- 1Department of Biosciences, KEMRI-Wellcome Trust Programme, Kilifi, Kenya
- 2Immunology and Infection Department, London School of Hygiene and Tropical Medicine, London, United Kingdom
- 3Radboud Institute for Health Sciences, Radboud University Medical Center, Nijmegen, Netherlands
- 4Department of Life Sciences, Imperial College London, London, United Kingdom
- 5Department of Pathology, University of Cambridge, Cambridge, United Kingdom
- 6Nuffield Department of Medicine, Centre for Tropical Medicine and Global Health, University of Oxford, Oxford, United Kingdom
Background: Malaria elimination remains a priority research agenda with the need for interventions that reduce and/or block malaria transmission from humans to mosquitoes. Transmission-blocking vaccines (TBVs) are in development, most of which target the transmission stage (i.e., gametocyte) antigens Pfs230 and Pfs48/45. For these interventions to be implemented, there is a need to understand the naturally acquired immunity to gametocytes. Several studies have measured the prevalence of immune responses to Pfs230 and Pfs48/45 in populations in malaria-endemic areas.
Methods: We conducted a systematic review of studies carried out in African populations that measured the prevalence of immune responses to the gametocyte antigens Pfs230 and Pfs48/45. We assessed seroprevalence of antibody responses to the two antigens and investigated the effects of covariates such as age, transmission intensity/endemicity, season, and parasite prevalence on the prevalence of these antibody responses by meta-regression.
Results: We identified 12 studies covering 23 sites for inclusion in the analysis. We found that the range of reported seroprevalence to Pfs230 and Pfs48/45 varied widely across studies, from 0 to 64% for Pfs48/45 and from 6 to 72% for Pfs230. We also found a modest association between increased age and increased seroprevalence to Pfs230: adults were associated with higher seroprevalence estimates in comparison to children (β coefficient 0.21, 95% CI: 0.05–0.38, p = 0.042). Methodological factors were the most significant contributors to heterogeneity between studies which prevented calculation of pooled prevalence estimates.
Conclusions: Naturally acquired sexual stage immunity, as detected by antibodies to Pfs230 and Pfs48/45, was present in most studies analyzed. Significant between-study heterogeneity was seen, and methodological factors were a major contributor to this, and prevented further analysis of epidemiological and biological factors. This demonstrates a need for standardized protocols for conducting and reporting seroepidemiological analyses.
Introduction
Progress has been made in controlling malaria with marked reductions in the global disease burden reported from 2000 onwards (1, 2). However, the 2018 World Malaria Report indicates stalling progress in malaria control in the past two years (1) which threatens the gains made in malaria control and hence efforts to develop new methods to control malaria must remain a priority. Vector control and treatment of acute malaria episodes remain key strategies. In addition to these malaria control strategies, there has been renewed interest in developing transmission-blocking vaccines (TBVs). While TBVs do not protect against clinical disease, they aim to reduce the infectiousness of mosquitoes thereby interrupting transmission (3, 4). The concept of a TBV was first demonstrated in early experiments in the late 1950s (5) and later in 1976 (6, 7) where reduced infectivity of gametocytes was demonstrated following immunization of avian hosts with inactivated gametocytes and gametes. Further experiments aimed to identify the targets of this transmission-reducing immunity by utilizing murine monoclonal antibodies to immunoprecipitate radioisotope-labeled female gametes (8, 9). The first TBV candidate antigens, Pfs25, Pfs230, and Pfs48/45 were thus identified, so named due to their observed molecular weight after separation by SDS-PAGE. Functional characterization of the Pfs230 and Pfs48/45 proteins has shown them to be essential for male gamete fertility and zygote formation (10, 11) while Pfs25 plays a role in ookinete to oocyst transition (9, 12).
Unlike Pfs25, which is expressed on the surface of activated female gametes and ookinetes post-fertilization in the mosquito midgut, Pfs230, and Pfs48/45 are expressed pre-fertilization in the mature gametocyte stages (13, 14) that are present in peripheral circulation awaiting uptake during a mosquito blood meal. The majority of these circulating gametocytes, however, are destroyed by the host immune system prior to transmission thus antigens present on the surface of the gametocytes are presented to the immune system (15, 16). Naturally acquired immune responses to Pfs230 and Pfs48/45 have been detected in the sera of individuals living in malaria-endemic areas (16–20) providing evidence that pre-fertilization sexual stage antigens are immune targets. Additionally, sera from individuals with high responses to Pfs230 and Pfs48/45 have been shown to exhibit transmission-reducing immunity (18, 20–23). Such naturally acquired transmission-reducing immunity (NA-TRI) has also been recently shown to reduce infectiousness in field settings (24). Though considered lead vaccine candidates, challenges in developing immunogenic vaccine constructs for Pfs230 and Pfs48/45 have delayed their evaluation in clinical trials. Both proteins contain a cysteine-rich domain whose disulphide-bonding pattern results in a complex tertiary structure that presents a challenge for producing properly folded recombinant protein (25, 26). The mapping of immunodominant epitopes (27, 28) and the development of various platforms for the production of properly folded Pfs230 and Pfs48/45 (29–32) have aided not only vaccine design but also seroepidemiological studies.
Several studies have now been carried out across multiple sites looking at naturally acquired immune responses to Pfs230 and Pfs48/45 (19, 20, 30, 33, 34) in a bid to improve our understanding of naturally acquired immunity (NAI) to sexual stage antigens. Classical indicators of parasite exposure such as age, transmission setting, malaria transmission season and parasite prevalence have been evaluated in a bid to describe the dynamics of sexual stage immunity. Discrepancies exist, however, in the observed associations of the aforementioned factors and seroprevalence of antibodies to sexual-stage antigens. For instance, there is no consensus on the impact of age on the development of sexual stage immunity, with some studies showing an increase in antibody prevalence with age (19, 33, 35) while other studies show no association with age (16, 36). Furthermore, while some studies have shown an increase in prevalence of responses in high transmission settings in comparison to low transmission settings (19, 34), other studies report higher responses in low transmission settings (36). Additionally, varied study designs and sampling protocols may affect the estimates of seroprevalence reported, making it difficult to provide an estimate of how common responses to sexual stage antigens are in the population.
Rationale
A better understanding of transmission-reducing immunity can offer important insights for the design, assessment and implementation of TBVs. This is of particular importance as though Pfs48/45 and Pfs230 are currently the most widely evaluated pre-fertilization TBV candidate antigens, evidence for the role of other antigens in the acquisition of NA-TRI (20, 36) would drastically increase the number of candidate antigens for evaluation. Understanding the dynamics of NA-TRI can provide important insights into the prioritization of candidate antigens for clinical testing. This work aims at providing a description of the prevalence of antibodies to sexual stage antigens and factors influencing sexual stage immune responses by analyzing responses to Pfs230 and Pfs48/45.
Objectives
Our study investigates naturally acquired transmission-reducing immunity in malaria-endemic populations in Africa. Specifically, it aims to:
a. Describe the prevalence of antibodies to the widely studied gametocyte antigens Pfs230 and Pfs48/45.
b. Identify factors associated with the acquisition of naturally acquired transmission-reducing immunity.
c. Describe the dynamics of naturally acquired transmission reducing immunity.
Research Question
This systematic review and meta-analysis aims to address the question “What is the seroprevalence and, at a population level, what are the factors that influence the development of naturally acquired anti-gametocyte immune responses in individuals living in falciparum-malaria endemic areas in Africa?”
Methods
We performed a systematic review of studies of naturally acquired Plasmodium falciparum transmission-reducing immunity in Africa that reported the prevalence of antibodies to the widely studied gametocyte antigens Pfs230 and Pfs48/45. We followed the Meta-analysis Of Observational Studies in Epidemiology (MOOSE) guidelines to conduct our analyses (37) and report our results according to the PRISMA (Preferred Reported Items for Systematic Reviews and Meta-Analyses) guidelines (38) (Supplementary Table 1). The study protocol is registered on PROSPERO (number CRD42019126701).
Study Design
We considered cross-sectional and longitudinal studies in our analyses. The inclusion of longitudinal studies that were spread over the malaria transmission season allowed for examination of transmission season as a potential modulator of sexual stage immune responses. We excluded hospital-based studies as they potentially would confound our results since these studies recruited participants with acute malaria infection. Our goal was to describe seroprevalence in a way that was generalizable at a population level.
Participants
The study population investigated was individuals living in malaria-endemic areas in Africa. We included studies recruiting both children and adults to be as representative as possible and our outcome was the development of antibodies to Pfs230 and/or Pfs48/45.
Search Strategy
The search strategy was based on the keywords: (pfs230 OR pfs48 OR pfs45) AND (antibodies OR immunity OR response) AND (plasmodium OR falciparum OR malaria). Reference lists of relevant studies were also searched for additional studies.
Data Sources, Studies Selection, and Data Extraction
Data Sources
Databases searched were MEDLINE/PubMed, SCOPUS, Web of Science, African Index Medicus, Embase, and African Journals Online from 1st February 2019 to 31st March 2019. We contacted study authors to provide prevalence data where it was not possible to extract the information directly from the published source. Alternatively, if raw data were available in public repositories, we used these data to estimate seroprevalence.
Study Selection
Criteria for study inclusion were: (1) studies reporting data from Africa (2) studies that measured antibody responses to Pfs230 and/or Pfs48/45. Studies from all years and written in all languages were included. Studies were excluded if: (1) they only reported antibody responses to non-falciparum antigens (2) they were vaccine, drug, or any other interventional trial (3) they analyzed responses in pregnant women (4) they did not measure antibody responses quantitatively (5) they sampled fewer than 30 participants (where studies recruited both children and adults, studies with fewer than 30 participants in each category were excluded). Where two studies had analyzed the same cohort, we considered the study where seroprevalence was evaluated in relation to a larger number of variables that were to be tested in the analyses.
Data Extraction
Data on seroprevalence to Pfs230 and/or Pfs48/45 were extracted from the studies using a standardized data extraction form. The data extraction form was developed to capture information on the study site, transmission intensity of the study site, season during which the participants were recruited, asexual and sexual parasite prevalence, study population, study design, age categories investigated, type of immunoassay used to detect immune responses, antigen coating concentration, serum dilution, source of antigen for the immunoassay, type of negative controls used, and method used to assess seropositivity.
Data Analysis
Heterogeneity between studies was assessed using the Cochran's Q, I2, and H statistics with I2 values of <30, 30–75, and >75% as cutoffs for low, moderate, and high estimates of heterogeneity, respectively. Sources of heterogeneity were explored through moderator analysis using sub-grouping and meta-regression. Conservative p-values were calculated using the “Knapp-Hartung” method. Additionally, we adjusted p-values for multiple comparisons using the Benjamini and Hochberg correction (39). We calculated the change in heterogeneity observed after each univariable analysis using the formula [(overall heterogeneity – residual heterogeneity/overall heterogeneity) * 100].
Variables explored in the meta-regression included: age group (broadly categorized as children [0–17 years of age] vs. adults [≥18 years of age]), parasite prevalence, antigen source for immunoassay (recombinant protein vs. gametocyte extract), antigen coating concentration used in the immunoassay, and seropositivity cut-off (2 or 3 standard deviations above the antibody reactivity of malaria naïve individuals or seronegative individuals identified using maximum likelihood methods to define Gaussian populations of low and high responders). Where a study reported seroprevalence for age-categories that slightly overlapped our pre-defined age categories (i.e., children 0–19 years of age or adults >16 years of age) and data were not available to reanalyze the age-category, we used the original study's defined age categories for children and adults in our analyses. For parasite prevalence, we used microscopy-based estimates as that was the parasite detection method used by majority of the studies.
To infer transmission intensity at the time of sampling in a uniform way across studies, we used data from the study by Snow et al. collected from across Africa from the early 1900s to 2015 that reported the predicted parasite rate standardized for 2–10-year olds (Pf PR2−10) (40). We used previously defined endemicity cut-offs (41) to categorize study sites as either hypoendemic; Pf PR2−10 ≤ 10%, mesoendemic; Pf PR2−10 > 10–50% or hyperendemic Pf PR2−10 > 50%. Information on the study site from where participants were sampled was then used to define the site's administrative region which was then used to guide the estimation of the Pf PR2−10 for that site.
For longitudinal studies, the seroprevalence estimates reported at the separate cross-sectional surveys over the follow-up period were combined to give an overall estimate. Where the data were seasonally spaced, each cross-section carried out at either the dry or the rainy season was considered separately in the univariable analysis. However, where a study measured responses at the peak and at the end of the rainy season, these data were pooled and analyzed as responses measured in the rainy season.
Results
Flow Diagram of Studies Retrieved for the Review
Five hundred twenty-five potentially relevant studies were identified from the literature search, 205 of these were unique studies and these were then screened by title and abstract. From the screen, 34 studies were deemed to contain relevant information and after full-text evaluation and assessment against our inclusion criteria, 12 studies were included in the systematic review and meta-analysis. A summary of the selection process is provided in Figure 1.
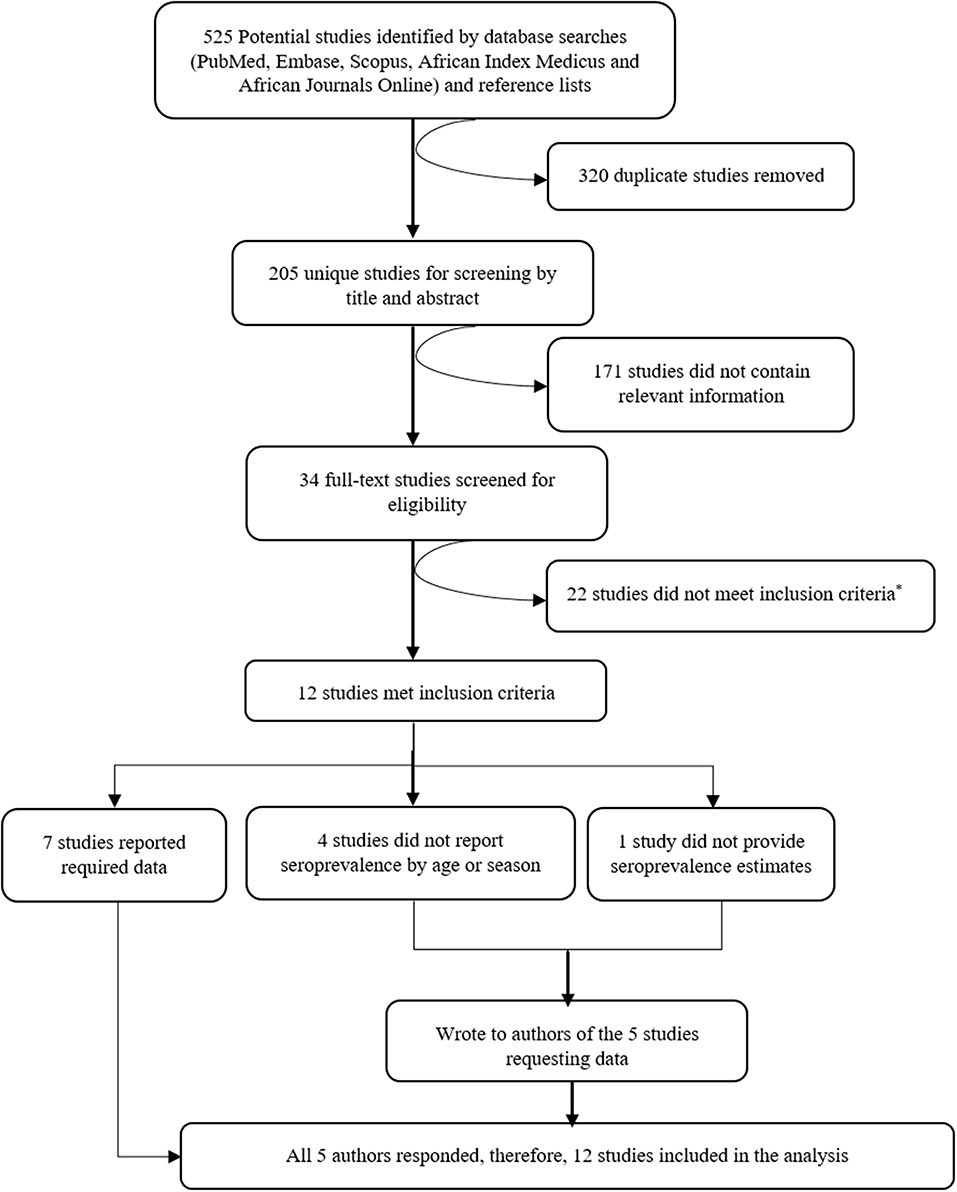
Figure 1. Consort diagram showing the selection of studies to include in the systematic review and meta-analysis. Reasons for exclusion are included at each step. *Reasons for exclusion: six studies measured immune responses semi-quantitatively (four of these in the same population), 11 studies had a sample size of < 30, five studies were healthcare facility-based studies (i.e., primary care facilities or hospitals).
Study Selection and Characteristics
The 12 studies were carried out across 17 study sites, majority of which were in West Africa (Burkina Faso, Senegal, Gabon, Cameroon, Ghana, and Mali) with only one study site in East Africa (Tanzania) and two study sites in Southern Africa (Zimbabwe) (Table 1). Ten articles (from 15 study sites) measured responses to Pfs230 and nine articles (13 study sites) measured responses to Pfs48/45. Six studies were longitudinal studies spread over the malaria transmission season with all but one measuring responses to both Pfs230 and Pfs48/45. Studies predominantly used ELISA as the immunoassay with only one study measuring responses using protein microarrays.
Pfs230
Seroprevalence
Ten studies from across 15 study sites in Africa analyzed immune responses to Pfs230. The range of seroprevalence estimates was quite wide, ranging from 6% reported by Stone et al. in Soumousso and Dande villages, Burkina Faso (20) to 72% reported by Amoah et al. (34) (Figure 2). Significant heterogeneity was observed between the studies (I2 = 97%; 95% CI: 96–98%; p < 0.01) therefore, a pooled prevalence estimate was not calculated.
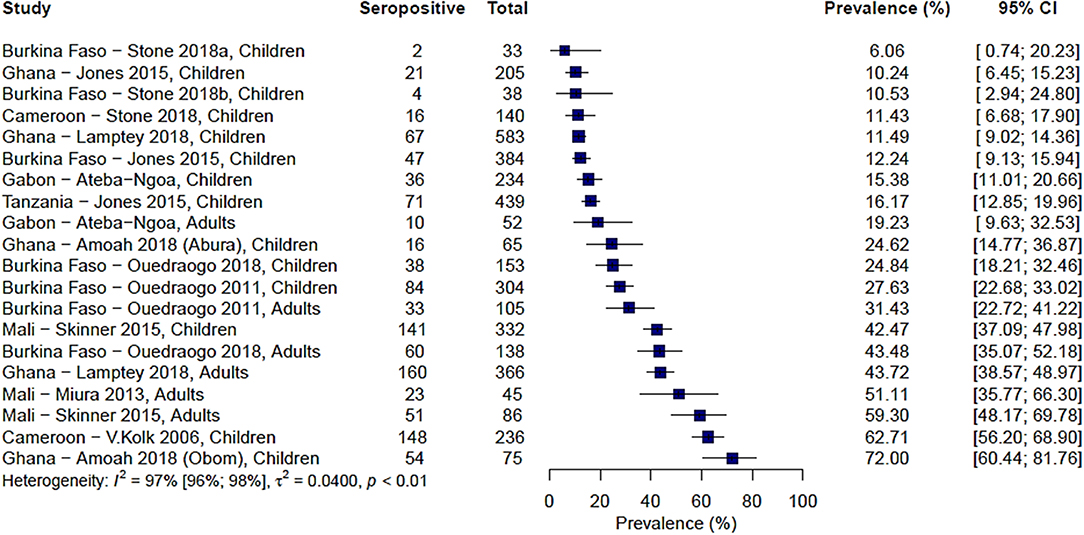
Figure 2. Forest plot of the prevalence of antibodies to Pfs230 in endemic sera from Africa. Seropositive individuals were defined as study participants with an antibody reactivity above a set cut-off defined from seronegative individuals as measured in an immunoassay.
Factors Associated With Reported Seroprevalence to Pfs230
We sought to explore how differences in the study population as well as in the immunoassay protocol employed affected reported seroprevalence to Pfs230 using meta-regression. Additionally, we tested whether the variables explored explained the between-study heterogeneity observed. The data presented in Table 2 show the results of a univariable analysis. Compared to children, adults were associated with higher seroprevalence estimates (β coefficient 0.21, 95% CI: 0.05–0.38, p = 0.042). On the other hand, higher asexual parasite and gametocyte prevalence were associated with lower seroprevalence estimates, however, neither of these associations was statistically significant. Similarly, transmission intensity and sampling season (dry season vs. rainy season) were not significantly associated with seroprevalence estimates.
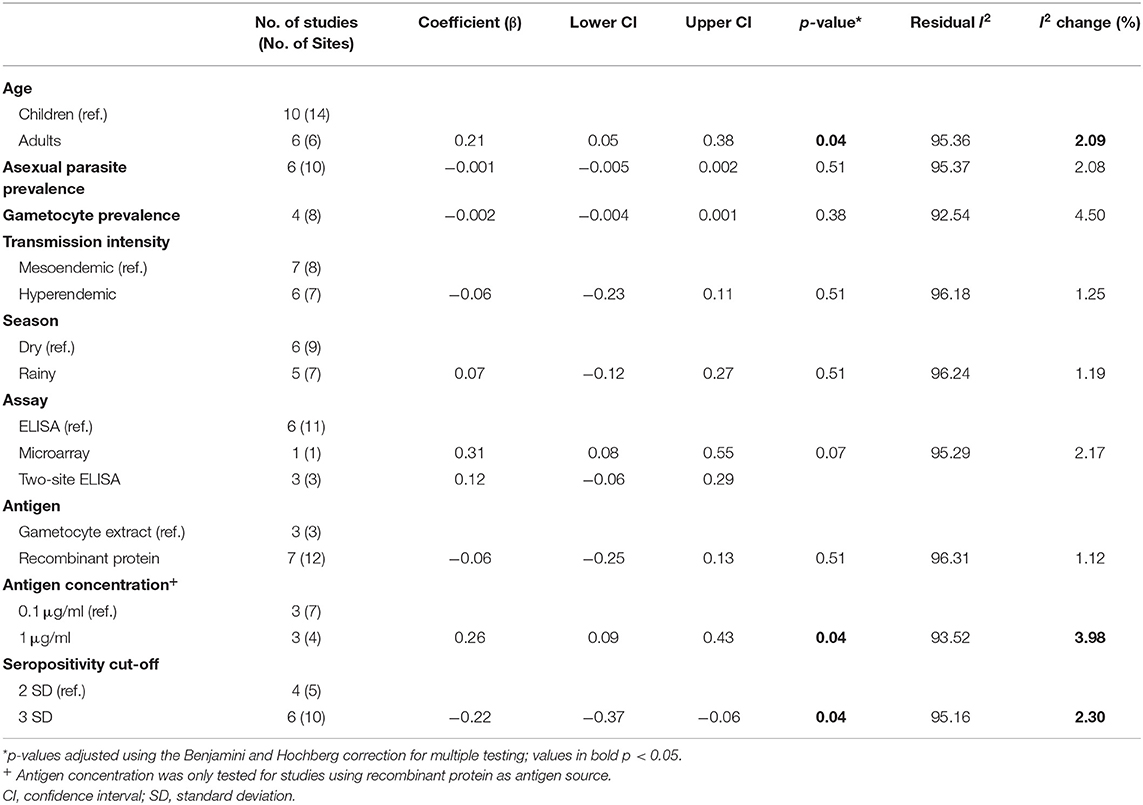
Table 2. Univariable meta-regression analysis of factors influencing reported seroprevalence to Pfs230.
Of the methodological variables tested, in the six studies that used recombinant protein as the source of antigen (19, 20, 34, 35, 44, 45), studies using 1 μg/ml coating concentration of antigen in the immunoassay were associated with higher seroprevalence estimates (β coefficient 0.26, 95% CI: 0.09–0.43, p = 0.042). Moreover, compared to studies that used a seropositivity cut-off of 2 standard deviations (SD) above the mean response of seronegative individuals, studies that used a cut-off of 3 SD were associated with lower seroprevalence estimates (β coefficient −0.22, 95% CI: −0.37 to −0.06, p = 0.042). Though modestly significant (p = 0.07), antibody detection using the microarray platform was associated with higher seroprevalence estimates to Pfs230 (β coefficient 0.31, 95% CI 0.08, 0.55) in comparison to studies using indirect ELISA. Source of antigen (gametocyte extract vs. recombinant protein) was not significantly associated with seroprevalence estimates. Due to the limited number of studies included in the analysis and the fact that not all the variables were reported for each study, we did not attempt further multivariable meta-regression.
When we analyzed the degree of heterogeneity explained by the variables tested, none of the variables resulted in a decrease in residual heterogeneity below 90% precluding calculation of a pooled seroprevalence estimate. This was further confirmed by sub group analyses using age group, antigen coating concentration and seropositivity cut-off as variables (Supplementary Figures 1–3) where the heterogeneity between studies in each category was still greater than our threshold of 75%.
Pfs48/45
Seroprevalence
A total of 9 studies carried out over 13 study sites measured immune responses to Pfs48/45. The range of seroprevalence estimates reported was 0% from Stone et al.'s study sites in Burkina Faso (20) to 64% reported by Paul et al. from their study in the Makoni district in Zimbabwe (43). As with Pfs230, there was significant heterogeneity between the studies, I2 =96% (95% CI: 95–97%), and hence no pooled estimate was calculated (Figure 3).
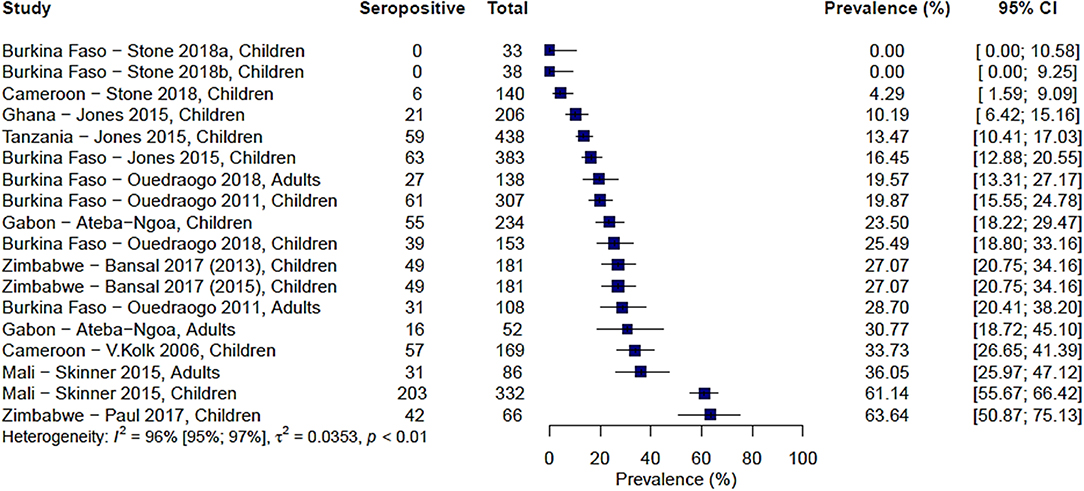
Figure 3. Forest plot of the prevalence of antibodies to Pfs48/45 in endemic sera from Africa. Seropositive individuals were defined as study participants with an antibody reactivity above a set cut-off defined from seronegative individuals as measured in an immunoassay.
We also carried out a pairwise comparison of seroprevalence estimates between Pfs230 and Pfs48/45 in five studies where both antigens were tested using the same protocol per study. Some studies reported higher responses to Pfs230 and others to Pfs48/45, and therefore we found no consistent pattern to suggest higher seroprevalence estimates to either antigen (Supplementary Figure 4).
Factors Associated With Reported Seroprevalence to Pfs48/45
As with Pfs230, we assessed how differences in the study population and in the immunoassay protocol affect reported seroprevalence to Pfs48/45 using meta-regression. From the univariable analysis, adults were associated with modestly higher seroprevalence estimates (β coefficient 0.07, 95% CI: −0.12–0.27) as with Pfs230, however, this was not statistically significant (p = 0.49). Higher parasite prevalence was associated with lower seroprevalence estimates, and this was statistically significant for gametocyte prevalence (β coefficient −0.003, 95% CI: −0.005–0.002, p = 0.003). Similar to Pfs230, transmission intensity and sampling season were not significantly associated with seroprevalence estimates.
Type of immunoassay was significantly associated with seroprevalence estimates as higher seroprevalence was reported where microarray was used in comparison to indirect ELISA (β coefficient 0.36, 95% CI: 0.15–0.56, p = 0.016). Furthermore, studies using 1 μg/ml coating concentration of antigen in the immunoassay were associated with higher seroprevalence estimates (β coefficient 0.30, 95% CI: 0.06–0.54, p = 0.043) while studies that used a seropositivity cut-off of 3 SD were associated with lower seroprevalence estimates (β coefficient −0.26, 95% CI: −0.39, −0.12, p = 0.003). We did not have statistical power to carry out multivariable meta-regression.
Gametocyte prevalence explained a high degree of heterogeneity (i.e., 25%), resulting in a residual heterogeneity of 71%, suggesting that seroprevalence was lower at higher gametocyte prevalence. We then decided to carry out a sub-group analysis of the four studies reporting gametocyte prevalence data (16, 19, 20, 24) with grouping by gametocyte prevalence coded as a categorical variable: <10, 10–50%, and >50% (Figure 4). We found that the observed association of decreased seroprevalence with increased gametocyte prevalence was highly influenced by Stone et al.'s study, where 99% of children sampled were gametocyte positive but reported low seroprevalence to Pfs48/45. This was confirmed by sensitivity analysis carried out in the absence of the Stone et al. study (Supplementary Table 2). Therefore, we do not have strong evidence of an association between seroprevalence and gametocyte prevalence when comparing these studies. Although assay, antigen coating concentration and seropositivity were statistically significant predictors in the meta-regression, sub-group analysis by assay, antigen coating concentration or seropositivity cut-off did not reduce the heterogeneity to below 75% as seen in Table 3 and Supplementary Figures 5–7.
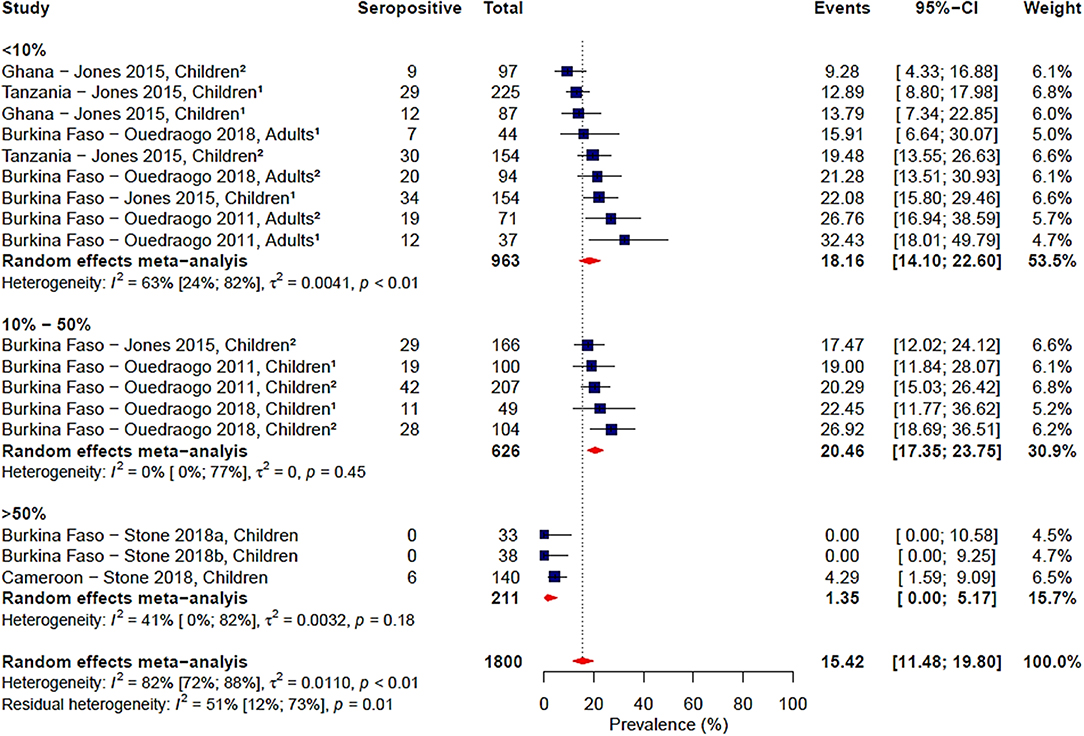
Figure 4. Forest plot of the prevalence of antibodies to Pfs48/45 in endemic sera from Africa grouped by gametocyte prevalence. Seropositive individuals were defined as study participants with an antibody reactivity above a set cut-off defined from seronegative individuals as measured in an immunoassay. 1Participants samples during the dry season; 2Participants sampled during the rainy season.
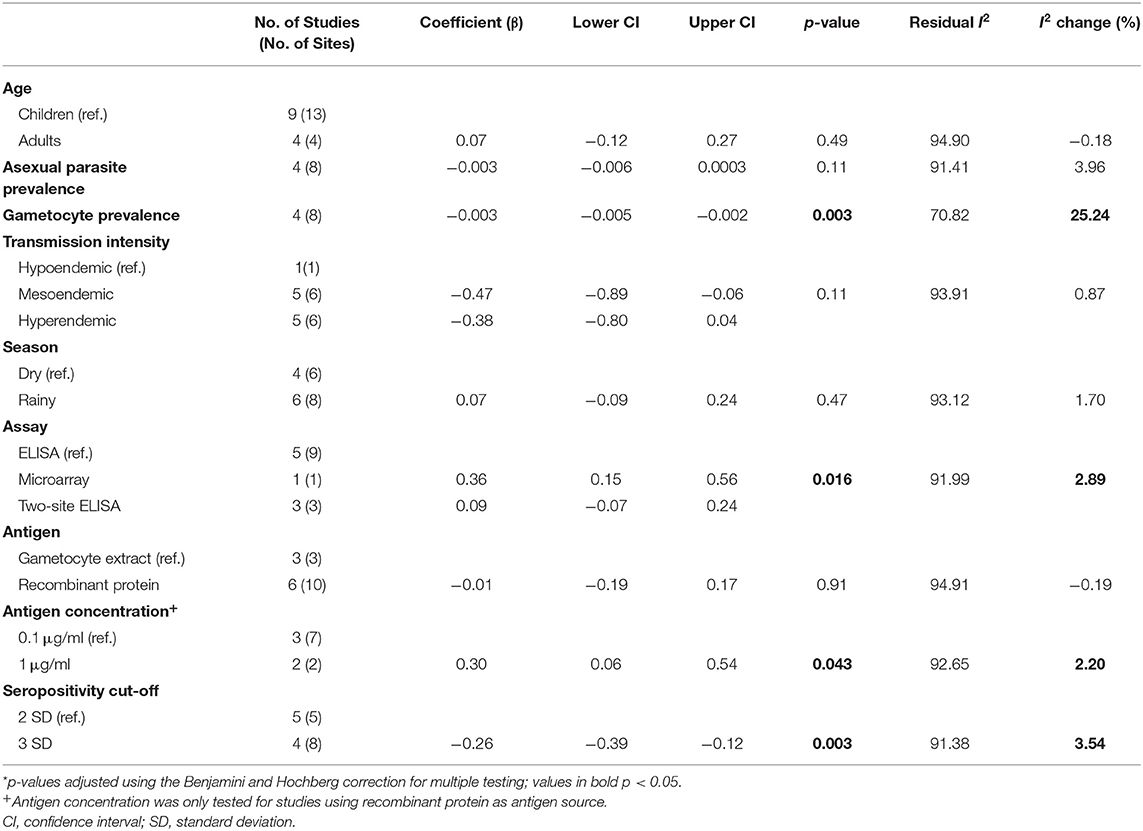
Table 3. Univariable meta-regression analysis of factors influencing reported seroprevalence to Pfs48/45.
Risk of Bias
As the studies included in these analyses were observational and non-comparative, we did not feel it appropriate to test for publication bias. We observed that studies reporting low prevalence were just as likely to report their results as studies reporting a high prevalence of antibodies to Pfs230 and Pfs48/45 as neither of these outcomes are linked to a statistically significant result that would be considered desirable or undesirable.
Discussion
With a renewed interest in developing a malaria TBV, a better understanding of naturally acquired immunity (NAI) to malaria is required to aid vaccine design, evaluation and implementation. We carried out a systematic review and meta-analysis of studies that evaluated naturally acquired immune responses to the widely study vaccine candidate antigens Pfs230 and Pfs48/45 in African populations. By combining results from different studies carried out across the continent, we aimed to define the prevalence of NAI to the two well-characterized sexual stage antigens. Our analysis largely focused on age, transmission intensity, season and parasite prevalence as markers of malaria exposure, and sought to describe their association with seroprevalence to Pfs230 and Pfs48/45 at the population level.
When all studies were examined in combination, the reported seroprevalence to both antigens ranged from 0% (20) to 64% (45) for Pfs48/45 and from 6% (19) to 72% for Pfs230 (34). Between-study heterogeneity did not allow for the pooling of studies to arrive at a single reliable seroprevalence estimate for either antigen. We therefore sought to explore potential factors affecting seroprevalence and their possible contribution to the heterogeneity observed. Factors we considered were age, transmission setting, sampling season and parasite prevalence. Furthermore, noting that there was considerable variation in study design, we also considered methodological factors in the analysis.
The influence of age on NAI to sexual stage antigens has been the subject of much debate with some studies showing no age-dependent acquisition of immune responses (16, 36, 47) while others demonstrate increasing antibody prevalence with age. NAI to asexual stage antigens, for instance, merozoite surface proteins (48, 49) or the infected erythrocyte protein family Plasmodium falciparum erythrocyte membrane protein 1 (50), has been more extensively studied than immunity to sexual stage antigens and reports indicate that immune responses increase with age. This may reflect the time taken to acquire a repertoire of antibodies, particularly to clonally variant antigens such as PfEMP1, and/or the gradual acquisition of long-lived plasma cells and memory B cells with repeated parasite exposure (49, 51). This meta-analysis found a modest association between increased age and an increase in the prevalence of antibodies to the gametocyte antigens Pfs230 and Pfs48/45 which was statistically significant for Pfs230 (Table 2). Some studies have argued that immune responses to sexual stage antibodies are short-lived [potentially due to a largely T-cell independent response (52)] and reflect recent rather than cumulative exposure to gametocytes (16, 36). Though not conclusive, this analysis suggests that there may be a case for long-lived responses to sexual stage antigens. Indeed, Ouedraogo et al. in their recent study found higher prevalence and density of antibodies to Pfs230 and Pfs48/45 in adults which positively correlated with higher transmission-reducing activity (24).
Our combined analysis did not find a definitive association between sampling season and seroprevalence to Pfs230 or Pfs48/45, though there was a trend toward higher seroprevalence during the rainy season. In the individual studies considered in the analysis (16, 19, 24) boosting of responses to gametocyte antigens following the malaria transmission season associated with increased seroprevalence in responses to both antigens. Boosting of responses to pre-fertilization sexual stage antigens during natural malaria infection is an argument put forth in favor of prioritizing antigens such as Pfs230 and Pfs48/45 for TBV design (29, 30, 33). Maintaining high titers of transmission-reducing responses would be essential in reducing malaria transmission potential. The study by Ouedraogo et al. (24) that directly assessed the impact of NAI to Pfs230 and Pfs48/45 on infectiousness to mosquitoes found decreased infectiousness to mosquitoes during the malaria transmission season that also coincided with boosted responses to the two antigens. This observation may provide evidence to support natural boosting of TBV responses.
In addition to looking at age and season, we also evaluated the relationship between transmission intensity and seroprevalence. Though seroprevalence estimates in individual studies conducted across multiple study sites described an increase in seroprevalence with increased transmission settings, we were not able to demonstrate this in our meta-analysis. Likewise, we did not observe an association between asexual parasite or gametocyte prevalence and seroprevalence to either Pfs230 or Pfs48/45.
Substantial heterogeneity between studies persisted even after sub-group analysis by our pre-specified variables relating to malaria exposure, and so we also investigated the contribution of methodological variability to the observed heterogeneity. Studies differed in their source of antigen, choice of immunoassay, assay protocol and seropositivity cut-off determination. Early studies of NAI to Pfs230 and Pfs48/45 relied on whole antigen for the determination of immune responses owing to the difficulties in producing recombinant protein for analysis. Assays with whole antigen involve a two-site ELISA where an epitope-targeted monoclonal antibody is used to “capture” the target antigen for detection by antibodies present in the immune sera. Such assays, however, are reportedly less sensitive (19, 30) which could lower seroprevalence estimates. In our analysis, however, we did not observe an influence of antigen source on seroprevalence. We did, however, find that type of immunoassay influenced seroprevalence with seroprevalence measured using protein microarray reporting higher results than either indirect ELISA or two-site ELISA. This is likely due to the greater dynamic range afforded by microarray (53) that may allow better distinction between seropositive and seronegative individuals. We believe that the field will gradually move to high-throughput multiplex methods such as microarray or Luminex, although there will remain a place for ELISAs when large populations are examined for single-antigen responses.
We found that studies using a lower antigen coating concentration reported lower seroprevalence estimates. Seroprevalence to sexual stage antigens is lower than to asexual stage antigens (23, 54), possibly due to lower immunogenicity of these antigens or a higher ratio of circulating asexual parasites to gametocytes (33). For this reason, lower antigen coating concentration may reduce assay sensitivity, thus it is paramount that studies optimize antigen coating concentration and serum dilution combinations prior to measuring immune responses. Subsequent to measuring immune responses, studies need to clearly define seronegative and seropositive participants to estimate seroprevalence. Typically, this is done by defining cut-offs based on either two or three standard deviations (SD) of the mean antibody responses in malaria naïve individuals or in a statistically-defined population of low responders. We found that studies using a 3 SD cut-off reported lower seroprevalence, potentially a reflection of the higher stringency in comparison to a 2 SD cut-off.
What recommendations could be made? First, seropositive status should be assigned by comparison with a negative control group (i.e., a population with no malaria exposure). In practice, the most widely accessible means of doing this is using malaria naïve European or American sera as a reference. Second, given the variability of assays, we would recommend the use of more stringent cut-offs and therefore proposed the mean plus 3 SD of a malaria-naïve population to be used to determine seropositivity. Establishing a recognized and broadly accepted “gold-standard” for seropositivity estimates would allow a more robust comparison between seroepidemiological studies (55). The increased availability of recombinant protein with defined conformational properties for use in immunoassays makes the goal of standardized methodologies more attainable.
Heterogeneity was more evident in the studies reporting immune responses to Pfs230 than to studies analyzing Pfs48/45. We hesitate to ascribe this to biological differences between the two antigens, but rather to persisting heterogeneity in the studies that analyzed immune responses to Pfs230. Factors associated with seroprevalence estimates to the two antigens were largely methodological rather than epidemiological underscoring the importance of more standardized methods for seroepidemiological studies of sexual stage antigens. With the increasing number of assays performed for seroepidemiology studies, including but not limited to ELISA, protein microarrays, Luminex and AlphaScreen, there is need for minimum reporting parameters to ensure reproducibility, standardization, and to an extent generalizability of findings. For the analysis of Malaria Immunoepidemiology Observational Studies (MIOS), Fowkes et al. (56) have provided reporting standards. In addition to these standards, we propose that the following minimum methodological criteria be adopted in lieu of a gold standard: (1) use of recombinant proteins (with indications of the protein expression system utilized, protein region targeted—full length vs. fragments) and if gametocyte/gamete extract is used, a further analysis of responses to a dominant recombinant antigen (e.g., Pfs230 and/or Pfs48/45); (2) use of a 3 SD cut-off to assign seropositivity from a population of naïve controls; and (3) mention of antigen coating concentration and serum dilutions used.
Summary of Main Findings
In summary, from the studies analyzed, this systematic review shows that the range of reported seroprevalence to Pfs230 and Pfs48/45 varies widely across populations. Of the factors thought to influence seroprevalence, we found a case for age as an important determinant of seroprevalence. This is particularly important as the demonstration that functional TRI likely involves antigens other than Pfs230 and Pfs48/45 (18, 20, 21, 46), and with the identification of new TBV candidate antigens (20), criteria for identifying and prioritizing candidate antigens would be required. Screening for NAI and selecting antigens which show increased recognition with age could eliminate antigens that are simply markers of gametocyte exposure, thus prioritizing more important candidates for functional characterization. Though the association was not significant, we did observe modest evidence for increased prevalence of antibodies to Pfs230 and Pfs48/45 following the malaria transmission season. The potential for boosting of vaccine-induced immune responses during natural infection would enhance vaccine efficacy in the field (57). The extent and implications of this boosting are yet to be explored, presenting an interesting question for future studies. Heterogeneity between studies remained significant even after assessing study-specific and methodological variation thus limiting our ability to describe a clear picture of the dynamics of NAI to sexual stages.
Limitations
As parasite detection in all the studies was by microscopy, with few studies also reporting PCR prevalence, we were restricted to using microscopy-based parasite prevalence for our analysis. The insensitivity of microscopy for parasite detection (58, 59) would lead to an underestimation of parasite prevalence. Better estimates of parasite prevalence may offer novel insights into the association between parasite prevalence and seroprevalence to sexual stage antigens improving our understanding of the dynamics of NAI in relation to sexual stage immunity. Furthermore, few studies currently report antibody titer in addition to prevalence though titer has been shown to positively correlate with transmission-reducing activity (23, 60, 61). Information on antibody titer can help generate useful information on the impact of naturally acquired sexual-stage immunity on malaria transmission and this should be considered in future studies.
Another limitation was that our search revealed only a few studies looking at naturally acquired immunity to sexual stage antigens which could limit the generalizability of our results. Study designs were also varied between studies complicating attempts to pool the studies in a meta-analysis to arrive at an overall seroprevalence estimate. While we were able to identify potential sources of heterogeneity in a univariable analysis, we could not further test the combined significance of the associated variables.
Conclusions
The combined analysis presented here showed that antibody responses to sexual stage antigens are identified in most studies conducted, suggesting that immunity to Pfs230 and Pfs48/45 is acquired following exposure to malaria parasites. Variability in study design and methodological differences contributed to significant between-study heterogeneity that was not fully addressed by the variables we examined in this analysis. This highlights the importance of harmonized protocols for carrying out and reporting seroepidemiological studies to enable comparability across different settings. Agreed guidelines for reporting seroepidemiological studies would be valuable for sexual antigens and could be based on the comprehensive set of criteria, MIOS, proposed by Fowkes et al. (56).
Data Availability Statement
Publicly available datasets were analyzed in this study. This data can be found here: doi: 10.5061/dryad.8bp05, doi: 10.5061/dryad.v60jk42, doi: 10.1038/nature24059.
Author Contributions
MM, MK, and AB developed the protocol for the systematic review and meta-analysis. MM identified studies for review, carried out the data extraction, analyzed the data, and wrote the manuscript. AK carried out data extraction, assisted with the statistical analysis, and participated in drafting of the manuscript. TB assisted with data collection and participated in drafting of the manuscript. AB participated in design of the study and drafting the manuscript. PB participated in design of the study, assisted with the statistical analysis, and participated in drafting the manuscript. MK conceived of the study, participated in its design, identified articles for review, and participated in drafting the manuscript.
Funding
MM and AK are funded via IDeAL Ph.D. Studentships awarded by the KEMRI-Wellcome Trust Research Programme through funding from the DELTAS Africa programme via the Wellcome Trust (107769). TB was supported by a grant from The Netherlands Organization for Scientific Research (Vidi fellowship; NWO Project 016.158.306). AB was funded by the MRC (New Investigator Research Grant; award number MR/N00227X/1) and the University of Cambridge JRG. PB and MK were supported by a Wellcome Trust grant (107499).
Conflict of Interest
The authors declare that the research was conducted in the absence of any commercial or financial relationships that could be construed as a potential conflict of interest.
Acknowledgments
We sincerely thank Colin Sutherland, André-Lin Ouédraogo, Vincent Robert, Ulysse Ateba-Ngoa, Maria Yazdanbakhsh, Linda Amoah, Sophie Jones, Jeff Skinner, and Will Stone for providing information and/or data for this review. This work was supported through the DELTAS Africa Initiative [DEL-15-003]. The DELTAS Africa Initiative is an independent funding scheme of the African Academy of Sciences (AAS)'s Alliance for Accelerating Excellence in Science in Africa (AESA) and supported by the New Partnership for Africa's Development Planning and Coordinating Agency (NEPAD Agency) with funding from the Wellcome Trust and the UK government. The views expressed in this publication are those of the author(s) and not necessarily those of AAS, NEPAD Agency, Wellcome Trust, or the UK government. This paper is published with permission from the Director of KEMRI.
Supplementary Material
The Supplementary Material for this article can be found online at: https://www.frontiersin.org/articles/10.3389/fimmu.2019.02480/full#supplementary-material
References
1. Ghebreyesus TA, Admasu K. Countries must steer new response to turn the malaria tide. Lancet. (2018) 392:2246–7. doi: 10.1016/S0140-6736(18)32943-X
2. Weiss DJ, Lucas TCD, Nguyen M, Nandi AK, Bisanzio D, Battle KE, et al. Mapping the global prevalence, incidence, and mortality of Plasmodium falciparum, 2000-17: a spatial and temporal modelling study. Lancet. (2019) 394:322–31. doi: 10.1016/S0140-6736(19)31097-9
3. Blagborough AM, Churcher TS, Upton LM, Ghani AC, Gething PW, Sinden RE. Transmission-blocking interventions eliminate malaria from laboratory populations. Nat Commun. (2013) 4:1812–7. doi: 10.1038/ncomms2840
4. Doumbo OK, Niaré K, Healy SA, Sagara I, Duffy PE. Malaria transmission-blocking vaccines: present status and future perspectives. In: Manguin S, Dev V, editors. Towards Malaria Elimination - A Leap Forward. London: IntechOpen (2018). p. 363–85.
5. Huff CG, Marchbank DF, Shiroishi T. Changes in infectiousness of malarial gametocytes. II. Analysis of the possible causative factors. Exp Parasitol. (1958) 7:399–417. doi: 10.1016/0014-4894(58)90036-5
6. Gwadz RW. Malaria: successful immunization against the sexual stages of Plasmodium gallinaceum. Science. (1976) 193:1150–1. doi: 10.1126/science.959832
7. Carter R, Chen DH. Malaria transmission blocked by immunisation with gametes of the malaria parasite. Nature. (1976) 263:57–60. doi: 10.1038/263057a0
8. Rener J, Graves PM, Carter R, Williams JL, Burkot TR. Target antigens of transmission-blocking immunity on gametes of Plasmodium falciparum. J Exp Med. (1983) 158:976–81. doi: 10.1084/jem.158.3.976
9. Vermeulen AN, Ponnudurai T, Beckers PJ, Verhave JP, Smits MA, Meuwissen JH. Sequential expression of antigens on sexual stages of Plasmodium falciparum accessible to transmission-blocking antibodies in the mosquito. J Exp Med. (1985) 162:1460–76. doi: 10.1084/jem.162.5.1460
10. Eksi S, Czesny B, Van Gemert GJ, Sauerwein RW, Eling W, Williamson KC. Malaria transmission-blocking antigen, Pfs230, mediates human red blood cell binding to exflagellating male parasites and oocyst production. Mol Microbiol. (2006) 61:991–8. doi: 10.1111/j.1365-2958.2006.05284.x
11. Van Dijk MR, Janse CJ, Thompson J, Waters AP, Braks JAM, Dodemont HJ, et al. A central role for P48/45 in malaria parasite male gamete fertility. Cell. (2001) 104:153–64. doi: 10.1016/S0092-8674(01)00199-4
12. Kaslow DC, Quakyi IA, Syin C, Raum MG, Keister DB, Coligan JE, et al. A vaccine candidate from the sexual stage of human malaria that contains EGF-like domains. Nature. (1988) 333:74–6. doi: 10.1038/333074a0
13. Vermeulen AN, van Deursen J, Brakenhoff RH, Lensen THW, Ponnudurai T, Meuwissen JHET. Characterization of Plasmodium falciparum sexual stage antigens and their biosynthesis in synchronised gametocyte cultures. Mol Biochem Parasitol. (1986) 20:155–63. doi: 10.1016/0166-6851(86)90027-7
14. Kumar N, Carter R. Biosynthesis of the target antigens of antibodies blocking transmission of Plasmodium falciparum. Mol Biochem Parasitol. (1984) 13:333–42. doi: 10.1016/0166-6851(84)90124-5
15. Stone WJR, Dantzler KW, Nilsson SK, Drakeley CJ, Marti M, Bousema T, et al. Naturally acquired immunity to sexual stage P. falciparum parasites. Parasitology. (2016) 143:1–12. doi: 10.1017/S0031182015001341
16. Ouédraogo AL, Roeffen W, Luty AJF, de Vlas SJ, Nebie I, Ilboudo-Sanogo E, et al. Naturally acquired immune responses to Plasmodium falciparum sexual stage antigens Pfs48/45 and Pfs230 in an area of seasonal transmission. Infect Immun. (2011) 79:4957–64. doi: 10.1128/IAI.05288-11
17. Graves PM, Carter R, Burkot TR, Quakyi IA, Kumar N. Antibodies to Plasmodium falciparum gamete surface antigens in Papua New Guinea sera. Parasite Immunol. (1988) 10:209–18. doi: 10.1111/j.1365-3024.1988.tb00215.x
18. Drakeley CJ, Bousema JT, Akim NIJ, Teelen K, Roeffen W, Lensen AH, et al. Transmission-reducing immunity is inversely related to age in Plasmodium falciparum gametocyte carriers. Parasite Immunol. (2006) 28:185–90. doi: 10.1111/j.1365-3024.2005.00818.x
19. Jones S, Grignard L, Nebie I, Chilongola J, Dodoo D, Sauerwein R, et al. Naturally acquired antibody responses to recombinant Pfs230 and Pfs48/45 transmission blocking vaccine candidates. J Infect. (2015) 71:117–27. doi: 10.1016/j.jinf.2015.03.007
20. Stone WJR, Campo JJ, Ouédraogo AL, Meerstein-Kessel L, Morlais I, Da DF, et al. Unravelling the immune signature of Plasmodium falciparum transmission-reducing immunity. Nat Commun. (2018) 9:1–14. doi: 10.1038/s41467-018-03769-w
21. Healer J, McGuinness D, Carter R, Riley E. Transmission-blocking immunity to Plasmodium falciparum in malaria-immune individuals is associated with antibodies to the gamete surface protein Pfs230. Parasitology. (1999) 119:425–33. doi: 10.1017/S0031182099005041
22. Roeffen W, Mulder B, Teelen K, Bolmer M, Eling W, Targett GAT, et al. Association between anti-Pfs48/45 reactivity and P. falciparum transmission-blocking activity in sera from Cameroon. Parasite Immunol. (1996) 18:103–9. doi: 10.1046/j.1365-3024.1996.d01-54.x
23. Bousema T, Roeffen W, Meijerink H, Mwerinde H, Mwakalinga S, van Gemert G-J, et al. The dynamics of naturally acquired immune responses to Plasmodium falciparum sexual stage antigens Pfs230 and Pfs48/45 in a low endemic area in Tanzania. PLoS ONE. (2010) 5:e14114. doi: 10.1371/journal.pone.0014114
24. Oueadraogo AL, Eckhoff PA, Luty AJFF, Roeffen W, Sauerwein RW, Bousema T, et al. Modeling the impact of Plasmodium falciparum sexual stage immunity on the composition and dynamics of the human infectious reservoir for malaria in natural settings. PLoS Pathog. (2018) 14:e1007034. doi: 10.1371/journal.ppat.1007034
25. Carter R, Coulson A, Bhatti S, Taylor BJ, Elliott JF. Predicted disulfide-bonded structures for three uniquely related proteins of Plasmodium falciparum, Pfs230, Pfs4845 and Pf12. Mol Biochem Parasitol. (1995) 71:203–10. doi: 10.1016/0166-6851(94)00054-Q
26. Gerloff DL, Creasey A, Maslau S, Carter R. Structural models for the protein family characterized by gamete surface protein Pfs230 of Plasmodium falciparum. Proc Natl Acad Sci USA. (2005) 102:13598–603. doi: 10.1073/pnas.0502378102
27. Bustamante PJ, Woodruff DC, Oh J, Keister DB, Muratova O, Williamson KC. Differential ability of specific regions of Plasmodium falciparum sexual-stage antigen, Pfs230, to induce malaria transmission-blocking immunity. Parasite Immunol. (2000) 22:373–80. doi: 10.1046/j.1365-3024.2000.00315.x
28. Outchkourov N, Vermunt A, Jansen J, Kaan A, Roeffen W, Teelen K, et al. Epitope analysis of the malaria surface antigen Pfs48/45 identifies a subdomain that elicits transmission blocking antibodies. J Biol Chem. (2007) 282:17148–56. doi: 10.1074/jbc.M700948200
29. Tachibana M, Wu Y, Iriko H, Muratova O, Macdonald NJ, Sattabongkot J, et al. N-terminal prodomain of Pfs230 synthesized using a cell-free system is sufficient to induce complement-dependent malaria transmission-blocking activity. Clin Vaccine Immunol. (2011) 18:1343–50. doi: 10.1128/CVI.05104-11
30. Acquah FK, Obboh EK, Asare K, Boampong JN, Nuvor SV, Singh SK, et al. Antibody responses to two new Lactococcus lactis-produced recombinant Pfs48/45 and Pfs230 proteins increase with age in malaria patients living in the Central Region of Ghana. Malar J. (2017) 16:1–11. doi: 10.1186/s12936-017-1955-0
31. Outchkourov NS, Roeffen W, Kaan A, Jansen J, Luty A, Schuiffel D, et al. Correctly folded Pfs48/45 protein of Plasmodium falciparum elicits malaria transmission-blocking immunity in mice. Proc Natl Acad Sci USA. (2008) 105:4301–5. doi: 10.1073/pnas.0800459105
32. Farrance CE, Rhee A, Jones RM, Musiychuk K, Shamloul M, Sharma S, et al. A plant-produced Pfs230 vaccine candidate blocks transmission of Plasmodium falciparum. Clin Vaccine Immunol. (2011) 18:1351–7. doi: 10.1128/CVI.05105-11
33. Skinner J, Huang CY, Waisberg M, Felgner PL, Doumbo OK, Ongoib A, et al. Plasmodium falciparum gametocyte-specific antibody profiling reveals boosting through natural infection and identifies potential markers of gametocyte exposure. Infect Immun. (2015) 83:4229–36. doi: 10.1128/IAI.00644-15
34. Amoah LE, Acquah FK, Ayanful-Torgby R, Oppong A, Abankwa J, Obboh EK, et al. Dynamics of anti-MSP3 and Pfs230 antibody responses and multiplicity of infection in asymptomatic children from southern Ghana. Parasit Vectors. (2018) 11:5–13. doi: 10.1186/s13071-017-2607-5
35. Lamptey H, Ofori MF, Kusi KA, Adu B, Owusu-Yeboa E, Kyei-Baafour E, et al. The prevalence of submicroscopic Plasmodium falciparum gametocyte carriage and multiplicity of infection in children, pregnant women and adults in a low malaria transmission area in Southern Ghana. Malar J. (2018) 17:331. doi: 10.1186/s12936-018-2479-y
36. Bousema JT, Drakeley CJ, Kihonda J, Hendriks JCM, Akim NIJ, Roeffen W, et al. A longitudinal study of immune responses to Plasmodium falciparum sexual stage antigens in Tanzanian adults. Parasite Immunol. (2007) 29:309–17. doi: 10.1111/j.1365-3024.2007.00948.x
37. Stroup DF, Berlin JA, Morton SC, Olkin I, Williamson GD, Rennie D, et al. Meta-analysis of observational studies in epidemiology: a proposal for reporting. JAMA. (2000) 283:2008–12. doi: 10.1001/jama.283.15.2008
38. Shamseer L, Moher D, Clarke M, Ghersi D, Liberati A, Petticrew M, et al. Preferred reporting items for systematic review and meta-analysis protocols (PRISMA-P) 2015: elaboration and explanation. BMJ. (2015) 350:g7647. doi: 10.1136/bmj.g7647
39. Benjamini Y, Hochberg Y. Controlling the false discovery rate: a practical and powerful approach to multiple testing. J R Stat Soc Ser B. (1995) 57:289–300. doi: 10.1111/j.2517-6161.1995.tb02031.x
40. Snow RW, Sartorius B, Kyalo D, Maina J, Amratia P, Mundia CW, et al. The prevalence of Plasmodium falciparum in sub-Saharan Africa since 1900. Nature. (2017) 550:515–8. doi: 10.1038/nature24059
41. Noor AM, Kinyoki DK, Mundia CW, Kabaria CW, Mutua JW, Alegana VA, et al. The changing risk of Plasmodium falciparum malaria infection in Africa: 2000-10: a spatial and temporal analysis of transmission intensity. Lancet. (2014) 383:1739–47. doi: 10.1016/S0140-6736(13)62566-0
42. Bansal GP, Vengesai A, Cao Y, Mduluza T, Kumar N. Antibodies elicited during natural infection in a predominantly Plasmodium falciparum transmission area cross-react with sexual stage-specific antigen in P. vivax. Acta Trop. (2017) 170:105–11. doi: 10.1016/j.actatropica.2017.02.032
43. Paul NH, Vengesai A, Mduluza T, Chipeta J, Midzi N, Bansal GP, et al. Prevalence of Plasmodium falciparum transmission reducing immunity among primary school children in a malaria moderate transmission region in Zimbabwe. Acta Trop. (2016) 163:103–8. doi: 10.1016/j.actatropica.2016.07.023
44. Ateba-Ngoa U, Jones S, Zinsou JF, Honkpehedji J, Adegnika AA, Agobe JCD, et al. Associations between helminth infections, Plasmodium falciparum parasite carriage and antibody responses to sexual and asexual stage malarial antigens. Am J Trop Med Hyg. (2016) 95:394–400. doi: 10.4269/ajtmh.15-0703
45. Miura K, Takashima E, Deng B, Tullo G, Diouf A, Moretz SE, et al. Functional comparison of Plasmodium falciparum transmission-blocking vaccine candidates by the standard membrane-feeding assay. Infect Immun. (2013) 81:4377–82. doi: 10.1128/IAI.01056-13
46. van der Kolk M, de Vlas SJ, Sauerwein RW. Reduction and enhancement of Plasmodium falciparum transmission by endemic human sera. Int J Parasitol. (2006) 36:1091–5. doi: 10.1016/j.ijpara.2006.05.004
47. Riley EM, Bennet S, Jepson A, Hassan-King M, Whittle H, Olerup O, Carter R. Human antibody responses to Pfs 230, a sexual stage-specific surface antigen of Plasmodium falciparum: non-responsiveness is a stable phenotype but does not appear to be genetically regulated. Parasite Immunol. (1994) 16:55–62. doi: 10.1111/j.1365-3024.1994.tb00323.x
48. Osier FHA, Polley SD, Mwangi T, Lowe B, Conway DJ, Marsh K. Naturally acquired antibodies to polymorphic and conserved epitopes of Plasmodium falciparum merozoite surface protein 3. Parasite Immunol. (2007) 29:387–94. doi: 10.1111/j.1365-3024.2007.00951.x
49. Weiss GE, Traore B, Kayentao K, Ongoiba A, Doumbo S, Doumtabe D, et al. The Plasmodium falciparum-specific human memory B cell compartment expands gradually with repeated malaria infections. PLoS Pathog. (2010) 6:e1000912. doi: 10.1371/journal.ppat.1000912
50. Barry AE, Trieu A, Fowkes FJI, Pablo J, Kalantari-Dehaghi M, Jasinskas A, et al. The stability and complexity of antibody responses to the major surface antigen of Plasmodium falciparum are associated with age in a malaria endemic area. Mol Cell Proteomics. (2011) 10:1–13. doi: 10.1074/mcp.M111.008326
51. Crompton PD, Kayala MA, Traore B, Kayentao K, Ongoiba A, Weiss GE, et al. A prospective analysis of the Ab response to Plasmodium falciparum before and after a malaria season by protein microarray. Proc Natl Acad Sci USA. (2010) 107:6958–63. doi: 10.1073/pnas.1001323107
52. Mendis KN, Carter R. Transmission blocking immunity may provide clues that antimalarial immunity is largely T-independent. Res Immunol. (1991) 142:687–90. doi: 10.1016/0923-2494(91)90150-H
53. Selvarajah S, Negm OH, Hamed MR, Tubby C, Todd I, Tighe PJ, et al. Development and validation of protein microarray technology for simultaneous inflammatory mediator detection in human sera. Mediators Inflamm. (2014) 2014:1–12. doi: 10.1155/2014/820304
54. Ouédraogo AL, Gonçalves BP, Gnémé A, Wenger EA, Guelbeogo MW, Ouédraogo A, et al. Dynamics of the human infectious reservoir for malaria determined by mosquito feeding assays and ultrasensitive malaria diagnosis in Burkina Faso. J Infect Dis. 213:90–9. doi: 10.1093/infdis/jiv370
55. Rogier E, Wiegand R, Moss D, Priest J, Angov E, Dutta S, et al. Multiple comparisons analysis of serological data from an area of low Plasmodium falciparum transmission. Malar J. (2015) 14:1–12. doi: 10.1186/s12936-015-0955-1
56. Fowkes FJI, Richards JS, Simpson JA, Beeson JG. The relationship between anti-merozoite antibodies and incidence of Plasmodium falciparum malaria: A systematic review and meta-analysis. PLoS Med. (2010) 7:e1000218. doi: 10.1371/journal.pmed.1000218
57. Nikolaeva D, Illingworth JJ, Miura K, Alanine DG, Brian IJ, Li Y, et al. Functional characterization and comparison of Plasmodium falciparum proteins as targets of transmission-blocking antibodies. Mol Cell Proteomics. (2017). doi: 10.1074/mcp.RA117.000036. [Epub ahead of print].
58. Okell LC, Ghani AC, Lyons E, Drakeley CJ. Submicroscopic infection in Plasmodium falciparum –endemic populations: a systematic review and meta-analysis. J Infect Dis. (2009) 200:1509–17. doi: 10.1086/644781
59. Gonçalves D, Hunziker P. Transmission-blocking strategies: the roadmap from laboratory bench to the community. Malar J. (2016) 15:95. doi: 10.1186/s12936-016-1163-3
60. Miura K, Keister DB, Muratova OV, Sattabongkot J, Long CA, Saul A. Transmission-blocking activity induced by malaria vaccine candidates Pfs25/Pvs25 is a direct and predictable function of antibody titer. Malar J. (2007) 6:107. doi: 10.1186/1475-2875-6-107
Keywords: immunity, Plasmodium falciparum, gametocytes, Pfs230, Pfs48/45
Citation: Muthui MK, Kamau A, Bousema T, Blagborough AM, Bejon P and Kapulu MC (2019) Immune Responses to Gametocyte Antigens in a Malaria Endemic Population—The African falciparum Context: A Systematic Review and Meta-Analysis. Front. Immunol. 10:2480. doi: 10.3389/fimmu.2019.02480
Received: 28 June 2019; Accepted: 04 October 2019;
Published: 22 October 2019.
Edited by:
Jude Ezeh Uzonna, University of Manitoba, CanadaReviewed by:
V. Ann Stewart, Uniformed Services University of the Health Sciences, United StatesJulius Clemence Hafalla, London School of Hygiene and Tropical Medicine, United Kingdom
Copyright © 2019 Muthui, Kamau, Bousema, Blagborough, Bejon and Kapulu. This is an open-access article distributed under the terms of the Creative Commons Attribution License (CC BY). The use, distribution or reproduction in other forums is permitted, provided the original author(s) and the copyright owner(s) are credited and that the original publication in this journal is cited, in accordance with accepted academic practice. No use, distribution or reproduction is permitted which does not comply with these terms.
*Correspondence: Michelle K. Muthui, bW11dGh1aUBrZW1yaS13ZWxsY29tZS5vcmc=