- 1Pathogen and Microbiome Institute, Northern Arizona University, Flagstaff, AZ, United States
- 2Department of Medical Microbiology and Immunology, University of California, Davis, Davis, CA, United States
- 3Division of Infectious Diseases, Department of Internal Medicine, University of California Davis Medical Center, Sacramento, CA, United States
- 4Valley Fever Center for Excellence, Department of Medicine, University of Arizona College of Medicine-Tucson, Tucson, AZ, United States
Coccidioidomycosis (Valley fever) is a fungal disease caused by the inhalation of Coccidioides posadasii or C. immitis. This neglected disease occurs in the desert areas of the western United States, most notably in California and Arizona, where infections continue to rise. Clinically, coccidioidomycosis ranges from asymptomatic to severe pulmonary disease and can disseminate to the brain, skin, bones, and elsewhere. New estimates suggest as many as 350,000 new cases of coccidioidomycosis occur in the United States each year. Thus, there is an urgent need for the development of a vaccine and new therapeutic drugs against Coccidioides infection. In this review, we discuss the battle against Coccidioides including the development of potential vaccines, the quest for new therapeutic drugs, and our current understanding of the protective host immune response to Coccidioides infection.
Introduction
The Coccidioides genus contains C. immitis and C. posadasii, the etiological agents of Valley fever. This neglected disease occurs primarily in the southwestern United States, most notably in California and Arizona; however, cases have appeared in Washington pointing to an underappreciation of the geographic distribution of this organism (1, 2). Furthermore, cases outside the United States have been occurring in the northern region of Mexico (3) and areas of Central and South America (4, 5). Coccidioides is considered both a primary and opportunistic fungal pathogen occurring in both immunocompetent and immunocompromised individuals causing a spectrum of coccidioidomycosis. Most cases (~60%) are asymptomatic. For the remainder, pulmonary symptoms from underlying acute or progressive pneumonia are the most common reason patients seek medical help (6, 7). Additionally, dissemination can occur affecting a multitude of organs (Figure 1) and lead to the most severe complication, coccidioidal meningitis. Originally, the literature stated that an estimated 150,000 infections occur each year in the United States, and about 1% lead to disseminated disease with a third of those being fatal (7). Host factors strongly influence risk of disseminated disease such as immunosuppression, third trimester of pregnancy, old age, and ethnicity (i.e., African Americans and Filipinos) (6–12). Additionally, host response to treatment varies, current antifungals cause potential adverse side effects, and resistance to antifungals has recently become a concern (13, 14). Furthermore, infections caused by Coccidioides are on the rise (15), and new estimates of the annual number of new U.S. infections are more than twice (350,000) that of previous estimates (16). The reason for the increase in Coccidioides cases is largely unknown; however, factors such as changes in the environment and surveillance methodology could be contributing factors (15). Taken together, there is an urgent need for new antifungal agents, a better of understanding of host response to infection, and the development of a vaccine to combat coccidioidomycosis. Here, we review the current understanding of the host immune response to infection and protection, advances in drug development, and discuss promising approaches to developing a Coccidioides vaccine; a one stop-shop to understand current research in the battle against the Dust Devil.
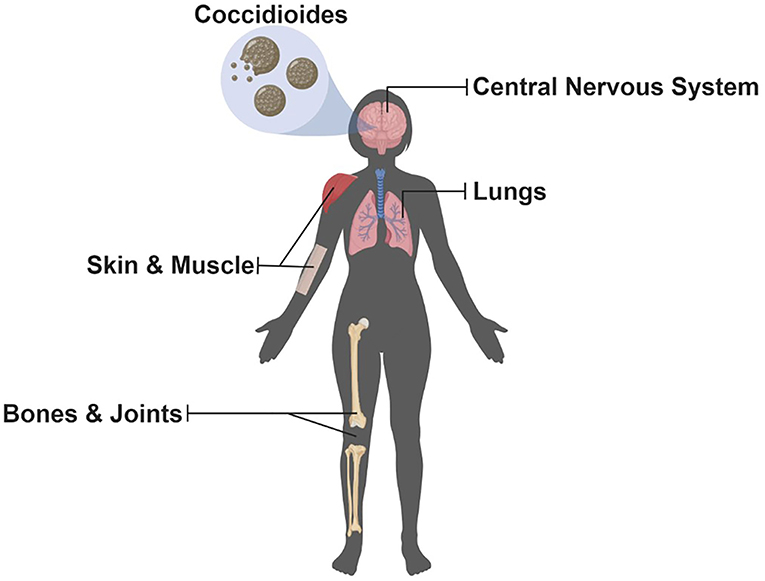
Figure 1. Potential Organs Infected by Coccidioides. Since inhalation is the most common route of infection, the lungs are the most common organ that becomes infected with Coccidioides; however, dissemination can occur allowing for multiple organs, highlighted above, to become infected but are uncommon (Illustration created with BioRender).
Host Immune Response to Coccidioides
Coccidioides grow in the soil as fungal mycelia which segment into arthroconidia (spores) that can then become aerosolized, inhaled, and cause infection. Once a host is infected, arthroconidia transition into mature rupturing spherules within 5 days of infection (17). Therefore, during the early days of infection, morphological variation of Coccidioides is high as the organism is switching from arthroconidia to its parasitic stage, the spherule. In this section, we will discuss what is known about the host immune response to Coccidioides infection, first focusing on the early immune response, and then discussing the protective host immune response to battle coccidioidomycosis.
Early Innate Immune Response to Coccidioides
The innate immune response is the first line of defense against fungal pathogens and clearance relies heavily on phagocytosis by macrophages and neutrophils (18). Phagocytosis can occur on inhaled arthroconidia (3–5 μm) (17) and endospores (2–7 μm); however, mature spherules are too large (15–80 μm) (19) and phagocytic cells fail to engulf these fungal organisms (20). Neutrophils for example can only partially engulf cells that are about 11 μm (21) which is below the threshold of the size of a mature spherule. Studies have shown an influx of neutrophils during infection with Coccidioides (22) and when spherules burst releasing hundreds of endospores (23, 24). Past reports have shown C57BL/6 mice depleted of neutrophils are as susceptible as wild-type mice when infected with wild type Coccidioides (19). Conversely, when mice are vaccinated with a live-attenuated strain of Coccidioides (ΔT, genetically engineered mutant originally designated Δcts2/ard1/cts3), protection relies on the presence of neutrophils (19). Additionally, studies conducted by Gonzalez et al. showed that mice deficient in NADPH oxidase (NOX2) were more susceptible to infection with C. posadasii compared to wild type mice while inducible nitric oxide synthase (iNOS) knock-out mice demonstrated that iNOS does not play a significant role in the control of Coccidioides infection (25, 26). Interestingly, NOX2−/− mice had substantially more infiltration of neutrophils in the lungs compared to wild type mice while iNOS−/− mice had a significant increase of neutrophils at day 7 but not day 11 post challenge. Overall these studies demonstrate that neutrophils play a role in the proper inflammatory response during a Coccidioides infection, and dysregulation of an inflammatory response can be detrimental to the host.
Coccidioides spherules can also escape phagocytosis from macrophages (23). Macrophages vary in size depending on location in the host: 5 μm spleen, 10 μm peritoneal surface, and 15 μm alveoli (27). Studies have demonstrated an evolutionary conserved particle/pathogen size ratio contributes to pathogen clearance and recognition (28), suggesting the inability of macrophages to phagocytose mature spherules. Vaccination studies have demonstrated the influx of macrophages to the lungs of vaccinated mice compared to unvaccinated mice after challenge with Coccidioides (22). However, the role of macrophage subsets (i.e., classically and alternatively activated macrophages or M1 and M2 macrophages) in the protective host immune response against Coccidioides has yet to be elucidated. Studies have shown that mouse peritoneal macrophages stimulated with Coccidioides spherules produce tumor necrosis factor alpha (TNF-α) (29). Furthermore, studies have shown increases in cytokines such as interferon gamma (IFNγ), tumor necrosis factor alpha (TNFα), and interleukin (IL)-17 in mononuclear cells from bronchoalveolar lavage fluid (BALF) from patients with pulmonary coccidioidomycosis (30).
Studies further determined which pattern recognition receptors (PRRs) on peritoneal macrophages were important for recognition of C. posadasii spherules. Using peritoneal macrophages from wild-type cells compared to different knockout mice (i.e., TLR2−/− and MyD88−/−), results demonstrate the response to spherules is dependent on Toll-like receptor 2 (TRL2), myeloid differentiation factor 88 (MyD88), and Dectin-1 (31). Dectin-1 is a C-type lectin receptor shown to interact with components of the fungal cell wall. Studies have shown the importance of this C-type lectin receptor where Dectin1−/− mice infected with Coccidioides demonstrated increased pulmonary fungal burden and decreased Th17 cytokines (32). Studies further suggest that increased susceptibility of C57BL/6 mice to coccidioidomycosis is due to alternative splicing of the Dectin-1 gene (33). Furthermore, studies have identified that null mutations in Dectin-1 predispose hosts to chronic mucosal candidiasis (34). Additionally, people with mutations in the CARD9 gene, Dectin-1, and other C-type lectin receptors signaling through this gene have increased susceptibility to fungal infections (35). Another C-type lectin receptor, the mannose receptor, has been shown to be important in the immune response of human coccidioidomycosis but does not play a role in a murine model of coccidioidomycosis (36–38). Studies demonstrated an association with low mannose-binding lectin (MBL), a collectin that is part of the innate immune system, serum levels among patients exhibiting an active Coccidioides infection compared to otherwise healthy individuals; however, the role of MBL in the pathogenesis of Coccidioides has yet to be determined (37). Recent studies further investigated the role of multiple receptors that use MyD88 to determine which of these receptors are required for resistance against coccidioidomycosis. Of all the surface receptors investigated, results from the studies determined IL-1R1 signaling to be important for protection against coccidioidomycosis (39). Overall, these studies demonstrate the potentially crucial role of C-type lectin receptors and certain TLRs to protect against coccidioidomycosis, but much remains to be done.
Dendritic cells (DCs) act as a bridge between the innate and adaptive immune response. DCs initiate the immune response by capturing antigens and then activate and modulate lymphocytes. Mature DCs have the ability prime naïve T cells toward phenotypes (Th1 and Th17) protective against coccidioidomycosis (discussed below) (40). Studies have demonstrated that DCs pulsed with Coccidioides antigen (spherulin, spherule lysate) can activate DC maturation and lymphocyte proliferation in non-immune individual cells (41). Furthermore, studies investigated the effects of DCs pulsed with a coccidioidal antigen preparation, T27K, using PBMCs from patients with disseminated coccidioidomycosis compared to healthy individuals (42). Results from these studies demonstrate that DCs can be generated by patients with disseminated coccidioidomycosis, and stimulation with T27K led to increased IFN-γ levels in both disseminated and healthy patient samples. Furthermore, studies have demonstrated that suppressing DC responses led to defective T cell responses. BALB/c mice are highly susceptible to infection with Coccidioides, whereas DBA/2 mice are more resistant. Bone-marrow derived DCs (BMDCs) from DBA/2 mice infected with Coccidioides demonstrated an increase in IL-12 secretion and T cell co-stimulatory cell surface molecules compared to BALB/c mice (43). Thus, these studies suggest BALB/c mice could be more susceptible due to impaired DC responses; however, more studies are needed using other mouse strains that are susceptible to infection with Coccidioides.
Despite species divergence of C. immitis and C. posadasii about 5.1 million years ago (44), many studies state that these two species cause similar disease clinically. However, studies from our laboratory allude to differential early host innate responses among species of Coccidioides in a murine model of coccidioidomycosis (45). Since host responses strongly influence clinical disease, differences in the first line of defense against coccidioidomycosis could attribute to differences in outcome of disease. Mice were infected with 1 × 105 arthroconidia of either a C. immitis pure strain (2006), C. immitis hybrid strain (RS), or a C. posadasii pure strain (Silveira). Real-time RT-PCR analysis of mouse lungs shows differential responses across strains. Expression of proinflammatory cytokine levels (IL-1α and IL-17α) were significantly increased in the mice infected with the 2006 strain (C. immitis) at day 5 post infection compared to all other infected mice. Silveira (C. posadasii) infected mice demonstrated an increase in proinflammatory cytokine IL-1β at day 1 post infection and immunoregulatory cytokine IL-10 at day 5 post infection compared to other strains (45).
Coccidioides has other means of avoiding phagocytosis and evading the immune response. Spherule outer wall glycoprotein (SOWgp) is a major antigen present on the cell surface of Coccidioides (46, 47). This glycoprotein is highly expressed during the transition to spherules, and demonstrates immunogenic properties (46, 47). Interestingly, studies have shown that a specific metalloproteinase (Mep1) is secreted during endosporulation, which then digests SOWgp to prevent host recognition (48). Furthermore, mice vaccinated with recombinant SOWgp and then challenged with a C. posadasii strain with the MEP1 gene disrupted demonstrated increased survival compared to the parental or revertant strain (48). Other studies have demonstrated that Coccidioides can suppress nitric oxide (NO) production in macrophages; however, these studies show NO is not critical for in vitro killing of Coccidioides (49). Although these studies give us insights into Coccidioides pathogenesis, more studies are needed to understand the immune evasion strategies of this pathogen.
Protective T-Cell Host Immune Response to Combat Coccidioides
Results from both clinical data and mouse models of coccidioidomycosis have demonstrated that T cell immunity is crucial for protection against coccidioidomycosis. Additionally, deficiency in CD4+ T cells results in increased susceptibility to infection with Coccidioides (50). CD4+ T cells can differentiate into distinct lineages that produce certain cytokines in response to a pathogen. Cytokines such as IL-12 and IFN-γ are associated with T cell helper 1 (Th1) responses, which has been shown to be important for protection in mouse models of coccidioidomycosis (51, 52) and in vitro studies using human PBMCs (53). Additionally, patients with IL-12 and IL-1 receptor deficiencies demonstrate increased dissemination of Coccidioides (54, 55). A Th2 immune response is activated by cytokines such as IL-4 and IL-5 and has been shown to downregulate the host immune response during infection with Coccidioides (51). On the other hand, these cytokines can induce B cell responses which have been shown to play a role in protection in a mouse model of coccidioidomycosis (51, 56, 57). However, the role of Th2 and antibodies in the clearance of Coccidioides has yet to be resolved and requires further study. Additionally, the detection of anti-Coccidioides antibodies for the diagnosis of coccidioidomycosis is not reliable in humans (58). Recently, the role of Th17 responses which produce proinflammatory cytokines such as IL-17 and IL-22 has been investigated (59). Vaccination studies by Hung et al. demonstrate the critical role of Th17 responses in protection against coccidioidomycosis (22). In these studies, mice lacking the IL-17 receptor that were vaccinated with the ΔT strain were highly susceptible to challenge with Coccidioides. Furthermore, mice deficient in IFN-γ and IL-4 receptors were still protected against challenge with Coccidioides equivalent to wild-type mice. Thus, demonstrating conflicting results of the importance of IFN-γ in the protection against coccidioidomycosis. These studies also demonstrate the immune response of ΔT vaccinated mice challenged with Coccidioides is a mixed Th1, Th2, and Th17 response (22). Overall, studies demonstrate that each of these subsets play a role in the protection against coccidioidomycosis.
Along with CD4+ T cells, mouse studies show that CD8+ T cells play a role in protection against infection with Coccidioides (60). Studies have shown an increased percentage of CD8+ T cells were present post challenge among ΔT vaccinated mice compared to non-vaccinated mice (22). Importantly, BALF from patients with coccidioidomycosis demonstrated an increased proportion of CD8+ T cells in patients with acute pulmonary Coccidioides infection compared to all other groups (30). Additionally, studies have shown that CD8+ T cells can compensate for the lack of CD4+ T cells and confer protection against fungal pathogens (60–63). Studies analyzing pediatric patients with coccidioidomycosis demonstrated an overall lower adaptive immune response in persistent disease patients with a trend toward lower CD4+ and CD8+ T cells, and significantly fewer B cells compared to control and resolved patients (64). Additionally, these studies found no difference in Th1 frequencies among patient populations; however, patients with persistent disease had a lower frequency of Th17 and higher T regulatory (Treg) frequencies compared to patients with resolved disease. Therefore, studies from both human and mouse models of coccidioidomycosis have demonstrated an association between increased Th17 responses and resolution of infection.
Development of a Coccidioides Vaccine
Despite earnest efforts, there is currently no clinically available vaccine against any fungal organism; although, early results have been favorable in the development of a Candida vaccine (65). The overall goal of an anti-coccidioidal vaccine is to prevent disease. Immunization against coccidioidomycosis appears possible since patients who have recovered from an initial coccidioidal infection rarely become ill from a second infection and additional exposure (66). The first experimental anti-Coccidioides vaccine developed was the formalin-killed spherule (FKS) vaccine that demonstrated promising results in mice (67). However, human trails established no differences between FKS-vaccinated group and the placebo group (68). Additionally, the FKS-vaccinated group experienced severe side effects at the local injection site. Herein, we discuss various strategies to develop a vaccine to combat coccidioidomycosis.
Live Attenuated Vaccines
Live attenuated strains have proven to be successful in stimulating the immune response similar to a naturally occurring infection (69–72). However, an ideal vaccine candidate needs to have an impeccable safety profile in all populations such as the immunocompromised (73). Although a live vaccine may not be useful in a human clinical setting, understanding the protective host immune response against Coccidioides is imperative to design a suitable and effective recombinant vaccine to combat coccidioidomycosis. For example, chitinase activity in C. posadasii was inhibited by disrupting two chitinase genes (CTS2 and CTS3) and a third gene contiguous to CTS3, to obtain an attenuated mutant that was no longer able to endosporulate, Δcts2/ard1/cts3 (51). This genetically engineered strain demonstrated protection in mice against coccidioidomycosis and is now designated as the ΔT vaccine strain (51, 74). Using this vaccine strain, studies have demonstrated the important parameters for eliciting a protective host immune response against coccidioidomycosis. As discussed above, the ΔT vaccine helped to elucidate the important role of CD4+ T cells, particularly Th1 and Th17 immune responses that are critical for protection (22).
More recently, a homolog of the gene CPS1, a virulence factor found in a maize pathogen (75), was deleted in a strain of C. posadasii (76). This deletion resulted in essentially complete attenuation of pathogenicity in both wild type and immunodeficient mice. Furthermore, mice vaccinated with live ΔCPS1 were protected against an otherwise lethal infection with wild type C. posadasii and C. immitis (76, 77). Further studies demonstrated a primarily Th1-type response in mice vaccinated with ΔCPS1 and challenged with wild-type C. posadasii compared to unvaccinated mice (77). Both the ΔT and ΔCPS1 strains are vital tools needed to determine the protective host immune response needed to battle Coccidioides. Interestingly, both of these mutant strains undergo initial spherulation in the host before arresting growth.
A practical attraction of a live attenuated Coccidioides vaccine candidate is that manufacturing costs to make a clinically feasible product should be low. Production costs have been a road block for an earlier recombinant vaccine (78); however, as with any live vaccine, safety is a critical consideration. Since ΔCPS1 is a complete gene-deletion, reversion is hard to imagine. On the other hand, new mutations in other genes might compensate for the missing gene and result in gain-of-function and cause disease, especially in more immunosuppressed individuals (73, 79). ΔCPS1 is currently being developed as a live vaccine candidate to prevent Valley fever in dogs (80). Should this prove successful, it would provide a proof-of-concept supporting further development to prevent Valley fever in humans. The exact path for this vaccine candidate to humans has yet to be determined. There is no precedent since a live attenuated eukaryotic vaccine has yet to be given FDA approval. Furthermore, the market for a vaccine to prevent Valley fever is relatively small. While there is a very strong public case for preventing this disease (81), it is much more challenging to make a business model with a return on investment competitive with other opportunities for investors. It is likely that a Valley fever vaccine will only be developed if public resources, state or federal, are deemed appropriate for this purpose.
Novel Adjuvants and Protein Vaccines
A safer alternative to attenuated vaccines is the use of recombinant proteins; yet, these may require an adjuvant to strengthen the immune response and optimize efficacy (82). Studies sought to characterize a novel adjuvant, a peptide agonist of the biologically active C-terminal region of human complement C5a referred to as EP67, conjugated to the live ΔT vaccine strain (83). These studies found that BALB/c mice immunized with the EP67-conjugated vaccine demonstrated increased survival rates and reduced fungal burden compared to the non-conjugated vaccine. Additionally, mice given the conjugated vaccine had increased infiltration of macrophages and DCs by day 7 post challenge while neutrophil numbers were decreased by 11 days post challenge compared to the non-conjugated vaccinated mice. Furthermore, the novel adjuvant EP67 increased Th1 and Th17 immune responses; therefore, augmenting T cell immunity and enhancing protective efficacy of the live ΔT vaccine strain (83).
Early studies suggest multivalent vaccines are more effective against coccidioidomycosis compared to a single peptide vaccine (84–86). Early studies introduced rAg2/Pra as a potential vaccine candidate; however, varying routes of challenge led to conflicting results (87, 88). Thus, improved protection efficacy against Coccidioides infection in mice by adding an Coccidioides-specific antigen (CSA) to the rAg2/Pra were completed (84). The inclusion of another antigen Prp2, and development of a combined vaccination of rAg2/Pra+rPrp2, produced significantly improved protection compared to either of the recombinant proteins alone (89). Additionally, recent studies have demonstrated a Ag2/Pra-specific response in mice using a DC-based vaccine which was prepared by transfecting primary bone marrow-derived DCs with a plasmid encoding Ag2/Pra (90). Prior studies demonstrated that the DC-based vaccine reduced fungal burden and increased IFNγ levels in the lung homogeneates from vaccinated mice compared to control mice (91).
Using two-dimensional gel electrophoresis and high-performance liquid chromatography-tandem mass spectrometry (HPLC-MS/MS), studies identified another protein, PMP1 (peroxisomal matrix protein 1), which also demonstrated protection in a mouse model of coccidioidomycosis (92). Additional protective antigens that were used as potential vaccine candidates include PEP1, PLB, and AMN1, which demonstrated enhanced protection as a multivalent vaccine compared to a single antigen alone (85). An alternative approach to a multivalent vaccine to lower cost is the used of epitope-based vaccines (EBV) which has been shown to effectively induce an immune response, is relatively easy to produce, and expected to be safe to use in humans (93, 94). Studies conducted by Hurtgen et al. created a recombinant EBV (rEBV) which incorporated PEP1, AMN1, and PLB into a single epitope-based vaccine which was either admixed with an adjuvant or loaded into glucan particles (GPs) (95). Overall, these studies demonstrated that the rEBV plus GP vaccination was superior to all formulations tested in this study showing enhanced survival, reduced fungal burden, and robust Th1 and Th17 immune responses compared to control mice with GPs alone. GPs are purified, hollow, porous yeast cell-wall particles derived from Saccharomyces cerevisiae. There have been several types of yeast particles created for vaccine development (96).
Recently, studies created a recombinant chimeric polypeptide antigen, rCPa1, that consist of Ag2/Pra, Cs-Ag, Pmp1, and 5 T cell epitopes from PEP1, PLB, and AMN1 from C. posadasii (97). Additionally, they tested the efficacy of rCpa1 encapsulated in differently formulated yeast cell-wall particles. These studies identified a promising vaccine candidate, rCpa1, encapsulated in glucan-chitin particles (GCP-rCpa1) that showed increased survival, significantly reduced fungal burden, and a mixed protective Th1 and Th17 response (97). Additionally, recent studies conducted by Hayden et al. demonstrated that mice immunized with recombinant Ag2 expressed in maize and loaded into GCPs had reduced fungal burden in Coccidioides challenged mice similar to Ag2 derived from Escherichia coli (98). Furthermore, oral administration of Ag2 fused onto a DC carrier peptide (DCpep) demonstrated protective Th17 responses. More studies are needed to characterize these new vaccine candidates to determine if clinical trials are on the horizon. To move into clinical trials, we need to test potential vaccine candidates in multiple animal models including transgenic mice expressing human receptors.
Current Treatments and Drug Discovery
Coccidioidomycosis represents a spectrum of illness ranging from asymptomatic acquisition with resultant immunity to severe and life-threatening disseminated infections. Even in otherwise uncomplicated primary pulmonary infection the symptoms of fever, chills, cough, joint pain, and malaise can last weeks to months (99). Severe cases including dissemination to the skin, bone, or brain (Figure 1) can be difficult to treat and in some cases require life-long antifungal therapy. Currently, the most common management of coccidioidomycosis includes antifungal agents such as fluconazole or itraconazole; however, guidelines suggest an individualized approach to patient management (13). Nevertheless, new concerns of toxicities and side effects, with either acute or long-term use, caused by these agents have seen renewed interest in the development of new agents to combat this disease. Herein, we discuss briefly the current and future treatment options for patients with coccidioidomycosis. A comprehensive review of current treatment options against coccidioidomycosis has recently been published (100).
Polyenes
Amphotericin B has been a widely used agent in the treatment of coccidioidomycosis over the last 50 years (101) and is currently available in multiple intravenous formulations: amphotericin B deoxycholate (AmBd), liposomal amphotericin B (L-AMB), amphotericin B colloidal dispersion (ABCD), and amphotericin B lipid complex (ABLC) (Table 1) (100). Overall, these formulations are met with adverse effects such as nephrotoxicity, hypokalaemia, phlebitis, fever, chills, hepatotoxicity, and anemia (102–105). Historically, long courses of amphotericin B therapy was prescribed in an attempt to provide curative therapy given the lack of an orally available efficacious agent. With the availability of the less toxic triazole antifungals, amphotericin B therapy is reserved for the treatment of patients who are intolerant or refractory to the other available antifungal agents or those with severe disease.
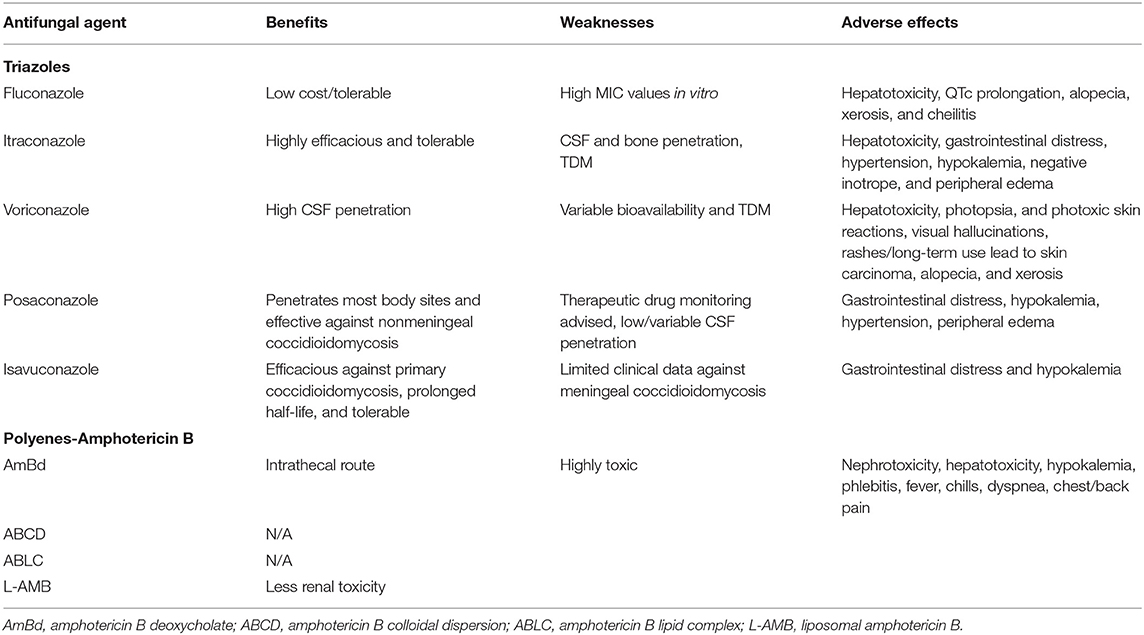
Table 1. A brief overview of antifungal agents benefits, weaknesses, and adverse effects in the treatment of coccidioidomycosis.
There have been numerous studies using animal models that have demonstrated the efficacy of the lipid formulations of amphotericin B therapy against coccidioidomycosis (106–109). Although clinical studies have been sparse, the use of amphotericin B against multiple forms of coccidioidomycosis has demonstrated its efficacy. A retrospective study demonstrated similar efficacy of ABLC and L-AMB in the treatment of severe coccidioidomycosis; however, L-AMB may be the preferred agent with less renal toxicity during treatment compared to ABLC (110). Studies have shown that coccidioidal meningitis treated with amphotericin B deoxycholate via the intrathecal route demonstrates a much more successful treatment compared to the intravenous route (111). However, discussion with those experienced in the treatment of intrathecal therapy is highly recommended if intrathecal therapy is needed during clinical care given the potential morbidity with treatment via this approach (112, 113).
Triazoles
The triazoles used to combat coccidioidomycosis include: fluconazole, itraconazole, voriconazole, posaconazole, and isavuconazole. These triazoles prevent the conversion of lanosterol to ergosterol thus affecting ergosterol synthesis. More specifically, these agents, with significant affinity differences, inhibit cytochrome P450 (CYP)-dependent 14-α-demethylase (114). This affinity difference leads to variability among the antifungal agents in their efficacy, spectrum of activity, and side effect profile. Despite the commercial availability of the triazoles, few have been evaluated in prospective clinical trials due to the regional nature of the disease and the high financial burden of these types of studies. However, the designation of coccidioidomycosis as an orphan disease may facilitate these efforts and allow future antifungal agents to be fully evaluated in prospective fashion.
Fluconazole
Fluconazole is the most frequently prescribed antifungal agent and clinical guidelines suggest it to be a first line agent against multiple forms of coccidioidomycosis (13). Advantages of this agent include low cost, tolerability, the availability of both an oral and intravenous formulations, long half-life, and excellent bioavailability (see Table 1 for an overview of benefits, weaknesses, and adverse effects) [for pharmacokinetics of antifungal agents see recent review article (100)]. Fluconazole has the ability to penetrate most tissues with adequate concentrations within the cerebrospinal (CSF) fluid allowing for the treatment of coccidioidal meningitis (CM) (13, 115). Although adverse effects from the use of fluconazole are largely benign, patients have experienced hepatotoxicity, heart corrected QT interval prolongation, alopecia, xerosis, and cheilitis (100, 116).
A recent study performed a large-scale susceptibility test to understand triazole minimum inhibitory concentrations (MICs) of Coccidioides isolates. These results revealed increased fluconazole MICs across multiple Coccidioides isolates tested (≥16 μg/ml, 37.3% of isolates; ≥32 μg/ml, 7.9% of isolates) (14). This decreased in vitro susceptibility of fluconazole may explain the need for higher fluconazole doses during treatment of coccidioidomycosis (13) and a dose-dependent response to fluconazole has been observed using a murine model of systemic coccidioidomycosis (117); however, this in vitro data has yet to be correlated with clinical outcomes. At this time, no comparative trial has evaluated the dose-dependent response of fluconazole in a randomized study; although, efficacy has been definitively demonstrated (118). Recently, tolerability of long-term fluconazole therapy was assessed, and it was demonstrated that out of 124 patients ~50% had adverse effects (116). The most common adverse effects patients experienced included xerosis, alopecia, and fatigue, which resulted in 65% of patients requiring a therapeutic change.
Itraconazole
Itraconazole is also frequently prescribed to treat coccidioidomycosis (13). This antifungal agent is available primarily as a capsule or oral solution (100). Advantages of using itraconazole include long half-life, efficacy, and tolerability (Table 1), although gastrointestinal side-effects are common with the oral solution, negative inotropic effects on cardiac output have been reported (119). Also, recent reports describe the development of hypertension following itraconazole initiation (120). However, the bioavailability is highly variable and studies have shown itraconazole to exhibit poor CSF (121, 122) and bone penetration (123). Additionally, due to variable bioavailability, therapeutic drug monitoring is recommended to ensure adequate absorption (124).
Despite poor CSF and bone penetration, studies have shown itraconazole to be highly efficacious in the treatment of both osseous coccidioidomycosis and coccidioidal meningitis (118, 125–127). Galgiani et al. compared fluconazole and itraconazole therapy in non-meningeal coccidioidal infections. These studies demonstrated an enhanced response in itraconazole treated patients compared to fluconazole treated patients with osseous coccidioidomycosis (118). Overall, they found itraconazole tended to be slightly more efficacious with fewer relapses compared to fluconazole treated patients. Studies using a murine model of CM demonstrated prolonged survival of mice infected with Coccidioides treated with either 50 mg/kg of itraconazole or fluconazole (125). At this same dose, they found equal clearing of fungi from both brain and kidney; however, itraconazole demonstrated an enhanced clearing of fungi in spinal cord and lungs.
Voriconazole
Voriconazole is often used for patients who are intolerant or refractory to other triazoles in the treatments of coccidioidomycosis (128, 129). The advantages of this antifungal agent include the availability of in both intravenous and oral formulations, high oral bioavailability, wide distribution throughout body, and the ability to penetrate the CSF (Table 1) (100). Nevertheless, voriconazole exhibits many attributes necessitating a working knowledge of its differences compared to other agents. Voriconazole possesses a variable half-life (patient dependent), many drug-drug interactions, hepatotoxicity, visual disturbances, rashes, alopecia, xerosis, and long-term toxicity concerns including cutaneous malignancy (129–134). Due to the variable half-life and the contraindication in patients with renal dysfunction, therapeutic drug monitoring is highly recommended (135).
The efficacy of voriconazole in the treatment of coccidioidomycosis has been demonstrated in retrospective series with favorable outcomes observed in the majority of reported cases including those with bone meningeal and non-meningeal disease (129, 130).
Posaconazole
Posaconazole was initially available only as an oral solution; however, bioavailability was a problem (136). Currently, an intravenous formulation and delayed release oral tablet are now available and the latter demonstrates significant improvement of absorption (137). Posaconazole has been shown to penetrate most sites of the body, but exhibits poor CSF penetration (138, 139). Common adverse effects caused by posaconazole treatment include gastrointestinal distress, hypokalemia, hypertension, peripheral edema, dry mouth, and headache (140, 141). Additionally, there are concerns of potential toxicity with high posaconazole concentrations (142); therefore, therapeutic drug monitoring is suggested (Table 1) (143).
Studies have shown the efficacy of posaconazole for the treatment of coccidioidomycosis in murine models (144, 145). One study demonstrated that mice treated with 10 mg of posaconazole showed >70% sterilization in the spleens and livers of Coccidioides infected mice while itraconazole treated mice resulted in no sterilization in the same tissues tested (144). Clinically, posaconazole treatment has shown efficiency in the treatment of refractory cases of coccidioidomycosis (129, 140, 146, 147).
Isavuconazole
Isavuconazole exist as a prodrug, isavuconazonium sulfate, which is cleaved by plasma esterases into the active moiety. This novel triazole is available in both oral and IV formulations, has a prolonged half-life (~130 h), high bioavailability, and is widely distributed through-out the body (Table 1). Additionally, isavuconazole has shown efficacy clinically against multiple disparate fungal pathogens including the endemic mycoses (148–152). Isavuconazole has been shown to cause adverse effects; the most commonly observed include gastrointestinal disorders (diarrhea and nausea/vomiting) and hypokalaemia (149).
Thus far, there is limited clinical data for the use of isavuconazole therapy on patients with coccidioidomycosis. A prospective study has demonstrated efficacy in the treatment of primary infection with Coccidioides (151) and a retrospective study has demonstrated the potential use of isavuconazole in coccidioidal meningitis in the salvage setting (153).
Combination Therapy
It stands to reason that targeting multiple pathways using a combination of drugs would improve efficacy. However, clinical trials are lacking in the case of combination therapy against coccidioidomycosis. Interestingly, studies using a murine model of coccidioidomycosis have demonstrated the synergistic effects of combination therapy with caspofungin and amphotericin B deoxycholate increasing survival and decreasing fungal burden of mice compared to monotherapy with either treatment (154). This is noteworthy as the echinocandins have little activity against Coccidioides species and should not be used as monotherapy or outside of the salvage setting. Additional reports on the potential utility of combination therapy against coccidioidomycosis is scant, and includes murine models of infection and a single case reports/case series in the salvage setting (111, 155–157). Overall, these cases demonstrate the potential promise of the use of combination therapy against refractory coccidioidomycosis.
New Drug Development
Although recent development of new and less toxic triazoles have been a welcome advance, there is a clear need for more effective and less toxic antifungal agents/therapies, particularly fungicidal oral agents. There are numerous agents currently in development with new modes of action and potentially reduced toxicity. A new formulation of itraconazole (SUBA-itraconazole) (158) has recently become available and clinical studies are ongoing. Novel amphotericin B formulations are currently in development (159). Additionally, some of the drugs in development exhibit broad-spectrum activity against multiple mycoses. Olorofim (formerly F901318) is an orotomide (inhibitor of dihydroorotate dehydrogenase) and has shown excellent in vitro activity against a number of fungal pathogens including Coccidioides, and murine models have suggested fungicidal activity (160) with a phase II clinical trial currently ongoing. Fosmanogepix (formerly APX001), a GPI-anchor inhibitor, has shown activity against a broad spectrum of fungal pathogens (161–166). A recent study evaluated the activity of prodrug APX001 and prodrug analogs against C. immitis and treatment with APX001 in Coccidioides infected mice resulted in significantly longer survival rates and reduced fungal burden than fluconazole or control treated mice (167). Another potential new drug, nikkomycin Z, a chitin synthase inhibitor, is nearing phase 2 clinical trials (168) and has shown similar promise in murine models of infection (169). Also in development are new glucan synthase inhibitors [rezafungin and ibrexafungerp (formerly SCY-078)] (170), a fungal mitochondrial inhibitor (T2307), and a histone deacetylase inhibitor (MGCD290), some with an unknown mode of action (ASP2397), and some repurposed from cancer therapy (sertraline and auranofin) (100, 159, 171, 172).
Conclusion
Due to the rise of Coccidioides infections and concerns regarding toxicity of current antifungals, further research is needed to understand the protective host immune response, new less toxic antifungal drugs, and development of an effective vaccine to prevent coccidioidomycosis. Figure 2 demonstrates each of the three arsenals discussed in this paper in the battle against Coccidioides: host immunity, vaccines, and antifungal drugs. A prophylactic anti-Coccidioides vaccine would help to reduce cost associated with long term medical care and frequently needed life-long antifungal drugs. Live attenuated strains have been useful to elucidate our understanding of the protective host immune response against Coccidioides which requires T cell mediated immunity, particularly a Th1 and Th17 response. Novel formulations of adjuvants/delivery systems along with immunogenic Coccidioides antigens have also been discovered as vaccine candidates. Either could potentially be developed for clinical use. While fluconazole is currently the main antifungal of choice to battle coccidioidomycosis, studies are underway to find less toxic and effective drugs. Altogether, there remains a battle at hand to combat Coccidioides, the Dust Devil.
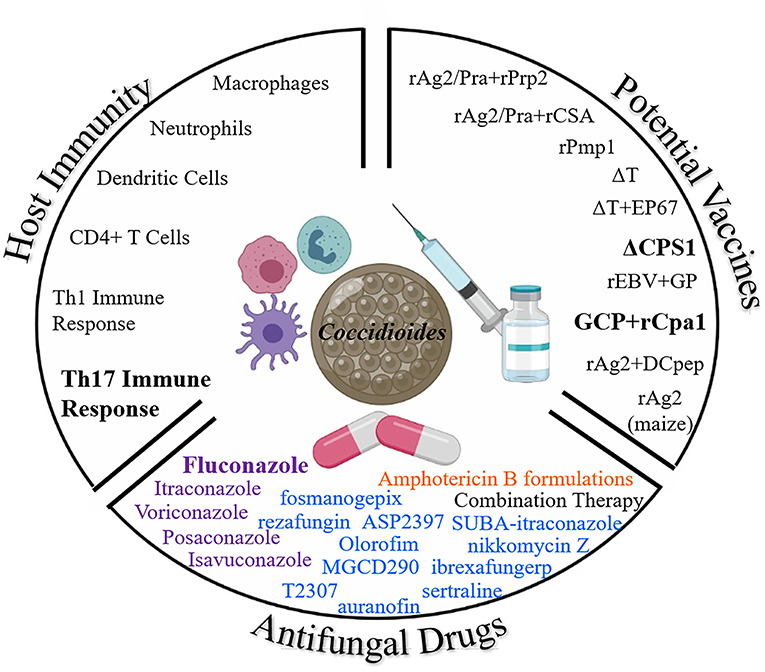
Figure 2. Three Arsenals to Combat Coccidioides. Here we highlight the current battle against Coccidioides from antifungals, potential vaccines, and the protective host immune response. Bolded terms: important for host protection, most common antifungal drug, and most promising current vaccine candidates against coccidioidomycosis. Color coding for antifungal drug classes: purple, Azoles; blue, drugs in development; and orange, Polyenes (Illustration created with BioRender).
Author Contributions
MV, GT, JG, and BB contributed to the writing, editing, and revision of the manuscript.
Funding
This work was supported by the NIH/NIAID 5R21-AI128536 to BB. NIH/NIAID 5U01-AI122275 and 5R01-AI132140 to JG.
Conflict of Interest Statement
JG is Chairman of the Board and a significant stockholder of Valley Fever Solutions, a company developing nikkomycin Z for the treatment of Valley fever.
The remaining authors declare that the research was conducted in the absence of any commercial or financial relationships that could be construed as a potential conflict of interest.
Acknowledgments
GT wishes to acknowledge the Burden Family Gift Fund for Coccidioidomycosis.
References
1. Brown J, Benedict K, Park BJ, Thompson GR 3rd. Coccidioidomycosis: epidemiology. Clin Epidemiol. (2013) 5:185–97. doi: 10.2147/CLEP.S34434
2. Marsden-Haug N, Goldoft M, Ralston C, Limaye AP, Chua J, Hill H, et al. Coccidioidomycosis acquired in Washington State. Clin Infect Dis. (2013) 56:847–50. doi: 10.1093/cid/cis1028
3. Gaona-Flores VA, Campos-Navarro LA, Cervantes-Tovar RM, Alcalá-Martínez E. The epidemiology of fungemia in an infectious diseases hospital in Mexico city: a 10-year retrospective review. Med Mycol. (2016) 54:600–4. doi: 10.1093/mmy/myw017
4. Laniado-Laborín R, Arathoon EG, Canteros C, Muñiz-Salazar R, Rendon A. Coccidioidomycosis in Latin America. Med Mycol. (2019) 57:S46–55. doi: 10.1093/mmy/myy037
5. Van Dyke MCC, Teixeira MM, Barker BM. Fantastic yeasts and where to find them: the hidden diversity of dimorphic fungal pathogens. Curr Opin Microbiol. (2019) 52:55–63. doi: 10.1016/j.mib.2019.05.002
6. Nguyen C, Barker BM, Hoover S, Nix DE, Ampel NM, Frelinger JA, et al. Recent advances in our understanding of the environmental, epidemiological, immunological, and clinical dimensions of coccidioidomycosis. Clin Microbiol Rev. (2013) 26:505–25. doi: 10.1128/CMR.00005-13
7. Odio CD, Marciano BE, Galgiani JN, Holland SM. Risk factors for disseminated coccidioidomycosis, United States. Emerg Infect Dis. (2017) 23:308–11. doi: 10.3201/eid2302.160505
8. Mease L. Pulmonary and extrapulmonary coccidioidomycosis, active component, U.S. Armed Forces, 1999-2011. MSMR. (2012) 19:2–4.
9. Pappagianis D. Epidemiology of coccidioidomycosis. Curr Top Med Mycol. (1988) 2:199–238. doi: 10.1007/978-1-4612-3730-3_6
10. Smith CE, Beard RR. Varieties of coccidioidal infection in relation to the epidemiology and control of the diseases. Am J Public Health Nations Health. (1946) 36:1394–402. doi: 10.2105/AJPH.36.12.1394
11. Sondermeyer G, Lee L, Gilliss D, Tabnak F, Vugia D. Coccidioidomycosis-associated hospitalizations, California, USA, 2000-2011. Emerg Infect Dis. (2013) 19:1590–7. doi: 10.3201/eid1910.130427
12. Wheeler C, Lucas KD, Mohle-Boetani JC. Rates and risk factors for coccidioidomycosis among prison inmates, California, USA, 2011. Emerg Infect Dis. (2015) 21:70–5. doi: 10.3201/eid2101.140836
13. Galgiani JN, Ampel NM, Blair JE, Catanzaro A, Geertsma F, Hoover SE, et al. 2016 Infectious Diseases Society of America (IDSA) clinical practice guideline for the treatment of coccidioidomycosis. Clin Infect Dis. (2016) 63:e112–46. doi: 10.1093/cid/ciw360
14. Thompson GR, Barker BM, Wiederhold NP. Large-scale evaluation of in vitro amphotericin B, triazole, and echinocandin activity against coccidioides species from U.S. Institutions. Antimicrob Agents Chemother. (2017) 61:e02634–16. doi: 10.1128/AAC.02634-16
15. Centers for Disease Control and Prevention. Increase in reported coccidioidomycosis–United States, 1998-2011. MMWR Morb Mortal Wkly Rep. (2013) 62:217–21.
16. Chiller T. Overview of endemic mycoses. In: Vaccine Strategies for Endemic Fungal Pathogens. Rockville, MD: NIAID (2019).
17. Lewis ER, Bowers JR, Barker BM. Dust devil: the life and times of the fungus that causes valley Fever. PLoS Pathog. (2015) 11:e1004762. doi: 10.1371/journal.ppat.1004762
18. Erwig LP, Gow NA. Interactions of fungal pathogens with phagocytes. Nat Rev Microbiol. (2016) 14:163–76. doi: 10.1038/nrmicro.2015.21
19. Hung CY, Jiménez-Alzate Mdel P, Gonzalez A, Wüthrich M, Klein BS, Cole GT. Interleukin-1 receptor but not Toll-like receptor 2 is essential for MyD88-dependent Th17 immunity to Coccidioides infection. Infect Immun. (2014) 82:2106–14. doi: 10.1128/IAI.01579-13
20. Lee CY, Thompson GR, Hastey CJ, Hodge GC, Lunetta JM, Pappagianis D, et al. Coccidioides endospores and spherules draw strong chemotactic, adhesive, and phagocytic responses by individual human neutrophils. PLoS ONE. (2015) 10:e0129522. doi: 10.1371/journal.pone.0129522
21. Herant M, Heinrich V, Dembo M. Mechanics of neutrophil phagocytosis: experiments and quantitative models. J Cell Sci. (2006) 119:1903–13. doi: 10.1242/jcs.02876
22. Hung CY, Gonzalez A, Wüthrich M, Klein BS, Cole GT. Vaccine immunity to coccidioidomycosis occurs by early activation of three signal pathways of T helper cell response (Th1, Th2, and Th17). Infect Immun. (2011) 79:4511–22. doi: 10.1128/IAI.05726-11
23. Drutz DJ, Huppert M. Coccidioidomycosis: factors affecting the host-parasite interaction. J Infect Dis. (1983) 147:372–90. doi: 10.1093/infdis/147.3.372
24. Hung CY, Castro-Lopez N, Cole GT. Card9- and MyD88-mediated gamma interferon and nitric oxide production is essential for resistance to subcutaneous Coccidioides posadasii infection. Infect Immun. (2016) 84:1166–75. doi: 10.1128/IAI.01066-15
25. Gonzalez A, Hung CY, Cole GT. Absence of phagocyte NADPH oxidase 2 leads to severe inflammatory response in lungs of mice infected with Coccidioides. Microb Pathog. (2011) 51:432–41. doi: 10.1016/j.micpath.2011.08.003
26. Gonzalez A, Hung CY, Cole GT. Nitric oxide synthase activity has limited influence on the control of Coccidioides infection in mice. Microb Pathog. (2011) 51:161–8. doi: 10.1016/j.micpath.2011.03.013
28. Champion JA, Walker A, Mitragotri S. Role of particle size in phagocytosis of polymeric microspheres. Pharm Res. (2008) 25:1815–21. doi: 10.1007/s11095-008-9562-y
29. Slagle DC, Cox RA, Kuruganti U. Induction of tumor necrosis factor alpha by spherules of Coccidioides immitis. Infect Immun. (1989) 57:1916–21.
30. Nesbit LA, Knox KS, Nguyen CT, Roesch J, Wheat LJ, Johnson SM, et al. Immunological characterization of bronchoalveolar lavage fluid in patients with acute pulmonary coccidioidomycosis. J Infect Dis. (2013) 208:857–63. doi: 10.1093/infdis/jit246
31. Viriyakosol S, Fierer J, Brown GD, Kirkland TN. Innate immunity to the pathogenic fungus Coccidioides posadasii is dependent on Toll-like receptor 2 and Dectin-1. Infect Immun. (2005) 73:1553–60. doi: 10.1128/IAI.73.3.1553-1560.2005
32. Viriyakosol S, Jimenez Mdel P, Gurney MA, Ashbaugh ME, Fierer J. Dectin-1 is required for resistance to coccidioidomycosis in mice. MBio. (2013) 4:e00597–12. doi: 10.1128/mBio.00597-12
33. del Pilar Jiménez-A M, Viriyakosol S, Walls L, Datta SK, Kirkland T, Heinsbroek SE, et al. Susceptibility to Coccidioides species in C57BL/6 mice is associated with expression of a truncated splice variant of Dectin-1 (Clec7a). Genes Immun. (2008) 9:338–48. doi: 10.1038/gene.2008.23
34. Ferwerda B, Ferwerda G, Plantinga TS, Willment JA, van Spriel AB, Venselaar H, et al. Human dectin-1 deficiency and mucocutaneous fungal infections. N Engl J Med. (2009) 361:1760–7. doi: 10.1056/NEJMoa0901053
35. Lanternier F, Pathan S, Vincent QB, Liu L, Cypowyj S, Prando C, et al. Deep dermatophytosis and inherited CARD9 deficiency. N Engl J Med. (2013) 369:1704–14. doi: 10.1056/NEJMoa1208487
36. Ampel NM. The complex immunology of human coccidioidomycosis. Ann N Y Acad Sci. (2007) 1111:245–58. doi: 10.1196/annals.1406.032
37. Ampel NM, Dionne SO, Giblin A, Podany AB, Galgiani J. Mannose-binding lectin serum levels are low in persons with clinically active coccidioidomycosis. Mycopathologia. (2009) 167:173–80. doi: 10.1007/s11046-008-9172-6
38. Viriyakosol S, Jimenez Mdel P, Saijo S, Fierer J. Neither dectin-2 nor the mannose receptor is required for resistance to Coccidioides immitis in mice. Infect Immun. (2014) 82:1147–56. doi: 10.1128/IAI.01355-13
39. Viriyakosol S, Walls L, Okamoto S, Raz E, Williams DL, Fierer J. Myeloid differentiation factor 88 and interleukin-1R1 signaling contribute to resistance to Coccidioides immitis. Infect Immun. (2018) 86:e00028–18. doi: 10.1128/IAI.00028-18
40. Roy RM, Klein BS. Dendritic cells in antifungal immunity and vaccine design. Cell Host Microbe. (2012) 11:436–46. doi: 10.1016/j.chom.2012.04.005
41. Richards JO, Ampel NM, Galgiani JN, Lake DF. Dendritic cells pulsed with Coccidioides immitis lysate induce antigen-specific naive T cell activation. J Infect Dis. (2001) 184:1220–4. doi: 10.1086/323664
42. Richards JO, Ampel NM, Lake DF. Reversal of coccidioidal anergy in vitro by dendritic cells from patients with disseminated coccidioidomycosis. J Immunol. (2002) 169:2020–5. doi: 10.4049/jimmunol.169.4.2020
43. Awasthi S, Magee DM. Differences in expression of cell surface co-stimulatory molecules, Toll-like receptor genes and secretion of IL-12 by bone marrow-derived dendritic cells from susceptible and resistant mouse strains in response to Coccidioides posadasii. Cell Immunol. (2004) 231:49–55. doi: 10.1016/j.cellimm.2004.11.006
44. Fisher MC, Koenig GL, White TJ, San-Blas G, Negroni R, Alvarez IG, et al. Biogeographic range expansion into South America by Coccidioides immitis mirrors New World patterns of human migration. Proc Natl Acad Sci USA. (2001) 98:4558–62. doi: 10.1073/pnas.071406098
45. Lewis ER, David VR, Doyle AL, Rajabi K, Kiefer JA, Pirrotte P, et al. Differences in host innate responses among Coccidioides isolates in a murine model of pulmonary coccidioidomycosis. Eukaryot Cell. (2015) 14:1043–53. doi: 10.1128/EC.00122-15
46. Hung CY, Yu JJ, Seshan KR, Reichard U, Cole GT. A parasitic phase-specific adhesin of Coccidioides immitis contributes to the virulence of this respiratory Fungal pathogen. Infect Immun. (2002) 70:3443–56. doi: 10.1128/IAI.70.7.3443-3456.2002
47. Hung CY, Ampel NM, Christian L, Seshan KR, Cole GT. A major cell surface antigen of Coccidioides immitis which elicits both humoral and cellular immune responses. Infect Immun. (2000) 68:584–93. doi: 10.1128/IAI.68.2.584-593.2000
48. Hung CY, Seshan KR, Yu JJ, Schaller R, Xue J, Basrur V, et al. A metalloproteinase of Coccidioides posadasii contributes to evasion of host detection. Infect Immun. (2005) 73:6689–703. doi: 10.1128/IAI.73.10.6689-6703.2005
49. Gonzalez A, Hung CY, Cole GT. Coccidioides releases a soluble factor that suppresses nitric oxide production by murine primary macrophages. Microb Pathog. (2011) 50:100–8. doi: 10.1016/j.micpath.2010.11.006
50. Cole GT, Hurtgen BJ, Hung CY. Progress toward a human vaccine against coccidioidomycosis. Curr Fungal Infect Rep. (2012) 6:235–44. doi: 10.1007/s12281-012-0105-y
51. Xue J, Chen X, Selby D, Hung CY, Yu JJ, Cole GT. A genetically engineered live attenuated vaccine of Coccidioides posadasii protects BALB/c mice against coccidioidomycosis. Infect Immun. (2009) 77:3196–208. doi: 10.1128/IAI.00459-09
52. Xue J, Hung CY, Yu JJ, Cole GT. Immune response of vaccinated and non-vaccinated mice to Coccidioides posadasii infection. Vaccine. (2005) 23:3535–44. doi: 10.1016/j.vaccine.2005.01.147
53. Nesbit L, Johnson SM, Pappagianis D, Ampel NM. Polyfunctional T lymphocytes are in the peripheral blood of donors naturally immune to coccidioidomycosis and are not induced by dendritic cells. Infect Immun. (2010) 78:309–15. doi: 10.1128/IAI.00953-09
54. Vinh DC, Masannat F, Dzioba RB, Galgiani JN, Holland SM. Refractory disseminated coccidioidomycosis and mycobacteriosis in interferon-gamma receptor 1 deficiency. Clin Infect Dis. (2009) 49:e62–5. doi: 10.1086/605532
55. Vinh DC, Schwartz B, Hsu AP, Miranda DJ, Valdez PA, Fink D, et al. Interleukin-12 receptor beta1 deficiency predisposing to disseminated coccidioidomycosis. Clin Infect Dis. (2011) 52:e99–102. doi: 10.1093/cid/ciq215
56. Magee DM, Friedberg RL, Woitaske MD, Johnston SA, Cox RA. Role of B cells in vaccine-induced immunity against coccidioidomycosis. Infect Immun. (2005) 73:7011–3. doi: 10.1128/IAI.73.10.7011-7013.2005
57. Cole GT, Xue JM, Okeke CN, Tarcha EJ, Basrur V, Schaller RA, et al. A vaccine against coccidioidomycosis is justified and attainable. Med Mycol. (2004) 42:189–216. doi: 10.1080/13693780410001687349
58. Kassis C, Zaidi S, Kuberski T, Moran A, Gonzalez O, Hussain S, et al. Role of Coccidioides antigen testing in the cerebrospinal fluid for the diagnosis of coccidioidal meningitis. Clin Infect Dis. (2015) 61:1521–6. doi: 10.1093/cid/civ585
59. Lin Y, Slight SR, Khader SA. Th17 cytokines and vaccine-induced immunity. Semin Immunopathol. (2010) 32:79–90. doi: 10.1007/s00281-009-0191-2
60. Fierer J, Waters C, Walls L. Both CD4+ and CD8+ T cells can mediate vaccine-induced protection against Coccidioides immitis infection in mice. J Infect Dis. (2006) 193:1323–31. doi: 10.1086/502972
61. Nanjappa SG, Heninger E, Wüthrich M, Gasper DJ, Klein BS. Tc17 cells mediate vaccine immunity against lethal fungal pneumonia in immune deficient hosts lacking CD4+ T cells. PLoS Pathog. (2012) 8:e1002771. doi: 10.1371/journal.ppat.1002771
62. Nanjappa SG, Heninger E, Wüthrich M, Sullivan T, Klein B. Protective antifungal memory CD8(+) T cells are maintained in the absence of CD4(+) T cell help and cognate antigen in mice. J Clin Invest. (2012) 122:987–99. doi: 10.1172/JCI58762
63. Wuthrich M, Filutowicz HI, Warner T, Deepe GS, Klein BS. Vaccine immunity to pathogenic fungi overcomes the requirement for CD4 help in exogenous antigen presentation to CD8+ T cells: implications for vaccine development in immune-deficient hosts. J Exp Med. (2003) 197:1405–16. doi: 10.1084/jem.20030109
64. Davini D, Naeem F, Phong A, Al-Kuhlani M, Valentine KM, McCarty J, et al. Elevated regulatory T cells at diagnosis of Coccidioides infection associates with chronicity in pediatric patients. J Allergy Clin Immunol. (2018) 142:1971–4.e7. doi: 10.1016/j.jaci.2018.10.022
65. Edwards JE Jr, Schwartz MM, Schmidt CS, Sobel JD, Nyirjesy P, Schodel F, et al. A fungal immunotherapeutic vaccine (NDV-3A) for treatment of recurrent vulvovaginal candidiasis-A phase 2 randomized, double-blind, placebo-controlled trial. Clin Infect Dis. (2018) 66:1928–36. doi: 10.1093/cid/ciy185
66. Kirkland TN. The quest for a vaccine against coccidioidomycosis: a neglected disease of the Americas. J Fungi (Basel). (2016) 2:E34. doi: 10.3390/jof2040034
67. Levine HB, Cobb JM, Smith CE. Immunity to coccidioi-domycosis induced in mice by purified spherule, arthrospore, and mycelial vaccines. Trans N Y Acad Sci. (1960) 22:436–49. doi: 10.1111/j.2164-0947.1960.tb00711.x
68. Pappagianis D. Evaluation of the protective efficacy of the killed Coccidioides immitis spherule vaccine in humans. The Valley Fever Vaccine Study Group. Am Rev Respir Dis. (1993) 148:656–60. doi: 10.1164/ajrccm/148.3.656
69. Saville SP, Lazzell AL, Chaturvedi AK, Monteagudo C, Lopez-Ribot JL. Efficacy of a genetically engineered Candida albicans tet-NRG1 strain as an experimental live attenuated vaccine against hematogenously disseminated candidiasis. Clin Vaccine Immunol. (2009) 16:430–2. doi: 10.1128/CVI.00480-08
70. Van Dyke MCC, Chaturvedi AK, Hardison SE, Leopold Wager CM, Castro-Lopez N, Hole CR, et al. Induction of broad-spectrum protective immunity against disparate Cryptococcus serotypes. Front Immunol. (2017) 8:1359. doi: 10.3389/fimmu.2017.01359
71. Wormley FL Jr, Perfect JR, Steele C, Cox GM. Protection against cryptococcosis by using a murine gamma interferon-producing Cryptococcus neoformans strain. Infect Immun. (2007) 75:1453–62. doi: 10.1128/IAI.00274-06
72. Wozniak KL, Ravi S, Macias S, Young ML, Olszewski MA, Steele C, et al. Insights into the mechanisms of protective immunity against Cryptococcus neoformans infection using a mouse model of pulmonary cryptococcosis. PLoS ONE. (2009) 4:e6854. doi: 10.1371/journal.pone.0006854
73. Levine MM, Sztein MB. Vaccine development strategies for improving immunization: the role of modern immunology. Nat Immunol. (2004) 5:460–4. doi: 10.1038/ni0504-460
74. Hung CY, Castro-Lopez N, Cole GT. Vaccinated C57BL/6 mice develop protective and memory T cell responses to Coccidioides posadasii infection in the absence of interleukin-10. Infect Immun. (2014) 82:903–13. doi: 10.1128/IAI.01148-13
75. Lu SW, Kroken S, Lee BN, Robbertse B, Churchill AC, Yoder OC, et al. A novel class of gene controlling virulence in plant pathogenic ascomycete fungi. Proc Natl Acad Sci USA. (2003) 100:5980–5. doi: 10.1073/pnas.0931375100
76. Narra HP, Shubitz LF, Mandel MA, Trinh HT, Griffin K, Buntzman AS, et al. A Coccidioides posadasii CPS1 deletion mutant is avirulent and protects mice from lethal infection. Infect Immun. (2016) 84:3007–16. doi: 10.1128/IAI.00633-16
77. Shubitz LF, Powell DA, Trinh HT, Lewis ML, Orbach MJ, Frelinger JA, et al. Viable spores of Coccidioides posadasii Deltacps1 are required for vaccination and provide long lasting immunity. Vaccine. (2018) 36:3375–80. doi: 10.1016/j.vaccine.2018.04.026
78. Galgiani JN. Vaccines to prevent systemic mycoses: holy grails meet translational realities. J Infect Dis. (2008) 197:938–40. doi: 10.1086/529205
79. Pirofski LA, Casadevall A. Use of licensed vaccines for active immunization of the immunocompromised host. Clin Microbiol Rev. (1998) 11:1–26. doi: 10.1128/CMR.11.1.1
80. Shubitz LFBR, Robb EJ, Powell Da, Bosco-Lauth AM, Hartwig A, Trinh HT, et al. A canine target species challenge model to evaluate efficacy of a coccidioidomycosis vaccine. In: Infectious Diseases of Society of America. Washington, DC (2019).
81. Wilson L, Ting J, Lin H, Shah R, MacLean M, Peterson MW, et al. The rise of valley fever: prevalence and cost burden of coccidioidomycosis infection in California. Int J Environ Res Public Health. (2019) 16:1113. doi: 10.3390/ijerph16071113
82. Levitz SM, Golenbock DT. Beyond empiricism: informing vaccine development through innate immunity research. Cell. (2012) 148:1284–92. doi: 10.1016/j.cell.2012.02.012
83. Hung CY, Hurtgen BJ, Bellecourt M, Sanderson SD, Morgan EL, Cole GT. An agonist of human complement fragment C5a enhances vaccine immunity against Coccidioides infection. Vaccine. (2012) 30:4681–90. doi: 10.1016/j.vaccine.2012.04.084
84. Shubitz LF, Yu JJ, Hung CY, Kirkland TN, Peng T, Perrill R, et al. Improved protection of mice against lethal respiratory infection with Coccidioides posadasii using two recombinant antigens expressed as a single protein. Vaccine. (2006) 24:5904–11. doi: 10.1016/j.vaccine.2006.04.002
85. Tarcha EJ, Basrur V, Hung CY, Gardner MJ, Cole GT. Multivalent recombinant protein vaccine against coccidioidomycosis. Infect Immun. (2006) 74:5802–13. doi: 10.1128/IAI.00961-06
86. Tarcha EJ, Basrur V, Hung CY, Gardner MJ, Cole GT. A recombinant aspartyl protease of Coccidioides posadasii induces protection against pulmonary coccidioidomycosis in mice. Infect Immun. (2006) 74:516–27. doi: 10.1128/IAI.74.1.516-527.2006
87. Jiang C, Magee DM, Ivey FD, Cox RA. Role of signal sequence in vaccine-induced protection against experimental coccidioidomycosis. Infect Immun. (2002) 70:3539–45. doi: 10.1128/IAI.70.7.3539-3545.2002
88. Jiang C, Magee DM, Quitugua TN, Cox RA. Genetic vaccination against Coccidioides immitis: comparison of vaccine efficacy of recombinant antigen 2 and antigen 2 cDNA. Infect Immun. (1999) 67:630–5.
89. Herr RA, Hung CY, Cole GT. Evaluation of two homologous proline-rich proteins of Coccidioides posadasii as candidate vaccines against coccidioidomycosis. Infect Immun. (2007) 75:5777–87. doi: 10.1128/IAI.00807-07
90. Awasthi S, Vilekar P, Conkleton A, Rahman N. Dendritic cell-based immunization induces Coccidioides Ag2/PRA-specific immune response. Vaccine. (2019) 37:1685–91. doi: 10.1016/j.vaccine.2019.01.034
91. Awasthi S, Awasthi V, Magee DM, Coalson JJ. Efficacy of antigen 2/proline-rich antigen cDNA-transfected dendritic cells in immunization of mice against Coccidioides posadasii. J Immunol. (2005) 175:3900–6. doi: 10.4049/jimmunol.175.6.3900
92. Orsborn KI, Shubitz LF, Peng T, Kellner EM, Orbach MJ, Haynes PA, et al. Protein expression profiling of Coccidioides posadasii by two-dimensional differential in-gel electrophoresis and evaluation of a newly recognized peroxisomal matrix protein as a recombinant vaccine candidate. Infect Immun. (2006) 74:1865–72. doi: 10.1128/IAI.74.3.1865-1872.2006
93. Rosa DS, Ribeiro SP, Cunha-Neto E. CD4+ T cell epitope discovery and rational vaccine design. Arch Immunol Ther Exp (Warsz). (2010) 58:121–30. doi: 10.1007/s00005-010-0067-0
94. Cole GT, Hung CY, Sanderson SD, Hurtgen BJ, Wüthrich M, Klein BS, et al. Novel strategies to enhance vaccine immunity against coccidioidomycosis. PLoS Pathog. (2013) 9:e1003768. doi: 10.1371/journal.ppat.1003768
95. Hurtgen BJ, Hung CY, Ostroff GR, Levitz SM, Cole GT. Construction and evaluation of a novel recombinant T cell epitope-based vaccine against coccidioidomycosis. Infect Immun. (2012) 80:3960–74. doi: 10.1128/IAI.00566-12
96. Young SH, Ostroff GR, Zeidler-Erdely PC, Roberts JR, Antonini JM, Castranova V. A comparison of the pulmonary inflammatory potential of different components of yeast cell wall. J Toxicol Environ Health A. (2007) 70:1116–24. doi: 10.1080/15287390701212224
97. Hung CY, Zhang H, Castro-Lopez N, Ostroff GR, Khoshlenar P, Abraham A, et al. Glucan-chitin particles enhance Th17 response and improve protective efficacy of a multivalent antigen (rCpa1) against pulmonary Coccidioides posadasii infection. Infect Immun. (2018) 86:e00070–18. doi: 10.1128/IAI.00070-18
98. Hayden CA, Hung CY, Zhang H, Negron A, Esquerra R, Ostroff G, et al. Maize-produced Ag2 as a subunit vaccine for valley fever. J Infect Dis. (2019) 220:615–23. doi: 10.1093/infdis/jiz196
99. Blair JE, Chang YH, Cheng MR, Vaszar LT, Vikram HR, Orenstein R, et al. Characteristics of patients with mild to moderate primary pulmonary coccidioidomycosis. Emerg Infect Dis. (2014) 20:983–90. doi: 10.3201/eid2006.131842
100. Thompson GR 3rd, Lewis JS 2nd, Nix DE, Patterson TF. Current concepts and future directions in the pharmacology and treatment of coccidioidomycosis. Med Mycol. (2019) 57:S76–84. doi: 10.1093/mmy/myy029
101. Einstein HE, Holeman CW Jr, Sandidge LL, Holden DH. Coccidioidal meningitis. The use of amphotericin B in treatment. Calif Med. (1961) 94:339–43.
102. Roden MM, Nelson LD, Knudsen TA, Jarosinski PF, Starling JM, Shiflett SE, et al. Triad of acute infusion-related reactions associated with liposomal amphotericin B: analysis of clinical and epidemiological characteristics. Clin Infect Dis. (2003) 36:1213–20. doi: 10.1086/374553
103. Sawaya BP, Briggs JP, Schnermann J. Amphotericin B nephrotoxicity: the adverse consequences of altered membrane properties. J Am Soc Nephrol. (1995) 6:154–64.
104. Sawaya BP, Weihprecht H, Campbell WR, Lorenz JN, Webb RC, Briggs JP, et al. Direct vasoconstriction as a possible cause for amphotericin B-induced nephrotoxicity in rats. J Clin Invest. (1991) 87:2097–107. doi: 10.1172/JCI115240
105. Yeo EJ, Ryu JH, Cho YS, Chun YS, Huang LE, Kim MS, et al. Amphotericin B blunts erythropoietin response to hypoxia by reinforcing FIH-mediated repression of HIF-1. Blood. (2006) 107:916–23. doi: 10.1182/blood-2005-06-2564
106. Albert MM, Adams K, Luther MJ, Sun SH, Graybill JR. Efficacy of AmBisome in murine coccidioidomycosis. J Med Vet Mycol. (1994) 32:467–71. doi: 10.1080/02681219480000621
107. Clemons KV, Capilla J, Sobel RA, Martinez M, Tong AJ, Stevens DA. Comparative efficacies of lipid-complexed amphotericin B and liposomal amphotericin B against coccidioidal meningitis in rabbits. Antimicrob Agents Chemother. (2009) 53:1858–62. doi: 10.1128/AAC.01538-08
108. Clemons KV, Sobel RA, Williams PL, Pappagianis D, Stevens DA. Efficacy of intravenous liposomal amphotericin B (AmBisome) against coccidioidal meningitis in rabbits. Antimicrob Agents Chemother. (2002) 46:2420–6. doi: 10.1128/AAC.46.8.2420-2426.2002
109. González GM, Tijerina R, Najvar LK, Bocanegra R, Rinaldi MG, Graybill JR. Efficacies of amphotericin B (AMB) lipid complex, AMB colloidal dispersion, liposomal AMB, and conventional AMB in treatment of murine coccidioidomycosis. Antimicrob Agents Chemother. (2004) 48:2140–3. doi: 10.1128/AAC.48.6.2140-2143.2004
110. Sidhu R, Lash DB, Heidari A, Natarajan P, Johnson RH. Evaluation of amphotericin B lipid formulations for treatment of severe coccidioidomycosis. Antimicrob Agents Chemother. (2018) 62:e02293–17. doi: 10.1128/AAC.02293-17
111. Mathisen G, Shelub A, Truong J, Wigen C. Coccidioidal meningitis: clinical presentation and management in the fluconazole era. Medicine (Baltimore). (2010) 89:251–84. doi: 10.1097/MD.0b013e3181f378a8
112. Hodge G, Cohen SH, Thompson GR 3rd. In vitro interactions between amphotericin B and hydrocortisone: potential implications for intrathecal therapy. Med Mycol. (2015) 53:749–53. doi: 10.1093/mmy/myv047
113. Stevens DA, Shatsky SA. Intrathecal amphotericin in the management of coccidioidal meningitis. Semin Respir Infect. (2001) 16:263–9. doi: 10.1053/srin.2001.29298
114. van den Bossche H, Willemsens G, Cools W, Lauwers WF, Le Jeune L. Biochemical effects of miconazole on fungi. II. Inhibition of ergosterol biosynthesis in Candida albicans. Chem Biol Interact. (1978) 21:59–78. doi: 10.1016/0009-2797(78)90068-6
115. Arndt CA, Walsh TJ, McCully CL, Balis FM, Pizzo PA, Poplack DG. Fluconazole penetration into cerebrospinal fluid: implications for treating fungal infections of the central nervous system. J Infect Dis. (1988) 157:178–80. doi: 10.1093/infdis/157.1.178
116. Davis MR, Nguyen MH, Donnelley MA, Thompson IIIGR. Tolerability of long-term fluconazole therapy. J Antimicrob Chemother. (2019) 74:768–71. doi: 10.1093/jac/dky501
117. Clemons KV, Hanson LH, Perlman AM, Stevens DA. Efficacy of SCH39304 and fluconazole in a murine model of disseminated coccidioidomycosis. Antimicrob Agents Chemother. (1990) 34:928–30. doi: 10.1128/AAC.34.5.928
118. Galgiani JN, Catanzaro A, Cloud GA, Johnson RH, Williams PL, Mirels LF, et al. Comparison of oral fluconazole and itraconazole for progressive, nonmeningeal coccidioidomycosis. A randomized, double-blind trial. Mycoses Study Group. Ann Intern Med. (2000) 133:676–86. doi: 10.7326/0003-4819-133-9-200011070-00009
119. Qu Y, Fang M, Gao B, Amouzadeh HR, Li N, Narayanan P, et al. Itraconazole decreases left ventricular contractility in isolated rabbit heart: mechanism of action. Toxicol Appl Pharmacol. (2013) 268:113–22. doi: 10.1016/j.taap.2013.01.029
120. Hoffmann WJ, McHardy I, Thompson GR 3rd. Itraconazole induced hypertension and hypokalemia: Mechanistic evaluation. Mycoses. (2018) 61:337–9. doi: 10.1111/myc.12749
121. Perfect JR, Durack DT. Penetration of imidazoles and triazoles into cerebrospinal fluid of rabbits. J Antimicrob Chemother. (1985) 16:81–6. doi: 10.1093/jac/16.1.81
122. Van Cauteren H, Heykants J, De Coster R, Cauwenbergh G. Itraconazole: pharmacologic studies in animals and humans. Rev Infect Dis. (1987) 9(Suppl 1):S43–6. doi: 10.1093/clinids/9.Supplement_1.S43
123. Zhu ES, Thompson GR, Kreulen C, Giza E. Amphotericin B-impregnated bone cement to treat refractory coccidioidal osteomyelitis. Antimicrob Agents Chemother. (2013) 57:6341–3. doi: 10.1128/AAC.00963-13
124. Andes D, Pascual A, Marchetti O. Antifungal therapeutic drug monitoring: established and emerging indications. Antimicrob Agents Chemother. (2009) 53:24–34. doi: 10.1128/AAC.00705-08
125. Kamberi P, Sobel RA, Clemons KV, Waldvogel A, Striebel JM, Williams PL, et al. Comparison of itraconazole and fluconazole treatments in a murine model of coccidioidal meningitis. Antimicrob Agents Chemother. (2007) 51:998–1003. doi: 10.1128/AAC.00332-06
126. Sorensen KN, Sobel RA, Clemons KV, Pappagianis D, Stevens DA, Williams PL. Comparison of fluconazole and itraconazole in a rabbit model of coccidioidal meningitis. Antimicrob Agents Chemother. (2000) 44:1512–7. doi: 10.1128/AAC.44.6.1512-1517.2000
127. Tucker RM, Denning DW, Dupont B, Stevens DA. Itraconazole therapy for chronic coccidioidal meningitis. Ann Intern Med. (1990) 112:108–12. doi: 10.7326/0003-4819-112-2-108
128. Johnson RH, Einstein HE. Coccidioidal meningitis. Clin Infect Dis. (2006) 42:103–7. doi: 10.1086/497596
129. Kim MM, Vikram HR, Kusne S, Seville MT, Blair JE. Treatment of refractory coccidioidomycosis with voriconazole or posaconazole. Clin Infect Dis. (2011) 53:1060–6. doi: 10.1093/cid/cir642
130. Freifeld A, Proia L, Andes D, Baddour LM, Blair J, Spellberg B, et al. Voriconazole use for endemic fungal infections. Antimicrob Agents Chemother. (2009) 53:1648–51. doi: 10.1128/AAC.01148-07
131. Haylett AK, Felton S, Denning DW, Rhodes LE. Voriconazole-induced photosensitivity: photobiological assessment of a case series of 12 patients. Br J Dermatol. (2013) 168:179–85. doi: 10.1111/j.1365-2133.2012.11196.x
132. Kinoshita J, Iwata N, Ohba M, Kimotsuki T, Yasuda M. Mechanism of voriconazole-induced transient visual disturbance: reversible dysfunction of retinal ON-bipolar cells in monkeys. Invest Ophthalmol Vis Sci. (2011) 52:5058–63. doi: 10.1167/iovs.11-7183
133. Malani AN, Kerr L, Obear J, Singal B, Kauffman CA. Alopecia and nail changes associated with voriconazole therapy. Clin Infect Dis. (2014) 59:e61–5. doi: 10.1093/cid/ciu275
134. Pascual A, Calandra T, Bolay S, Buclin T, Bille J, Marchetti O. Voriconazole therapeutic drug monitoring in patients with invasive mycoses improves efficacy and safety outcomes. Clin Infect Dis. (2008) 46:201–11. doi: 10.1086/524669
135. Kim SH, Kwon JC, Park C, Han S, Yim DS, Choi JK, et al. Therapeutic drug monitoring and safety of intravenous voriconazole formulated with sulfobutylether beta-cyclodextrin in haematological patients with renal impairment. Mycoses. (2016) 59:644–51. doi: 10.1111/myc.12517
136. Thompson GR, Rinaldi MG, Pennick G, Dorsey SA, Patterson TF, Lewis JS. Posaconazole therapeutic drug monitoring: a reference laboratory experience. Antimicrob Agents Chemother. (2009) 53:2223–4. doi: 10.1128/AAC.00240-09
137. Pham AN, Bubalo JS, Lewis JS 2nd. Comparison of posaconazole serum concentrations from haematological cancer patients on posaconazole tablet and oral suspension for treatment and prevention of invasive fungal infections. Mycoses. (2016) 59:226–33. doi: 10.1111/myc.12452
138. Reinwald M, Uharek L, Lampe D, Grobosch T, Thiel E, Schwartz S. Limited penetration of posaconazole into cerebrospinal fluid in an allogeneic stem cell recipient with invasive pulmonary aspergillosis. Bone Marrow Transplant. (2009) 44:269–70. doi: 10.1038/bmt.2009.17
139. Rüping MJ, Albermann N, Ebinger F, Burckhardt I, Beisel C, Müller C, et al. Posaconazole concentrations in the central nervous system. J Antimicrob Chemother. (2008) 62:1468–70. doi: 10.1093/jac/dkn409
140. Catanzaro A, Cloud GA, Stevens DA, Levine BE, Williams PL, Johnson RH. Safety, tolerance, and efficacy of posaconazole therapy in patients with nonmeningeal disseminated or chronic pulmonary coccidioidomycosis. Clin Infect Dis. (2007) 45:562–8. doi: 10.1086/519937
141. Thompson GR 3rd, Chang D, Wittenberg RR, McHardy I, Semrad A. In vivo 11beta-hydroxysteroid dehydrogenase inhibition in posaconazole-induced hypertension and hypokalemia. Antimicrob Agents Chemother. (2017) 61:e00760–17. doi: 10.1128/AAC.00760-17
142. Nguyen MH, Davis MR, Wittenberg R, Mchardy I, Baddley JW, Young BY, et al. Posaconazole serum drug levels associated with pseudohyperaldosteronism. Clin Infect Dis. (2019). doi: 10.1093/cid/ciz741. [Epub ahead of print]
143. Patterson TF, Thompson GR 3rd, Denning DW, Fishman JA, Hadley S, Herbrecht R, et al. Practice guidelines for the diagnosis and management of aspergillosis: 2016 update by the Infectious Diseases Society of America. Clin Infect Dis. (2016) 63:e1–60. doi: 10.1093/cid/ciw326
144. González GM, Tijerina R, Najvar LK, Bocanegra R, Rinaldi M, Loebenberg D, et al. In vitro and in vivo activities of posaconazole against Coccidioides immitis. Antimicrob Agents Chemother. (2002) 46:1352–6. doi: 10.1128/AAC.46.5.1352-1356.2002
145. Lutz JE, Clemons KV, Aristizabal BH, Stevens DA. Activity of the triazole SCH 56592 against disseminated murine coccidioidomycosis. Antimicrob Agents Chemother. (1997) 41:1558–61. doi: 10.1128/AAC.41.7.1558
146. Anstead GM, Corcoran G, Lewis J, Berg D, Graybill JR. Refractory coccidioidomycosis treated with posaconazole. Clin Infect Dis. (2005) 40:1770–6. doi: 10.1086/430303
147. Stevens DA, Rendon A, Gaona-Flores V, Catanzaro A, Anstead GM, Pedicone L, et al. Posaconazole therapy for chronic refractory coccidioidomycosis. Chest. (2007) 132:952–8. doi: 10.1378/chest.07-0114
148. Donnelley MA, Zhu ES, Thompson GR 3rd. Isavuconazole in the treatment of invasive aspergillosis and mucormycosis infections. Infect Drug Resist. (2016) 9:79–86. doi: 10.2147/IDR.S81416
149. Maertens JA, Raad II, Marr KA, Patterson TF, Kontoyiannis DP, Cornely OA, et al. Isavuconazole versus voriconazole for primary treatment of invasive mould disease caused by Aspergillus and other filamentous fungi (SECURE): a phase 3, randomised-controlled, non-inferiority trial. Lancet. (2016) 387:760–9. doi: 10.1016/S0140-6736(15)01159-9
150. Marty FM, Ostrosky-Zeichner L, Cornely OA, Mullane KM, Perfect JR, Thompson GR, et al. Isavuconazole treatment for mucormycosis: a single-arm open-label trial and case-control analysis. Lancet Infect Dis. (2016) 16:828–37. doi: 10.1016/S1473-3099(16)00071-2
151. Thompson GR, Rendon A, Ribeiro Dos Santos R, Queiroz-Telles F, Ostrosky-Zeichner L, Azie N, et al. Isavuconazole treatment of cryptococcosis and dimorphic mycoses. Clin Infect Dis. (2016) 63:356–62. doi: 10.1093/cid/ciw305
152. Wiederhold NP, Kovanda L, Najvar LK, Bocanegra R, Olivo M, Kirkpatrick WR, et al. isavuconazole is effective for the treatment of experimental cryptococcal meningitis. Antimicrob Agents Chemother. (2016) 60:5600–3. doi: 10.1128/AAC.00229-16
153. Heidari A, Quinlan M, Benjamin DJ, Laurence B, Mu A, Ngai T, et al. Isavuconazole in the treatment of coccidioidal meningitis. Antimicrob Agents Chemother. (2019) 63:e02232–18. doi: 10.1128/AAC.02232-18
154. González GM, González G, Najvar LK, Graybill JR. Therapeutic efficacy of caspofungin alone and in combination with amphotericin B deoxycholate for coccidioidomycosis in a mouse model. J Antimicrob Chemother. (2007) 60:1341–6. doi: 10.1093/jac/dkm383
155. Glenn TJ, Blair JE, Adams RH. Coccidioidomycosis in hematopoietic stem cell transplant recipients. Med Mycol. (2005) 43:705–10. doi: 10.1080/13693780500147840
156. Levy ER, McCarty JM, Shane AL, Weintrub PS. Treatment of pediatric refractory coccidioidomycosis with combination voriconazole and caspofungin: a retrospective case series. Clin Infect Dis. (2013) 56:1573–8. doi: 10.1093/cid/cit113
157. McCarty JM, Demetral LC, Dabrowski L, Kahal AK, Bowser AM, Hahn JE. Pediatric coccidioidomycosis in central California: a retrospective case series. Clin Infect Dis. (2013) 56:1579–85. doi: 10.1093/cid/cit114
158. Lindsay J, Sandaradura I, Wong K, Arthur C, Stevenson W, Kerridge I, et al. Serum levels, safety and tolerability of new formulation SUBA-itraconazole prophylaxis in patients with haematological malignancy or undergoing allogeneic stem cell transplantation. J Antimicrob Chemother. (2017) 72:3414–9. doi: 10.1093/jac/dkx295
159. Seyedmousavi S, Rafati H, Ilkit M, Tolooe A, Hedayati MT, Verweij P. Systemic antifungal agents: current status and projected future developments. Methods Mol Biol. (2017) 1508:107–39. doi: 10.1007/978-1-4939-6515-1_5
160. Wiederhold NP, Najvar LK, Jaramillo R, Olivo M, Birch M, Law D, et al. The orotomide olorofim is efficacious in an experimental model of central nervous system coccidioidomycosis. Antimicrob Agents Chemother. (2018) 62:e00999–18. doi: 10.1128/AAC.00999-18
161. Castanheira M, Duncanson FP, Diekema DJ, Guarro J, Jones RN, Pfaller MA. Activities of E1210 and comparator agents tested by CLSI and EUCAST broth microdilution methods against Fusarium and Scedosporium species identified using molecular methods. Antimicrob Agents Chemother. (2012) 56:352–7. doi: 10.1128/AAC.05414-11
162. Miyazaki M, Horii T, Hata K, Watanabe NA, Nakamoto K, Tanaka K, et al. In vitro activity of E1210, a novel antifungal, against clinically important yeasts and molds. Antimicrob Agents Chemother. (2011) 55:4652–8. doi: 10.1128/AAC.00291-11
163. Pfaller MA, Duncanson F, Messer SA, Moet GJ, Jones RN, Castanheira M. In vitro activity of a novel broad-spectrum antifungal, E1210, tested against Aspergillus spp. determined by CLSI and EUCAST broth microdilution methods. Antimicrob Agents Chemother. (2011) 55:5155–8. doi: 10.1128/AAC.00570-11
164. Pfaller MA, Hata K, Jones RN, Messer SA, Moet GJ, Castanheira M. In vitro activity of a novel broad-spectrum antifungal, E1210, tested against Candida spp. as determined by CLSI broth microdilution method. Diagn Microbiol Infect Dis. (2011) 71:167–70. doi: 10.1016/j.diagmicrobio.2011.05.001
165. Pfaller MA, Watanabe N, Castanheira M, Messer SA, Jones RN. Pre-clinical development of antifungal susceptibility test methods for the testing of the novel antifungal agent E1210 versus Candida: comparison of CLSI and European Committee on Antimicrobial Susceptibility Testing methods. J Antimicrob Chemother. (2011) 66:2581–4. doi: 10.1093/jac/dkr342
166. Shaw KJ, Schell WA, Covel J, Duboc G, Giamberardino C, Kapoor M, et al. In vitro and in vivo evaluation of APX001A/APX001 and other Gwt1 inhibitors against cryptococcus. Antimicrob Agents Chemother. (2018) 62:e00523–18. doi: 10.1128/AAC.00523-18
167. Viriyakosol S, Kapoor M, Okamoto S, Covel J, Soltow QA, Trzoss M, et al. APX001 and other Gwt1 inhibitor prodrugs are effective in experimental Coccidioides immitis pneumonia. Antimicrob Agents Chemother. (2019) 63:e01715–18. doi: 10.1128/AAC.01715-18
168. Shubitz LF, Trinh HT, Perrill RH, Thompson CM, Hanan NJ, Galgiani JN, et al. Modeling nikkomycin Z dosing and pharmacology in murine pulmonary coccidioidomycosis preparatory to phase 2 clinical trials. J Infect Dis. (2014) 209:1949–54. doi: 10.1093/infdis/jiu029
169. Hector RF, Zimmer BL, Pappagianis D. Evaluation of nikkomycins X and Z in murine models of coccidioidomycosis, histoplasmosis, and blastomycosis. Antimicrob Agents Chemother. (1990) 34:587–93. doi: 10.1128/AAC.34.4.587
170. Wiederhold NP. Antifungal resistance: current trends and future strategies to combat. Infect Drug Resist. (2017) 10:249–59. doi: 10.2147/IDR.S124918
171. Osherov N, Kontoyiannis DP. The anti-Aspergillus drug pipeline: is the glass half full or empty? Med Mycol. (2017) 55:118–24. doi: 10.1093/mmy/myw060
Keywords: Coccidioides, valley fever, fungal vaccines, antifungal drugs, immunity
Citation: Van Dyke MCC, Thompson GR 3rd, Galgiani JN and Barker BM (2019) The Rise of Coccidioides: Forces Against the Dust Devil Unleashed. Front. Immunol. 10:2188. doi: 10.3389/fimmu.2019.02188
Received: 14 July 2019; Accepted: 30 August 2019;
Published: 11 September 2019.
Edited by:
Agostinho Carvalho, University of Minho, PortugalReviewed by:
Bruce Klein, University of Wisconsin-Madison, United StatesTheo N. Kirkland, University of California, San Diego, United States
Copyright © 2019 Van Dyke, Thompson, Galgiani and Barker. This is an open-access article distributed under the terms of the Creative Commons Attribution License (CC BY). The use, distribution or reproduction in other forums is permitted, provided the original author(s) and the copyright owner(s) are credited and that the original publication in this journal is cited, in accordance with accepted academic practice. No use, distribution or reproduction is permitted which does not comply with these terms.
*Correspondence: Bridget M. Barker, YnJpZGdldC5iYXJrZXJAbmF1LmVkdQ==