mice is reduced by ibrutinib or acalabrutinib. Mice were randomly assigned to undergo CLP or sham surgery. One hour later, mice were treated with ibrutinib (30 mg/kg i.v.), acalabrutinib (3 mg/kg i.v.), or vehicle (5% DMSO + 30% cyclodextrin i.v.). At 24 h cardiac tissue was collected and signaling was assessed (n = 5 per group). Densitometric analysis of the bands is expressed as relative OD of (A) phosphorylation of IKKα/β at Ser176/180 corrected for the corresponding total IKKα/β and normalized using the related sham band. (B) Phosphorylation of IκBα at Ser32/36 corrected for the corresponding total IκBα and normalized using the related sham band. (C) NF-κB p65 in both nucleus and cytosol and expressed as a ratio, normalized using the sham related bands. All data are expressed as mean ± SEM for n number of observations. A value of ****P < 0.0001 was considered to be statistically significant when compared to the control by one-way ANOVA followed by a Bonferroni's post-hoc test.
Cardiac NLRP3 Activation in CLP Mice Is Reduced by Ibrutinib or Acalabrutinib
We next assessed the potential involvement of NLRP3 in the cardiac dysfunction of CLP mice. When compared to sham operated mice, CLP-sepsis (vehicle-treatment) resulted in the increased expression of the NLRP3 inflammasome and cleavage of pro-caspase-1 to caspase-1 in the heart and a rise in serum IL-1β (P < 0.0001; Figures 5A–C). When compared to CLP mice treated with vehicle, treatment of CLP mice with ibrutinib or acalabrutinib significantly inhibited the expression of NLRP3, cleavage of pro-caspase-1 to caspase-1 and the rise in IL-1β (P < 0.01; Figures 5A–C), without any significant difference between the two drug treatments.
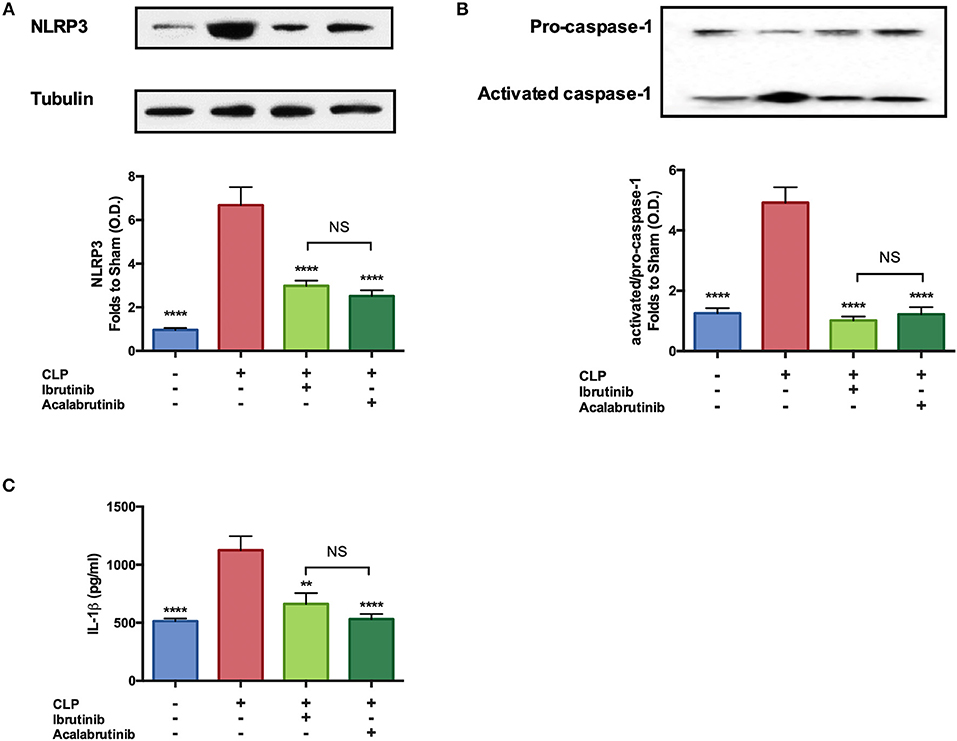
Figure 5. Cardiac NLRP3 activation in CLP mice is reduced by ibrutinib or acalabrutinib. Mice were randomly assigned to undergo CLP or sham surgery. One hour later, mice were treated with ibrutinib (30 mg/kg i.v.), acalabrutinib (3 mg/kg i.v.), or vehicle (5% DMSO + 30% cyclodextrin i.v.). At 24 h after CLP surgery, the assembly and activation of NLRP3 in the heart was analyzed by western blot analysis (n = 5 per group). Specifically, densitometric analysis of the bands is expressed as relative OD of (A) NLRP3 activation, corrected against tubulin and normalized using the sham related bands. (B) Pro-caspase-1 against activated caspase-1 and normalized using the sham related bands. (C) IL-1β serum concentration analyzed by multiplex assay (n = 8). All data are expressed as mean ± SEM for n number of observations. A value of ****P < 0.0001, **P < 0.01 was considered to be statistically significant when compared to the control by one-way ANOVA followed by a Bonferroni's post-hoc test.
Relationship Between BTK Activation and Cardiac Dysfunction in CLP-sepsis
To address the question whether the degree of activation of BTK correlates with alterations in cardiac function, we correlated the degree of phosphorylation of BTK at Tyr223 (Figure 6A) and the phosphorylation of PLCγ at Tyr1217 (Figure 6B) with EF. We found a highly significant negative correlation between the degree of BTK and PLCγ activation and the decline in EF, strongly suggesting that BTK activation drives or precedes the cardiac dysfunction associated with sepsis. To address the question whether the degree of activation of BTK also correlates with alterations in the activation of NF-κB, we correlated the degree of phosphorylation of BTK at Tyr223 with the translocation of p65 (Figure 6C) and the phosphorylation of IKKα/β at Ser176/180 (Figure 6D). We found a highly significant positive correlation between the degree of BTK activation and the activation of NF-κB when measured as either the translocation of p65 (Figure 6C) and the phosphorylation of IKKα/β at Ser176/180 (Figure 6D). To address the question whether the degree of activation of BTK also correlates with alterations in the assembly and activation of the inflammasome, we correlated the degree of phosphorylation of BTK at Tyr223 with either NLRP3 assembly (Figure 6E) or the activation of caspase-1 (Figure 6F). We found a highly significant positive correlation between the degree of BTK activation and the NLRP3 (Figure 6E) increased expression and the activation of caspase-1 (Figure 6F).
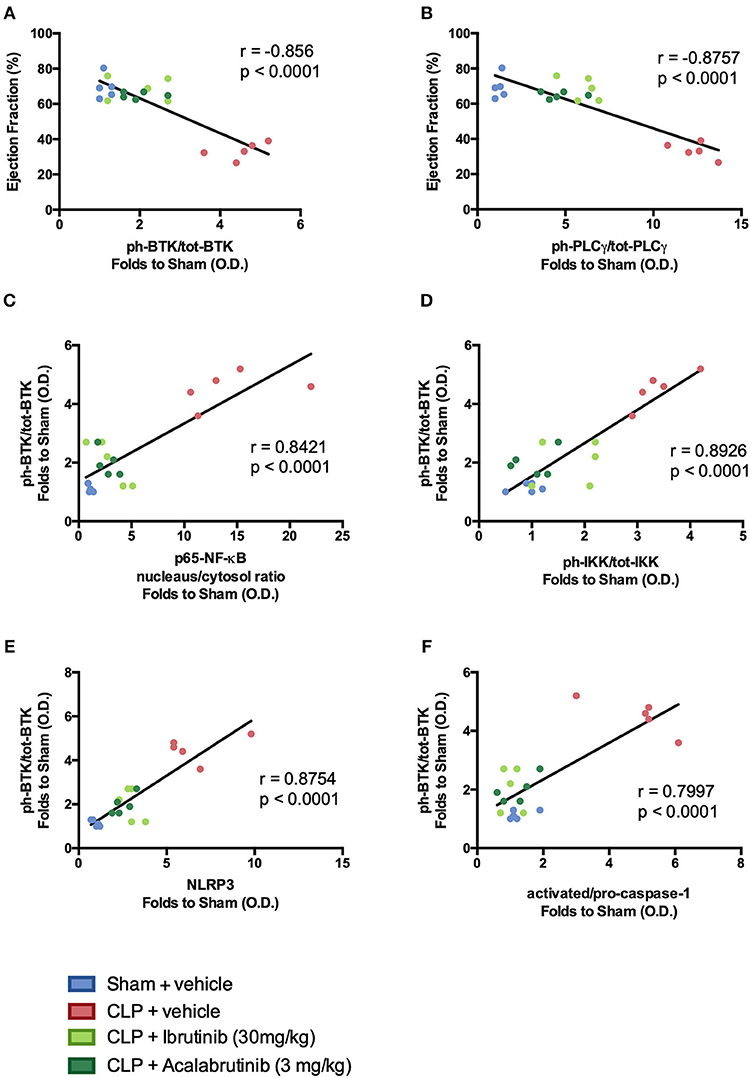
Figure 6. Relationship between BTK activation and cardiac dysfunction in CLP-sepsis. Correlation data to show (A) ejection fraction (%) vs. phosphorylation of BTK at Tyr223. (B) ejection fraction (%) vs. of PLCγ at Tyr1217. (C) Phosphorylation of BTK at Tyr223 vs. NF-κB p65. (D) Phosphorylation of BTK at Tyr223 vs. phosphorylation of IKKα/β atSer176/180. (E) Phosphorylation of BTK at Tyr223 vs. NLRP3. (F) Phosphorylation of BTK at Tyr223 vs. activated/pro-caspase-1. Data was analyzed by the Pearson correlation coefficient test to calculate the r value and a two tailed T-test for significance.
Systemic Inflammation in CLP Mice Is Reduced by Ibrutinib or Acalabrutinib
We also studied the effect of CLP (in the absence and presence of BTK inhibitors) on the systemic synthesis of pro-inflammatory cytokines, anti-inflammatory cytokines and pro-inflammatory chemokines. When compared to sham operated mice, CLP (vehicle) resulted in a significant rise in the serum levels of (a) the pro-inflammatory cytokines TNF-α, IFN-γ, IL-6; (b) the anti-inflammatory cytokines IL-4 and IL-10, and (c) the pro-inflammatory chemokines KC/CXCL1, eotaxin-1/CCL11, eotaxin-2/CCL24 (P < 0.05; Figures 7A–H). The sepsis-induced increase in these cytokines and chemokines was significantly attenuated by both BTK inhibitors, the only exception being IL-6, which was not significantly reduced by ibrutinib but a trend in reduction was observed. No significant differences were observed in the levels of cytokines or chemokines in CLP animals treated with either ibrutinib or acalabrutinib (P > 0.05; Figures 7A–H).
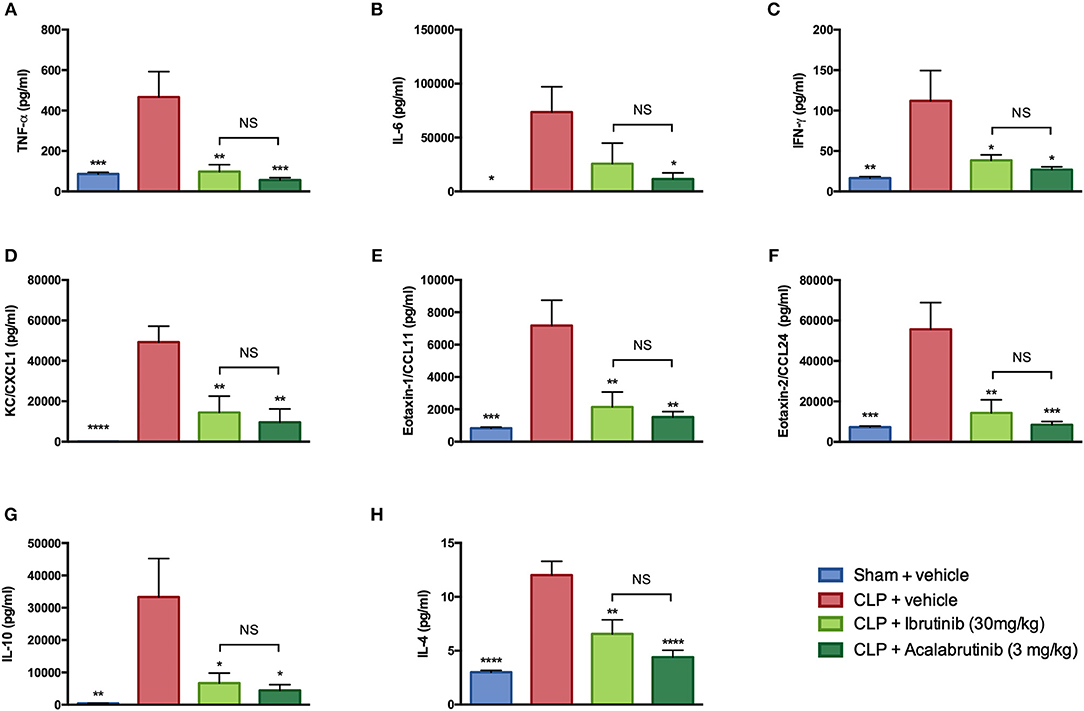
Figure 7. Systemic inflammation in CLP mice is reduced by ibrutinib or acalabrutinib. Mice were randomly assigned to undergo CLP or sham surgery. One hour later, mice were treated with ibrutinib (30 mg/kg i.v.), acalabrutinib (3 mg/kg i.v.), or vehicle (5% DMSO + 30% cyclodextrin i.v.). At 24 h after CLP, blood samples were collected, and the serum concentration of cytokines and chemokines were measured by a multiplex assay (n = 8 per group). (A) TNF-α serum concentration (pg/ml). (B) IL-6 serum concentration (pg/ml). (C) IFN-γ serum concentration (pg/ml). (D) KC/CXCL1 serum concentration (pg/ml). (E) Eotaxin-1/CCL11 serum concentration (pg/ml). (F) Eotaxin-2/CCL24 serum concentration (pg/ml). (G) IL-10 serum concentration (pg/ml). (H) IL-4 serum concentration (pg/ml). All data are expressed as mean ± SEM for n number of observations. Correlations coefficients were determined by Pearson's correlation with P-values based on two-tailed tests a value of ****P < 0.0001, ***P < 0.001, **P < 0.01, *P < 0.05 was considered to be statistically significant.
The data of all other cytokines/chemokines/growth factors that we measured in all groups are provided in Supplementary Figure 1.
Discussion
We show here, for the first time, that administration of two structurally different BTK inhibitors (ibrutinib and acalabrutinib) both ameliorate the cardiac dysfunction (measured as decline in EF, FS, or FAC by echocardiography) caused by CLP-sepsis. The observed decline in EF also was associated with a significant increase in the serum levels of two, well-known biomarkers of left ventricular dysfunction, namely CXCL10 and CXCL11 (24–26). Most notably, ibrutinib or acalabrutinib also attenuated the rises in CXCL10 and CXCL11 caused by CLP-sepsis. In addition, ibrutinib or acalabrutinib also reduced the renal dysfunction (measured as increase in serum urea or creatinine) caused by CLP-sepsis. Thus, both BTK inhibitors reduced the cardiac and renal dysfunction caused by sepsis.
What, then, is the mechanism by which ibrutinib or acalabrutinib reduce the cardiac (renal) dysfunction caused by sepsis? Ibrutinib is a potent BTK inhibitor, but not very specific (as it also inhibits a multitude of other kinases), which is approved by the FDA and the EMA for the use in CLL, MCL, and WM. Acalabrutinib is a potent, but highly specific BTK inhibitor: At a (relatively high) concentration of 1 μM, acalabrutinib strongly inhibited only the following 5 kinases: BTK, Bmx, ErbB4, RIPK2, and TEC, while the same concentration of ibrutinib inhibited 35 kinases. It should be noted that the doses of acalabrutinib and ibrutinib that we used in our study in the mouse resulted in a similar, ~70%, inhibition of BTK activity in septic hearts. We, therefore, propose that inhibition of BTK activity explains the observed beneficial effects of ibrutinib or acalabrutinib in sepsis. The activation of BTK and the subsequent activation of BTK signaling involves (a) phosphorylation of BTK at Tyr223 and (b) the phosphorylation of PLCγ at Tyr1217 by phosphorylated (activated) BTK as the first step in the BTK signaling cascade (27). We report here that sepsis results in significant increases in the phosphorylation of cardiac BTK at Tyr223 and the phosphorylation of PLCγ at Tyr1217, indicating that BTK is activated in septic hearts. Most notably, the activation of BTK negatively correlated with EF indicating that activation of BTK is associated with the cardiac dysfunction in sepsis. Indeed, inhibition of BTK activity with ibrutinib or acalabrutinib in the heart of septic animals reduces the cardiac dysfunction in sepsis suggesting that activation of BTK plays a pivotal role in the pathophysiology of the cardiac dysfunction in sepsis.
What are the mechanisms by which the activation of BTK (in the heart) leads to cardiac dysfunction in sepsis? There is good evidence that (a) the activation of BTK precedes the activation of NF-κB (16), and (b) the activation of NF-κB plays an important role in the cardiac dysfunction in sepsis (28, 29). Specifically, inhibition of the activity of NF-κB attenuates the cardiac dysfunction in sepsis (30, 31). We report here, for the first time, that (a) activation of BTK is associated activation of NF-κB in septic hearts, and (b) inhibition of BTK activity with ibrutinib or acalabrutinib reduces both the activation of NF-κB in septic hearts and the cardiac dysfunction caused by sepsis. Thus, we propose that inhibition of the activation of NF-κB contributes to the observed beneficial effects of the BTK inhibitors ibrutinib and acalabrutinib in sepsis. When challenging BTK KO-mice with LPS, Gabhann and colleagues observed reduced (i) activation of NF-κB p65, (ii) Akt phosphorylation, and (iii) M1 polarization of macrophages (32).
Activation of NF-κB drives the formation of a number of pro- and anti-inflammatory cytokines and chemokines. We report here that CLP-sepsis leads to a significant increase in the serum levels of the pro-inflammatory cytokines TNF-α, IL-6, IFN-γ, anti-inflammatory cytokines IL-10, IL-4 and the chemokines KC/CXCL1, eotaxin-1/CCL11, eotaxin-2/CCL24, all of which importantly contribute to the local and systemic inflammation and organ injury associated with sepsis (33). Most notably, we see the powerful pro-inflammatory cytokine TNF-α to be ameliorated by both BTK inhibitors. TNF-α has been implicated in murine models of sepsis and in humans with sepsis. TNF-α acts in an autocrine and paracrine manner leading to macrophage production and activation, resulting in the release of other proinflammatory cytokines such as IL-6 and IL-8 (34, 35).
Similarly, there is also good evidence that activation of BTK plays a crucial role in the assembly and activation of the NLRP3 inflammasome (17, 18). The activation of the NLRP3 inflammasome has been suggested to play a role in the cardiac dysfunction (36) and the pathophysiology of sepsis (37). Others have reported that inhibition of the assembly and activation of NLRP3 inflammasome protects against microbial sepsis (37). We report here for the first time that (a) activation of BTK is associated with the activation of the NLRP3 inflammasome in septic hearts, and (b) inhibition of BTK activity with ibrutinib and acalabrutinib reduces both the assembly and subsequent activation of the NLRP3 inflammasome in septic hearts (and the cardiac dysfunction caused by sepsis). Thus, we propose that inhibition of the activation of the NLRP3 inflammasome may also contribute to the observed beneficial effects of the BTK inhibitors ibrutinib and acalabrutinib in sepsis.
Activation of the NLRP3 inflammasome drives the formation of IL-1β and IL-18, both of which play an important role in the systemic inflammation and/or organ dysfunction in sepsis (38). Specifically, inhibition of caspase-1 results in an inhibition of IL-18 and IL-1β secretion, which, in turn, attenuated the cardiac dysfunction caused by myocardial ischemia (39). The role of the inflammasome in the pathophysiology of sepsis, however, is still controversial: For example, survival was similar in wild-type and caspase-1/11 knockout mice with sepsis, while the neutralization of IL-1 and IL-18 reduced mortality in endotoxemia (38). Here we show that BTK inhibition results in reduced serum levels of IL-1β, and this was associated with an improvement of cardiac function.
The evaluation of the efficacy of the BTK inhibitors used in our study depends on the assumption that the development of organ dysfunction (and specifically cardiac and renal dysfunction) correlates with outcome. There is good evidence that the occurrence of cardiac and/or renal dysfunction correlates positively with an increase in mortality in patients with sepsis (6). We have, however, not investigated the effects of BTK inhibition on survival in animals with sepsis due to ethical reasons. It would be useful to confirm whether inhibition of BTK activity does, indeed, improve survival in longer models of sepsis (rather than the very acute model employed here).
In addition to inhibiting BTK, ibrutinib and acalabrutinib also strongly inhibit four other kinases. To ensure that inhibition of BTK, indeed, accounts for the inhibition of NF-kB and the inflammasome and ultimately the observed beneficial effects in sepsis, it would be useful to repeat our study in BTK knockout mice. Interestingly, of the kinases which are strongly inhibited by ibrutinib and acalabrutinib, expression of ErbB4 (rather than its activation) may play a role in the cardiac dysfunction and cognitive impairment associated with sepsis (40). In contrast, RIP2 kinase is unlikely to play a significant role in sepsis, as the CLP-induced septic peritonitis was similar in RIP2 knockout mice and their wild-type litter mates (41).
Conclusions
There are currently no specific treatments, which reduce the cardiac dysfunction or, indeed, mortality in sepsis. Our data shows for the first time that two commercially available BTK inhibitors, ibrutinib or acalabrutinib, attenuate the CLP-induced cardiac dysfunction through inhibition of the activation of BTK/NF-κB and/or the NLRP3 inflammasome, which in turn reduces the formation of a number of chemokines and cytokines including TNF-α. Notably, no significant qualitative or quantitative differences were found with two, chemically distinct BTK-inhibitors suggesting that the observed beneficial effects of both compounds in experimental sepsis are likely to be a drug class related effect. Thus, BTK inhibitors are FDA-approved drugs that maybe repurposed for the use in sepsis, but also for other diseases associated with either local or systemic inflammation.
Data Availability
All datasets generated for this study are included in the manuscript and/or the Supplementary Files.
Ethics Statement
This study was carried out in accordance with the recommendations of Home Office guidance on the operation of Animals (Scientific Procedure Act, 1986) published by Her Majesty's Stationary Office, the Guide for the Care and Use of Laboratory Animals of the National Research Council and the ARRIVE guidelines. The protocol was approved by The Animal Welfare Ethics Review Board of Queen Mary University of London and conducted under U.K. home office license number PC5F29685.
Author Contributions
CO'R, GP, SC, MC, and CT conceived and designed the experiment. CO'R, GP, DC, FC, BW, SA, and LS performed the experiments. CO'R, MC, SC, BW, LM, and CT analyzed the data. CO'R, and CT contributed to the writing of the manuscript. MC, FC, SC, BW, LM, and SA contributed to the revision prior to submission.
Funding
CO'R was sponsored by Barts and The London School of Medicine and Dentistry, Queen Mary University of London. This work was, in part, supported by William Harvey Research Limited and the William Harvey Research Foundation, the British Heart Foundation (Award number: FS/13/58/30648 to GP), the Federal Ministry of Education and Research, Germany (Grant 03Z22JN12 to SC, Research Group Translational Septomics, Center for Innovation Competence Septomics), and the German Research Foundation DFG (MA 7082/3-1).
Conflict of Interest Statement
The authors declare that the research was conducted in the absence of any commercial or financial relationships that could be construed as a potential conflict of interest.
Acknowledgments
We would like to thank Jacqueline Fischer for technical assistance.
Supplementary Material
The Supplementary Material for this article can be found online at: https://www.frontiersin.org/articles/10.3389/fimmu.2019.02129/full#supplementary-material
Supplementary Figure 1. Systemic inflammation in CLP mice is reduced by ibrutinib or acalabrutinib. Mice were randomly assigned to undergo CLP or sham surgery. One hour later, mice were treated with ibrutinib (30 mg/kg i.v.), acalabrutinib (3 mg/kg i.v.), or vehicle (5% DMSO + 30% cyclodextrin i.v.). At 24 h after CLP, blood samples were collected, and the serum concentration of cytokines and chemokines were measured by a multiplex assay (n = 8 per group). All data are expressed as mean ± SEM for n number of observations. A value of ****P < 0.0001, *** P < 0.001, **P < 0.01, *P < 0.05 was considered to be statistically significant when compared to the control by one-way ANOVA followed by a Bonferroni's post-hoc test. A value of $$$$P < 0.0001, $$P < 0.01 was considered to be statistically significant when compared to the sham by one-way ANOVA followed by a Bonferroni's post-hoc test.
References
1. Singer M, Deutschman CS, Seymour CW, Shankar-Hari M, Annane D, Bauer M, et al. The third international consensus definitions for sepsis and septic shock (Sepsis-3). JAMA. (2016) 315:801–10. doi: 10.1001/jama.2016.0287
2. Fleischmann C, Scherag A, Adhikari NKJ, Hartog CS, Tsaganos T, Schlattmann P, et al. Assessment of global incidence and mortality of hospital-treated sepsis. Current estimates and limitations. Am J Respir Crit Care Med. (2016) 193:259–72. doi: 10.1164/rccm.201504-0781OC
3. Daniels R. Surviving the first hours in sepsis: getting the basics right (an intensivist's perspective). J Antimicrob Chemother. (2011) 66:ii11–23. doi: 10.1093/jac/dkq515
4. Richards M. Sepsis Management as an NHS Clinical Priority. UK Sepsis Group (2014). Available online at: http://www.england.nhs.uk/wp-content/uploads/2013/12/sepsis-brief.pdf (accessed March 1, 2019).
6. Parrillo JE, Parker MM, Natanson C, Suffredini AF, Danner RL, Cunnion RE, et al. Septic shock in humans. Ann Intern Med. (1990) 113:227. doi: 10.7326/0003-4819-113-3-227
7. Kumar A, Thota V, Dee L, Olson J, Uretz E, Parrillo JE. Tumor necrosis factor alpha and interleukin 1beta are responsible for in vitro myocardial cell depression induced by human septic shock serum. J Exp Med. (1996) 183:949–58. doi: 10.1016/S0300-9572(96)90072-6
8. Martin L, Derwall M, Al Zoubi S, Zechendorf E, Reuter DA, Thiemermann C, et al. The septic heart: current understanding of molecular mechanisms and clinical implications. Chest. (2019) 155:427–37. doi: 10.1016/j.chest.2018.08.1037
9. Tsukada S, Saffran DC, Rawlings DJ, Parolini O, Allen RC, Klisak I, et al. Deficient expression of a B cell cytoplasmic tyrosine kinase in human X-linked agammaglobulinemia. Cell. (1993) 72:279–90. doi: 10.1016/0092-8674(93)90667-F
10. Sanford DS, Wierda WG, Burger JA, Keating MJ, O'Brien SM. Three newly approved drugs for chronic lymphocytic leukemia: incorporating ibrutinib, idelalisib, and obinutuzumab into clinical practice. Clin Lymphoma Myeloma Leuk. (2015) 15:385–91. doi: 10.1016/j.clml.2015.02.019
11. Markham A, Dhillon S. Acalabrutinib: first global approval. Drugs. (2018) 78:139–45. doi: 10.1007/s40265-017-0852-8
12. European Medicines Agency. Imbruvica. (2019). Available online at: https://www.ema.europa.eu/en/medicines/human/EPAR/imbruvica (accessed August 21, 2019).
13. European Medicines Agency. Orphan designation: Acalabrutinib for: Treatment of Chronic Lymphocytic Leukaemia/Small Lymphocytic Lymphoma. (2016). Available online at: https://www.ema.europa.eu/en/medicines/human/orphan-designations/eu3161626 (accessed August 21, 2019).
14. European Medicines Agency. Orphan Designation: Acalabrutinib for: Treatment of Lymphoplasmacytic Lymphoma. (2016). Available online at: https://www.ema.europa.eu/en/medicines/human/orphan-designations/eu3161626 (accessed August 21, 2019).
15. European Medicines Agency. Orphan Designation: Acalabrutinib for: Treatment of Mantle Cell Lymphoma. (2016). Available online at: https://www.ema.europa.eu/en/medicines/human/orphan-designations/eu3161625 (accessed August 21, 2019).
16. Jefferies CA, Doyle S, Brunner C, Dunne A, Brint E, Wietek C, et al. Bruton's tyrosine kinase is a Toll/interleukin-1 receptor domain-binding protein that participates in nuclear factor kappaB activation by Toll-like receptor 4. J Biol Chem. (2003) 278:26258–64. doi: 10.1074/jbc.M301484200
17. Liu X, Pichulik T, Wolz OO, Dang TM, Stutz A, Dillen C, et al. Human NACHT, LRR, and PYD domain–containing protein 3 (NLRP3) inflammasome activity is regulated by and potentially targetable through Bruton tyrosine kinase. J Allergy Clin Immunol. (2017) 140:1054–67.e10. doi: 10.1016/j.jaci.2017.01.017
18. Ito M, Shichita T, Okada M, Komine R, Noguchi Y, Yoshimura A, et al. Bruton's tyrosine kinase is essential for NLRP3 inflammasome activation and contributes to ischaemic brain injury. Nat Commun. (2015) 6:7360. doi: 10.1038/ncomms8360
19. Wu H, Huang Q, Qi Z, Chen Y, Wang A, Chen C, et al. Irreversible inhibition of BTK kinase by a novel highly selective inhibitor CHMFL-BTK-11 suppresses inflammatory response in rheumatoid arthritis model. Sci Rep. (2017) 7:466. doi: 10.1038/s41598-017-00482-4
20. Kilkenny C, Browne WJ, Cuthill IC, Emerson M, Altman DG. Improving bioscience research reporting: the ARRIVE guidelines for reporting animal research. PLoS Biol. (2010) 8:e1000412. doi: 10.1371/journal.pbio.1000412
21. Collino M, Pini A, Mugelli N, Mastroianni R, Bani D, Fantozzi R, et al. Beneficial effect of prolonged heme oxygenase 1 activation in a rat model of chronic heart failure. Dis Model Mech. (2013) 6:1012–20. doi: 10.1242/dmm.011528
22. Morgan E, Varro R, Sepulveda H, Ember JA, Apgar J, Wilson J, et al. Cytometric bead array: a multiplexed assay platform with applications in various areas of biology. Clin Immunol. (2004) 110:252–66. doi: 10.1016/j.clim.2003.11.017
23. Varro R, Chen R, Sepulveda H, Apgar J. Bead-based multianalyte flow immunoassays. In: Methods in Molecular Biology (Clifton, NJ: Humana Press). p. 125–52.
24. Altara R, Mallat Z, Booz GW, Zouein FA. The CXCL10/CXCR3 axis and cardiac inflammation: implications for immunotherapy to treat infectious and noninfectious diseases of the heart. J Immunol Res. (2016) 2016:4396368. doi: 10.1155/2016/4396368
25. Altara R, Manca M, Hessel MH, Gu Y, van Vark LC, Akkerhuis KM, et al. CXCL10 is a circulating inflammatory marker in patients with advanced heart failure: a pilot study. J Cardiovasc Transl Res. (2016) 9:302–14. doi: 10.1007/s12265-016-9703-3
26. Altara R, Gu YM, Struijker-Boudier HAJ, Thijs L, Staessen JA, Blankesteijn WM. Left ventricular dysfunction and CXCR3 ligands in hypertension: from animal experiments to a population-based pilot study. PLoS ONE. (2015) 10:e0141394. doi: 10.1371/journal.pone.0141394
27. Kurosaki T, Maeda A, Ishiai M, Hashimoto A, Inabe K, Takata M. Regulation of the phospholipase C-gamma2 pathway in B cells. Immunol Rev. (2000) 176:19–29. doi: 10.1034/j.1600-065X.2000.00605.x
28. Pritts TA, Moon MR, Wang Q, Hungness ES, Salzman AL, Fischer JE, et al. Activation of NF-kappaB varies in different regions of the gastrointestinal tract during endotoxemia. Shock. (2000) 14:118–22. doi: 10.1097/00024382-200014020-00007
29. Liu SF, Ye X, Malik AB. Pyrrolidine dithiocarbamate prevents I-kappaB degradation and reduces microvascular injury induced by lipopolysaccharide in multiple organs. Mol Pharmacol. (1999) 55:658–67.
30. Al Zoubi S, Chen J, Murphy C, Martin L, Chiazza F, Collotta D, et al. Linagliptin attenuates the cardiac dysfunction associated with experimental sepsis in mice with Pre-existing Type 2 diabetes by inhibiting NF-κB. Front Immunol. (2018) 9:2996. doi: 10.3389/fimmu.2018.02996
31. Chen J, Kieswich JE, Chiazza F, Moyes AJ, Gobbetti T, Purvis GSD, et al. IκB Kinase inhibitor attenuates sepsis-induced cardiac dysfunction in CKD. J Am Soc Nephrol. (2017) 28:94–105. doi: 10.1681/ASN.2015060670
32. Ní Gabhann J, Hams E, Smith S, Wynne C, Byrne JC, Brennan K, et al. Btk regulates macrophage polarization in response to lipopolysaccharide. PLoS ONE. (2014) 9:e85834. doi: 10.1371/journal.pone.0085834
33. Chaudhry H, Zhou J, Zhong Y, Ali MM, McGuire F, Nagarkatti PS, et al. Role of cytokines as a double-edged sword in sepsis. In Vivo. (2013) 27:669–84.
34. Fong Y, Tracey KJ, Moldawer LL, Hesse DG, Manogue KB, Kenney JS, et al. Antibodies to cachectin/tumor necrosis factor reduce interleukin 1 beta and interleukin 6 appearance during lethal bacteremia. J Exp Med. (1989) 170:1627–33. doi: 10.1084/jem.170.5.1627
36. Zhang W, Xu X, Kao R, Mele T, Kvietys P, Martin CM, et al. Cardiac fibroblasts contribute to myocardial dysfunction in mice with sepsis: the role of NLRP3 inflammasome activation. PLoS ONE. (2014) 9:e107639. doi: 10.1371/journal.pone.0107639
37. Lee S, Nakahira K, Dalli J, Siempos II, Norris PC, Colas RA, et al. NLRP3 Inflammasome deficiency protects against microbial sepsis via increased Lipoxin B4 synthesis. Am J Respir Crit Care Med. (2017) 196:713–26. doi: 10.1164/rccm.201604-0892OC
38. Berghe TV, Demon D, Bogaert P, Vandendriessche B, Goethals A, Depuydt B, et al. Simultaneous targeting of IL-1 and IL-18 is required for protection against inflammatory and septic shock. Am J Respir Crit Care Med. (2014) 189:282–91. doi: 10.1164/rccm.201308-1535OC
39. Pomerantz BJ, Reznikov LL, Harken AH, Dinarello CA. Inhibition of caspase 1 reduces human myocardial ischemic dysfunction via inhibition of IL-18 and IL-1beta. Proc Natl Acad Sci USA. (2001) 98:2871–6. doi: 10.1073/pnas.041611398
40. An R, Feng J, Xi C, Xu J, Sun L. miR-146a attenuates sepsis-induced myocardial dysfunction by suppressing IRAK1 and TRAF6 via targeting ErbB4 expression. Oxid Med Cell Longev. (2018) 2018:1–9. doi: 10.1155/2018/7163057
Keywords: Bruton's tyrosine kinase (BTK), sepsis, cardiac dysfunction, ibrutinib, acalabrutinib, NLRP3, NF-κB, mice
Citation: O'Riordan CE, Purvis GSD, Collotta D, Chiazza F, Wissuwa B, Al Zoubi S, Stiehler L, Martin L, Coldewey SM, Collino M and Thiemermann C (2019) Bruton's Tyrosine Kinase Inhibition Attenuates the Cardiac Dysfunction Caused by Cecal Ligation and Puncture in Mice. Front. Immunol. 10:2129. doi: 10.3389/fimmu.2019.02129
Received: 10 April 2019; Accepted: 23 August 2019;
Published: 06 September 2019.
Edited by:
Rudolf Lucas, Augusta University, United StatesReviewed by:
Basilia Zingarelli, Cincinnati Children's Hospital Medical Center, United StatesHelder Mota-Filipe, University of Lisbon, Portugal
Copyright © 2019 O'Riordan, Purvis, Collotta, Chiazza, Wissuwa, Al Zoubi, Stiehler, Martin, Coldewey, Collino and Thiemermann. This is an open-access article distributed under the terms of the Creative Commons Attribution License (CC BY). The use, distribution or reproduction in other forums is permitted, provided the original author(s) and the copyright owner(s) are credited and that the original publication in this journal is cited, in accordance with accepted academic practice. No use, distribution or reproduction is permitted which does not comply with these terms.
*Correspondence: Caroline E. O'Riordan, c.e.oriordan@qmul.ac.uk; Christoph Thiemermann, c.thiemermann@qmul.ac.uk