- 1Matrix Biology Program, Benaroya Research Institute at Virginia Mason, Seattle, WA, United States
- 2Division of Pulmonary/Critical Care Medicine, Center for Lung Biology, University of Washington School of Medicine, Seattle, WA, United States
- 3Centre for the Tumour Microenvironment, Barts Cancer Institute, Queen Mary University of London, London, United Kingdom
The extracellular matrix (ECM) proteoglycan, versican increases along with other ECM versican binding molecules such as hyaluronan, tumor necrosis factor stimulated gene-6 (TSG-6), and inter alpha trypsin inhibitor (IαI) during inflammation in a number of different diseases such as cardiovascular and lung disease, autoimmune diseases, and several different cancers. These interactions form stable scaffolds which can act as “landing strips” for inflammatory cells as they invade tissue from the circulation. The increase in versican is often coincident with the invasion of leukocytes early in the inflammatory process. Versican interacts with inflammatory cells either indirectly via hyaluronan or directly via receptors such as CD44, P-selectin glycoprotein ligand-1 (PSGL-1), and toll-like receptors (TLRs) present on the surface of immune and non-immune cells. These interactions activate signaling pathways that promote the synthesis and secretion of inflammatory cytokines such as TNFα, IL-6, and NFκB. Versican also influences inflammation by interacting with a variety of growth factors and cytokines involved in regulating inflammation thereby influencing their bioavailability and bioactivity. Versican is produced by multiple cell types involved in the inflammatory process. Conditional total knockout of versican in a mouse model of lung inflammation demonstrated significant reduction in leukocyte invasion into the lung and reduced inflammatory cytokine expression. While versican produced by stromal cells tends to be pro-inflammatory, versican expressed by myeloid cells can create anti-inflammatory and immunosuppressive microenvironments. Inflammation in the tumor microenvironment often contains elevated levels of versican. Perturbing the accumulation of versican in tumors can inhibit inflammation and tumor progression in some cancers. Thus versican, as a component of the ECM impacts immunity and inflammation through regulating immune cell trafficking and activation. Versican is emerging as a potential target in the control of inflammation in a number of different diseases.
Introduction
Inflammation occurs during tissue infection or injury and involves the migration of leukocytes out of the blood vessels and into damaged areas of tissue (1, 2). Inflammation is driven by receptors on the surface of immune and non-immune cells (pattern recognition receptors, PRR) (1). PRRs recognize three classes of molecular patterns resulting from either pathogens generated by infectious agents such as virus and bacteria (pathogen-associated molecular patterns or PAMPs), molecules released from damaged tissues (damage-associated molecular patterns or DAMPs), or from molecular patterns on “self” tissues, often upregulated during malignancy (self-associated molecular patterns, SAMPs) (3). Activation of these receptors initiates an inflammatory response involving inflammatory cytokine production and recruitment of leukocytes. While inflammation is important in repairing tissue after insult, it often results in an exacerbation of tissue injury and promotion of disease.
Recent studies have indicated an important role for the ECM in the inflammatory response (4–10). Leukocytes cross the endothelial barrier and interact with the ECM which influences their adhesion, retention, migration, and activation. Leukocyte trafficking and localization are critical to events associated with the immune response. Specific components of the ECM can act as DAMPs or matrikines (11) promoting inflammatory cytokine synthesis and release by immune and non-immune cells (4, 12, 13). Proteoglycans, as components of the ECM, play a key part in providing intrinsic signals needed to coordinate critical events in the inflammatory cascade (4–6, 10, 14–23).
We have been interested in versican, which is a chondroitin sulfate proteoglycan (CSPG) and a member of the hyalectin family of ECM components (24), as one of the principal drivers of immunity and inflammation in a variety of different diseases, such as cardiovascular and lung disease, autoimmune diseases, and several different cancers. Interestingly, like many other ECM components, versican has “two faces”—functioning both in a pro- and an anti-inflammatory manner. The goal of this review will be to highlight the involvement of versican as a component in inflammation, discuss its role in recruiting and activating leukocytes, and provide examples and possible mechanisms by which this “versatile” ECM molecule can exhibit both pro- and anti-inflammatory properties.
Versican
The expression and accumulation of versican, a large ECM proteoglycan, increases dramatically during inflammation in most diseases [reviewed in (4–6, 10, 23, 25, 26)]. Versican, named for its versatility in being a highly interactive molecule (27), is encoded from a single gene locus on chromosome 5q14.3 in humans (28) and its full-length isoform shares 76% nucleotide and 62% amino acid sequence identity between mouse and human.
There are at least five different isoforms of versican, V0, V1, V2, V3, V4, due to the alternative splicing of the major exons that code for the attachment regions for the chondroitin sulfate (CS) glycosaminoglycans (GAG) in the core protein (27, 29–33). Four of these isoforms contain CS GAGs that are attached by covalent linkage to the core protein, while one of the isoforms, V3, contains no GAGs due to the splicing together of the N- and C- terminal regions. V0, V1, V2, and V3 differ in the size of the core proteins and the size and number of the GAG chains. Additional variation within these isoforms has also been observed with an alternatively spliced C-terminus “Vint tail” (33).
The calculated molecular masses from cDNA sequencing studies for human versican core proteins are 370 kDa for V0, 262 kDa for V1, and 72 kDa for V3. These theoretical values are significantly lower than deduced from SDS PAGE gels where V0 migrates at around 550 kDa, and V1 around 500 kDa after chondroitin ABC lyase digestion (34). These differences are due to the high content of O- and N-linked oligosaccharides associated with the versican core protein (34–36). Interestingly, the different isoforms exhibit functional differences regarding their impact on cell phenotype, such as the ability of V1 to promote proliferation and inhibit apoptosis, while V2 exhibits antiproliferative activity (37, 38). In contrast, V3 regulates ECM assembly and inhibits cell proliferation and migration (39–45). A new V5 isoform has been recently described and shown to be expressed by injured rat neurons (46). While the biological importance of the “Vint tail” has yet to be elucidated, it likely provides fine tuning of interactions with the G3 region of the molecule. Thus, the different molecular domains of versican make the biology of this molecule quite complex given that both the alternative splicing of the mRNA as well as the controlled degradation of the intact versican molecule generate many different molecular forms which themselves can have their own effects on key events associated with immunity and inflammation. Much work remains to be done to sort out these complex interactions and their effects on the biology of this highly interactive molecule.
There is variation in the number, size, and composition of the CS chains attached to the different isoforms of versican. For example, consensus sequence for the number of CS attachment sites on the core protein of human versican reveals 17–23 sites for V0, 12–15 for V1, 5–8 for V2, and 0 for V3 (36, 47). Growth factors that increase during inflammation such as platelet-derived growth factor (PDGF) and transforming growth factor-beta (TGFβ) increase CS chains length and alter CS composition, impacting the ability of versican to interact with other molecules (35, 48–50). The CS isolated from versican interacts with inflammatory cytokines and impacts cytokine activity (51) [also see reviews (52, 53)]. Interestingly, the machinery and signaling pathways that control the composition and elongation of the CS chain differ from those controlling the transcription of versican core protein synthesis (48). For example, we found that stimulation of versican core protein synthesis by PDGF in non-human primate arterial smooth muscle cells encoded both the PKC and ERK pathways, whereas the elongation of the CS chains attached to the versican core protein was PKC dependent, but ERK independent. Since GAG chain length is a critical factor in determining the interactive nature of versican as well as other proteoglycans (54), targeting the pathways that regulate GAG chain elongation (55) may be a useful therapeutic approach to alter the immune and inflammatory properties of this ECM component.
Versican is synthesized by many different types of cells, including epithelial, endothelial, and stromal cells as well as leukocytes. This synthesis is regulated by a host of proinflammatory cytokines and growth factors [reviewed in (26, 56)]. Versican expression is regulated through two signaling pathways. Rhamani et al. first described the role of the canonical Wnt/β-catenin/T-cell factor (TCF) pathway in regulating versican expression in airway smooth muscle cells (57–59). Using polyinosinic-polycytidylic acid (poly I:C) and lipopolysaccharide (LPS) to activate TLR3 and TLR4, respectively, we showed that the signaling cascade that includes TLR3 or TLR4, the TLR adaptor molecule, Trif, type I interferons (IFNs), and the type I IFN receptor (IFNAR1), increases versican expression by mouse macrophages, which implicates versican as an IFN-stimulated gene (60). Versican synthesis is also controlled by several different miRNAs which are modulated during inflammation (61–63).
The degradation of versican is affected by several different families of proteases that increase during inflammation. Such proteases include matrix metalloproteinases (MMPs), i.e., MMP-1,−2,−3,−7, and−9 (64–66), serine protease plasmin (67), and at least five ADAMTS (a disintegrin and metalloproteinase with thrombospondin motifs) MMPs, specifically ADAMTS-1,−4,−5,−9, and−20 [see reviews (12, 13, 68–70)]. Cleavage of versican by ADAMTS-1,−4,−5, and−9 leads to production of an amino-terminal fragment, termed versikine, that can be detected using an antibody recognizing the neoepitope sequence DPEAAE (DPE) (12, 71, 72). Fragments such as versikine can act as DAMPs, interacting with immune and non-immune cells stimulating pro- and anti-inflammatory cytokine release and a modified immune response in both human and murine models (13, 73–75). Thus, many mechanisms are in place to regulate the expression and degradation of versican during the inflammatory process which ultimately influence key cellular events including cell adhesion, proliferation, migration, and ECM remodeling.
Versican: A Component of the Inflammatory Response
Versican is essential during development (76, 77) and it is now becoming apparent that it is an important component of the tissue inflammation caused by infection and tissue injury (10). Versican accumulates as part of the early inflammatory response in a number of human diseases often associated with the invasion of leukocytes including those in the vascular system (10, 40, 78–84), lung (5, 6, 60, 77, 85–89), brain and spinal cord (53, 90–92), intestine (93–96), heart (97), liver (63), skin (98, 99), eye (100, 101), pancreatic islets (102), and many different forms of cancer [reviewed in (103–105)]. The accumulation of versican in these tissues is usually associated with other ECM components that bind versican, such as hyaluronan (106, 107), link protein, TSG-6, IαI, and CD44 (95, 96, 108–111) (Figure 1). Complexes form as a result of this interaction which shape the microenvironment which impacts immunity and inflammation (95, 96, 109–113). Versican is usually found co-localized with hyaluronan, however, inflammatory situations exist where each can be found separately (114, 115). Like versican, hyaluronan is also well-known for having both pro- and anti-inflammatory properties [reviewed in (95, 96, 111, 113, 116, 117)]. It remains to be determined whether the respective pro- or anti-inflammatory activities of versican and hyaluronan are interdependent. Usually, when interventions are used to reduce either versican or hyaluronan in cells and tissues, both components are similarly affected, adding to the challenge of determining the independent contributions of each molecule in the inflammatory process.
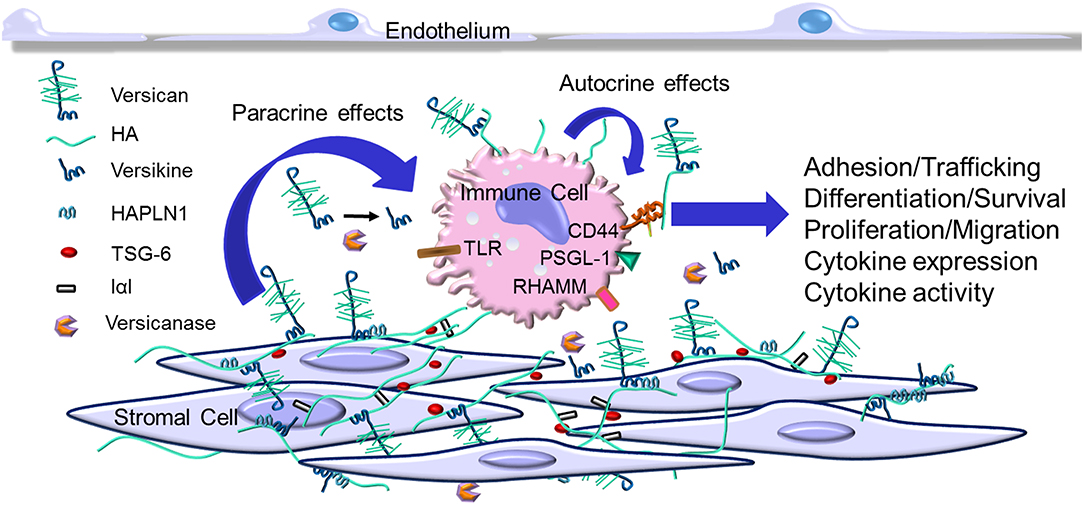
Figure 1. Versican increases in the extracellular matrix (ECM) as part of the early inflammatory response. Immune cells invade the tissue from the blood stream and interact with specific ECM components including versican, versican fragments such as versikine (generated by versicanases), and other ECM components that associate with versican. This interaction may involve receptors on the immune cells such as CD44, P-selectin glycoprotein 1 (PSGL-1), receptor for hyaluronan-mediated motility (RHAMM), and toll-like receptors (TLRs). This interaction impacts several aspects of immune cell activation as part of the immune and inflammatory response.
Versican interacts with receptors, such as CD44, PSGL-1, TLR2 (118), found on the surface of immune cells, and P- or L-selectins (20, 51, 52, 119–123). This interaction initiates a signaling cascade that influences the phenotypes of immune and inflammatory cells. For the TLRs, versican, like hyaluronan, does not possess the biochemical homology common to ligands for TLR2 and TLR4, such as LPS, so the precise interaction of versican with the TLRs is a bit unclear. No doubt interaction with the TLRs for versican, and maybe hyaluronan as well, involves a complex with molecules that have an affinity for the TLRs (113). In addition, it is interesting to compare the consequences of postulated versican–TLR interactions to other proteoglycans that have peptide motifs that bind TLRs, such as biglycan (18, 124). Whereas biglycan impacts inflammation by activating TLR4 and inflammasomes through activation of Trif-dependent signaling pathways (4, 6, 14, 19, 125), versican appears to mediate TLR2 interaction and activate MyD88-dependent signaling (118, 119). The functional significance of these two separate pathways in governing the inflammatory response is not clear. It will be important to determine if versican has unique immunogenic properties when compared to other ECM molecules.
The negatively charged CS chains in large part control the ability of versican to interact with a multitude of other molecules including chemokines, growth factors and proteases (51, 52, 122, 126–128) impacting their bioavailability and bioactivity (129–131). A number of studies demonstrate that CS chains can also promote the release of proinflammatory cytokines from macrophages and splenocytes, regulate MHCII intracellular trafficking, antigen presentation and T-cell activation [reviewed in (53)].
While a majority of studies show versican as having proinflammatory properties (see below), some studies have indicated that versican can operate in an immunosuppressive manner under certain conditions. For example, Xu et al. (132) found that treatment of mice exposed to LPS with siRNA to versican V1 resulted in increases in TNFα, NFκB, and TLR2 which were accompanied by increases in leukocytes in the lung. Interestingly, we found that LPS stimulated the expression of versican by macrophages in a type I IFN-dependent manner and that deletion of versican from macrophages promoted increased leukocyte invasion in mice exposed to poly I:C (60, 87). These studies are important since they suggest that macrophage-derived versican may possess immunosuppressive characteristics. Additionally, Coulson-Thomas and colleagues showed that the surface of human umbilical cord mesenchymal stem cells contains a glycocalyx enriched in hyaluronan, versican, TSG-6, and IαI, protecting the stem cells from immunodestruction (133) and affecting T-cell and macrophage phenotype. Interestingly, in tumors, human cancer stem cells synthesize and secrete prominent pericellular coat matrices enriched in hyaluronan and versican (134). Such a “cell coat” may account, in part, for the resistance to chemotherapy that these cells exhibit, as well as protection from immune surveillance as has been described for hyaluronan (135, 136) (see below).
Versican: Interplay With Leukocytes
Leucocytes interact with the ECM as they invade tissue as part of the inflammatory response (5, 7–9, 17, 21) (see Figure 1). To explore this interaction, Carol de la Motte's laboratory at the Cleveland Clinic pioneered the use of co-cultures of leukocytes with stromal cells to explore the mechanisms and consequences responsible for the interaction of leukocytes with the ECM. Using agonists that promote ER stress, these models demonstrated that stromal cells produce an ECM organized into cable like structures that bound leukocytes [see reviews (96, 116, 137)]. These cable-like structures contain hyaluronan, versican, TSG-6, and IαI that bind different subsets of leukocytes (93, 94, 112, 114–116, 137–147). Such structures have not only been found in vitro, but also in diseased tissues, such as in atherosclerosis (10, 84), inflammatory bowel disease (IBD) (94), and in brain and spinal cord injury (53, 91). Further studies are needed to determine the contribution of each of the components in the complex on leukocyte phenotype. For example, we found that blocking versican accumulation in the ECM generated by cultured human lung fibroblasts using a neutralizing antibody significantly reduced monocyte adhesion in vitro (115). In addition, we showed that manipulating the expression of versican by overexpressing the V3 isoform in experimentally-induced atherosclerotic lesions in rabbits significantly reduced macrophage infiltration, inhibiting the development of lipid-filled atherosclerotic lesions (84). Furthermore, our in vitro studies using rat arterial smooth muscle cells demonstrated that the V3 expression effect is anti-inflammatory and decreased the expression of the CS-containing versican isoforms, V0 and V1, and the formation of elastic fibers which are a poor substrate for macrophages (42, 43). Such results further support a critical role for versican in myeloid cell accumulation in atherosclerosis and perhaps in other diseases as well (39).
Lymphoid cells also interact with the ECM. An effective immune response requires that T cells are able to adhere to and migrate through the ECM (7, 145). For example, activated human CD4+ T cells bind to the ECM generated by human synoviocytes and human lung fibroblasts treated with poly I:C, but not to the ECM generated by the stromal cells in the absence of poly I:C treatment (145). This binding blocked the ability of T cells to spread and migrate and was reversed by pretreatment of the ECM with chondroitin ABC lyase. Additionally, versican blocked hyaluronan binding to T cells and inhibited IL-10 synthesis reducing the immunosuppressive capacity of these cells (145). Versican also inhibited human T-cell invasion of collagen gels consistent with influencing T-cell migration and immunosuppression. These activities, of course, could be critical to influencing the ability of T cells to invade and destroy tumor cells (see below). In addition, versican was identified as one of the most upregulated genes in lymphocytes isolated from patients with Sezary syndrome which is a leukemic variant of cutaneous T cell lymphoma. In this setting, versican promoted the invasive and homing capacity of the lymphocytes from these patients (148). Interestingly, the cleavage of versican by ADAMTS enzymes is critical for T-cell trafficking in mouse models of influenza virus infection (149).
Whether versican is intact or degraded may affect its impact on disease cell phenotype. For example, we showed that the G1 fragment of versican promoted extensive ECM cable formation that interconnected adjacent cells and inhibited proliferation, while the intact form had no activity (150). Using the CRISPR/Cas9 system, Hideto Watanabe's group recently developed mice that synthesized versican lacking the ADAMTS versicanase cleavage site (99). They found that accumulation of intact versican in these mice facilitated TGFβ activity coupled to fibroblast proliferation and myofibroblast differentiation and accelerated wound healing. Interestingly, wounds from the versican cleavage-resistant mice contained elevated levels of M1 macrophages and T cells, suggesting in this context that intact versican enhances inflammatory cell infiltration. These studies highlight the importance of ECM molecules such as versican in regulating the ability of both myeloid cells and T cells to invade tissue which is critical to the immune destruction of tumors in many forms of cancer (see below).
Versican: Expression by Myeloid Cells and the Impact on Inflammation
Versican is also expressed by myeloid cells and upregulated as part of the inflammatory response (see Figure 1). For example, versican expression is elevated by myeloid cells in autoimmunity (127, 151, 152), coronary stenosis (153), myocardial infarction (154), and in response to proinflammatory stimulants such as hypoxia (155, 156) and LPS (60, 87, 157). Versican is differentially expressed in M1 macrophages, as opposed to M2 macrophages, as they differentiate from monocytes (87, 156, 158). On the other hand, a recent study shows that versican enhances mesothelioma growth by promoting M2 polarization and inhibiting phagocytosis (159). More work is needed to sort out whether specific isoforms of versican play a role in determining and/or regulating macrophage phenotype. In patients with systemic sclerosis, CD14-positive monocytes (127) show elevated expression of versican that is accompanied by elevated expression of CCL2 [also known as Monocyte Chemoattractant Protein 1 (MCP-1)]. Interestingly, versican protects CCL2 from degradation which in turn promotes monocyte migration (127). Earlier studies showed that in a model of neuronal inflammation hyperalgesia, CCL2 binds to versican and impacts inflammation (160). A similar relationship has been seen among versican, macrophages, and CCL2 and the promotion of inflammation in mouse models of cancer (161, 162) (see below). Versican also interacts with CCL5 which is important in recruiting CD8 T cells in the inflammatory response (51). Versican has also been identified as a gene common for classical monocytes (CD14++ CD16−) and classical CD11c dendritic cells (163).
LPS and poly I:C, two TLR agonists, stimulate versican expression in both murine bone marrow-derived macrophages and alveolar macrophages in vitro (87) and in murine alveolar macrophages as well as in stromal cells in vivo (60, 88). To determine the role of versican derived from macrophages in the innate immune response in vivo, we developed two models of conditional versican deficiency by floxing exon 4 of the versican gene. LysM/Vcan−/− mice have constitutive myeloid cell-specific versican deficiency (60), and R26Rert2Cre+/Vcan−/− mice, when treated with tamoxifen, are globally deficient in versican (88). In vitro studies with macrophages from LysM/Vcan−/− mice indicate that versican is important for production of type I IFNs and IL-10 by macrophages in response to poly I:C. This is supported by in vivo studies with LysM/Vcan−/− mice which indicate that myeloid-derived versican restrains recruitment of inflammatory cells into lungs and promotes production of the key anti-inflammatory cytokines, IFN-β and IL-10, in the pulmonary response to poly I:C. In contrast, in vivo studies with R26Rert2Cre+/Vcan−/− mice suggest that stromal-derived versican promotes pulmonary inflammatory cell recruitment in response to poly I:C. When considered in tandem, these results identify versican derived from macrophages as an immunomodulatory molecule with anti-inflammatory properties whereas versican derived from stromal cells has proinflammatory properties. Some of the factors that determine the pro- or anti-inflammatory properties of versican include cellular source, surface receptors, signaling pathways affected, nature of the binding partners that associate with versican and/or whether versican is intact or degraded. It should also be noted that reducing versican by genetic manipulation or other means frequently reduces the accumulation of hyaluronan (88) raising some question as to whether the opposing inflammatory properties of versican may be governed by hyaluronan?
Versican and Inflammation in Cancer: Pro- and/or Anti-Inflammatory?
Versican is a central player in cancer development in that it impacts tumor-promoting inflammation, immune surveillance evasion, and immunomodulation (164). Versican expression increases as part of the inflammatory response in a number of cancers [reviewed in (22, 103, 105, 165)]. In both breast cancer and Lewis lung carcinoma, the presence of versican produced by the tumor cells leads to an accumulation and activation of tumor-associated macrophages (TAMs) via TLR2 and its co-receptors TLR6 and CD14 (118, 119, 159, 165–167). Tumor cell-derived versican in turn promotes accumulation and secretion of proinflammatory TNFα and IL-6. In addition, we found significantly elevated expression and accumulation of versican in leiomyosarcoma (LMS), a metastatic uterine cancer, when compared to the more benign leiomyomas and control healthy tissue (103, 168). Importantly, cultured human LMS cells synthesized large quantities of versican, forming extensive pericellular coats around the tumor cells. Blocking versican synthesis with siRNA to versican reduced the thickness of the cell coats and inhibited their proliferation and migration in vitro and tumor formation in vivo (103, 168).
Stromal cells are a major source of versican as well. In breast and ovarian cancer, TGFβ is overexpressed and contributes to strong stromal versican upregulation (169, 170). Cervical and endometrial cancers are characterized by increases in versican originating from both tumor and stromal cells (171, 172). On the other hand, lack of versican expression in a mouse fibrosarcoma model resulted in a decrease in the number and density of cancer-associated fibroblasts (CAFs) in stroma (173). Such changes led to larger tumors and poorer prognosis in pancreatic cancer (174). In Lewis lung carcinoma, stromal cell-derived versican and its fragment, versikine are associated with increased angiogenesis as part of the inflammatory response contributing to this tumor (175). Accumulation of versican in tumors is positively correlated with the number of microvessels within tumor stroma (176, 177). We found, for example, that human stromal stem cells that produce elevated levels of versican formed an extensive vascular network enriched in hyaluronan and versican when cultured with vascular endothelial cells (178). Furthermore, when patches containing these proangiogenic cells were transplanted onto athymic rat hearts they developed 50-fold more vessels than patches containing stromal cells with low versican expression (178). In other studies, we demonstrated that versican was actively processed in the early stages of VEGF-induced pathological angiogenesis generating extensive DPEAAE (versikine) fragments that associated with the endothelial cells (179). These results suggest that versican, in some form, may be critical for the early stages of angiogenesis as part of the events associated with inflammation.
Myeloid cells are also a major source of versican in tumor inflammation. Versican from myeloid cells promotes tumor metastasis in breast cancer (180). Versican expressed by CD11b+ Ly6Chigh myeloid cells promotes lung metastasis in a TGFβ-dependent manner in mouse models (181). Intriguingly, versican expression is upregulated by macrophages when co-cultured with carcinoma cells (161, 162), suggesting that the source of versican includes both myeloid cells associated with cancer cells (182, 183). These studies raise the possibility of “crosstalk” among different cell types within the tumor that may influence the nature of versican accumulation and bioactivity which would provide key links to the inflammation associated with cancer initiation, promotion, and metastatic progression (see Figure 1).
Infiltrating myeloid cells secrete versican which can also exhibit immunosuppressive activities in some cancers (74, 75, 103, 118, 184, 185). For example, ADAMTS-generated versikine regulates mouse BATF3-dendritic cell (BATF3-DC) differentiation (74). BATF3-DCs control CD8+ abundance in the tumor microenvironment (186). These observations support a role for versican as being tolerogenic. In addition, a recent study (187) using bone marrow biopsies from 35 myeloma patients revealed a significant correlation of versikine accumulation with infiltration of CD8+ T cells supporting a model in which macrophages and regulatory DCs secrete tolerogenic versican which is subsequently degraded, generating versikine and further altering the immunosuppressive nature of the tumor microenvironment. Thus, taken together, these studies establish that both intact versican and a proteolytic degradation product of versican have immunomodulatory properties and suggest that the anti-tumor properties of versikine might antagonize the pro-tumor properties of intact versican (13, 23, 73, 75, 188). The juxtaposition of these findings indicates that the consequences of ECM-derived–DAMP interactions with PRRs can have sharply differing outcomes depending on the contextual specifics of versican structure.
The tumor stromal ECM microenvironment is characteristic of a wound that does not heal (189, 190) and is functionally analogous to an immune privileged site in normal tissue such as in the eye [reviewed in (191)]. Versican and hyaluronan are enriched in immune privileged sites. The expression and accumulation of versican in the microenvironment of some tumors is associated with reduced numbers of CD8-positive T cells indicating that versican may interfere with T-cell invasion as part of an immunosuppressive activity (192). In addition, versican may be a player in regulating the expression of PD-L1 as part of the autoimmune checkpoint involved in tumor escape from the T-cell immune response. Hartley and colleagues showed that versican produced by mouse tumor cells stimulated monocytes to produce TNFα in a TLR2-dependent manner which in turn upregulated the expression of PD-L1 by mouse monocyte/macrophages (193). The involvement of versican in regulating PD-L1 expression in T cells awaits further investigation. However, such results overall suggest that versican may be part of the exclusionary zone in the microenvironment impacting and preventing T cells access to the tumor (191, 194–196). Such an involvement should be considered as a potential target in immunotherapy treatment of cancer. For example, versican accumulation in the stroma could interfere with T cell-mediated tumor destruction by displacing the T cells from the appropriate tumor target (191).
While the CS containing isoforms of versican appear to be pro-inflammatory, V3 which contains no CS chains when overexpressed in arterial smooth muscle cells inhibits the expression of pro inflammatory cytokines such as CXCL1, CCL20, and CCL2 resulting in blockade of epidermal growth factor receptor and NFκB signaling activity (42, 43). V3 expression also reduced the rate of tumor growth of melanoma by inhibiting tumor cell proliferation as well as increasing the rate of apoptosis. Such experiments highlight a potential role for V3 in counteracting the inflammatory response associated with cancer (197–199).
Conclusions
Studies indicating a causal role of versican regulating events that drive immunity and inflammation are increasing. There is no doubt that versican, either intact or degraded, as an ECM participant, has a role, but whether it acts alone or in combination with other components during inflammatory events is still not fully understood. Published studies indicate that versican has both pro- and anti-inflammatory activities depending upon the context in which versican is presented to cells. Versican's role in inflammation also depends on temporal and spatial considerations and it may play different roles whether it is involved early or late in disease and whether it is intact or degraded. Furthermore, versican's versatility in binding to multiple receptors and other components involved in the inflammatory response identifies it as a “keystone molecule” regulating inflammation. Developing targeted reagents and therapeutic strategies to interfere with versican accumulation should further identify key mechanisms regulating versican's biological activity. Versican is increasingly being seen as a potential therapeutic target in multiple diseases.
Thus, future directions will be to take advantage of the mouse models we have developed in which versican has been deleted conditionally in the whole animal or specifically in myeloid cells and determine the impact of selectively removing versican in mouse models of cardiovascular and lung disease, autoimmune diseases such as type 1 diabetes and multiple sclerosis, and in some cancers, particularly breast cancer. Such studies have not been possible in the past due to the unavailability of the versican KO mouse. We are interested in focusing on the impact of the different versican variants, such as V3 to better define their role in inflammation and immunity since our preliminary data indicates that V3 acts differently than V0 or V1 in influencing events that drive the immune and inflammatory response. Our cell biology studies will focus on how versican influences immune cells and the immune response focusing on its role in antigen presentation, immune synapse formation, and immune activation important, for example, in the destruction of tumors. Indeed versican, as part of the ECM, is “versatile” and a “keystone” molecule in the regulation of immunity and inflammation.
Author Contributions
TW wrote the manuscript. IK, SE, IH, MC, OP, CA, and CF edited and revised the manuscript. All authors have reviewed and approved the final version of this article.
Funding
This study was supported by National Institutes of Health grants R01 DK 096087 and U19 AI 125378 (TW) and R01 AI 130280 (CF and TW) and by Cancer Research UK career establishment award A27947 (OMTP).
Conflict of Interest
The authors declare that the research was conducted in the absence of any commercial or financial relationships that could be construed as a potential conflict of interest.
Acknowledgments
We thank Dr. Virginia M. Green for her careful editing and preparation of the manuscript. We also thank all past and present collaborators and lab members for their dedication to furthering our understanding of the role of this important ECM macromolecule in disease pathogenesis. We apologize to those authors whose original contributions were not cited in lieu of reviews due to space requirements.
References
1. Liu J, Cao X. Cellular and molecular regulation of innate inflammatory responses. Cell Mol Immunol. (2016) 13:711–21. doi: 10.1007/1038/cmi.2016.58
2. Fullerton JN, Gilroy DW. Resolution of inflammation: a new therapeutic frontier. Nat Rev Drug Discov. (2016) 15:551–67. doi: 10.1007/1038/nrd.2016.39
3. Laubli H, Varki A. Sialic acid-binding immunoglobulin-like lectins (Siglecs) detect self-associated molecular patterns to regulate immune responses. Cell Mol Life Sci. (2019). doi: 10.1007/s00018-019-03288-x
4. Frevert CW, Felgenhauer J, Wygrecka M, Nastase MV, Schaefer L. Danger-associated molecular patterns derived from the extracellular matrix provide temporal control of innate immunity. J Histochem Cytochem. (2018) 66:213–27. doi: 10.1007/1369/0022155417740880
5. Gill S, Wight TN, Frevert CW. Proteoglycans: key regulators of pulmonary inflammation and the innate immune response to lung infection. Anat Rec. (2010) 293:968–81. doi: 10.1002/ar.21094
6. Kang I, Chang MY, Wight TN, Frevert CW. Proteoglycans as immunomodulators of the innate immune response to lung infection. J Histochem Cytochem. (2018) 66:241–59. doi: 10.1369/0022155417751880
7. Sorokin L. The impact of the extracellular matrix on inflammation. Nat Rev Immunol. (2010) 10:712–23. doi: 10.1038/nri2852
8. Vaday GG, Franitza S, Schor H, Hecht I, Brill A, Cahalon L, et al. Combinatorial signals by inflammatory cytokines and chemokines mediate leukocyte interactions with extracellular matrix. J Leukoc Biol. (2001) 69:885–92. doi: 10.1189/jlb.69.6.885
9. Vaday GG, Lider O. Extracellular matrix moieties, cytokines, and enzymes: dynamic effects on immune cell behavior and inflammation. J Leukoc Biol. (2000) 67:149–59. doi: 10.1002/jlb.67.2.149
10. Wight TN, Kang I, Merrilees MJ. Versican and the control of inflammation. Matrix Biol. (2014) 35:152–61. doi: 10.1016/j.matbio.2014.01.015
11. Maquart FX, Pasco S, Ramont L, Hornebeck W, Monboisse JC. An introduction to matrikines: extracellular matrix-derived peptides which regulate cell activity. Implication in tumor invasion. Crit Rev Oncol Hematol. (2004) 49:199–202. doi: 10.1016/j.critrevonc.2003.06.007
12. Nandadasa S, Foulcer S, Apte SS. The multiple, complex roles of versican and its proteolytic turnover by ADAMTS proteases during embryogenesis. Matrix Biol. (2014) 35:34–41. doi: 10.1016/j.matbio.2014.01.005
13. Timms K, Maurice SB. Context-dependent bioactivity of versican fragments. Glycobiology. (2019). doi: 10.1093/glycob/cwz090. [Epub ahead of print].
14. Frey H, Schroeder N, Manon-Jensen T, Iozzo RV, Schaefer L. Biological interplay between proteoglycans and their innate immune receptors in inflammation. FEBS J. (2013) 280:2165–79. doi: 10.1111/febs.12145
15. Karamanos NK, Piperigkou Z, Theocharis AD, Watanabe H, Franchi M, Baud S, et al. Proteoglycan chemical diversity drives multifunctional cell regulation and therapeutics. Chem Rev. (2018) 118:9152–232. doi: 10.1021/acs.chemrev.8b00354
16. Karamanos NK, Theocharis AD, Neill T, Iozzo RV. Matrix modeling and remodeling: a biological interplay regulating tissue homeostasis and diseases. Matrix Biol. (2019) 75–76:1–11. doi: 10.1016/j.matbio.2018.08.007
17. Parish CR. The role of heparan sulphate in inflammation. Nat Rev Immunol. (2006) 6:633–43. doi: 10.1038/nri1918
18. Roedig H, Damiescu R, Zeng-Brouwers J, Kutija I, Trebicka J, Wygrecka M, et al. Danger matrix molecules orchestrate CD14/CD44 signaling in cancer development. Semin Cancer Biol. (2019). doi: 10.1016/j.semcancer.2019.07.026
19. Schaefer L, Babelova A, Kiss E, Hausser HJ, Baliova M, Krzyzankova M, et al. The matrix component biglycan is proinflammatory and signals through Toll-like receptors 4 and 2 in macrophages. J Clin Invest. (2005) 115:2223–33. doi: 10.1172/JCI23755
20. Taylor KR, Gallo RL. Glycosaminoglycans and their proteoglycans: host-associated molecular patterns for initiation and modulation of inflammation. FASEB J. (2006) 20:9–22. doi: 10.1096/fj.05-4682rev
21. Wight TN, Frevert CW, Debley JS, Reeves SR, Parks WC, Ziegler SF. Interplay of extracellular matrix and leukocytes in lung inflammation. Cell Immunol. (2017) 312:1–14. doi: 10.1016/j.cellimm.2016.12.003
22. Theocharis AD, Skandalis SS, Tzanakakis GN, Karamanos NK. Proteoglycans in health and disease: novel roles for proteoglycans in malignancy and their pharmacological targeting. FEBS J. (2010) 277:3904–23. doi: 10.1111/j.1742-4658.2010.07800.x
23. Zhang Z, Miao L, Wang L. Inflammation amplification by versican: the first mediator. Int J Mol Sci. (2012) 13:6873–82. doi: 10.3390/ijms13066873
24. Wight TN, Heinegård DK, Hascall VC. Proteoglycans: structure and function. In: Hay ED, editor. Cell Biology of Extracellular Matrix. New York, NY: Plenum Press (1991). p. 45–78.
25. Wight TN. The pathobiology of versican. In: Karamanos N, editor. Extracellular Matrix: Pathobiology and Signaling. Berlin: Walter De Gruyter GMBH & Co., KG (2012). p. 154–70.
26. Wight TN, Kinsella MG, Evanko SP, Potter-Perigo S, Merrilees MJ. Versican and the regulation of cell phenotype in disease. Biochim Biophys Acta. (2014) 1840:2441–51. doi: 10.1016/j.bbagen.2013.12.028
27. Zimmermann DR, Ruoslahti E. Multiple domains of the large fibroblast proteoglycan, versican. EMBO J. (1989) 8:2975–81. doi: 10.1002/j.1460-2075.1989.tb08447.x
28. Iozzo RV, Naso MF, Cannizzaro LA, Wasmuth JJ, McPherson JD. Mapping of the versican proteoglycan gene (CSPG2) to the long arm of human chromosome 5 (5q12-5q14). Genomics. (1992) 14:845–51. doi: 10.1016/s0888-7543(05)80103-x
29. Zako M, Shinomura T, Ujita M, Ito K, Kimata K. Expression of PG-M (V3), an alternatively spliced form of PG-M without a chondroitin sulfate attachment region in mouse and human tissues. J Biol Chem. (1995) 270:3914–8. doi: 10.1074/jbc.270.8.3914
30. Dours-Zimmermann MT, Zimmerman DR. A novel glycosaminoglycan attachment domain identified in two alternative splice variants of human versican. J Biol Chem. (1994) 269:32992–8.
31. Ito K, Shinomura T, Zako M, Ujita M, Kimata K. Multiple forms of mouse PG-M, a large chondroitin sulfate proteoglycan generated by alternative splicing. J Biol Chem. (1995) 270:958–65. doi: 10.1074/jbc.270.2.958
32. Kischel P, Waltregny D, Dumont B, Turtoi A, Greffe Y, Kirsch S, et al. Versican overexpression in human breast cancer lesions: known and new isoforms for stromal tumor targeting. Int J Cancer. (2010) 126:640–50. doi: 10.1002/ijc.24812
33. Lemire JM, Braun KR, Maurel P, Kaplan ED, Schwartz SM, Wight TN. Versican/PG-M isoforms in vascular smooth muscle cells. Arterioscler Thromb Vasc Biol. (1999) 19:1630–9. doi: 10.1161/01.atv.19.7.1630
34. Evanko SP, Chan CK, Johnson PY, Frevert CW, Wight TN. The biochemistry and immunohistochemistry of versican. In: Mecham RP, editor. Methods in Extracellular Matrix Biology. Cambridge, MA: Academic Press (Elsevier Inc.) (2018). p. 261–79.
35. Chang Y, Yanagishita M, Hascall VC, Wight TN. Proteoglycans synthesized by smooth muscle cells derived from monkey (Macaca nemestrina) aorta. J Biol Chem. (1983) 258:5679–88.
36. Zimmermann D. Versican. In: Iozzo R, editor. Proteoglycans: Structure, Biology and Molecular Interactions. New York, NY: Marcel Dekker, Inc. (2000). p. 327–41.
37. Sheng W, Wang G, Wang Y, Liang J, Wen J, Zheng PS, et al. The roles of versican V1 and V2 isoforms in cell proliferation and apoptosis. Mol Biol Cell. (2005) 16:1330–40. doi: 10.1091/mbc.e04-04-0295
38. Wu Y, Zhang Y, Cao L, Chen L, Lee V, Zheng PS, et al. Identification of the motif in versican G3 domain that plays a dominant-negative effect on astrocytoma cell proliferation through inhibiting versican secretion and binding. J Biol Chem. (2001) 276:14178–86. doi: 10.1074/jbc.M100618200
39. Merrilees MJ, Wight TN. Targeting the Matrix: Potential Benefits for Versican Therapeutics. Current Comments. Elsevier (2012). Available online at: http://www.elsevierblogs.com/currentcomments/?p=519 (accessed June 04, 2019).
40. Wight TN. A role for proteoglycans in vascular disease. Matrix Biol. (2018) 71–72:396–420. doi: 10.1016/j.matbio.2018.02.019
41. Wight TN, Merrilees MJ. Proteoglycans in atherosclerosis and restenosis: key roles for versican. Circ Res. (2004) 94:1158–67. doi: 10.1161/01.RES.0000126921.29919.51
42. Kang I, Barth JL, Sproul EP, Yoon DW, Braun KR, Argraves WS, et al. Expression of V3 versican by rat arterial smooth muscle cells promotes differentiated and anti-inflammatory phenotypes. J Biol Chem. (2015) 290:21629–41. doi: 10.1074/jbc.M115.657486
43. Kang I, Yoon DW, Braun KR, Wight TN. Expression of versican V3 by arterial smooth muscle cells alters TGFβ-, EGF-, and NFκB-dependent signaling pathways, creating a microenvironment that resists monocyte adhesion. J Biol Chem. (2014) 289:15393–404. doi: 10.1074/jbc.M113.544338
44. Lemire JM, Merrilees MJ, Braun KR, Wight TN. Overexpression of the V3 variant of versican alters arterial smooth muscle cell adhesion, migration, and proliferation in vitro. J Cell Physiol. (2002) 190:38–45. doi: 10.1002/jcp.10043
45. Merrilees MJ, Lemire JM, Fischer JW, Kinsella MG, Braun KR, Clowes AW, et al. Retrovirally mediated overexpression of versican v3 by arterial smooth muscle cells induces tropoelastin synthesis and elastic fiber formation in vitro and in neointima after vascular injury. Circ Res. (2002) 90:481–7. doi: 10.1161/hh0402.105791
46. Bogen O, Bender O, Alvarez P, Kern M, Tomiuk S, Hucho F, et al. Expression of a novel versican variant in dorsal root ganglia from spared nerve injury rats. Mol Pain. (2019) 15:1744806919874557. doi: 10.1177/1744806919874557
47. Naso MF, Zimmermann DR, Iozzo RV. Characterization of the complete genomic structure of the human versican gene and functional analysis of its promoter. J Biol Chem. (1994) 269:32999–3008.
48. Cardoso LE, Little PJ, Ballinger ML, Chan CK, Braun KR, Potter-Perigo S, et al. Platelet-derived growth factor differentially regulates the expression and post-translational modification of versican by arterial smooth muscle cells through distinct protein kinase C and extracellular signal-regulated kinase pathways. J Biol Chem. (2010) 285:6987–95. doi: 10.1074/jbc.M109.088674
49. Little PJ, Tannock L, Olin KL, Chait A, Wight TN. Proteoglycans synthesized by arterial smooth muscle cells in the presence of transforming growth factor-beta1 exhibit increased binding to LDLs. Arterioscler Thromb Vasc Biol. (2002) 22:55–60. doi: 10.1161/hq0102.101100
50. Schönherr E, Järveläinen HT, Sandell LJ, Wight TN. Effects of platelet-derived growth factor and transforming growth factor-β 1 on the synthesis of a large versican-like chondroitin sulfate proteoglycan by arterial smooth muscle cells. J Biol Chem. (1991) 266:17640–7.
51. Hirose J, Kawashima H, Yoshie O, Tashiro K, Miyasaka M. Versican interacts with chemokines and modulates cellular responses. J Biol Chem. (2001) 276:5228–34. doi: 10.1074/jbc.M007542200
52. Wu YJ, La Pierre DP, Wu J, Yee AJ, Yang BB. The interaction of versican with its binding partners. Cell Res. (2005) 15:483–94. doi: 10.1038/sj.cr.7290318
53. Stephenson EL, Yong VW. Pro-inflammatory roles of chondroitin sulfate proteoglycans in disorders of the central nervous system. Matrix Biol. (2018) 71–72:432–42. doi: 10.1016/j.matbio.2018.04.010
54. Chait A, Wight TN. Interaction of native and modified low-density lipoproteins with extracellular matrix. Curr Opin Lipidol. (2000) 11:457–63. doi: 10.1097/00041433-200010000-00003
55. Little PJ, Osman N, O'Brien KD. Hyperelongated biglycan: the surreptitious initiator of atherosclerosis. Curr Opin Lipidol. (2008) 19:448–54. doi: 10.1097/MOL.0b013e32830dd7c4
56. Kinsella MG, Bressler SL, Wight TN. The regulated synthesis of versican, decorin, and biglycan: extracellular matrix proteoglycans that influence cellular phenotype. Crit Rev Eukaryot Gene Expr. (2004) 14:203–34. doi: 10.1615/critreveukaryotgeneexpr.v14.i3.40
57. Rahmani M, Carthy JM, McManus BM. Mapping of the Wnt/beta-catenin/TCF response elements in the human versican promoter. Methods Mol Biol. (2012) 836:35–52. doi: 10.1007/978-1-61779-498-8_3
58. Rahmani M, Read JT, Carthy JM, McDonald PC, Wong BW, Esfandiarei M, et al. Regulation of the versican promoter by the beta-catenin-T-cell factor complex in vascular smooth muscle cells. J Biol Chem. (2005) 280:13019–28. doi: 10.1074/jbc.M411766200
59. Rahmani M, Wong BW, Ang L, Cheung CC, Carthy JM, Walinski H, et al. Versican: signaling to transcriptional control pathways. Can J Physiol Pharmacol. (2006) 84:77–92. doi: 10.1139/y05-154
60. Chang MY, Kang I, Gale M Jr, Manicone AM, Kinsella MG, Braun KR, et al. Versican is produced by Trif- and type I interferon-dependent signaling in macrophages and contributes to fine control of innate immunity in lungs. Am J Physiol Lung Cell Mol Physiol. (2017) 313:L1069–86. doi: 10.1152/ajplung.00353.2017
61. Rutnam ZJ, Wight TN, Yang BB. miRNAs regulate expression and function of extracellular matrix molecules. Matrix Biol. (2013) 32:74–85. doi: 10.1016/j.matbio.2012.11.003
62. Wang X, Hu G, Zhou J. Repression of versican expression by microRNA-143. J Biol Chem. (2010) 285:23241–50. doi: 10.1074/jbc.M109.084673
63. Yan Y, Qin D, Hu B, Zhang C, Liu S, Wu D, et al. Deletion of miR-126a promotes hepatic aging and inflammation in a mouse model of cholestasis. Mol Ther Nucleic Acids. (2019) 16:494–504. doi: 10.1016/j.omtn.2019.04.002
64. Halpert I, Sires U, Potter-Perigo S, Wight TN, Shapiro DS, Welgus HG, et al. Matrilysin is expressed by lipid-laden macrophages at sites of potential rupture in atherosclerotic lesions and localized to areas of versican deposits. Proc Natl Acad Sci USA. (1996) 93:9748–53.
65. Passi A, Negrini D, Albertini R, Miserocchi G, De Luca G. The sensitivity of versican from rabbit lung to gelatinase A (MMP-2) and B (MMP-9) and its involvement in the development of hydraulic lung edema. FEBS Lett. (1999) 456:93–6. doi: 10.1016/s0014-5793(99)00929-1
66. Perides G, Asher RA, Lark MW, Lane WS, Robinson RA, Bignami A. Glial hyaluronate-binding protein: a product of metalloproteinase digestion of versican? Biochem J. (1995) 312:377–84. doi: 10.1042/bj3120377
67. Kenagy RD, Fischer JW, Davies MG, Berceli SA, Hawkins SM, Wight TN, et al. Increased plasmin and serine proteinase activity during flow-induced intimal atrophy in baboon PTFE grafts. Arterioscler Thromb Vasc Biol. (2002) 22:400–4. doi: 10.1161/hq0302.105376
68. Apte SS. A disintegrin-like and metalloprotease (reprolysin type) with thrombospondin type 1 motifs: the ADAMTS family. Int J Biochem Cell Biol. (2004) 36:981–5. doi: 10.1016/j.biocel.2004.01.014
69. Apte SS. A disintegrin-like and metalloprotease (reprolysin-type) with thrombospondin type 1 motif (ADAMTS) superfamily: functions and mechanisms. J Biol Chem. (2009) 284:31493–7. doi: 10.1074/jbc.R109.052340
70. Kenagy RD, Plaas AH, Wight TN. Versican degradation and vascular disease. Trends Cardiovasc Med. (2006) 16:209–15. doi: 10.1016/j.tcm.2006.03.011
71. Sandy JD, Westling J, Kenagy RD, Iruela-Arispe ML, Verscharen C, Rodriguez-Mazaneque JC, et al. Versican V1 proteolysis in human aorta in vivo occurs at the Glu441-Ala442 bond, a site that is cleaved by recombinant ADAMTS-1 and ADAMTS-4. J Biol Chem. (2001) 276:13372–8. doi: 10.1074/jbc.M009737200
72. Somerville RP, Longpre JM, Jungers KA, Engle JM, Ross M, Evanko S, et al. Characterization of ADAMTS-9 and ADAMTS-20 as a distinct ADAMTS subfamily related to Caenorhabditis elegans GON-1. J Biol Chem. (2003) 278:9503–13. doi: 10.1074/jbc.M211009200
73. Schmitt M. Versican vs versikine: tolerance vs attack. Blood. (2016) 128:612–3. doi: 10.1182/blood-2016-06-721092
74. Hope C, Emmerich PB, Papadas A, Pagenkopf A, Matkowskyj KA, Van De Hey DR, et al. Versican-derived matrikines regulate Batf3-dendritic cell dfferentiation and pomote T cell infiltration in colorectal cancer. J Immunol. (2017) 199:1933–41. doi: 10.4049/jimmunol.1700529
75. Hope C, Foulcer S, Jagodinsky J, Chen SX, Jensen JL, Patel S, et al. Immunoregulatory roles of versican proteolysis in the myeloma microenvironment. Blood. (2016) 128:680–5. doi: 10.1182/blood-2016-03-705780
76. Mjaatvedt CH, Yamamura H, Capehart AA, Turner D, Markwald RR. The Cspg2 gene, disrupted in the hdf mutant, is required for right cardiac chamber and endocardial cushion formation. Dev Biol. (1998) 202:56–66. doi: 10.1006/dbio.1998.9001
77. Snyder JM, Washington IM, Birkland T, Chang MY, Frevert CW. Correlation of versican expression, accumulation, and degradation during embryonic development by quantitative immunohistochemistry. J Histochem Cytochem. (2015) 63:952–67. doi: 10.1369/0022155415610383
78. Otsuka F, Kramer MC, Woudstra P, Yahagi K, Ladich E, Finn AV, et al. Natural progression of atherosclerosis from pathologic intimal thickening to late fibroatheroma in human coronary arteries: a pathology study. Atherosclerosis. (2015) 241:772–82. doi: 10.1016/j.atherosclerosis.2015.05.011
79. Burke AP, Jarvelainen H, Kolodgie FD, Goel A, Wight TN, Virmani R. Superficial pseudoaneurysms: clinicopathologic aspects and involvement of extracellular matrix proteoglycans. Mod Pathol. (2004) 17:482–8. doi: 10.1038/modpathol.3800060
80. Evanko S, Raines EW, Ross R, Gold LI, Wight TN. Proteoglycan distribution in lesions of atherosclerosis depends on lesion severity, structural characteristics and the proximity of platelet-derived growth factor and transforming growth factor-b. Am J Pathol. (1998) 152:533–46.
81. Farb A, Kolodgie FD, Hwang JY, Burke AP, Tefera K, Weber DK, et al. Extracellular matrix changes in stented human coronary arteries. Circulation. (2004) 110:940–7. doi: 10.1161/01.CIR.0000139337.56084.30
82. Olive M, Harten I, Mitchell R, Beers J, Djabali K, Cao K, et al. Cardiovascular pathology in Hutchinson-Gilford Progeria: Correlation with the vascular pathology of aging. Arterioscler Thromb Vasc Biol. (2010) 30:2301–9. doi: 10.1161/ATVBAHA.110.209460
83. Wight T, Evanko S, Kinsella MG, Chang MY, Yeop Han C, Sakr S, et al. The pro-inflammatory nature of the extracellular matrix. In: Matsuzawa Y, Kita T, Nagai R, Teramoto T, editors. Atherosclerosis XIII. Amsterdam: Elsevier B.V. (2004). p. 404–6.
84. Merrilees MJ, Beaumont BW, Braun KR, Thomas AC, Kang I, Hinek A, et al. Neointima formed by arterial smooth muscle cells expressing versican variant v3 is resistant to lipid and macrophage accumulation. Arterioscler Thromb Vasc Biol. (2011) 31:1309–16. doi: 10.1161/ATVBAHA.111.225573
85. Andersson-Sjoland A, Hallgren O, Rolandsson S, Weitoft M, Tykesson E, Larsson-Callerfelt AK, et al. Versican in inflammation and tissue remodeling: the impact on lung disorders. Glycobiology. (2015) 25:243–51. doi: 10.1093/glycob/cwu120
86. Bensadoun ES, Burke AK, Hogg JC, Roberts CR. Proteoglycan deposition in pulmonary fibrosis. Am J Respir Crit Care Med. (1996) 154:1819–28.
87. Chang MY, Tanino Y, Vidova V, Kinsella MG, Chan CK, Johnson PY, et al. A rapid increase in macrophage-derived versican and hyaluronan in infectious lung disease. Matrix Biol. (2014) 34:1–12. doi: 10.1016/j.matbio.2014.01.011
88. Kang I, Harten IA, Chang MY, Braun KR, Sheih A, Nivison MP, et al. Versican deficiency significantly reduces lung inflammatory response induced by polyinosine-polycytidylic acid stimulation. J Biol Chem. (2017) 292:51–63. doi: 10.1074/jbc.M116.753186
89. Reeves SR, Kaber G, Sheih A, Cheng G, Aronica MA, Merrilees MJ, et al. Subepithelial accumulation of versican in a cockroach antigen-induced murine model of allerigic asthma. J Histochem Cytochem. (2016) 64:364–80. doi: 10.1369/0022155416642989
90. Sobel RA, Ahmed AS. White matter extracellular matrix chondroitin sulfate/dermatan sulfate proteoglycans in multiple sclerosis. J Neuropathol Exp Neurol. (2001) 60:1198–207. doi: 10.1093/jnen/60.12.1198
91. Stephenson EL, Mishra MK, Moussienko D, Laflamme N, Rivest S, Ling CC, et al. Chondroitin sulfate proteoglycans as novel drivers of leucocyte infiltration in multiple sclerosis. Brain. (2018) 141:1094–110. doi: 10.1093/brain/awy033
92. Haylock-Jacobs S, Keough MB, Lau L, Yong VW. Chondroitin sulphate proteoglycans: extracellular matrix proteins that regulate immunity of the central nervous system. Autoimmun Rev. (2011) 10:766–72. doi: 10.1016/j.autrev.2011.05.019
93. de la Motte C, Hascall VC, Drazba JA, Strong SA. Poly I:C induces mononuclear leukocyte-adhesive hyaluronan structures on colon smooth muscle cells: IαI and versican facilitate adhesion. In: Kennedy JF, Phillips GO, Williams PA, Hascall VC, editors. Hyaluronan: Chemical, Biochemical and Biological Aspects. Cambridge, UK: Woodhead Publishing Limited. (2002). p. 381–8.
94. de la Motte CA, Hascall VC, Drazba J, Bandyopadhyay SK, Strong SA. Mononuclear leukocytes bind to specific hyaluronan structures on colon mucosal smooth muscle cells treated with polyinosinic acid:polycytidylic acid: inter-α-trypsin inhibitor is crucial to structure and function. Am J Pathol. (2003) 163:121–33. doi: 10.1016/s0002-9440(10)63636-x
95. Petrey AC, de la Motte CA. Hyaluronan, a crucial regulator of inflammation. Front Immunol. (2014) 5:101. doi: 10.3389/fimmu.2014.00101
96. Petrey AC, de la Motte CA. Hyaluronan in inflammatory bowel disease: cross-linking inflammation and coagulation. Matrix Biol. (2019) 78–79:314–23. doi: 10.1016/j.matbio.2018.03.011
97. Rienks M, Papageorgiou AP, Frangogiannis NG, Heymans S. Myocardial extracellular matrix: an ever-changing and diverse entity. Circ Res. (2014) 114:872–88. doi: 10.1161/CIRCRESAHA.114.302533
98. Kunisada M, Yogianti F, Sakumi K, Ono R, Nakabeppu Y, Nishigori C. Increased expression of versican in the inflammatory response to UVB- and reactive oxygen species-induced skin tumorigenesis. Am J Pathol. (2011) 179:3056–65. doi: 10.1016/j.ajpath.2011.08.042
99. Islam S, Chuensirikulchai K, Khummuang S, Keratibumrungpong T, Kongtawelert P, Kasinrerk W, et al. Accumulation of versican facilitates wound healing: implication of its initial ADAMTS-cleavage site. Matrix Biol. (2020) 87:77–93. doi: 10.1016/j.matbio.2019.10.006
100. Mukhopadhyay A, Nikopoulos K, Maugeri A, de Brouwer AP, van Nouhuys CE, Boon CJ, et al. Erosive vitreoretinopathy and wagner disease are caused by intronic mutations in CSPG2/Versican that result in an imbalance of splice variants. Invest Ophthalmol Vis Sci. (2006) 47:3565–72. doi: 10.1167/iovs.06-0141
101. Tang PH, Velez G, Tsang SH, Bassuk AG, Mahajan VB. VCAN canonical splice site mutation is associated with vitreoretinal degeneration and disrupts an MMP proteolytic site. Invest Ophthalmol Vis Sci. (2019) 60:282–93. doi: 10.1167/iovs.18-25624
102. Bogdani M, Johnson PY, Potter-Perigo S, Nagy N, Day AJ, Bollyky PL, et al. Hyaluronan and hyaluronan binding proteins accumulate in both human type 1 diabetic islets and lymphoid tissues and associate with inflammatory cells in insulitis. Diabetes. (2014) 63:2727–43. doi: 10.2337/db13-1658
103. Keire PA, Kang I, Wight TN. Versican: Role in cancer tumorigenesis. In: Brekken RA, Stupack DG, editors. Extracellular Matrix in Tumor Biology. Cham: Springer International Publishing (2017). p. 51–74.
104. Theocharis AD, Karamanos NK. Proteoglycans remodeling in cancer: underlying molecular mechanisms. Matrix Biol. (2019) 75–76:220–59. doi: 10.1016/j.matbio.2017.10.008
105. Ricciardelli C, Sakko AJ, Ween MP, Russell DL, Horsfall DJ. The biological role and regulation of versican levels in cancer. Cancer Metastasis Rev. (2009) 28:233–45. doi: 10.1007/s10555-009-9182-y
106. LeBaron RG, Zimmermann DR, Ruoslahti E. Hyaluronate binding properties of versican. J Biol Chem. (1992) 267:10003–10.
107. Matsumoto K, Shionyu M, Go M, Shimizu K, Shinomura T, Kimata K, et al. Distinct interaction of versican/PG-M with hyaluronan and link protein. J Biol Chem. (2003) 278:41205–12. doi: 10.1074/jbc.M305060200
108. Kohda D, Morton CJ, Parkar AA, Hatanaka H, Inagaki FM, Campbell ID, et al. Solution structure of the link module: a hyaluronan-binding domain involved in extracellular matrix stability and cell migration. Cell. (1996) 86:767–75.
109. Day AJ, Prestwich GD. Hyaluronan-binding proteins: tying up the giant. J Biol Chem. (2002) 277:4585–8. doi: 10.1074/jbc.R100036200
110. Day AJ, Milner CM. TSG-6: A multifunctional protein with anti-inflammatory and tissue-protective properties. Matrix Biol. (2019) 78–79:60–83. doi: 10.1016/j.matbio.2018.01.011
111. Garantziotis S, Savani RC. Hyaluronan biology: A complex balancing act of structure, function, location and context. Matrix Biol. (2019) 78–79:1–10. doi: 10.1016/j.matbio.2019.02.002
112. Day AJ, de la Motte CA. Hyaluronan cross-linking: a protective mechanism in inflammation? Trends Immunol. (2005) 26:637–43. doi: 10.1016/j.it.2005.09.009
113. Tighe RM, Garantziotis S. Hyaluronan interactions with innate immunity in lung biology. Matrix Biol. (2019) 78–79:84–99. doi: 10.1016/j.matbio.2018.01.027
114. Gaucherand L, Falk BA, Evanko SP, Workman G, Chan CK, Wight TN. Crosstalk between T lymphocytes and lung fibroblasts: generation of a hyaluronan-enriched extracellular matrix adhesive for monocytes. J Cell Biochem. (2017) 118:2118–30. doi: 10.1002/jcb.25842
115. Potter-Perigo S, Johnson PY, Evanko SP, Chan CK, Braun KR, Wilkinson TS, et al. Polyinosine-polycytidylic acid stimulates versican accumulation in the extracellular matrix promoting monocyte adhesion. Am J Respir Cell Mol Biol. (2010) 43:109–20. doi: 10.1165/rcmb.2009-0081OC
116. Hascall VC, Majors AK, De La Motte CA, Evanko SP, Wang A, Drazba JA, et al. Intracellular hyaluronan: a new frontier for inflammation? Biochim Biophys Acta. (2004) 1673:3–12. doi: 10.0.1016/j.bbagen.2004.02.013
117. Jiang D, Liang J, Noble PW. Hyaluronan as an immune regulator in human diseases. Physiol Rev. (2011) 91:221–64. doi: 10.1152/physrev.00052.2009
118. Tang M, Diao J, Gu H, Khatri I, Zhao J, Cattral MS. Toll-like receptor 2 activation promotes tumor dendritic cell dysfunction by regulating IL-6 and IL-10 receptor signaling. Cell Rep. (2015) 13:2851–64. doi: 10.1016/j.celrep.2015.11.053
119. Kim S, Takahashi H, Lin WW, Descargues P, Grivennikov S, Kim Y, et al. Carcinoma-produced factors activate myeloid cells through TLR2 to stimulate metastasis. Nature. (2009) 457:102–6. doi: 10.1038/nature07623
120. Zheng PS, Vais D, Lapierre D, Liang YY, Lee V, Yang BL, et al. PG-M/versican binds to P-selectin glycoprotein ligand-1 and mediates leukocyte aggregation. J Cell Sci. (2004) 117:5887–95. doi: 10.1242/jcs.01516
121. Kawashima H, Atarashi K, Hirose M, Hirose J, Yamada S, Sugahara K, et al. Oversulfated chondroitin/dermatan sulfates containing GlcAbeta1/IdoAalpha1-3GalNAc(4,6-O-disulfate) interact with L- and P-selectin and chemokines. J Biol Chem. (2002) 277:12921–30. doi: 10.1074/jbc.M200396200
122. Kawashima H, Hirose M, Hirose J, Nagakubo D, Plaas AH, Miyasaka M. Binding of a large chondroitin sulfate/dermatan sulfate proteoglycan, versican, to L-selectin, P-selectin, and CD44. J Biol Chem. (2000) 275:35448–56. doi: 10.1074/jbc.M003387200
123. Wang W, Xu GL, Jia WD, Ma JL, Li JS, Ge YS, et al. Ligation of TLR2 by versican: a link between inflammation and metastasis. Arch Med Res. (2009) 40:321–3. doi: 10.1016/j.arcmed.2009.04.005
124. Poluzzi C, Nastase MV, Zeng-Brouwers J, Roedig H, Hsieh LT, Michaelis JB, et al. Biglycan evokes autophagy in macrophages via a novel CD44/Toll-like receptor 4 signaling axis in ischemia/reperfusion injury. Kidney Int. (2019) 95:540–62. doi: 10.1016/j.kint.2018.10.037
125. Zeng-Brouwers J, Beckmann J, Nastase MV, Iozzo RV, Schaefer L. De novo expression of circulating biglycan evokes an innate inflammatory tissue response via MyD88/TRIF pathways. Matrix Biol. (2014) 35:132–42. doi: 10.1016/j.matbio.2013.12.003
126. Kawashima H, Li YF, Watanabe N, Hirose J, Hirose M, Miyasaka M. Identification and characterization of ligands for L-selectin in the kidney. I. Versican, a large chondroitin sulfate proteoglycan, is a ligand for L-selectin. Int Immunol. (1999) 11:393–405. doi: 10.1093/intimm/11.3.393
127. Masuda A, Yasuoka H, Satoh T, Okazaki Y, Yamaguchi Y, Kuwana M. Versican is upregulated in circulating monocytes in patients with systemic sclerosis and amplifies a CCL2-mediated pathogenic loop. Arthritis Res Ther. (2013) 15:R74. doi: 10.1186/ar4251
128. Malla N, Berg E, Theocharis AD, Svineng G, Uhlin-Hansen L, Winberg JO. In vitro reconstitution of complexes between pro-matrix metalloproteinase-9 and the proteoglycans serglycin and versican. FEBS J. (2013) 280:2870–87. doi: 10.1111/febs.12291
129. Ra HJ, Harju-Baker S, Zhang F, Linhardt RJ, Wilson CL, Parks WC. Control of promatrilysin (MMP7) activation and substrate-specific activity by sulfated glycosaminoglycans. J Biol Chem. (2009) 284:27924–32. doi: 10.1074/jbc.M109.035147
130. Ra HJ, Parks WC. Control of matrix metalloproteinase catalytic activity. Matrix Biol. (2007) 26:587–96. doi: 10.1016/j.matbio.2007.07.001
131. Tocchi A, Parks WC. Functional interactions between matrix metalloproteinases and glycosaminoglycans. FEBS J. (2013) 280:2332–41. doi: 10.1111/febs.12198
132. Xu L, Xue T, Zhang J, Qu J. Knockdown of versican V1 induces a severe inflammatory response in LPS-induced acute lung injury via the TLR2-NF-kappaB signaling pathway in C57BL/6J mice. Mol Med Rep. (2016) 13:5005–12. doi: 10.3892/mmr.2016.5168
133. Coulson-Thomas VJ, Gesteira TF, Hascall V, Kao W. Umbilical cord mesenchymal stem cells suppress host rejection: The role of the glycocalyx. J Biol Chem. (2014) 289:23465–81. doi: 10.1074/jbc.M114.557447
134. Avnet S, Cortini M. Role of pericellular matrix in the regulation of cancer stemness. Stem Cell Rev. (2016) 12:464–75. doi: 10.1007/s12015-016-9660-x
135. Schwertfeger KL, Cowman MK, Telmer PG, Turley EA, McCarthy JB. Hyaluronan, inflammation, and breast cancer progression. Front Immunol. (2015) 6:236. doi: 10.3389/fimmu.2015.00236
136. Turley EA, Wood DK, McCarthy JB. Carcinoma cell hyaluronan as a “portable” cancerized prometastatic microenvironment. Cancer Res. (2016) 76:2507–12. doi: 10.1158/0008-5472.CAN-15-3114
137. de la Motte CA. Hyaluronan in intestinal homeostasis and inflammation: implications for fibrosis. Am J Physiol Gastrointest Liver Physiol. (2011) 301:G945–9. doi: 10.1152/ajpgi.00063.2011
138. de la Motte CA, Hascall VC, Calabro A, Yen-Lieberman B, Strong SA. Mononuclear leukocytes preferentially bind via CD44 to hyaluronan on human intestinal mucosal smooth muscle cells after virus infection or treatment with poly(I.C). J Biol Chem. (1999) 274:30747–55. doi: 10.1074/jbc.274.43.30747
139. Evanko SP, Potter-Perigo S, Johnson PY, Wight TN. Organization of hyaluronan and versican in the extracellular matrix of human fibroblasts treated with the viral mimetic poly I:C. J Histochem Cytochem. (2009) 57:1041–60. doi: 10.1369/jhc.2009.953802
140. Lauer ME, Fulop C, Mukhopadhyay D, Comhair S, Erzurum SC, Hascall VC. Airway smooth muscle cells synthesize hyaluronan cable structures independent of inter-alpha-inhibitor heavy chain attachment. J Biol Chem. (2009) 284:5313–23. doi: 10.1074/jbc.M807979200
141. Lauer ME, Mukhopadhyay D, Fulop C, de la Motte CA, Majors AK, Hascall VC. Primary murine airway smooth muscle cells exposed to poly(I,C) or tunicamycin synthesize a leukocyte-adhesive hyaluronan matrix. J Biol Chem. (2009) 284:5299–312. doi: 10.1074/jbc.M807965200.
142. Majors AK, Austin RC, de la Motte CA, Pyeritz RE, Hascall VC, Kessler SP, et al. Endoplasmic reticulum stress induces hyaluronan deposition and leukocyte adhesion. J Biol Chem. (2003) 278:47223–31. doi: 10.1074/jbc.M304871200
143. Wang A, Hascall VC. Hyaluronan structures synthesized by rat mesangial cells in response to hyperglycemia induce monocyte adhesion. J Biol Chem. (2004) 279:10279–85. doi: 10.1074/jbc.M312045200
144. Lauer ME, Erzurum SC, Mukhopadhyay D, Vasanji A, Drazba J, Wang A, et al. Differentiated murine airway epithelial cells synthesize a leukocyte-adhesive hyaluronan matrix in response to endoplasmic reticulum stress. J Biol Chem. (2008) 283:26283–96. doi: 10.1074/jbc.M803350200
145. Evanko SP, Potter-Perigo S, Bollyky PL, Nepom GT, Wight TN. Hyaluronan and versican in the control of human T-lymphocyte adhesion and migration. Matrix Biol. (2012) 31:90–100. doi: 10.1016/j.matbio.2011.10.004
146. Selbi W, de la Motte CA, Hascall VC, Day AJ, Bowen T, Phillips AO. Characterization of hyaluronan cable structure and function in renal proximal tubular epithelial cells. Kidney Int. (2006) 70:1287–95. doi: 10.1038/sj.ki.5001760
147. Wang A, de la Motte C, Lauer M, Hascall V. Hyaluronan matrices in pathobiological processes. FEBS J. (2011) 278:1412–8. doi: 10.1111/j.1742-4658.2011.08069.x
148. Fujii K, Karpova MB, Asagoe K, Georgiev O, Dummer R, Urosevic-Maiwald M. Versican upregulation in Sezary cells alters growth, motility and resistance to chemotherapy. Leukemia. (2015) 29:2024–32. doi: 10.1038/leu.2015.103
149. McMahon M, Ye S, Izzard L, Dlugolenski D, Tripp RA, Bean AG, et al. ADAMTS5 Is a critical regulator of virus-specific T cell immunity. PLoS Biol. (2016) 14:e1002580. doi: 10.1371/journal.pbio.1002580
150. Merrilees MJ, Zuo N, Evanko SP, Day AJ, Wight TN. G1 domain of versican regulates hyaluronan organization and the phenotype of cultured human dermal fibroblasts. J Histochem Cytochem. (2016) 64:353–63. doi: 10.1369/0022155416643913
151. Olsen NJ, Moore JH, Aune TM. Gene expression signatures for autoimmune disease in peripheral blood mononuclear cells. Arthritis Res Ther. (2004) 6:120–8. doi: 10.1136/ard.2003.017194
152. Shou J, Bull CM, Li L, Qian HR, Wei T, Luo S, et al. Identification of blood biomarkers of rheumatoid arthritis by transcript profiling of peripheral blood mononuclear cells from the rat collagen-induced arthritis model. Arthritis Res Ther. (2006) 8:R28. doi: 10.1186/ar1883
153. Wingrove JA, Daniels SE, Sehnert AJ, Tingley W, Elashoff MR, Rosenberg S, et al. Correlation of peripheral-blood gene expression with the extent of coronary artery stenosis. Circ Cardiovasc Genet. (2008) 1:31–8. doi: 10.1161/CIRCGENETICS.108.782730
154. Toeda K, Nakamura K, Hirohata S, Hatipoglu OF, Demircan K, Yamawaki H, et al. Versican is induced in infiltrating monocytes in myocardial infarction. Mol Cell Biochem. (2005) 280:47–56. doi: 10.1007/s11010-005-8051-4
155. Asplund A, Ostergren-Lunden G, Camejo G, Stillemark-Billton P, Bondjers G. Hypoxia increases macrophage motility, possibly by decreasing the heparan sulfate proteoglycan biosynthesis. J Leukoc Biol. (2009) 86:381–8. doi: 10.1189/jlb.0908536
156. Asplund A, Friden V, Stillemark-Billton P, Camejo G, Bondjers G. Macrophages exposed to hypoxia secrete proteoglycans for which LDL has higher affinity. Atherosclerosis. (2011) 215:77–81. doi: 10.1016/j.atherosclerosis.2010.12.017
157. Lang R, Patel D, Morris JJ, Rutschman RL, Murray PJ. Shaping gene expression in activated and resting primary macrophages by IL-10. J Immunol. (2002) 169:2253–63. doi: 10.4049/jimmunol.169.5.2253
158. Martinez FO, Gordon S, Locati M, Mantovani A. Transcriptional profiling of the human monocyte-to-macrophage differentiation and polarization: new molecules and patterns of gene expression. J Immunol. (2006) 177:7303–11. doi: 10.4049/jimmunol.177.10.7303
159. Pappas AG, Magkouta S, Pateras IS, Skianis I, Moschos C, Vazakidou ME, et al. Versican modulates tumor-associated macrophage properties to stimulate mesothelioma growth. Oncoimmunology. (2019) 8:e1537427. doi: 10.1080/2162402X.2018.1537427
160. Bogen O, Dina OA, Gear RW, Levine JD. Dependence of monocyte chemoattractant protein 1 induced hyperalgesia on the isolectin B4-binding protein versican. Neuroscience. (2009) 159:780–6. doi: 10.1016/j.neuroscience.2008.12.049
161. Said N, Sanchez-Carbayo M, Smith SC, Theodorescu D. RhoGDI2 suppresses lung metastasis in mice by reducing tumor versican expression and macrophage infiltration. J Clin Invest. (2012) 122:1503–18. doi: 10.1172/JCI61392
162. Said N, Theodorescu D. RhoGDI2 suppresses bladder cancer metastasis via reduction of inflammation in the tumor microenvironment. Oncoimmunology. (2012) 1:1175–7. doi: 10.4161/onci.20594
163. Villani AC, Satija R, Reynolds G, Sarkizova S, Shekhar K, Fletcher J, et al. Single-cell RNA-seq reveals new types of human blood dendritic cells, monocytes, and progenitors. Science. (2017) 356:eaah4573. doi: 10.1126/science.aah4573
164. Hanahan D, Weinberg RA. Hallmarks of cancer: the next generation. Cell. (2011) 144:646–74. doi: 10.1016/j.cell.2011.02.013
165. Du WW, Yang W, Yee AJ. Roles of versican in cancer biology–tumorigenesis, progression and metastasis. Histol Histopathol. (2013) 28:701–13. doi: 10.14670/HH-28.701
166. Grivennikov SI, Greten FR, Karin M. Immunity, inflammation, and cancer. Cell. (2010) 140:883–99. doi: 10.1016/j.cell.2010.01.025
167. Dos Reis DC, Damasceno KA, de Campos CB, Veloso ES, Pegas GRA, Kraemer LR, et al. Versican and tumor-associated macrophages promotes tumor progression and metastasis in canine and murine models of breast carcinoma. Front Oncol. (2019) 9:577. doi: 10.3389/fonc.2019.00577
168. Keire PA, Bressler SL, Lemire JM, Edris B, Rubin BP, Rahmani M, et al. A role for versican in the development of leiomyosarcoma. J Biol Chem. (2014) 289:34089–103. doi: 10.1074/jbc.M114.607168
169. Derynck R, Goeddel DV, Ullrich A, Gutterman JU, Williams RD, Bringman TS, et al. Synthesis of messenger RNAs for transforming growth factors alpha and beta and the epidermal growth factor receptor by human tumors. Cancer Res. (1987) 47:707–12.
170. Van Bockstal M, Lambein K, Van Gele M, De Vlieghere E, Limame R, Braems G, et al. Differential regulation of extracellular matrix protein expression in carcinoma-associated fibroblasts by TGF-beta1 regulates cancer cell spreading but not adhesion. Oncoscience. (2014) 1:634–48. doi: 10.18632/oncoscience.87
171. Kodama J, Hasengaowa, Kusumoto T, Seki N, Matsuo T, Nakamura K, et al. Versican expression in human cervical cancer. Eur J Cancer. (2007) 43:1460–6. doi: 10.1016/j.ejca.2007.02.007
172. Kodama J, Hasengaowa, Kusumoto T, Seki N, Matsuo T, Ojima Y, et al. Prognostic significance of stromal versican expression in human endometrial cancer. Ann Oncol. (2007) 18:269–74. doi: 10.1093/annonc/mdl370
173. Fanhchaksai K, Okada F, Nagai N, Pothacharoen P, Kongtawelert P, Hatano S, et al. Host stromal versican is essential for cancer-associated fibroblast function to inhibit cancer growth. Int J Cancer. (2016) 138:630–41. doi: 10.1002/ijc.29804
174. Ozdemir BC, Pentcheva-Hoang T, Carstens JL, Zheng X, Wu CC, Simpson TR, et al. Depletion of carcinoma-associated fibroblasts and fibrosis induces immunosuppression and accelerates pancreas cancer with reduced survival. Cancer Cell. (2014) 25:719–34. doi: 10.1016/j.ccr.2014.04.005
175. Asano K, Nelson CM, Nandadasa S, Aramaki-Hattori N, Lindner DJ, Alban T, et al. Stromal versican regulates tumor growth by promoting angiogenesis. Sci Rep. (2017) 7:17225. doi: 10.1038/s41598-017-17613-6
176. Ghosh S, Albitar L, LeBaron R, Welch WR, Samimi G, Birrer MJ, et al. Up-regulation of stromal versican expression in advanced stage serous ovarian cancer. Gynecol Oncol. (2010) 119:114–20. doi: 10.1016/j.ygyno.2010.05.029
177. Labropoulou VT, Theocharis AD, Ravazoula P, Perimenis P, Hjerpe A, Karamanos NK, et al. Versican but not decorin accumulation is related to metastatic potential and neovascularization in testicular germ cell tumours. Histopathology. (2006) 49:582–93. doi: 10.1111/j.1365-2559.2006.02558.x
178. Kreutziger KL, Muskheli V, Johnson P, Braun K, Wight TN, Murry CE. Developing vasculature and stroma in engineered human myocardium. Tissue Eng Part A. (2011) 17:1219–28. doi: 10.1089/ten.TEA.2010.0557
179. Fu Y, Nagy JA, Brown LF, Shih SC, Johnson PY, Chan CK, et al. Proteolytic cleavage of versican and involvement of ADAMTS-1 in VEGF-A/VPF-induced pathological angiogenesis. J Histochem Cytochem. (2011) 59:463–73. doi: 10.1369/0022155411401748
180. Gao D, Vahdat LT, Wong S, Chang JC, Mittal V. Microenvironmental regulation of epithelial-mesenchymal transitions in cancer. Cancer Res. (2012) 72:4883–9. doi: 10.1158/0008-5472.CAN-12-1223
181. Gao D, Joshi N, Choi H, Ryu S, Hahn M, Catena R, et al. Myeloid progenitor cells in the premetastatic lung promote metastases by inducing mesenchymal to epithelial transition. Cancer Res. (2012) 72:1384–94. doi: 10.1158/0008-5472.CAN-11-2905
182. Gutmann DH. Microglia in the tumor microenvironment: taking their TOLL on glioma biology. Neuro Oncol. (2015) 17:171–3. doi: 10.1093/neuonc/nou346
183. Senda M, Fukuyama R, Nagasaka T. Kinetics of versican-expressing macrophages in bone marrow after cord blood stem cell transplantation for treatment of acute myelogenous leukaemia. J Clin Pathol. (2016) 69:906–11. doi: 10.1136/jclinpath-2015-203496
184. Hope C, Ollar SJ, Heninger E, Hebron E, Jensen JL, Kim J, et al. TPL2 kinase regulates the inflammatory milieu of the myeloma niche. Blood. (2014) 123:3305–15. doi: 10.1182/blood-2014-02-554071
185. Arana P, Zabaleta A, Lasa M, Maiso P, Alignani D, Jelinek T, et al. High-throughput characterization and new insight into the role of tumor associated macrophages (TAMs) in multiple myeloma (MM). Blood. (2016) 128:482. doi: 10.1182/blood.V128.22.482.482
186. Spranger S, Dai D, Horton B, Gajewski TF. Tumor-residing Batf3 dendritic cells are required for effector T cell trafficking and adoptive T cell therapy. Cancer Cell. (2017) 31:711–23 e4. doi: 10.1016/j.ccell.2017.04.003
187. Dhakal B, Pagenkopf A, Umair Mushtaq M, Cunningham AM, Flietner E, Morrow Z, et al. Versican proteolysis predicts immune effector infiltration and post-transplant survival in myeloma. Leuk Lymphoma. (2019) 60:2558–62. doi: 10.1080/10428194.2019.1585836
188. Binder MJ, McCoombe S, Williams ED, McCulloch DR, Ward AC. The extracellular matrix in cancer progression: Role of hyalectan proteoglycans and ADAMTS enzymes. Cancer Lett. (2017) 385:55–64. doi: 10.1016/j.canlet.2016.11.001
189. Dvorak HF. Tumors: wounds that do not heal. Similarities between tumor stroma generation and wound healing. N Engl J Med. (1986) 315:1650–9.
190. Dvorak HF. Tumors: wounds that do not heal-redux. Cancer Immunol Res. (2015) 3:1–11. doi: 10.1158/2326-6066.CIR-14-0209
191. Joyce JA, Fearon DT. T cell exclusion, immune privilege, and the tumor microenvironment. Science. (2015) 348:74–80. doi: 10.1126/science.aaa6204
192. Gorter A, Zijlmans HJ, van Gent H, Trimbos JB, Fleuren GJ, Jordanova ES. Versican expression is associated with tumor-infiltrating CD8-positive T cells and infiltration depth in cervical cancer. Mod Pathol. (2010) 23:1605–15. doi: 10.1038/modpathol.2010.154
193. Hartley G, Regan D, Guth A, Dow S. Regulation of PD-L1 expression on murine tumor-associated monocytes and macrophages by locally produced TNF-alpha. Cancer Immunol Immunother. (2017) 66:523–35. doi: 10.1007/s00262-017-1955-5
194. Jiang H, Hegde S, DeNardo DG. Tumor-associated fibrosis as a regulator of tumor immunity and response to immunotherapy. Cancer Immunol Immunother. (2017) 66:1037–48. doi: 10.1007/s00262-017-2003-1
195. Nicholas NS, Apollonio B, Ramsay AG. Tumor microenvironment (TME)-driven immune suppression in B cell malignancy. Biochim Biophys Acta. (2016) 1863:471–82. doi: 10.1016/j.bbamcr.2015.11.003
196. Tang H, Wang Y, Chlewicki LK, Zhang Y, Guo J, Liang W, et al. Facilitating T cell infiltration in tumor microenvironment overcomes resistance to PD-L1 blockade. Cancer Cell. (2016) 29:285–96. doi: 10.1016/j.ccell.2016.02.004
197. Hernandez D, Miquel-Serra L, Docampo MJ, Marco-Ramell A, Cabrera J, Fabra A, et al. V3 versican isoform alters the behavior of human melanoma cells by interfering with CD44/ErbB-dependent signaling. J Biol Chem. (2011) 286:1475–85. doi: 10.1074/jbc.M110.127522
198. Miquel-Serra L, Serra M, Hernández D, Domenzain C, Docampo MJ, Rabanal R, et al. V3 versican isoform expression has a dual role in human melanoma tumor growth and metastasis. Lab Invest. (2006) 86:889–901. doi: 10.1038/labinvest.3700449
Keywords: versican, hyaluronan, immunity, inflammation, macrophages, T lymphocytes
Citation: Wight TN, Kang I, Evanko SP, Harten IA, Chang MY, Pearce OMT, Allen CE and Frevert CW (2020) Versican—A Critical Extracellular Matrix Regulator of Immunity and Inflammation. Front. Immunol. 11:512. doi: 10.3389/fimmu.2020.00512
Received: 08 January 2020; Accepted: 06 March 2020;
Published: 24 March 2020.
Edited by:
Aaron C. Petrey, The University of Utah, United StatesReviewed by:
Anna Maria Piccinini, University of Nottingham, United KingdomKatherina Psarra, Evaggelismos General Hospital, Greece
Copyright © 2020 Wight, Kang, Evanko, Harten, Chang, Pearce, Allen and Frevert. This is an open-access article distributed under the terms of the Creative Commons Attribution License (CC BY). The use, distribution or reproduction in other forums is permitted, provided the original author(s) and the copyright owner(s) are credited and that the original publication in this journal is cited, in accordance with accepted academic practice. No use, distribution or reproduction is permitted which does not comply with these terms.
*Correspondence: Thomas N. Wight, dHdpZ2h0QGJlbmFyb3lhcmVzZWFyY2gub3Jn