- 1Institute of Virology, Technische Universität München, Munich, Germany
- 2Institute for Infectious Diseases and Zoonoses, Ludwig-Maximilians-Universität München, Munich, Germany
- 3Department of Informatics, Technische Universität München, Munich, Germany
C-C motif chemokine ligand 2 (CCL2) is a chemoattractant for leukocytes including monocytes, T cells, and natural killer cells and it plays an important role in maintaining the integrity and function of the brain. However, there is accumulating evidence that many neurological diseases are attributable to a dysregulation of CCL2 expression. Acquired immune deficiency syndrome (AIDS) encephalopathy is a severe and frequent complication in individuals infected with the human immunodeficiency virus (HIV) or the simian immunodeficiency virus (SIV). The HIV and SIV Nef protein, a progression factor in AIDS pathology, can be transferred by microvesicles including exosomes and tunneling nanotubes (TNT) within the host even to uninfected cells, and Nef can induce CCL2 expression. This review focuses on findings which collectively add new insights on how Nef-induced CCL2 expression contributes to neurotropism and neurovirulence of HIV and SIV and elucidates why adjuvant targeting of CCL2 could be a therapeutic option for HIV-infected persons.
Introduction
Acquired immune deficiency syndrome (AIDS), caused by the human immunodeficiency virus (HIV) (1, 2), has to date resulted in the deaths of over 32 million people. According to the 2019 UNAIDS Global AIDS Update, 1.7 million people became newly infected with HIV in 2018 resulting in a total number of 37.9 million people living with HIV worldwide. To date, there is no effective protective vaccine against HIV or even a feasible cure available for HIV-infected patients (3, 4).
In the mid-1990s combined anti-retroviral therapy (ART) was introduced, which considerably reduced the mortality of HIV-infected patients. However, since then, the prevalence of HIV-associated diseases has increased. A major obstacle toward the development of therapies against these diseases that affect a number of organs such as the heart, lungs, kidneys, and the brain is due mainly to the fact that the disease pathogenesis is poorly understood (5–8). In the meantime, there exists at best a consensus that a systemic and persistent activation of the immune system plays a major role in the disease pathogenesis (9–11). Moreover, it is difficult to accurately differentiate between age-related neurodegeneration, other neurodegenerative diseases and HIV-associated neurocognitive disorders (HAND) (12). However, attempts have been made to identify biomarkers to diagnose neurocognitive impairment in HIV-infected persons and activated monocytes/macrophages and C-C motif chemokine ligand 2 (CCL2) appear to be the most promising amongst them (13).
CCL2, also named monocyte chemoattractant protein 1, is a chemotactic cytokine for monocytes (14) and T cells (15), which are the main target cells of HIV-1. CCL2 decreases interferon-alpha expression (16), and promotes HIV/SIV replication by up-regulation of surface C-X-C motif chemokine receptor 4 expression (17).
CCL2 binds to the C-C motif chemokine receptor 2 (CCR2), which is expressed by neurons (18), human fetal astrocytes (19) and brain microvascular endothelial cells (BMECs) (20). CCL2 also binds to the D6 chemokine decoy receptor, which is expressed on adult human astrocytes (21).
The CCL2-CCR2 axis has been shown to play a key role in multiple sclerosis and in experimental autoimmune encephalomyelitis (22), in addition to exacerbating neuronal damage after status epilepticus (23), eliciting itch- and pain-like behavior in allergic contact dermatitis (24), as well as mediating alcohol-induced neuroinflammation and neurotoxicity (25).
HIV and SIV Associated Dementia, Encephalitis and Neuronal Damage
Without combined ART, HIV causes dementia which is characterized by deficiencies in cognition, motor disorders, and behavior abnormalities (26). Pathological manifestations of HIV-associated dementia (HAD) appear as meningitis, encephalitis and vacuolar myelopathy (27). A similar clinical picture has been observed in the SIV/macaque model (28, 29). Even after the introduction of combined ART, HAND remain (8), and, in fact SIV-infected macaques treated with suppressive ART also show ongoing neurodegeneration and inflammation (30). The reason for this phenomenon is unknown although several explanations have been proposed, e.g., that anti-retroviral drugs cannot access the central nervous system (CNS), are not effective in eliminating viral reservoirs, or themselves contribute to HAND (31, 32). However, since specific CNS-targeted ART failed to improve neurocognition in HIV-infected patients compared to non-CNS-targeted (33), it has been hypothesized that early events after primary infection with HIV/SIV are critical for initiating the development of HAND (8).
Indeed, SIV was detected in the brains of macaques within a few days after intravenous infection (34, 35). Further, HIV nucleic acid was detected in the brain of an HIV naïve patient who died 15 days after intravenous inoculation of indium-111-labeled white blood cells, which originated from an HIV-infected individual (36). Additionally, a more recent study showed that HIV RNA is present in the cerebrospinal fluid (CSF) of humans as early as 8 days after HIV infection (37). This suggests that HIV/SIV is capable of exploiting a distinct mechanism to enter the brain rapidly.
Entry of SIV into the brain and induction of neuropathology does not appear to depend on a sustained high viral load because the SIVmac32H(pC8) strain, whose replication is attenuated in vivo (38, 39), was detected in the brain 3 days after infection of macaques where it caused persisting neuroinflammation (40). The attenuated phenotype of SIVmac32H(pC8) is most probably due to a 12 base-pair deletion in its nef gene, which results in an in-frame deletion of the amino acids 143–146 of the translational product (38). Although this Nef variant was detected at lower levels in vitro compared to other variants (41), it was definitely detected in the brain of macaques infected with SIVmac32H(pC8) (42). However, SIV strains containing nucleotide deletions in the nef long-terminal repeat (nef /LTR) overlap region, analogous to the HIV strain of the Sydney blood bank cohort (SBBC), could not be detected in the brains of macaques despite viral replication in the periphery (43). Of note, members of the SBBC who had become infected with an HIV strain containing the nucleotide sequence deletions in the nef /LTR region that results in a truncated Nef protein of 24 amino acids (44), did not or only slowly progressed to AIDS including HAD (45).
The Nef Protein of HIV/SIV: Importance for AIDS Progression and Its Intercellular Transfer
The importance of Nef for AIDS progression was confirmed in SIV-infected rhesus monkeys and HIV-transgenic mice (46, 47). Additionally, it was shown that Nef is required for high viral load in vivo (47). These findings have stimulated a series of studies aiming to identify the mechanistic background with the ultimate goal to exploit the knowledge for therapeutic intervention. Indeed, numerous cellular interaction partners and pathophysiological functions of Nef have been detected (48, 49), and several models of how Nef executes its role in HIV/SIV replication and immunopathogenesis have been proposed (50). In 2009, Kyei et al. showed that HIV Nef inhibits autophagic maturation in human macrophages and thereby provided a convincing explanation of how Nef acts at the molecular level to enable efficient replication of HIV (51). Inhibition of autophagy increases the production of proinflammatory cytokines (52, 53) including CCL2 (54, 55). Thus, Nef also seems to contribute to chronic inflammation, which occurs in HIV-infected persons (56).
HIV Nef was found in supernatants of nef-expressing BHK cells (57), yeast (58), and HEK293 cells (59), which was surprising at the time of these discoveries because nef does not code for an N-terminal signal sequence that would direct the protein to the cell secretory pathway leading to export. Thus, the mechanism by which Nef is released from infected cells was regarded as an open question. In the past, on analyzing the supernatants of BHK cells infected with recombinant vaccinia virus expressing HIV Nef, it was assumed that Nef could be released by vesicles (57). Today, it is recognized that not only proteins but also lipids and RNA can be released from a cell by extracellular vesicles (60).
In 2003, it was shown that HIV Nef induces an accumulation of multivesicular bodies (MVBs) and that Nef itself is present in MVBs (61). MVBs can fuse with the cell plasma membrane, leading to the release of 40–90 nm diameter vesicles, termed exosomes, into the extracellular environment (62). Consequently, it was tempting to speculate that Nef could be released from cells by exosomes. However, it was challenging to test this hypothesis in HIV-infected cells because Nef is incorporated in virions (63, 64).
The astrocytoma cell line TH4-7-5 is persistently infected with the HIV isolate TH4-7-5 which has a mutation in the nef gene (GenBank accession number: L31963.1), resulting in a myristoylation-deficient Nef (65). However, myristoylation of Nef is required for optimal HIV replication in vitro (66). Thus, a myristoylation-deficient Nef and a block in HIV Rev function most probably effected a very low production of infectious virus but a high production of Nef in astrocytoma TH4-7-5 cells (65, 67). We took advantage of the astrocytoma cell line TH4-7-5 and examined whether Nef is present in the supernatants of these cells. Application of a two-step centrifugation protocol, previously shown to enable the enrichment of microvesicles including exosomes from cellular supernatants (68), resulted in the detection of Nef in the pellets of centrifuged supernatants of these cells (69).
It was later confirmed that Nef is released from HIV-infected cells (70, 71) by microvesicles and it was even claimed that this occurs via exosomes (72). However, there is still an ongoing debate regarding the type of vesicle by which Nef leaves the cell (73, 74). Further, Nef was detected in microvesicles and exosomes isolated from the plasma of HIV-infected persons despite them receiving ART, and it has been shown that exosomes derived from HAD patients can transfer nef mRNA to cells, leading to Nef expression and subsequent induction of cellular genes (75, 76).
Nef was also found in uninfected human peripheral blood mononuclear cells (PBMCs), which can transfer Nef to human umbilical cord vein endothelial cells (77). A recent study not only reported that Nef is released by vesicles from HIV-infected cells, but also confirmed the result for SIV-infected cells and has additionally shown that extracellular vesicles containing Nef circulate in the blood of SIV-infected macaques (78). Meanwhile, the process of protein and mRNA transfer by exosomes and other extracellular vesicles even between different types of cells is well understood (79). In summary, irrespective of the type of extracellular vesicle from which Nef is released by HIV/SIV infected cells, Nef is present in the extracellular environment independently of virions and can enter uninfected cells where it affects cellular functions and gene expression.
Additionally, cells can exchange molecules and organelles directly via tunneling nanotubes (TNTs), which are about 50–200 nm long thin actin rich membrane conduits, even between different types of cells (80). Nef can induce TNT formation (81, 82), and it can also be transferred to B cells via TNTs from HIV-infected macrophages (83), from macrophages to T cells (82), from nef-expressing T cells to hepatocytic cells (84) and also between macrophages (81). Importantly, Nef is transferred from T cells and monocytes to human coronary arterial endothelial cells via TNTs, leading to apoptosis and CCL2 expression (85).
Nef-induced CCL2 Expression and the Function of the Blood-Brain-Barrier
CCL2 increases the blood-brain barrier (BBB) permeability (86, 87), and much progress has been made in revealing the molecular mechanism of how leukocytes, governed by CCL2, pass the BBB (88). Therein, astrocyte and BMEC-derived CCL2 play complementary roles (89).
A natural repair mechanism to restore damaged brain tissue after experimentally-induced ischemia starts with the recruitment of CCR2+Iba1+ monocytes from the periphery, which then differentiate into brain Iba1+NG2+ cells within the brain parenchyma (90, 91). Transmigration of CCR2+Iba1+ monocytes through the BBB is enabled by a transient expression of CCL2 in astrocytes and endothelial cells that lasts for only 2 days (92). Indeed, under normal physiological conditions, the BBB is impermeable for circulating monocytes (93, 94), and therefore invasion of a healthy brain by HIV/SIV should not happen as fast as it has been observed. But a specific HIV/SIV-triggered mechanism leading to CCL2 expression in BMECs may enable HIV/SIV to get access to the brain either in the form of free virions or via infected CCR2+ cells. In this respect, it was significant to observe that Nef (i) can be transferred from human PBMC to human endothelial cells (77), (ii) was detected in endothelial cells of nef-transgenic mice and macaques infected with SHIV-nefSF33, and (iii) induces CCL2 expression in endothelial cells (85).
Invasion of the brain by leukocytes would additionally require an upregulation of adhesion molecules on both endothelial and infected cells. Indeed, it has been shown that HIV Nef upregulates the intercellular adhesion molecule 1 (ICAM-1) in vascular endothelial cells (95). ICAM-1 interacts with the lymphocyte function-associated antigen 1 (LFA-1), and its subunits, CD11a and CD18, are upregulated in HIV-infected monocytes (96, 97). Endothelial-derived CCL2 activates CD11a, leading to a firm arrest of monocytes on endothelial cells (98, 99), and mediates the subsequent transendothelial migration (100).
In summary, the findings collectively result in a model in which Nef-containing PBMCs and extracellular vesicles carrying Nef attach to and transfer Nef into endothelial cells, leading to CCL2 production that can cause BBB leakiness and subsequent entry of HIV/SIV by infected cells into the brain (Figure 1). Of note, this provides a simple explanation of why SIV with a deleted nef gene cannot enter the brain (43).
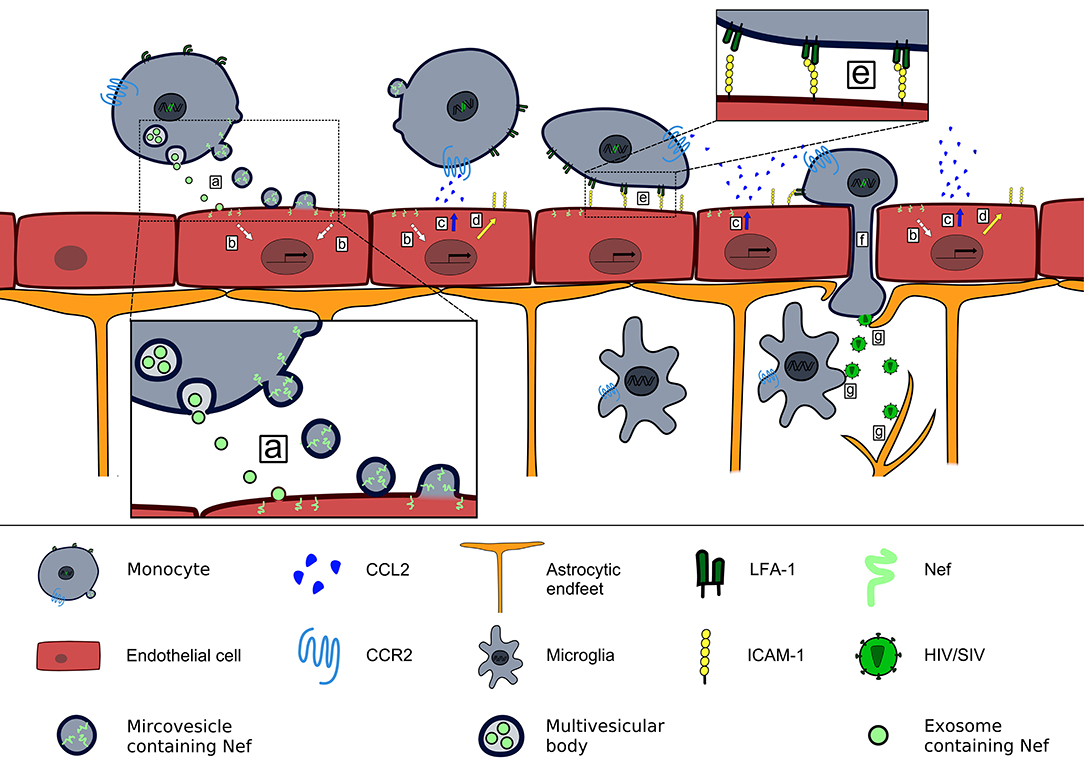
Figure 1. Contribution of Nef-induced CCL2 expression to HIV/SIV neurotropism. HIV/SIV infected monocytes release microvesicles and exosomes that transfer Nef into brain endothelial cells (a), where Nef induces a signaling pathway (b) that leads to release of CCL2 at the luminal side of the BBB (c) and upregulation of ICAM-1 (d). CCL2 binding to CCR2+cells triggers a conformational change of LFA-1 that enables their firm adhesion to brain endothelial cells via LFA-1–ICAM-1 interaction (e). Endothelial CCL2 expression enables transendothelial migration of HIV/SIV-infected CCR2+monocytes into the brain parenchyma (101) (f). There HIV/SIV infects astrocytes and microglia (g).
Nef-induced CCL2 Expression and Neuronal Dysfunctions
Once in the brain, HIV/SIV cannot be eliminated by ART. The virus persists and triggers a chronic inflammation leading to sustained leukocyte infiltration, astrogliosis and neuronal degeneration (102, 103). In brain tissues of HIV-infected patients, HIV DNA was detected in the cells of the macrophage lineage and in astrocytes, the most abundant cell type in the brain. However, it was not found in neurons (104), which is in accordance with the finding that perivascular macrophages and microglia, but not neurons, can be productively infected with HIV/SIV (105, 106). These findings indicate that an indirect mechanism causes neuronal dysfunction and damage, and microglia that release exosomes and microvesicles containing Nef (107) may play an important role therein. It has long been known that HIV and SIV antigens are present in astrocytes of primary infected tissues (106, 108). Recently, a hypothesis was proposed that explains this finding (109) and challenges the consensus that HIV/SIV can infect astrocytes (110).
Significantly, Nef is highly expressed in astrocytes (111), promotes replication of HIV (112), and is also released by exosomes (113) or any other extracellular vesicle (114). Human astrocytes infected with recombinant Sindbis virus vector encoding HIV nef produced elevated CCL2 mRNA levels, which was independent of the nef variant tested (115). Induction of CCL2 expression by HIV Nef was confirmed in U-251 MG astroglioma cells transfected stably with nef (116), in primary rat astrocytes in vivo (117), and in primary murine macrophages and microglia (118). Animal models have provided evidence that there is a direct link between Nef-induced CCL2 expression and neuronal dysfunction and damage. Macrophages expressing HIV Nef, which were implanted into the rat hippocampus, triggered immigration of monocytes/macrophages, tumor necrosis factor expression, and astrogliosis, a hallmark of HIV encephalitis (HIVE). In addition, the neurotoxicity triggered by Nef was associated with cognitive deficits (119). Cognitive deficits in particular spatial and recognition memory were observed in rat brains in which primary astrocytes were implanted that expressed HIV Nef. This was associated with Nef-induced CCL2 expression, which resulted in immigration of macrophages in the hippocampus and loss of hippocampal CA3 neurons in these animals (117). In transgenic mice, in which HIV Nef was expressed specifically in macrophages and microglia, CCL2 was increased in the brain, and the dopamine system was affected, leading to mania-like behavior, especially in males (118).
There are several studies demonstrating that increased CCL2 concentrations correlate with HAD/HAND. Elevated levels of CCL2 were detected in the CSF of HIV-infected individuals positively diagnosed with HAD (120, 121). Microglia and astrocytes of HIV-infected persons suffering from HIVE produce CCL2 (122), which was confirmed for SIV infected macaques (123). Additionally, a specific small nucleotide polymorphism in the CCL2 promoter, which leads to increased CCL2 expression and infiltration of mononuclear phagocytes into tissues correlates positively with the risk of HAD (124). Cocaine, known to exacerbate neurodegeneration in persons infected with HIV, induces CCL2 expression in microglia and leads to increased transmigration of monocytes into the brain (125).
It is now also known that CCL2 affects neurons directly in addition to enhancing the transmigration of infected leukocytes through the BBB (126). For example, over-induction of CCL2 in astrocytes causes dopaminergic neurodegeneration in 1-methyl-4-phenyl-1,2,3,6-tetrahydropyridine mice (127), and an inhibition of CCL2 expression protects neurons against amyloid-beta-induced toxicity (128). Indeed, CCL2 mediates cell death in neurons of the hippocampal CA3 region after kainic acid-induced seizures in mice. Neuronal degeneration was associated with behavioral impairment, memory decline, and anxiety (129), all characteristics which have been observed early after infection of humans with HIV and even in HIV-infected persons receiving ART (130–132).
CCR2, the receptor of CCL2, is present on neurons (18), and its absence reduced brain damage as well as BBB permeability in an experimental stroke model in mice (133). Similar to the process in an HIV/SIV infection, CCR2 plays a key role in the accumulation of myeloid cells in the brain and the activation of hippocampal myeloid cells upon infection with Theiler's murine encephalitis virus (TMV). Notably, CCR2 deficient mice had almost no hippocampal damage during TMV infection (134). Thus, CCL2 represents a convincing candidate to explain neuronal dysfunction and damage (135) which occur in HIV/SIV infected humans and animals (Figure 2). Additionally, CCL2 is major mediator of pain (137), and chronic pain is a common burden in people living with HIV/AIDS (138).
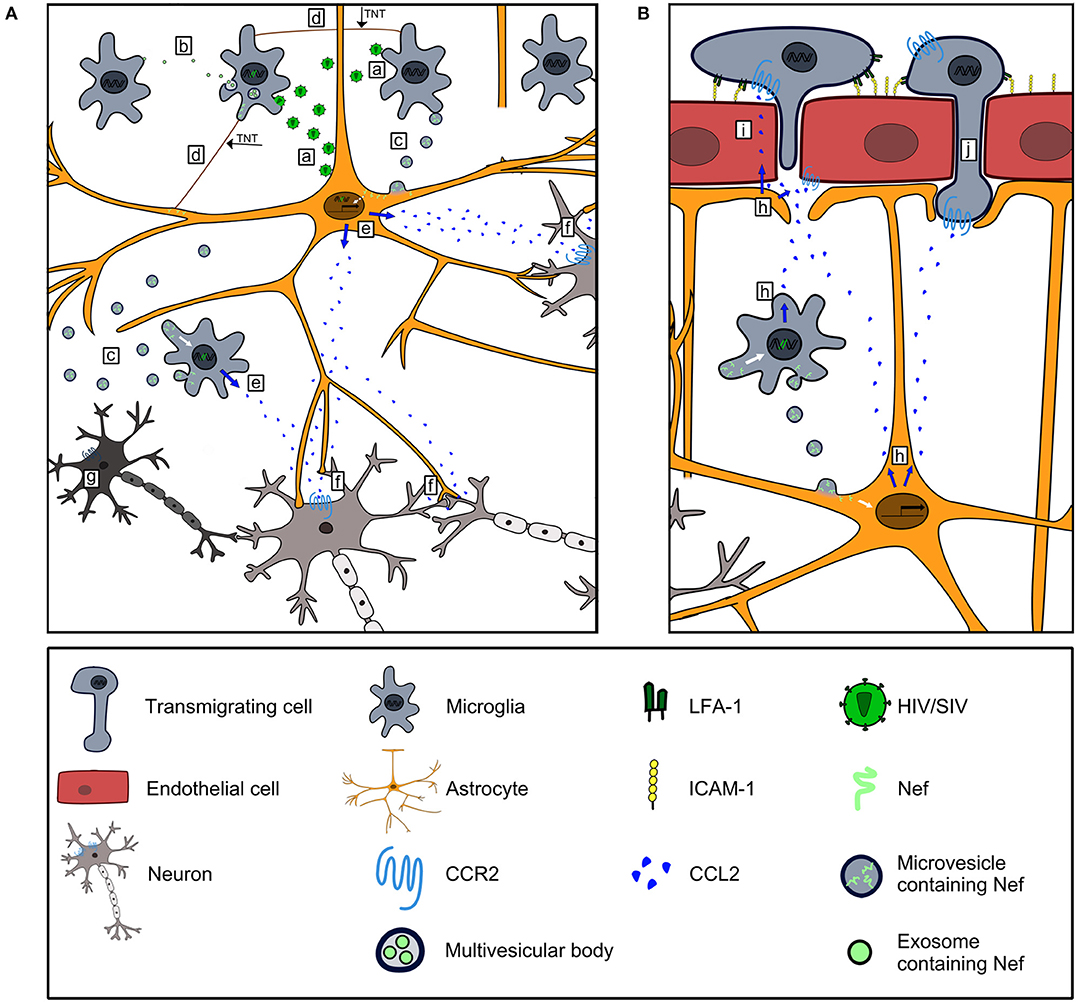
Figure 2. Contribution of Nef-induced CCL2 expression to HIV/SIV neurovirulence. (A) HIV/SIV-infected microglia and astrocytes infect uninfected microglia and astrocytes (a), and disseminate Nef via exosomes (b), microvesicles (c) and TNTs (d) to uninfected cells. Nef harboring astrocytes and microglia express CCL2 (e). CCL2 stimulates CCR2 signaling in neurons leading to their dysfunction (f) and death (g). (B) CCL2 produced by Nef harboring astrocytes and microglia (h) is transported transcellularly across BMEC (136) to act on CCR2+cells along the luminal side of the BBB (i). CCL2 binds to CCR2 on BMEC and mediates disruption of endothelial junctions (86, 87) to foster invasion of CCR2+cells into the brain (j).
Summary
The findings summarized herein not only integrate well into the “Trojan horse” model that states that a cell infected with HIV/SIV enters the brain leading to a persistent infection and consequently HAND (139) but also add to this model the fact that the transfer of Nef by microvesicles into endothelial cells and the subsequent induction of CCL2, mimics a pathophysiological state of the brain to which monocytes are recruited normally. Nef, in combination with other HIV/SIV proteins and even anti-retroviral drugs, possibly work together more efficiently to enable a rapid entry of HIV/SIV-infected cells into the brain (140). This interplay presumably plays a general role in HIV-associated diseases (141).
In the brain, HIV/SIV-infected cells such as astrocytes and microglia distribute Nef to uninfected cells via microvesicles and TNTs. Thereby, there is a steady increase in the number of Nef-bearing, non-infected cells which produce CCL2. HIV Tat in astrocytes seems to contribute to an increase in the levels of CCL2 in the brain (142, 143). The persistent non-physiological expression of CCL2 leads to sustained cell infiltration into the brain and a disturbance of neuronal functions. If a person is infected with HIV subtype B then Tat could enhance CCR2 activation through its acidic region (144, 145). Moreover, when present in sufficiently high concentrations in the brain, Tat could definitely exacerbate neuronal dysfunctions through its basic region (146). Moreover, besides CCL2, the C-X-C motif chemokine 10 (CXCL10) has also been identified as a biomarker for HAND (13), especially in HIV-infected women (147) and this chemokine can also be induced by Nef (115).
Conclusion
The findings summarized here classify HIV/SIV Nef-induced CCL2 expression in the complex pathogenesis of HAND, and once again highlight the special role which the CCL2-CCR2 axis can play in a neurological disease. Consequently, drugs which have been developed to target this chemokine or its receptor could also be an option for an adjuvant therapy in HIV-infected persons.
Author Contributions
ML wrote the initial draft. ML and VE discussed the manuscript. JL, ML, and VE edited the manuscript. JL and ML designed and drew the illustrations.
Funding
This work was supported by the German Research Foundation (DFG) and the Technical University of Munich (TUM) in the framework of the Open Access Publishing Program.
Conflict of Interest
The authors declare that the research was conducted in the absence of any commercial or financial relationships that could be construed as a potential conflict of interest.
Acknowledgments
The authors would like to thank Jacqueline Weber-Lehmann for critical reading of the manuscript.
References
1. Barre-Sinoussi F, Chermann JC, Rey F, Nugeyre MT, Chamaret S, Gruest J, et al. Isolation of a T-lymphotropic retrovirus from a patient at risk for acquired immune deficiency syndrome (AIDS). Science. (1983) 220:868–71. doi: 10.1126/science.6189183
2. Gallo RC, Salahuddin SZ, Popovic M, Shearer GM, Kaplan M, Haynes BF, et al. Frequent detection and isolation of cytopathic retroviruses (HTLV-III) from patients with AIDS and at risk for AIDS. Science. (1984) 224:500–3. doi: 10.1126/science.6200936
3. Kent SJ, Davenport MP. Moving the HIV vaccine field forward: concepts of protective immunity. Lancet HIV. (2019) 6:e406–10. doi: 10.1016/S2352-3018(19)30134-1
4. Lopez-Galindez C, Pernas M, Casado C, Olivares I, Lorenzo-Redondo R. Elite controllers and lessons learned for HIV-1 cure. Curr Opin Virol. (2019) 38:31–6. doi: 10.1016/j.coviro.2019.05.010
5. Fitzpatrick ME, Kunisaki KM, Morris A. Pulmonary disease in HIV-infected adults in the era of antiretroviral therapy. AIDS. (2018) 32:277–92. doi: 10.1097/QAD.0000000000001712
6. Naicker S, Rahmanian S, Kopp JB. HIV and chronic kidney disease. Clin Nephrol. (2015) 83(suppl. 1):32–8. doi: 10.5414/CNP83S032
7. Pinto DSM, da Silva M. Cardiovascular disease in the setting of human immunodeficiency virus infection. Curr Cardiol Rev. (2018) 14:25–41. doi: 10.2174/1573403X13666171129170046
8. Saylor D, Dickens AM, Sacktor N, Haughey N, Slusher B, Pletnikov M, et al. HIV-associated neurocognitive disorder–pathogenesis and prospects for treatment. Nat Rev Neurol. (2016) 12:234–48. doi: 10.1038/nrneurol.2016.27
9. Paiardini M, Muller-Trutwin M. HIV-associated chronic immune activation. Immunol Rev. (2013) 254:78–101. doi: 10.1111/imr.12079
10. Plaeger SF, Collins BS, Musib R, Deeks SG, Read S, Embry A. Immune activation in the pathogenesis of treated chronic HIV disease: a workshop summary. AIDS Res Hum Retroviruses. (2012) 28:469–77. doi: 10.1089/aid.2011.0213
11. Younas M, Psomas C, Reynes J, Corbeau P. Immune activation in the course of HIV-1 infection: causes, phenotypes and persistence under therapy. HIV Med. (2016) 17:89–105. doi: 10.1111/hiv.12310
12. Rosenthal J, Tyor W. Aging, comorbidities, and the importance of finding biomarkers for HIV-associated neurocognitive disorders. J Neurovirol. (2019). doi: 10.1007/s13365-019-00735-0. [Epub ahead of print].
13. Bandera A, Taramasso L, Bozzi G, Muscatello A, Robinson JA, Burdo TH, et al. HIV-associated neurocognitive impairment in the modern ART era: are we close to discovering reliable biomarkers in the setting of virological suppression? Front Aging Neurosci. (2019) 11:187. doi: 10.3389/fnagi.2019.00187
14. Robinson EA, Yoshimura T, Leonard EJ, Tanaka S, Griffin PR, Shabanowitz J, et al. Complete amino acid sequence of a human monocyte chemoattractant, a putative mediator of cellular immune reactions. Proc Natl Acad Sci USA. (1989) 86:1850–4. doi: 10.1073/pnas.86.6.1850
15. Loetscher P, Seitz M, Clark-Lewis I, Baggiolini M, Moser B. Monocyte chemotactic proteins MCP-1, MCP-2, and MCP-3 are major attractants for human CD4+ and CD8+ T lymphocytes. FASEB J. (1994) 8:1055–60. doi: 10.1096/fasebj.8.13.7926371
16. Williams DW, Askew LC, Jones E, Clements JE. CCR2 signaling selectively regulates IFN-alpha: role of beta-arrestin 2 in IFNAR1 internalization. J Immunol. (2019) 202:105–18. doi: 10.4049/jimmunol.1800598
17. Campbell GR, Spector SA. CCL2 increases X4-tropic HIV-1 entry into resting CD4+ T cells. J Biol Chem. (2008) 283:30745–53. doi: 10.1074/jbc.M804112200
18. Coughlan CM, McManus CM, Sharron M, Gao Z, Murphy D, Jaffer S, et al. Expression of multiple functional chemokine receptors and monocyte chemoattractant protein-1 in human neurons. Neuroscience. (2000) 97:591–600. doi: 10.1016/S0306-4522(00)00024-5
19. Andjelkovic AV, Song L, Dzenko KA, Cong H, Pachter JS. Functional expression of CCR2 by human fetal astrocytes. J Neurosci Res. (2002) 70:219–31. doi: 10.1002/jnr.10372
20. Dzenko KA, Andjelkovic AV, Kuziel WA, Pachter JS. The chemokine receptor CCR2 mediates the binding and internalization of monocyte chemoattractant protein-1 along brain microvessels. J Neurosci. (2001) 21:9214–23. doi: 10.1523/JNEUROSCI.21-23-09214.2001
21. Fouillet A, Mawson J, Suliman O, Sharrack B, Romero IA, Woodroofe MN. CCL2 binding is CCR2 independent in primary adult human astrocytes. Brain Res. (2012) 1437:115–26. doi: 10.1016/j.brainres.2011.11.049
22. Mahad DJ, Ransohoff RM. The role of MCP-1 (CCL2) and CCR2 in multiple sclerosis and experimental autoimmune encephalomyelitis (EAE). Semin Immunol. (2003) 15:23–32. doi: 10.1016/S1044-5323(02)00125-2
23. Varvel NH, Neher JJ, Bosch A, Wang W, Ransohoff RM, Miller RJ, et al. Infiltrating monocytes promote brain inflammation and exacerbate neuronal damage after status epilepticus. Proc Natl Acad Sci USA. (2016) 113:E5665–74. doi: 10.1073/pnas.1604263113
24. Jiang H, Cui H, Wang T, Shimada SG, Sun R, Tan Z, et al. CCL2/CCR2 signaling elicits itch- and pain-like behavior in a murine model of allergic contact dermatitis. Brain Behav Immun. (2019) 80:464–73. doi: 10.1016/j.bbi.2019.04.026
25. Zhang K, Luo J. Role of MCP-1 and CCR2 in alcohol neurotoxicity. Pharmacol Res. (2019) 139:360–6. doi: 10.1016/j.phrs.2018.11.030
26. Price RW, Brew B, Sidtis J, Rosenblum M, Scheck AC, Cleary P. The brain in AIDS: central nervous system HIV-1 infection and AIDS dementia complex. Science. (1988) 239:586–92. doi: 10.1126/science.3277272
27. Petito CK. Review of central nervous system pathology in human immunodeficiency virus infection. Ann Neurol. (1988) 23:S54–7. doi: 10.1002/ana.410230715
28. Rausch DM, Murray EA, Eiden LE. The SIV-infected rhesus monkey model for HIV-associated dementia and implications for neurological diseases. J Leukoc Biol. (1999) 65:466–74. doi: 10.1002/jlb.65.4.466
29. Zink MC, Spelman JP, Robinson RB, Clements JE. SIV infection of macaques–modeling the progression to AIDS dementia. J Neurovirol. (1998) 4:249–59. doi: 10.3109/13550289809114526
30. Beck SE, Queen SE, Metcalf Pate KA, Mangus LM, Abreu CM, Gama L, et al. An SIV/macaque model targeted to study HIV-associated neurocognitive disorders. J Neurovirol. (2018) 24:204–12. doi: 10.1007/s13365-017-0582-4
31. Nabha L, Duong L, Timpone J. HIV-associated neurocognitive disorders: perspective on management strategies. Drugs. (2013) 73:893–905. doi: 10.1007/s40265-013-0059-6
32. Fois AF, Brew BJ. The potential of the CNS as a reservoir for HIV-1 infection: implications for HIV eradication. Curr HIV AIDS Rep. (2015) 12:299–303. doi: 10.1007/s11904-015-0257-9
33. Ellis RJ, Letendre S, Vaida F, Haubrich R, Heaton RK, Sacktor N, et al. Randomized trial of central nervous system-targeted antiretrovirals for HIV-associated neurocognitive disorder. Clin Infect Dis. (2014) 58:1015–22. doi: 10.1093/cid/cit921
34. Chakrabarti L, Hurtrel M, Maire MA, Vazeux R, Dormont D, Montagnier L, et al. Early viral replication in the brain of SIV-infected rhesus monkeys. Am J Pathol. (1991) 139:1273–80.
35. Matsuda K, Dang Q, Brown CR, Keele BF, Wu F, Ourmanov I, et al. Characterization of simian immunodeficiency virus (SIV) that induces SIV encephalitis in rhesus macaques with high frequency: role of TRIM5 and major histocompatibility complex genotypes and early entry to the brain. J Virol. (2014) 88:13201–11. doi: 10.1128/JVI.01996-14
36. Davis LE, Hjelle BL, Miller VE, Palmer DL, Llewellyn AL, Merlin TL, et al. Early viral brain invasion in iatrogenic human immunodeficiency virus infection. Neurology. (1992) 42:1736–9. doi: 10.1212/WNL.42.9.1736
37. Valcour V, Chalermchai T, Sailasuta N, Marovich M, Lerdlum S, Suttichom D, et al. Central nervous system viral invasion and inflammation during acute HIV infection. J Infect Dis. (2012) 206:275–82. doi: 10.1093/infdis/jis326
38. Rud EW, Cranage M, Yon J, Quirk J, Ogilvie L, Cook N, et al. Molecular and biological characterization of simian immunodeficiency virus macaque strain 32H proviral clones containing nef size variants. J Gen Virol. (1994) 75:529–43. doi: 10.1099/0022-1317-75-3-529
39. Stahl-Hennig C, Dittmer U, Nisslein T, Petry H, Jurkiewicz E, Fuchs D, et al. Rapid development of vaccine protection in macaques by live-attenuated simian immunodeficiency virus. J Gen Virol. (1996) 77:2969–81. doi: 10.1099/0022-1317-77-12-2969
40. Ferguson D, Clarke S, Berry N, Almond N. Attenuated SIV causes persisting neuroinflammation in the absence of a chronic viral load and neurotoxic antiretroviral therapy. AIDS. (2016) 30:2439–48. doi: 10.1097/QAD.0000000000001178
41. Carl S, Iafrate AJ, Skowronski J, Stahl-Hennig C, Kirchhoff F. Effect of the attenuating deletion and of sequence alterations evolving in vivo on simian immunodeficiency virus C8-Nef function. J Virol. (1999) 73:2790–7.
42. Clarke S, Berry N, Ham C, Alden J, Almond N, Ferguson D. Neuropathology of wild-type and nef-attenuated T cell tropic simian immunodeficiency virus (SIVmac32H) and macrophage tropic neurovirulent SIVmac17E-Fr in cynomolgus macaques. J Neurovirol. (2012) 18:100–12. doi: 10.1007/s13365-012-0084-3
43. Thompson KA, Kent SJ, Gahan ME, Purcell DF, McLean CA, Preiss S, et al. Decreased neurotropism of nef long terminal repeat (nef/LTR)-deleted simian immunodeficiency virus. J Neurovirol. (2003) 9:442–51. doi: 10.1080/13550280390218715
44. Deacon NJ, Tsykin A, Solomon A, Smith K, Ludford-Menting M, Hooker DJ, et al. Genomic structure of an attenuated quasi species of HIV-1 from a blood transfusion donor and recipients. Science. (1995) 270:988–91. doi: 10.1126/science.270.5238.988
45. Gorry PR, Churchill M, Learmont J, Cherry C, Dyer WB, Wesselingh SL, et al. Replication-dependent pathogenicity of attenuated nef-deleted HIV-1 in vivo. J Acquir Immune Defic Syndr. (2007) 46:390–4. doi: 10.1097/QAI.0b013e31815aba08
46. Hanna Z, Kay DG, Rebai N, Guimond A, Jothy S, Jolicoeur P. Nef harbors a major determinant of pathogenicity for an AIDS-like disease induced by HIV-1 in transgenic mice. Cell. (1998) 95:163–75. doi: 10.1016/S0092-8674(00)81748-1
47. Kestler HW III, Ringler DJ, Mori K, Panicali DL, Sehgal PK, Daniel MD, et al. Importance of the nef gene for maintenance of high virus loads and for development of AIDS. Cell. (1991) 65:651–62. doi: 10.1016/0092-8674(91)90097-I
48. Fackler OT, Baur AS. Live and let die: Nef functions beyond HIV replication. Immunity. (2002) 16:493–7. doi: 10.1016/S1074-7613(02)00307-2
49. Quaranta MG, Mattioli B, Giordani L, Viora M. The immunoregulatory effects of HIV-1 Nef on dendritic cells and the pathogenesis of AIDS. FASEB J. (2006) 20:2198–208. doi: 10.1096/fj.06-6260rev
50. Kirchhoff F, Schindler M, Specht A, Arhel N, Munch J. Role of Nef in primate lentiviral immunopathogenesis. Cell Mol Life Sci. (2008) 65:2621–36. doi: 10.1007/s00018-008-8094-2
51. Kyei GB, Dinkins C, Davis AS, Roberts E, Singh SB, Dong C, et al. Autophagy pathway intersects with HIV-1 biosynthesis and regulates viral yields in macrophages. J Cell Biol. (2009) 186:255–68. doi: 10.1083/jcb.200903070
52. Nakahira K, Haspel JA, Rathinam VA, Lee SJ, Dolinay T, Lam HC, et al. Autophagy proteins regulate innate immune responses by inhibiting the release of mitochondrial DNA mediated by the NALP3 inflammasome. Nat Immunol. (2011) 12:222–30. doi: 10.1038/ni.1980
53. Saitoh T, Fujita N, Jang MH, Uematsu S, Yang BG, Satoh T, et al. Loss of the autophagy protein Atg16L1 enhances endotoxin-induced IL-1beta production. Nature. (2008) 456:264–8. doi: 10.1038/nature07383
54. Ghosh AK, Mau T, O'Brien M, Garg S, Yung R. Impaired autophagy activity is linked to elevated ER-stress and inflammation in aging adipose tissue. Aging. (2016) 8:2525–37. doi: 10.18632/aging.101083
55. Xu W, Wei Q, Han M, Zhou B, Wang H, Zhang J, et al. CCL2-SQSTM1 positive feedback loop suppresses autophagy to promote chemoresistance in gastric cancer. Int J Biol Sci. (2018) 14:1054–66. doi: 10.7150/ijbs.25349
56. Appay V, Sauce D. Immune activation and inflammation in HIV-1 infection: causes and consequences. J Pathol. (2008) 214:231–41. doi: 10.1002/path.2276
57. Guy B, Riviere Y, Dott K, Regnault A, Kieny MP. Mutational analysis of the HIV nef protein. Virology. (1990) 176:413–25. doi: 10.1016/0042-6822(90)90011-F
58. Macreadie IG, Castelli LA, Lucantoni A, Azad AA. Stress- and sequence-dependent release into the culture medium of HIV-1 Nef produced in Saccharomyces cerevisiae. Gene. (1995) 162:239–43. doi: 10.1016/0378-1119(95)00316-X
59. James CO, Huang MB, Khan M, Garcia-Barrio M, Powell MD, Bond VC. Extracellular Nef protein targets CD4+ T cells for apoptosis by interacting with CXCR4 surface receptors. J Virol. (2004) 78:3099–109. doi: 10.1128/JVI.78.6.3099-3109.2004
60. Robbins PD, Morelli AE. Regulation of immune responses by extracellular vesicles. Nat Rev Immunol. (2014) 14:195–208. doi: 10.1038/nri3622
61. Stumptner-Cuvelette P, Jouve M, Helft J, Dugast M, Glouzman AS, Jooss K, et al. Human immunodeficiency virus-1 Nef expression induces intracellular accumulation of multivesicular bodies and major histocompatibility complex class II complexes: potential role of phosphatidylinositol 3-kinase. Mol Biol Cell. (2003) 14:4857–70. doi: 10.1091/mbc.e03-04-0211
62. Denzer K, Kleijmeer MJ, Heijnen HF, Stoorvogel W, Geuze HJ. Exosome: from internal vesicle of the multivesicular body to intercellular signaling device. J Cell Sci. (2000) 113:3365–74.
63. Pandori MW, Fitch NJ, Craig HM, Richman DD, Spina CA, Guatelli JC. Producer-cell modification of human immunodeficiency virus type 1: Nef is a virion protein. J Virol. (1996) 70:4283–90.
64. Welker R, Kottler H, Kalbitzer HR, Krausslich HG. Human immunodeficiency virus type 1 Nef protein is incorporated into virus particles and specifically cleaved by the viral proteinase. Virology. (1996) 219:228–36. doi: 10.1006/viro.1996.0240
65. Brack-Werner R, Kleinschmidt A, Ludvigsen A, Mellert W, Neumann M, Herrmann R, et al. Infection of human brain cells by HIV-1: restricted virus production in chronically infected human glial cell lines. AIDS. (1992) 6:273–85. doi: 10.1097/00002030-199203000-00004
66. Chowers MY, Spina CA, Kwoh TJ, Fitch NJ, Richman DD, Guatelli JC. Optimal infectivity in vitro of human immunodeficiency virus type 1 requires an intact nef gene. J Virol. (1994) 68:2906–14.
67. Neumann M, Felber BK, Kleinschmidt A, Froese B, Erfle V, Pavlakis GN, et al. Restriction of human immunodeficiency virus type 1 production in a human astrocytoma cell line is associated with a cellular block in Rev function. J Virol. (1995) 69:2159–67.
68. Raposo G, Nijman HW, Stoorvogel W, Liejendekker R, Harding CV, Melief CJ, et al. B lymphocytes secrete antigen-presenting vesicles. J Exp Med. (1996) 183:1161–72. doi: 10.1084/jem.183.3.1161
69. Lehmann MH, Walter S, Ylisastigui L, Striebel F, Kohleisen B, Ovod V, et al. Exogenous HIV-1 Nef protein increases migration of monocytes. In: XXII Symposium of the International Association for Comparative Research on Leukemia and Related Diseases. Heidelberg (2005).
70. Campbell TD, Khan M, Huang MB, Bond VC, Powell MD. HIV-1 Nef protein is secreted into vesicles that can fuse with target cells and virions. Ethn Dis. (2008) 18(suppl. 2):S2–14–9.
71. Muratori C, Cavallin LE, Kratzel K, Tinari A, De Milito A, Fais S, et al. Massive secretion by T cells is caused by HIV Nef in infected cells and by Nef transfer to bystander cells. Cell Host Microbe. (2009) 6:218–30. doi: 10.1016/j.chom.2009.06.009
72. Lenassi M, Cagney G, Liao M, Vaupotic T, Bartholomeeusen K, Cheng Y, et al. HIV Nef is secreted in exosomes and triggers apoptosis in bystander CD4+ T cells. Traffic. (2010) 11:110–22. doi: 10.1111/j.1600-0854.2009.01006.x
73. Luo X, Fan Y, Park IW, He JJ. Exosomes are unlikely involved in intercellular Nef transfer. PLoS ONE. (2015) 10:e0124436. doi: 10.1371/journal.pone.0124436
74. Ellwanger JH, Veit TD, Chies JAB. Exosomes in HIV infection: a review and critical look. Infect Genet Evol. (2017) 53:146–54. doi: 10.1016/j.meegid.2017.05.021
75. Khan MB, Lang MJ, Huang MB, Raymond A, Bond VC, Shiramizu B, et al. Nef exosomes isolated from the plasma of individuals with HIV-associated dementia (HAD) can induce Abeta(1–42) secretion in SH-SY5Y neural cells. J Neurovirol. (2016) 22:179–90. doi: 10.1007/s13365-015-0383-6
76. Raymond AD, Campbell-Sims TC, Khan M, Lang M, Huang MB, Bond VC, et al. HIV Type 1 Nef is released from infected cells in CD45(+) microvesicles and is present in the plasma of HIV-infected individuals. AIDS Res Hum Retroviruses. (2011) 27:167–78. doi: 10.1089/aid.2009.0170
77. Wang T, Green LA, Gupta SK, Amet T, Byrd DJ, Yu Q, et al. Intracellular Nef detected in peripheral blood mononuclear cells from HIV patients. AIDS Res Hum Retroviruses. (2015) 31:217–20. doi: 10.1089/aid.2013.0250
78. McNamara RP, Costantini LM, Myers TA, Schouest B, Maness NJ, Griffith JD, et al. Nef secretion into extracellular vesicles or exosomes is conserved across human and simian immunodeficiency viruses. MBio. (2018) 9:e02344–17. doi: 10.1128/mBio.02344-17
79. Mathieu M, Martin-Jaular L, Lavieu G, Thery C. Specificities of secretion and uptake of exosomes and other extracellular vesicles for cell-to-cell communication. Nat Cell Biol. (2019) 21:9–17. doi: 10.1038/s41556-018-0250-9
80. Dupont M, Souriant S, Lugo-Villarino G, Maridonneau-Parini I, Verollet C. Tunneling nanotubes: intimate communication between myeloid cells. Front Immunol. (2018) 9:43. doi: 10.3389/fimmu.2018.00043
81. Hashimoto M, Bhuyan F, Hiyoshi M, Noyori O, Nasser H, Miyazaki M, et al. Potential role of the formation of tunneling nanotubes in HIV-1 spread in macrophages. J Immunol. (2016) 196:1832–41. doi: 10.4049/jimmunol.1500845
82. Uhl J, Gujarathi S, Waheed AA, Gordon A, Freed EO, Gousset K. Myosin-X is essential to the intercellular spread of HIV-1 Nef through tunneling nanotubes. J Cell Commun Signal. (2018) 13:209–24. doi: 10.1007/s12079-018-0493-z
83. Xu W, Santini PA, Sullivan JS, He B, Shan M, Ball SC, et al. HIV-1 evades virus-specific IgG2 and IgA responses by targeting systemic and intestinal B cells via long-range intercellular conduits. Nat Immunol. (2009) 10:1008–17. doi: 10.1038/ni.1753
84. Park IW, Fan Y, Luo X, Ryou MG, Liu J, Green L, et al. HIV-1 Nef is transferred from expressing T cells to hepatocytic cells through conduits and enhances HCV replication. PLoS ONE. (2014) 9:e99545. doi: 10.1371/journal.pone.0099545
85. Wang T, Green LA, Gupta SK, Kim C, Wang L, Almodovar S, et al. Transfer of intracellular HIV Nef to endothelium causes endothelial dysfunction. PLoS ONE. (2014) 9:e91063. doi: 10.1371/journal.pone.0091063
86. Roberts TK, Eugenin EA, Lopez L, Romero IA, Weksler BB, Couraud PO, et al. CCL2 disrupts the adherens junction: implications for neuroinflammation. Lab Invest. (2012) 92:1213–33. doi: 10.1038/labinvest.2012.80
87. Stamatovic SM, Keep RF, Kunkel SL, Andjelkovic AV. Potential role of MCP-1 in endothelial cell tight junction 'opening': signaling via Rho and Rho kinase. J Cell Sci. (2003) 116:4615–28. doi: 10.1242/jcs.00755
88. Yao Y, Tsirka SE. Monocyte chemoattractant protein-1 and the blood-brain barrier. Cell Mol Life Sci. (2014) 71:683–97. doi: 10.1007/s00018-013-1459-1
89. Paul D, Ge S, Lemire Y, Jellison ER, Serwanski DR, Ruddle NH, et al. Cell-selective knockout and 3D confocal image analysis reveals separate roles for astrocyte-and endothelial-derived CCL2 in neuroinflammation. J Neuroinflammation. (2014) 11:10. doi: 10.1186/1742-2094-11-10
90. Matsumoto H, Kumon Y, Watanabe H, Ohnishi T, Shudou M, Chuai M, et al. Accumulation of macrophage-like cells expressing NG2 proteoglycan and Iba1 in ischemic core of rat brain after transient middle cerebral artery occlusion. J Cereb Blood Flow Metab. (2008) 28:149–63. doi: 10.1038/sj.jcbfm.9600519
91. Smirkin A, Matsumoto H, Takahashi H, Inoue A, Tagawa M, Ohue S, et al. Iba1(+)/NG2(+) macrophage-like cells expressing a variety of neuroprotective factors ameliorate ischemic damage of the brain. J Cereb Blood Flow Metab. (2010) 30:603–15. doi: 10.1038/jcbfm.2009.233
92. Tei N, Tanaka J, Sugimoto K, Nishihara T, Nishioka R, Takahashi H, et al. Expression of MCP-1 and fractalkine on endothelial cells and astrocytes may contribute to the invasion and migration of brain macrophages in ischemic rat brain lesions. J Neurosci Res. (2013) 91:681–93. doi: 10.1002/jnr.23202
93. Ajami B, Bennett JL, Krieger C, McNagny KM, Rossi FM. Infiltrating monocytes trigger EAE progression, but do not contribute to the resident microglia pool. Nat Neurosci. (2011) 14:1142–9. doi: 10.1038/nn.2887
94. Ajami B, Bennett JL, Krieger C, Tetzlaff W, Rossi FM. Local self-renewal can sustain CNS microglia maintenance and function throughout adult life. Nat Neurosci. (2007) 10:1538–43. doi: 10.1038/nn2014
95. Fan Y, Liu C, Qin X, Wang Y, Han Y, Zhou Y. The role of ERK1/2 signaling pathway in Nef protein upregulation of the expression of the intercellular adhesion molecule 1 in endothelial cells. Angiology. (2010) 61:669–78. doi: 10.1177/0003319710364215
96. Dhawan S, Weeks BS, Soderland C, Schnaper HW, Toro LA, Asthana SP, et al. HIV-1 infection alters monocyte interactions with human microvascular endothelial cells. J Immunol. (1995) 154:422–32.
97. Stent G, Crowe SM. Effects of HIV-1 on the surface expression of LFA-1 on cultured monocytes. J Acquir Immune Defic Syndr Hum Retrovirol. (1997) 15:95–103. doi: 10.1097/00042560-199706010-00001
98. Maus U, Henning S, Wenschuh H, Mayer K, Seeger W, Lohmeyer J. Role of endothelial MCP-1 in monocyte adhesion to inflamed human endothelium under physiological flow. Am J Physiol Heart Circ Physiol. (2002) 283:H2584–91. doi: 10.1152/ajpheart.00349.2002
99. Weber KS, Klickstein LB, Weber C. Specific activation of leukocyte beta2 integrins lymphocyte function-associated antigen-1 and Mac-1 by chemokines mediated by distinct pathways via the alpha subunit cytoplasmic domains. Mol Biol Cell. (1999) 10:861–73. doi: 10.1091/mbc.10.4.861
100. Dzenko KA, Song L, Ge S, Kuziel WA, Pachter JS. CCR2 expression by brain microvascular endothelial cells is critical for macrophage transendothelial migration in response to CCL2. Microvasc Res. (2005) 70:53–64. doi: 10.1016/j.mvr.2005.04.005
101. Williams DW, Veenstra M, Gaskill PJ, Morgello S, Calderon TM, Berman JW. Monocytes mediate HIV neuropathogenesis: mechanisms that contribute to HIV associated neurocognitive disorders. Curr HIV Res. (2014) 12:85–96. doi: 10.2174/1570162X12666140526114526
102. Kraft-Terry SD, Stothert AR, Buch S, Gendelman HE. HIV-1 neuroimmunity in the era of antiretroviral therapy. Neurobiol Dis. (2010) 37:542–8. doi: 10.1016/j.nbd.2009.12.015
103. Mangus LM, Beck SE, Queen SE, Brill SA, Shirk EN, Metcalf Pate KA, et al. Lymphocyte-dominant encephalitis and meningitis in simian immunodeficiency virus-infected macaques receiving antiretroviral therapy. Am J Pathol. (2018) 188:125–34. doi: 10.1016/j.ajpath.2017.08.035
104. Churchill MJ, Gorry PR, Cowley D, Lal L, Sonza S, Purcell DF, et al. Use of laser capture microdissection to detect integrated HIV-1 DNA in macrophages and astrocytes from autopsy brain tissues. J Neurovirol. (2006) 12:146–52. doi: 10.1080/13550280600748946
105. Hurtrel B, Chakrabarti L, Hurtrel M, Montagnier L. Target cells during early SIV encephalopathy. Res Virol. (1993) 144:41–6. doi: 10.1016/S0923-2516(06)80010-5
106. Michaels J, Sharer LR, Epstein LG. Human immunodeficiency virus type 1 (HIV-1) infection of the nervous system: a review. Immunodefic Rev. (1988) 1:71–104.
107. Raymond AD, Diaz P, Chevelon S, Agudelo M, Yndart-Arias A, Ding H, et al. Microglia-derived HIV Nef+ exosome impairment of the blood-brain barrier is treatable by nanomedicine-based delivery of Nef peptides. J Neurovirol. (2016) 22:129–39. doi: 10.1007/s13365-015-0397-0
108. Ward JM, O'Leary TJ, Baskin GB, Benveniste R, Harris CA, Nara PL, et al. Immunohistochemical localization of human and simian immunodeficiency viral antigens in fixed tissue sections. Am J Pathol. (1987) 127:199–205.
109. Russell RA, Chojnacki J, Jones DM, Johnson E, Do T, Eggeling C, et al. Astrocytes resist HIV-1 fusion but engulf infected macrophage material. Cell Rep. (2017) 18:1473–83. doi: 10.1016/j.celrep.2017.01.027
110. Al-Harti L, Joseph J, Nath A. Astrocytes as an HIV CNS reservoir: highlights and reflections of an NIMH-sponsored symposium. J Neurovirol. (2018) 24:665–9. doi: 10.1007/s13365-018-0691-8
111. Ranki A, Nyberg M, Ovod V, Haltia M, Elovaara I, Raininko R, et al. Abundant expression of HIV Nef and Rev proteins in brain astrocytes in vivo is associated with dementia. AIDS. (1995) 9:1001–8. doi: 10.1097/00002030-199509000-00004
112. Bencheikh M, Bentsman G, Sarkissian N, Canki M, Volsky DJ. Replication of different clones of human immunodeficiency virus type 1 in primary fetal human astrocytes: enhancement of viral gene expression by Nef. J Neurovirol. (1999) 5:115–24. doi: 10.3109/13550289909021993
113. Puzar Dominkus P, Ferdin J, Plemenitas A, Peterlin BM, Lenassi M. Nef is secreted in exosomes from Nef.GFP-expressing and HIV-1-infected human astrocytes. J Neurovirol. (2017) 23:713–24. doi: 10.1007/s13365-017-0552-x
114. Sami Saribas A, Cicalese S, Ahooyi TM, Khalili K, Amini S, Sariyer IK. HIV-1 Nef is released in extracellular vesicles derived from astrocytes: evidence for Nef-mediated neurotoxicity. Cell Death Dis. (2017) 8:e2542. doi: 10.1038/cddis.2016.467
115. van Marle G, Henry S, Todoruk T, Sullivan A, Silva C, Rourke SB, et al. Human immunodeficiency virus type 1 Nef protein mediates neural cell death: a neurotoxic role for IP-10. Virology. (2004) 329:302–18. doi: 10.1016/j.virol.2004.08.024
116. Lehmann MH, Masanetz S, Kramer S, Erfle V. HIV-1 Nef upregulates CCL2/MCP-1 expression in astrocytes in a myristoylation- and calmodulin-dependent manner. J Cell Sci. (2006) 119:4520–30. doi: 10.1242/jcs.03231
117. Chompre G, Cruz E, Maldonado L, Rivera-Amill V, Porter JT, Noel RJ Jr. Astrocytic expression of HIV-1 Nef impairs spatial and recognition memory. Neurobiol Dis. (2013) 49:128–36. doi: 10.1016/j.nbd.2012.08.007
118. Acharjee S, Branton WG, Vivithanaporn P, Maingat F, Paul AM, Dickie P, et al. HIV-1 Nef expression in microglia disrupts dopaminergic and immune functions with associated mania-like behaviors. Brain Behav Immun. (2014) 40:74–84. doi: 10.1016/j.bbi.2014.02.016
119. Mordelet E, Kissa K, Cressant A, Gray F, Ozden S, Vidal C, et al. Histopathological and cognitive defects induced by Nef in the brain. FASEB J. (2004) 18:1851–61. doi: 10.1096/fj.04-2308com
120. Cinque P, Vago L, Mengozzi M, Torri V, Ceresa D, Vicenzi E, et al. Elevated cerebrospinal fluid levels of monocyte chemotactic protein-1 correlate with HIV-1 encephalitis and local viral replication. AIDS. (1998) 12:1327–32. doi: 10.1097/00002030-199811000-00014
121. Conant K, Garzino-Demo A, Nath A, McArthur JC, Halliday W, Power C, et al. Induction of monocyte chemoattractant protein-1 in HIV-1 Tat-stimulated astrocytes and elevation in AIDS dementia. Proc Natl Acad Sci USA. (1998) 95:3117–21. doi: 10.1073/pnas.95.6.3117
122. Persidsky Y, Ghorpade A, Rasmussen J, Limoges J, Liu XJ, Stins M, et al. Microglial and astrocyte chemokines regulate monocyte migration through the blood-brain barrier in human immunodeficiency virus-1 encephalitis. Am J Pathol. (1999) 155:1599–611. doi: 10.1016/S0002-9440(10)65476-4
123. Zink MC, Coleman GD, Mankowski JL, Adams RJ, Tarwater PM, Fox K, et al. Increased macrophage chemoattractant protein-1 in cerebrospinal fluid precedes and predicts simian immunodeficiency virus encephalitis. J Infect Dis. (2001) 184:1015–21. doi: 10.1086/323478
124. Gonzalez E, Rovin BH, Sen L, Cooke G, Dhanda R, Mummidi S, et al. HIV-1 infection and AIDS dementia are influenced by a mutant MCP-1 allele linked to increased monocyte infiltration of tissues and MCP-1 levels. Proc Natl Acad Sci USA. (2002) 99:13795–800. doi: 10.1073/pnas.202357499
125. Yao H, Yang Y, Kim KJ, Bethel-Brown C, Gong N, Funa K, et al. Molecular mechanisms involving sigma receptor-mediated induction of MCP-1: implication for increased monocyte transmigration. Blood. (2010) 115:4951–62. doi: 10.1182/blood-2010-01-266221
126. Eugenin EA, Osiecki K, Lopez L, Goldstein H, Calderon TM, Berman JW. CCL2/monocyte chemoattractant protein-1 mediates enhanced transmigration of human immunodeficiency virus (HIV)-infected leukocytes across the blood-brain barrier: a potential mechanism of HIV-CNS invasion and NeuroAIDS. J Neurosci. (2006) 26:1098–106. doi: 10.1523/JNEUROSCI.3863-05.2006
127. Parillaud VR, Lornet G, Monnet Y, Privat AL, Haddad AT, Brochard V, et al. Analysis of monocyte infiltration in MPTP mice reveals that microglial CX3CR1 protects against neurotoxic over-induction of monocyte-attracting CCL2 by astrocytes. J Neuroinflammation. (2017) 14:60. doi: 10.1186/s12974-017-0830-9
128. Severini C, Passeri PP, Ciotti M, Florenzano F, Possenti R, Zona C, et al. Bindarit, inhibitor of CCL2 synthesis, protects neurons against amyloid-beta-induced toxicity. J Alzheimers Dis. (2014) 38:281–93. doi: 10.3233/JAD-131070
129. Tian DS, Peng J, Murugan M, Feng LJ, Liu JL, Eyo UB, et al. Chemokine CCL2-CCR2 signaling induces neuronal cell death via STAT3 activation and IL-1beta production after status epilepticus. J Neurosci. (2017) 37:7878–92. doi: 10.1523/JNEUROSCI.0315-17.2017
130. Brandt C, Zvolensky MJ, Woods SP, Gonzalez A, Safren SA, O'Cleirigh CM. Anxiety symptoms and disorders among adults living with HIV and AIDS: a critical review and integrative synthesis of the empirical literature. Clin Psychol Rev. (2017) 51:164–84. doi: 10.1016/j.cpr.2016.11.005
131. Prakash A, Hou J, Liu L, Gao Y, Kettering C, Ragin AB. Cognitive function in early HIV infection. J Neurovirol. (2017) 23:273–82. doi: 10.1007/s13365-016-0498-4
132. Smail RC, Brew BJ. HIV-associated neurocognitive disorder. Handb Clin Neurol. (2018) 152:75–97. doi: 10.1016/B978-0-444-63849-6.00007-4
133. Dimitrijevic OB, Stamatovic SM, Keep RF, Andjelkovic AV. Absence of the chemokine receptor CCR2 protects against cerebral ischemia/reperfusion injury in mice. Stroke. (2007) 38:1345–53. doi: 10.1161/01.STR.0000259709.16654.8f
134. Kaufer C, Chhatbar C, Broer S, Waltl I, Ghita L, Gerhauser I, et al. Chemokine receptors CCR2 and CX3CR1 regulate viral encephalitis-induced hippocampal damage but not seizures. Proc Natl Acad Sci USA. (2018) 115:E8929–38. doi: 10.1073/pnas.1806754115
135. Conductier G, Blondeau N, Guyon A, Nahon JL, Rovere C. The role of monocyte chemoattractant protein MCP1/CCL2 in neuroinflammatory diseases. J Neuroimmunol. (2010) 224:93–100. doi: 10.1016/j.jneuroim.2010.05.010
136. Ge S, Song L, Serwanski DR, Kuziel WA, Pachter JS. Transcellular transport of CCL2 across brain microvascular endothelial cells. J Neurochem. (2008) 104:1219–32. doi: 10.1111/j.1471-4159.2007.05056.x
137. Van Steenwinckel J, Reaux-Le Goazigo A, Pommier B, Mauborgne A, Dansereau MA, Kitabgi P, et al. CCL2 released from neuronal synaptic vesicles in the spinal cord is a major mediator of local inflammation and pain after peripheral nerve injury. J Neurosci. (2011) 31:5865–75. doi: 10.1523/JNEUROSCI.5986-10.2011
138. Parker R, Stein DJ, Jelsma J. Pain in people living with HIV/AIDS: a systematic review. J Int AIDS Soc. (2014) 17:18719. doi: 10.7448/IAS.17.1.18719
139. Fischer-Smith T, Rappaport J. Evolving paradigms in the pathogenesis of HIV-1-associated dementia. Expert Rev Mol Med. (2005) 7:1–26. doi: 10.1017/S1462399405010239
140. Marincowitz C, Genis A, Goswami N, De Boever P, Nawrot TS, Strijdom H. Vascular endothelial dysfunction in the wake of HIV and ART. FEBS J. (2019) 286:1256–70. doi: 10.1111/febs.14657
141. Covino DA, Sabbatucci M, Fantuzzi L. The CCL2/CCR2 axis in the pathogenesis of HIV-1 infection: a new cellular target for therapy? Curr Drug Targets. (2016) 17:76–110. doi: 10.2174/138945011701151217110917
142. Dickens AM, Yoo SW, Chin AC, Xu J, Johnson TP, Trout AL, et al. Chronic low-level expression of HIV-1 Tat promotes a neurodegenerative phenotype with aging. Sci Rep. (2017) 7:7748. doi: 10.1038/s41598-017-07570-5
143. Khiati A, Chaloin O, Muller S, Tardieu M, Horellou P. Induction of monocyte chemoattractant protein-1 (MCP-1/CCL2) gene expression by human immunodeficiency virus-1 Tat in human astrocytes is CDK9 dependent. J Neurovirol. (2010) 16:150–67. doi: 10.3109/13550281003735691
144. Campbell GR, Watkins JD, Singh KK, Loret EP, Spector SA. Human immunodeficiency virus type 1 subtype C Tat fails to induce intracellular calcium flux and induces reduced tumor necrosis factor production from monocytes. J Virol. (2007) 81:5919–28. doi: 10.1128/JVI.01938-06
145. Albini A, Ferrini S, Benelli R, Sforzini S, Giunciuglio D, Aluigi MG, et al. HIV-1 Tat protein mimicry of chemokines. Proc Natl Acad Sci USA. (1998) 95:13153–8. doi: 10.1073/pnas.95.22.13153
146. Sabatier JM, Vives E, Mabrouk K, Benjouad A, Rochat H, Duval A, et al. Evidence for neurotoxic activity of tat from human immunodeficiency virus type 1. J Virol. (1991) 65:961–7. doi: 10.1007/978-94-011-2264-1_282
Keywords: AIDS, astrocyte, autophagy, chemokine, dementia, inflammation, neuron, virus
Citation: Lehmann MH, Lehmann JM and Erfle V (2019) Nef-induced CCL2 Expression Contributes to HIV/SIV Brain Invasion and Neuronal Dysfunction. Front. Immunol. 10:2447. doi: 10.3389/fimmu.2019.02447
Received: 27 June 2019; Accepted: 01 October 2019;
Published: 15 October 2019.
Edited by:
Serge Benichou, Centre National de la Recherche Scientifique (CNRS), FranceReviewed by:
Laura Fantuzzi, National Institute of Health (ISS), ItalyTetsuo Tsukamoto, Kindai University, Japan
Fan Wu, Fudan University, China
Matthias Clauss, Indiana University Bloomington, United States
Copyright © 2019 Lehmann, Lehmann and Erfle. This is an open-access article distributed under the terms of the Creative Commons Attribution License (CC BY). The use, distribution or reproduction in other forums is permitted, provided the original author(s) and the copyright owner(s) are credited and that the original publication in this journal is cited, in accordance with accepted academic practice. No use, distribution or reproduction is permitted which does not comply with these terms.
*Correspondence: Michael H. Lehmann, T3JsYXRhbGVyQHdlYi5kZQ==