- 1National Institutes of Health-NIRT-International Center for Excellence in Research, Chennai, India
- 2National Institute for Research in Tuberculosis (NIRT), Chennai, India
- 3Government Stanley Medical Hospital, Chennai, India
- 4Laboratory of Parasitic Diseases, National Institute of Allergy and Infectious Diseases, National Institutes of Health, Bethesda, MD, United States
- 5Frederick National Laboratory for Cancer Research Sponsored by the National Cancer Institute, Frederick, MD, United States
Filarial infections are known to modulate cytokine responses in pulmonary tuberculosis by their propensity to induce Type 2 and regulatory cytokines. However, very little is known about the effect of filarial infections on extra-pulmonary forms of tuberculosis. Thus, we have examined the effect of filarial infections on the plasma levels of various families of (IL-1, IL-12, γC, and regulatory) cytokines and (CC and CXC) chemokines in tuberculous lymphadenitis coinfection. We also measured lymph node culture grades in order to assess the burden of Mycobacterium tuberculosis in the two study groups [Fil+ (n = 67) and Fil– (n = 109)]. Our data reveal that bacterial burden was significantly higher in Fil+ compared to Fil– individuals. Plasma levels of IL-1 family (IL-1α, IL-β, IL-18) cytokines were significantly lower with the exception of IL-33 in Fil+ compared to Fil– individuals. Similarly, plasma levels of IL-12 family cytokines -IL-12 and IL-23 were significantly reduced, while IL-35 was significantly elevated in Fil+ compared to Fil– individuals. Filarial infection was also associated with diminished levels of IL-2, IL-9 and enhanced levels of IL-4, IL-10, and IL-1Ra. Similarly, the Fil+ individuals were linked to elevated levels of different CC (CCL-1, CCL-2, CCL-3, CCL-11) and CXC (CXCL-2, CXCL-8, CXCL-9, CXCL-11) chemokines. Therefore, we conclude that filarial infections exert powerful bystander effects on tuberculous lymphadenitis, effects including modulation of protective cytokines and chemokines with a direct impact on bacterial burdens.
Introduction
Lymphatic filariasis is a tropical disease afflicting about 70 million people worldwide (1). It is an infection caused by the nematodes, Wuchereria bancrofti, Brugia malayi, and Brugia timori and is transmitted by mosquitoes (2). Filarial infections occur predominantly in tropical regions of the world where pulmonary and extra-pulmonary tuberculosis (TB) are co-prevalent (3). Tuberculous lymphadenitis (TBL) is the most common manifestation of extra-pulmonary TB and constitutes 35% of all forms of extra-pulmonary TB (4). The incidence of TBL has increased in parallel with the increase of mycobacterial infections worldwide. Cervical lymphadenopathy is the most common manifestation of TBL but other lymph nodes including inguinal, axillary, mesenteric and mediastinal can be involved (5). The pathogenesis of TBL is still poorly understood (5).
Cytokines are crucial players in host resistance to both pulmonary TB and TBL (6, 7). Thus, Type 1, Type 17 and other pro-inflammatory cytokines (including IL-1, IL-12, and γC family of cytokines) are important for host protection, while Type 2 and regulatory cytokines are detrimental for host immunity against active TB (7). A variety of chemokines are known to play an important role in the immune response to active TB (8, 9). Filarial infections are known to modulate cytokine and chemokine responses in both active and latent tuberculosis (TB) (3). However, the effect of filarial infections on the cytokine and chemokine response to TBL is not known.
Therefore, we aimed to study the cytokine and chemokine profile established during filarial infection to assess its impact on TBL disease. To address this, we measured the plasma levels of different families of (IL-1, IL-12, γC, and regulatory) cytokines and (CC and CXC) chemokines in filaria-TBL coinfection. We show that filarial coinfected individuals have significantly increased bacterial burden in the affected lymph nodes when compared to filarial-uninfected individuals. Our results also show significantly altered cytokine and chemokine responses in filaria-TBL coinfection.
Results
Study Population Demographics
The demographics of the study population are listed in (Table 1). There were no significant differences in age and gender between Fil+ and Fil– individuals. Neither age nor gender had any significant effect on the cytokine or chemokine profiles. However, Fil+ individuals had moderately higher bacterial burdens as determined by the culture grade in solid media of the affected lymph node (p = 0.045). Hence, filarial coinfection is associated with higher bacterial burdens in TBL.
Fil+ Individuals Exhibit Significantly Diminished Levels of IL-1α, IL-1β, IL-18, IL-12, and IL-23 and Significantly Enhanced Levels of IL-33 and IL-35
Filarial infection is associated with diminished levels of pro-inflammatory cytokines in pulmonary TB (3). To assess the impact of coincident filarial infection on IL-1 family (IL-1α, IL-1β, IL-18, IL-33) and IL-12 family (IL-12, IL-23, IL-27, IL-35) of cytokines in TBL individuals, we measured the plasma levels of these cytokines (Figure 1). The plasma levels of IL-1α (Geometric mean (GM) of 83.82 vs. 123.4 pg/ml, p = 0.0047), IL-β (GM of 16.78 vs. 21.27 pg/ml, p < 0.0001) and IL-18 (GM of 167.7 vs. 310.0 pg/ml, p < 0.0001) were significantly lower in Fil+ compared to Fil– individuals. In contrast, the plasma levels of IL-33 (GM of 749.5 vs. 545.9 pg/ml, p < 0.0001) was significantly higher in Fil+ compared to Fil– individuals (Figure 1A). The plasma levels of IL-12 (GM of 82.12 vs. 428.3 pg/ml, p < 0.0001) and IL-23 (GM of 12.89 vs. 19.09 pg/ml, p < 0.0001) were significantly lower and the plasma levels of IL-35 (GM of 27.44 vs. 17.63 pg/ml, p < 0.0001) was significantly higher in Fil+ compared to Fil- individuals (Figure 1B). Hence, filarial coinfection is characterized by altered plasma levels of IL-1 and IL-12 family of cytokines.
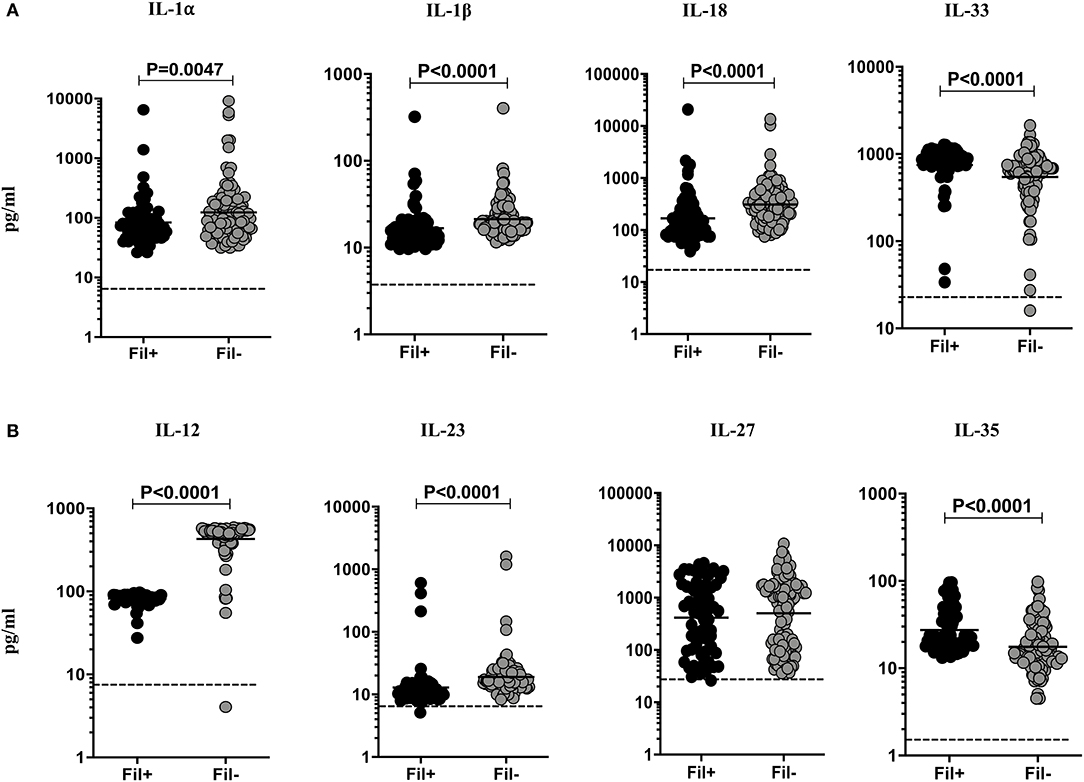
Figure 1. Altered plasma levels of IL-1 and IL-12 family of cytokines in filarial coinfected individuals with TBL. The plasma levels of (A) IL-1 (IL-1α, IL-1β, IL-18, and IL-33) and (B) IL-12 family (IL-12, IL-23, IL-27, and IL-35) of cytokines were measured in Fil+ (n = 67) and Fil– (n = 109) individuals with TBL. The results are represented as scatter plots with each circle representing a single individual. The bar indicates the geometric mean and the significant differences were calculated using non-parametric Mann-Whitney U test. The dotted line indicates the limit of detection for each cytokine.
Fil+ Individuals Exhibit Significantly Diminished Levels of IL-2 and IL-9 and Significantly Enhanced Levels of IL-4
Filarial infections are associated with alterations in the γc family of cytokines in pulmonary TB (3). Hence, we measured the circulating levels of γc family (IL-2, IL-4, IL-7, IL-9, IL-15, and IL-21) of cytokines to examine the influence of filarial coinfection among TBL individuals (Figure 2). The plasma levels of IL-2 (GM of 49.02 vs. 82.09 pg/ml, p < 0.0001) and IL-9 (GM of 15.26 vs. 38.79 pg/ml, p < 0.0001) were significantly lower and the plasma levels of IL-4 (GM of 41.93 vs. 10.18 pg/ml, p < 0.0001) was significantly higher in Fil+ compared to Fil– individuals. In contrast, there were no significant differences in the levels of IL-7, IL-15, and IL-21 between the two groups (Figure 2). Hence, filarial coinfection is characterized by altered plasma levels of γc family of cytokines.
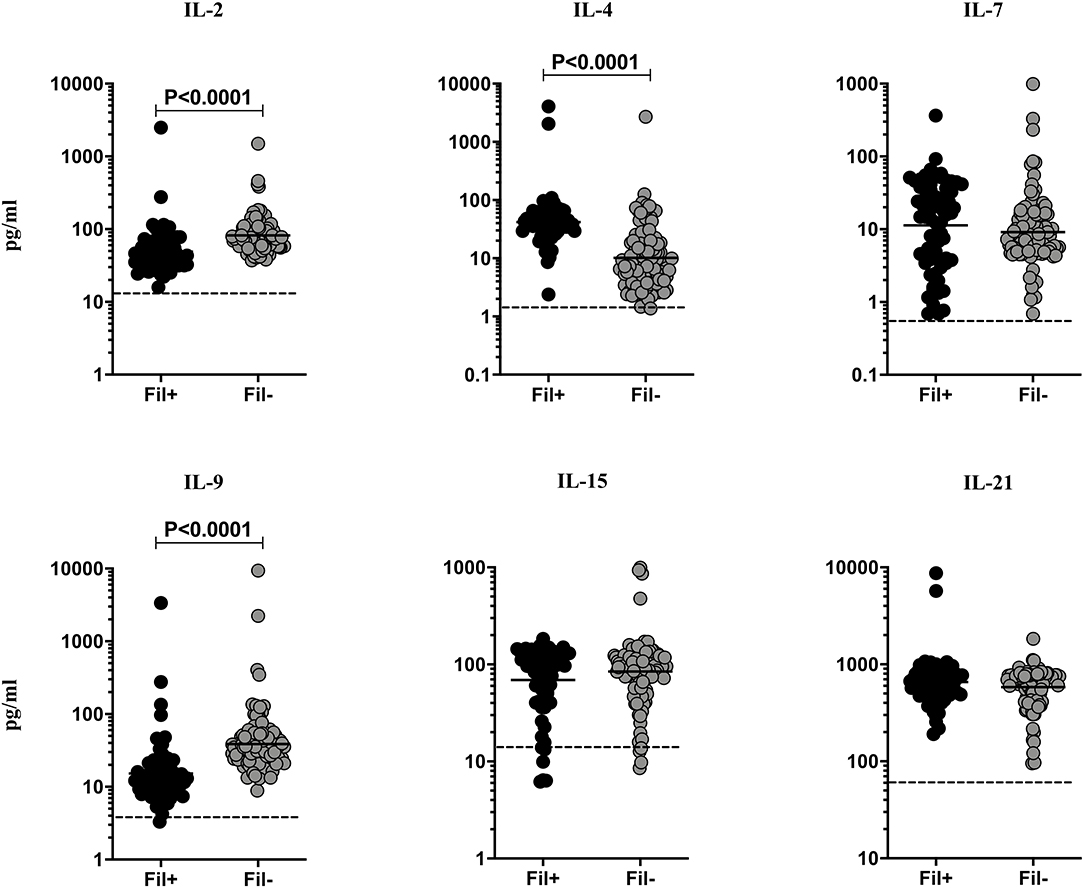
Figure 2. Altered plasma levels of γC cytokines in filarial coinfected individuals with TBL. The plasma levels of γC family (IL-2, IL-4, IL-7, IL-9, IL-15, and IL-21) of cytokines were measured in Fil+ (n = 67) and Fil– (n = 109) individuals with TBL. The results are represented as scatter plots with each circle representing a single individual. The bar indicates the geometric mean and the significant differences were calculated using non-parametric Mann-Whitney U test. The dotted line indicates the limit of detection for each cytokine.
Fil+ Individuals Exhibit Significantly Enhanced Levels of IL-10 and IL-1Ra
Filarial infection is associated with elevated regulatory cytokines (IL-10 and TGF β) in pulmonary TB (3). Hence, we studied the influence of filarial coinfection on regulatory cytokines in TBL, by measuring the plasma levels of these cytokines (Figure 3). The plasma levels of IL-10 (GM of 366.9 vs. 102.0 pg/ml, p < 0.0001) and IL-1Ra (GM of 1148.0 vs. 755.7 pg/ml, p = 0.0136) were significantly higher in Fil+ compared to Fil– individuals. In contrast, there were no significant differences in the levels of TGF β between the two groups (Figure 3). Hence, filarial coinfection is characterized by increased plasma levels of regulatory cytokines.
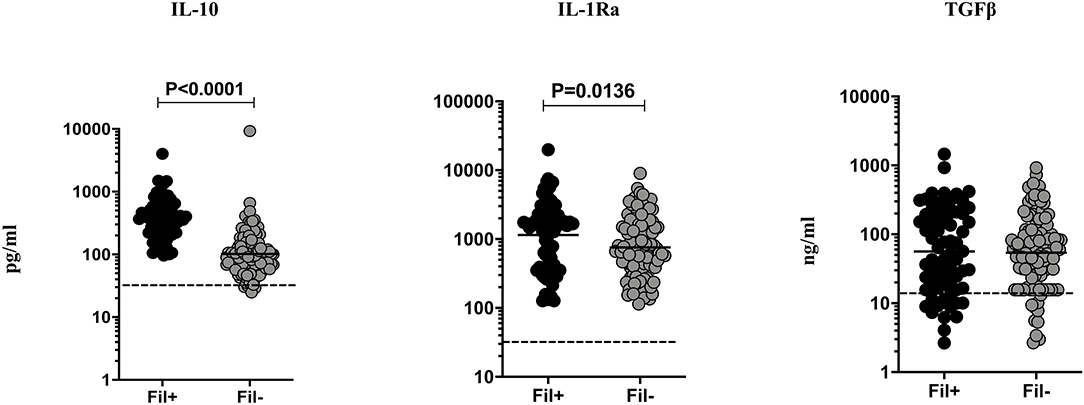
Figure 3. Elevated plasma levels of regulatory cytokines in filarial coinfected individuals with TBL. The plasma levels of regulatory (IL-10, IL-1Ra, and TGF-β) cytokines were measured in Fil+ (n = 67) and Fil– (n = 109) individuals with TBL. The results are represented as scatter plots with each circle representing a single individual. The bar indicates the geometric mean and the significant differences were calculated using non-parametric Mann-Whitney U test. The dotted line indicates the limit of detection for each cytokine.
Fil+ Individuals Exhibit Significantly Enhanced Levels of CC Chemokines
Helminth infections are associated with diminished CC chemokines in latent TB (10). To study the association of filarial coinfection on CC chemokines in TBL, we measured the plasma levels of CCL1, CCL2, CCL3, and CCL11 (Figure 4). The plasma levels of CCL1 (GM of 24.64 vs. 11.31 pg/ml, p = 0.0024), CCL2 (GM of 33.59 vs. 10.43 pg/ml, p < 0.0001) and CCL11 (GM of 169.7 vs. 43.82 pg/ml, p < 0.0001) were significantly higher in Fil+ compared to Fil– individuals (Figure 4). Hence, filarial coinfection is characterized by increased plasma levels of CC chemokines.
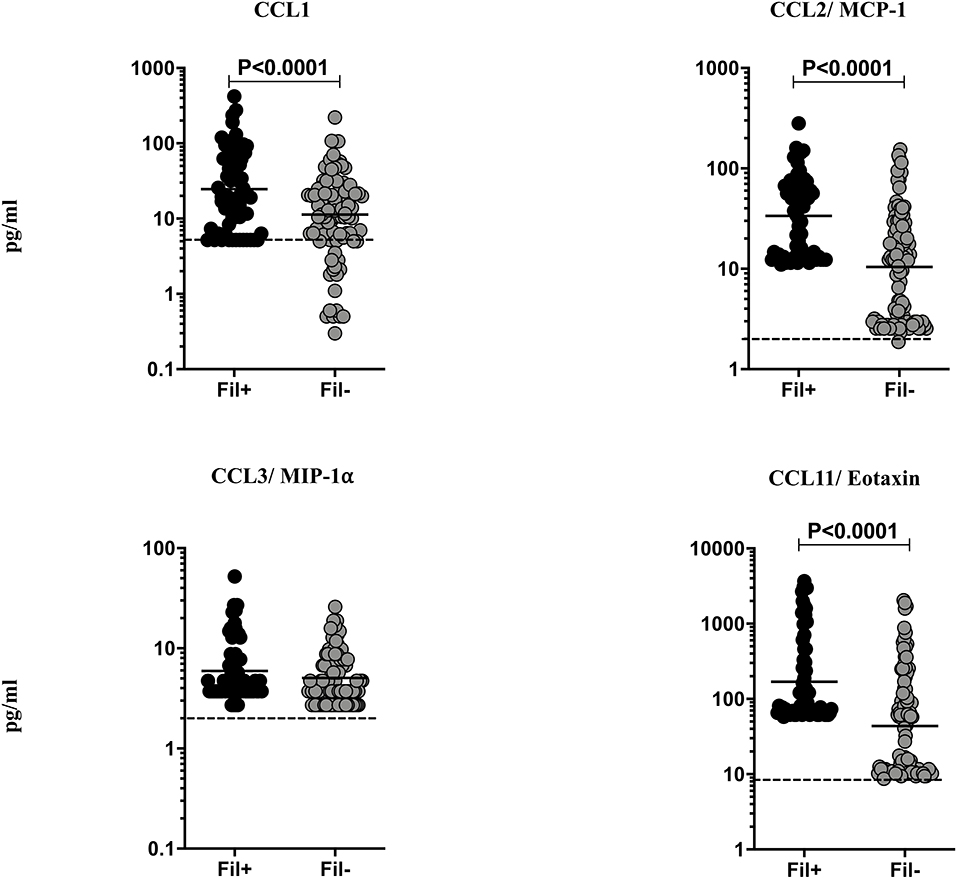
Figure 4. Elevated plasma levels of CC chemokines in filarial coinfected individuals with TBL. The plasma levels of CC (CCL1, CCL-2/MCP-1, CCL3/MIP1α, and CCL11/eotaxin) chemokines were measured in Fil+ (n = 67) and Fil– (n = 109) individuals with TBL. The results are represented as scatter plots with each circle representing a single individual. The bar indicates the geometric mean and the significant differences were calculated using non-parametric Mann-Whitney U test. The dotted line indicates the limit of detection for each chemokine.
Fil+ Individuals Exhibit Significantly Enhanced Levels of Most CXC Chemokines
Helminth infections are associated with diminished CXC chemokines in latent TB (10). To study the association of filarial coinfection on CXC chemokines in TBL, we measured the systemic levels of CXCL1, CXCL2, CXCL8, CXCL9, CXCL10, and CXCL11 (Figure 5). The plasma levels of CXCL2 (GM of 74.75 vs. 12.04 pg/ml, p < 0.0001), CXCL8 (GM of 92.34 vs. 19.64 pg/ml, p < 0.0001), CXCL9 (GM of 199.4 vs. 48.40 pg/ml, p < 0.0001) and CXCL11 (GM of 43.07 vs. 10.27 pg/ml, p < 0.0001) were significantly higher and plasma levels of CXCL1 (GM of 69.71 vs. 104.1 pg/ml, p < 0.0001) was significantly lower in Fil+ compared to Fil– individuals (Figure 5). Hence, filarial coinfection is characterized by increased plasma levels of most CXC chemokines.
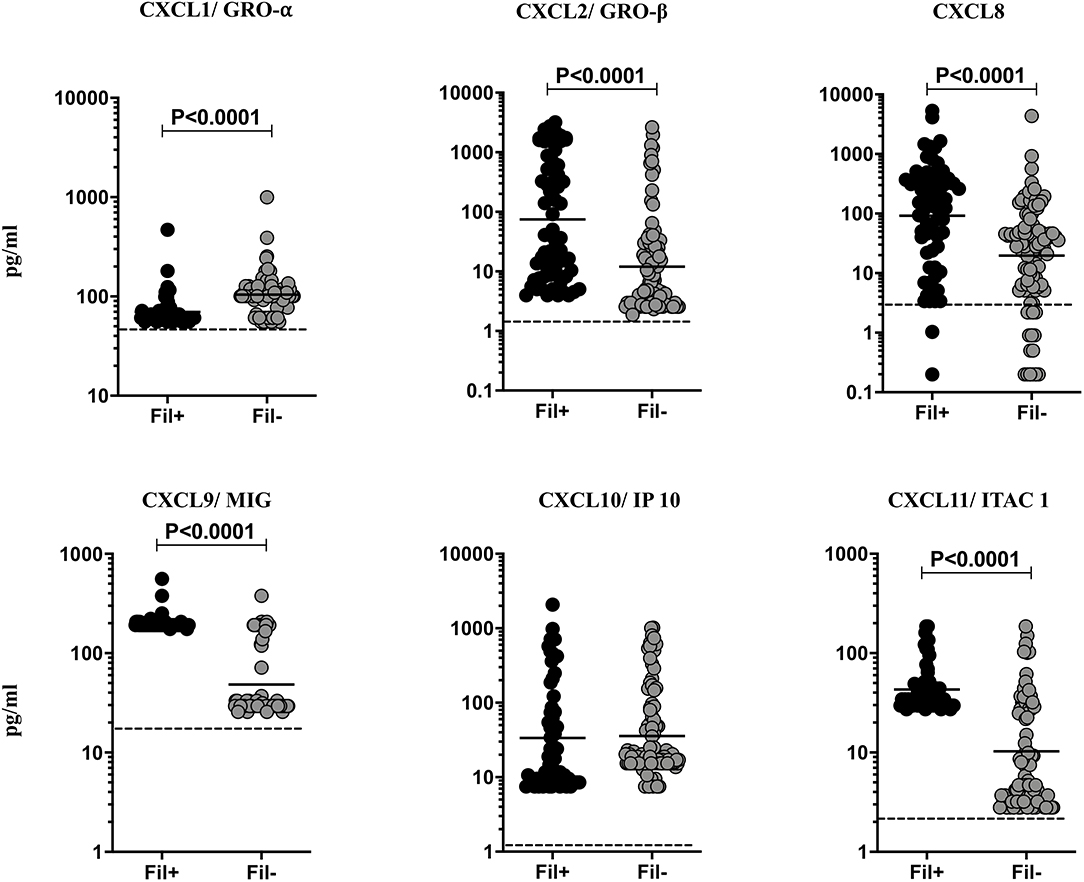
Figure 5. Elevated plasma levels of CXC chemokines in filarial coinfected individuals with TBL. The plasma levels of CXC (CXCL1/GRO-α, CXCL2/GRO-β, CXCL9/MIG, CXCL8, CXCL10/IP-10, CXCL11/ITAC1) chemokines were measured in Fil+ (n = 67) and Fil– (n = 109) individuals with TBL. The results are represented as scatter plots with each circle representing a single individual. The bar indicates the geometric mean and the significant differences were calculated using non-parametric Mann-Whitney U test. The dotted line indicates the limit of detection for each chemokine.
Discussion
Extra-pulmonary TB accounts for ~20% of all TB cases and lymph nodes are often the most common site (4). Previous studies have shown that helminth infections can influence the pathogenesis and immune response to pulmonary TB (3, 11). To our knowledge, our study is the first to examine the bacteriological and immunological outcomes in filaria-TBL coinfections and the second to examine the same in helminth-TBL coinfections in total (12). Our previous study had demonstrated that Stronglyoides stercoralis coinfection could modulate cytokine (but not chemokine) responses both systemically and in an antigen—specific manner (12). We had also demonstrated elevated bacterial burdens in that helminth-TBL coinfection (12). We expand our data on those findings and report the influence of filarial coinfection on the cytokine and chemokine response in TBL disease. We also validate our previous report that co-infected helminth infection is associated with higher bacterial burdens in the affected lymph node of TBL individuals. This study offers new insights into the pathogenesis of TBL disease in the presence of co-prevalent helminth infections and suggests that treatment of helminth infections could influence the outcome of TBL disease.
Among cytokines involved in host immunity to TB disease, the IL-1 and IL-12 family of cytokines play a major role. IL-1α, IL-1β, and IL-18 are known to be essential for immunity to TB infection (13, 14). Both IL-1 cytokines signal through the same IL-1 receptor and are known to be independently required for host resistance and deficiency in either cytokine renders mice more susceptible to TB infection (15). Similarly, IL-18 is important for host immunity and functions by induction of IFNγ (16). Finally, TBL is characterized by diminished plasma and TB—antigen stimulated levels of IL-1β and IL-18 (17). Our data therefore suggest that in the presence of filarial infection, protective cytokines are dampened in the circulation of TBL individuals. In contrast, IL-33 is a predominantly anti-inflammatory cytokine belonging to the IL-1 family (18). It is mostly expressed in non-hematopoietic cells like adipocytes, fibroblasts, intestinal and bronchial epithelial cells and endothelial cells (19–21). IL-33 is also expressed by hematopoietic cells mainly by dendritic cells (DCs) to lesser extent (22). IL-33 acts an alarmin in the induction of Type 2 cytokine responses (23) and is known to help attenuate ongoing TB infection in mice (24). Since IL-33 is induced in a variety of Th2 settings, it is not surprising to see that IL-33 levels were enhanced in filarial coinfected individuals. However, whether IL-33 has a beneficial or detrimental effect on the TBL disease remains to be determined.
IL-12 and IL-23 are the two primary cytokines fundamentally important in resistance to TB infection as demonstrated both by animal models and human immune deficiencies (25–30). IL-12 by its induction of Type 1 responses and IL-23 by driving Type 17 cytokine responses are critical in host immunity to TB. Our data showing diminished levels of both the cytokines also suggest that filarial infections modulate immunity in TBL by decreasing Type 1 and Type 17 cytokine responses. IL-27 has an important role in immunity to TB (31) but is not modulated in the presence of coinfection. Finally, IL-35 is an anti-inflammatory cytokine of the IL-12 family (32), whose role in TB is not clear. Our data suggest that filarial modulation of TBL disease results in higher levels of IL-35, which might be potentially host-detrimental. The role of γc cytokines in host immunity to TB infection and disease is not well understood. IL-2 and IL-21 have been shown to be important to host resistance in animal models (33, 34) and regulation of these cytokines has been demonstrated in human pulmonary TB (35). Our data on the modulation of γc cytokines in the presence of filarial coinfection suggests that IL-2 and IL-9 (but not IL-7, IL-15 or IL-21) levels are significantly diminished. The implication of the alterations in IL-2 and IL-9 needs to be examined further.
One potential mechanism for the decrease in baseline levels of proinflammatory cytokines in TBL individuals with filarial coinfection could be a concomitant increase in regulatory cytokines. Our data suggest that IL-4, IL-10, and IL-1Ra are significantly increased in Fil+ individuals suggesting a plausible biological mechanism for the downmodulation of IL-1 and IL-12 family of cytokines. Also, since IL-4, IL-10, and IL-1Ra are known to play a detrimental role in enhancing susceptibility to infection (36–38), our data indicate that alteration of the balance between protective and regulatory cytokines plays a pivotal role in the establishment of cytokine responses in filarial-TBL coinfection. In addition, this balance could also be impacted by the elevated levels of the anti-inflammatory cytokines—IL-33 and IL-35. Interestingly, no difference in TGFβ levels was observed between coinfected and uninfected individuals in TBL disease.
Chemokines play an important role in cell migration to TB infected organs and are critical for TB control (8, 9). Indeed, productive granuloma formation is known to be tightly regulated by chemokines (8, 9). However, chemokine dysregulation can shift the balance from protection to inflammation and pathology in TB disease (8, 9). We have previously shown that plasma chemokines are markers of disease severity, bacterial burdens and delayed culture conversion in pulmonary TB (39). Our data clearly show that both CC (CCL1, CCL2, CCL11) and CXC (CXCL2, CXCL8, CXCL9, CXCL11) are clearly elevated in filarial-TBL coinfection. Due to their ability to recruit neutrophils, eosinophils and other inflammatory cell types (40), these chemokines could play a potentially pathogenic role in TBL. Finally, dysregulation of chemokine production has been shown to enhance TB cavitation and pathology and therefore, excessive circulating levels of chemokines could also contribute to this process (41).
Our study suffers from the limitation of being cross-sectional, lacking the ability to attribute cause-effect mechanisms, measuring only plasma (and not local) levels of cytokines and chemokines and not performing stool examinations for other helminth infections. Nevertheless, our study clearly shows that coexistent filarial infection has major effects on TBL bacteriology and immunology. In addition to being associated with higher bacterial burdens, filarial infections appear to downmodulate the systemic levels of key protective cytokine families and upregulate the systemic levels of key pathogenic cytokines and chemokines. Thus, by its ability to influence the cytokine and chemokine milieu in TBL, coexistent filarial infection imparts certain deleterious effects in the course of TBL disease. The role of immune cell subsets in helminth—TBL coinfection is currently under investigation. Overall, our results suggest the crucial role of filarial coinfection in modulating the necessary protective cytokines and chemokines in TBL disease. Therefore, our future approach is to study the association of those cytokines and chemokines in those groups of study individuals after the completion of anthelmintic treatment to understand the effect of anthelmintic treatment on this important component of the immune response against the TB pathogen.
Materials and Methods
Ethics
All individuals (participants above 18 years of age were enrolled in the study) were assessed as part of a natural history study protocol approved by Institutional Review Boards of National Institute for Research in Tuberculosis (NIRT, NIRTIEC2010002) and informed written consent was obtained from all participants involved in the study.
Study Population
We studied a group of 176 individuals with TBL, 67 of whom were infected with filarial infection (hereafter Fil+) and 109 of whom were negative for filarial infection and only had TBL (hereafter Fil–) (Table 1). This was a nested case control study within a larger study on the immune responses in pulmonary and extrapulmonary TB conducted at the Government Stanley Medical Hospital, Chennai. Out of 252 extrapulmonary TB cases, 176 TBL cases were used for this study. TBL diagnosis was made on the basis of excision biopsy (i.e., affected lymph nodes) showing culture positivity for M. tuberculosis. Culture was performed using homogenized lymph node tissue and culture grades [1+ (1–20 colonies)/2+ (20–100 colonies)/3+(>100 colonies)] were used to identify the bacterial burdens as ascertained by growth of M. tuberculosis on Lowenstein-Jensen solid media [11]. Culture scoring was done independently and blinded to the filarial infection status. Filarial (Wuchereria bancrofti) infection was diagnosed by assessing the filarial (both microfilaremic and amicrofilaremic) antigen levels using TropBio Og4C3 enzyme-linked immunosorbent assay (ELISA) (Trop Bio Pty. Ltd., Townsville, Queensland, Australia). The cut off range to detect filarial antigen is >128 antigen unit (U/ml) and considered as positive and <128 (U/ml) is considered as negative for the presence of filarial antigen. All the study individuals were BCG vaccinated, negative for HIV and not under any steroid treatment. We did not perform any stool microscopy examination for these individuals and they were anti-tuberculosis and anthelmintic treatment naive. All these individuals were from a peri-urban area in Chennai, India.
Plasma Collection and Measurement of Cytokines by ELISA
Blood samples (10 ml) were collected in sodium heparin tubes and plasma was collected by centrifugation at 2,600 revolutions per minute (rpm) for 10 min at 4°C and stored at −80°C. The following cytokines-IL-1α, IL-Ra, IL-1β, IL-2, IL-4, IL-7, IL-9, IL-10, IL-12, IL-15, IL-18, IL-21 (DuoSet R&D Systems), IL-23, IL-27, IL-33, IL-35 (e-Biosciences) and TGFβ (BioLegend) were measured using ELISA.
Measurement of Chemokines by Multiplex Immunoassay
Plasma chemokine levels were measured using a multiplex immunoassay system (Luminex, Biorad) with a kit from R&D Systems. The chemokines measured were: CC (CCL1, CCL2/MCP-1, CCL3/MIP1α, CCL4/MIP-1β, CCL11/eotaxin) and CXC (CXCL1/GRO-α, CXCL2/GRO-β, CXCL9/MIG, CXCL8/IL-8, CXCL10/IP-10, CXCL11/ITAC1).
Statistical Analysis
All the data were analyzed using GraphPad PRISM (GraphPad Software, Inc., San Diego, CA, USA) tool. Geometric means (GM) were used for measurements of central tendency and nonparametric Chi-square test was used to compare statistically significant differences in age, gender and bacterial burdens. Mann-Whitney U test was used to compare the statistically significant differences among the cytokines and chemokines analyzed.
Data Availability Statement
All datasets generated for this study are included in the article/supplementary material.
Ethics Statement
The studies involving human participants were reviewed and approved by Institutional Review Boards of National Institute for Research in Tuberculosis (NIRT, NIRTIEC2010002). The patients/participants provided their written informed consent to participate in this study.
Author Contributions
GK and SB: conceived and designed the experiments, analyzed the data, and wrote the paper. GK and SM: performed the experiments. VB, DN, RS, and SB: contributed materials, reagents, analysis tools.
Funding
This research was supported [in part] by the National Institutes of Health.
Conflict of Interest
The authors declare that the research was conducted in the absence of any commercial or financial relationships that could be construed as a potential conflict of interest.
Acknowledgments
The authors thank the staff of the Department of Clinical Research, NIRT and Government Stanley Hospital, Chennai, India, for valuable assistance in recruiting the patients for this study.
References
1. Specht S, Suma TK, Pedrique B, Hoerauf A. Elimination of lymphatic filariasis in South East Asia. BMJ. (2019) 364:k5198. doi: 10.1136/bmj.k5198
2. Taylor MJ, Hoerauf A, Bockarie M. Lymphatic filariasis and onchocerciasis. Lancet. (2010) 376:1175–85. doi: 10.1016/S0140-6736(10)60586-7
3. Babu S, Nutman TB. Helminth-tuberculosis co-infection: an immunologic perspective. Trends Immunol. (2016) 37:597–607. doi: 10.1016/j.it.2016.07.005
4. WHO. Global Tuberculosis Report 2018. (2019). Available online at: www.hoint/tb/data (accessed February 3, 2020)
5. Handa U, Mundi I, Mohan S. Nodal tuberculosis revisited: a review. J Infect Dev Ctries. (2012) 6:6–12. doi: 10.3855/jidc.2090
6. Cooper AM, Khader SA. The role of cytokines in the initiation, expansion, and control of cellular immunity to tuberculosis. Immunol Rev. (2008) 226:191–204. doi: 10.1111/j.1600-065X.2008.00702.x
7. O'Garra A, Redford PS, McNab FW, Bloom CI, Wilkinson RJ, Berry MP. The immune response in tuberculosis. Annu Rev Immunol. (2013) 31:475–527. doi: 10.1146/annurev-immunol-032712-095939
8. Monin L, Khader SA. Chemokines in tuberculosis: the good, the bad and the ugly. Semin Immunol. (2014) 26:552–8. doi: 10.1016/j.smim.2014.09.004
9. Slight SR, Khader SA. Chemokines shape the immune responses to tuberculosis. Cytokine Growth Factor Rev. (2013) 24:105–13. doi: 10.1016/j.cytogfr.2012.10.002
10. Rajamanickam A, Munisankar S, Bhootra Y, Dolla CK, Nutman TB, Babu S. Coexistent helminth infection-mediated modulation of chemokine responses in latent tuberculosis. J Immunol. (2019) 202:1494–500. doi: 10.4049/jimmunol.1801190
11. Salgame P, Yap GS, Gause WC. Effect of helminth-induced immunity on infections with microbial pathogens. Nat Immunol. (2013) 14:1118–26. doi: 10.1038/ni.2736
12. Kathamuthu GR, Munisankar S, Sridhar R, Baskaran D, Babu S. Helminth mediated modulation of the systemic and mycobacterial antigen - stimulated cytokine profiles in extra-pulmonary tuberculosis. PLoS Negl Trop Dis. (2019) 13:e0007265. doi: 10.1371/journal.pntd.0007265
13. Cooper AM, Mayer-Barber KD, Sher A. Role of innate cytokines in mycobacterial infection. Mucosal Immunol. (2011) 4:252–60. doi: 10.1038/mi.2011.13
14. Mayer-Barber KD, Sher A. Cytokine and lipid mediator networks in tuberculosis. Immunol Rev. (2015) 264:264–75. doi: 10.1111/imr.12249
15. Mayer-Barber KD, Andrade BB, Barber DL, Hieny S, Feng CG, Caspar P, et al. Innate and adaptive interferons suppress IL-1alpha and IL-1beta production by distinct pulmonary myeloid subsets during Mycobacterium tuberculosis infection. Immunity. (2011) 35:1023–34. doi: 10.1016/j.immuni.2011.12.002
16. Sugawara I, Yamada H, Kaneko H, Mizuno S, Takeda K, Akira S. Role of interleukin-18 (IL-18) in mycobacterial infection in IL-18-gene-disrupted mice. Infect Immun. (1999) 67:2585–9. doi: 10.1128/IAI.67.5.2585-2589.1999
17. Kathamuthu GR, Moideen K, Bhaskaran D, Sekar G, Sridhar R, Vidyajayanthi B, et al. Reduced systemic and mycobacterial antigen-stimulated concentrations of IL-1beta and IL-18 in tuberculous lymphadenitis. Cytokine. (2017) 90:66–72. doi: 10.1016/j.cyto.2016.10.013
18. Mantovani A, Dinarello CA, Molgora M, Garlanda C. Interleukin-1 and related cytokines in the regulation of inflammation and immunity. Immunity. (2019) 50:778–95. doi: 10.1016/j.immuni.2019.03.012
19. Wood IS, Wang B, Trayhurn P. IL-33, a recently identified interleukin-1 gene family member, is expressed in human adipocytes. Biochem Biophys Res Commun. (2009) 384:105–9. doi: 10.1016/j.bbrc.2009.04.081
20. Pichery M, Mirey E, Mercier P, Lefrancais E, Dujardin A, Ortega N, et al. Endogenous IL-33 is highly expressed in mouse epithelial barrier tissues, lymphoid organs, brain, embryos, and inflamed tissues: in situ analysis using a novel Il-33-LacZ gene trap reporter strain. J Immunol. (2012) 188:3488–95. doi: 10.4049/jimmunol.1101977
21. Moussion C, Ortega N, Girard JP. The IL-1-like cytokine IL-33 is constitutively expressed in the nucleus of endothelial cells and epithelial cells in vivo: a novel 'alarmin'? PLoS ONE. (2008) 3:e3331. doi: 10.1371/journal.pone.0003331
22. Schmitz J, Owyang A, Oldham E, Song Y, Murphy E, McClanahan TK, et al. IL-33, an interleukin-1-like cytokine that signals via the IL-1 receptor-related protein ST2 and induces T helper type 2-associated cytokines. Immunity. (2005) 23:479–90. doi: 10.1016/j.immuni.2005.09.015
23. Liew FY, Pitman NI, McInnes IB. Disease-associated functions of IL-33: the new kid in the IL-1 family. Nat Rev Immunol. (2010) 10:103–10. doi: 10.1038/nri2692
24. Pineros AR, Campos LW, Fonseca DM, Bertolini TB, Gembre AF, Prado RQ, et al. M2 macrophages or IL-33 treatment attenuate ongoing Mycobacterium tuberculosis infection. Sci Rep. (2017) 7:41240. doi: 10.1038/srep41240
25. Khader SA, Bell GK, Pearl JE, Fountain JJ, Rangel-Moreno J, Cilley GE, et al. IL-23 and IL-17 in the establishment of protective pulmonary CD4+ T cell responses after vaccination and during Mycobacterium tuberculosis challenge. Nat Immunol. (2007) 8:369–77. doi: 10.1038/ni1449
26. Cooper AM, Roberts AD, Rhoades ER, Callahan JE, Getzy DM, Orme IM. The role of interleukin-12 in acquired immunity to Mycobacterium tuberculosis infection. Immunology. (1995) 84:423–32.
27. Flynn JL, Goldstein MM, Triebold KJ, Sypek J, Wolf S, Bloom BR. IL-12 increases resistance of BALB/c mice to Mycobacterium tuberculosis infection. J Immunol. (1995) 155:2515–24.
28. Boisson-Dupuis S, Ramirez-Alejo N, Li Z, Patin E, Rao G, Kerner G, et al. Tuberculosis and impaired IL-23-dependent IFN-gamma immunity in humans homozygous for a common TYK2 missense variant. Sci Immunol. (2018) 3:eaau8714. doi: 10.1126/sciimmunol.aau8714
29. Martinez-Barricarte R, Markle JG, Ma CS, Deenick EK, Ramirez-Alejo N, Mele F, et al. Human IFN-gamma immunity to mycobacteria is governed by both IL-12 and IL-23. Sci Immunol. (2018) 3:eaau6759. doi: 10.1126/sciimmunol.aau6759
30. Altare F, Durandy A, Lammas D, Emile JF, Lamhamedi S, Le Deist F, et al. Impairment of mycobacterial immunity in human interleukin-12 receptor deficiency. Science. (1998) 280:1432–5. doi: 10.1126/science.280.5368.1432
31. Robinson CM, Nau GJ. Interleukin-12 and interleukin-27 regulate macrophage control of Mycobacterium tuberculosis. J Infect Dis. (2008) 198:359–66. doi: 10.1086/589774
32. Banchereau J, Pascual V, O'Garra A. From IL-2 to IL-37: the expanding spectrum of anti-inflammatory cytokines. Nat Immunol. (2012) 13:925–31. doi: 10.1038/ni.2406
33. Leveton C, Barnass S, Champion B, Lucas S, De Souza B, Nicol M, et al. T-cell-mediated protection of mice against virulent Mycobacterium tuberculosis. Infect Immun. (1989) 57:390–5. doi: 10.1128/IAI.57.2.390-395.1989
34. Booty MG, Barreira-Silva P, Carpenter SM, Nunes-Alves C, Jacques MK, Stowell BL, et al. IL-21 signaling is essential for optimal host resistance against Mycobacterium tuberculosis infection. Sci Rep. (2016) 6:36720. doi: 10.1038/srep36720
35. Kumar NP, Banurekha VV, Nair D, Babu S. Diminished plasma levels of common gamma-chain cytokines in pulmonary tuberculosis and reversal following treatment. PLoS ONE. (2017) 12:e0176495. doi: 10.1371/journal.pone.0176495
36. Redford PS, Murray PJ, O'Garra A. The role of IL-10 in immune regulation during M. tuberculosis infection. Mucosal Immunol. (2011) 4:261–70. doi: 10.1038/mi.2011.7
37. Ji DX, Yamashiro LH, Chen KJ, Mukaida N, Kramnik I, Darwin KH, et al. Type I interferon-driven susceptibility to Mycobacterium tuberculosis is mediated by IL-1Ra. Nat Microbiol. (2019) 4:2128–35. doi: 10.1038/s41564-019-0578-3
38. Rook GA. Th2 cytokines in susceptibility to tuberculosis. Curr Mol Med. (2007) 7:327–37. doi: 10.2174/156652407780598557
39. Kumar NP, Moideen K, Nancy A, Viswanathan V, Shruthi BS, Sivakumar S, et al. Plasma chemokines are biomarkers of disease severity, higher bacterial burden and delayed sputum culture conversion in pulmonary tuberculosis. Sci Rep. (2019) 9:18217. doi: 10.1038/s41598-019-54803-w
40. Cyster JG. Chemokines and cell migration in secondary lymphoid organs. Science. (1999) 286:2098–102. doi: 10.1126/science.286.5447.2098
Keywords: tuberculosis, lymphadenitis, filariasis, cytokines, chemokines
Citation: Kathamuthu GR, Munisankar S, Banurekha VV, Nair D, Sridhar R and Babu S (2020) Filarial Coinfection Is Associated With Higher Bacterial Burdens and Altered Plasma Cytokine and Chemokine Responses in Tuberculous Lymphadenitis. Front. Immunol. 11:706. doi: 10.3389/fimmu.2020.00706
Received: 25 February 2020; Accepted: 30 March 2020;
Published: 21 April 2020.
Edited by:
Christoph Hölscher, Research Center Borstel (LG), GermanyReviewed by:
William Harnett, University of Strathclyde, United KingdomSuraj Prakash Parihar, University of Cape Town, South Africa
Copyright © 2020 Kathamuthu, Munisankar, Banurekha, Nair, Sridhar and Babu. This is an open-access article distributed under the terms of the Creative Commons Attribution License (CC BY). The use, distribution or reproduction in other forums is permitted, provided the original author(s) and the copyright owner(s) are credited and that the original publication in this journal is cited, in accordance with accepted academic practice. No use, distribution or reproduction is permitted which does not comply with these terms.
*Correspondence: Gokul Raj Kathamuthu, Z29rdWwuckBuaXJ0LnJlcy5pbg==